- 1Texas A&M AgriLife Research and Extension Center, Weslaco, TX, United States
- 2Department of Agriculture, Agribusiness, and Environmental Sciences, Texas A&M University-Kingsville, Weslaco, TX, United States
- 3Department of Horticultural Sciences, Texas A&M University, College Station, TX, United States
- 4Department of Entomology, Texas A&M University, College Station, TX, United States
- 5Department of Plant Pathology & Microbiology, Texas A&M University, College Station, TX, United States
- 6Institute for Advancing Health Through Agriculture, Texas A&M AgriLife, College Station, TX, United States
Potato zebra chip (ZC) disease, associated with the uncultured phloem-limited bacterium, Candidatus Liberibacter solanacearum (CLso), is transmitted by the potato psyllid Bactericera cockerelli. Potato ZC disease poses a significant threat to potato production worldwide. Current management practices mainly rely on the control of the psyllid to limit the spread of CLso. The present study investigated new sources of ZC resistance among wild Solanum species. A taxonomically diverse collection of tuber-bearing Solanum species was screened; one ZC-resistant accession and three ZC-tolerant accessions were identified among the 52 screened accessions. Further characterization of the resistant accession showed that the resistance was primarily associated with antibiosis effects due to differences in leaf trichome density and morphology of the wild accession, which could limit the psyllid feeding and oviposition. This germplasm offers a good resource for further understanding ZC and psyllid resistance mechanisms, contributing to potato breeding efforts to develop ZC resistance cultivars. Alternatively, it could be used as a potential trap crop to manage psyllid and control ZC disease.
Introduction
Potato (Solanum tuberosum L.) is cultivated in over 160 countries and is rated as the fourth most important staple food crop after wheat, corn, and rice. It is a rich source of carbohydrates and provides other essential nutrients, such as dietary fiber, vitamins, minerals, protein, and antioxidants (Dahal et al., 2019). Based on its global consumption, nutritional benefits, tonnage production, and cash value, the global production was estimated at ~395 metric tons in 2019. Its importance in the United States was at 3.94 billion (Faostat, 2020; USDA, 2020). However, increasing the incidence of biotic (diseases and pests) as well as abiotic (drought, heat, and salinity) stresses limit potato production (Savary et al., 2019).
Since its identification in 1994 in Mexico and in 2000 in the United States, the zebra chip (ZC) disease of potato has spread into several commercial potato-growing regions of Central America, Australia, New Zealand, Northern Africa, and the Middle East (Munyaneza et al., 2007, 2009a, 2010; Tahzima et al., 2014; Mawassi et al., 2018; Mora et al., 2021) and recently in Spain (Portal, 2017). ZC disease could result in potato yield losses of up to 94% (Greenway, 2014). The disease is associated with the uncultured phloem-limited bacterium Candidatus Liberibacter solanacearum (CLso) and vectored by the potato psyllid Bactericera cockerelli Šulc (Hemiptera: Triozidae) (Munyaneza, 2012; Nwugo et al., 2017; Mora et al., 2021). Typical foliar symptoms of ZC-affected plants include purplish discoloration/chlorosis of young leaves, upward rolling of top leaves, presence of axillary buds, presence of aerial tubers, wilting, stunted growth, and ultimately plant death. ZC symptoms in tubers are associated with the synthesis and accumulation of phenolic compounds, reducing sugars and defense enzymes (Navarre et al., 2009; Wallis et al., 2012), giving chips a bitter taste and a dark brown striped, zebra-like appearance when fried (Munyaneza, 2012), ultimately causing the entire tubers to become unmarketable (Mora et al., 2021). If left uncontrolled, ZC disease can become one of the potato's economically significant diseases.
Current ZC management strategies rely on controlling the psyllid vector, involving insecticides (Guenthner et al., 2012; Greenway, 2014; Greenway and Rondon, 2018) and the possibility of increased insecticide resistance. Recently, resistance to neonicotinoid-based insecticides has been reported in Texas potato psyllids, which threatens future control of this insect (Prager et al., 2013; Szczepaniec et al., 2019). The primary mode of acquisition and spreading of CLso is by psyllids feeding on CLso-infected plants and then on healthy plants transferring CLso (Munyaneza et al., 2009b; Buchman et al., 2011). Hence, identifying novel genetic resistance and tolerance to CLso or the psyllid can be valuable components of ZC's integrated pest/diseases management. Previous studies have reported variations in the psyllid preference for the wild potato species, namely, Solanum bulbocastanum, Solanum habrochaites, and Solanum Verrucosum, and the breeding clones, namely, Solanum berthaultii and Solanum tuberosum (Butler et al., 2011; Cooper and Bamberg, 2014, 2016; Diaz-Montano et al., 2014; Levy and Tamborindeguy, 2014). Variations in the tolerance response to CLso have also been noted among various Solanum breeding clones (Prager et al., 2013; Rashidi et al., 2017; Vigue, 2018; Fife et al., 2020; Vigue et al., 2020).
A 2015 taxonomic and genetic study established a Solanum section Petota panel of tuber-bearing relatives of cultivated potatoes, representing a genetic resource of broadly diverse germplasm of highly regarded agronomic traits and tuber size (Hawkes, 1990; Spooner and Castillo, 1997; Hardigan et al., 2015). In this study, screening and identification of new ZC-resistant and -tolerant germplasm in the Solanum sect. Petota panels were performed. The ZC resistance of one accession (S. berthaultii) could be attributed to modifications in leaf trichome shape and density, affecting potato psyllid fecundity and survival.
Materials and Methods
Plant Materials, Propagation, and Maintenance
Plant material consisted of 52 wild potato accessions grown from true potato seeds obtained from the U.S. National Plant Germplasm System (NPGS) in Wisconsin, USA. The introductions belong to the Solanum sect. Petota diversity panel (Supplementary Table 1). This panel represents the germplasm of tuber-bearing Solanum species exhibiting morphological variations (Supplementary Figure 1) (Hardigan et al., 2015). Solanum tuberosum L. var. Atlantic (chip processing market class) was used as a susceptible control in all experiments and was initially propagated from certified disease-free seed tubers. For in vitro germination and propagation, the Solanum botanical seeds were pretreated with 10% (w/v) gibberellic acid (GA3) overnight in a 1.5 ml microcentrifuge tube to break dormancy and enhance seed germination. Furthermore, seeds were surface sterilized in 70% (v/v) ethanol for 3 min and then with 10% (v/v) bleach and 2% (v/v) Tween® 20 (Sigma-Aldrich, St. Louis, MO, USA) for 10 min, followed by rinsing four times with sterile water. Sterilized seeds were placed on a sterile wetted Whatman™ paper inside a sterile 100 × 25 mm Petri dish and kept at 22°C in the dark for 1 week. Germinated seeds were grown on Murashige and Skoog (Murashige and Skoog, 1962) solid media supplemented with pre-made MS vitamins (Caisson Labs, North Logan, Utah, USA), 2% sucrose (w/v), and 2 g/L of Gelrite™ (Research Products International, Mt. Prospect, IL, USA), pH 5.8. When plants were 4 weeks old (about 8 cm tall), micropropagation was done using internode cutting as explants; sub-culturing every 4 weeks was necessary to increase plant material for each wild potato accession for screening against ZC. All explants were maintained in a temperature-controlled growth chamber at 22°C under 14-h light/10-h dark photoperiod.
Psyllid Maintenance
Potato psyllid (Bactericera cockerelli Šulc.) colonies consisted of CLso-free (CLso−) and CLso-positive (CLso+) haplotype B, which were obtained and reared for several generations at Texas A&M AgriLife Research and Extension Center in Weslaco in 60 × 60 × 60 cm nylon mesh cages (Bugdorm, BioQuip Products Rancho Dominguez, CA, USA). Psyllid colonies were reared and maintained on potato plants (Atlantic) and periodically diagnosed for the presence of CLso (>90% CLso+) and the absence of CLso (CLso−) by polymerase chain reaction (PCR) using genomic DNA isolated from adult psyllids (Sengoda et al., 2014) and primers specific to the 16s rDNA of CLso (OA2-F and OI2c-R) (Supplementary Table 2) (French-Monar et al., 2010). PCR conditions were given as follows: one denaturing cycle at 95°C for 30 s; 35 cycles each at 95°C for 30 s, 68°C for 30 s, and 68°C for 2 min; and a final extension cycle at 68°C for 5 min. PCR amplicons were separated by electrophoresis on a 1.0% (w/v) agarose gel stained with ethidium bromide (0.5 μg/ml).
Screening of Wild Solanum Sect. Petota Accessions for ZC Resistance
For primary screening, 52 wild potato accessions were grown in a professional growth mix (Berger BM6 All-Purpose mix, pH 5.4–6.2) in 2.84-l pots at 22°C under 14-h light/10-h dark photoperiod and 50% relative humidity in controlled growth chambers. No choice feeding was performed on 4-week-old plants by inoculating each plant with 20 CLso+ psyllids enclosed into two separate organza drawstring bags (10 psyllids/bag), which were tied to the second and third fully expanded leaves (Supplementary Figure 2B), with four replicates of inoculated plants per accession. The bags and psyllids were removed from plants 1 week after placement. Phenotypic evaluations of foliar ZC disease symptoms were done at 14, 21, and 28 days post-inoculation (dpis) in an environment-controlled growth chamber. Foliar symptoms were evaluated and rated based on a scale of 1–3, where 1 = being resistant (no symptoms), 2 = moderately susceptible (some symptoms), and 3 = highly susceptible (severe symptoms and morbidity) (Supplementary Figures 2C, 3). Chip frying and ZC scoring were performed as described in other studies (Henne et al., 2010; Harrison et al., 2019).
A second screening of the ZC resistant and moderately susceptible accessions chosen from the primary screening and a screening of the single seed lines from true potato seeds were done using 1-month-old plants. To increase quantity, all plants were vegetatively propagated by tissue culture and were planted in modified enclosed transparent 32 oz. plastic cups. No choice feeding was performed by releasing five CLso+ psyllids (presence of CLso was confirmed by PCR diagnostics) into each enclosed cup containing two plants in a professional growth mix Berger BM6 All-Purpose mix, pH 5.4–6.2, with two cups per accession. CLso− psyllid (absence of CLso was confirmed by PCR diagnostics) challenged and non-challenged plants were used as negative controls (Supplementary Figure 2A). Phenotypic evaluations of inoculated plants consisted of assessing foliar symptoms of ZC at 14, 21, and 28 dpi. At 7 dpi, the soil was drenched with Admire Pro (Bayer Crop Science, Germany) to kill all psyllids in enclosed cups and prevent further plant damage. Leaf tissue was collected at 21 and 28 dpi, with 3–4 leaves from the top, middle, and bottom of each plant, cut into pieces with a single edge blade, and pooled to produce four replicates before freezing in liquid nitrogen (VWR Reinforced 2 ml Bead Mill Tubes, Radnor, PA, USA). Tissues were lyophilized before genomic DNA extraction for PCR and quantitative PCR (qPCR) analyses.
Molecular Diagnostics for CLso Detection and Quantification
Genomic DNA was extracted from leaf tissue using a modified protocol as previously published (Edwards et al., 1991). For the detection of CLso in tissues collected from wild potato accessions and Atlantic control, conventional PCR was performed on a ProFlex™ PCR System (Applied Biosystems, Life Technologies, Carlsbad, CA) in a total reaction volume of 20 μl, using 150 ng of DNA, 0.5 μM of each target-specific primer, 10 μl of AccuStart Tough Mix (Quantabio, Beverly, MA, USA), 0.5 μl of 50 × loading dye, and 6.6 μl of nuclease-free water (Ambion, Life Technologies, Austin, TX, USA). For initial conventional (gel) PCR analysis, a primer pair, OI2C-F and OA2-R, was used to specifically amplify CLso 16S rDNA (Levy et al., 2011). The potato Ribosomal Protein L2(RPL2)-specific primers RPL2-F and RPL2-R were used to amplify an endogenous reference gene (Supplementary Table 2) (Avila et al., 2012). PCR conditions were given as follows: one denaturing cycle at 95°C for 30 s; 28 cycles each at 95°C for 30 s, 50°C for 30 s, and 68°C for 2 min; and a final extension cycle at 68°C for 5 min. PCR amplicons were separated by electrophoresis on a 1.0% (w/v) agarose gel stained with ethidium bromide (0.5 μg/ml).
The CLso relative titer was quantified in tissues collected from wild potato accessions and Atlantic control using quantitative PCR (qPCR) in a CFX384™ Real-Time System (Bio-Rad Laboratories, Inc., Hercules, CA, USA) in a total reaction of 10 μl, using 50 ng of DNA, 0.4 μM of each target-specific primer, and 5 μl of iTaq™ Universal SYBR Green Supermix (Bio-Rad Laboratories, Inc., Hercules, CA, USA). Three biological replicates were used with two technical replicates. Lso-F and HLB-R primers (Supplementary Table 2) were used to specifically amplify CLso 16S rDNA, while Sotu-RPL2-F and Sotu-RPL2-R primers (Supplementary Table 2) were used for the amplification of an endogenous reference gene, RPL2 (Levy et al., 2011; Irigoyen et al., 2020). PCR conditions were given as follows: one denaturing cycle at 95°C for 3 min; 40 two-step cycles each at 95°C for 15 s and at 55°C for 30 s; and a final melting curve of 65–95°C for 55 s. The results were analyzed and recorded as CT (threshold cycle) values, which were normalized against the CT values of the potato RPL2 (Supplementary Table 2) for quantification using the comparative CT method () (Zuñiga et al., 2020). The Student's t-test was used to determine statistically significant (p ≤ 0.05 or 0.01) differences between the controls and treatments.
Host Selection and Olfactometer Assays
The olfactory host-preference assays of B. cockerelli adults (CLso−), reared and maintained on Atlantic potato plants, were conducted using 2-month-old Sb-PI310927 and Atlantic plants and a Y-tube olfactometer (35 cm long × 2.5 cm diameter). Twenty B. cockerelli adults per set of plants (n = 10 sets of plants, Sb-PI310927 and Atlantic plants) were collected into plastic vials for 16 h before initiating the behavioral assays to promote insect host choice. The Y-tube was connected to a 2-port Humidified Air Delivery system (ARS, Gainesville, FL, USA). The air was filtered through an activated carbon filter (16 cm, ARS). Airflow of each arm (bifurcated at a 45 angle) was set up at a constant rate of 10 L/min, leading into two separated sealed cylindrical glass chambers (15 cm diameter, 35 cm high) that contained individual plants, Sb-PI310927 or Atlantic, as odor sources. The Y-tube was placed at a 30° angle from the surface and adult psyllids were released at the end of the tube. To examine the insect olfactory behavior, observations were recorded by counting the number of psyllids at each arm's terminal end (set threshold = 4 cm) every 15 min for a 60-min period. All others were considered as a non-responding group. All measurements were conducted between 2 and 4 p.m. Central Standard Time (CST) at ambient temperature (21°C) and under constant light (~250 μmol/m2 s).
Survival and Oviposition of B. cockerelli on Sb-PI310927 and Atlantic Plants
The survival of B. cockerelli adults (CLso−) on 2-month-old Sb-PI310927 and Atlantic plants (n = 10 plants; 10 psyllids/plant) was evaluated to record the surviving insects every day for 7 days. To examine the oviposition of B. cockerelli on Sb-PI310927 and Atlantic plants, a non-choice assay was performed on insect-proof mesh cages (30 × 30 × 30 cm). Before the assay was initiated, couples (female and male psyllids) were allowed to mate for 4 h in a 1.7-ml tube and subsequently transferred onto plants (n = 10 plants; 10 psyllids/plant). After 7 days, adult psyllids were removed, and the total number of eggs was recorded.
Trichome Evaluation of Sb-PI310927
One-month-old in vitro culture plants of ZC-resistant single seed line 10 of Sb-PI310927 accession and Atlantic control were hardened for 1 month in the growth mix. Three 2-mm diameter disks were excised from one leaflet of the second and third fully expanded leaves from two plants of each of Sb-PI310927-resistant line 10 and Atlantic control, using a 2-mm sampling tool (Electron Microscopy Sciences, Hatfield, PA, USA), and observed at 60 × on an Olympus BX51 stereomicroscope (Olympus Corporation, Tokyo, Japan). Leaflet disks were evaluated for leaf trichome shape and density based on two biological samplings and 12 technical replications. Trichome density was calculated as the number of trichomes per mm2 leaf area on both the adaxial and abaxial leaflet surfaces.
Data Analysis and Statistics
The data analysis, statistics, and graphs were displayed as described in the figure legends using the Microsoft Excel software (version 2009). The Student's t-test was used to determine statistically significant (p ≤ 0.05, 0.01, or 0.001) differences in CLso titer and oviposition between control and treatment. The Chi-square (χ2) test was used to compare the number of potato psyllids responding to plant odor sources using a Y-tube olfactometer. The survival of potato psyllids was estimated using the Kaplan–Meier survival analysis using the RStudio environment (Allaire, 2011).
Results
ZC Resistance Exists Among the Wild Solanum Sect. Petota Collection
Primary screening of 52 wild potato accessions was performed from the Solanum sect. Petota collection (Supplementary Table 1) to identify potential lines with resistance and tolerance to CLso or the psyllid. Multiple in vitro propagated plants of each accession were hardened in pots for 2 months, followed by exposure to CLso+ psyllids. Foliar symptoms of ZC were then observed every 7 days for up to 4 weeks (Supplemental Figure 2A, B) and rated based on a scale of 1–3, where 1 = being resistant (no symptoms), 2 = moderately susceptible (some symptoms), and 3 = highly susceptible (severe symptoms and morbidity) (Supplementary Figure 2C). Several of the 52 accessions were susceptible and moderately susceptible, showing some upward leaf rolling, chlorosis, and plant stunting, but they grew new leaves at 21–28 dpi. Interestingly, one diploid accession Solanum berthaultii Hawkes (Sb-PI310927), sourced initially from Bolivia, seemed resistant with no visible ZC symptoms. The nine accessions (one resistant and eight moderately susceptible) were selected, and the challenges with CLso+ psyllids were repeated using 1-month-old plants grown in terrariums (enclosed cups). In addition to phenotypic observation for ZC symptoms, the relative CLso titers were estimated at 28 dpi when CLso could be sufficiently detected. The single resistant accession, Sb-PI310927, reproducibly showed no visible symptoms and continued to grow after 28 dpi with a severity index of 1, compared with the susceptible Atlantic control that displayed severe symptoms and died at 28 dpi (severity index 3). Three accessions, namely, Sk-PI498359, So-PI498130, and Sr-PI310953, again showed reproducible ZC symptoms with a severity index scale of 2 (Figure 1A). The remaining five accessions displayed variable symptoms and died at 28 dpi, with a severity index of 3. In this experiment, both conventional and quantitative PCR confirmed the presence or absence of CLso (Figures 1B,C). The resistant accession Sb-PI310927 and the three moderately susceptible accessions (i.e., Sk-PI498359, So-PI498130, and Sr-PI310953) displayed CLso titers of 6.05% and 52.44–119.36%, respectively, relative to that of the Atlantic control (set to 100%) (Figure 1C). Further comparative studies were carried on with the resistant (Sb-PI310927) and highly susceptible control (Atlantic) plants.
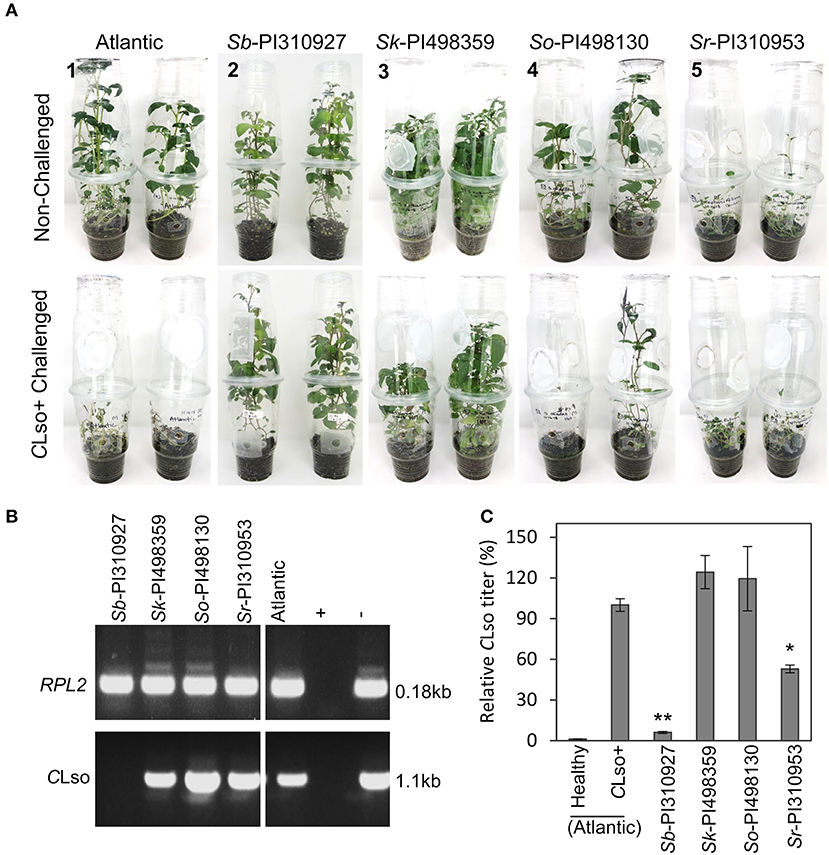
Figure 1. Phenotypic evaluation and CLso quantification of ZC resistant and tolerant wild Solanum accessions. A no-choice psyllid feeding assay was performed by releasing five CLso+ psyllids on two plants (1-month-old) in a professional growth mix per enclosed cup in an environment-controlled growth chamber. (A) The top panel shows representative photos of non-challenged accessions, and the bottom panel representative photos of CLso+ challenged accessions at 28 dpi [1, Atlantic control, severity index (SI) = 3. 2; S. berthaultii PI310927, SI = 1; 3, S. kurtzianum PI498359, SI = 2; 4, S. okadae PI498130, SI = 2. 5; and S. raphanifolium PI310953, SI = 2). (B) Detection of CLso by PCR amplification of CLso 16s rDNA in leaf tissues of the resistant accession (Sb-PI310927) and the three tolerant accessions (Sk-PI498359, So-PI498130, and Sr-PI310953) at 28 days post-inoculation (dpi). The potato RPL2 endogenous gene was used as the PCR control. (C) Relative quantification of CLso titers of the accessions at 28 dpi by qPCR. Relative CLso titers were calculated from three biological replicates; error bars represent the mean CLso titer ± standard error of the mean. The p-values were calculated by Student's t-test relative to the infected control. *, **P ≤ 0.05 and 0.01, respectively.
Sb-PI310927 Is ZC Resistant
The ZC resistance trait of Sb-PI310927 was confirmed by repeating the ZC challenges, using 2-month-old plants under greenhouse conditions, and evaluating the ZC tuber symptoms. Typically, CLso-psyllid feeding for the brief period of the challenge (~7 days) causes the inoculated lower leaves to wilt, while the newly emerging (upper) non-inoculated leaves remain asymptomatic. In contrast, CLso+ psyllids induce symptoms associated with ZC on new upper non-inoculated leaves ranging from leaf wilting, upward curling, and chlorosis/necrosis. They can also lead to severe stunting of the plants (Supplementary Figure 4). Sb-PI310927 plants consistently showed no visual ZC foliar symptoms with a severity index of 1 when challenged with CLso+ or CLso− psyllids. However, the susceptible Atlantic variety displayed typical ZC foliar symptoms with a severity index of 3 when infected with CLso+ psyllids at 28 dpi (Figure 2B). Freshly cut tubers and fried chips of CLso-infected Sb-PI310927 accession showed no brown discoloration, whereas Atlantic displayed characteristic ZC tuber symptoms (Figure 2C). Furthermore, molecular diagnostic analyses by qPCR and conventional PCR indicated that Sb-PI310927 had a very low relative CLso titer of 4.87% compared with Atlantic plants (CLso titer set to 100%) (Figure 2D,E).

Figure 2. ZC resistance trait evaluation of the Solanum berthaultii accession PI310927. (A) Two-month-old Sb-PI310927 accession and Atlantic control plants in pots were randomized and challenged with CLso+ and CLso− psyllids inside cages under greenhouse conditions. (B, C) The above-ground phenotype of 4-week-old Sb-PI310927 accession and Atlantic plants and their corresponding tuber and fried chip phenotypes. (D) Detection of CLso by PCR amplification of CLso 16s rDNA in leaf tissues of Sb-PI310927 accession and Atlantic at 28 dpi. The potato RPL2 endogenous gene was used as the PCR control (NC, negative control; PC, positive control). (E) Relative quantification of CLso titer of Sb-PI310927 accession and Atlantic control plants at 28 dpi by qPCR. Relative CLso titers were calculated from three biological replicates; error bars represent ± standard error of the mean. The p-values were calculated by Student's t-test relative to the infected control. **P ≤ 0.01.
Segregation of ZC Resistance Among True Seed Progeny of Sb-PI310927
All screening was conducted using in vitro micro-propagated plants from a true seed sourced from the USDA-ARS germplasm bank. As with most wild germplasm collections, Sb-PI310927 true seeds could be heterozygous and pooled from multiple plants during germplasm maintenance, thus having the potential for segregating alleles (Bamberg and Del Rio, 2020). To determine if there would be any segregation of the ZC-resistant trait of Sb-PI310927 among true seed progeny and to recover a stable resistant line for further studies, 10 true botanical seeds of Sb-PI310927 were germinated and multiplied individually by micropropagation. The ZC resistance trait of the 10 single seed lines was then evaluated following no-choice challenges with CLso+, CLso−, or no psyllids under growth chamber conditions (Figure 3A). At 21 dpi, the susceptible Atlantic control showed characteristic ZC symptoms with high titers of CLso transmission, whereas almost all Sb-PI310927 lines did not show any ZC symptoms. However, conventional PCR revealed that only ~50% of Sb-PI310927 single seed lines were negative for CLso (Figure 3B). Further confirmation by quantitative PCR showed that six Sb-PI310927 single seed lines (#3, 4, 5, 7, 8, and 10) were CLso− or had undetectable levels of CLso. Three lines (#2, 6, and 9) showed low to moderate levels of CLso compared with Atlantic control (CLso titer set to 100%). We also noted some segregation in plant height among the 10 lines, which can be observed even among unchallenged (healthy) plants. Together, these results suggest some degree of segregating alleles among the true seed pools of these wild accessions possible for multiple traits (Bamberg and Del Rio, 2020).
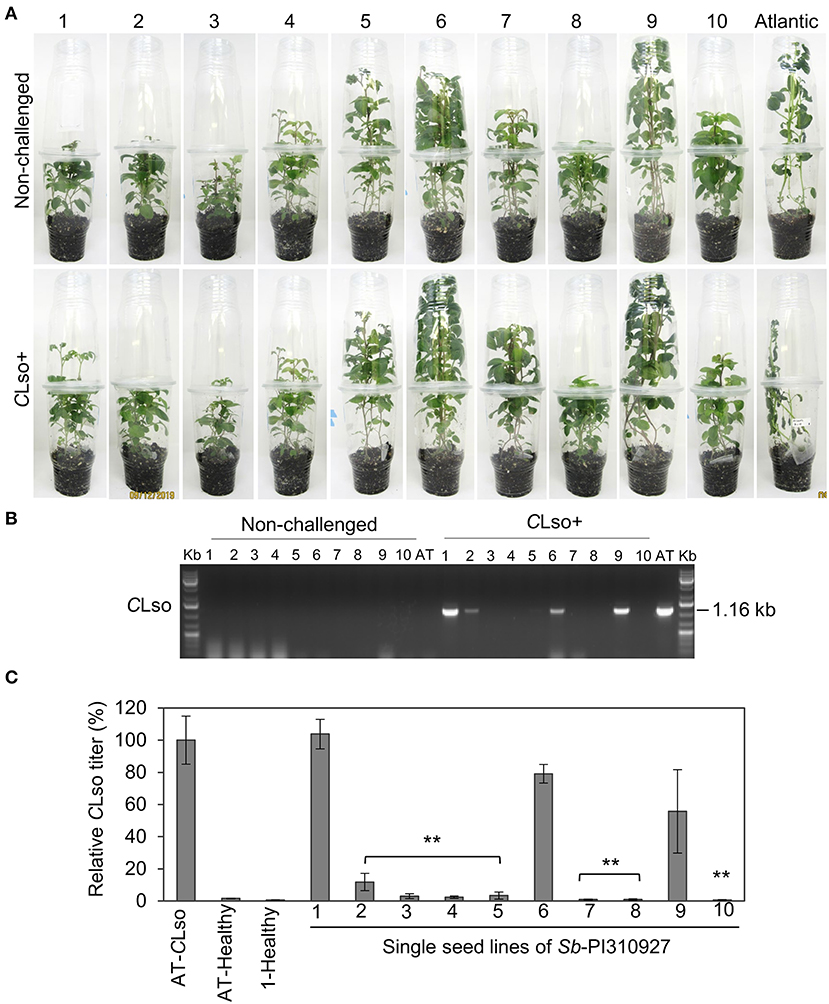
Figure 3. ZC resistance trait evaluation of single seed lines of Solanum berthaultii accession PI310927. (A) Photos of representative lines 1–10 of Sb-PI310927 and Atlantic controls following challenge with CLso+ psyllids at 28 dpi. (B) Detection of CLso by PCR amplification of CLso 16s rDNA in leaf tissues of 10 single seed lines of Sb-PI310927 accession and Atlantic (AT) control plants challenged with CLso+ psyllids or no psyllids at 28 dpi. Controls included a positive control, CLso-infected Atlantic, a negative control, healthy Atlantic (H), and water (W). (C) Relative quantification of CLso titers of the single seed lines and Atlantic (AT) at 28 dpi by qPCR. Relative CLso titers were calculated from three biological replicates; error bars represent ± standard error of the mean. The p-values were calculated by Student's t-test relative to the infected control. **P ≤ 0.01.
Host Preference, Fecundity, and Survival of the Psyllids on Sb-PI310927
The following two possible mechanisms explain the ZC resistance trait of Sb-PI310927: (i) resistance to the psyllid either by antixenosis or antibiosis effects and (ii) immune gene-mediated resistance to the bacteria or the insect. Although not mutually exclusive, we first explored if there were antixenosis or antibiosis effects. For this, we evaluated whether there was a host preference between Sb-PI310927 line#10 and Atlantic plants equidistantly placed in an olfactometer-based choice assay (n = 10 plants; 20 psyllids/plant). Of the total psyllids analyzed in the experiment (20 psyllids × 10 plants = 200 psyllids), the majority (76%) of them responded within 60 min after the assay was initiated, i.e., reached a 4-cm set threshold distance in the arms toward the plant odor sources (Figure 4A). However, no significant differences in host-selection were found between Sb-PI310927 and Atlantic odor sources at the sampling points tested (Figure 4B; 15 min: χ2 = 0.053, df = 1, P = 0.82; 30 min: χ2 = 0.644, df = 1, P = 0.42; 45 min: χ2 = 0.65, df = 1, P = 0.42; and 60 min: χ2 = 0.118, df = 1, P = 0.73).
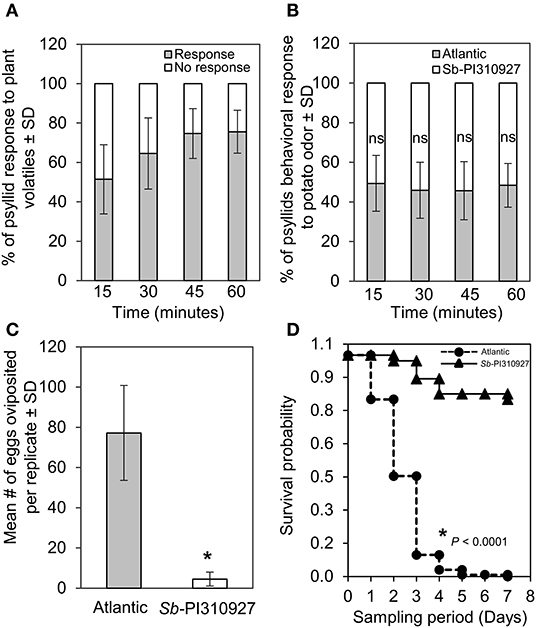
Figure 4. Olfactometer, oviposition, and survival evaluations of Bactericera cockerelli adults on Solanum berthaultii PI310927. (A) Olfactometer (Y-tube) behavioral response of potato psyllid adults to plant volatiles under stable conditions observed every 15 min for a maximum of 60 min. Bar graphs represent the overall mean percentages of adults choosing either odor source ± standard deviation (n = 10). (B) Potato psyllid's behavioral response to Sb-PI310927 and susceptible Atlantic (control). Bar graphs represent the mean percentages of adults ± standard deviation (n = 10). (C) Female psyllids oviposition at day 7 in no-choice assays using whole plants. Bar graphs represent the mean number of oviposited eggs per replicate ± standard deviation (n = 10); the p-value was calculated by Student's t-test relative to the Atlantic control, *P ≤ 0.0001. (D) Survival analysis of potato psyllid adults (n = 10) for 7 days showed significant psyllids mortality after exposure to Sb-PI310927 when compared with Atlantic plants. The p-value was calculated by the Kaplan–Meier analysis, P < 0.0001. SD, standard deviation; ns, no significance.
As there were no differences in host preference, we next evaluated if there were any adverse effects on oviposition, hatching, and survival of psyllids using no-choice assays with 2-month-old Sb-PI310927 line#10 and Atlantic plants (n = 10 plants; 10 psyllids/plant). The results showed a significant reduction (F = 113.68, df = 1, P < 0.001) in the number of oviposited eggs after 7 days on Sb-PI310927 when compared with Atlantic plants (Figure 4C). The few oviposited eggs on Sb-PI310927 were also defective in hatching. Furthermore, survival analysis showed that adult psyllids on Sb-PI310927 had a significantly (Kaplan Meier analysis, P < 0.0001) lowered probability of survival beyond day 3 when compared with Atlantic plants (Figure 4D).
Leaf Trichome Density and Morphological Differences of Sb-PI310927
Lastly, in our experiments, we observed that the leaves of S. berthaultii were “sticky,” and the movement of psyllids appeared to be impaired (Figure 5C). Hence, under a microscope, we examined the adaxial and abaxial surfaces of 1-month-old Sb-PI310927 line #10. Sb-PI310927 leaves had a more significant number of trichomes, and they appear longer than those of Atlantic leaves (Figures 5A,B). The number of trichomes was significantly (P < 0.01) higher in Sb-PI310927 than in Atlantic leaves on both the adaxial and abaxial surfaces (Figures 5A,B). It is possible that the observed reduced fecundity and survival of psyllids effects on Sb-PI310927 could be associated with the increased trichome density and the presence of glandular trichomes (Figures 5C,D).
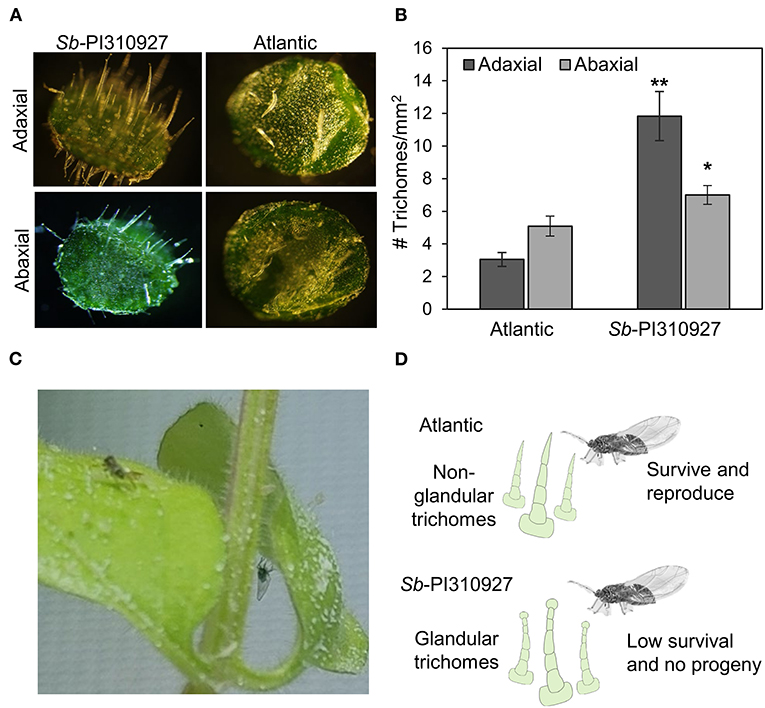
Figure 5. Evaluation of leaf trichomes on Solanum berthaultii PI310927 and Atlantic. (A) Microscopic evaluation of shape and density of trichomes on the abaxial and adaxial surfaces of leaves of Sb-PI310927 line 10 and Atlantic controls. (B) Estimation of trichome density on the adaxial and abaxial leaf surfaces of Sb-PI310927 and Atlantic controls. Errors bars represent the mean number of trichomes per mm2 ± standard error of the mean (n = 12). The p-values were calculated by Student's t-test relative to the Atlantic control. * P ≤ 0.01; ** P ≤ 0.001. (C) Dead psyllids on abaxial and adaxial leaf surfaces of Sb-PI310927 plants using non-choice assays. (D) Proposed mechanism of negative effects of Sb-PI310927 on potato psyllid.
Discussion
Zebra chip, a devastating potato disease worldwide, is complex due to its association with the phloem-limited and uncultured CLso and the potato psyllid vector. Using chemical measures has helped control the psyllid population, but it is associated with high costs and positive selection for insecticide resistance (Szczepaniec et al., 2019). In the present study, new sources of ZC resistance were identified among a wild collection of tuber-bearing Solanum spp. (Solanum sect. Petota) (Hardigan et al., 2015). This panel is a taxonomically well-characterized and diverse collection to mine for valuable traits (Hardigan et al., 2015).
Following the screening, phenotypic evaluations, and quantitation of CLso titers of 52 accessions infected with CLso carrying psyllids, we identified one ZC-resistant accession, S. berthaultii PI310927, and three ZC moderately tolerant accessions, namely, Solanum kurtzianum PI498359, Solanum okadae PI498130, and Solanum raphanifolium PI310953. Phenotypically, although the three ZC-tolerant wild Solanum accessions (S. kurtzianum PI498359, S. okadae PI498130, and S. raphanifolium PI310953) showed some ZC foliar symptoms at 28 dpi, they were able to tolerate and survive CLso. In contrast, the susceptible Atlantic control plants showed severe symptoms, wilting, and eventual death. There are some commonalities among the three tolerant and one resistant accessions. They were all sourced from countries that are relatively closer to the South American continent (Hardigan et al., 2015). The four species are diploids and belong to the same species, clade 4, as described by Spooner and Castillo (1997). Several of these accessions were also previously reported to be tolerant to other potato pests, including green peach aphid, potato aphid, Colorado potato beetle, potato flea beetle, and potato leafhopper (Flanders et al., 1992).
The S. berthaultii PI310927 is a highly promising candidate among the ZC-resistant lines. Characterization of resistance among the S. berthaultii PI310927 true seeds showed a low-to-moderate level of transmission of CLso among true seed progeny, indicating the presence of heterozygosity or allelic variation among the true seed progeny, which is expected from wild germplasm where traits and alleles are not fixed. Potato breeding efforts with hybrids that incorporated S. berthaultii as one of the parents reported variable levels of tolerance to CLso, likely caused due to the heterogeneity of the progeny (Butler et al., 2011; Prager et al., 2013; Rashidi et al., 2017). Our results suggest that natural allelic variations present among the true seed progenies of wild germplasm can impact trait phenotypes and underscore the need to evaluate multiple seed progeny independently propagated.
Importantly, we were able to recover several true seed lines of S. berthaultii PI310927 (#3, 4, 5,7, 8, and 10) that showed no ZC symptoms and detectable CLso, when compared with the Atlantic control (100%) (Figures 3B,C). Further characterization of the resistant single seed line #10 showed no differences in psyllid preference to odor sources of Sb-PI310927 and Atlantic plants (Figures 4A,B). However, we found greater leaf trichome density that may cause the negative impacts observed on psyllid fecundity and survival (Figures 4C,D, 5A–D). Previous studies on other wild potato relatives and hybrids suggested similar effects of the glandular trichomes against several potato pests (Tingey and Gibson, 1978; Tingey and Laubengayer, 1981; Yencho and Tingey, 1994; Malakar and Tingey, 2000; Butler et al., 2011; Diaz-Montano et al., 2014; Rubio-Covarrubias et al., 2017). Additionally, specific secondary metabolites produced by glandular trichomes were reported to influence host selection (Prager et al., 2018). Further investigation into potential chemical signatures or metabolites synthesized by the S. berthaultii PI310927 glandular trichomes may bring new insights into the mechanisms of the observed ZC and psyllid resistance.
Conclusion
In this study, screening 52 tuber-bearing wild Solanum species resulted in identifying a ZC resistant and several tolerant accessions. The resistant S. berthaultii accession has dense glandular leaf trichomes. This foliar structural modification could be responsible for much of the observed ZC resistance. We suggest the S. berthaultii as a promising source for ZC/psyllid resistance that can be further studied to understand insect resistance mechanisms and incorporated into the potato production system as a trap crop for psyllid or breeding new potato cultivars.
Data Availability Statement
The original contributions presented in the study are included in the article/Supplementary Material, further inquiries can be directed to the corresponding author/s.
Author Contributions
KM conceived the project and supervised the study. KM, FI, CA, VA, and MV designed the experiments and interpreted the results. VM, MR, MD, and SI conducted the experiments, analyzed the data, and prepared the manuscript. All authors contributed to the editing and review of the manuscript.
Funding
This study was supported in part by funds from Texas A&M AgriLife Research Insect-Vectored Disease Seed Grant (124185-96210) and the Texas A&M AgriLife Institute for Advancing Health Through Agriculture, USDA-NIFA (HATCH 1023984) to KM and USDA-NIFA (2019-03814) to MIV. We thank Jesse Schartner and John Bamberg (U.S. Potato Genebank, NRSP-6, Sturgeon Bay, WI) for providing the Solanum accessions. We also thank the technical assistance of Denise Rossi, Ashley Jacques, Briana Jacques, and Renesh Bedre (Texas A&M AgriLife Research).
Conflict of Interest
The authors declare that the research was conducted in the absence of any commercial or financial relationships that could be construed as a potential conflict of interest.
The reviewer XW is currently organizing a Research Topic with the author KM.
Publisher's Note
All claims expressed in this article are solely those of the authors and do not necessarily represent those of their affiliated organizations, or those of the publisher, the editors and the reviewers. Any product that may be evaluated in this article, or claim that may be made by its manufacturer, is not guaranteed or endorsed by the publisher.
Supplementary Material
The Supplementary Material for this article can be found online at: https://www.frontiersin.org/articles/10.3389/fmicb.2022.857493/full#supplementary-material
Supplementary Figure 1. Morphological diversity among the tuber-bearing Solanum section Petota species.
Supplementary Figure 2. Phenotypic evaluation of zebra chip (ZC) disease symptoms in wild Solanum section Petota accessions. Screening of accessions was done using a no-choice feeding assay and Candidatus Liberibacter solanacearum-carrying psyllids in the soil in modified cups (A) and pots (B) inside cages. (C) ZC severity index (SI) scale (1, resistant; 2, moderately susceptible; 3, highly susceptible).
Supplementary Figure 3. Preliminary screening of Solanum section Petota accessions for resistance or tolerance to zebra chip disease, with a no-choice feeding assay with Candidatus Liberibacter solanacearum-carrying psyllids. Morphological differences in ZC symptoms of 28-day-old Solanum sect. Petota accessions.
Supplementary Figure 4. The phenotypes of 4-week-old Atlantic and Sb-PI310927 accession zebra chip disease, with a no-choice feeding assay with Candidatus Liberibacter solanacearum-carrying psyllids. Morphological differences in ZC symptoms (leaf curling and wilting) of Atlantic (28-day-old) (A) and Sb-PI310927 (B) are shown. A closeup of ZC symptoms is shown in the inset box.
Supplementary Table 1. List of the Solanum species and accessions screened for zebra chip resistance.
Supplementary Table 2. List of primers used in this study.
References
Allaire, J. J. (2011). “RStudio: Integrated development environment for R,” in The R User Conference (Coventry: University of Warwick). Available online at: https://www.r-project.org/conferences/useR-2011/abstracts/180111-allairejj.pdf
Avila, C. A., Arévalo-Soliz, L. M., Jia, L., Navarre, D. A., Chen, Z., Howe, G. A., et al. (2012). Loss of function of fatty acid desaturase 7 in tomato enhances basal aphid resistance in a salicylate-dependent manner. Plant Physiol. 158, 2028–2041. doi: 10.1104/pp.111.191262
Bamberg, J., and Del Rio, A. (2020). Assessing under-estimation of genetic diversity within wild potato (Solanum) species populations. Am. J. Pot. Res. 97, 547–553. doi: 10.1007/s12230-020-09802-3
Buchman, J. L., Heilman, B. E., and Munyaneza, J. E. (2011). Effects of liberibacter-infective Bactericera cockerelli (Hemiptera: Triozidae) density on zebra chip potato disease incidence, potato yield, and tuber processing quality. J. Econ. Entom. 104, 1783–1792. doi: 10.1603/EC11146
Butler, C. D., Gonzalez, B., Manjunath, K. L., Lee, R. F., Novy, R. G., Miller, J. C., et al. (2011). Behavioral responses of adult potato psyllid, Bactericera cockerelli (Hemiptera: Triozidae), to potato germplasm and transmission of Candidatus Liberibacter psyllaurous. J. Crop Protect. 30, 1233–1238. doi: 10.1016/j.cropro.2011.05.006
Cooper, W. R., and Bamberg, J. B. (2014). Variation in Bactericera cockerelli (Hemiptera: Triozidae) oviposition, survival, and development on Solanum bulbocastanum germplasm. Am. J. Pot. Res. 91, 532–537. doi: 10.1007/s12230-014-9384-x
Cooper, W. R., and Bamberg, J. B. (2016). Variation in susceptibility to potato psyllid, Bactericera cockerelli (Hemiptera: Triozidae), among Solanum verrucosum germplasm accessions. Am. J. Pot. Res. 93, 386–391. doi: 10.1007/s12230-016-9512-x
Dahal, K., Li, X.-Q., Tai, H., Creelman, A., and Bizimungu, B. (2019). Improving potato stress tolerance and tuber yield under a climate change scenario–a current overview. Front. Plant Sci. 10, 563. doi: 10.3389/fpls.2019.00563
Diaz-Montano, J., Vindiola, B. G., Drew, N., Novy, R. G., Miller, J. C., and Trumble, J. T. (2014). Resistance of selected potato genotypes to the potato psyllid (Hemiptera: Triozidae). Am. J. Pot. Res. 91, 363–367. doi: 10.1007/s12230-013-9356-6
Edwards, K., Johnstone, C., and Thompson, C. (1991). A simple and rapid method for the preparation of plant genomic DNA for PCR analysis. Nucleic Acids Res. 19, 1349. doi: 10.1093/nar/19.6.1349
Faostat, F. (2020). FAOSTAT [Online]. Available online at: http://www.fao.org/faostat/en/#data/QC (accessed May 04, 2020).
Fife, A. N., Cruzado, K., Rashed, A., Novy, R. G., and Wenninger, E. J. (2020). Potato psyllid (Hemiptera: Triozidae) behavior on three potato genotypes with tolerance to ‘Candidatus Liberibacter solanacearum’. J. Insect Sci. 20, 15. doi: 10.1093/jisesa/ieaa020
Flanders, K. L., Hawkes, J. G., Radcliffe, E. B., and Lauer, F. I. (1992). Insect resistance in potatoes: sources, evolutionary relationships, morphological and chemical defenses, and ecogeographical associations. Euphytica 61, 83–111. doi: 10.1007/BF00026800
French-Monar, R., Patton Iii, A., Douglas, J., Abad, J., Schuster, G., Wallace, R., et al. (2010). First report of “Candidatus Liberibacter solanacearum” on field tomatoes in The United States. Plant Dis. 94, 481–481. doi: 10.1094/PDIS-94-4-0481A
Greenway, G. (2014). Economic impact of zebra chip control costs on grower returns in seven US states. Am. J. Potato Res. 91, 714–719. doi: 10.1007/s12230-014-9404-x
Greenway, G. A., and Rondon, S. (2018). Economic impacts of zebra chip in Idaho, Oregon, and Washington. Am. J. Pot. Res. 95, 362–367. doi: 10.1007/s12230-018-9636-2
Guenthner, J., Goolsby, J., and Greenway, G. (2012). Use and cost of insecticides to control potato psyllids and zebra chip on potatoes. Southwestern Entomol. 37, 263–270, 268. doi: 10.3958/059.037.0302
Hardigan, M. A., Bamberg, J., Buell, C. R., and Douches, D. S. (2015). Taxonomy and genetic differentiation among wild and cultivated germplasm of Solanum sect. Petota. Plant Genome 8, 1–16. doi: 10.3835/plantgenome2014.06.0025
Harrison, K., Tamborindeguy, C., Scheuring, D. C., Herrera, A. M., Silva, A., Badillo-Vargas, I. E., et al. (2019). Differences in Zebra Chip severity between ‘Candidatus Liberibacter solanacearum’ haplotypes in Texas. Am. J. Pot. Res. 96, 86–93. doi: 10.1007/s12230-018-9692-7
Henne, D., Workneh, F., Wen, A., Price, J., Pasche, J., Gudmestad, N., et al. (2010). Characterization and epidemiological significance of potato plants grown from seed tubers affected by zebra chip disease. Plant Dis. 94, 659–665. doi: 10.1094/PDIS-94-6-0659
Irigoyen, S., Ramasamy, M., Pant, S., Niraula, P., Bedre, R., Gurung, M., et al. (2020). Plant hairy roots enable high throughput identification of antimicrobials against Candidatus Liberibacter spp. Nature Comm. 11, 1–14. doi: 10.1038/s41467-020-19631-x
Levy, J., Ravindran, A., Gross, D., Tamborindeguy, C., and Pierson, E. (2011). Translocation of Candidatus Liberibacter solanacearum, the zebra chip pathogen, in potato and tomato. Phytopathology 101, 1285–1291. doi: 10.1094/PHYTO-04-11-0121
Levy, J., and Tamborindeguy, C. (2014). Solanum habrochaites, a potential source of resistance against Bactericera cockerelli (Hemiptera: Triozidae) and “Candidatus Liberibacter solanacearum”. J. Econ. Entom. 107, 1187–1193. doi: 10.1603/EC13295
Malakar, R., and Tingey, W. M. (2000). Glandular trichomes of Solanum berthaultii and its hybrids with potato deter oviposition and impair growth of potato tuber moth. Entomol. Exp. Appl. 94, 249–257. doi: 10.1046/j.1570-7458.2000.00627.x
Mawassi, M., Dror, O., Bar-Joseph, M., Piasezky, A., Sjölund, J., Levitzky, N., et al. (2018). ‘Candidatus Liberibacter solanacearum’ is tightly associated with carrot yellows symptoms in Israel and transmitted by the prevalent psyllid vector Bactericera trigonica. Phytopathology 108, 1056–1066. doi: 10.1094/PHYTO-10-17-0348-R
Mora, V., Ramasamy, M., Damaj, M. B., Irigoyen, S., Ancona, V., Ibanez, F., et al. (2021). Potato zebra chip: an overview of the disease, control strategies and prospects. Front. Microbiol. 12:700663. doi: 10.3389/fmicb.2021.700663
Munyaneza, J., Crosslin, J., and Upton, J. (2007). Association of Bactericera cockerelli (Homoptera: Psyllidae) with “zebra chip,” a new potato disease in southwestern United States and Mexico. J. Econ. Entom. 100, 656–663. doi: 10.1603/0022-0493(2007)100[656:AOBCHP]2.0.CO;2
Munyaneza, J., Fisher, T., Sengoda, V., Garczynski, S., Nissinen, A., and Lemmetty, A. (2010). First report of “Candidatus Liberibacter solanacearum” associated with psyllid-affected carrots in Europe. Plant Disease 94, 639. doi: 10.1094/PDIS-94-5-0639A
Munyaneza, J., Sengoda, V., Crosslin, J., Garzon-Tiznado, J., and Cardenas-Valenzuela, O. (2009a). First report of “Candidatus Liberibacter solanacearum” in tomato plants in Mexico. Plant Dis. 93, 1076–1076. doi: 10.1094/PDIS-93-10-1076A
Munyaneza, J. E. (2012). Zebra chip disease of potato: biology, epidemiology, and management. Am. J. Potato Res. 89, 329–350. doi: 10.1007/s12230-012-9262-3
Munyaneza, J. E., Crosslin, J. M., and Buchman, J. L. (2009b). Seasonal occurrence and abundance of the potato psyllid, Bactericera cockerelli, in south central Washington. Am. J. Pot. Res. 86, 513. doi: 10.1007/s12230-009-9108-9
Murashige, T., and Skoog, F. (1962). A revised medium for rapid growth and bio assays with tobacco tissue cultures. Physiol. Plant. 15, 473–497. doi: 10.1111/j.1399-3054.1962.tb08052.x
Navarre, D. A., Shakya, R., Holden, J., and Crosslin, J. M. (2009). LC-MS analysis of phenolic compounds in tubers showing zebra chip symptoms. Am. J. Pot. Res 86, 88–95. doi: 10.1007/s12230-008-9060-0
Nwugo, C. C., Sengoda, V. G., Tian, L., and Lin, H. (2017). Characterization of physiological and molecular processes associated with potato response to zebra chip disease. Hortic. Res. 4, 1–9. doi: 10.1038/hortres.2017.69
Portal, U. P. H. I. (2017). Pest Alert: Zebra Chip Disease of Potato. Available online at: https://planthealthportal.defra.gov.uk/pests-and-diseases/high-profile-pests-and-diseases/zebra-chip-disease-of-potato/
Prager, S., Wallis, C., Jones, M., Novy, R., and Trumble, J. (2018). Examining the potential role of foliar chemistry in imparting potato germplasm tolerance to potato psyllid, green peach aphid, and zebra chip disease. J. Econ. Entom. 111, 327–336. doi: 10.1093/jee/tox255
Prager, S. M., Vindiola, B., Kund, G. S., Byrne, F. J., and Trumble, J. T. (2013). Considerations for the use of neonicotinoid pesticides in management of Bactericera cockerelli (Šulk)(Hemiptera: Triozidae). Journal of Crop Protection 54, 84–91. doi: 10.1016/j.cropro.2013.08.001
Rashidi, M., Novy, R. G., Wallis, C. M., and Rashed, A. (2017). Characterization of host plant resistance to zebra chip disease from species-derived potato genotypes and the identification of new sources of zebra chip resistance. PLOS ONE 12, e0183283. doi: 10.1371/journal.pone.0183283
Rubio-Covarrubias, O., Cadena-Hinojosa, M., Prager, S., Wallis, C., and Trumble, J. (2017). Characterization of the tolerance against zebra chip disease in tubers of advanced potato lines from Mexico. Am. J. Potato Res. 94, 342–356. doi: 10.1007/s12230-017-9570-8
Savary, S., Willocquet, L., Pethybridge, S. J., Esker, P., Mcroberts, N., and Nelson, A. (2019). The global burden of pathogens and pests on major food crops. Nat. Ecol. Evol 3, 430–439. doi: 10.1038/s41559-018-0793-y
Sengoda, V. G., Cooper, W. R., Swisher, K. D., Henne, D. C., and Munyaneza, J. E. (2014). Latent period and transmission of “Candidatus Liberibacter solanacearum” by the potato psyllid Bactericera cockerelli (Hemiptera: Triozidae). PLoS ONE 9, e93475. doi: 10.1371/journal.pone.0093475
Spooner, D. M., and Castillo, R. (1997). Reexamination of series relationships of South American wild potatoes (Solanaceae: Solanum sect. Petota): evidence from chloroplast DNA restriction site variation. Am. J. Bot. 84, 671–685. doi: 10.2307/2445904
Szczepaniec, A., Varela, K. A., Kiani, M., Paetzold, L., and Rush, C. M. (2019). Incidence of resistance to neonicotinoid insecticides in Bactericera cockerelli across Southwest US. Crop Protect. 116, 188–195. doi: 10.1016/j.cropro.2018.11.001
Tahzima, R., Maes, M., Achbani, E., Swisher, K., Munyaneza, J., and De Jonghe, K. (2014). First report of ‘Candidatus Liberibacter solanacearum’ on carrot in Africa. Plant Dis. 98, 1426. doi: 10.1094/PDIS-05-14-0509-PDN
Tingey, W. M., and Gibson, R. W. (1978). Feeding and mobility of the potato leafhopper impaired by glandular trichomes of Solanum berthaultii and S. polyadenium. J. Econ. Entom. 71, 856–858. doi: 10.1093/jee/71.6.856
Tingey, W. M., and Laubengayer, J. E. (1981). Defense against the green peach aphid and potato leafhopper by glandular trichomes of Solanum berthaultii. J. Econ. Entom. 74, 721–725. doi: 10.1093/jee/74.6.721
USDA (2020). Potatoes 2019 Summary [Online]. Available online at: https://www.nass.usda.gov›reports›pots0919 (accessed July 02, 2020).
Vigue, S. J. (2018). Identification of Zebra Chip Tolerant Diploid and Tetraploid Potato Genotypes with Good Processing Quality. College Station, TX: Texas A&M University.
Vigue, S. J., Scheuring, D. C., Koym, J. W., Rush, C. M., Workneh, F., Tamborindeguy, C., et al. (2020). Identification of tetraploid potato clones with good processing quality among genotypes with reduced zebra chip disease symptomatology. Am. J. Pot. Res. 97, 565–579. doi: 10.1007/s12230-020-09804-1
Wallis, C. M., Chen, J., and Civerolo, E. L. (2012). Zebra chip-diseased potato tubers are characterized by increased levels of host phenolics, amino acids, and defense-related proteins. Physiol. Mol. Plant Pathol. 78, 66–72. doi: 10.1016/j.pmpp.2012.02.001
Yencho, G. C., and Tingey, W. M. (1994). Glandular trichomes of Solanum berthaultii alter host preference of the Colorado potato beetle, Leptinotarsa decemlineata. Entomol. Exp. Appl. 70, 217–225. doi: 10.1111/j.1570-7458.1994.tb00750.x
Keywords: Candidatus Liberibacter solanacearum, Fastidious bacteria, Bactericera cockerelli, zebra chip (ZC), wild accessions, resistant traits, antibiosis
Citation: Mora V, Ramasamy M, Damaj MB, Irigoyen S, Ancona V, Avila CA, Vales MI, Ibanez F and Mandadi KK (2022) Identification and Characterization of Potato Zebra Chip Resistance Among Wild Solanum Species. Front. Microbiol. 13:857493. doi: 10.3389/fmicb.2022.857493
Received: 18 January 2022; Accepted: 16 June 2022;
Published: 27 July 2022.
Edited by:
Xiangming Xu, National Institute of Agricultural Botany (NIAB), United KingdomReviewed by:
Assunta Bertaccini, University of Bologna, ItalyMaria Julissa Ek-Ramos, Autonomous University of Nuevo León, Mexico
Xuefeng Wang, Chinese Academy of Agricultural Sciences (CAAS), China
Copyright © 2022 Mora, Ramasamy, Damaj, Irigoyen, Ancona, Avila, Vales, Ibanez and Mandadi. This is an open-access article distributed under the terms of the Creative Commons Attribution License (CC BY). The use, distribution or reproduction in other forums is permitted, provided the original author(s) and the copyright owner(s) are credited and that the original publication in this journal is cited, in accordance with accepted academic practice. No use, distribution or reproduction is permitted which does not comply with these terms.
*Correspondence: Kranthi K. Mandadi, a2ttYW5kYWRpJiN4MDAwNDA7dGFtdS5lZHU=
†These authors have contributed equally to this work