- 1Henan Engineering Laboratory for Bioconversion Technology of Functional Microbes, College of Life Sciences, Henan Normal University, Xinxiang, China
- 2School of Life Sciences & Basic Medicine, Xinxiang University, Xinxiang, China
- 3Key Laboratory of Biomedicine and Health Risk Warning of Xinxiang City, Xinxiang, China
Benzalkonium chloride (BC) is widely used for disinfection in food industry. However, prolonged exposure to BC may lead to the emergence of BC adapted strains of Listeria monocytogenes, an important foodborne pathogen. Until now, two communication systems, the LuxS/AI-2 system and the Agr system, have been identified in L. monocytogenes. This study aimed to investigate the role of communication systems in BC adaptation and the effect of BC adaptation on two communication systems and the communication-controlled behaviors in L. monocytogenes. Results demonstrated that the Agr system rather than the LuxS system plays an important role in BC adaptation of L. monocytogenes. Neither luxS expression nor AI-2 production was affected by BC adaptation. On the other hand, the expression of the agr operon and the activity of the agr promoter were significantly increased after BC adaptation. BC adaptation enhanced biofilm formation of L. monocytogenes. However, swarming motility was reduced by BC adaptation. Data from qRT-PCR showed that flagella-mediated motility-related genes (flaA, motA, and motB) were downregulated in BC adapted strains. BC adaptation increased the ability of L. monocytogenes to adhere to and invade Caco-2 cells but did not affect the hemolytic activity. Compared with the wild-type strains, the expression levels of virulence genes prfA, plcA, mpl, actA, and plcB increased more than 2-fold in BC adapted strains; however, lower than 2-fold changes in the expression of hemolysis-associated gene hly were observed. Our study suggests that BC adaptation could increase the expression of the Agr system and enhance biofilm formation, invasion, and virulence of L. monocytogenes, which brings about threats to food safety and public health. Therefore, effective measures should be taken to avoid the emergence of BC adapted strains of L. monocytogenes.
Introduction
Disinfection is an important operation in food industry, which can avoid the microbiological contamination of food products and reduce the risk of foodborne diseases (Pricope et al., 2013). Quaternary ammonium compounds (QACs) are widely used for disinfecting food processing environments. Benzalkonium chloride (BC) is one of the most studied quaternary ammonium disinfectants, and it exhibits good antibacterial activity against many important foodborne pathogens (Langsrud et al., 2003).
Listeria monocytogenes, the causative agent of listeriosis in humans and animals, represents a major foodborne pathogen. It can be found in a wide variety of raw and processed foods (Montero et al., 2015; Shamloo et al., 2019; Falardeau et al., 2021). Since L. monocytogenes has the ability to persist for long periods on equipments and within environments of food industry, the main contamination route for this pathogen is considered to be cross-contamination from food producing environments to food during processing (Mendonça et al., 2012; Jami et al., 2014; Smith et al., 2019). The recommended concentrations of BC for food industry are 200–1,000 μg/ml, which is high enough to inhibit the growth of L. monocytogenes completely (Møretrø et al., 2017). However, niches with sublethal concentrations of BC occur quite often due to improper use of BC, such as insufficient rinsing after disinfection and inadequate dosage (Møretrø et al., 2017). Previous studies have reported the emergence of adaptation to BC (it refers to “adaptive resistance to BC”) when L. monocytogenes are frequently exposed to sublethal concentrations of BC (Aase et al., 2000; Lundén et al., 2003; Romanova et al., 2006). Adaptation to BC can alter cell morphology, tolerance to environmental stresses, and sensitivity to antimicrobial agents in L. monocytogenes (To et al., 2002; Romanova et al., 2006; Bisbiroulas et al., 2011; Rakic-Martinez et al., 2011; Yu et al., 2018). To et al. (2002) have found that cells of BC adapted strains (it refers to “strains with adaptive resistance to BC”) were elongated and slimmer compared with the parent strains. In our previous study, we have reported decreased tolerance to acid, alkali, osmotic, ethanol, and oxidative stresses in BC adapted strains of L. monocytogenes (Yu et al., 2018). Besides, after BC adaptation, L. monocytogenes exhibits cross-adaptation not only to other disinfectants but also to antimicrobial agents, such as cephalosporin, ciprofloxacin, gentamicin, kanamycin, and ethidium bromide (Romanova et al., 2006; Rakic-Martinez et al., 2011; Yu et al., 2018).
Cell-to-cell communication is an important regulatory mechanism that allows bacteria to behave coordinately by producing and releasing chemical signal molecules. Two communication systems have been identified in L. monocytogenes, the autoinducer-2 (AI-2) LuxS and the autoinducing peptide (AIP) mediated Agr. The LuxS enzyme that involves biosynthesis of the signal molecule AI-2 is associated with the repression of biofilm formation of L. monocytogenes (Belval et al., 2006; Sela et al., 2006). The Agr system is composed of four genes (agrB, agrD, agrC, and agrA) organized as an operon, and it plays an important role in biofilm formation, virulence, and invasion in L. monocytogenes (Autret et al., 2003; Rieu et al., 2007; Riedel et al., 2009).
In the current study, we aimed to investigate (i) the role of two communication systems in BC adaptation, (ii) the effect of BC adaptation on expression of the communication systems, and (iii) the effect of BC adaptation on the communication-controlled behaviors, including biofilm formation and virulence in L. monocytogenes.
Materials and Methods
Bacterial Strains and Growth Conditions
Four previously characterized strains of L. monocytogenes (HL09, HL11, HL28, and HL50) were used in this study (Table 1). BC adapted strains of the four wild-type strains were also used in our study, and the sensitivity to BC of four adapted strains has been reported in our previous study (Yu et al., 2018). Each adapted strain carried the wild-type strain designation followed by BCA. Minimum inhibitory concentrations (MICs) of BC for all L. monocytogenes are presented in Table 1. BC adapted strains showed the similar growth curves as their parental strains when incubated in BHI broth at 37°C (data not shown). The increased resistance to BC of adapted strains remained stable for 1 week of daily subcultivation in BHI without BC (data not shown). Listeria monocytogenes strains were grown at 37°C on brain heart infusion (BHI; Oxoid Ltd., Basingstoke, Hampshire, England) agar or in BHI broth.
Construction of Gene Deletion Mutant Strains
The gene deletion mutants of luxS and the entire agr operon derived from HL28 were generated by allelic replacement using a pMAD shuttle vector as described previously (Yu et al., 2018). Primers for construction of gene deletion mutants are listed in Table 2. In brief, an insert containing homologous arms up- and down-stream of the target gene was obtained by the splicing by overlap extension (SOE) PCR. The insert and pMAD were digested and ligated. The recombinant plasmid was electroporated into HL28 and transformants were seleced on BHI agar plates with erythromycin (5 μg/ml; Sigma-Aldrich, St. Louis, MO, United States).
Determination of MICs
The MICs of BC for L. monocytogenes were determined using the broth microdilution method as described previously (Romanova et al., 2006). Briefly, strains were tested in BHI broth using 96-well microtiter plates (Corning Inc., Kennebunk, ME, United States), with an inoculum of 104–105 CFU/ml. The plates were incubated at 37°C for 24 h. The lowest concentration of BC totally preventing growth was taken to be the MIC.
Adaptation to BC
Adaptation to BC of L. monocytogenes was conducted as described previously (Aase et al., 2000). Briefly, strains were subcultivated in BHI containing BC at a concentration of 1/2MIC at 37°C from the start. Then subcultures were serially subcultivated in BHI with increasing BC concentration by steps of 0.5 μg/ml, until there was no growth within 7 days.
Quantitative Real Time-PCR
In this study, the relative expression levels of luxS, agrBDCA, flagella gene (flaA), motility-related genes (motA and motB) and virulence-associated genes (prfA, plcA, hly, mpl, actA and plcB) were assessed by quantitative real time-PCR (qRT-PCR) as previously described (Jiang et al., 2021). qRT-PCR primers for six virulence genes are shown in Table 2, and the primers for luxS, agr, flaA, motA and motB have been reported in our previous study (Jiang et al., 2021).
Detection of AI-2
A bioluminescence assay was used to detect AI-2 as described previously (Taga and Xavier, 2011). Briefly, overnight cultures of L. monocytogenes were centrifugated at 12,000 g for 10 min and the supernatants were filtered using a 0.22-μm-pore size filter (Millipore, Bedford, MA, United States). Then, the culture of Vibrio harveyi BB170 (an AI-2 reporter strain) and the filtered supernatants of L. monocytogenes were mixed at the ratio of 9:1 and incubated at 30°C. The fluorescence values of samples were measured by an EnSpire Multimode Plate Reader (PerkinElmer, Waltham, MA, United States). Vibrio harveyi BB170 and Escherichia coli DH5α were used as the positive control and the negative control, respectively. The relative activity of AI-2 was presented as a percentage of the positive control.
Analysis of the agr Promoter (P2) Activity by β-Galactosidase Assays
The agr promoter (P2)-lacZ fusion was constructed as described previously (O’Driscoll et al., 2004; Collins et al., 2012). Briefly, the DNA fragment containing P2 was cloned into pPTPL, a promoter probe vector and then the recombinant plasmid was electroporated into the wild-type strains and the BC adapted strains. Transformants were selected by BHI agar plates with tetracycline (Sigma-Aldrich). β-galactosidase activity assay was performed based on the method by Miller (Deng et al., 2013). The assays were performed in triplicate independently, and results were presented as the mean in Miller unit.
Microtiter Plate Biofilm Formation Assay
Biofilms were assayed using the microplate method with crystal violet staining as described previously (Djordjevic et al., 2002). Briefly, 200 μl of diluted (1:100 in BHI broth) bacterial culture (bacterial concentration of approximately 104 CFU/ml) were transferred into microtiter plate (Corning). The plates were statically incubated at 37°C for 24 h, 48 h and 72 h. To assess the number of planktonic cells, the cultures (100 μl) were centrifuged, and the pellets were resuspended in 1 ml of sterile saline. The bacterial cultures were then serially diluted and 100 μl volumes were taken for colony counting. To quantify the biofilm production, the medium was removed after incubation and then the wells were gently washed five times with sterile water. Biofilms were stained with 1% crystal violet for 45 min and washed with sterile water. Finally, biofilms were decolorized by 95% ethanol. The absorbance at OD595 nm was measured to determine biofilm production. Finally, the biofilms were visualized under a DMi1 inverted microscope (Leica-Microsystems, Wetzlar, Germany).
Confocal Laser Scanning Microscopy Biofilm Formation Assay
Biofilm formation assay by confocal laser scanning microscopy (CLSM) was performed as described previously (Jiang et al., 2021). Biofilms were prepared by immersing the cover glasses in the wells of 24-well polystyrene plates (Corning). After 48 h of incubation at 37°C, biofilms were washed with sterile water. The Live/Dead BacLight Bacterial viability kit (Molecular Probes, Eugene, OR, United States) was used to stain biofilms. A Leica TCS-SP8 Confocal Laser Scanning Microscope (Leica-Microsystems) was applied for image acquisition. Three-dimensional projections of the biofilms were constructed from the CLSM acquisitions using the IMARIS 7.1 software (Bitplane, Zürich, Switzerland). The COMSTAT software was applied for quantification of biofilm biomass and thickness (Heydorn et al., 2000).
Motility Assay
The swarming motility of all strains was tested on soft tryptic soy broth (TSB; Huankai Ltd., Guangzhou, Guangdong, China) agar plate (0.3% w/v agar) at 25°C and 37°C (Jiang et al., 2021). Bacteria were inoculated onto the agar plate using sterile toothpicks and the diameter of the bacterial swarm was measured 48 h later.
Hemolysis Assay
The hemolytic activity of L. monocytogenes was assayed as described previously (Liu et al., 2016). The bacterial cultures were centrifuged (5,500 × g, 4°C, 10 min), and 250 μl of supernatant were mixed with 900 μl of hemolysin buffer (Solarbio Science & Technology, Beijing, China) and 100 μl of sheep red blood cells (Solarbio). Sterile BHI broth and the sheep red blood cells treated with 1% Triton X-100 (Solarbio) served as the negative control and positive control, respectively. The absorbance of the samples at 543 nm was measured and the relative hemolysis was determined as the percentage of the absorbance presented by the positive control.
Caco-2 Adhesion and Invasion Assays
The ability of wild type and BC adapted strains to adhere to and invade Caco-2 cells was evaluated as described previously (Riedel et al., 2009). Briefly, bacterial cultures of L. monocytogenes were diluted in DMEM (Solarbio) to 1 × 108 CFU/ml and then added to Caco-2 cells at a multiplicity of infection (MOI) of 100. The mixed cells were incubated at 37°C + 5% CO2 for 1 h. For adhesion assay, cells were washed with pre-warmed phosphate buffered saline (PBS; Solarbio) and then lysed with ice-cold distilled water. For invasion assay, cells were incubated in DMEM with 10 μg/ml gentamicin after washing once with pre-warmed PBS. The cells were washed and lysed according to the steps described above. The lysed cells were plated on BHI agar and incubated at 37°C for 24 h.
Statistical Analysis
All data comparisons were analyzed using the unpaired two-tailed Student t test (Microsoft Excel 2010). Differences with values of p lower than 0.05 were considered as statistically significant.
Results
The Agr System Plays an Important Role in BC Adaptation
To investigate the role of two communication systems in BC adaptation of L. monocytogenes, the gene deletion mutant strains of luxS and agr derived from HL28 were constructed in this study. Our results displayed that deletion of luxS or agr had no effect on BC MICs for HL28 (Table 3). After BC adaptation, HL28ΔluxSBCA showed the MIC of BC with 14 μg/ml, the same value as that of HL28BCA; however, the BC MIC of HL28ΔagrBCA was lower than that of HL28BCA (Table 3). These results indicate that the Agr system rather than the LuxS system plays an important role in BC adaptation of L. monocytogenes.
Neither luxS Expression Nor AI-2 Production Was Affected by BC Adaptation
Results from qRT-PCR showed that no obvious changes in the expression levels of luxS were observed in the adapted strains (Figure 1A). As presented in Figure 1B, four wild-type strains of L. monocytogenes had the ability to produce AI-2 and the highest level was observed in HL11. The amount of AI-2 of four BC adapted strains was similar to that of their corresponding wild-type strains.
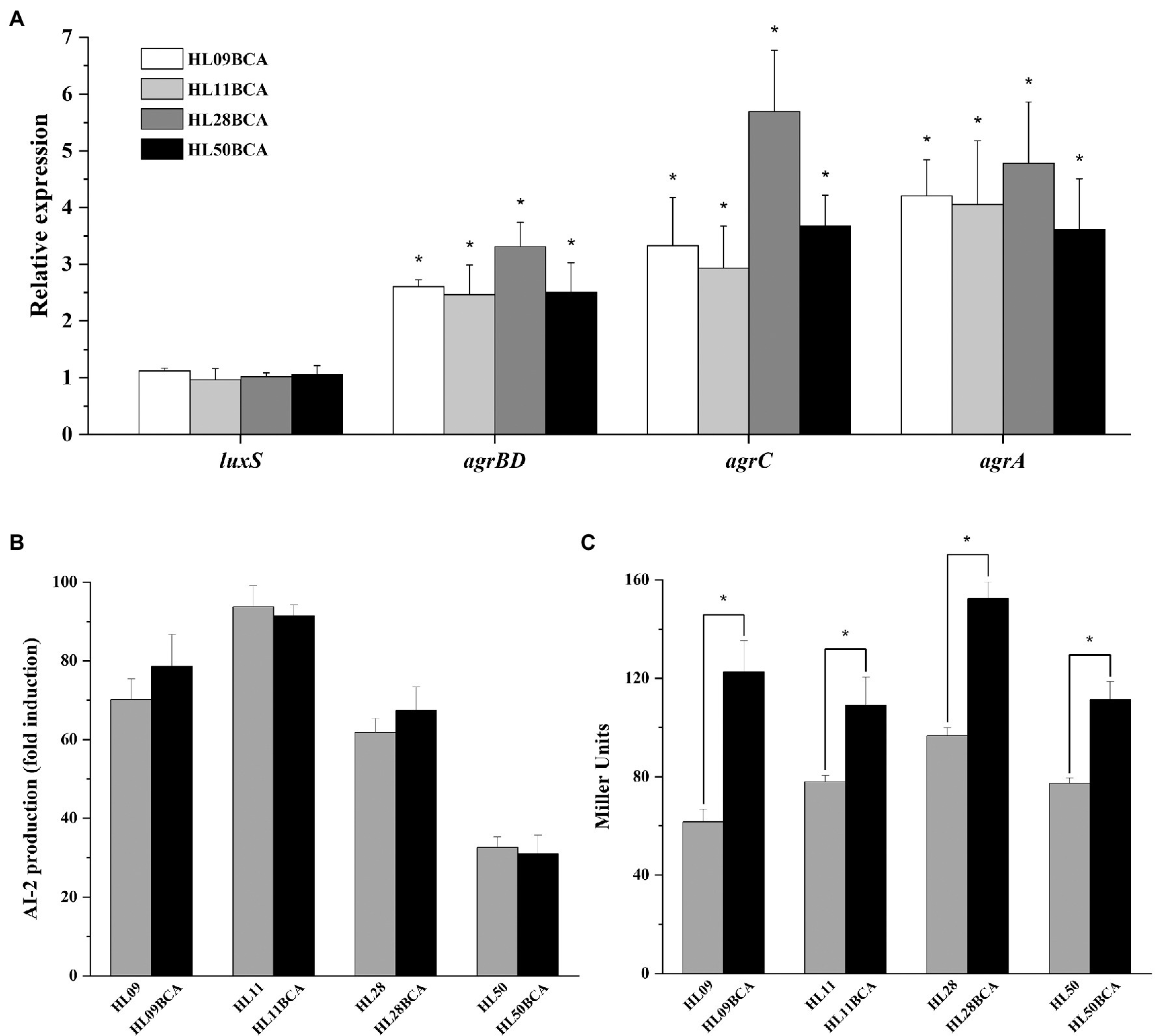
Figure 1. Effect of BC adaptation on (A) the expression levels of luxS and agr, (B) AI-2 production, and (C) the agr promoter (P2) activity. Relative expression levels of luxS and agr are presented as fold changes of the gene tested in BC adapted strain compared to that in its wild-type strain. Error bars represent the standard deviation of triplicate experiments (n = 3). The asterisk indicates a value statistically different from that of the wild-type strain, with p < 0.05.
BC Adaptation Increases the Expression of the Agr System
The expression levels of agr genes in the BC adapted strains were significantly higher (p < 0.05) than those in their corresponding parental strains (Figure 1A). As shown in Figure 1C, the P2 activity of four BC adapted strains was increased significantly (p < 0.05) in relative to the corresponding wild-type strains. BC adaptation resulted in 1.99-, 1.40-, 1.58-, and 1.44-fold increase in the activity of the P2 promoter in HL09, HL11, HL28, and HL50, respectively.
BC Adaptation Enhances Biofilm Formation of Listeria monocytogenes
The biofilm production of L. monocytogenes was quantified by the crystal violet staining method. As shown in Figures 2A–C, all the BC adapted strains demonstrated significantly increased biofilm biomass (p < 0.05) compared with their corresponding wild-type strains over each of 3 days at 37°C. For example, the biofilm biomass of HL09BCA was 47.5%, 60.0%, and 32.1% higher than that of HL09 at 24, 48, and 72 h, respectively. Each wild-type strain grew comparably with its BC adapted strain (Figures 2D–F). Therefore, the difference in biofilm formation is not due to a difference in growth.
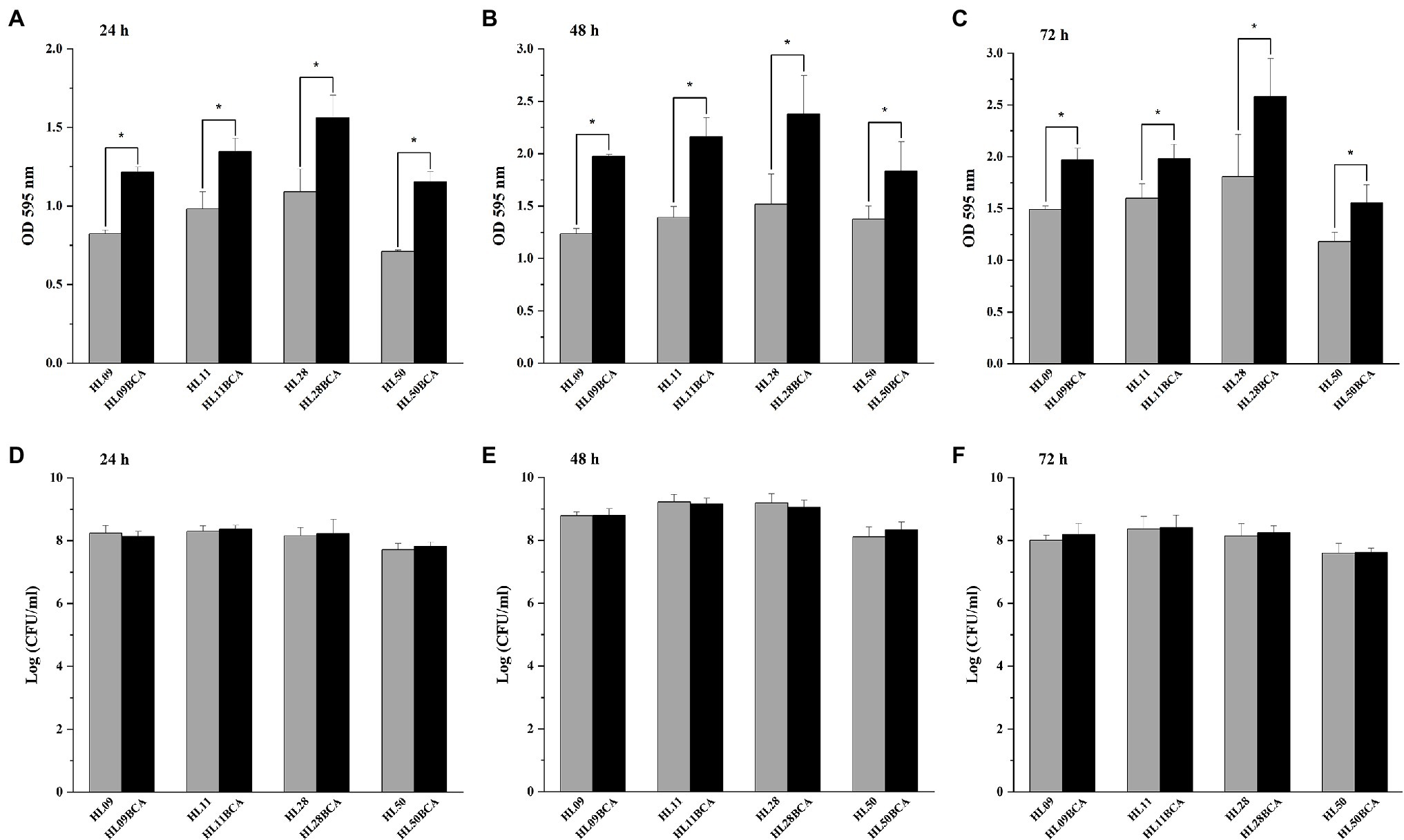
Figure 2. Biofilm formation assay by microtiter plate with crystal violet staining of the wild-type strains and the BC adapted strains of Listeria monocytogenes at 37°C for (A) 24 h, (B) 48 h, and (C) 72 h. Surviving planktonic cells in the bacterial culture incubated at 37°C for (D) 24 h, (E) 48 h, and (F) 72 h. Error bars represent the standard deviation of triplicate experiments (n = 3). The asterisk indicates a value statistically different from that of the wild-type strain, with p < 0.05.
The morphology of L. monocytogenes biofilms was observed using inverted microscope and CLSM. Inverted microscopic images showed that compact biofilm structure with smaller pores was observed in the BC adapted strains, but loose biofilm structure with bigger pores was observed in their corresponding parental strains (Figure 3). CLSM showed that each BC adapted strain formed a denser and thicker biofilm than did its wild type (Figure 4). The results from microscopic analyses of biofilm formation were consistent with those from biofilm biomass determined by the microplate assay, indicating that biofilm formation of L. monocytogenes is enhanced after BC adaptation.
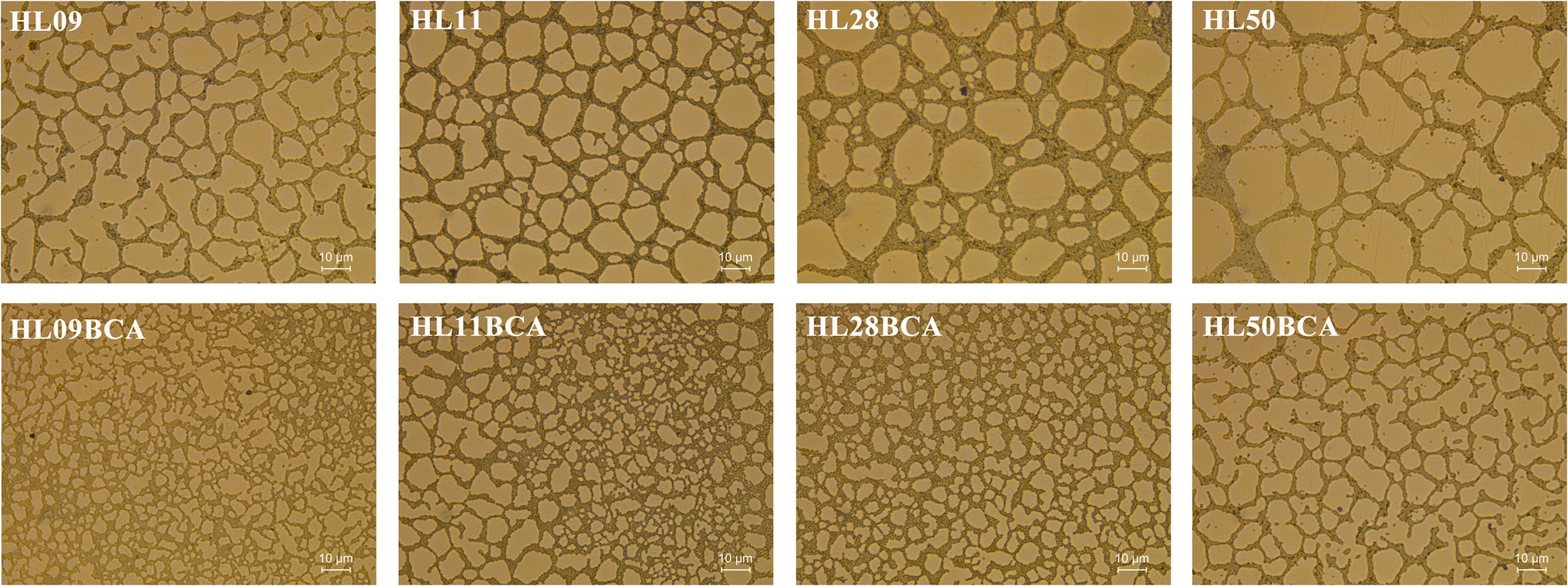
Figure 3. Inverted microscopic analysis of Listeria monocytogenes biofilm. All biofilms were grown at 37°C for 48 h.
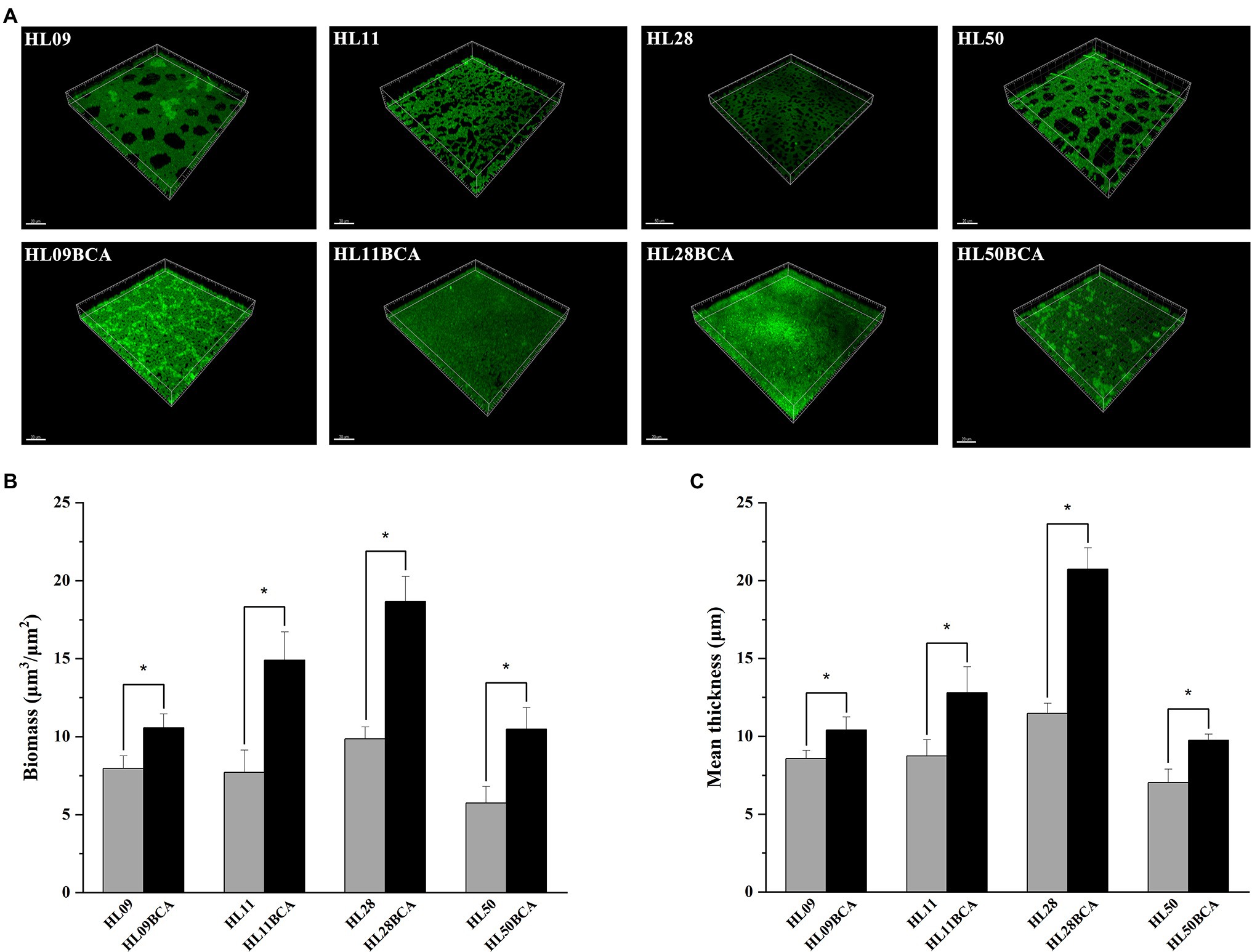
Figure 4. CLSM analysis of Listeria monocytogenes biofilm. (A) CLSM images of Listeria monocytogenes strains. (B) The biofilm biomass. (C) Mean thickness of biofilm. The asterisk indicates a value statistically different from that of the wild-type strain, with p < 0.05.
The biofilm-forming ability of four mutant strains HL28ΔluxS, HL28Δagr, HL28ΔluxSBCA, and HL28ΔagrBCA was also investigated in our study. As shown in Figure 5, the absence of luxS had no influence on biofilm formation of HL28. HL28ΔluxSBCA exhibited a similar biofilm biomass as that of HL28BCA. Compared with the wild type strain HL28, the biofilm biomass was significantly reduced in the gene deletion mutant strain HL28Δagr (p < 0.05). After BC adaptation, the biofilm biomass of HL28ΔagrBCA was higher than that of HL28Δagr (p < 0.05) but still lower than that of HL28BCA (p < 0.05).
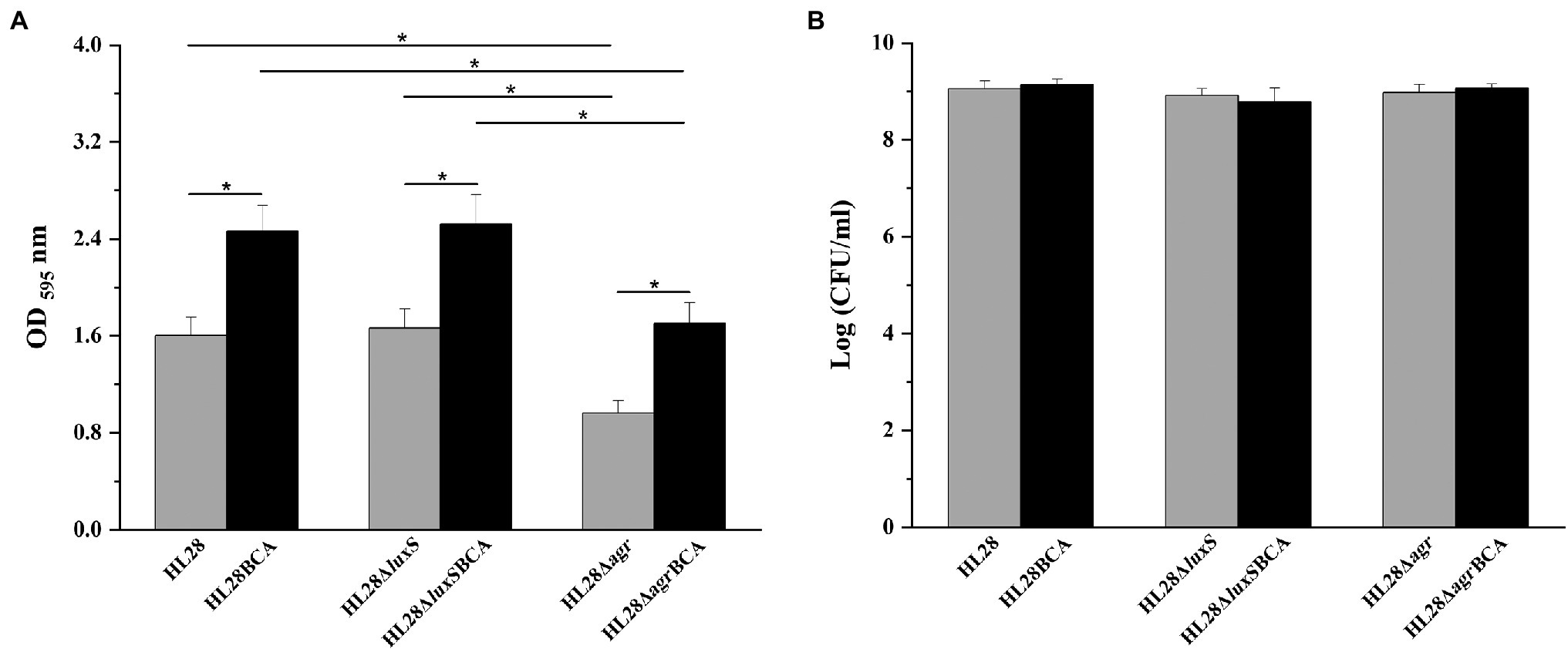
Figure 5. (A) Biofilm biomass of HL28, HL28BCA, HL28ΔluxS, HL28ΔluxSBCA, HL28Δagr and HL28ΔagrBCA at 37°C for 48 h. (B) Surviving planktonic cells in the bacterial culture incubated at 37°C for 48 h. Error bars represent the standard deviation of triplicate experiments (n = 3). The asterisk indicates a value statistically different from that of the wild-type strain, with p < 0.05.
BC Adaptation Reduces Swarming Motility
In this study, we analyzed the motility of L. monocytogenes strains by semisolid swarm plate assays. After incubation at 25°C, all the strains were motile, and each BC adapted strain had a smaller swarm ring than that of its wild type (Figure 6A). At 37°C, none of the strains showed swarming (Figure 6A). Relative expression levels of flagella gene (flaA) and motility-related genes (motA and motB) were also measured by qRT-PCR. As shown in Figure 6B, the flaA, motA, and motB genes in the BC adapted strains were downregulated when compared to their corresponding wild-type strains, however, not all differences were significant. Expression levels of flaA in four BC adapted strains and motA in HL28BCA and HL50BCA were significantly decreased (p < 0.05). These data suggested that BC adaptation reduces the swarming motility of L. monocytogenes.
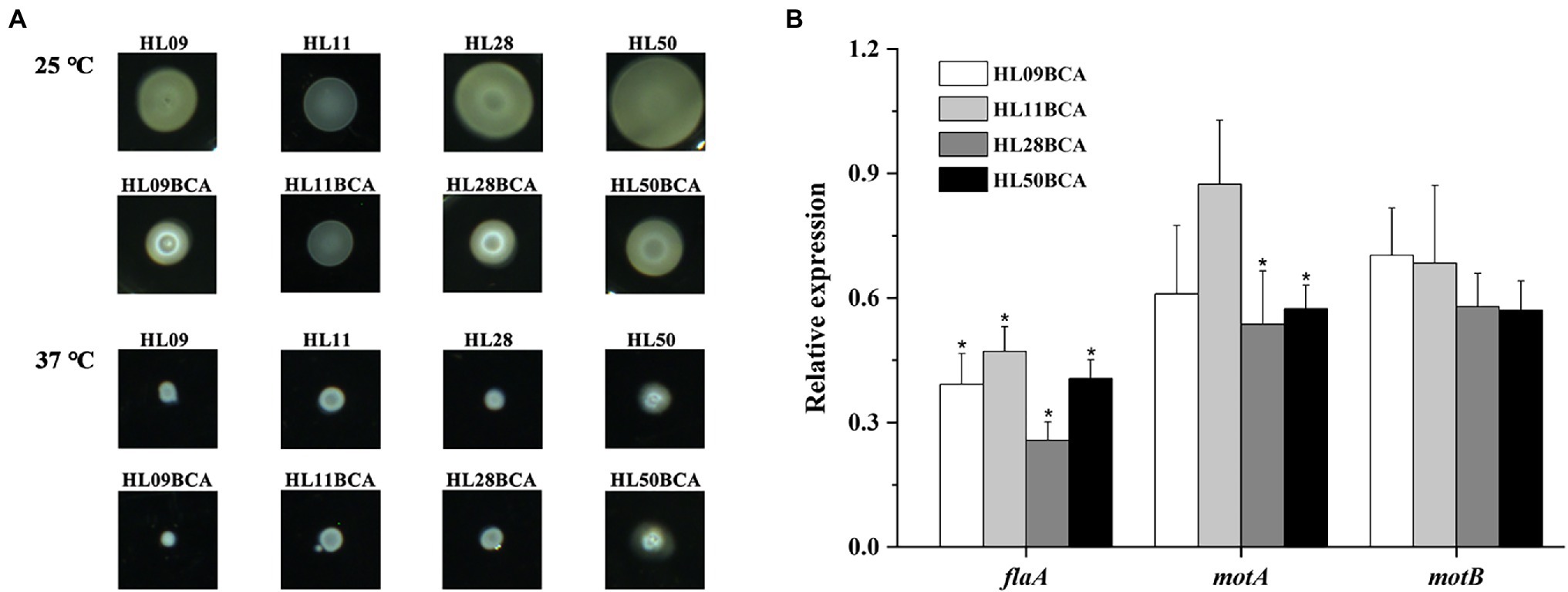
Figure 6. (A) Swarming motility of the wild-type strains and the BC adapted strains of Listeria monocytogenes at 25°C and 37°C. (B) Relative expression levels of flagella gene (flaA) and motility-related genes (motA and motB). The asterisk indicates a value statistically different from that of the wild-type strain, with p < 0.05.
BC Adaptation Increases Cell Adhesion and Invasion
As presented in Figure 7A, there was no significant difference in the hemolytic activity between BC adapted strains and their corresponding wild type strains. The percentage of four BC adapted strains adhesion to Caco-2 cells was significantly higher (p < 0.05) than that of their corresponding wild-type strains. Specifically, BC adaptation increased the percent adherence by 12.6%, 19.9%, 37.3%, and 18.2% in HL09, HL11, HL28, and HL50, respectively. The percent invasion of HL11BCA, HL28BCA, and HL50BCA was increased by 8.79%, 13.9%, and 11.2%, respectively. No significant difference in the percent invasion was observed between HL09 and HL09BCA.
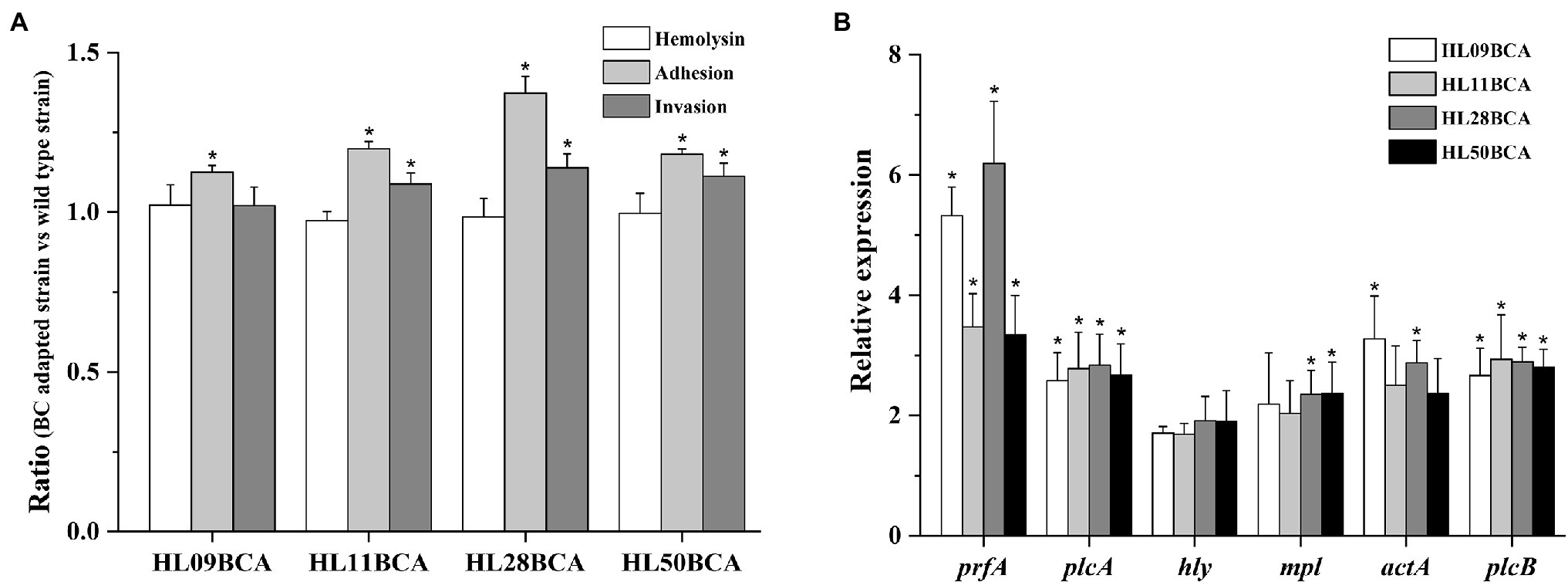
Figure 7. Effect of BC adaptation on (A) hemolysis, Caco-2 adhesion and invasion, and (B) expression of prfA virulence gene cluster. “Ratio” refers to the value of BC adapted strain relative to that of its wild type strain. Error bars indicate standard deviations. The asterisk indicates a value statistically different from that of the wild-type strain, with p < 0.05.
Expression levels of the prfA virulence gene cluster were investigated in this study (Figure 7B). Compared with the wild-type strains, the prfA gene was significantly upregulated (p < 0.05) in the BC adapted strains. The expression levels of plcA, mpl, actA, and plcB increased more than twofold; however, lower than twofold changes in expression of hly were observed in four adapted strains.
Discussion
In the present study, the role of two communication systems in BC adaptation of L. monocytogenes was first investigated. The luxS gene and the agr operon were deleted from HL28, respectively. Since the mutants HL28ΔluxS and HL28Δagr had the same BC MICs as that of HL28, these two systems may not be associated with resistance to BC. The same BC MICs were observed in HL28BCA and HL28ΔluxSBCA, indicating that BC adaptation was not affected by the absence of luxS. Compared with HL28BCA, HL28ΔagrBCA exhibited increased susceptibility to BC, suggesting the role of the Agr system in BC adaptation of L. monocytogenes. The Agr system is not the only mechanism of BC adaptation, because the BC MIC of HL28ΔagrBCA was twice that of HL28Δagr.
To investigate the effect of BC adaptation on the communication systems, four strains of L. monocytogenes isolated from retail food with four different serotypes and their BC adapted strains were selected in our study. The LuxS/AI-2 system is ubiquitous in Gram-negative and Gram-positive bacteria and the production of AI-2 is dependent on LuxS, the key enzyme in the biosynthesis pathway of AI-2 (Sela et al., 2006). Our results suggested that BC adaptation affects neither luxS expression nor AI-2 production.
The agr system in L. monocytogenes consists of the four-gene operon agrBDCA. Of the four proteins encoded by the agr operon, a precursor peptide AgrD is processed into an active signaling molecule AIP by AgrB and then the AIP is released outside the cells (Autret et al., 2003; Garmyn et al., 2009). Upon accumulation in the extracellular space, the AIP activates the two-component system consisting of AgrC (receptor-histidine kinase) and AgrA (response regulator; Autret et al., 2003; Garmyn et al., 2009). Our results showed that BC adaptation increased the expression of the agr operon. The putative promoter of the agr operon, the P2 promoter, has been found upstream of agrB in L. monocytogenes (Autret et al., 2003). In our study, the effect of BC adaptation on the P2 promoter activity was also investigated. After BC adaptation, the activity of P2 was significantly increased in four tested strains, providing further evidence that BC adaptation could activate the transcription of the Agr system.
Listeria monocytogenes is capable of forming biofilm on various food processing surfaces. Biofilms by L. monocytogenes represent an important source of contamination of raw materials and processed products, which brings about a great potential threat to food safety. Given that the Agr system positively regulates biofilm formation of L. monocytogenes (Rieu et al., 2007; Riedel et al., 2009), a promotion of Agr may enhance the development of bacterial biofilms. BC adaptation has been confirmed to induce the Agr system in this study. Thus, we speculated that BC adaptation also affects biofilm formation of L. monocytogenes. Our results demonstrated that the biofilm-forming ability of BC adapted strains was much stronger than that of wild-type strains, suggesting that BC adaptation could enhance biofilm formation mediated by the Agr system in L. monocytogenes.
Romanova et al. (2007) assessed biofilm formation of three L. monocytogenes strains and their BC adapted strains by crystal violet staining, and they found that the adaptation to BC does not significantly affect biofilm-forming ability. Their findings were different from our results, which may be caused by different experimental protocols. In the previous study, biofilm was incubated at 30°C for 5 days and the medium was replaced with fresh broth every 24 h (Romanova et al., 2007). In our study, biofilm was incubated at 37°C for 24, 48, and 72 h, and the medium was not changed during the incubation period. Additionally, biofilm biomass was determined by measuring the absorbance at 530 nm and the observed OD530 nm values were in the range from 0.03 to 0.17 in the study of Romanova et al. (2007), which were much lower than our OD600 nm values.
Previous studies have reported that the luxS deletion mutant strain exhibited an enhanced ability to form biofilm compared with the wild type strain of L. monocytogenes EGD-e (Belval et al., 2006; Sela et al., 2006). However, the absence of luxS had no influence on biofilm formation of HL28 in our study. The different results may be due to the different strains used. Our results also showed the biofilm biomass of HL28Δagr reduced when compared with that of HL28, providing further evidence for the positive regulation of Agr on biofilm formation of L. monocytogenes. The biofilm forming ability of HL28ΔluxSBCA and HL28ΔagrBCA was higher than that of HL28ΔluxS and HL28Δagr, confirming that BC adaptation could enhance biofilm formation of L. monocytogenes.
Many studies have confirmed the importance of flagellum-mediated motility in the first stages of biofilm formation (Shrout et al., 2011; Guttenplan and Kearns, 2013). Swarming motility is a specialized form of movement and enables flagellated bacteria to coordinately move atop solid surfaces (Fraser and Hughes, 1999). Increased swarming motility may improve biofilm-forming ability. The flagellum of L. monocytogenes is composed of flagellin monomers encoded by the flaA gene. The motA and motB genes encode the flagellar motor protein MotA and the flagellar motor rotation MotB, respectively. Previous studies have reported that these flagellar motility genes (flaA, motA, and motB) are critical for L. monocytogenes biofilm formation (Lemon et al., 2007; Todhanakasem and Young, 2008). In this study, BC adaptation reduced not only swarming motility but also the expression levels of flaA, motA and motB. Our results suggested that BC adaptation had opposite effects on swarming motility and biofilm formation. Although swarming motility is considered as one of the factors affecting biofilm formation, there is no clear positive correlation between them (O’May et al., 2012; Henly et al., 2019). Thus, it was not surprising that BC adapted strains exhibited enhanced biofilm formation and decreased swarming motility.
Listeria monocytogenes is an intracellular pathogen that can cause severe invasive infections mainly in the newborn, the elderly, and the immunocompromised. Actually, the process of L. monocytogenes infecting host cells is under the modulation of virulence factors (Camejo et al., 2011). The virulence gene cluster composed of prfA, plcA, hly, mpl, actA, and plcB has been identified in L. monocytogenes, the products of which play a crucial role in pathogenesis (Sheehan et al., 1995). Among these genes, hly encodes listeriolysin O (LLO), which is a Listeria-specific hemolysin and major virulence factor required for escape of bacteria from the phagocytic compartment into the cytoplasm (Sheehan et al., 1995). All the genes in this virulence gene cluster are controlled by the transcriptional activator PrfA (Sheehan et al., 1995). Previous studies reported that the deletion of agrD resulted in reduced virulence and expression of prfA-dependent virulence gene cluster, indicating that Agr system is involved in virulence mediated by this gene cluster (Riedel et al., 2009). Our results found that BC adaptation increased the ability of L. monocytogenes to adhere to and invade Caco-2 cells. Indeed, prfA and several prfA-controlled virulence genes were upregulated in BC adapted strains. However, the hemolysis activity of L. monocytogenes was not affected by BC adaptation. The expression levels of hly were not significantly changed in BC adapted strains. There is the possibility that hly is controlled by other regulator(s).
Previous studies reported that the sublethal concentrations of BC inhibited biofilm formation and Caco-2 cell invasion of L. monocytogenes (Pricope et al., 2013; Ortiz et al., 2014). In the current study, results suggested that BC adaptation increased the ability to form biofilms and invade Caco-2 cells in L. monocytogenes. These findings are not contradictory because BC adaptation is different from BC exposure. Specifically, prolonged exposure to BC is required for L. monocytogenes strains to develop adaptive tolerance to this disinfectant. Compared with its wild-type strain, BC adapted strain could exhibit changes in cell morphology, environmental stress response, antimicrobial resistance, and so on. These phenotype changes may be due to mutations.
The Agr system plays an important role in BC adaptation of L. monocytogenes. BC adaptation promotes the Agr system of L. monocytogenes and consequently enhances bacterial behaviors controlled by this communication system. BC adapted strains of L. monocytogenes exhibited increased ability to form biofilm and adhere to and invade Caco-2 cells. Therefore, the presence of BC adapted strains in food processing environments may increase the risk of food contamination by L. monocytogenes and bring about threats to food safety and public health. Our study indicates the importance of proper use of disinfectants. The operators should use the disinfectant BC according to standardized protocols in food industry, which may reduce bacterial exposure to sublethal concentrations of BC and the emergence of BC adapted strains.
Data Availability Statement
The raw data supporting the conclusions of this article will be made available by the authors, without undue reservation.
Author Contributions
XjJ, CJ, SR, and RK performed the experiments. XbJ analyzed the data and drafted the manuscript. TY and SQ designed and supervised the study. All authors contributed to the article and approved the submitted version.
Funding
This work was supported by the Cultivation Fund of the National Scientific Research Project of Henan Normal University (2020PL04), the Natural Science Foundation of Henan Province (202300410018), and the Key Project of Natural Science of the Education Department of Henan Province (22A180003).
Conflict of Interest
The authors declare that the research was conducted in the absence of any commercial or financial relationships that could be construed as a potential conflict of interest.
Publisher’s Note
All claims expressed in this article are solely those of the authors and do not necessarily represent those of their affiliated organizations, or those of the publisher, the editors and the reviewers. Any product that may be evaluated in this article, or claim that may be made by its manufacturer, is not guaranteed or endorsed by the publisher.
Acknowledgments
The authors are grateful to Douwe van Sinderen for providing the pPTPL plasmid and Jean-Francois Collet for providing E. coli MC1000 (cloning host for pPTPL).
References
Aase, B., Sundheim, G., Langsrud, S., and Rørvik, L. M. (2000). Occurrence of and a possible mechanism for resistance to a quaternary ammonium compound in Listeria monocytogenes. Int. J. Food Microbiol. 62, 57–63. doi: 10.1016/S0168-1605(00)00357-3
Autret, N., Raynaud, C., Dubail, I., Berche, P., and Charbit, A. (2003). Identification of the agr locus of listeria monocytogenes: role in bacterial virulence. Infect. Immun. 71, 4463–4471. doi: 10.1128/IAI.71.8.4463-4471.2003
Belval, S. C., Gal, L., Margiewes, S., Garmyn, D., Piveteau, P., and Guzzo, J. (2006). Assessment of the roles of LuxS, S-ribosyl homocysteine, and autoinducer 2 in cell attachment during biofilm formation by Listeria monocytogenes EGD-e. Appl. Environ. Microbiol. 72, 2644–2650. doi: 10.1128/AEM.72.4.2644-2650.2006
Bisbiroulas, P., Psylou, M., Iliopoulou, I., Diakogiannis, I., Berberi, A., and Mastronicolis, S. K. (2011). Adaptational changes in cellular phospholipids and fatty acid composition of the food pathogen Listeria monocytogenes as a stress response to disinfectant sanitizer benzalkonium chloride. Lett. Appl. Microbiol. 52, 275–280. doi: 10.1111/j.1472-765X.2010.02995.x
Camejo, A., Carvalho, F., Reis, O., Leitão, E., Sousa, S., and Cabanes, D. (2011). The arsenal of virulence factors deployed by Listeria monocytogenes to promote its cell infection cycle. Virulence 2, 379–394. doi: 10.4161/viru.2.5.17703
Collins, B., Guinane, C. M., Cotter, P. D., Hill, C., and Paul Ross, R. (2012). Assessing the contributions of the liaS histidine kinase to the innate resistance of Listeria monocytogenes to nisin, cephalosporins, and disinfectants. Appl. Environ. Microbiol. 78, 2923–2929. doi: 10.1128/AEM.07402-11
Deng, Z., Shan, Y., Pan, Q., Gao, X., and Yan, A. (2013). Anaerobic expression of the gadE-mdtEF multidrug efflux operon is primarily regulated by the two-component system ArcBA through antagonizing the H-NS mediated repression. Front. Microbiol. 4:194. doi: 10.3389/fmicb.2013.00194
Djordjevic, D., Wiedmann, M., and McLandsborough, L. A. (2002). Microtiter plate assay for assessment of listeria monocytogenes biofilm formation. Appl. Environ. Microbiol. 68, 2950–2958. doi: 10.1128/AEM.68.6.2950-2958.2002
Falardeau, J., Trmčić, A., and Wang, S. (2021). The occurrence, growth, and biocontrol of Listeria monocytogenes in fresh and surface-ripened soft and semisoft cheeses. Compr. Rev. Food Sci. Food Saf. 20, 4019–4048. doi: 10.1111/1541-4337.12768
Fraser, G. M., and Hughes, C. (1999). Swarming motility. Curr. Opin. Microbiol. 2, 630–635. doi: 10.1016/S1369-5274(99)00033-8
Garmyn, D., Gal, L., Lemaitre, J. P., Hartmann, A., and Piveteau, P. (2009). Communication and autoinduction in the species Listeria monocytogenes: A central role for the agr system. Commun. Integr. Biol. 2, 371–374. doi: 10.4161/cib.2.4.8610
Guttenplan, S. B., and Kearns, D. B. (2013). Regulation of flagellar motility during biofilm formation. FEMS Microbiol. Rev. 37, 849–871. doi: 10.1111/1574-6976.12018
Henly, E. L., Dowling, J. A. R., Maingay, J. B., Lacey, M. M., Smith, T. J., and Forbes, S. (2019). Biocide exposure induces changes in susceptibility, pathogenicity, and biofilm formation in uropathogenic Escherichia coli. Antimicrob. Agents Chemother. 63, e01892–e01818. doi: 10.1128/AAC.01892-18
Heydorn, A., Nielsen, A. T., Hentzer, M., Sternberg, C., Givskov, M., Ersboll, B. K., et al. (2000). Quantification of biofilm structures by the novel computer program COMSTAT. Microbiology 146, 2395–2407. doi: 10.1099/00221287-146-10-2395
Jami, M., Ghanbari, M., Zunabovic, M., Domig, K. J., and Kneifel, W. (2014). Listeria monocytogenes in aquatic food products—a review. Compr. Rev. Food Sci. Food Saf. 13, 798–813. doi: 10.1111/1541-4337.12092
Jiang, X., Ren, S., Geng, Y., Jiang, C., Liu, G., Wang, H., et al. (2021). Role of the VirSR-VirAB system in biofilm formation of Listeria monocytogenes EGD-e. Food Res. Int. 145:110394. doi: 10.1016/j.foodres.2021.110394
Langsrud, S., Singh, M., Heir, E., and Holck, A. L. (2003). Bacterial disinfectant resistance-a challenge for the food industry. Int. Biodeterior. Biodegradation. 51, 283–290. doi: 10.1016/S0964-8305(03)00039-8
Lemon, K. P., Higgins, D. E., and Kolter, R. (2007). Flagellar motility is critical for listeria monocytogenes biofilm formation. J. Bacteriol. 189, 4418–4424. doi: 10.1128/JB.01967-06
Liu, Z., Meng, R., Zhao, X., Shi, C., Zhang, X., Zhang, Y., et al. (2016). Inhibition effect of tea tree oil on Listeria monocytogenes growth and exotoxin proteins listeriolysin O and p60 secretion. Lett. Appl. Microbiol. 63, 450–457. doi: 10.1111/lam.12666
Lundén, J., Autio, T., Markkula, A., Hellström, S., and Korkeala, H. (2003). Adaptive and cross-adaptive responses of persistent and non-persistent Listeria monocytogenes strains to disinfectants. Int. J. Food Microbiol. 82, 265–272. doi: 10.1016/S0168-1605(02)00312-4
Mendonça, K. S., Michael, G. B., von Laer, A. E., Menezes, D. B., Cardoso, M. R. I., and Da Silva, W. P. (2012). Genetic relatedness among Listeria monocytogenes isolated in foods and food production chain in southern Rio Grande do Sul, Brazil. Food Control 28, 171–177. doi: 10.1016/j.foodcont.2012.04.014
Montero, D., Bodero, M., Riveros, G., Lapierre, L., Gaggero, A., Vidal, R. M., et al. (2015). Molecular epidemiology and genetic diversity of Listeria monocytogenes isolates from a wide variety of ready-to-eat foods and their relationship to clinical strains from listeriosis outbreaks in Chile. Front. Microbiol. 6:384. doi: 10.3389/fmicb.2015.00384
Møretrø, T., Schirmer, B. C. T., Heir, E., Fagerlund, A., Hjemli, P., and Langsrud, S. (2017). Tolerance to quaternary ammonium compound disinfectants may enhance growth of Listeria monocytogenes in the food industry. Int. J. Food Microbiol. 241, 215–224. doi: 10.1016/j.ijfoodmicro.2016.10.025
O’Driscoll, J., Glynn, F., Cahalane, O., O’Connell-Motherway, M., Fitzgerald, G. F., and Van Sinderen, D. (2004). Lactococcal plasmid pNP40 encodes a novel, temperature-sensitive restriction-modification system. Appl. Environ. Microbiol. 70, 5546–5556. doi: 10.1128/AEM.70.9.5546-5556.2004
O’May, C., Ciobanu, A., Lam, H., and Tufenkji, N. (2012). Tannin derived materials can block swarming motility and enhance biofilm formation in Pseudomonas aeruginosa. Biofouling 28, 1063–1076. doi: 10.1080/08927014.2012.725130
Ortiz, S., López, V., and Martínez-Suárez, J. V. (2014). The influence of subminimal inhibitory concentrations of benzalkonium chloride on biofilm formation by Listeria monocytogenes. Int. J. Food Microbiol. 189, 106–112. doi: 10.1016/j.ijfoodmicro.2014.08.007
Pricope, L., Nicolau, A., Wagner, M., and Rychli, K. (2013). The effect of sublethal concentrations of benzalkonium chloride on invasiveness and intracellular proliferation of Listeria monocytogenes. Food Control 31, 230–235. doi: 10.1016/j.foodcont.2012.09.031
Rakic-Martinez, M., Drevets, D. A., Dutta, V., Katic, V., and Kathariou, S. (2011). Listeria monocytogenes strains selected on ciprofloxacin or the disinfectant benzalkonium chloride exhibit reduced susceptibility to ciprofloxacin, gentamicin, benzalkonium chloride, and other toxic compounds. Appl. Environ. Microbiol. 77, 8714–8721. doi: 10.1128/AEM.05941-11
Riedel, C. U., Monk, I. R., Casey, P. G., Waidmann, M. S., Gahan, C. G. M., and Hill, C. (2009). AgrD-dependent quorum sensing affects biofilm formation, invasion, virulence and global gene expression profiles in Listeria monocytogenes. Mol. Microbiol. 71, 1177–1189. doi: 10.1111/j.1365-2958.2008.06589.x
Rieu, A., Weidmann, S., Garmyn, D., Piveteau, P., and Guzzo, J. (2007). Agr system of Listeria monocytogenes EGD-e: role in adherence and differential expression pattern. Appl. Environ. Microbiol. 73, 6125–6133. doi: 10.1128/AEM.00608-07
Romanova, N. A., Wolffs, P. F. G., Brovko, L. Y., and Griffiths, M. W. (2006). Role of efflux pumps in adaptation and resistance of Listeria monocytogenes to benzalkonium chloride. Appl. Environ. Microbiol. 72, 3498–3503. doi: 10.1128/AEM.72.5.3498
Romanova, N. A., Gawande, P. V., Brovko, L. Y., and Griffiths, M. W. (2007). Rapid methods to assess sanitizing efficacy of benzalkonium chloride to Listeria monocytogenes biofilms. J. Microbiol. Methods 71, 231–237. doi: 10.1016/j.mimet.2007.09.002
Sela, S., Frank, S., Belausov, E., and Pinto, R. (2006). A mutation in the luxS gene influences Listeria monocytogenes biofilm formation. Appl. Environ. Microbiol. 72, 5653–5658. doi: 10.1128/AEM.00048-06
Shamloo, E., Hosseini, H., Moghadam, A. Z., Larsen, H. M., Haslberger, A., and Alebouyeh, M. (2019). Importance of Listeria monocytogenes in food safety: A review of its prevalence, detection, and antibiotic resistance. Iran. J. Vet. Res. 20, 241–254.
Sheehan, B., Klarsfeld, A., Msadek, T., and Cossart, P. (1995). Differential activation of virulence gene expression by PrfA, the Listeria monocytogenes virulence regulator. J. Bacteriol. 177, 6469–6476. doi: 10.1128/jb.177.22.6469-6476.1995
Shrout, J. D., Tolker-Nielsen, T., Givskov, M., and Parsek, M. R. (2011). The contribution of cell-cell signaling and motility to bacterial biofilm formation. MRS Bull. 36, 367–373. doi: 10.1557/mrs.2011.67
Smith, A., Hearn, J., Taylor, C., Wheelhouse, N., Kaczmarek, M., Moorhouse, E., et al. (2019). Listeria monocytogenes isolates from ready to eat plant produce are diverse and have virulence potential. Int. J. Food Microbiol. 299, 23–32. doi: 10.1016/j.ijfoodmicro.2019.03.013
Taga, M. E., and Xavier, K. B. (2011). Methods for analysis of bacterial autoinducer-2 production. Curr. Protoc. Microbiol. Chapter 1, Unit1C.1–Unit1C.15. doi: 10.1002/9780471729259.mc01c01s23
To, M. S., Favrin, S., Romanova, N., and Griffiths, M. W. (2002). Postadaptational resistance to benzalkonium chloride and subsequent physicochemical modifications of Listeria monocytogenes. Appl. Environ. Microbiol. 68, 5258–5264. doi: 10.1128/AEM.68.11.5258-5264.2002
Todhanakasem, T., and Young, G. M. (2008). Loss of flagellum-based motility by Listeria monocytogenes results in formation of hyperbiofilms. J. Bacteriol. 190, 6030–6034. doi: 10.1128/JB.00155-08
Keywords: Listeria monocytogenes, benzalkonium chloride, adaptation, the Agr system, biofilm formation
Citation: Jiang X, Jiang C, Yu T, Jiang X, Ren S, Kang R and Qiu S (2022) Benzalkonium Chloride Adaptation Increases Expression of the Agr System, Biofilm Formation, and Virulence in Listeria monocytogenes. Front. Microbiol. 13:856274. doi: 10.3389/fmicb.2022.856274
Edited by:
Romain Briandet, Institut National de recherche pour l’agriculture, l’alimentation et l’environnement (INRAE), FranceReviewed by:
Efstathios D. Giaouris, University of the Aegean, GreecePierre-Emmanuel Douarre, Agence Nationale de Sécurité Sanitaire de l’Alimentation, de l’Environnement et du Travail (ANSES), France
Copyright © 2022 Jiang, Jiang, Yu, Jiang, Ren, Kang and Qiu. This is an open-access article distributed under the terms of the Creative Commons Attribution License (CC BY). The use, distribution or reproduction in other forums is permitted, provided the original author(s) and the copyright owner(s) are credited and that the original publication in this journal is cited, in accordance with accepted academic practice. No use, distribution or reproduction is permitted which does not comply with these terms.
*Correspondence: Tao Yu, yutao@xxu.edu.cn