- 1Department of Bacteriology, Capital Institute of Pediatrics, Beijing, China
- 2Children’s Hospital Affiliated to Capital Institute of Pediatrics, Beijing, China
With the increasingly severe problem of bacterial resistance, colistin, as the last line of defense, has attracted attention again. Mobile colistin resistance (mcr-1) gene is involved in the horizontal transmission of colistin resistance in Gram-negative bacteria (GNB), which is a serious threat to human health. Therefore, rapid detection of mcr-1 gene presence in clinical samples is crucial. In this study, a Recombinase-aided amplification(RAA) method for mcr-1 was successfully constructed, with sensitivity of 20 copies/reaction. In addition, amplification signal could only be detected in the strain containing mcr-1 gene among 14 different bacterial species. The method was then used to test a total of 672 clinical samples from a pediatric hospital in Beijing. Five strains harbored mcr-1 genes were isolated from mcr-1-positive clinical samples and identified as Escherichia coli. Multi-locus sequence typing (MLST) analysis showed that the five E. coli belonged to different ST types. Notably, the mcr-1 gene from the isolates could be transferred conjugately to the recipient strain E. coli J53, with highest transfer efficiency up to 57–58%, suggesting that the mcr-1 gene was located on the plasmid. These findings showed that the RAA assay has potential to be a rapid and sensitive mcr-1 gene screening test for clinical samples, and mcr-1 could be transmitted vertically and horizontally between and within bacterial species in a plasmid-mediated manner.
Introduction
For the past few decades, polymyxins are used for the treatment of multidrug-resistant GNB when better treatment options are not available (Li et al., 2006; Lim et al., 2010). The main mechanism of action of polymyxin is that its positive charge binds to the negative charge of the phosphate groups of lipid A on lipopolysaccharide (LPS) localized on the outer membrane of Gram-negative bacteria (GNB) (Schindler and Osborn, 1979; Srimal et al., 1996; Moffatt et al., 2019). Ca2+ is essential for maintaining the structural stability of LPS, and polymyxin can replace LPS-bound Mg2+ and Ca2+, increasing the permeability of bacterial outer membrane and causing bacterial death (Schindler and Osborn, 1979). Many factors, such as two-component systems PhoP/PhoQ (Elizabeth et al., 2021) and efflux pumps MexXY-OprM (Poole et al., 2015), can influence the resistance of bacteria to polymyxin. However, the plasmid-mediated mcr genes can rapidly spread via horizontal gene transfer (HGT) among humans, animals, and the environment, posing the greatest risk to human health (Hussein et al., 2021).
The mcr genes encode phosphoethanolamine (pEtN) transferase enzymes, which can reduce the electrostatic interaction between polymyxin and lipid A of LPS by binding pEtN moiety to the lipid A of GNB, creating bacteria resistant to polymyxin (Baron et al., 2016). So far, ten variants of the mcr gene, mcr-1 to mcr-10, have been identified in various bacteria (Wang et al., 2020). The plasmid-mediated colistin resistance gene mcr-1 was the first to be identified from E. coli in 2015 and is more widely disseminated than the other nine variants, while mcr-2 to mcr-10 have only occasionally been reported (Liu et al., 2016; Xiaomin et al., 2020). The spread of the mcr-1 gene makes multidrug-resistant GNB more resistant to polymyxins, which poses a serious threat to public health (Xiaomin et al., 2020). Hence, a rapid and accurate method of detecting the mcr-1 gene carrier strain would be helpful to guide clinical medication and inhibit the mcr-1 gene spread.
The polymerase chain reaction (PCR) method has been used to detect the presence of mcr genes, but it is time consuming (Rebelo et al., 2018; Mentasti et al., 2021). RAA assay is a highly efficient method for the rapid detection of specific target genes. Based on isothermal amplification technology, the RAA assay can be completed within 15–30 min at 39°C and has been widely used in clinical applications, such as for identifying New Delhi Metallo-β-Lactamase Gene (Feng et al., 2021), blaKPC (Zhang et al., 2021) and other applications (Fan et al., 2019; Qi et al., 2019; Shen et al., 2019; Xue et al., 2020a,b). Here, an RAA assay was developed to detect the mcr-1 gene in clinical samples, which was proven to have high specificity and sensitivity. To further analyze the characteristics of strains harboring mcr-1 genes obtained from clinical samples, the minimum inhibitory concentrations (MICs) and the HGT of mcr-1 to these isolates were investigated.
Materials and Methods
Bacterial Strains, Growth Conditions, and Primers
Information on all of the strains used in this study is listed in Table 1. All strains were cultured in Luria-Bertani (LB) broth (5 g/L yeast extract, 10 g/L sodium chloride, and 10 g/L tryptone) at 37°C in a shaker at 200 rpm. All primers involved in the construction of plasmid, PCR and RAA assay are listed in Table 2.
Acquisition, Isolation, and Identification of Clinical Strains
Six hundred and seventy-two stool samples were collected from inpatients of Capital Institute of Pediatrics, Beijing, China. After dilution, stool samples with different dilution gradients were plated on LB plates with 2 mg/L of colistin sulfate salt and incubated at 35–37°C for 24 h. The screened single colonies were identified using the VITEK® 2 compact system (bioMérieux, Nürtingen, Germany). Standard PCR and RAA assay were used simultaneously to detect whether the strains harbored the mcr-1 gene. Meanwhile, a sample of approximately 200 mg was subjected to DNA extraction using a kit (Vazyme Biotech Co., Ltd., Nanjing, China) for further use.
Primer Design for the Recombinase-Aided Amplification Assay
The sequence of the mcr-1 gene was downloaded from the National Center for Biotechnology Information (NCBI) GenBank database (NCBI Reference Sequence: NG_050417.1). The primers and probes were manually designed under the principles of RAA primer and probe. Briefly, the primer size was between 30 and 35 bp, the probe size was between 46 and 52 bp and the final product size was between 100 and 200 bp. The specificity of primers and probes was confirmed by NCBI primer-specific BLAST analysis and the hairpins and primer dimers were analyzed by Primer Primier 5. As an internal positive control, the primers and the probe of the 16S rRNA gene were designed in its conserved region. Related primers and probes involved in this study were synthesized by Sangon Biotech (Shanghai, China) and purified by high-performance liquid chromatography.
Analytical Sensitivity and Specificity of the Recombinase-Aided Amplification Assay
The full-length mcr-1 gene was amplified by PCR and cloned into vector pUC57 (Tiangen Biotech Co., Ltd., Beijing, China) by TA cloning, and the recombinant plasmid was called pUC57-mcr-1. The analytical sensitivity of the RAA assay was determined using 10-fold serial dilutions of the recombinant plasmid pUC57-mcr-1 ranging from 107 to 100 copies/μL. The analytical specificity of the RAA assay was evaluated by amplifying the mcr-1 and 16S rRNA genes from 14 different strains, respectively E. colimcr–1, Klebsiella pneumoniae 2146, E. coli ATCC 25922, Pseudomonas aeruginosa ATCC 27853, Shigella sonnei, Salmonella enteritidis, Acinetobacter baumannii, K. oxytoca, Enterobacter aerogenes, Proteus mirabilis, Enterobacter cloacae, Serratia marcescens, Campylobacter jejuni, and Citrobacter freundii. The E. colimcr–1 was used as a positive control and sterile water was used as a negative control.
Recombinase-Aided Amplification Assay
A commercial RAA kit (Jiangsu Qitian Bio-Tech Co., Ltd., China) was used for the RAA assays. The RAA assays were performed as described previously (Feng et al., 2021). Briefly, a 50 μL reaction mixture was prepared first, which was made of reaction buffer (25 μL), DNase-free water (15.7 μL), 10 μM primer F (2.1 μL), 10 μM primer R (2.1 μL), DNA template (2 μL), 280 mM magnesium acetate (2.5 μL), and 10 μM probe (0.6 μL). Then, the reaction mixture was added to a tube with the lyophilized form of RAA enzyme mix and the tube was mixed briefly and incubated for 4 min in a B6100 Oscillation mixer (QT-RAA-B6100; Jiangsu Qitian Bio-Tech Co., Ltd., China). Finally, a fluorescence detector (QT-RAA-1620; Jiangsu Qitian Bio-Tech Co., Ltd.) was used to measure the fluorescence for 20 min at 39°C.
Standard Polymerase Chain Reaction Assay
To detect mcr-1 gene, PCR was performed in a 20 μL reaction mix containing the following: 10 μL of PCR Master Mix reagent (Tiangen Biotech Co., Ltd., Beijing, China), 1 μL of 10 μM mcr-1-F primer (5′-CGTTCAGCAGTCATTATGCCAGTTTCTTTCGCGTGC-3′) and mcr-1-R primer (5′-CTTACGCATATCAGGCTTGGT TGCTTGTACCGC-3′), 1 μL of DNA template and 7 μL of double-distilled water. The PCR cycling conditions were 95°C for 3 min, followed by 35 cycles at 95°C for 30 s, 58°C for 30 s, and 72°C for 1 min. The final extension step was 72°C for 15 min. The PCR products were sent to Sangon Biotech for sequencing.
Multi-Locus Sequence Typing Analysis
All mcr-1-positive E. coli isolates were identified using the VITEK® 2 compact system and screened in accordance with the protocols presented on the MLST website.1 Eight housekeeping genes, namely, dinB (DNA polymerase), icdA (isocitrate dehydrogenase), pabB (p-aminobenzoate synthase), polB (polymerase PolII), putP (proline permease), trpA (tryptophan synthase subunit A), trpB (tryptophan synthase subunit B), and uidA (beta-glucuronidase), were detected.
Antimicrobials Susceptibility Testing
To analyze the characteristics of strains harboring mcr-1 genes, MICs were mainly determined using a VITEK® 2 system (bioMérieux, Nürtingen, Germany). Escherichia coli ATCC25922 was used for quality control. The 2020 Clinical Laboratory Standards Institute’s threshold was used to as reference. In addition, the MICs of colistin and polymyxin B were determined by the twofold serial dilution method, as previously described (Fan et al., 2021). Briefly, the strains harboring mcr-1 genes were grown in LB broth at 37°C until the optical density at 600 nm (OD600) reached 1.0. Next, 100 μL of the bacterial suspension (5 × 105 CFU/mL) and different concentrations of diluted antibiotics were added to each well of a 96-well plate (Corning). The 96-well plate was incubated without agitation at 37°C for 24 h. The minimum antibiotic concentration to visibly inhibit bacterial growth was recorded as the MIC.
Horizontal Gene Transfer Assay
To detect the HGT of mcr-1, colistin-resistant isolates served as donor strains, with sodium azide-resistant E. coli J53 as recipient. Briefly, 500 μL of each donor strain and 500 μL of the recipient in LB broth were mixed, centrifuged at 8,000 rpm, and the bacterial precipitates were resuspended on 50 μL of LB broth. The resuspended bacteria were then added to round filter papers pre-placed on nutritional agar plates and cultured overnight at 37°C. Transconjugant bacteria were selected on LB plates containing sodium azide (100 mg/mL) and colistin (4 μg/mL). Meanwhile, the same number of bacteria were plated on LB plates only containing sodium azide (100 mg/mL). The number of bacterial cells was determined by serial dilution and plating. The transfer efficiency was calculated by dividing the number of successfully transformed strains by the total number of receptor strains.
Statistical Analysis
All trials were conducted three times. The p-values and kappa values of the RAA and standard PCR assays were calculated. The statistical analysis was conducted with SPSS 21.0 (IBM, Armonk, NY, United States).
Results
Primers and Probe Design for the Recombinase-Aided Amplification Assay
Since the first mcr-1 sequence was released in 2015 (NCBI Reference Sequence: NG_050417.1), 31 variants of it have been identified. The genome sequences of all mcr-1 genes are almost identical. The primers and probes for this study were manually designed on the specific and conserved region (Figure 1 and Table 2).
Specificity and Sensitivity Analysis of the Recombinase-Aided Amplification Assay
Fourteen different strains were used as templates to amplify the mcr-1 gene (Table 1). Only from E. colimcr–1 did we succeed in detecting amplification signals, while the others were all negative (Figure 2A). As an internal control, the amplification signals of the 16S rRNA were detected from all bacteria (Figure 2B). Therefore, the RAA assay for the detection of mcr-1 was 100% specific.
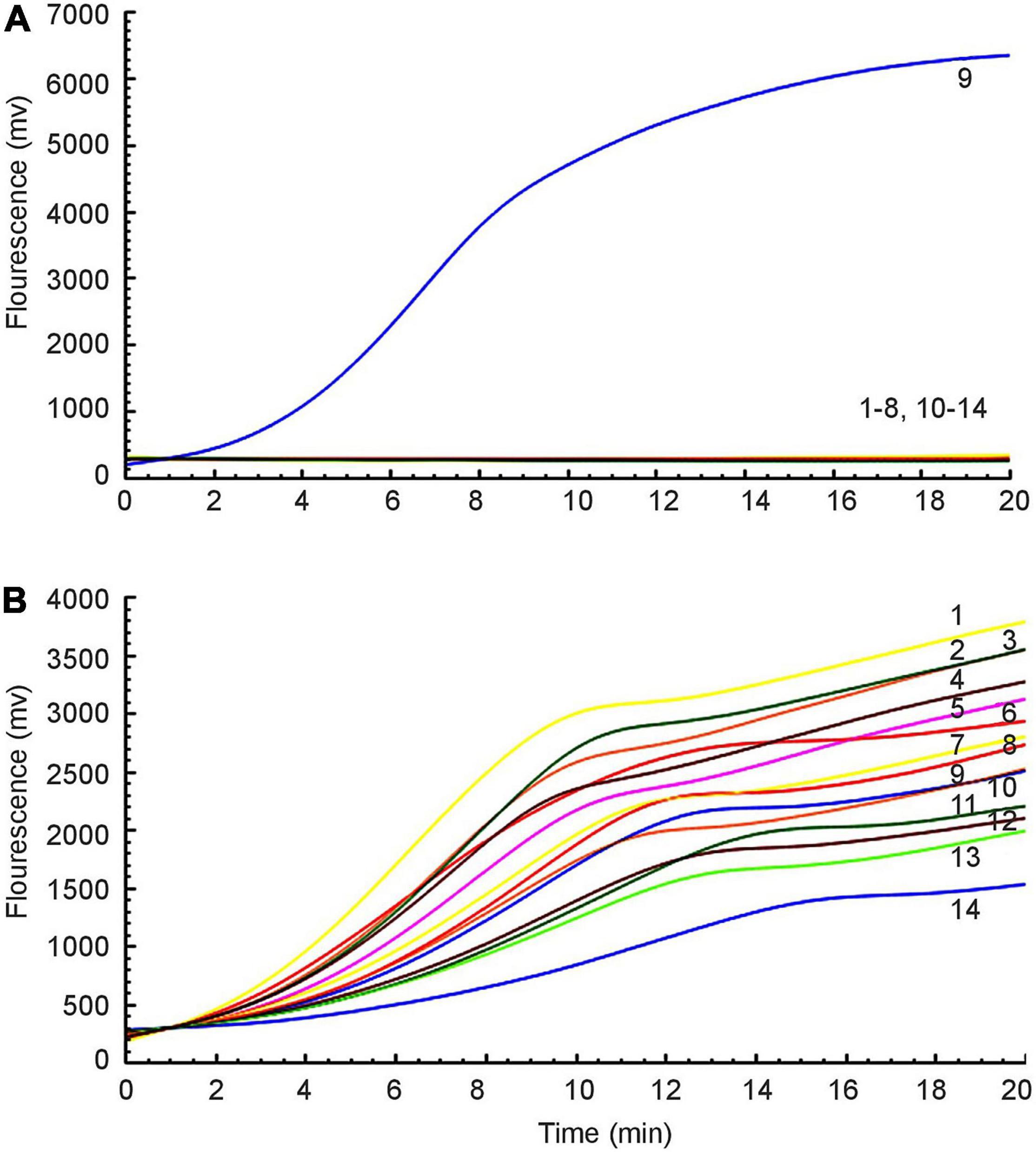
Figure 2. Specificity of the RAA assay. (A) Amplification signal was only amplified from E. colimcr–1, and no relevant signal was detected in other mcr-1-lacking strains. (B) All strains produced 16S rRNA gene amplification signals: 1: P. mirabilis, 2: K. pneumoniae 2146, 3: K. oxytoca, 4: P. aeruginosa, 5: S. sonnei, 6: A. baumannii, 7: C. jejuni, 8: E. aerogenes, 9: E. colimcr–1, 10: S. enteritidis, 11: E. cloacae, 12: C. freundii, 13: P. mirabilis, 14: E. coli ATCC 25922.
Furthermore, a 10-fold gradient dilution series of recombinant plasmids pUC57-mcr-1 was used to detect the sensitivity of the RAA assay. As the copies/μL increased from 1 × 101 to 1 × 107, the fluorescence signal increased (Figure 3). The detection limit of the RAA assay was 20 copies per reaction.
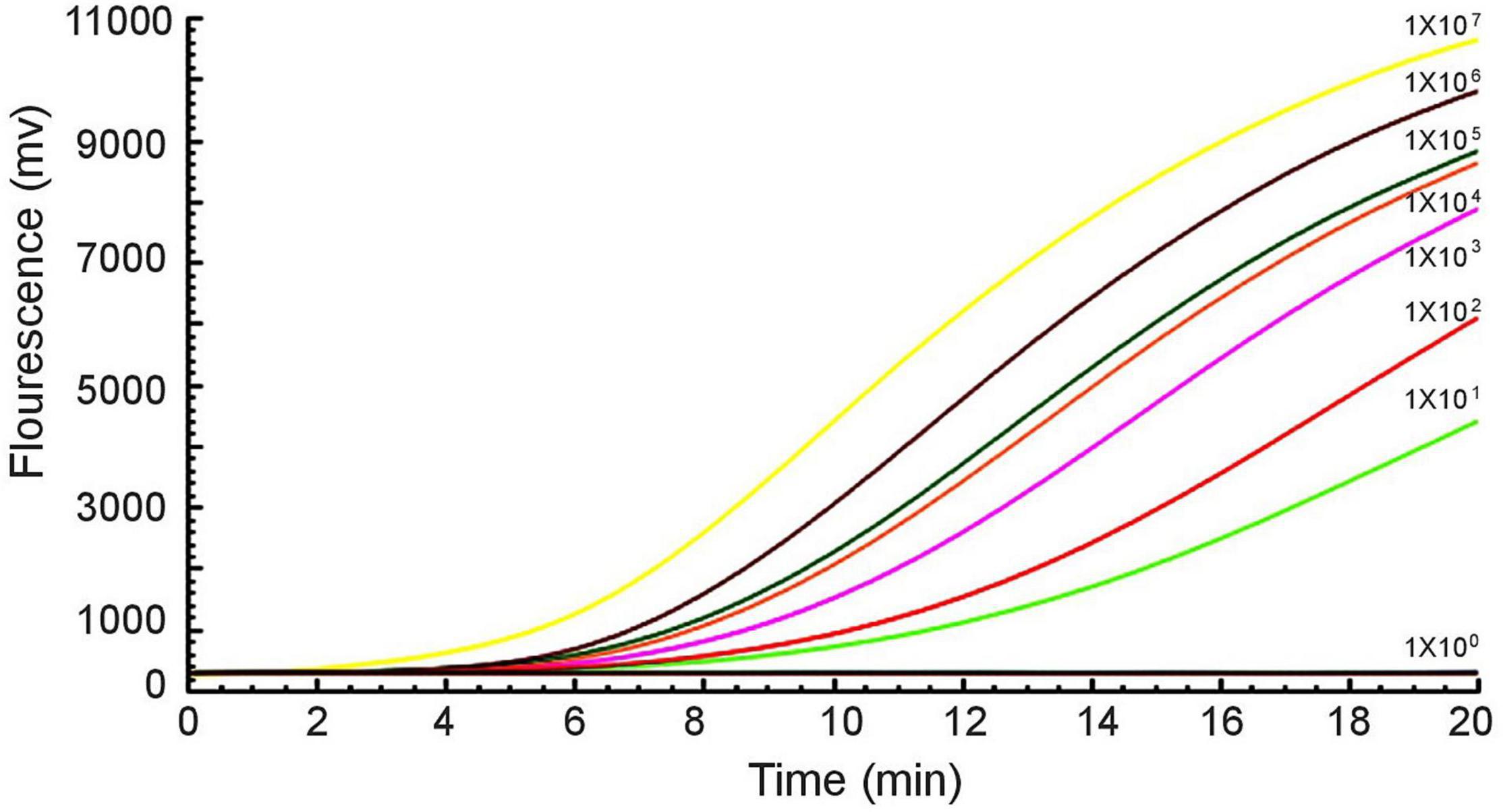
Figure 3. Sensitivity of the RAA assay. An increase in the fluorescence signal was observed from 1 × 101 to 1 × 107 copies/reaction.
Evaluating the Recombinase-Aided Amplification Assay on Clinical Samples
The RAA assay and the standard PCR assay were simultaneously used to detect mcr-1 in 672 samples. Among these, the presence of the mcr-1 gene was found in five samples. The two methods gave the same experimental results (Figure 4 and Table 3).
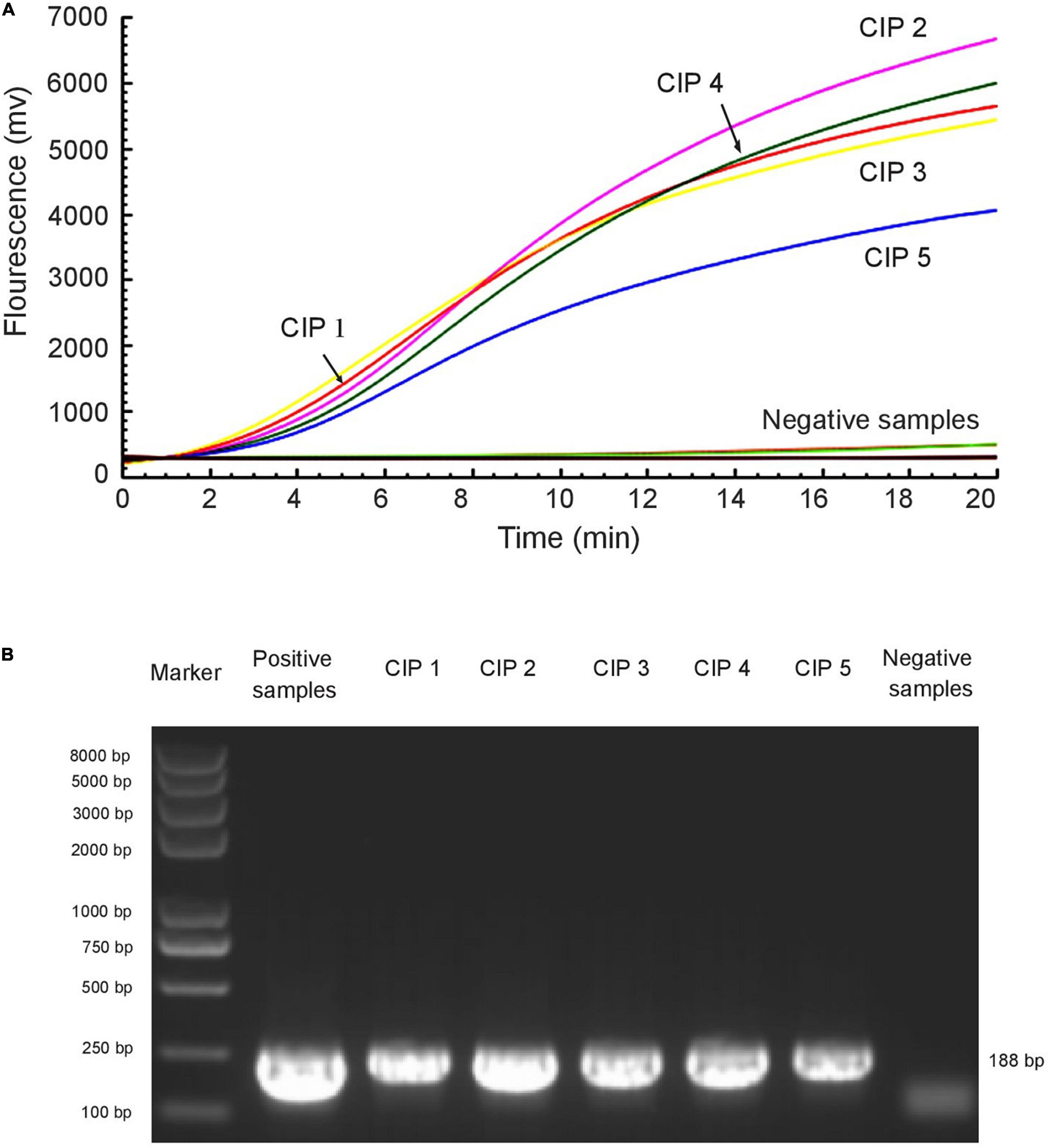
Figure 4. RAA assay was applied to clinical samples. Five mcr-1-positive samples were identified among 672 samples by RAA detection (A) and standard PCR (B). The E. colimcr–1 was used as a positive control and sterile water was used as a negative control.
By screening for colistin resistance, five colistin-resistant E. coli were isolated from five clinical samples. All of these E. coli had the mcr-1 gene, as determined by PCR and sequencing (Table 3). MLST analysis showed that the five E. coli belonged to different ST types: E. coli/mcr-1 1 (ST21), E. coli/mcr-1 2 (ST740), E. coli/mcr-1 3 (ST48), E. coli/mcr-1 5 (ST809), and E. coli/mcr-1 4 (a new ST type).
Susceptibility Test for the Mobile Colistin Resistance-Positive Bacteria
Following testing with the VITEK® 2 compact system, most of the mcr-1-positive E. coli were shown to be resistant to colistin, ciprofloxacin, levofloxacin, doxycycline, and trimethoprim, and sensitive to imipenem, amikacin, and meropenem (Table 4). E. coli/mcr-1 2 was resistant to ticarcillin/clavulanic acid, piperacillin/tazobactam, and cefoperazone, while the other bacteria were sensitive to these antibiotics. The MICs of colistin and polymyxin B were simultaneously determined by the twofold serial dilution method (Table 4).
Transconjugation of Mobile Colistin Resistance
To investigate the transferability of mcr-1, HGT assays were performed using colistin-resistant isolates as donors and sodium azide-resistant E. coli J53 as recipient. mcr-1 from all five colistin-resistant strains was successfully transferred to E. coli J53 recipient strain with some efficiency (Table 3). In contrast to the parental recipient strain, E. coli J53 transconjugants showed varying degrees of resistance to colistin and polymyxin B (Table 4). It was not difficult to find that other resistance did not transfer with the mcr-1 gene transfer (Table 4). Interestingly, the transfer efficiency of mcr-1 from E. coli/mcr-1 4 and E. coli/mcr-1 5 was much higher than that from other isolated E. coli strains, at up to 57–58%. The mechanisms related to this are under investigation.
Discussion
Polymyxins are a class of medications used in the treatment of systemic infections caused by susceptible strains of multidrug-resistant GNB (Shatri and Tadi, 2022). In GNB, modification of lipid A of LPS is the main mechanism of polymyxin resistance and pEtN transferase enzyme encoded by mcr-1 can add a pEtN to the phosphate groups in lipid A (Liu et al., 2016, 2017). mcr-1 has been identified in more than 50 countries across the globe, and detected from many different species of GNB (Nang et al., 2019; Xiaomin et al., 2020). Transmission of mcr-1 has been observed in all kinds of environment including a variety of water resources, raising the possibility that it could be transferred to humans through HGT (Fernandes et al., 2017; Hembach et al., 2017; Sun et al., 2017). Therefore, an efficient, sensitive and reliable method to detect mcr-1 genes is crucial for early diagnosis and infection control in clinical samples.
RAA assay, loop-mediated isothermal amplification (LAMP), and nucleic acid sequence-based amplification (NASBA) are all isothermal amplification techniques, among which RAA assay is the cheapest. As an experimental method with high sensitivity and specificity, RAA assay takes only 20 min to obtain results, while LAMP and real-time PCR take 1–2 h (Du et al., 2021). In addition, RAA assay has been successfully used in identifying SARS-CoV-2, blaNDM, blaKPC, respiratory syntactical virus, hepatitis B virus, salmonella, and other pathogens (Zhang et al., 2017; Fan et al., 2019; Qi et al., 2019; Shen et al., 2019; Xue et al., 2020a,b). In this study, we developed an RAA assay to detect mcr-1 in clinical specimens from children. Among 672 samples, only five of them were mcr-1-positive, and the results from RAA assay and standard PCR were identical, indicating that RAA assay is efficient and accurate for detecting the mcr-1 gene in clinical samples. The detection rate was close to those in other studies among children (Hu et al., 2017; Wu et al., 2021). Human fecal carriage of mcr-1-positive E. coli has been detected in many regions (Chan et al., 2018; Zhong et al., 2018); in this study, all mcr-1-positive isolated strains were identified as E. coli. Five distinct STs detected by MLST showed that mcr-1-positive E. coli isolates from different origins have high clonal diversity.
HGT of mcr-1 is a threat to human health, so it is necessary to calculate the efficiency of mcr-1 transfer. The reported transfer efficiency of mcr-1 varies greatly depending on the experimental conditions, methods, and strains, ranging from 10–9 to 10–1 (Anjum et al., 2016; Liu et al., 2016; Quesada et al., 2016; Gutiérrez et al., 2019; Lu et al., 2019; Li et al., 2022). In our study, mcr-1 from all five colistin-resistant strains was successfully transferred into the E. coli J53 recipient strain by conjugation. Interestingly, the transfer efficiency of mcr-1 from E. coli/mcr-1 4 and E. coli/mcr-1 5 was as high as 57–58%, which is quite high among reported rates. Whether there is a new plasmid that mediates gene transfer will be further studied in the future.
In conclusion, we constructed an RAA assay for mcr-1, screened many clinical samples from a pediatric hospital in Beijing, and confirmed the sensitivity, specificity and effectiveness of our method, which will greatly contribute to clinical diagnosis.
Data Availability Statement
The original contributions presented in the study are included in the article/supplementary material, further inquiries can be directed to the corresponding author/s.
Ethics Statement
The present project was performed in compliance with the Helsinki Declaration (Ethical Principles for Medical Research Involving Human Subjects) and was approved by the research board of the Ethics Committee of the Capital Institute of Pediatrics, Beijing, China (SHERLLM2022004). All specimens used in this study are part of routine patient management without any additional collection, and all patient data were anonymously reported. Based on the guidelines of the Ethics Committee of the Capital Institute of Pediatrics, no consent was needed in this study.
Author Contributions
JY and YF designed the study. ZF, YF, CY, NL, RZ, and SL performed the experiments. WX, GX, HZ, and YF collected the clinical samples. ZF, SD, CY, JC, LG, TF, and JF analyzed the results. ZF, YF, and GX wrote the manuscript. JY and GX revised the manuscript. All authors read and approved the final manuscript.
Funding
This work was supported by grants from the National Natural Science Foundation for Key Programs of China Grant (82130065), the National Natural Science Foundation of China (82002191 and 32170201), FENG Foundation (FFBR 202103), the Beijing Talents Fund (2018000021469G280), the Research Foundation of Capital Institute of Pediatrics (PY-2019-06 and CXYJ-2021-04), and Public Service Development and Reform Pilot Project of the Beijing Medical Research Institute (BMR2019-11).
Conflict of Interest
The authors declare that the research was conducted in the absence of any commercial or financial relationships that could be construed as a potential conflict of interest.
Publisher’s Note
All claims expressed in this article are solely those of the authors and do not necessarily represent those of their affiliated organizations, or those of the publisher, the editors and the reviewers. Any product that may be evaluated in this article, or claim that may be made by its manufacturer, is not guaranteed or endorsed by the publisher.
Acknowledgments
We thank Liwen Bianji (Edanz) (www.liwenbianji.cn) for editing the language of a draft of this manuscript.
Footnotes
References
Anjum, M. F., Duggett, N. A., AbuOun, M., Randall, L., Nunez-Garcia, J., Ellis, R. J., et al. (2016). Colistin resistance in Salmonella and Escherichia coli isolates from a pig farm in Great Britain. J. Antimicrob. Chemother. 71, 2306–2313. doi: 10.1093/jac/dkw149
Baron, S., Hadjadj, L., Rolain, J. M., and Olaitan, A. O. (2016). Molecular mechanisms of polymyxin resistance: knowns and unknowns. Int. J. Antimicrob. Agents 48, 583–591. doi: 10.1016/j.ijantimicag.2016.06.023
Chan, W. S., Au, C. H., Ho, D. N., Chan, T. L., Ma, E. S., and Tang, B. S. (2018). Prospective study on human fecal carriage of Enterobacteriaceae possessing mcr-1 and mcr-2 genes in a regional hospital in Hong Kong. BMC Infect. Dis. 18:81. doi: 10.1186/s12879-018-2987-y
Du, S., Yan, C., Du, B., Zhao, H., Xue, G., Zheng, P., et al. (2021). Development of loop-mediated isothermal amplification assay targeting lytA and psaA genes for rapid and visual diagnosis of streptococcus pneumoniae pneumonia in children. Front. Microbiol. 12:816997. doi: 10.3389/fmicb.2021.816997
Elizabeth, R., Wangkheimayum, J., Singha, K. M., Chanda, D. D., and Bhattacharjee, A. (2021). Subinhibitory concentration stress of colistin enhanced PhoPQ expression in Escherichia coli harboring mcr-1. J. Basic Microbiol. 61, 1029–1034. doi: 10.1002/jobm.202100355
Fan, G. H., Shen, X. X., Li, F., Li, X. N., Bai, X. D., Zhang, R. Q., et al. (2019). Development of an internally controlled reverse transcription recombinase-aided amplification assay for the rapid and visual detection of West Nile virus. Biomed. Environ. Sci. 32, 926–929. doi: 10.3967/bes2019.116
Fan, Z., Pan, X., Wang, D., Chen, R., Fu, T., Yang, B., et al. (2021). Pseudomonas aeruginosa polynucleotide phosphorylase controls tolerance to aminoglycoside antibiotics by regulating the MexXY multidrug efflux pump. Antimicrob. Agents Chemother. 65, e1846–e1820. doi: 10.1128/aac.01846-20
Feng, Y., Xue, G., Feng, J., Yan, C., Cui, J., Gan, L., et al. (2021). Rapid detection of New Delhi Metallo-β-Lactamase gene using recombinase-aided amplification directly on clinical samples from children. Front. Microbiol. 12:691289. doi: 10.3389/fmicb.2021.691289
Fernandes, M. R., Sellera, F. P., Esposito, F., Sabino, C. P., Cerdeira, L., and Lincopan, N. (2017). Colistin-Resistant mcr-1-Positive Escherichia coli on public beaches, an infectious threat emerging in recreational waters. Antimicrob. Agents Chemother. 61, e234–e217. doi: 10.1128/aac.00234-17
Gutiérrez, C., Zenis, J., Legarraga, P., Cabrera-Pardo, J. R., García, P., Bello-Toledo, H., et al. (2019). Genetic analysis of the first mcr-1 positive Escherichia coli isolate collected from an outpatient in Chile. Braz. J. Infect. Dis. 23, 203–206. doi: 10.1016/j.bjid.2019.05.008
Hembach, N., Schmid, F., Alexander, J., Hiller, C., Rogall, E. T., and Schwartz, T. (2017). Occurrence of the mcr-1 colistin resistance gene and other clinically relevant antibiotic resistance genes in microbial populations at different municipal wastewater treatment plants in Germany. Front. Microbiol. 8:1282. doi: 10.3389/fmicb.2017.01282
Hu, Y. Y., Wang, Y. L., Sun, Q. L., Huang, Z. X., Wang, H. Y., Zhang, R., et al. (2017). Colistin resistance gene mcr-1 in gut flora of children. Int. J. Antimicrob. Agents 50, 593–597. doi: 10.1016/j.ijantimicag.2017.06.011
Hussein, N. H., Al-Kadmy, I. M. S., Taha, B. M., and Hussein, J. D. (2021). Mobilized colistin resistance (mcr) genes from 1 to 10: a comprehensive review. Mol. Biol. Rep. 48, 2897–2907. doi: 10.1007/s11033-021-06307-y
Li, J., Nation, R. L., Turnidge, J. D., Milne, R. W., Coulthard, K., Rayner, C. R., et al. (2006). Colistin: the re-emerging antibiotic for multidrug-resistant Gram-negative bacterial infections. Lancet Infect. Dis. 6, 589–601. doi: 10.1016/s1473-3099(06)70580-1
Li, R., Lu, X., Munir, A., Abdullah, S., Liu, Y., Xiao, X., et al. (2022). Widespread prevalence and molecular epidemiology of tet(X4) and mcr-1 harboring Escherichia coli isolated from chickens in Pakistan. Sci. Total Environ. 806(Pt 2):150689. doi: 10.1016/j.scitotenv.2021.150689
Lim, L. M., Ly, N., Anderson, D., Yang, J. C., Macander, L., and Jarkowski, A. 3rd., et al. (2010). Resurgence of colistin: a review of resistance, toxicity, pharmacodynamics, and dosing. Pharmacotherapy 30, 1279–1291. doi: 10.1592/phco.30.12.1279
Liu, Y. Y., Chandler, C. E., Leung, L. M., McElheny, C. L., Mettus, R. T., Shanks, R. M. Q., et al. (2017). Structural modification of lipopolysaccharide conferred by mcr-1 in gram-negative ESKAPE pathogens. Antimicrob. Agents Chemother. 61, e580–e517. doi: 10.1128/aac.00580-17
Liu, Y. Y., Wang, Y., Walsh, T. R., Yi, L. X., Zhang, R., Spencer, J., et al. (2016). Emergence of plasmid-mediated colistin resistance mechanism MCR-1 in animals and human beings in China: a microbiological and molecular biological study. Lancet Infect. Dis. 16, 161–168. doi: 10.1016/s1473-3099(15)00424-7
Lu, X., Zeng, M., Xu, J., Zhou, H., Gu, B., Li, Z., et al. (2019). Epidemiologic and genomic insights on mcr-1-harbouring Salmonella from diarrhoeal outpatients in Shanghai, China, 2006-2016. EBioMedicine 42, 133–144. doi: 10.1016/j.ebiom.2019.03.006
Mentasti, M., David, S., Sands, K., Khan, S., Davies, L., Turner, L., et al. (2021). Rapid detection and differentiation of mobile colistin resistance (mcr-1 to mcr-10) genes by real-time PCR and melt-curve analysis. J. Hosp. Infect. 110, 148–155. doi: 10.1016/j.jhin.2021.01.010
Moffatt, J. H., Harper, M., and Boyce, J. D. (2019). Mechanisms of polymyxin resistance. Adv Exp Med Biol 1145, 55–71. doi: 10.1007/978-3-030-16373-0_5
Nang, S. C., Li, J., and Velkov, T. (2019). The rise and spread of mcr plasmid-mediated polymyxin resistance. Crit. Rev. Microbiol. 45, 131–161. doi: 10.1080/1040841x.2018.1492902
Poole, K., Lau, C. H., Gilmour, C., Hao, Y., and Lam, J. S. (2015). Polymyxin susceptibility in Pseudomonas aeruginosa linked to the MexXY-OprM multidrug efflux system. Antimicrob. Agents Chemother. 59, 7276–7289. doi: 10.1128/aac.01785-15
Qi, J., Li, X., Zhang, Y., Shen, X., Song, G., Pan, J., et al. (2019). Development of a duplex reverse transcription recombinase-aided amplification assay for respiratory syncytial virus incorporating an internal control. Arch. Virol. 164, 1843–1850. doi: 10.1007/s00705-019-04230-z
Quesada, A., Ugarte-Ruiz, M., Iglesias, M. R., Porrero, M. C., Martínez, R., Florez-Cuadrado, D., et al. (2016). Detection of plasmid mediated colistin resistance (MCR-1) in Escherichia coli and Salmonella enterica isolated from poultry and swine in Spain. Res. Vet. Sci. 105, 134–135. doi: 10.1016/j.rvsc.2016.02.003
Rebelo, A. R., Bortolaia, V., Kjeldgaard, J. S., Pedersen, S. K., Leekitcharoenphon, P., Hansen, I. M., et al. (2018). Multiplex PCR for detection of plasmid-mediated colistin resistance determinants, mcr-1, mcr-2, mcr-3, mcr-4 and mcr-5 for surveillance purposes. Euro Surveill 23, 17–00672. doi: 10.2807/1560-7917.Es.2018.23.6.17-00672
Schindler, M., and Osborn, M. J. (1979). Interaction of divalent cations and polymyxin B with lipopolysaccharide. Biochemistry 18, 4425–4430. doi: 10.1021/bi00587a024
Shatri, G., and Tadi, P. (2022). “Polymyxin,” in StatPearls, Treasure Island, FL: StatPearls Publishing Copyright © 2022, StatPearls Publishing LLC).
Shen, X. X., Qiu, F. Z., Shen, L. P., Yan, T. F., Zhao, M. C., Qi, J. J., et al. (2019). A rapid and sensitive recombinase aided amplification assay to detect hepatitis B virus without DNA extraction. BMC Infect. Dis. 19:229. doi: 10.1186/s12879-019-3814-9
Srimal, S., Surolia, N., Balasubramanian, S., and Surolia, A. (1996). Titration calorimetric studies to elucidate the specificity of the interactions of polymyxin B with lipopolysaccharides and lipid A. Biochem. J. 315(Pt 2), 679–686. doi: 10.1042/bj3150679
Sun, P., Bi, Z., Nilsson, M., Zheng, B., Berglund, B., Stålsby Lundborg, C., et al. (2017). Occurrence of bla(KPC-2), bla(CTX-M), and mcr-1 in Enterobacteriaceae from Well Water in Rural China. Antimicrob. Agents Chemother. 61, e2569–e2516. doi: 10.1128/aac.02569-16
Wang, C., Feng, Y., Liu, L., Wei, L., Kang, M., and Zong, Z. (2020). Identification of novel mobile colistin resistance gene mcr-10. Emerg. Microbes Infect. 9, 508–516. doi: 10.1080/22221751.2020.1732231
Wu, P. C., Cheng, M. F., Chen, W. L., Hung, W. Y., Wang, J. L., and Hung, C. H. (2021). Risk factors and prevalence of mcr-1-Positive Escherichia coli in fecal carriages among community children in southern Taiwan. Front. Microbiol. 12:748525. doi: 10.3389/fmicb.2021.748525
Xiaomin, S., Yiming, L., Yuying, Y., Zhangqi, S., Yongning, W., and Shaolin, W. (2020). Global impact of mcr-1-positive Enterobacteriaceae bacteria on “one health”. Crit. Rev. Microbiol. 46, 565–577. doi: 10.1080/1040841x.2020.1812510
Xue, G., Li, S., Zhang, W., Du, B., Cui, J., Yan, C., et al. (2020a). Reverse-transcription recombinase-aided amplification assay for rapid detection of the 2019 novel coronavirus (SARS-CoV-2). Anal. Chem. 92, 9699–9705. doi: 10.1021/acs.analchem.0c01032
Xue, G., Li, S., Zhao, H., Yan, C., Feng, Y., Cui, J., et al. (2020b). Use of a rapid recombinase-aided amplification assay for Mycoplasma pneumoniae detection. BMC Infect. Dis. 20:79. doi: 10.1186/s12879-019-4750-4
Zhang, W., Feng, Y., Zhao, H., Yan, C., Feng, J., Gan, L., et al. (2021). A recombinase aided amplification assay for rapid detection of the Klebsiella pneumoniae carbapenemase gene and its characteristics in Klebsiella pneumoniae. Front. Cell Infect. Microbiol. 11:746325. doi: 10.3389/fcimb.2021.746325
Zhang, X., Guo, L., Ma, R., Cong, L., Wu, Z., Wei, Y., et al. (2017). Rapid detection of salmonella with recombinase aided amplification. J. Microbiol. Methods 139, 202–204. doi: 10.1016/j.mimet.2017.06.011
Zhong, L. L., Phan, H. T. T., Shen, C., Vihta, K. D., Sheppard, A. E., Huang, X., et al. (2018). High rates of human fecal carriage of mcr-1-positive multidrug-resistant enterobacteriaceae emerge in china in association with successful plasmid families. Clin. Infect. Dis. 66, 676–685. doi: 10.1093/cid/cix885
Keywords: RAA assay, mcr-1, E. coli, colistin, children
Citation: Fan Z, Feng Y, Xu W, Feng J, Yan C, Fu T, Zhao H, Cui J, Gan L, Liu S, Du S, Zhang R, Xu Z, Li N, Xue G and Yuan J (2022) Rapid Detection of Multi-Resistance Strains Carrying mcr-1 Gene Using Recombinase-Aided Amplification Directly on Clinical Samples. Front. Microbiol. 13:852488. doi: 10.3389/fmicb.2022.852488
Received: 11 January 2022; Accepted: 25 February 2022;
Published: 31 March 2022.
Edited by:
Rustam Aminov, University of Aberdeen, United KingdomCopyright © 2022 Fan, Feng, Xu, Feng, Yan, Fu, Zhao, Cui, Gan, Liu, Du, Zhang, Xu, Li, Xue and Yuan. This is an open-access article distributed under the terms of the Creative Commons Attribution License (CC BY). The use, distribution or reproduction in other forums is permitted, provided the original author(s) and the copyright owner(s) are credited and that the original publication in this journal is cited, in accordance with accepted academic practice. No use, distribution or reproduction is permitted which does not comply with these terms.
*Correspondence: Guanhua Xue, eGdoNjE4QDE2My5jb20=; Jing Yuan, eXVhbmppbmc2MjE2QDE2My5jb20=
†These authors have contributed equally to this work and share first authorship