- 1Department of Virology, State Key Laboratory of Pathogen and Biosecurity, Beijing Institute of Microbiology and Epidemiology, AMMS, Beijing, China
- 2Department of Nutrition and Food Hygiene, School of Public Health, Cheeloo College of Medicine, Shandong University, Jinan, China
- 3Key Laboratory of Carcinogenesis and Translational Research (Ministry of Education/Beijing), Department of Thoracic Medical Oncology, Peking University Cancer Hospital & Institute, Beijing, China
- 4Key Laboratory for the Prevention and Control of Infectious Diseases, Department of Microbiological Laboratory Technology, School of Public Health, Cheeloo College of Medicine, Shandong University, Jinan, China
- 5Department of Clinical Laboratory, Cheeloo College of Medicine, Shandong University, Jinan, China
Lung cancer is the second most common cancer worldwide and the leading cause of cancer death in the world. Therefore, there is an urgent need to develop new and effective biomarkers for diagnosis and treatment. Under this circumstance, human endogenous retroviruses (HERVs) were recently introduced as novel biomarkers for cancer diagnosis. This study focused on the correlation between lung cancer and HERV-K (HML-2) transcription levels. At the cellular level, different types of lung cancer cells and human normal lung epithelial cells were used to analyze the transcription levels of the HERV-K (HML-2) gag, pol, and env genes by RT–qPCR. At the level of lung cancer patients, blood samples with background information from 734 lung cancer patients and 96 healthy persons were collected to analyze the transcription levels of HERV-K (HML-2) gag, pol, and env genes. The results showed that the transcriptional levels of the HERV-K (HML-2) gag, pol, and env genes in lung cancer cells and lung cancer patient blood samples were significantly higher than those in the healthy controls, which was also verified by RNAScope ISH technology. In addition, we also found that there was a correlation between the abnormal transcription levels of HERV-K (HML-2) genes in lung cancer patients and the clinicopathological parameters of lung cancer. We also identified the distribution locations of the gag, pol, and env primer sequences on each chromosome and analyzed the function of these loci. In conclusion, HERV-K (HML-2) genes may be a potential biomarker for the diagnosis of lung cancer.
Introduction
Lung cancer, a bronchogenic malignancy originating from epithelial tissue (Wadowska et al., 2020), is the second most commonly diagnosed cancer and the leading cause of cancer deaths worldwide (Schwartz and Cote, 2016; Siegel et al., 2020). According to the latest global cancer burden data for 2020 released by the International Agency for Research on Cancer (IARC) of the World Health Organization, in 2020, there were 2.2 million new cases of lung cancer worldwide (1.44 million males and 770,000 females), accounting for 11.4% of new cases of cancer (14.3% of males and 8.4% of females). In the same period, the global number of lung cancer deaths was 1.8 million (1.19 million males and 610,000 females), accounting for 18% of cancer deaths (21.5% of males and 13.7% of females). The morbidity and mortality of males are almost twice those of females (Sung et al., 2021). The number of deaths from lung cancer is expected to increase to 3 million by 2035 (Siegel et al., 2015; Didkowska et al., 2016). Traditionally, lung cancer is divided into non-small cell lung cancer (NSCLC) and small cell lung cancer (SCLC; Howlader et al., 2020). The most common type of NSCLC includes three histological subtypes: adenocarcinoma (~50%), squamous cell carcinoma (~35%), and large cell carcinoma (~15%; Cagle et al., 2013).
The diagnosis of lung cancer is mainly carried out through imaging technology and pathological examination, both of which have limitations in the comprehensive description of lung cancer (Patz et al., 2014; Konopka, 2016), with only 10–15% of new cases being diagnosed at an early clinical stage (Xi et al., 2018). Therefore, in most cases, lung cancer patients are diagnosed with locally advanced or metastatic diseases (Santarpia et al., 2018), and their prognosis is relatively poor, with 5 year survival rates ranging from 4 to 17% depending on stage and regional differences (Hirsch et al., 2017). In addition, some studies have identified biomarkers associated with lung cancer (Seijo et al., 2019) that can be used in disease diagnosis and treatment response, such as carcinoembryonic antigen (CEA; Hammarström, 1999) and cytokeratin fraction 21-1 (CYFRA 21-1; Shirasu et al., 2018). It is mainly used to help and monitor the treatment process. Unfortunately, these two detection methods can only cover a small number of patients with NSCLC. At this stage, we cannot achieve the prevention and early detection of lung cancer through the determination of biomarkers (Cagle et al., 2013). Therefore, in lung cancer, it is necessary to further understand the changes in other genes. Finding high-efficiency lung cancer biomarkers and their clinical application is one of the important means to improve the early diagnosis rate and treatment effectiveness of lung cancer. Some achievements have been made in this field, but the specificity and sensitivity of the verified lung cancer biomarkers still need to be further improved.
Human endogenous retroviruses (HERVs) are novel biomarkers that have recently been introduced for cancer diagnostic purposes (Schmitt et al., 2015; Mareschi et al., 2019; Cao et al., 2020; Curty et al., 2020). HERVs are remnants of retroviruses that infected humans millions of years ago and are integrated into the cellular genome, comprising approximately 8% of the human genome, and are inherited in a Mendelian fashion, with a gene structure consisting of the gag, pro, pol, and env genes (Lander et al., 2001; Balestrieri et al., 2015; Johanning et al., 2017; Gruchot et al., 2019). According to their genetic origin, HERVs can be divided into three main groups: ① ERV-Class I: HERV-W, HERV-R, HERV-E, HERV-H, ERV-9, and HERV-I; ② ERV-Class II: HERV-K; and ③ ERV-Class III: HERV-S and HERV-L (Kim et al., 2008; Escalera-Zamudio and Greenwood, 2016; Vargiu et al., 2016). Through the process of recombination, deletion, and inactivation mutations (Hughes and Coffin, 2001; Lander et al., 2001; Grandi et al., 2017; Grandi, 2018), HERVs are inserted into the human genome in the form of gene fragments, weakening their ability to form infectious virus particles (Blomberg et al., 2009; Dewannieux and Heidmann, 2013), but HERV elements can be transcriptionally active, and many sequences retain open reading frames capable of producing viral proteins (de Parseval et al., 2003; Büscher et al., 2006). HERV-K is the HERV with the most complete viral genetic structure and is capable of producing biologically active retroviral-like particles (Medstrand and Mager, 1998; Buzdin et al., 2003). In recent years, it has been found that abnormal expression of HERV-K proteins and reverse transcription elements may lead to the development of pathological states (Zhou et al., 2016; Lemaitre et al., 2017). HERV-K RNA expression and protein products are widely present in a variety of malignancies (Büscher et al., 2006; Ehlhardt et al., 2006; Fuchs et al., 2011). A study comparing the mRNA expression of HERVs in tumors and adjacent normal tissues showed that HERV-R and H were expressed at high levels in lung tumor tissue compared to normal adjacent tissue (Ahn and Kim, 2009). Another study showed that the blood levels of HERV-R, HERV-H, HERV-K, and HERV-P env mRNA in lung cancer patients were significantly higher than those in healthy controls, and the level of HERV env mRNA in patients with adenocarcinoma was generally higher than that in patients with squamous cell carcinoma and SCLC (Zare et al., 2018).
The above studies have proven that the abnormal expression of HERV-K env is closely related to the occurrence of lung cancer, but there is no related literature on the analysis of HERV-K gag and pol transcription levels in PBMCs of lung cancer patients. In this case, it is necessary to analyze the transcriptional levels of HERV-K genes in lung cancer patients. Therefore, in this study, lung cancer cell lines and control cells, lung cancer patients, and healthy individuals were selected as subjects to analyze the transcriptional levels of HERV-K (HML-2) gag, pol, and env genes based on RT–qPCR technology and to analyze their correlation with the occurrence and development of lung cancer. Our results show that the occurrence of lung cancer is associated with HERV-K (HML-2) gag, pol, and env, indicating that HERV-K (HML-2) genes may be a potential biomarker for the diagnosis of lung cancer.
Materials and Methods
Patients and Samples
Blood samples were collected in tubes containing dipotassium EDTA anticoagulant from 734 lung cancer patients, including 356 adenocarcinomas, 159 squamous cell carcinoma, 17 large cell carcinoma, and 157 small cell lung cancer; the rest were unknown. According to the disease stage, there were 35 cases of I–II and 213 cases of III-IV, and according to the treatment protocol, there were 110 cases without treatment, 414 cases with CTx, and 185 cases with CTx + RTx. Additionally, 96 age- and sex-matched individuals without a history of tumors, autoimmune diseases, or neurological diseases were selected as healthy controls. Written informed consent was obtained from all lung cancer patients and healthy individuals, and the data were analyzed anonymously. All samples were transported to our laboratory through a cold chain and stored at −80°C. The demographic and basic information of the participants is presented in Table 1.
Cell Lines and Cell Culture
Human lung bronchial epithelial BEAS-2B cells, human small cell lung cancer NCI-H446 cells, human adenocarcinoma Calu-3 cells, human squamous cell carcinoma SK-MES-1 cells, and human large cell carcinoma NCI-H460 cells were obtained from Shanghai Chinese Academy of Sciences Cell Bank (Shanghai, China) and Procell (Wuhan, China). The cell lines BEAS-2B, NCI-H446, Calu-3, SK-MES-1, and NCI-H460 were cultured in RPMI 1640, DMEM/F12, or MEM (Gibco, United States) with 10% fetal calf serum (Gibco, United States), 100 U/ml penicillin, and 100 μg/ml streptomycin at 37°C with 5% CO2 and saturated humidity.
RNA Preparation and cDNA Synthesis
Total RNA was extracted using the MiniBEST Plant RNA Extraction Kit (TaKaRa, Cat No. 9769) according to the manufacturer’s protocol. To remove genomic DNA, all RNA samples were treated with 1 μl of gDNA eraser per 1 μg of RNA (TaKaRa, Cat No. RR047A). Then, real-time quantitative PCR was used to detect DNA contamination in all RNA samples, which showed that the CT value of β-actin was >40 in all samples. To further assess whether these gDNA-erased samples had any residual DNase activity or possible inhibition of PCR, we added 103 and 102 DNA standards to the treated samples or pure water controls and compared their CT values. We also performed double gDNA eraser treatments on the low-copy RNA standards, and we found no evidence of RNA copy loss, as there was no change in CT values for successive treatments (Hurst et al., 2016; Karamitros et al., 2016). Then, the mRNA was reverse transcribed into cDNA using a mixed primer pair that contained Oligo dT primer according to the manufacturer’s protocol (TaKaRa, Cat No. RR047A): 37°C for 15 min and 85°C for 5 s, followed by storage at 4°C.
Real-Time Quantitative Polymerase Chain Reaction
Real-time quantitative PCR was performed with a Roche LightCycler 480 II System, 96-well format, using TB Green® Premix Ex Taq™ (TaKaRa, Cat No. RR420A) in a 20 μl reaction with 2 μl cDNA and final concentrations of 0.25 μM forward primer and 0.25 μM reverse primer. All reactions were run using the following protocol: cycling conditions were a 10 min denaturation step at 95°C, followed by 40 cycles of 10 s at 95°C, 5 s at 60°C, and 10 s at 72°C. At the end of the reaction, the amplification curve and melting curve were analyzed. The presence of a single peak in the melting curve analysis was applied to validate the specificity of the PCR amplification. For each amplicon, cDNA samples were measured in triplicate. Primer sequences for amplification of HERV-K (HML-2) gag, pol, and env and β-actin were obtained from a previous study (Li et al., 2021). These sequences were checked by the Primer Blast, Oligocalc, and Gene Runner programs. Primer information is shown in Table 2.
Quantification of HERV-K (HML-2) Transcription in Cells by RNAscope® ISH Technology
RNAscope® (RNAscope® Multiplex Fluorescent Reagent Kit v2, Advanced Cell Diagnostics, United States, Cat No. 323100) was used according to the manufacturer’s protocol and was adapted for the dual detection of mRNA. The probe sets HERV-K (HML-2) env-C1, HERV-K (HML-2) pol-C2, or HERV-K (HML-2) gag-C3 (Advanced Cell Diagnostics, United States) consisted of 20 dual probes targeting different segments within the HERV-K (HML-2) env, HERV-K (HML-2) pol, or HERV-K (HML-2) gag. The standard sample was pretreated according to the manufacturer’s instructions and the previously reported literature (Wang et al., 2012; Li et al., 2021). After the cells were treated, they were inoculated on culture slides. The slide was dipped into 10% neutral buffer formalin (NBF) and fixed at room temperature (RT) for 30 min. Pretreatment for storage was performed by dehydration using 50, 70, and 100% ethanol for 5 min at room temperature and then rehydration at room temperature with 100, 50, and 70% ethanol. The slide was removed from 1× PBS, and a hydrophobic barrier was created by using an Immedge™ Hydrophobic Barrier Pen (Vector Laboratory, United States). The slide was then incubated with hydrogen peroxide and protease (RNAscope® H2O2 and Protease Reagents, Cat No. 322281) at 40°C for 10 and 30 min, respectively. The probe was mixed according to the dose requirement. Then, the mixed target probes were incubated with a HyBez™ oven (Advanced Cell Diagnostics, 110 V, Cat No. 310010) at 40°C for 2 h, and the corresponding probes were added dropwise to the negative (RNAscope® 3-plex Negative Control Probe, Cat No. 320871) and positive (RNAscope® 3-plex Positive Control Probe-Hs, Cat No. 320861) controls. The signal was amplified by incubating one drop each of AMP-1, AMP-2, and AMP-3 at 40°C for 30, 30, and 15 min, respectively, using a HyBez™ oven. Each target nucleic acid was fluorescence-stained with Opal™ 520 (PerkinElmer, Cat No. FP1487001KT), Opal™ 570 (PerkinElmer, Cat No. FP1488001KT), and Opal™ 690 (PerkinElmer, Cat No. FP1497001KT) for 30 min at 40°C and washed twice with wash buffer. Prolong Gold Antifade Mountant (Prolong™, Invitrogen, Cat No. P10144) was used to prevent fluorescence quenching. Then, the coverslips were covered, and a picture was taken as soon as possible. The excitation/emission and pass wavelengths used to detect DAPI, Opal™ 520, Opal™ 570, and Opal™ 690 were set to 340–370/410–470, 460–480/490–530, 510–550/570–590, and 630–650/640–670 nm, respectively. Superresolution images were captured using a confocal microscope (NIKON TI2-E; CRESTOPTICS X-LIGHT V3).
Bioinformatic Analyses
In this study, bioinformatic analyses were performed to predict the chromosome distribution loci and related functions of the HERV-K (HML-2) gag, pol, and env genes. Based on HERV-K (HML-2) gag, pol, and env gene primer sequences, the UCSC genome browser in genome assembly HG381 Blat is used to quickly search the chromosomal coordinates of short nucleotide or protein sequences encoded within the genome. The functional role of these chromosomal loci was then analyzed using the GREAT tool2 and WebGestalt.3
Statistical Analysis
Statistical analysis was performed using GraphPad Prism 8 (GraphPad, CA, United States) and SPSS version 25.0 (SPSS Inc., IL, United States). The Shapiro–Wilk test was applied to test for a normal distribution. Normally distributed numerical variables were expressed as the mean ± standard deviation (SD) taken from at least three independent experiments using an unpaired Student’s t-test. p values <0.05 were considered significant, and *p < 0.05, **p < 0.01, ***p < 0.001, and ****p < 0.0001.
Results
Expression of HERV-K (HML-2) gag, pol, and env in the Lung Cancer Cell Lines NCI-H446, Calu-3, SK-MES-1, and NCI-H460 by RT–qPCR
We analyzed the transcriptional levels of HERV-K (HML-2) gag, pol, and env mRNA in the lung cancer cell lines NCI-H446, Calu-3, SK-MES-1, NCI-H460, and BEAS-2B by RT–qPCR. The transcriptional levels of the HERV-K (HML-2) gag, pol, and env genes in lung cancer were significantly higher than those in the healthy controls (p < 0.0001, Figures 1A–C). Analysis of the expression of HERV-K (HML-2) gag, pol, and env in lung cancers showed that the transcriptional levels of gag, pol, and env mRNA were significantly higher in SCLC, adenocarcinoma, squamous cell carcinoma, and large cell carcinoma than in healthy controls (Figures 1D–F).
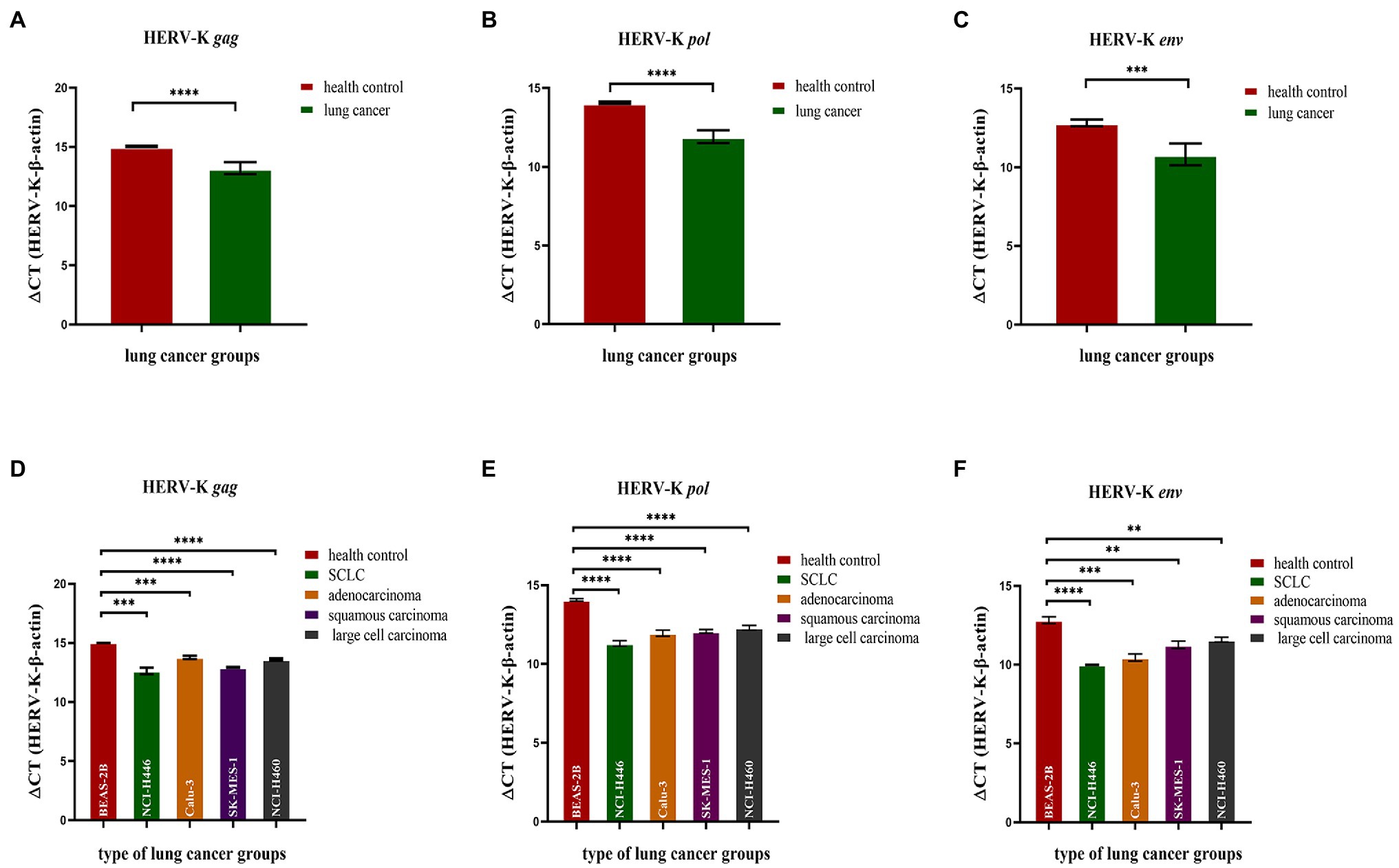
Figure 1. Analysis by real-time quantitative RT–PCR of HERV-K (HML-2) gag, pol, and env gene expression in the different human lung cancer cell lines. The expression of the HERV-K (HML-2) gag (A,D); HERV-K (HML-2) pol (B,E); and HERV-K (HML-2) env (C,F) genes in the lung cancer cell lines NCI-H446, Calu-3, SK-MES-1, NCI-H460, and human bronchial epithelial BEAS-2B cells was analyzed. Real-time quantitative RT–PCR was performed with cell total RNA from the different human lung cancer cell lines, including the healthy control groups, lung cancer groups and lung cancer groups. Real-time quantitative RT-PCRs were coupled to melting-curve analysis to confirm the amplification specificity. Nontemplate controls were included for each primer pair to check for any significant levels of contaminants. The relative expression levels of the HERV-K (HML-2) gag, pol, and env genes were normalized to the expression level of the β-actin gene. Experiments were repeated three times to ascertain the reproducibility of the results. The Y-axis is ΔCT = CTHERV−K−CTβ−actin. *p < 0.05, **p < 0.01, ***p < 0.001, ****p < 0.0001.
Expression of HERV-K (HML-2) gag, pol, and env in Lung Cancer Cell Lines Was Assessed by the RNAscope® ISH Technique
We used NCI-H446, Calu-3, SK-MES-1, NCI-H460, and BEAS-2B cells to show the in situ HERV-K (HML-2) gag, pol, and env RNA expression sites and relative abundance using RNAScope® ISH technology. Sections were made when the cell density was 80%. At the same time, positive control and negative control sections were prepared. Compared with the healthy controls, the transcriptional levels of HERV-K (HML-2) gag, pol, and env in the lung cancers were significantly increased (Figure 2). The experimental results of RNAScope® are consistent with the results of RT–qPCR at the cellular level.
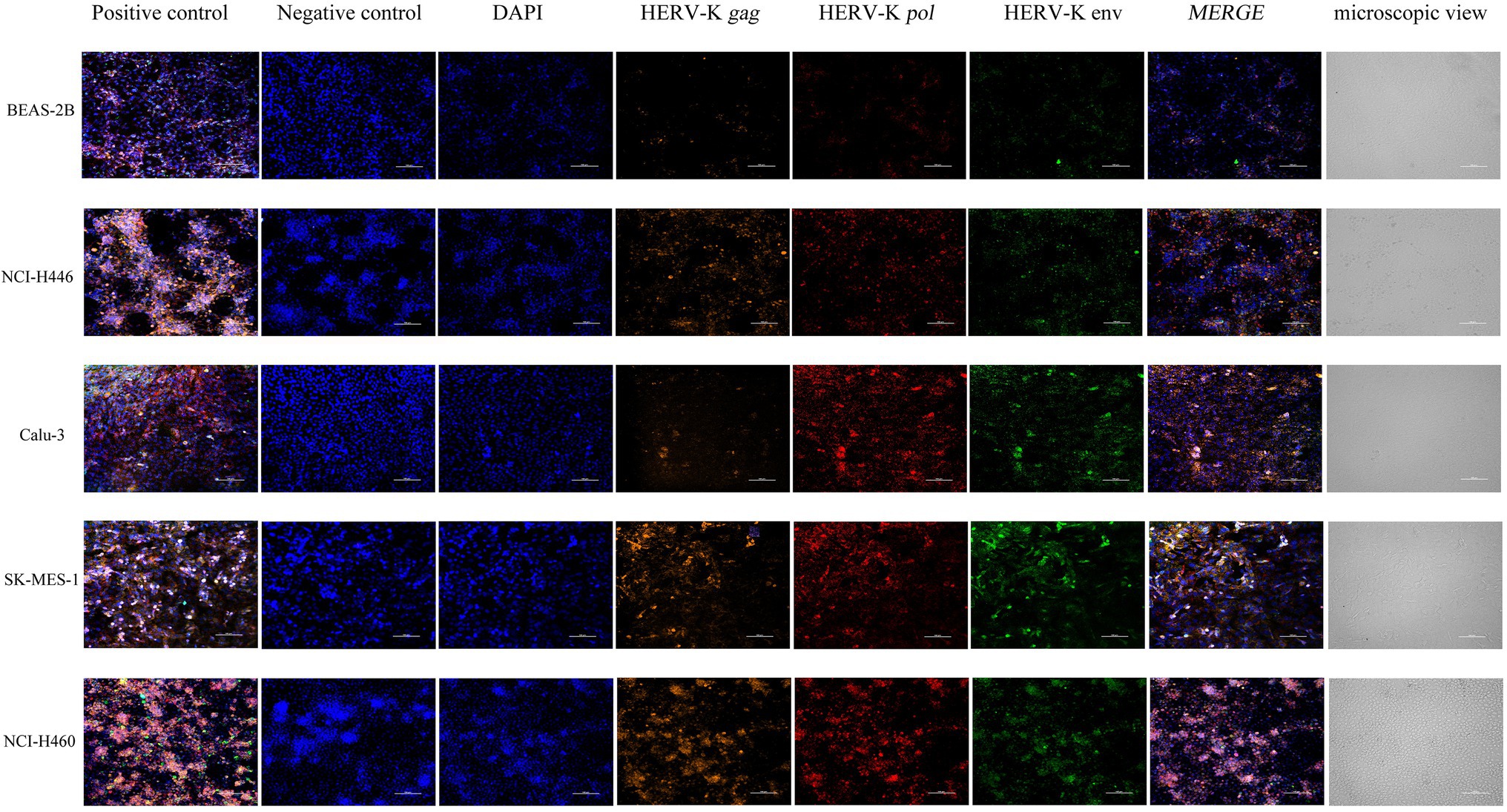
Figure 2. Analysis by RNAscope® ISH technology of HERV-K (HML-2) gag, pol, and env gene expression in the different human lung cancer cell lines. DAPI was used to stain and label the nuclei, Opal™ 520 fluorescent dye with a special probe was used to stain and label HERV-K (HML-2) env region mRNA (green), Opal™ 570 fluorescent dye with a special probe was used to stain and label HERV-K (HML-2) pol region mRNA (red), and Opal™ 690 fluorescent dye with a special probe was used to stain and label HERV-K (HML-2) gag region mRNA (orange). The positive and negative controls used standard control probes provided in the kit (ACD). Original magnification: 20×; scale bars: 100 μM.
Expression of HERV-K (HML-2) gag, pol, and env in the Blood of Lung Cancer Patients by RT–qPCR
We collected background information and blood samples from 734 lung cancer patients and 96 healthy controls and then analyzed the transcriptional levels of the HERV-K (HML-2) gag, pol, and env genes in different lung cancer patients by RT–qPCR. The transcriptional levels of HERV-K (HML-2) gag, pol, and env in lung cancers were significantly upregulated compared with those in healthy controls (p < 0.0001, p < 0.05, and p < 0.01, respectively, Figures 3A–C). Analysis of HERV-K (HML-2) gag, pol, and env gene transcription in lung cancers showed that the transcriptional levels of gag, pol, and env mRNA were significantly higher in SCLC, adenocarcinoma, squamous cell carcinoma, and large cell carcinoma than in healthy controls (Figures 3D–F).
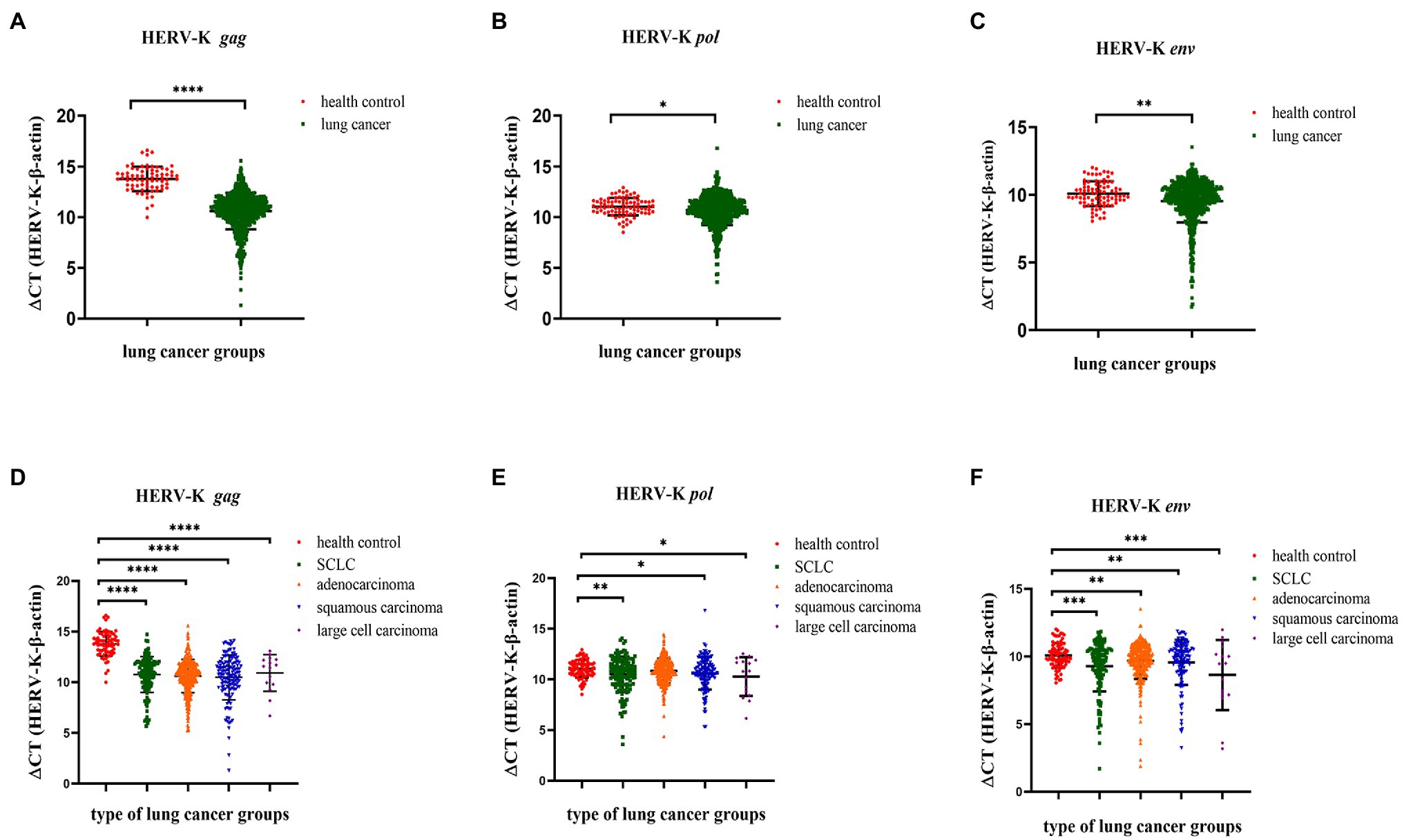
Figure 3. Analysis by real-time quantitative RT–PCR of HERV-K (HML-2) gag, pol, and env gene expression in the different lung cancer patients. The expression of the HERV-K (HML-2) gag (A,D); HERV-K (HML-2) pol (B,E); and HERV-K (HML-2) env (C,F) genes in the different lung cancer patients was analyzed. Real-time quantitative RT-PCRs were performed with blood total RNA of the different lung cancer patients, including healthy control groups, lung cancer groups and lung cancer groups. Real-time quantitative RT-PCRs were coupled to melting-curve analysis to confirm the amplification specificity. Nontemplate controls were included for each primer pair to check for any significant levels of contaminants. The relative expression levels of the HERV-K (HML-2) gag, pol, and env genes were normalized to the expression level of the β-actin gene. Experiments were repeated three times to ascertain the reproducibility of the results. The Y-axis is ΔCT = CTHERV−K−CTβ−actin. *p < 0.05, **p < 0.01, ***p < 0.001, ****p < 0.0001.
Correlation Between HERV-K (HML-2) gag, pol, and env and the Pathologic Features of Lung Cancer
We found a significant correlation between HERV-K (HML-2) and some pathologic data, such as stage and treatment protocol. Our results showed that the transcriptional levels of the HERV-K (HML-2) gag, pol, and env genes in different stages of lung cancer (stage I–II and stage III–IV) were higher than those in healthy controls (Figure 4A). There was also a significant difference in the transcriptional levels of the HERV-K (HML-2) gag gene between I-II and III-IV (p < 0.01). However, there was no significant difference in the transcriptional levels of HERV-K (HML-2) pol, env between I-II and III-IV. We compared treatment naive, chemotherapy included (CTx), and chemotherapy accompanying radiotherapy (CTx + RTx) and found that the transcriptional levels of HERV-K (HML-2) decreased in the chemotherapy groups, while the transcriptional levels of HERV-K (HML-2) in the chemotherapy with radiotherapy groups approached those of healthy controls (Figure 4B). The results suggest that the combination therapy is conducive to reducing the expression of HERV-K (HML-2) genes.
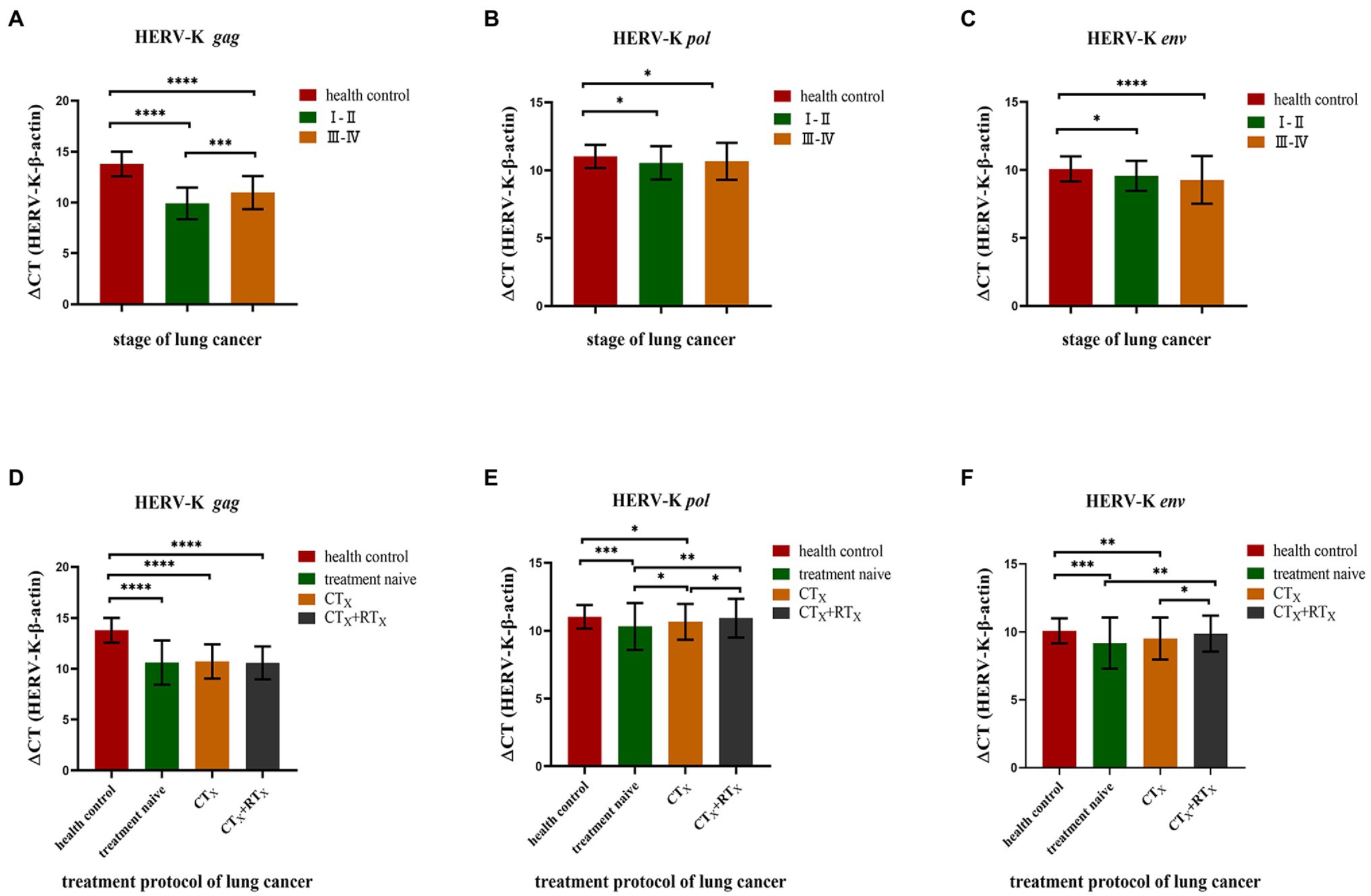
Figure 4. Analysis of HERV-K (HML-2) gag, pol, and env gene expression according to various stages of lung cancer and treatment protocols. (A) HERV-K (HML-2) gag, pol, and env gene transcript levels in the blood of patients with stage I–II and III–IV lung cancer. (B) Effect of chemotherapy and cotreatment with radiotherapy on the reduction of HERV-K (HML-2) gag, pol, and env genes in the blood of lung cancer patients. The expression of the HERV-K (HML-2) gag, pol, and env genes was compared by chemotherapy method, including treatment naive, chemotherapy included (CTx), and chemotherapy accompanying radiotherapy (CTx + RTx) groups. The Y-axis is ΔCT = CTHERV−K−CTβ−actin. *p < 0.05, **p < 0.01, ***p < 0.001, ****p < 0.0001.
GO Annotation and KEGG Pathway Enrichment Analyses
In this study, bioinformatic analyses were performed to predict the chromosome distribution loci. Based on HERV-K (HML-2) gag, pol, and env gene primer sequences, the UCSC genome browser in genome assembly HG38 Blat was used to quickly search the chromosomal coordinates (Figure 5A). To obtain deeper insight into the biological roles of these loci, GREAT and WebGestalt were employed to conduct Gene Ontology (GO) annotation and Kyoto Encyclopedia of Genes and Genomes (KEGG) pathway enrichment analyses. The top 10 enriched GO terms and KEGG pathways are shown in this study. Regarding HERV-K gag, GO biological process (BP) analysis revealed that these loci were markedly enriched in the developmental process. For GO cellular component (CC) analysis, the top significantly enriched term was membrane. The top significantly enriched molecular function (MF) term was protein binding. In addition, the top markedly enriched pathway for these gag loci was the activated neurotrophic receptor tyrosine kinase 3 (NTRK3) signal (Figure 5B). It is worth noting that the GO analysis of pol and env was similar. GO BP analysis revealed that the pol and env loci were markedly enriched in biological regulation. For GO CC analysis, the top significantly enriched term was membrane. The top significantly enriched MF term was protein binding. In addition, the top markedly enriched pathway for these pol loci was glucuronidation. The top markedly enriched pathway for these env loci was nerve growth factor (NGF)-independent tyrosine kinase (TRKA) (Figures 5C,D). The genes used for GO and KEGG analysis are listed in Supplementary Table S1 and the associations between each HERV-K (HML-2) and the genes it putatively regulates are shown in Supplementary Figure S1.
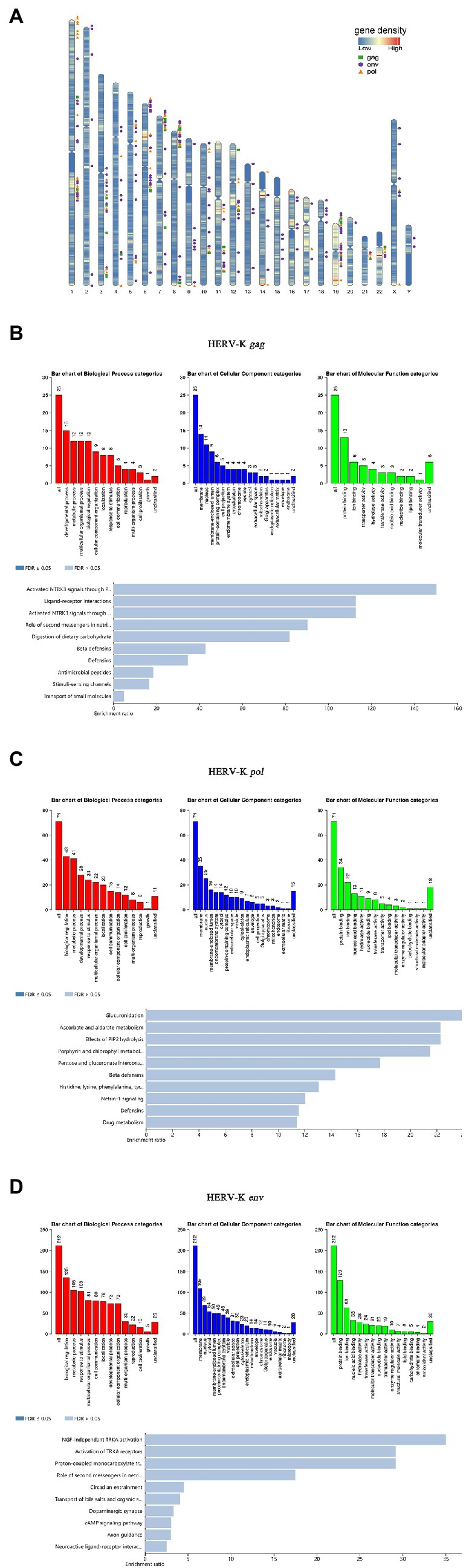
Figure 5. Chromosomal distribution of HERV-K (HML-2) loci and GO analyses. (A) HERV-K (HML-2) gene loci have been visualized on the human karyotype; the green square is HERV-K (HML-2) gag, the orange triangle is HERV-K (HML-2) pol, and the purple circle is HERV-K (HML-2) env. (B-D) GO annotation and KEGG pathway enrichment analyses. All host genes with transcript expression profiles correlated with HERV-K (HML-2) gag, pol, and env abundance were analyzed using the GREAT and WebGestalt tools for enriched GO terms. Each biological process, cellular component and molecular function category is represented by a red, blue, and green bar, respectively. The height of the bar represents the number of IDs in the user list and in the category. Using WebGestalt bioinformatics resources, the predicted target genes were classified according to KEGG functional annotations to determine the regulation pathway of HERV-K (HML-2) gag, pol, and env transcripts.
Discussion
Lung cancer has the characteristics of concealed pathogenesis, rapid progression, poor prognosis, and high mortality, which lead to tumor invasiveness, frequent intrapulmonary spread and extrapulmonary metastasis, and frequent postoperative recurrence. Due to the lack of effective early clinical diagnosis, only a small number of lung cancer patients can undergo potential radical resection, which can improve the 5 year survival rate. Therefore, to improve the diagnostic accuracy and therapeutic effect of lung cancer, it is essential to explore new and effective tumor biomarkers and their functional mechanism. In this study, we found that the transcription levels of HERV-K (HML-2) gag, pol, and env genes in lung cancer cells and lung cancer patients were significantly higher than those in healthy controls, showing that the occurrence of lung cancer is associated with the HERV-K (HML-2) gag, pol, and env genes, suggesting that HERV-K (HML-2) genes may be a potential biomarker for the diagnosis of lung cancer.
Human endogenous retrovirus genetic elements make up approximately 8% of the human genome and are distributed comprehensively in the human chromosome (Kurth and Bannert, 2010). Originally, they were considered “junk DNA.” Growing evidence has proven that they might be involved in certain physiological and pathological processes (Jeong et al., 2010; Romanish et al., 2010). For example, the Env protein of HERV-W has been confirmed to induce inflammation in patients with multiple sclerosis through the toll-like receptor 4 (TLR4) activation pathway (Perron et al., 2013). In addition, recent studies have shown that HERVs transcriptional activity is significantly associated with cancers (Matteucci et al., 2018). Furthermore, there are HERVs (mainly HERV-K) virus-like particles, transcripts, and proteins in different types of human cancers, as well as their specific antibodies in the sera of patients. Dube et al. (2014) found HERV-K viral particles in teratocarcinoma cell lines and patient plasma, and HERV-K can initiate reverse transcription activity of reverse transcriptase to convert RNA to DNA and infest host cells. Wang-Johanning et al. (2014) showed that high expression of HERV-K (HLM-2) was detected in the serum of patients with early breast cancer, and the level of mRNA was positively correlated with the aggravation of clinical manifestations of breast cancer. Fava et al. (2016) found that high expression of HERVs was also found in peripheral monocytes of patients with T-cell lymphoma, suggesting that it was abnormal in the early stage of the disease. Rycaj et al. (2015) studies showed that the level of HERV-K antibody increased significantly in the blood of patients with ovarian cancer and stimulated the immune response, viral T-cell proliferation, elevated levels of interferon-γ, and increased the activity of antigen-specific viral L cells. HERV activity is strictly regulated in the life cycle of each individual (Balestrieri et al., 2015) and is related to the state of chromatin (van de Lagemaat et al., 2006). Many mechanisms have evolved to silence the activity of HERVs. However, a very small number of HERVs are unstable and still active. Their reproduction and random insertion of cell DNA will lead to genetic changes, resulting in abnormal expression in a variety of diseases (Wildschutte et al., 2016).
Aberrant activation of HERV genes is a promising field of diagnosis and treatment in cancer research. HERV genes or gene products show pathogenic potential in a variety of human diseases, such as autoimmune diseases, neurological diseases, infectious diseases, and cancer (Suntsova et al., 2015). Compared with healthy controls, the increased transcriptional expression of HERV-K in malignant diseases such as colorectal cancer, breast cancer, liver cancer, melanoma, and prostate cancer has been studied. Pérot et al. (2015) specifically detected several gene loci of HERV-K and found that the transcriptional levels of HERV-K can stably reflect the progression of colorectal cancer and help to improve the sensitivity and specificity of clinical detection of colorectal cancer. The detection frequency of HERV-specific transcripts in the brain tissue of ALS patients was higher than that of non-ALS control patients (刘剑凯, 2008). The levels of HERV-K mRNA expression and the titer of anti-HERV-K antibody in the serum of patients with ductal carcinoma in situ or stage I breast cancer were significantly higher than those of normal controls, and those with metastasis were higher than those without metastasis, suggesting that it can be used as a serum biomarker for early breast cancer diagnosis (Wang-Johanning et al., 2014). With a large differential expression profile in hepatoblastoma and very low mRNA levels in liver control samples, HERV-K mRNA expression may be a useful biomarker in hepatoblastoma (Grabski et al., 2021). The mRNA expression level of the HERV-K family gag in peripheral blood mononuclear cells of patients with prostate cancer was significantly higher than that of healthy people, especially in elderly individuals and smokers (Wallace et al., 2014). As mentioned above, although HERV-K has been evaluated and introduced as a potential biomarker for breast cancer and melanoma, there are no systematic studies to evaluate the ability of the HERV-K gene to diagnose lung cancer. To clearly evaluate the correlation between HERV-K and development of lung cancer, this study focused on the correlation between lung cancer and HERV-K (HML-2) gene transcriptional levels. At the cellular level, SCLC, adenocarcinoma, squamous cell carcinoma, large cell lung cancer, and human normal lung epithelial cells were used to analyze the transcriptional levels of the HERV-K (HML-2) gag, pol, and env genes based on RT–qPCR. The transcriptional levels of the HERV-K (HML-2) gag, pol, and env genes in lung cancer cells were significantly higher than those in normal lung cells. This result was also verified by RNAscope® ISH technology. At the level of lung cancer patients, background information and blood samples were collected from 734 lung cancer patients (157 cases of SCLC, 356 cases of adenocarcinoma, 159 cases of squamous cell carcinoma, and 17 cases of large cell lung cancer) and 96 healthy controls. The transcriptional levels of the HERV-K (HML-2) gag, pol, and env genes in different lung cancer patients were analyzed. The transcriptional levels of the HERV-K (HML-2) gag, pol, and env genes in lung cancer patients were significantly higher than those in healthy controls. The reason why the experimental results at the cellular level and the patient population level are not very consistent may be due to individual differences and lymphocyte enrichment. In addition, interestingly, we found that there was a correlation between the abnormal expression levels of HERV-K (HML-2) genes in blood of lung cancer patients and the clinicopathological parameters of lung cancer, such as the disease stage and treatment protocol of lung cancer. The results showed that the transcriptional levels of the HERV-K (HML-2) gag, pol, and env genes in lung cancer patients with different stages (stage I–II and stage III–IV) were higher than those in healthy controls. Compared with untreated lung cancer patients, it was found that the transcriptional levels of HERV-K (HML-2) genes decreased in lung cancer patients treated with chemotherapy and radiotherapy, while the transcriptional levels of HERV-K (HML-2) in chemotherapy with radiotherapy group tended to be close to that of healthy controls, suggesting that combination therapy is more beneficial to reduce the expression of HERV-K (HML-2) genes.
In this study, we revealed HERV-K (HML-2) loci transcribed in lung cancer. The identified HERV-K (HML-2) loci are of immediate relevance if the HERV-K (HML-2) transcript is considered to play a role in lung cancer. Therefore, in this study, bioinformatics methods were used to predict the chromosome distribution loci and related functions of the HERV-K (HML-2) gag, pol, and env genes. In this study, the results of bioinformatics analysis showed that the number of chromosome loci where the HERV-K (HML-2) gag, pol, and env genes were distributed was 29, 71, and 158, respectively, suggesting that HERV-K (HML-2) genes exist widely in the human chromosome genome. In addition, the results of bioinformatics analysis showed that the top markedly enriched pathway for these HERV-K (HML-2) gag loci was the activation of the NTRK3 signal, and the NTRK fusion gene was one of the tumor-driving genes. NTRK gene fusion led to the fusion of NTRK gene family members (NTRK1, NTRK2, and NTRK3) with another unrelated gene. The NTRK fusion protein will be continuously active, triggering a permanent signal cascade that drives the spread and growth of NTRK fusion tumors, including lung cancer (Harada et al., 2021; Konig et al., 2021). Studies have shown that high HERV-K (HML-2) transcription alters cortical layer formation in forebrain organoids, and transcriptional activation leads to hyperactivation of NTRK3 expression and other neurodegeneration-related genes (Padmanabhan Nair et al., 2021). The top markedly enriched pathway for these HERV-K (HML-2) pol loci was glucuronidation, and recent studies have shown that glucuronidation increases in malignant tumors (Landmann et al., 2014; Zahreddine et al., 2014). The top markedly enriched pathway for these HERV-K (HML-2) env loci was NGF-independent TRKA activation. Tyrosine kinase (TRKA) is the functional receptor of nerve growth factor (NGF), which has been shown to be associated with tumorigenesis, proliferation, angiogenesis, and metastasis. NGF and its receptors are expressed in lung cancer, and this autocrine stimulus plays an important role in the development of malignant tumors and is associated with low tumor survival rates (Prakash et al., 2010). Kim and other studies have shown that TRKA inhibitors have a significant inhibitory effect on the proliferation of human lung cancer cells but have a low inhibitory effect on normal MRC5 cells (Yan et al., 2021). In summary, it is very important to identify specific HERV-K sites related to specific diseases, especially HERV-K polymorphic sites, which may affect the expression profile of the virus in different individuals and the regulation of host genes. HERV-K (HML-2) is closely related to the occurrence and development of lung cancer, so it is necessary to study the loci of HERV-K (HML-2) and its functional mechanism in the progression of lung cancer.
We confirmed that the transcriptional levels of HERV-K (HML-2) were significantly upregulated in lung cancer patients compared with healthy controls and provided insights into the relationship with the clinical characteristics of lung cancer patients. However, our study still has many limitations. First, we only analyzed the transcriptional levels of HERV-K (HML-2) genes in lung cancer patients, but unfortunately, we do not know which loci play a major role. From our research, we can prove that the occurrence of lung cancer is closely related to HERV-K (HML-2) gag, pol, and env, so we consider that HERV-K (HML-2) is a potential biomarker, rather than just identifying it as such. Second, the expression changes of non-HERV genes have not been studied, and it is not possible to link the data to the expression changes that occur in these cancers, which is also a limitation of our study. High-throughput sequencing technology is widely used to find candidate genes for diseases, which can better and more deeply study the interaction between lung cancer and HERV-K (HML-2). Next, we plan to identify specific HERV-K (HML-2) loci with altered transcript levels in lung cancer patients based on high-throughput sequencing in future studies. We will then conduct systematic functional validation experiments based on the specific loci to clarify the important role of the HERV-K (HML-2) gene in the development of lung cancer, and we will try to collect lung cancer tissue samples and control samples for experiments to validate the results against each other.
The biological function and disease association of HERVs are largely elusive. Obviously, more research is needed to understand the role of HERV-K (HML-2) in human lung cancer and to clarify its role in the pathogenesis of the disease, which may provide new ideas for the further discovery of tumor-associated antigens and the development of new treatments for lung cancer.
Data Availability Statement
The original contributions presented in the study are included in the article/Supplementary Material, further inquiries can be directed to the corresponding authors.
Ethics Statement
The studies involving human participants were reviewed and approved by Beijing Institute of Microbiology and Epidemiology. The patients/participants provided their written informed consent to participate in this study.
Author Contributions
CY, LL, and HL contributed to conception and design of the study. JL, XG, and ZW contributed to sample collection. CY contributed to organizing the database, performing the statistical analysis, and writing the first draft of the manuscript. LJ, JH, and XW contributed to the bibliographic survey. BZ and JH were involved in the critical revision of the article. All authors contributed to the article and approved the submitted version.
Funding
This study was supported by the National Key Research and Development Program of China (2020YFA0907000), the NSFC (81773493, 31800149, and 31900157), and the State Key Laboratory of Pathogen and Biosecurity (AMMS).
Conflict of Interest
The authors declare that the research was conducted in the absence of any commercial or financial relationship that could be construed as a potential conflict of interest.
Publisher’s Note
All claims expressed in this article are solely those of the authors and do not necessarily represent those of their affiliated organizations, or those of the publisher, the editors and the reviewers. Any product that may be evaluated in this article, or claim that may be made by its manufacturer, is not guaranteed or endorsed by the publisher.
Acknowledgments
The authors thank all of the participants and peer workers.
Supplementary Material
The Supplementary Material for this article can be found online at: https://www.frontiersin.org/articles/10.3389/fmicb.2022.850444/full#supplementary-material
Footnotes
References
Ahn, K., and Kim, H. S. (2009). Structural and quantitative expression analyses of HERV gene family in human tissues. Mol. Cells 28, 99–103. doi: 10.1007/s10059-009-0107-y
Balestrieri, E., Pica, F., Matteucci, C., Zenobi, R., Sorrentino, R., Argaw-Denboba, A., et al. (2015). Transcriptional activity of human endogenous retroviruses in human peripheral blood mononuclear cells. Biomed. Res. Int. 2015:164529. doi: 10.1155/2015/164529
Blomberg, J., Benachenhou, F., Blikstad, V., Sperber, G., and Mayer, J. (2009). Classification and nomenclature of endogenous retroviral sequences (ERVs): problems and recommendations. Gene 448, 115–123. doi: 10.1016/j.gene.2009.06.007
Büscher, K., Hahn, S., Hofmann, M., Trefzer, U., Ozel, M., Sterry, W., et al. (2006). Expression of the human endogenous retrovirus-K transmembrane envelope, Rec and Np9 proteins in melanomas and melanoma cell lines. Melanoma Res. 16, 223–234. doi: 10.1097/01.cmr.0000215031.07941.ca
Buzdin, A., Ustyugova, S., Khodosevich, K., Mamedov, I., Lebedev, Y., Hunsmann, G., et al. (2003). Human-specific subfamilies of HERV-K (HML-2) long terminal repeats: three master genes were active simultaneously during branching of hominoid lineages☆☆sequence data from this article have been deposited with the DDBJ/EMBL/GenBank data libraries under accession Nos. AY134884-AY134891 and AF532734-AF532738. Genomics 81, 149–156. doi: 10.1016/S0888-7543(02)00027-7
Cagle, P. T., Allen, T. C., and Olsen, R. J. (2013). Lung cancer biomarkers: present status and future developments. Arch. Pathol. Lab. Med. 137, 1191–1198. doi: 10.5858/arpa.2013-0319-CR
Cao, W., Kang, R., Xiang, Y., and Hong, J. (2020). Human endogenous retroviruses in clear cell renal cell carcinoma: biological functions and clinical values. Onco. Targets. Ther. 13, 7877–7885. doi: 10.2147/OTT.S259534
Curty, G., Marston, J. L., de Mulder, R. M., Leal, F. E., Nixon, D. F., and Soares, M. A. (2020). Human endogenous retrovirus K in cancer: a potential biomarker and immunotherapeutic target. Viruses 12:726. doi: 10.3390/v12070726
de Parseval, N., Lazar, V., Casella, J. F., Benit, L., and Heidmann, T. (2003). Survey of human genes of retroviral origin: identification and transcriptome of the genes with coding capacity for complete envelope proteins. J. Virol. 77, 10414–10422. doi: 10.1128/JVI.77.19.10414-10422.2003
Dewannieux, M., and Heidmann, T. (2013). Endogenous retroviruses: acquisition, amplification and taming of genome invaders. Curr. Opin. Virol. 3, 646–656. doi: 10.1016/j.coviro.2013.08.005
Didkowska, J., Wojciechowska, U., Manczuk, M., and Lobaszewski, J. (2016). Lung cancer epidemiology: contemporary and future challenges worldwide. Ann. Transl. Med. 4:150. doi: 10.21037/atm.2016.03.11
Dube, D., Contreras-Galindo, R., He, S., King, S. R., Gonzalez-Hernandez, M. J., Gitlin, S. D., et al. (2014). Genomic flexibility of human endogenous retrovirus type K. J. Virol. 88, 9673–9682. doi: 10.1128/JVI.01147-14
Ehlhardt, S., Seifert, M., Schneider, J., Ojak, A., Zang, K. D., and Mehraein, Y. (2006). Human endogenous retrovirus HERV-K(HML-2) Rec expression and transcriptional activities in normal and rheumatoid arthritis synovia. J. Rheumatol. 33, 16–23.
Escalera-Zamudio, M., and Greenwood, A. D. (2016). On the classification and evolution of endogenous retrovirus: human endogenous retroviruses may not be ‘human’ after all. APMIS 124, 44–51. doi: 10.1111/apm.12489
Fava, P., Bergallo, M., Astrua, C., Brizio, M., Galliano, I., Montanari, P., et al. (2016). Human endogenous retrovirus expression in primary cutaneous T-cell lymphomas. Dermatology 232, 38–43. doi: 10.1159/000438669
Fuchs, N. V., Kraft, M., Tondera, C., Hanschmann, K. M., Lower, J., and Lower, R. (2011). Expression of the human endogenous retrovirus (HERV) group HML-2/HERV-K does not depend on canonical promoter elements but is regulated by transcription factors Sp1 and Sp3. J. Virol. 85, 3436–3448. doi: 10.1128/JVI.02539-10
Grabski, D. F., Ratan, A., Gray, L. R., Bekiranov, S., Rekosh, D., Hammarskjold, M.-L., et al. (2021). Upregulation of human endogenous retrovirus-K (HML-2) mRNAs in hepatoblastoma: identification of potential new immunotherapeutic targets and biomarkers. J. Pediatr. Surg. 56, 286–292. doi: 10.1016/j.jpedsurg.2020.05.022
Grandi, N. E. T. (2018). Human endogenous retroviruses are ancient acquired elements still shaping innate immune responses. Front. Immunol. 9:2039. doi: 10.3389/fimmu.2018.02039
Grandi, N., Cadeddu, M., Pisano, M. P., Esposito, F., Blomberg, J., and Tramontano, E. (2017). Identification of a novel HERV-K(HML10): comprehensive characterization and comparative analysis in non-human primates provide insights about HML10 proviruses structure and diffusion. Mob. DNA 8:15. doi: 10.1186/s13100-017-0099-7
Gruchot, J., Kremer, D., and Kury, P. (2019). Neural cell responses upon exposure to human endogenous retroviruses. Front. Genet. 10:655. doi: 10.3389/fgene.2019.00655
Hammarström, S. (1999). The carcinoembryonic antigen (CEA) family structures, suggested functions and expression in normal and malignant tissues. Semin. Cancer Biol. 9, 67–81. doi: 10.1006/scbi.1998.0119
Harada, G., Santini, F. C., Wilhelm, C., and Drilon, A. (2021). NTRK fusions in lung cancer: From biology to therapy. Lung Cancer 161, 108–113. doi: 10.1016/j.lungcan.2021.09.005
Hirsch, F. R., Scagliotti, G. V., Mulshine, J. L., Kwon, R., Curran, W. J., Wu, Y.-L., et al. (2017). Lung cancer: current therapies and new targeted treatments. Lancet 389, 299–311. doi: 10.1016/S0140-6736(16)30958-8
Howlader, N., Forjaz, G., Mooradian, M. J., Meza, R., Kong, C. Y., Cronin, K. A., et al. (2020). The effect of advances in lung-cancer treatment on population mortality. N. Engl. J. Med. 383, 640–649. doi: 10.1056/NEJMoa1916623
Hughes, J. F., and Coffin, J. M. (2001). Evidence for genomic rearrangements mediated by human endogenous retroviruses during primate evolution. Nat. Genet. 29, 487–489. doi: 10.1038/ng775
Hurst, T., Pace, M., Katzourakis, A., Phillips, R., Klenerman, P., Frater, J., et al. (2016). Human endogenous retrovirus (HERV) expression is not induced by treatment with the histone deacetylase (HDAC) inhibitors in cellular models of HIV-1 latency. Retrovirology 13:10. doi: 10.1186/s12977-016-0242-4
Jeong, B. H., Lee, Y. J., Carp, R. I., and Kim, Y. S. (2010). The prevalence of human endogenous retroviruses in cerebrospinal fluids from patients with sporadic Creutzfeldt-Jakob disease. J. Clin. Virol. 47, 136–142. doi: 10.1016/j.jcv.2009.11.016
Johanning, G. L., Malouf, G. G., Zheng, X., Esteva, F. J., Weinstein, J. N., Wang-Johanning, F., et al. (2017). Expression of human endogenous retrovirus-K is strongly associated with the basal-like breast cancer phenotype. Sci. Rep. 7:41960. doi: 10.1038/srep41960
Karamitros, T., Paraskevis, D., Hatzakis, A., Psichogiou, M., Elefsiniotis, I., Hurst, T., et al. (2016). A contaminant-free assessment of endogenous retroviral RNA in human plasma. Sci. Rep. 6:33598. doi: 10.1038/srep33598
Kim, H. S., Ahn, K., and Kim, D. S. (2008). Quantitative expression of the HERV-W env gene in human tissues. Arch. Virol. 153, 1587–1591. doi: 10.1007/s00705-008-0159-x
Konig, D., Savic Prince, S., and Rothschild, S. I. (2021). Targeted therapy in advanced and metastatic non-small cell lung cancer. An update on treatment of the Most important actionable oncogenic driver alterations. Cancers 13:804. doi: 10.3390/cancers13040804
Konopka, K. (2016). Diagnostic pathology of lung cancer. Semin. Respir. Crit. Care Med. 37, 681–688. doi: 10.1055/s-0036-1592172
Kurth, R., and Bannert, N. (2010). Beneficial and detrimental effects of human endogenous retroviruses. Int. J. Cancer 126, 306–314. doi: 10.1002/ijc.24902
Lander, E. S., Linton, L. M., Birren, B., Nusbaum, C., Zody, M. C., Baldwin, J., et al. (2001). Initial sequencing and analysis of the human genome. Nature 409, 860–921. doi: 10.1038/35057062
Landmann, H., Proia, D. A., He, S., Ogawa, L. S., Kramer, F., Beissbarth, T., et al. (2014). UDP glucuronosyltransferase 1A expression levels determine the response of colorectal cancer cells to the heat shock protein 90 inhibitor ganetespib. Cell Death Dis. 5:e1411. doi: 10.1038/cddis.2014.378
Lemaitre, C., Tsang, J., Bireau, C., Heidmann, T., and Dewannieux, M. (2017). A human endogenous retrovirus-derived gene that can contribute to oncogenesis by activating the ERK pathway and inducing migration and invasion. PLoS Pathog. 13:e1006451. doi: 10.1371/journal.ppat.1006451
Li, X., Guo, Y., Li, H., Huang, X., Pei, Z., Wang, X., et al. (2021). Infection by diverse HIV-1 subtypes leads to different elevations in HERV-K transcriptional levels in human T cell lines. Front. Microbiol. 12:662573. doi: 10.3389/fmicb.2021.662573
Mareschi, K., Montanari, P., Rassu, M., Galliano, I., Dapra, V., Adamini, A., et al. (2019). Human endogenous retrovirus-H and K expression in human Mesenchymal stem cells as potential markers of Stemness. Intervirology 62, 9–14. doi: 10.1159/000499185
Matteucci, C., Balestrieri, E., Argaw-Denboba, A., and Sinibaldi-Vallebona, P. (2018). Human endogenous retroviruses role in cancer cell stemness. Semin. Cancer Biol. 53, 17–30. doi: 10.1016/j.semcancer.2018.10.001
Medstrand, P., and Mager, D. L. (1998). Human-specific integrations of the HERV-K endogenous retrovirus family. J. Virol. 72, 9782–9787. doi: 10.1128/JVI.72.12.9782-9787.1998
Padmanabhan Nair, V., Liu, H., Ciceri, G., Jungverdorben, J., Frishman, G., Tchieu, J., et al. (2021). Activation of HERV-K(HML-2) disrupts cortical patterning and neuronal differentiation by increasing NTRK3. Cell Stem Cell 28, 1566.e8–1581.e8. doi: 10.1016/j.stem.2021.04.009
Patz, E. F. Jr., Pinsky, P., Gatsonis, C., Sicks, J. D., Kramer, B. S., Tammemagi, M. C., et al. (2014). Overdiagnosis in low-dose computed tomography screening for lung cancer. JAMA Intern. Med. 174, 269–274. doi: 10.1001/jamainternmed.2013.12738
Pérot, P., Mullins, C. S., Naville, M., Bressan, C., Hühns, M., Gock, M., et al. (2015). Expression of young HERV-H loci in the course of colorectal carcinoma and correlation with molecular subtypes. Oncotarget 6, 40095–40111. doi: 10.18632/oncotarget.5539
Perron, H., Dougier-Reynaud, H. L., Lomparski, C., Popa, I., Firouzi, R., Bertrand, J. B., et al. (2013). Human endogenous retrovirus protein activates innate immunity and promotes experimental allergic encephalomyelitis in mice. PLoS One 8:e80128. doi: 10.1371/journal.pone.0080128
Prakash, Y., Thompson, M. A., Meuchel, L., Pabelick, C. M., Mantilla, C. B., Zaidi, S., et al. (2010). Neurotrophins in lung health and disease. Expert Rev. Respir. Med. 4, 395–411. doi: 10.1586/ers.10.29
Romanish, M. T., Cohen, C. J., and Mager, D. L. (2010). Potential mechanisms of endogenous retroviral-mediated genomic instability in human cancer. Semin. Cancer Biol. 20, 246–253. doi: 10.1016/j.semcancer.2010.05.005
Rycaj, K., Plummer, J. B., Yin, B., Li, M., Garza, J., Radvanyi, L., et al. (2015). Cytotoxicity of human endogenous retrovirus K-specific T cells toward autologous ovarian cancer cells. Clin. Cancer Res. 21, 471–483. doi: 10.1158/1078-0432.CCR-14-0388
Santarpia, M., Liguori, A., D’Aveni, A., Karachaliou, N., Gonzalez-Cao, M., Daffina, M. G., et al. (2018). Liquid biopsy for lung cancer early detection. J. Thorac. Dis. 10, S882–S897. doi: 10.21037/jtd.2018.03.81
Schmitt, K., Heyne, K., Roemer, K., Meese, E., and Mayer, J. (2015). HERV-K(HML-2) rec and np9 transcripts not restricted to disease but present in many normal human tissues. Mob. DNA 6:4. doi: 10.1186/s13100-015-0035-7
Schwartz, A. G., and Cote, M. L. (2016). Epidemiology of lung cancer. Adv. Exp. Med. Biol. 893, 21–41. doi: 10.1007/978-3-319-24223-1_2
Seijo, L. M., Peled, N., Ajona, D., Boeri, M., Field, J. K., Sozzi, G., et al. (2019). Biomarkers in lung cancer screening: achievements, promises, and challenges. J. Thorac. Oncol. 14, 343–357. doi: 10.1016/j.jtho.2018.11.023
Shirasu, H., Ono, A., Omae, K., Nakashima, K., Omori, S., Wakuda, K., et al. (2018). CYFRA 21-1 predicts the efficacy of nivolumab in patients with advanced lung adenocarcinoma. Tumour Biol. 40:1010428318760420. doi: 10.1177/1010428318760420
Siegel, R. L., Miller, K. D., and Jemal, A. (2015). Cancer statistics, 2015. CA Cancer J. Clin. 65, 5–29. doi: 10.3322/caac.21254
Siegel, R. L., Miller, K. D., and Jemal, A. (2020). Cancer statistics, 2020. CA Cancer J. Clin. 70, 7–30. doi: 10.3322/caac.21590
Sung, H., Ferlay, J., Siegel, R. L., Laversanne, M., Soerjomataram, I., Jemal, A., et al. (2021). Global cancer statistics 2020: GLOBOCAN estimates of incidence and mortality worldwide for 36 cancers in 185 countries. CA Cancer J. Clin. 71, 209–249. doi: 10.3322/caac.21660
Suntsova, M., Garazha, A., Ivanova, A., Kaminsky, D., Zhavoronkov, A., and Buzdin, A. (2015). Molecular functions of human endogenous retroviruses in health and disease. Cell. Mol. Life Sci. 72, 3653–3675. doi: 10.1007/s00018-015-1947-6
van de Lagemaat, L. N., Medstrand, P., and Mager, D. L. (2006). Multiple effects govern endogenous retrovirus survival patterns in human gene introns. Genome Biol. 7:R86. doi: 10.1186/gb-2006-7-9-r86
Vargiu, L., Rodriguez-Tomé, P., Sperber, G. O., Cadeddu, M., Grandi, N., Blikstad, V., et al. (2016). Classification and characterization of human endogenous retroviruses; mosaic forms are common. Retrovirology 13:7. doi: 10.1186/s12977-015-0232-y
Wadowska, K., Bil-Lula, I., Trembecki, L., and Sliwinska-Mosson, M. (2020). Genetic markers in lung cancer diagnosis: A review. Int. J. Mol. Sci. 21:4569. doi: 10.3390/ijms21134569
Wallace, T. A., Downey, R. F., Seufert, C. J., Schetter, A., Dorsey, T. H., Johnson, C. A., et al. (2014). Elevated HERV-K mRNA expression in PBMC is associated with a prostate cancer diagnosis particularly in older men and smokers. Carcinogenesis 35, 2074–2083. doi: 10.1093/carcin/bgu114
Wang, F., Flanagan, J., Su, N., Wang, L. C., Bui, S., Nielson, A., et al. (2012). RNAscope: a novel in situ RNA analysis platform for formalin-fixed, paraffin-embedded tissues. J. Mol. Diagn. 14, 22–29. doi: 10.1016/j.jmoldx.2011.08.002
Wang-Johanning, F., Li, M., Esteva, F. J., Hess, K. R., Yin, B., Rycaj, K., et al. (2014). Human endogenous retrovirus type K antibodies and mRNA as serum biomarkers of early-stage breast cancer. Int. J. Cancer 134, 587–595. doi: 10.1002/ijc.28389
Wildschutte, J. H., Williams, Z. H., Montesion, M., Subramanian, R. P., Kidd, J. M., and Coffin, J. M. (2016). Discovery of unfixed endogenous retrovirus insertions in diverse human populations. Proc. Natl. Acad. Sci. U. S. A. 113, E2326–E2334. doi: 10.1073/pnas.1602336113
Xi, K. X., Zhang, X. W., Yu, X. Y., Wang, W. D., Xi, K. X., Chen, Y. Q., et al. (2018). The role of plasma miRNAs in the diagnosis of pulmonary nodules. J. Thorac. Dis. 10, 4032–4041. doi: 10.21037/jtd.2018.06.106
Yan, W., Zhang, L., Lv, F., Moccia, M., Carlomagno, F., Landry, C., et al. (2021). Discovery of pyrazolo-thieno[3,2-d]pyrimidinylamino-phenyl acetamides as type-II pan-tropomyosin receptor kinase (TRK) inhibitors: design, synthesis, and biological evaluation. Eur. J. Med. Chem. 216:113265. doi: 10.1016/j.ejmech.2021.113265
Zahreddine, H. A., Culjkovic-Kraljacic, B., Assouline, S., Gendron, P., Romeo, A. A., Morris, S. J., et al. (2014). The sonic hedgehog factor GLI1 imparts drug resistance through inducible glucuronidation. Nature 511, 90–93. doi: 10.1038/nature13283
Zare, M., Mostafaei, S., Ahmadi, A., Azimzadeh Jamalkandi, S., Abedini, A., Esfahani-Monfared, Z., et al. (2018). Human endogenous retrovirus env genes potential blood biomarkers in lung cancer. Microb. Pathog. 115, 189–193. doi: 10.1016/j.micpath.2017.12.040
Zhou, F., Li, M., Wei, Y., Lin, K., Lu, Y., Shen, J., et al. (2016). Activation of HERV-K Env protein is essential for tumorigenesis and metastasis of breast cancer cells. Oncotarget 7, 84093–84117. doi: 10.18632/oncotarget.11455
Keywords: lung cancer, HERV-K, cell culture, RT–qPCR, in situ hybridization, biomarker, diagnosis
Citation: Yang C, Guo X, Li J, Han J, Jia L, Wen H-L, Sun C, Wang X, Zhang B, Li J, Chi Y, An T, Wang Y, Wang Z, Li H and Li L (2022) Significant Upregulation of HERV-K (HML-2) Transcription Levels in Human Lung Cancer and Cancer Cells. Front. Microbiol. 13:850444. doi: 10.3389/fmicb.2022.850444
Edited by:
Yongxiu Yao, The Pirbright Institute, United KingdomReviewed by:
Shaian Tavakolian, Shahid Beheshti University, IranElif Karlık, University of Istinye, Turkey
Copyright © 2022 Yang, Guo, Li, Han, Jia, Wen, Sun, Wang, Zhang, Li, Chi, An, Wang, Wang, Li and Li. This is an open-access article distributed under the terms of the Creative Commons Attribution License (CC BY). The use, distribution or reproduction in other forums is permitted, provided the original author(s) and the copyright owner(s) are credited and that the original publication in this journal is cited, in accordance with accepted academic practice. No use, distribution or reproduction is permitted which does not comply with these terms.
*Correspondence: Ziping Wang, d2FuZ3pwMjAwN0AxMjYuY29t; Hanping Li, aGFucGluZ2xpbmVAMTYzLmNvbQ==; Lin Li, ZGVhcndvb2RAc2luYS5jb20=
†These authors have contributed equally to this work and share first authorship