- 1Department of Medical Microbiology and Immunology, Faculty of Medicine, Minia University, Minya, Egypt
- 2Department of Pharmacology, Faculty of Medicine, Minia University, Minya, Egypt
- 3Department of Pathology, Faculty of Medicine, Minia University, Minya, Egypt
- 4Internal Medicine Department, Faculty of Medicine, Minia University, Minya, Egypt
- 5Chest Department, Faculty of Medicine, Minia University, Minya, Egypt
There is a persistent need to look for alternative therapeutic modalities to help control the pandemic of antimicrobial resistance. Assessment of antibacterial and anti-biofilm effects of vitamin C (ascorbic acid) was the aim of the current study. The micro-dilution method determined the minimal inhibitory concentration (MIC) of ascorbic acid or antibiotics alone and in combinations against Pseudomonas aeruginosa (P. aeruginosa) clinical isolates. The micro-titer plate method monitored the effect of ascorbic acid on the biofilm-producing isolates of P. aeruginosa. The effect of ascorbic acid on the differential expression of different antibiotic-resistant genes and biofilm encoding genes of P. aeruginosa isolates were also tested using real-time polymerase chain reaction (PCR). For in vivo assessment of the antibacterial effects of ascorbic acid alone or combined with an antibiotic, rats were infected with P. aeruginosa clinical isolate followed by different treatment regimens. MICs of ascorbic acid among P. aeruginosa isolates were in the range of 156.2–1,250 μg/ml, while MIC50 and MIC90 were 312.5 and 625 μg/ml, respectively. At sub-inhibitory concentrations (19.5–312.5 μg/ml), ascorbic acid had 100% biofilm inhibitory effect. Furthermore, ascorbic acid-treated bacteria showed downregulation of genes underpinning biofilm formation and antibiotic resistance. In vivo assessment of vitamin C and ceftazidime in rats showed that administration of both at a lower dose for treatment of pseudomonas infection in rats had a synergistic and more powerful effect. Vitamin C shows excellent in vitro results as an antibacterial and anti-biofilm agent. Vitamin C should be routinely prescribed with antibiotics to treat bacterial infections in the clinical setting.
Introduction
Pseudomonas aeruginosa (P. aeruginosa) is a versatile bacteria characterized by multiple resistance to various antimicrobial agents. It is one of the top causes of opportunistic infections and a primary causative agent of hospital-acquired infections (Stover et al., 2000). The emergence of multi-drug resistant (MDR) P. aeruginosa is a potential public health risk and may compromise effective antibiotic therapy. Biofilm formation is an essential cause of antibiotic resistance among P. aeruginosa clinical isolates. There are a list of factors considered to be responsible for biofilm resistance, which include restricted penetration of antimicrobials into a biofilm barrier, decreased growth rate of biofilm persister cells, and expression of possible biofilm-specific resistance genes (Lewis, 2001). Biofilm formation is leading to another form of resistance, also called tolerance or phenotypical resistance of biofilm embedded cells. Biofilm-forming P. aeruginosa has the ability to convert to an antibiotic-resistant phenotype after antibiotic exposure with enhanced antibiotic tolerance (Drenkard and Ausubel, 2002).
There is considerable concern about the pandemic increase in antibiotic-resistant bacteria leading to treatment failure (Hancock and Speert, 2000). Vitamin C (ascorbic acid) represents one such alternative, and many studies have demonstrated its antibacterial action. It has a strong growth inhibitory effect on Staphylococcus aureus, Enterococcus faecalis (Isela et al., 2013), Helicobacter pylori, Campylobacter jejuni (Zhang et al., 1997), and Mycobacterium tuberculosis (Vilchèze et al., 2013), and even on fungi such as Aspergillus (Gupta and Guha, 1941). At first, it was hypothesized that the antimicrobial effect of vitamin C was due to its pH-lowering effect. However, another study proved the potent antimicrobial properties of vitamin C directed against Streptocccus pyogenes, even in pH-neutral conditions. Also, vitamin C is a powerful antioxidant agent produced against free radicals and reactive oxygen species (Slade and Knox, 1950).
Vitamin C is cheap, available, and has few or no side effects. Vitamin C is frequently prescribed as a nutritional supplement, has known antioxidant effects, and has been used as an adjuvant in cancer chemotherapy. Vitamin C is a gluconic acid lactone derived from glucuronic acid and water-soluble ketolactone with two ionizable hydroxyl groups (Sorice et al., 2014). In nature, there are two isomeric molecules of vitamin C present in equal parts, the reduced form (D-ascorbic acid) and the chemically active and oxidized form (L-ascorbic acid) (Hong et al., 2016), which are mutually interchangeable. The natural supplies of vitamin C are citrus fruits, kiwi, mango, strawberries, papaya, tomatoes, green leafy vegetables, and broccoli (Farris, 2014). This study investigated the effect of ascorbic acid alone and in combination with antibiotics on the growth and biofilm-forming potential of clinical isolates of P. aeruginosa in vitro. We also examined the effect of ascorbic acid alone and in combination with an antibiotic on treating P. aeruginosa infection in rats.
Materials and Methods
Microorganisms
Previously identified P. aeruginosa clinical isolates collected from patients with burn or infected wounds, at the surgery department of Minia University Hospital, were included in this study. The identification of the isolates was done according to morphological characteristics and biochemical reactions using API Test Kits (bioMerieux, France). We selected 50 biofilm-producing P. aeruginosa from all other isolates to perform this study.
Determination of Minimal Inhibitory Concentration of Ascorbic Acid or Antimicrobials Alone and in Combinations
The minimal inhibitory concentration (MIC) of ascorbic acid or antibiotics alone and in combinations against all isolates was determined by micro-dilution method, according to the clinical and laboratory standard institute (CLSI) guidelines [Clinical and Laboratory Standard Institute [CLSI], 2019]. A stock solution of ascorbic acid was prepared by dissolving commercially purchased ascorbic acid (L-ascorbic acid, Sigma) in sterile distilled water (20 mg/ml). Stock solutions of the following antibiotics: piperacillin, piperacillin/tazobactam, ceftazidime, ciprofloxacin, and gentamicin were prepared by dissolving commercially purchased antibiotics in sterile distilled water (1 mg/ml). Müeller-Hinton broth (MHB) (Difco, United States) was used as a basal medium. Serial dilutions of ascorbic acid or antibiotics alone and in combinations were prepared in 100 μl volume of MHB in micro-titer plate wells. A quantity of 10 μl of bacterial suspension of each isolate with 0.5 McFarland turbidity was then inoculated in the wells. Wells containing a basal medium with and without bacterial suspension, but free of antibiotic and ascorbic acid, and wells containing the antibiotic or ascorbic acid were included in each assay as growth control. The plates were incubated for 24 h at 37°C. The lowest concentration of antibiotics separately or combined with ascorbic acid, which prevented the growth, was regarded as the MIC. The MIC results were interpreted according to CLSI guidelines [Clinical and Laboratory Standard Institute [CLSI], 2019].
Determination of Fractional Inhibitory Concentration
Fractional inhibitory concentration (FIC) was used to interpret the MIC results as follows (Mackay et al., 2000): FIC of antibiotic = MIC of the antibiotic combined with ascorbic acid/MIC of antibiotic alone. FIC ≤ 0.5 means syngergism, FIC > 0.5–4 means indifference, and FIC > 4 means antagonism.
Time-Kill Kinetics Assay
Vitamin C was tested to detect the time-kill kinetics. Briefly, grown culture of MHB with pure bacterial colonies (1.0 × 106 CFU/ml) was supplemented with ascorbic acid (625 μg/ml). Serial dilution was performed at each time point (0, 2, 4, 6, 12, and 24 h), and subculture on Muller Hinton agar plates was incubated at 37°C. Positive control test was performed for the tested strains without ascorbic acid. A graph of log CFU versus time was created. Each experiment was performed in triplicates (Appiah et al., 2017).
Effect of Ascorbic Acid on Biofilm Production
The effect of ascorbic acid on the biofilm-producing isolates of P. aeruginosa was assessed by the microtiter plate method according to the instructions by Samet et al., 2013. Initially, 190 μl of bacterial suspension equivalent to 0.5 McFarland in Luria Bertani broth (LB) was inoculated in 96 microtiter plates. Sub-MIC of ascorbic acid was added to each well, excluding the positive and negative control wells. Plates were incubated at 37°C for 24 h. After incubation, the content of the wells were gently removed. The wells were washed with phosphate-buffered saline solution to remove free-unattached bacteria. Biofilms formed by adherent bacteria were air- and heat-fixed at 60°C for 1 h, and then stained with crystal violet (0.1%). Excess stain was rinsed off and the wells were washed with water. Ethanol 95% was added to the wells, and after 15 min, the optical densities (ODs) of the stained bacteria were determined with an ELISA reader (model CS, Biotec) at 590 nm. These OD values were considered an index of bacteria-forming biofilms. Experiments were performed in triplicate, and the data were then averaged.
Effect of Ascorbic Acid on Relative Genes Expression
Pseudomonas aeruginosa isolates were tested to express different antibiotic-resistant genes and biofilm encoding genes before and after treating the isolates with ascorbic acid, using real-time reverse transcriptase-polymerase chain reaction (RT-PCR) according to the steps described below.
RNA Extraction
Isolated bacteria were inoculated in two tubes containing 2 ml LB broth with and without sub-MIC of ascorbic acid. The tubes were incubated at 37°C with shaking at 200 runs per minute (rpm) for 24 h. Bacterial RNA was extracted by the Direct-zol RNA extraction kit (CORP, Australia) according to the kit protocol. Absorbance was measured by a spectrophotometer (Genova, United States), and the ratio of absorbance at 260 nm and 280 nm was used to evaluate the purity of the extracted RNA. A result within the range of 1.8–2 was considered as acceptable purity. The quality of the extracted RNA was assessed via gel electrophoresis at 100 V for 60 min.
Real-Time Polymerase Chain Reaction
Quantitative RT-PCR was done using one-step SYBR green kits (SensiFAST SYBR Lo-ROX Kit, United Kingdom) in an ABI 7500 instrument (Applied Biosystems, United States). The RT-PCR reaction was prepared with a final volume of 20 μl (master mix: 10 μl, forward primer: 1 μl, reverse primer: 1 μl, reverse transcriptase: 0.2 μl, RNase inhibitor: 0.4 μl, water up to 16 μl, and template: 4 μl). Different genes and primers are listed in Table 1. Negative control samples containing deionized water instead of template, one for each gene, were included in the same PCR run.
We analyzed the PCR results with relative quantification to 16srRNA as a reference gene. We calculated the fold changes of mRNA levels using the comparative cycle threshold (ΔΔCt). ΔCt = mean Ct of gene of interest – mean Ct reference gene. ΔΔCt = ΔCT of the test sample –Δ CT of the control sample. The relative quantity (RQ) = 2–ΔΔCT (Livak and Schmittgen, 2001). The relative expression was then analyzed by using free data analysis tools. PCR products were examined by gel electrophoresis to exclude any unspecific products that may be present.
In vivo Assessment of the Antibacterial Effect of Ascorbic Acid
The experiment was conducted according to the standard practices concerning the ethics and animal procedures of the Institutional Research Ethics Committee.
Rats
Forty male Wistar rats weighing 250–280 g were obtained from National Research Center (Giza, Egypt). The 40 rats were divided into four groups (10 rats each), group 1 (Pneumonia; PN), group 2 (Vitamin C; VIT C), group 3 (Antibiotic; AB), and group 4 (VIT C+AB). The rats in groups 1, 2, 3, and 4 were intraperitoneally injected with P. aeruginosa clinical isolate. Three hours after injection, five rats were culled from group 1 to ensure that pneumonia had been established. Group 1 did not receive any treatment. Treatment options, given by intraperitoneal injection, were started 3 h after inoculation for groups 2, 3, and 4 for 72 h. Group 2 was treated with ascorbic acid (400 mg/kg/day), group 3 was treated with ceftazidime antibiotic (80 mg/kg/day), and group 4 was treated with ceftazidime (20 mg/kg/day) and ascorbic acid (200 mg/kg/day). The dose was calculated according to MIC results and rat pharmacokinetics.
Intraperitoneal P. aeruginosa Inoculation and Sample Collections
For intraperitoneal (i.p) inoculation in rats, the fresh cultured broth was prepared from isolated colonies of P. aeruginosa. Rats were injected with P. aeruginosa in 1,000 μl saline. The actual inoculum was quantitated by plating 10-fold serial dilutions on agar plates on brain-heart infusion (BHI). The clinical appearance of the rats was followed up. At the end of our experiment, the rats were culled. Initially, the rats were anesthetized, and then the peritoneal cavity and trachea were lavaged with 3 ml of phosphate-buffered saline. Blood samples were collected from the abdominal aorta for bacterial culture or serum separation. The lungs were aseptically removed, divided, and used for bacteriological, biochemical, and histological examination.
Bacterial Count
The lungs were aseptically homogenized in sterile saline using tissue homogenizers. A volume of 100 μl of 10-fold serial dilutions of the tissue homogenate, whole blood, bronchoalveolar lavage, and peritoneal lavage fluid were cultured on solid media. The bacterial count was calculated per 1 ml of blood and lavage fluid or per gram of tissue.
Quantification of Oxidative Stress Parameters
Blood was centrifuged at 3,000 rpm for 10 min (T30 centrifuge, Germany). Sera were kept frozen at −80°C for further analysis. The lungs were washed with saline and then divided. Specimens were homogenized in ice-cold phosphate buffer, centrifuged for 15 min at 5,000 rpm, and the supernatant was kept frozen at −80°C for further examination. The index of lipid peroxidation is the malondialdehyde (MDA) level, which was determined following the method of Buege and Aust, 1978. Glutathione (GSH) (Moron et al., 1979) and total antioxidant capacity (TAC) were evaluated using colorimetric kits and following the manufacturer’s guidelines.
Statistical Analyses
Statistical analyses were performed using the Graph Pad Prism, Version 8.0 for Windows (Graph Pad Software, San Diego, CA, United States). Data are presented as means ± standard error of the mean (SEM). The quantitative measurements were assessed by Student’s t-test or one-way ANOVA followed by Turkey’s multiple comparison tests, when appropriate. The difference was considered significant when p-value < 0.05.
Results
In vitro Antibacterial and Anti-biofilm Effect of Ascorbic Acid
Minimal inhibitory concentrations of ascorbic acid among P. aeruginosa isolates were 156–1,250 μg/ml, as presented in Figure 1. The MIC50 and MIC90 of ascorbic acid, which inhibit the growth of 50% and 90% of isolates, were 312.5 and 625 μg/ml, respectively.
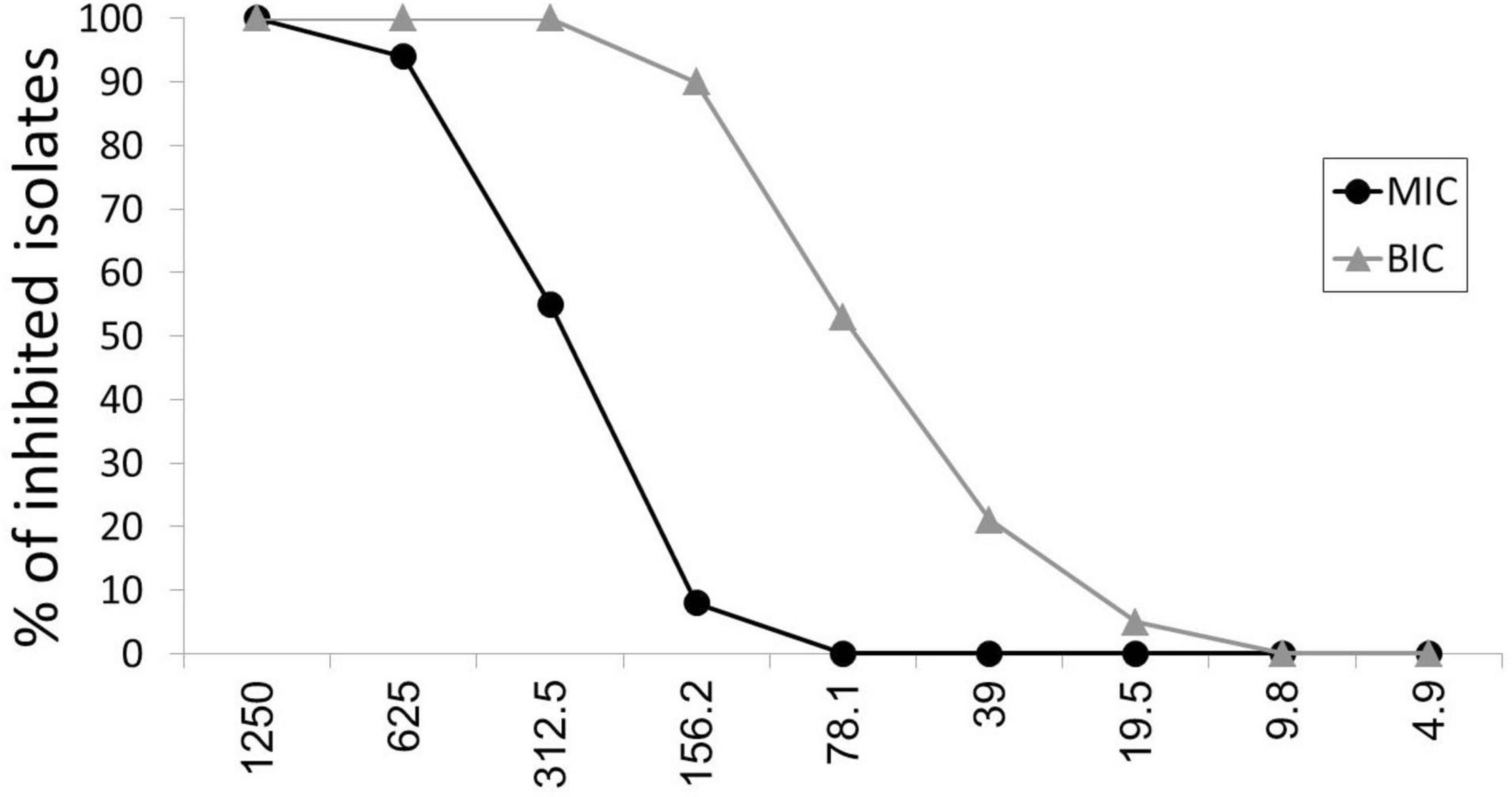
Figure 1. Antibacterial and anti-biofilm effect of ascorbic acid. MIC, minimal inhibitory concentration; BIC, biofilm inhibitory concentration.
At sub-inhibitory concentrations (19.5–312.5 μg/ml), ascorbic acid had 100% biofilm inhibitory effect as presented in Figure 1. BIC50 and BIC90, biofilm inhibitory concentrations of ascorbic acid that inhibits the biofilm formation in 50% and 90% of isolates, were 78.1 and 156.2 μg/ml, respectively.
The Results of Ascorbic Acid/Antimicrobial Combinations
The results of the combination studies are shown in Table 2. Synergy was detected in the five antimicrobial–ascorbic acid combinations tested.
Piperacillin and piperacillin/tazobactam MIC showed onefold decrease when combined with ascorbic acid (FIC = 0.5). Ceftazidime MIC showed twofold reductions when combined with ascorbic acid (FIC = 0.25). Ciprofloxacin and gentamicin MIC showed threefold decreases when combined with ascorbic acid (FIC = 0.125).
Time-Kill Kinetics Study
The time-kill kinetics study of ascorbic acid against the tested P. aeruginosa isolates significantly reduced the number of viable bacterial cells over the first 12, and 24 h, respectively, as shown in Figure 2.
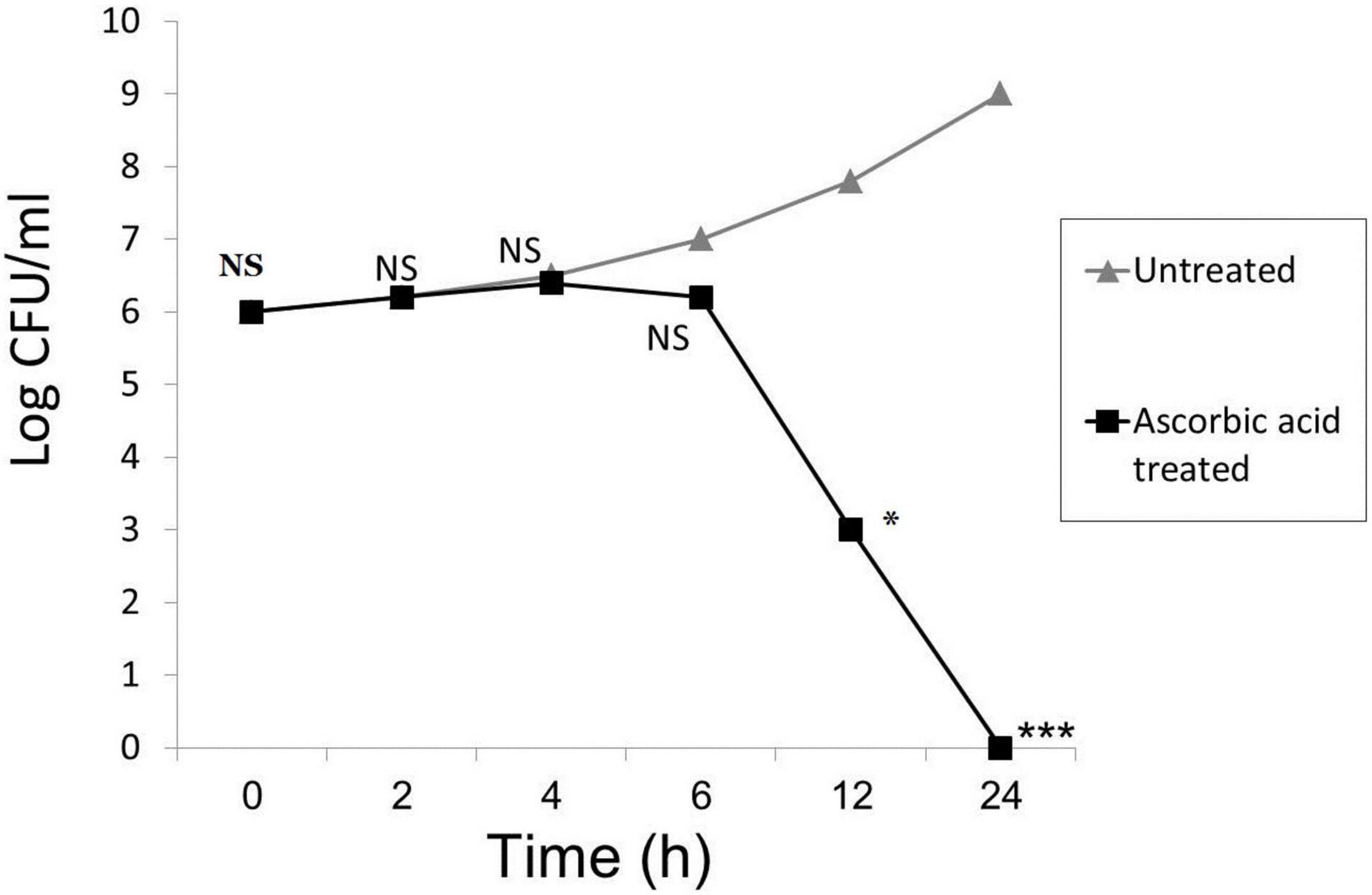
Figure 2. Time-kill kinetics of ascorbic acid. Mean from 3 replicates plotted for all panels; (* means significant, p- value* < 0.05, *** < 0.001, and NS means not significant).
Effect of Ascorbic Acid on Gene Expression
The results showed that the expression levels of all tested antimicrobial resistance genes (ampC, blaSHV, blaTEM, gyrA, and aacC1) and biofilm-associated gnes (lasR, lecA, and pelA) were down-regulated as shown in Figure 3. We observed a 46.1- and 40.5-fold decrease in the expression of the blaSHV and blaTEM genes, respectively, under ascorbic acid-treated condition (p value < 0.05). Results also confirmed that there was a significant fold change decrease (60.6 and 50.1) in transcription of biofilm-associated genes, lasR and pelA, respectively, (p value < 0.05) compared to untreated culture, thus confirming the anti-biofilm effect of ascorbic acid.
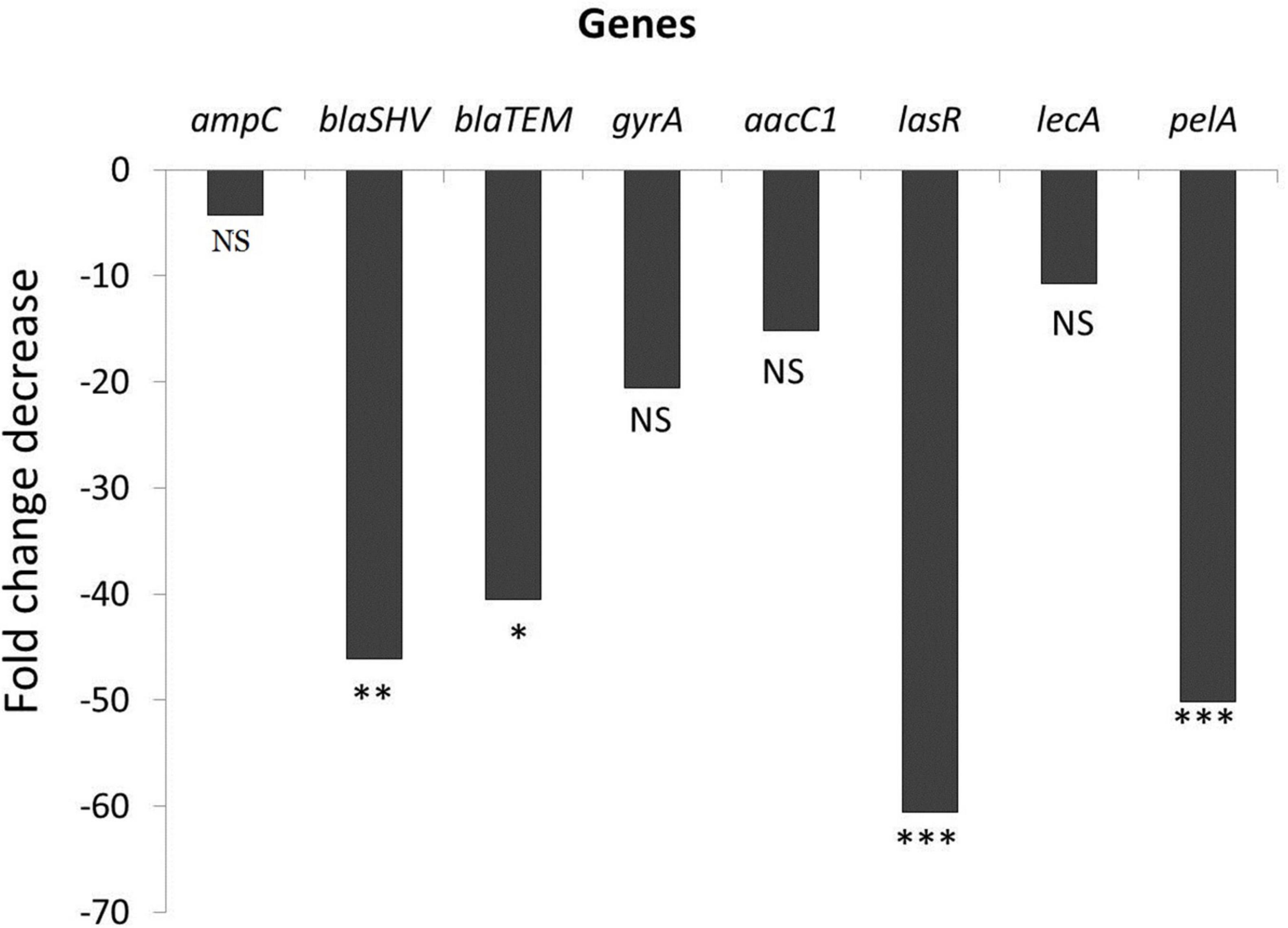
Figure 3. Effect of ascorbic acid on gene expression (* means significant, p- value* < 0.05, value** < 0.01, *** < 0.001).
In vivo Assessment of the Antibacterial Effect of Ascorbic Acid
All remaining rats in group 1 died after 24 h, and then all the rats were culled after 72 h (6 h after the last dose of treatment).
Bacterial Count
The five rats culled at the start of the experiment (3 h after inoculation of P. aeruginosa) presented bilateral pneumonia with a mean P. aeruginosa count of log10; 4.4, 7.5, 6.6, and 10.8 for peritoneal fluid, blood, bronchoalveolar lavage, and lung, respectively (Figure 4). The viable count after treatment was calculated in peritoneal fluid, blood, bronchoalveolar lavage, and lung, and the mean is presented in Figure 4. VIT C+AB group shows complete eradication of P. aeruginosa infection. A significant reduction of the bacterial count was seen in the AB group and VIT C group, respectively, in comparison with the untreated PN group (p value < 0.05).
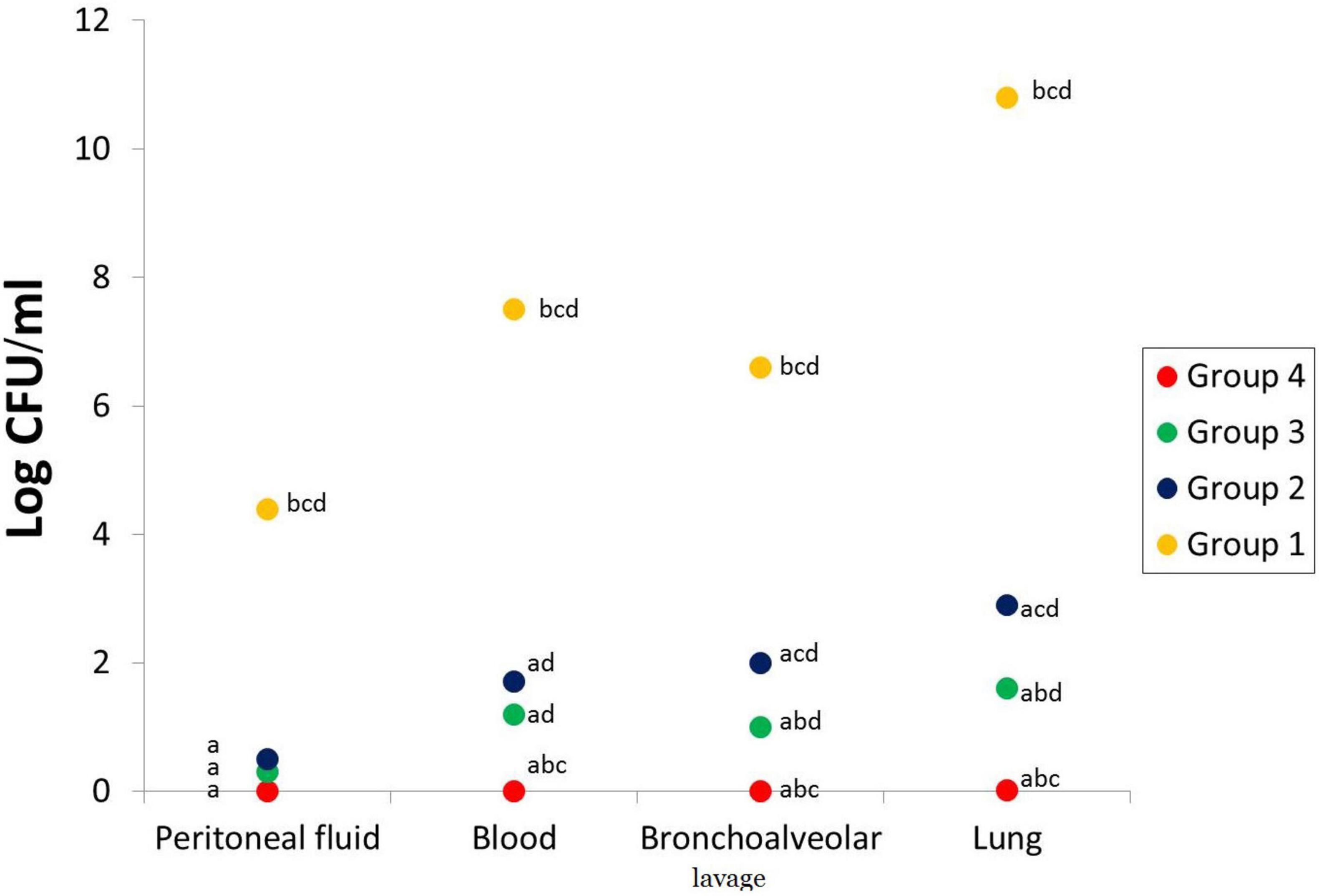
Figure 4. Bacterial count in the peritoneal lavage, blood, bronchoalveolar lavage, and lungs of different rat groups. aSignificant difference compared to PN group. bSignificant difference compared to VIT C group. cSignificant difference compared to AB group. dSignificant difference compared to VIT C+AB group. PN, pneumonia; VIT C, vitamin C; AB, antibiotic.
Oxidative Stress Parameters
VIT C alone, AB alone, and VIT C+AB groups showed a significant decrease in MDA with a substantial increase in GSH and TAC compared to the control group. Co-administration of VIT C + AB showed more improvement and normalization of oxidative stress parameters compared to VIT C or AB groups, as shown in Figures 5A–C.
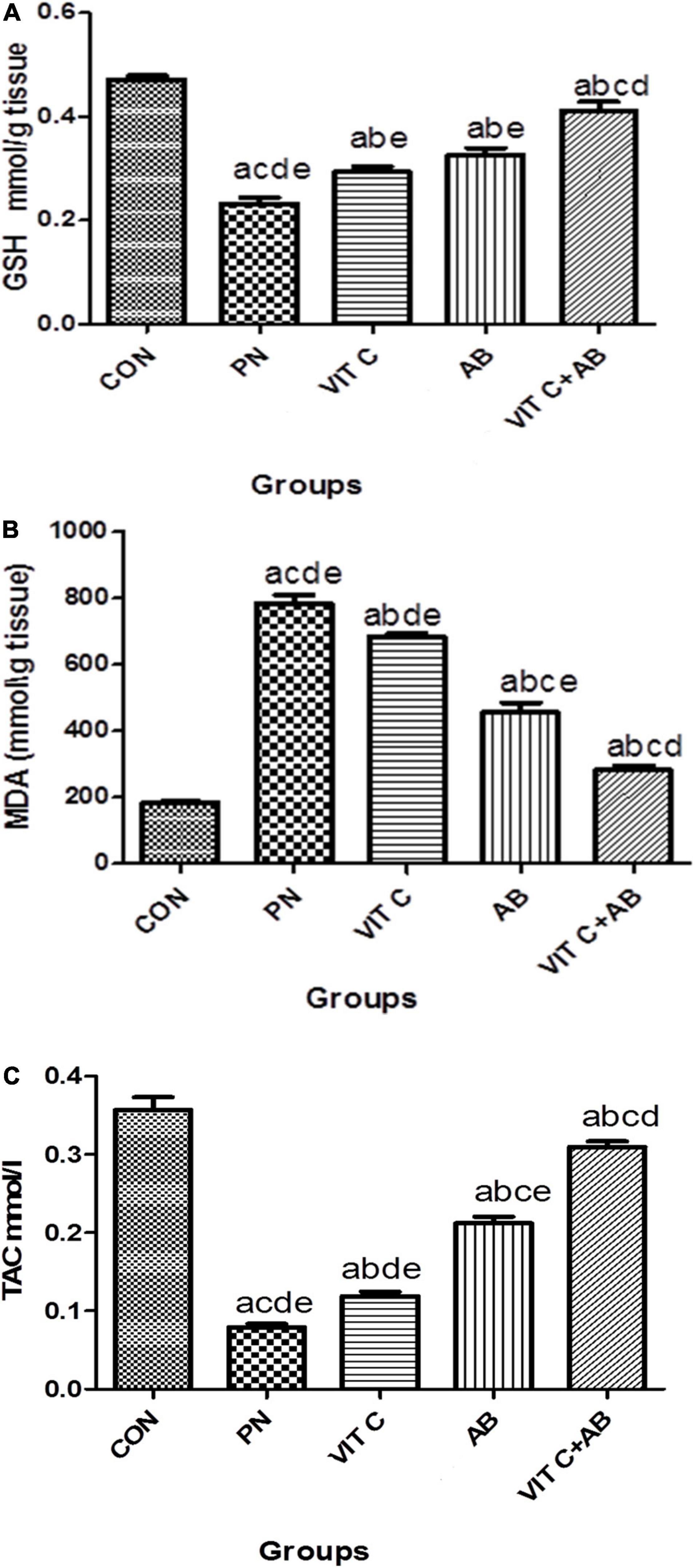
Figure 5. Oxidative stress parameters of different rat groups. (A) Glutathione (GSH) in lung tissue, (B) malondialdehyde (MDA) in lung tissue, (C) total antioxidant capacity (TAC) in serum. aSignificant difference compared to PN group. bSignificant difference compared to VIT C group. cSignificant difference compared to AB group. dSignificant difference compared to VIT C+AB group. PN, pneumonia group; VIT C, vitamin C; AB, antibiotic.
Histological Examination
Comparison of the histological picture of rat lung tissues of different groups is presented in Figures 6A–D. The five rats killed at the start of the experiment (PN group) showed a histological picture of bilateral pneumonia. Improvement of the histological picture in all treatment groups was detected but with different degrees. The VIT C+AB group showed nearly complete improvement of the signs of pneumonia. Signs of pneumonia were still present in VIT C and AB groups, respectively, but less than PN group.
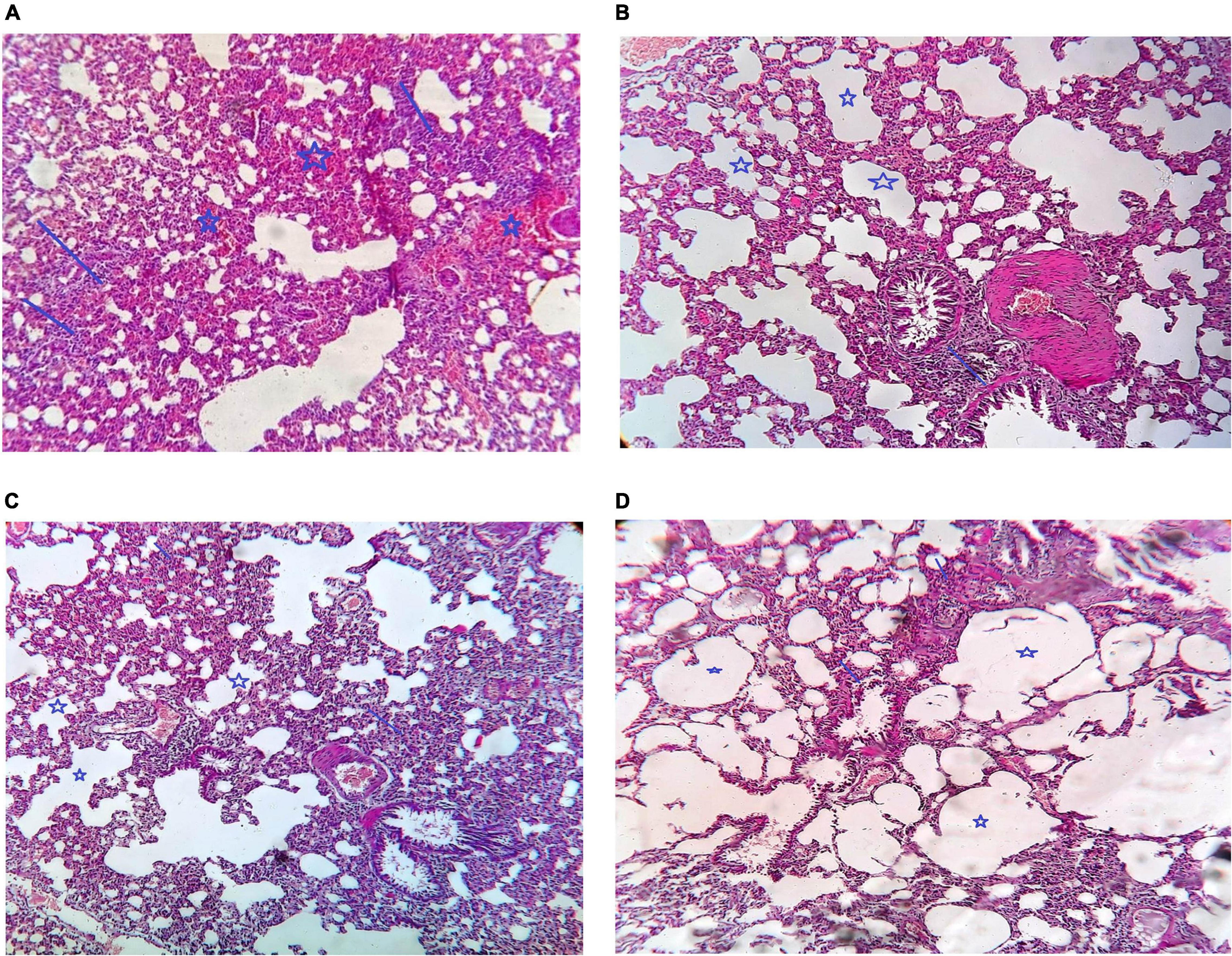
Figure 6. Histological examination of different groups of rat lungs. (A) PN group, Arrow show multiple foci of moderate inflammatory infiltrate. Star showed marked intraalveolar congestion, (B) VIT C group: Arrow showed that there is alveoli are still congested and contain inflammatory infiltrate but less than that in case of pneumonia only. Star showed that there is some alveoli are cleared from congestion and inflammatory infiltrate, (C) AB group: Arrow show moderate peribroncheal inflammatory infiltrate. Star show alveoli cleared from congestion and inflammatory infiltrate, (D) VIT C+ AB group: Arrow showed there is no peribronchial inflammatory infiltrate. Star showed cleared alveoli with no congestion or inflammation.
Discussion
This study is the first insight wherein we evaluated antimicrobial and anti-biofilm effect of vitamin C against P. aeruginosa clinical isolates in vitro and in vivo.
The antimicrobial effect of vitamin C exists against the all the P. aeruginosa clinical isolates tested. To our surprise, vitamin C inhibited the growth of P. aeruginosa clinical isolates at lower concentration ranges of 156.2–1,250 μg/ml, while MIC50 and MIC90 were 312.5 and 625 μg/ml, respectively.
The antimicrobial effect of vitamin C corroborates with the previous results by Golonka et al. (2017), which depict that the MIC 310 μg/mL of vitamin C could inhibit P. aeruginosa growth in vitro. In addition, vitamin C at low concentration (150 μg/ml) was shown to inhibit the growth of S. aureus and E. faecalis (Verghese et al., 2017). Further studies reported the antibacterial effect of vitamin C against E. coli and Klebsiella (Abd El-Baky and El-Gebaly, 2012).
This is in contrast to the findings reported by Pandit et al., 2017. They reported that vitamin C at low concentrations did not exhibit any inhibitory effect against planktonic bacteria. They suggested that vitamin C enhances the antibiotic bacterial susceptibility without demonstrating direct bactericidal activity.
With regard to the reports mentioned above, the differences of low or no antibacterial effect of vitamin C are probably attributed to the variations in the methodologies attributed during the study, i.e., differences in the bacterial strains, bacterial cell density, culture media composition, and concentration of vitamin C.
Moving on to the biofilm production, we found an anti-biofilm effect of vitamin C on the same isolates that were examined, based on the absorbance results that we obtained from the ELISA reader. Biofilm inhibitory concentrations of ascorbic acid among P. aeruginosa isolates were 19.5–312.5 μg/ml, with BIC50 and BIC90 being 78.1 and 156.2 μg/ml, respectively. In this aspect, Abd El-Baky and El-Gebaly (2012) reported that vitamin C at a concentration of 100 mg/ml could inhibit biofilm production on the catheter surface. There was up to a 92% reduction in biofilm production. Likewise, Sendamangalam et al. (2011) and Eydou et al. (2020) reported 2 mg/ml and 5.61 mg/ml, respectively, as the biofilm inhibitory concentration of vitamin C against S. mutans.
Furthermore, vitamin C could effectively counteract biofilm production by methicillin-resistant S. aureus (MRSA) at a lower concentration range of 8–16 μg/ml (Ali Mirani et al., 2018).
A synergistic effect was detected in the five antimicrobial–ascorbic acid combinations tested; piperacillin and piperacillin/tazobactam (FIC = 0.5), ceftazidime (FIC = 0.25), ciprofloxacin and gentamicin (FIC = 0.125). The enhancement of antibiotic activity or the reversal of antibiotic resistance by non-traditional antibiotics support the classification of vitamin C as a modifier of antibiotic activity. Similar synergistic effects between antimicrobials and ascorbic acid were previously reported by Cursino et al. (2005) and Abd El-Baky and El-Gebaly (2012).
The synergistic effect of ascorbic acid with antibiotics may be due to its effect on certain metabolic activities associated with protein synthesis inside bacterial cells, making the bacterial cells more permeable to antibiotics through its effect on the cytoplasmic membrane, or it could be due to the effect of hydrogen peroxide produced by the oxidation of ascorbic acid, which causes antibiotics to have a higher potency (Kramarenko et al., 2007). Certain antibiotics increase the production of reactive oxygen species (free radicals) in various bacterial species, suggesting that ascorbic acid may serve as a free radical scavenger (Albesa et al., 2004). Also, the synergistic effect of ascorbic acid with antibiotics may be due to down regulation of antibiotic-resistant genes.
Biofilm-forming P. aeruginosa strains demonstrated higher expression levels of lasR, lecA, and pelA genes (Abdelraheem et al., 2020).
Further, to confirm the anti-biofilm effect of vitamin C and the corresponding synergistic effect with antibiotics against P. aeruginosa tested isolates, a transcriptional response was analyzed in the presence of Vitamin C. The results showed that all tested biofilm-associated gene expression levels vital to P. aeruginosa biofilm formation (lasR, lecA, and pelA) were down-regulated after being treated with ascorbic acid, with significant decrease in lasR and pelA (p value < 0.05). Therefore, it was suggested that the anti-biofilm activity of vitamin C was due to the inhibition of biofilm-forming genes expression.
Also, the expression levels of all tested genes (ampC, blaSHV, blaTEM, aacC1, and gyrA) are essential for resistance to piperacillin, piperacillin/tazobactam, ceftazidime, ciprofloxacin and gentamicin were down-regulated after treating P. aeruginosa isolates with ascorbic acid, the expression levels of all tested genes (ampC, blaSHV, blaTEM, aacC1, and gyrA), which are essential for resistance to piperacillin, piperacillin/tazobactam, ceftazidime, ciprofloxacin and gentamicin, were down-regulated, with a significant decrease in blaSHV and blaTEM (P value < 0.05).
In our in vivo investigation, the efficacy of vitamin C treatment in rats after infection with P. aeruginosa showed that the administration of vitamin C in the infected animal partially resolved the disease and lowered the bacterial count. In vivo, the rats’ vitamin C and antibiotic assessment showed that antibiotic alone has a better antibacterial effect than vitamin C alone. Still, the administration of both at a lower dose for treating pseudomonas infection in rats has a synergistic and more powerful impact. Vitamin C shows excellent in vitro results as an antibacterial and anti-biofilm agent. Still, a combination of vitamin C and antibiotics is necessary to eradicate the bacterial infection in vivo. Vitamin C has a synergistic effect with antibiotics in vitro and in vivo. Vitamin C should be routinely prescribed with antibiotics to treat pseudomonas infections in clinical settings as this combination will shorten the antibiotic course, decrease the dose of the antibiotic, and reduce the development of bacterial resistance. However, further in vivo studies are needed before generalizing the concept about the effectiveness of ascorbic acid–antibiotics combined therapy. Future research on ascorbic acid–antimicrobial interactions is required to control MDR bacteria.
Data Availability Statement
The original contributions presented in the study are included in the article/supplementary material, further inquiries can be directed to the corresponding author/s.
Ethics Statement
The studies involving human participants were reviewed and approved by Ethical Committee of Minia University. The patients/participants provided their written informed consent to participate in this study. The animal study was reviewed and approved by Institutional Research Ethics Committee.
Author Contributions
WA, RR, AA, and YM: concept and study design. WA, MR, and RY: experiments and results analysis. All authors drafted and revised the manuscript and read and approved the final manuscript.
Conflict of Interest
The authors declare that the research was conducted in the absence of any commercial or financial relationships that could be construed as a potential conflict of interest.
Publisher’s Note
All claims expressed in this article are solely those of the authors and do not necessarily represent those of their affiliated organizations, or those of the publisher, the editors and the reviewers. Any product that may be evaluated in this article, or claim that may be made by its manufacturer, is not guaranteed or endorsed by the publisher.
Acknowledgments
We would like to thank the technicians at Pharmacology Department, Faculty of Medicine, Minia University, who helped us deal with mice.
References
Abd El-Baky, R., and El-Gebaly, E. (2012). Effect of levofloxacin and Vitamin C on bacterial adherence and preformed biofilm on urethral catheter surfaces. J. Microb. Biochem. Technol. 4, 131–136. doi: 10.4172/1948-5948.1000083
Abdelraheem, W. M. (2019). Fresh garlic extract has a synergistic effect with antibiotics on ESBLs producing E. coli urinary isolates. J. Adv. Microbiol. 14, 1–9.
Abdelraheem, W. M., and Mohamed, E. S. (2021). The effect of Zinc Oxide nanoparticles on Pseudomonas aeruginosa biofilm formation and virulence genes expression. J. Infect. Dev. Ctries 15, 826–832. doi: 10.3855/jidc.13958
Abdelraheem, W., Abdelkader, A., Mohamed, E., and Mohammed, M. (2020). Detection of biofilm formation and assessment of biofilm genes expression in different Pseudomonas aeruginosa clinical isolates. Meta Gene 23:100646.
Albesa, I., Becerra, M. C., Battan, P. C., and Paes, P. L. (2004). Oxidative stress involved in the antibacterial action of different antibiotics. Biochem. Biophys. Res. Commun. 317, 605–609. doi: 10.1016/j.bbrc.2004.03.085
Ali Mirani, Z., Khan, M. N., Siddiqui, A., Khan, F., Aziz, M., Naz, S., et al. (2018). Ascorbic acid augments colony spreading by reducing biofilm formation of methicillin-resistant Staphylococcus aureus. Iran. J. Basic Med. Sci. 21, 175–180. doi: 10.22038/IJBMS.2018.20714.5398
Aliakbarzade, K., Farajnia, S., KarimiNik, A., Zarei, F., and Tanomand, A. (2014). Prevalence of aminoglycoside resistance genes in Acinetobacter baumannii isolates. Jundishapur J. Microbiol. 7:e11924.
Appiah, T., Boakye, Y. D., and Agyare, C. (2017). Antimicrobial activities and time-kill kinetics of extracts of selected ghanaian mushrooms. Evid. Based Complement. Altern. Med. 2017:4534350. doi: 10.1155/2017/4534350
Clinical and Laboratory Standard Institute [CLSI] (2019). Performance Standards for Antimicrobial Susceptibility Testing. CLSI Supplement M100, 29th Edn. Wayne, PA: Clinical and Laboratory Standards Institute.
Cursino, L., Chartone-Souza, E., Nascimento, A., and Andréa Maria, A. (2005). Synergic interaction between ascorbic acid and antibiotics against Pseudomonas aeruginosa. Braz. Arch. Biol. Technol. 48, 379–384.
Drenkard, E., and Ausubel, F. M. (2002). Pseudomonas biofilm formation and antibiotic resistance are linked to phenotypic variation. Nature 416, 740–743. doi: 10.1038/416740a
Eydou, Z., Jad, B. N., Elsayed, Z., Ismail, A., Magaogao, M., and Hossain, A. (2020). Investigation on the effect of vitamin C on growth & biofilm-forming potential of Streptococcus mutans isolated from patients with dental caries. BMC Microbiol. 20:231. doi: 10.1186/s12866-020-01914-4
Farris, K. (2014). “Cosmeceutical vitamins: vitamin C,” in Cosmeceuticals, 3rd Edn. Vol. 2, ed. Z. D. Draelos (Amsterdam, Elsevier), 37–42.
Golonka, I., Oleksy, M., Junka, A., Matera-Witkiewicz, A., Bartoszewicz, M., and Musiał, W. (2017). Selected physicochemical and biological properties of EthylAscorbic acid compared to ascorbic acid. Biol. Pharm. Bulletin. 40, 1199–1206. doi: 10.1248/bpb.b16-00967
Gorgani, N., Ahlbrand, S., Patterson, A., and Pourmand, N. (2009). Detection of point mutations associated with antibiotic resistance in Pseudomonas aeruginosa. Int. J. Antimicrob. Agents 34, 414–418. doi: 10.1016/j.ijantimicag.2009.05.013
Gupta, G., and Guha, B. (1941). The effect of Vitamin C and certain other substances on the growth of microorganisms. Ann. Biochem. Exp. Med. 1, 14–26.
Hancock, R., and Speert, D. (2000). Antibiotic resistance in Pseudomonas aeruginosa: mechanisms and impact on treatment. Drug. Resist. Updat. 3, 247–255.
Hong, J., Kim, H., Kang, S., Lee, W., and Hwang, I. (2016). Vitamin C is taken up by human T cells via sodium-dependent vitamin C transporter 2 (SVCT2) and exerts inhibitory effects on the activation of these cells in vitro. Anat. Cell Biol. 49, 88–98. doi: 10.5115/acb.2016.49.2.88
Isela, S., Sergio, N., Jose, M., Rene, H., and Claudio, C. (2013). Ascorbic acid on oral microbial growth and biofilm formation. Pharma Innov. 28, 104–109.
Konings, A., Martin, L., Sharples, K., Roddam, L., Latham, R., Reid, D., et al. (2013). Pseudomonas aeruginosa uses multiple pathways to acquire iron during chronic infection in cystic fibrosis lungs. Infect. Immun. 81, 2697–2704. doi: 10.1128/IAI.00418-13
Kramarenko, G. G., Hummel, S. G., Martin, S. M., and Buettner, G. R. (2007). Ascorbate reacts with singlet oxygen to produce hydrogen peroxide. Photochem. Photobiol. 82, 1634–1637. doi: 10.1562/2006-01-12-RN-774
Lewis, K. (2001). Riddle of biofilm resistance. Antimicrob. Agents Chemother. 45, 999–1007. doi: 10.1128/AAC.45.4.999-1007.2001
Livak, K., and Schmittgen, T. (2001). Analysis of relative gene expression data using real-time quantitative PCR and the 2(-Delta DeltaC(T)) Method. Methods 25, 402–408. doi: 10.1006/meth.2001.1262
Mackay, M. L., Milne, K., and Gould, I. M. (2000). Comparison of methods for assessing synergic antibiotic interactions. Int. J. Antimicrob. Agents 15, 125–129. doi: 10.1016/s0924-8579(00)00149-7
Moron, M. S., Depierre, J. W., and Mannervik, B. (1979). Levels of glutathione, glutathione reductase and glutathione S-transferase activities in rat lung and liver. Biochim. Biophys. Acta 582, 67–78. doi: 10.1016/0304-4165(79)90289-7
Pandit, S., Ravikumar, V., Abdel-Haleem, A. M., Derouiche, A., Mokkapati, V. R., Sihlbom, C., et al. (2017). Low concentrations of VitC reduce the synthesis of extracellular polymers and destabilize bacterial biofilms. Front. Microbiol. 8:2599. doi: 10.3389/fmicb.2017.02599
Samet, M., Ghaemi, E., Jahanpur, S., and Jamalli, A. (2013). Evaluation of biofilm-forming capabilities of urinary Escherichia coli isolates in microtiter plate using two different culture media. Int. J. Mol. Clin. Microbiol. 3, 244–247.
Sendamangalam, V., Choi, O. K., Kim, D., and Seo, Y. (2011). The anti-biofouling effect of polyphenols against Streptococcus mutans. Biofouling 27, 13–19. doi: 10.1080/08927014.2010.535897
Slade, H., and Knox, G. (1950). Nutrition and the role of reducing agents in the formation of streptolysin O by a group A hemolytic streptococcus. J. Bacteriol. 60:301. doi: 10.1128/jb.60.3.301-310.1950
Sorice, A., Guerriero, E., Capone, F., Colonna, G., Castello, G., and Costantini, S. (2014). Ascorbic acid: its role in immune system and chronic inflammation diseases. Mini Rev. Med. Chem. 14, 444–452. doi: 10.2174/1389557514666140428112602
Stover, C., Pham, X., Erwin, A., Mizoguchi, S., Warrener, P., Hickey, M., et al. (2000). Complete genome sequence of Pseudomonas aeruginosa PAO1, an opportunistic pathogen. Nature 406, 959–964. doi: 10.1038/35023079
Verghese, R. J., Mathew, S. K., and David, A. (2017). Antimicrobial activity of vitamin C demonstrated on uropathogenic Escherichia coli and Klebsiella pneumoniae. J. Curr. Res. Sci. Med. 3, 88–93. doi: 10.4103/jcrsm.jcrsm_35_17
Vilchèze, C., Hartman, T., Weinrick, B., and Jacobs, J. (2013). Mycobacterium tuberculosis is extraordinarily sensitive to killing by a Vitamin C-induced Fenton reaction. Nat. Commun. 4:1881. doi: 10.1038/ncomms2898
Keywords: antimicrobial and anti-biofilm effects of vitamin C, anti-biofilm strategies, synergism, MDR, biofilm former P. aeruginosa strains
Citation: Abdelraheem WM, Refaie MMM, Yousef RKM, Abd El Fatah AS, Mousa YM and Rashwan R (2022) Assessment of Antibacterial and Anti-biofilm Effects of Vitamin C Against Pseudomonas aeruginosa Clinical Isolates. Front. Microbiol. 13:847449. doi: 10.3389/fmicb.2022.847449
Received: 02 January 2022; Accepted: 12 April 2022;
Published: 20 May 2022.
Edited by:
Ziad Daoud, Central Michigan University, United StatesReviewed by:
Veronica Lazar, University of Bucharest, RomaniaMurugan Kasi, Manonmaniam Sundaranar University, India
Copyright © 2022 Abdelraheem, Refaie, Yousef, Abd El Fatah, Mousa and Rashwan. This is an open-access article distributed under the terms of the Creative Commons Attribution License (CC BY). The use, distribution or reproduction in other forums is permitted, provided the original author(s) and the copyright owner(s) are credited and that the original publication in this journal is cited, in accordance with accepted academic practice. No use, distribution or reproduction is permitted which does not comply with these terms.
*Correspondence: Wedad M. Abdelraheem, d2VkYWQuYWJkZWxyZWhlYW1AbXUuZWR1LmVn, YWx0YXF3YS4yMDEyQHlhaG9vLmNvbQ==