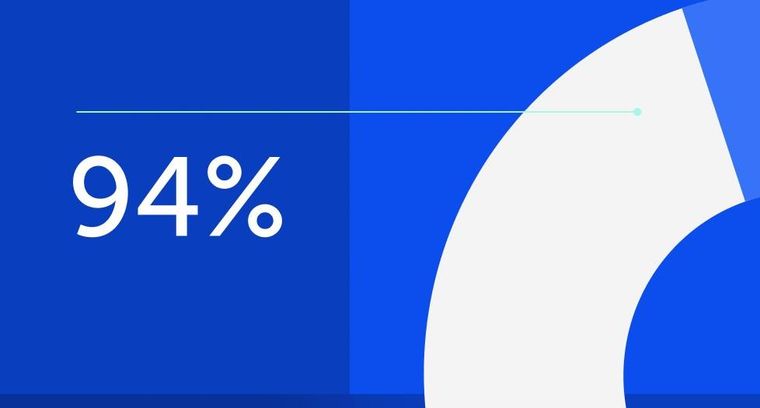
94% of researchers rate our articles as excellent or good
Learn more about the work of our research integrity team to safeguard the quality of each article we publish.
Find out more
MINI REVIEW article
Front. Microbiol., 23 March 2022
Sec. Infectious Agents and Disease
Volume 13 - 2022 | https://doi.org/10.3389/fmicb.2022.846959
This article is part of the Research TopicInsights in Infectious Agents and Disease: 2021View all 37 articles
Antimicrobial resistance is considered one of the three most important health problems by the World Health Organization. The emergence and spread of an increasing number of antimicrobial-resistant microorganisms make this a worldwide problem. Antibiotic-resistant bacteria are estimated to be the cause of 33,000 deaths in Europe and 700,000 worldwide each year. It is estimated that in 2050 bacterial infections will cause 10 million deaths across the globe. This problem is concomitant with a decrease in the investment and, therefore, the discovery and marketing of new antibiotics. Recently, there have been tremendous efforts to find new effective antimicrobial agents. Gold complexes, with their broad-spectrum antimicrobial activities and unique modes of action, are particularly relevant among several families of derivatives that have been investigated. This mini review revises the role of gold-derived molecules as antibacterial agents.
In spite of the important role of antibiotics in human health during the 60s and 70s, infectious diseases continue being the second cause of death worldwide (Spellberg et al., 2004). The emergence and spread of drug-resistant microorganisms (bacteria, fungi, virus, and parasites) are among the most important health threats today. This issue is especially alarming regarding antibiotic resistance in bacteria, as the rapid spread of multi- and pan-resistant bacteria in hospitals and in the community is associated with an increase in mortality and morbidity. It has been estimated that if no measure is taken in the next years, the number of deaths by infections caused by resistant bacteria could increase up to 10 million each year, exceeding the 1.8 million deaths by cancer. However, a recent study reported that infections associated with antimicrobial resistance are the first cause of death worldwide surpassing those caused by cancer, with 4.95 million of deaths in 2019 (Antimicrobial Resistance Collaborators, 2022). At an economic level, the infections by resistant bacteria represent 2.5 million days of extra hospitalization per year only in the European Union, with a cost of about 900 million euros (Glasner et al., 2013).
Following the discovery of cephalosporins in 1968, the discovery of new antibiotics with new mechanisms of action has slowed (Powers, 2004). After that, most of the antibiotics developed were derivatives from the existing classes and are considered as “new-generation” antibiotics. However, the use of new antibiotics has, sooner or later, been followed by the emergence of bacterial strains resistant to these antibiotics (Saga and Yamaguchi, 2009).
The decrease in investment for the search for antibiotics by pharmaceutical companies could be due to the time-consuming and financially taxing venture that traditional methods employed to discover new antibiotics require. This has led researchers to mine existing libraries of clinical molecules in order to repurpose old drugs for different clinical diseases as antimicrobials. This effort led to the discovery of the gold complex auranofin, a drug initially approved as an anti-rheumatic agent, which also possesses potent antibacterial activity in a clinically achievable range (Cassetta et al., 2014). Interest in antimicrobial gold complexes is not new. The work of Robert Koch at the end of the 19th century demonstrated that potassium dicyanidoaurate(I), K[Au(CN)2], showed activity against Mycobacterium tuberculosis, a causative agent of tuberculosis (Higby, 1982). Since then, interest in the anti-infective activity of gold complexes has prompted the evaluation of a large number of derivatives as potential agents against a high number of bacteria, fungi and parasite species.
So far, most studies have been focused on gold(I) complexes in connection with the remarkable bactericidal effects of Au(I) phosphine complex auranofin. By contrast, although gold(III) complexes have been intensively investigated as antitumor agents due to their similarities with platinum(II) (Nardón et al., 2014), until recently there were very few studies related to their antimicrobial activity (Glišić and Djuran, 2014). However, the serious threat of resistant “super-bugs” has led to the evaluation of these antitumor drugs also as antimicrobials.
In the present work, the antibacterial activity of both gold(I) and gold(III) representative complexes is revised.
In recent years, gold(I) compounds have been considered of clinical relevance due to their anti-rheumatic activity as well as their anticancer properties. In addition, the interest in these compounds as antibacterial agents is increasing, and different families of gold(I) have been investigated, including the Au(I) N-heterocyclic carbenes (NHCs) (Özdemir et al., 2004). A selection of gold(I) complexes with antimicrobial activity and their chemical structure is shown in Figure 1, as well as a compilation of their antibacterial (Table 1), antifungal (Table 2) and antibiofilm activities (Table 3).
The most known and studied gold(I) complex is auranofin [triethylphosphine (2,3,4,6-tetra-O-acetyl-β-1-D-thiopyranosato-S)Au(I)], a mixed ligand gold compound in clinical use since 1985 for the treatment of severe rheumatoid arthritis (Shaw, 1999), was first described recently as an antimicrobial agent (Cassetta et al., 2014). Auranofin possesses potent microbicidal effects in vitro against Staphylococcus spp. and Candida spp. (Fuchs et al., 2016; Wiederhold et al., 2017) and also moderate activity against Staphylococcus aureus and Pseudomonas aeruginosa biofilms (Torres et al., 2016, 2018). Auranofin (1) consists of a gold(I) center linearly coordinated to a triethylphosphine and a thiosugar ligand as shown in Figure 1 (Roder and Thomson, 2015). Subsequently, a relatively large number of linear phosphine gold(I) complexes, with a sulfur atom in the coordination sphere, have been synthesized and evaluated for their antibacterial effects, exhibiting a different spectrum of in vitro activity against several strains of Gram-positive and Gram-negative bacteria. For example, complexes 2 and 3 were comparably active as complex 1 against Gram-positive bacteria and were more active against Escherichia coli (Figure 1). When compared to the reference clinical antibiotics piperacillin and chloramphenicol, gold(I) complexes were found to be less active against Gram-negative bacteria but more active against Gram-positive strains (Novelli et al., 1999). It has been reported that this compound is unable to generate resistant mutants in Gram-positive bacteria. The hypothesis for explaining this fact is that auranofin has multiple targets or an unspecified mechanism of action (Thangamani et al., 2016). Thus, complex 1 causes a reduction in the cell wall and DNA biosynthesis pathway and also in bacterial protein synthesis. In addition, a decrease in the production of important toxins, such as hemolysin and Panton Valentine toxins, occurs when the bacteria are exposed to this compound (Thangamani et al., 2016).
Antifungal activity of complexes 2 and 3 against strains of C. albicans and A. niger was also determined (Novelli et al., 1999). Lipophilic compound 2 displayed excellent activity against both fungi, being comparable with miconazole nitrate. On contrary, more hydrophilic compound 3 was significantly less active on fungi but was more active than 2 on E. coli.
Other linear gold(I)–triphenylphosphine complexes displaying interesting antimicrobial profiles are gold(I)–triphenylphosphine complexes containing nitrogen-donor heterocyclic ligands. Thus, Nomiya et al. prepared complexes 4 and 5, which displayed selective and effective antimicrobial activities against two Gram-positive bacteria (Bacillus subtilis and S. aureus) and modest activities against one yeast (C. albicans). In contrast, poor activities observed in the corresponding silver(I) complexes (Nomiya et al., 2000). The mechanism of antimicrobial action of these heterocycle-containing gold(I)– complexes was not investigated in depth but, according to the authors, it is likely quite different from that of the corresponding silver(I) complexes.
Fillat et al. (2011) described a new family of (aminophosphane)gold(I) thiolate complexes 6 and 7, also belonging to the type of linear gold(I)–phosphine complexes, which exhibit powerful anti-bacterial activity. As expected, these linear gold(I) complexes are more efficient against Gram-positive microorganisms, presenting excellent activity against Enterococcus faecalis and S. aureus.
As water is the biologically most relevant solvent, the development of water-soluble gold-based metallodrugs is a matter of increasing interest (Hristova et al., 2013). In this regard, Elie et al. (2009) described water-soluble linear gold(I)– complexes 8 and 9 containing sulfonated phosphanes, which display moderate to high antibacterial activity and moderate antifungal activity. A relevant advantage of complexes 8 and 9 in comparison with the previously reported linear gold(I)– phosphine complexes is their lower hydrophobicity, enabling the preparation of aqueous solutions without the addition of organic co-solvents such as dimethyl sulfoxide (DMSO).
Frik et al. (2012) reported heterometallic gold-silver complexes 10 and 11 and compared their antimicrobial activity to silver salts. The Au2Ag complexes resulted up to 10 times more toxic than the silver salts for all Gram-positive (B. cereus, S. aureus) and Gram-negative bacteria (E. coli, Salmonella typhimurium), confirming that the biological activity of these complexes did not originate from the dissociation of the silver perchlorate or triflate from the heterometallic derivatives. The heterometallic compounds were also more toxic to Gram-negative bacteria than the corresponding dinuclear gold complexes, pointing to a synergistic or cooperative effect between the gold and silver metals. This result is of considerable importance for the development of novel metalloantibiotics which may be toxic for both Gram-negative as well as Gram-positive bacteria. For complex 11 containing ClO4– contraion, a remarkably high toxicity for Gram-positive bacteria B. cereus was observed. Both complexes 10 and 11 were inactive against yeast S. cerevisiae.
Barreiro et al. (2012) described several gold(I) sulfanylcarboxylates 12 showing significant activity against S. aureus and Bacillus subtilis. Similar to other gold(I) compounds, these complexes show good activity against Gram-positive strains (S. aureus and B. subtilis), but low sensitivity against Gram-positive microorganisms (E. coli and P. aeruginosa) and fungi (C. albicans). The silver complexes incorporating the same ligands were also synthetized, enabling comparison of the activity of silver and gold complexes. Gold compounds displayed better activity against the two Gram-positive bacteria, while the silver complexes generally showed better minimal inhibitory concentration (MIC) values against Gram-negative strains and yeast C. albicans. As previously suggested by Nomiya et al. (2000) a different mechanism of antimicrobial action for silver and gold complexes was also hypothesized in which the antibacterial activity of silver(I) complexes is related to the ease with which they undergo ligand metathesis. However, this process would not explain the activity of the related gold(I) complexes.
N-Heterocyclic carbenes (NHC) are extremely good σ-donor ligands, thus forming strong Au-C bonds and constructing Au-NHC complexes that are stable in the presence of biologically relevant thiol groups. Accordingly, for several years, there has been a growing interest in the biomedical applications of gold complexes based on NHC ligands (Mora et al., 2019). Thus, in 2004, Özdemir et al. (2004) reported a novel family of NHC gold(I) complexes of the type [Au(NHC)2]+.
Among them, p-methoxybenzyl derivative 13 was found to inhibit the growth of P. aeruginosa, S. epidermidis, S. aureus, and E. faecalis with MIC values of 3.12 μg mL–1, 6.25 μg mL–1, 3.12 μg mL–1, and 3.12 μg mL–1, respectively. However, complex 13 only showed modest activity against yeast C. albicans (Özdemir et al., 2004).
Later in 2007, Ray et al. (2007) reported the Au(I) complexes of 1-benzyl-3-tert-butylimidazol-2-ylidene, as well as the Pd and Ag counterparts. While the palladium complexes displayed potent anticancer activity, the gold and silver complexes inhibited the growth of Gram-positive B. subtilis but did not show any activity against Gram-negative E. coli strains. Specifically, complex 14 showed a twofold stronger antibacterial activity toward B. subtilis compared to the corresponding silver complex. Incubation of B. subtilis cells with the gold compound increased the cell length, indicating that complex 14 inhibits bacterial proliferation by blocking cytokinesis. In contrast, incubation of B. subtilis with the equivalent silver complex resulted in only a very small increase in cell length, which agrees with a different mechanism of antimicrobial action for silver and gold complexes.
N-heterocyclic carbene complex 15 inhibited E. faecium and two methicillin-resistant S. aureus (MRSA) strains at MIC values of 3.12, 0.64, and 0.64 μM, respectively, being more active than 1 (MICs of 18.40, 2.30, and 2.30 μM) (Schmidt et al., 2017). Complex 14 displayed effective inhibition of mammalian and bacterial thioredoxin reductases (TrxR). As the TrxR system is essential for the antioxidant system of Gram-positive bacteria, this result is in accordance with the particularly high activity observed against Gram-positive strains. TrxR was also hypothesized as one of the multiple bacterial targets of complex 1 (Thangamani et al., 2016), confirming the interest in bacterial Trx and TrxR as viable antibacterial drug targets for gold-based anti-infective agents (Felix et al., 2021).
Samanta et al. (2013) described a N,N’-olefin functionalized bis-imidazolium gold(I) salt 16 of interest for the development of antimicrobial therapies in patients with keratitis-associated eye infections caused by multidrug-resistant bacteria and fungi. Complex 16 was more active against P. aeruginosa and S. aureus than antibiotics ceftazidime, vancomycin, and piperacilline and more active against filamentous fungi C. albicans and A. fumigatus than amphotericin B, fluconazole, and voriconazol. The bacterial killing activity was concentration-dependent, and no cell growth was seen even using complex 16 concentrations of 0.5 and 2.5 μM for P. aeruginosa and S. aureus, respectively. In addition, this Au(I) compound was able to eradicate biofilm formed over contact lenses. In order to better understand the mechanisms of action, interactions between the bacterial cell and complex 16 were observed using a scanning electron microscope (SEM). The images produced (Figure 2) clearly showed a morphological change in the microbial envelope after treatment with complex 16, which evidenced that the target would be on cell membranes or cell walls. The high binding affinities to bacterial cell membranes displayed by complex 16 in docking studies point to a mechanism of action based on the destabilization of the bacterial membrane, causing loss of structural integrity and a change in bacterial shape. Samanta et al. (2013) hypothesized that the destabilizing effect on the bacterial membrane is caused by the insertion of multiple molecules of complex 16 intercalating the lipids on the membrane.
Figure 2. Control P. aeruginosa cells (A) and cells treated with complex 16 (B). Reproduced from Samanta et al. (2013) (CC BY-NC 4.0).
In summary, several Au(I) complexes tested so far display antibacterial effects with a marked preference for Gram-positive bacteria. This selectiveness toward Gram-positive pathogens could be explained in that: (i) gold(I) complexes inhibit the thioredoxin reductase enzyme and, in Gram-negative bacteria this is compensated by the presence of the glutathione system; and (ii) the permeability barrier conferred by the outer membrane of Gram-negative bacteria impairs the transfer of the complex to the cytoplasm. In addition, other possible mechanisms of action proposed include the disruption of the bacterial cell wall and cytosolic membrane. SEM images of Gram-positive bacteria submitted to gold(I) compounds revealed morphological changes on the bacterial surface and cytosolic leakage, probably due to destabilization of the peptidoglycan membrane by the insertion of these compounds in the lipid bilayer, inducing a loss of the structural integrity of the bacteria.
Even though gold(III) complexes have been less studied as antibacterial agents than their gold(I) counterparts, the threat of multiresistant bacteria has led to the evaluation of these classical anticancer metallodrugs also as antibacterials. A selection of gold(III) complexes with antimicrobial activity and their chemical structure is shown in Figure 3, as well as a compilation of their antibacterial (Table 4), antifungal (Table 5) and antibiofilm activities (Table 3).
The stabilization of the oxidation state +3 is of great importance to the biological activity of these compounds. Otherwise, the metal center can ultimately undergo a reduction process leading to the formation of Au(0) (Pacheco et al., 2009). Thus, the most common gold(III) complexes used as antibiotics are cyclometallated organometallic gold compounds with the general formula [AuX2(L)], in which L is a bifunctional ligand that forms a C-Au bond, as well as a coordinate N-Au bond. The two remaining positions at the square planar gold(III) center are usually occupied by two monodentate or one bidentate anion, leading to the formation of neutral complexes (Tiekink, 2002).
Parish et al. performed a variety of biological tests to evaluate [AuCl2(damp)] (17) for antimicrobial activity; the complex displayed moderate, broad-spectrum activity against bacteria and fungi, but was less active than the control drugs ciprofloxacin and amphotericin B (Parish, 1999). In order to improve the antibacterial activity of complex 17, both chloride atoms were replaced by acetate ligands, leading to water-soluble complex 18 (Parish et al., 1996). Similar to its chloride analog, complex 18 showed a broad spectrum of antibacterial activity, with some preference to Gram-positive S. aureus and E. faecalis. The MIC values of complex 18 against Gram-positive bacteria were of a lower order of magnitude than the cytotoxic concentrations against Chinese hamster ovary cells, indicating in vitro selectivity for these microorganisms. On the other hand, gold(III) complexes 19 and 20, containing chelating thiosalicylate and salicylate anions, respectively, showed significantly high activity against bacteria E. coli, B. subtilis, and P. aeruginosa, and fungi C. albicans and C. resinae (Dinger and Henderson, 1998).
The gold(III) 1,2-dithiolene cyclometalated complex 21 was tested against a panel of Gram-positive and Gram-negative bacteria and three yeasts of the Candida spp., showing remarkable bacteriostatic activity against S. haemolyticus and S. aureus, with MIC values of 1.56 and 3.13 μg/mL, respectively. However, none of the tested Gram-negative bacteria or fungi were sensitive to this gold(III) complex. The biofilm inhibitory effect if complex 21 was also described in this study against S. aureus and S. haemolyticus, where they highlight 3.125 μg/mL as the minimum concentration to interfere in biofilm formation. However, due to turbidity of the solution, a concentration that fully inhibits biofilm formation was not provided (Pintus et al., 2017).
The gold(III) bis (dithiolene) complex 22 containing an N-ethyl-1,3-thiazoline-2-thiane dithiolate ligand showed significant activity against Gram-positive bacteria S. aureus and fungi C. glabrata and C. albicans but not against Gram-negative E. coli (Fontinha et al., 2020). As in the case of gold(I) complexes, several studies support that thioredoxin reductase is a possible target of these complexes. In addition, complex 22 is stable under biological conditions, being an important characteristic if this compound is ever to be considered as a drug.
More recently, Chakraborty et al. (2021) reported several gold(III) complexes with bidentate N^N ligands, cyclometalated bidentate C^N and terdentate C^N^N scaffolds. Even though those cyclometallated Au(III) complexes do not show any antibacterial activity against E. coli, complexes 23 and 24 inhibited the growth of B. subtilis, S. aureus, and MRSA, with MICs ranging from 3.12 to 25 μM.
These compounds do not affect adenosine triphosphate (ATP) synthesis, or membrane potential or permeability. RNA sequencing revealed that these gold(III) complexes upregulated genes related to metal transport, major facilitator superfamily (MFS)-transporter, genes responsible for arsenic metabolism, oxidoreductases, and proteases, among others. However, they downregulated genes related to cell wall formation, contact dependent growth inhibition, and ABR transporter genes (Chakraborty et al., 2021).
In view of the biological interest of Au(I) complexes with NHC ligands, the corresponding gold (III) analogs were also prepared and investigated as organometallic antibacterial drugs. Au(III) NHC complexes 25–27 showed higher antibacterial activity against the Gram-positive MRSA and E. faecium than against the Gram-negative strains (A. baumannii, E. coli, Klebsiella pneumoniae, and P. aeruginosa), as previously reported for the related Au(I) NHC complexes. All target complexes were efficient inhibitors of bacterial TrxR, suggesting that inhibition of this enzyme might be involved in the mechanism of antibacterial activity (Büssing et al., 2021).
The interest of Au(III) dithiocarbamate complexes as antimicrobial agents dates from the 90 s, when the potential activity against Streptococcus pneumoniae of a series of Au(III) complexes with dithiocarbamates derived from α-amino acids (DL-alanine, DL-valine, L-valine, and DL-leucine) was reported (Criado et al., 1992).
Since the biological activity is strongly related to the coordination sphere of gold, a good strategy to modulate the biological properties of gold complexes would be to alter the ligands attached to the metallic center. This strategy has been widely applied to the development of gold-based anticancer drugs. Bertrand et al. (2015) studied cyclometalated Au(III) complexes with 1,3,5-triazaphosphaadamantane (PTA) and thio-α-D-glucose tetraacetate (GluS) ligands, chosen on account of the properties that could be conferred to the gold complexes. Thus, the PTA ligand was chosen for its good water solubility. As for the GluS ligand, Au(III) binding to thiolates would avoid ligand exchange reactions with biological nucleophiles that would lead to inactivation of the compound. In addition, the presence of the sugar unit would have important implications in molecular recognition phenomena.
Considering these previous studies, the effect of different dithiocarbamate ligands on the therapeutic index of dithiophenylphosphine Au(III) complexes has been extensively investigated (Soto et al., 2019). Thus, a group of (S^C)-cyclometallated gold(III) complexes containing a 1,1-dithio ligand was developed and tested against a wide panel of Gram-positive and Gram-negative bacteria. Among these, complex 28 not only exhibited antibacterial in vitro activity against multidrug resistant microorganisms, especially S. aureus, S. maltophilia, P. aeruginosa, and H. influenzae, but also displayed low cytotoxicity. Complex 28 was able to in inhibit biofilm formation by its addition before the adhesion phase at low concentrations in S. aureus strains. The minimum biofilm inhibitory concentration (MBIC) values ranged between 0.5 to 2 mg/L for both MRSA and MSSA, only two-fold higher than their MIC in planktonic phase. For Gram-negative bacteria, a similar trend was observed for biofilm forming strains of P. aeruginosa, K. pneumoniae, and A. baumannii, which showed MBICs two-fold higher than their corresponding MIC. In addition, 28 was also highly stable under physiological conditions and is expected to maintain its antibacterial activity in vivo in the same way.
In summary, albeit less studied than their Au(I) counterparts, Au(III) complexes exhibit potent antibacterial activities and do not generate bacterial resistance. However, very little attention has been given to the elucidation of their modes of action. The data available indicate that, opposed to Au(I) complexes, Au(III) complexes may not have any effect on reactive oxygen species production, membrane permeability or membrane depolarization (Chakraborty et al., 2021). The low probability of development of mutations also suggest multiple targets for the bactericidal action of Au(III) complexes (Soto et al., 2019). In-depth mechanistic studies are still required to fully elucidate the mode of bactericidal action of Au(III) complexes.
Increasing resistance to antibiotics is one of the more pressing problems in modern medicine. To tackle this, researchers soon turned their attention to gold. During the past decade, gold-based drugs have become an interesting topic in pharmaceutical research and metallodrug chemistry because many of these drugs have shown potent activity against several bacterial strains, including both Gram-positive and -negative organisms. Unfortunately, promising in vitro results are not always correlated with significant in vivo effects. Despite all the efforts, scientists have struggled to develop a practical gold-based antimicrobial therapy.
One of the fundamental reasons for this is the complicated biological chemistry of gold compounds; the ligand exchange behavior of the complexes in gold drugs and the Au(III)/Au(I) redox chemistry must be considered, in addition to the problems of hydrolysis and protein binding. In this regard, future developments in the field of gold-based antibiotics require more knowledge about the mechanisms of their in vivo distribution and/or degradation.
Gold complexes have unique modes of action, which is a plausible explanation for the observed lack of detectable resistance. However, as the mechanisms of antimicrobial metal toxicity remain uncertain, the identification of bacterial targets and uptake pathways are key issues for promoting the clinical application of gold-based antibiotics.
To overcome the present disadvantages of gold-based drug candidates, several strategies are currently being investigated. A new trend, which has also entered the pharmaceutical chemistry of gold complexes, is the use of encapsulating techniques. Accordingly, the biodistribution of gold antibiotics may be improved by inclusion in cyclodextrins (Morgen et al., 2019; Tomasello et al., 2021). Recent studies have also described a potent synergistic microbicidal effect with antibiotics, suggesting that gold compounds may have great application prospects in combination therapy, potentiating the efficacy of clinically used antibiotics (Ratia et al., 2022).
There is clearly much untapped potential of the use of gold complexes for antimicrobial applications. However, for these drug candidates to be effectively and safely used, more research is required. Better understanding of the structure, function, and mechanisms that give gold-based agents higher antibacterial activity and lower toxicity will lead to the design of better gold complexes that are safe and effective for systemic administration. As the chemical properties of gold complexes can be fine-tuned by proper ligand design (Storr et al., 2006), new generations of various gold-based antibiotics that exhibit more effective antibacterial activities can be foreseen.
To conclude, the future is bright for gold-based antibiotics and the coming years will surely see further advances in this exciting research field. Hopefully, a number of gold-based antibiotic drug candidates will be able to reach clinical trials and the market within the next decade and thereby become a solution for the treatment of bacterial infections resistant to antibiotics.
SS: conceptualization. RS and SS: writing-original draft preparation. CR, RS, and SS: writing—review and editing. All authors have read and agreed to the published version of the manuscript.
This work was funded by the Instituto de Salud Carlos III (PI19/00478 to SS), Subdirección General de Redes y Centros de Investigación Cooperativa, Ministerio de Economía y Competitividad, Spanish Network for Research in Infectious Diseases (REIPI RD12/0015/0013 and REIPI RD16/0016/0010 to SS) co-financed by the FEDER program, the European Development Regional Fund “A way to achieve Europe,” operative program Intelligent Growth 2014–2020, and the Principado de Asturias (FICYT AYUD/2021/51542).
The authors declare that the research was conducted in the absence of any commercial or financial relationships that could be construed as a potential conflict of interest.
All claims expressed in this article are solely those of the authors and do not necessarily represent those of their affiliated organizations, or those of the publisher, the editors and the reviewers. Any product that may be evaluated in this article, or claim that may be made by its manufacturer, is not guaranteed or endorsed by the publisher.
We acknowledge support from the Spanish Ministry of Science and Innovation and State Research Agency through the “Centro de Excelencia Severo Ochoa 2019–2023” Program (CEX2018-000806-S) and support from the Generalitat de Catalunya through the CERCA Program.
Antimicrobial Resistance Collaborators (2022). Global burden of bacterial antimicrobial resistance in 2019: a systematic analysis. Lancet 399, 629–655. doi: 10.1016/S0140-6736(21)02724-2720
Barreiro, E., Casas, J. S., Couce, M. D., Saìnchez, A., Seoane, R., and Perez-Esteìvez, A. (2012). Synthesis and antimicrobial activities of gold(I) sulfanylcarboxylates. Gold Bull. 45, 23–34. doi: 10.1007/s13404-011-0040-47
Bertrand, B., Spreckelmeyer, S., Bodio, E., Cocco, F., Picquet, M., Richard, P., et al. (2015). Exploring the potential of gold(III) cyclometallated compounds as cytotoxic agents: variations on the C^N theme. Dalton Trans. 44, 11911–11918. doi: 10.1039/c5dt01023c
Büssing, R., Karge, B., Lippmann, P., Jones, P. G., Broönstrup, M., and Ott, I. (2021). Gold(I) and gold(III) N-heterocyclic carbene complexes as antibacterial agents and inhibitors of bacterial thioredoxin reductase. ChemMedChem 16, 3402–3409. doi: 10.1002/cmdc.202100381
Cassetta, M. I., Marzo, T., Fallani, S., Novelli, A., and Messori, L. (2014). Drug repositioning: auranofin as a prospective antimicrobial agent for the treatment of severe Staphylococcal infections. Biometals 27, 787–791. doi: 10.1007/s10534-014-9743-9746
Chakraborty, P., Oosterhuis, D., Bonsignore, R., Casini, A., Olinga, P., and Scheffers, D. J. (2021). An organogold compound as potential antimicrobial agent against drug-resistant bacteria: initial mechanistic insights. ChemMedChem 16, 3060–3070. doi: 10.1002/cmdc.202100342
Criado, J. J. Lopez-Arias, J. A., Macias, B. Fernandez-Lago, L. R., and Salas, J. M. (1992). Au(III) complexes of tris-dithiocarbamate derivatives of α-amino acids: spectroscopic studies, thermal behaviour and antibacterial activity. Inorg. Chim. Acta 193, 229–235. doi: 10.1016/S0020-1693(00)80357-6
Dinger, M. B., and Henderson, W. (1998). Organogold(III) metallacyclic chemistry. Part 4. Organogold(III) metallacyclic chemistry. Part 4. Synthesis, characterisation, and biological activity of gold(III)-thiosalicylate and -salicylate complexes. J. Organomet. Chem. 560, 233–243. doi: 10.1016/s0022-328x(98)00493-8
Elie, B. T., Levine, C., Ubarretxena-Belandia, I., Varela-Ramírez, A., Aguilera, R. J., Ovalle, R., et al. (2009). Water-soluble (phosphane)gold(I) complexes - aplications as recyclable catalysts in a three-component coupling reaction and as antimicrobial and anticancer agents. Eur. J. Inorg. Chem. 2009, 3421–3430. doi: 10.1002/ejic.200900279
Felix, L. O., Mylonakis, E., and Fuchs, B. B. (2021). Thioredoxin Reductase is a valid target for antimicrobial therapeutic development against gram-positive bacteria. Front. Microbiol. 12:663481. doi: 10.3389/fmicb.2021.663481
Fillat, M. F., Gimeno, M. C., Laguna, A., Latorre, E., Ortego, L., and Villacampa, M. D. (2011). Synthesis, structure and bactericide activity of (aminophosphane)gold(I) thiolate complexes. J. Inor. Chem. 1487–1495. doi: 10.1002/ejic.201001195
Fontinha, D., Sousa, S. A., Morais, T. S., Prudêncio, M., Leitão, J. H., Le Gal, Y., et al. (2020). Gold(III) bis(dithiolene) complexes: from molecular conductors to prospective anticancer, antimicrobial and antiplasmodial agents. Metallomics 12, 974–987. doi: 10.1039/d0mt00064g
Frik, M., Jiménez, J., Gracia, I., Falvello, L. R., Abi-Habib, S., Suriel, K., et al. (2012). Luminescent di- and polynuclear organometallic gold(I)-metal (Au2, {Au2Ag}n and {Au2Cu}n) compounds containing bidentate phosphanes as active antimicrobial agents. Chem. Eur. J. 18, 3659–3674. doi: 10.1002/chem.201103145
Fuchs, B. B., RajaMuthiah, R., Souza, A. C., Eatemadpour, S., Rossoni, R. D., Santos, D. A., et al. (2016). Inhibition of bacterial and fungal pathogens by the orphaned drug auranofin. Future Med. Chem. 8, 117–132. doi: 10.4155/fmc.15.182
Glasner, C., Albiger, B., Buist, G., Tambić-Andrasević, A., Canton, R., and Carmeli, Y. (2013). Carbapenemase-producing Enterobacteriaceae in Europe: a survey among national experts from 39 countries, February 2013. Eurosurveillance 18:28. doi: 10.2807/1560-7917.es2013.18.28.20525
Glišić, B. D., and Djuran, M. I. (2014). Gold complexes as antimicrobial agents: an overview of different biological activities in relation to the oxidation state of the gold ion and the ligand structure. Dalton Trans. 43, 5950–5969. doi: 10.1039/c4dt00022f
Higby, G. J. (1982). Gold in medicine: a review of its use in the West before 1900. Gold Bull. 15, 130–140. doi: 10.1007/BF03214618
Hristova, Y. R., Kemper, B., and Besenius, P. (2013). Water-soluble Au(I) complexes, their synthesis and applications. Tetrahedron 69, 10525–10533. doi: 10.1016/j.tet.2013.09.096
Mora, M., Gimeno, M. C., and Visbal, R. (2019). Recent advances in gold-NHC complexes with biological properties. Chem. Soc. Rev. 48, 447–462. doi: 10.1039/C8CS00570B
Morgen, M., Fabrowski, P., Amtmann, E., Gunkel, N., and Miller, A. K. (2019). Inclusion complexes of gold(I)-dithiocarbamates with β-Cyclodextrin: a journey from drug repurposing towards drug discovery. Chemistry 27, 12156–12165. doi: 10.1002/chem.202101366
Nardón, C., Boscutti, G., and Fregona, D. (2014). Beyond platinums: gold complexes as anticancer agents. Anticancer. Res. 34, 487–492.
Nomiya, K., Noguchi, R., Ohsawa, K., Tsuda, K., and Oda, M. (2000). Synthesis, crystal structure and antimicrobial activities of two isomeric gold(I) complexes with nitrogen-containing heterocycle and triphenylphosphine ligands, [Au(L)(PPh3)] (HLspyrazole and imidazole). J. Inorg. Biochem. 78, 363–370. doi: 10.1016/s0162-0134(00)00065-69
Novelli, F., Recine, M., Sparatore, F., and Juliano, C. (1999). Gold(I) complexes as antimicrobial agents. Farmaco 54, 232–236. doi: 10.1016/s0014-827x(99)00019-11
Özdemir, I., Denizci, A., Tansel, Öztürk, H., and ÇetinkayaS, B. (2004). Synthetic and antimicrobial studies on new gold(I) complexes of imidazolidin-2-ylidenes. Bioorganometal Chem. 18, 318–322. doi: 10.1002/aoc.668
Pacheco, E. A., Tiekink, E. R. T., and Whitehouse, M. W. (2009). In Gold Chemistry. Weinheim: Wiley-VCH Verlag GmbH & Co.
Parish, R. V. (1999). Biologically-Active Gold(III) complexes. Met Based Drugs 6, 271–276. doi: 10.1155/MBD.1999.271
Parish, R. V., Howe, B. P., Wright, J. P., Mack, J., Pritchard, R. G., Buckley, R. G., et al. (1996). Chemical and Biological Studies of Dichloro(2-((dimethylamino)methyl)phenyl)gold(III). Inorg. Chem. 35, 1659–1666. doi: 10.1021/ic950343b
Pintus, A., Aragoni, M. C., Cinellu, M. A., Maiore, L., Isaia, F., Lippolis, V., et al. (2017). [Au(pyb-H)(mnt)]: a novel gold(III) 1,2-dithiolene cyclometalated complex with antimicrobial activity (pyb-H=C-deprotonated 2-benzylpyridine; mnt=1,2-dicyanoethene-1,2-dithiolate). J. Inorg. Biochem. 170, 188–194. doi: 10.1016/j.jinorgbio.2017.02.015
Powers, J. H. (2004). Antimicrobial drug development-the past, the present, and the future. Clin. Microbiol. Infect 10, 23–31. doi: 10.1111/j.1465-0691.2004.1007.x
Ratia, C., Cepas, V., Soengas, R., Navarro, Y., Velasco-de-Andrés, M., Iglesias, M. J., et al. (2022). A C∧S-cyclometallated gold(III) complex as a novel antibacterial candidate against drug-resistant bacteria. Front. Microbiol. 13:815622. doi: 10.3389/fmicb.2022.815622
Ray, S., Mohan, R., Singh, J. K., Samantaray, M. K., Shaikh, M. M., Panda, D., et al. (2007). Anticancer and antimicrobial metallopharmaceutical agents based on palladium, gold, and silver N-heterocyclic carbene complexes. J. Am. Chem. Soc. 129, 15042–15053. doi: 10.1021/ja075889z
Roder, C., and Thomson, M. J. (2015). Auranofin: repurposing an old drug for a golden new age. Drugs R. D. 15, 13–20. doi: 10.1007/s40268-015-0083-y
Saga, T., and Yamaguchi, K. (2009). History of antimicrobial agents and resistant bacteria. Japan Med. Assoc. J. 52, 103–108.
Samanta, T., Roymahapatra, G., Porto, W. F., Seth, S., Ghorai, S., Saha, S., et al. (2013). N, N’-Olefin functionalized bis-imidazolium gold(I) salt is an efficient candidate to control keratitis-associated eye infection. PLoS One 8:e58346. doi: 10.1371/journal.pone.0058346
Schmidt, C., Karge, B., Misgeld, R., Prokop, A., Franke, R., Brönstrup, M., et al. (2017). Gold(I) NHC complexes: antiproliferative activity, cellular uptake, inhibition of mammalian and bacterial thioredoxin reductases, and Gram-positive directed antibacterial effects. Chem. Eur. J. 23, 1869–1880. doi: 10.1002/chem.201604512
Soto, S., Ratia, C., Cepas, V., López, Y., López-Ortiz, F., Valdes, M. J., et al. (2019). A gold(III) complex, a conjugate of the gold(III) complex, a pharmaceutical composition comprising the gold(III) complex and uses and a process for preparing the gold(III) complex. WO 2019211222:A120191107.
Spellberg, B., Powers, J. H., Brass, E. P., Miller, L. G., and Edwards, J. E. Jr. (2004). Trends in antimicrobial drug development: implications for the future. Clin. Infect. Dis. 38, 1279–1286. doi: 10.1086/420937
Storr, T., Thompson, K. H., and Orvig, C. (2006). Design of targeting ligands in medicinal inorganic chemistry. Chem. Soc. Rev. 35, 534–544. doi: 10.1039/B514859F
Thangamani, S., Mohammad, H., Abushahba, M. F., Sobreira, T. J., Hedrick, V. E., Paul, L. N., et al. (2016). Antibacterial activity and mechanism of action of auranofin against multi-drug resistant bacterial pathogens. Sci. Rep. 6:22571. doi: 10.1038/srep22571
Tiekink, E. R. (2002). Gold derivatives for the treatment of cancer. Crit. Rev. Oncol. Hematol. 42, 225–248. doi: 10.1016/s1040-8428(01)00216-215
Tomasello, M. F., Nardon, C., Lanza, V., Di Natale, G., Pettenuzzo, N., Salmaso, S., et al. (2021). New comprehensive studies of a gold(III) dithiocarbamate complex with proven anticancer properties: aqueous dissolution with cyclodextrins, pharmacokinetics and upstream inhibition of the ubiquitin-proteasome pathway. Eur. J. Med. Chem. 138, 115–127. doi: 10.1016/j.ejmech.2017.06.013
Torres, N. S., Abercrombie, J. J., Srinivasan, A., Lopez-Ribot, J. L., Ramasubramanian, A. K., and Leung, K. P. (2016). Screening a commercial library of pharmacologically active small molecules against Staphylococcus aureus biofilms. Antimicrob. Agents Chemother. 60, 5663–5672. doi: 10.1128/AAC.00377-16
Torres, N. S., Montelongo-Jauregui, D., Abercrombie, J. J., Srinivasan, A., Lopez-Ribot, J. L., Ramasubramanian, A. K., et al. (2018). Antimicrobial and antibiofilm activity of synergistic combinations of a commercially available small compound library with colistin against Pseudomonas aeruginosa. Front. Microbiol. 9:2541. doi: 10.3389/fmicb.2018.02541
Keywords: gold(I) complexes, gold(III) complexes, antibacterial, mechanism of action, toxicity
Citation: Ratia C, Soengas RG and Soto SM (2022) Gold-Derived Molecules as New Antimicrobial Agents. Front. Microbiol. 13:846959. doi: 10.3389/fmicb.2022.846959
Received: 31 December 2021; Accepted: 21 February 2022;
Published: 23 March 2022.
Edited by:
Axel Cloeckaert, Institut National de Recherche pour l’Agriculture, L’Alimentation et l’Environnement (INRAE), FranceReviewed by:
Lara Massai, University of Florence, ItalyCopyright © 2022 Ratia, Soengas and Soto. This is an open-access article distributed under the terms of the Creative Commons Attribution License (CC BY). The use, distribution or reproduction in other forums is permitted, provided the original author(s) and the copyright owner(s) are credited and that the original publication in this journal is cited, in accordance with accepted academic practice. No use, distribution or reproduction is permitted which does not comply with these terms.
*Correspondence: Sara M. Soto, c2FyYS5zb3RvQGlzZ2xvYmFsLm9yZw==
Disclaimer: All claims expressed in this article are solely those of the authors and do not necessarily represent those of their affiliated organizations, or those of the publisher, the editors and the reviewers. Any product that may be evaluated in this article or claim that may be made by its manufacturer is not guaranteed or endorsed by the publisher.
Research integrity at Frontiers
Learn more about the work of our research integrity team to safeguard the quality of each article we publish.