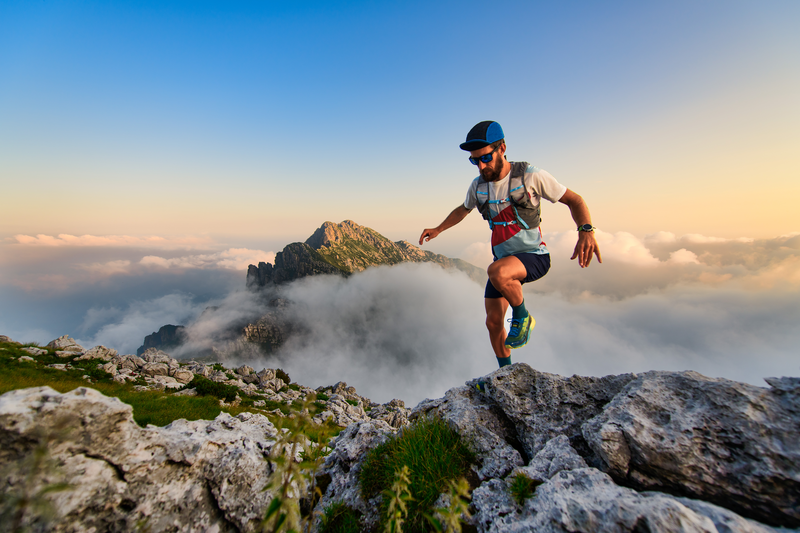
95% of researchers rate our articles as excellent or good
Learn more about the work of our research integrity team to safeguard the quality of each article we publish.
Find out more
ORIGINAL RESEARCH article
Front. Microbiol. , 17 March 2022
Sec. Virology
Volume 13 - 2022 | https://doi.org/10.3389/fmicb.2022.846770
This article is part of the Research Topic The Viral Evasion of Antiviral Innate Immunity View all 71 articles
African swine fever (ASF), caused by the African swine fever virus (ASFV), is an acute, deadly, infectious disease of domestic pigs and wild boars and has a tremendous negative socioeconomic impact on the swine industry. ASF is a notifiable disease to the World Organization for Animal Health (OIE). Currently, no effective vaccine or treatment against ASF is available. Early detection and rapid diagnosis are potentially significant to control ASF spread with the emerging ASFV mutant strains and non-classical symptoms. In this study, we developed a real-time recombinase-aid amplification (RAA) assay to detect the ASFV genome rapidly. Thirty samples were detected using commercial lysis buffer for DNA extraction and equipped with a portable testing instrument. The results showed that the sensitivity of RAA was 103 copies per reaction at 95% probability in 9 min at 39°C. The method was universally specific for three strains of ASFV, and there was no cross-reaction with other pathogens, including foot-and-mouth disease virus (FMDV), classical swine fever virus (CSFV), porcine reproductive and respiratory syndrome virus (PRRSV), porcine circovirus 2 (PCV2), pseudorabies virus (PRV), and porcine parvovirus (PPV). The coefficient of variation (C.V) of repetitive experiments was 0%, and the coincidence rate was 100% compared to the real-time qPCR. 123 field samples were detected by the real-time RAA assay, and the results showed that the clinical coincidence rate of the real-time RAA assay was 98% compared to the real-time qPCR assay. The advantages of this method were as follows: the extraction of DNA can be performed on site, the DNA template is directly used, a small battery-powered instrument is easily available, and the on-site diagnostic process is finished within an hour. These suggest that this assay could be used to detect different genotypes of ASFV and play a vital role in the control of ASF.
African swine fever (ASF) is caused by the African swine fever virus (ASFV) and is an acute, contagious, and hemorrhagic infectious disease, with a mortality rate reaching up to 100% (Galindo and Alonso, 2017). ASFV is a double-stranded DNA virus with a complex molecular structure and is the only member of the Asfarviridae family (Galindo and Alonso, 2017). ASF is a notifiable disease to the World Organization for Animal Health (OIE) due to its serious impact on pig production and related industries. The clinical symptoms include high fever, depression, anorexia, cyanosis, vomiting, nose bleeding, and bloody diarrhea, similar to classical swine fever (CSF) and porcine reproductive and respiratory syndrome (PRRS) and other swine diseases, which indicate that laboratory diagnosis is required (Blome et al., 2013). Twenty-four genotypes were presented based on sequence variation in the C-terminal region of the B646L gene that mainly encodes capsid protein p72, which is the most dominant structural component of the virion constituting about 31–33% of the total mass of the virion and is the major antigen detected in ASFV-infected pigs (Bastos et al., 2003; Boshoff et al., 2007; Revilla et al., 2018; Liu Q. et al., 2019). A previous study showed that the p72 gene nucleotide sequences of MAL/19/Karonga/1–4 showed 100% nucleotide identity with ASFV strains previously described in Tanzania, Zambia, Georgia, China, Vietnam, Estonia, Moldova, Czechia, Belgium, and Poland, which suggests that the p72 gene is relatively conserved (Hakizimana et al., 2020). The primers used for ASFV detection were designed based on the p72 gene.
At present, no efficient vaccine or treatment against ASF is available, even though much time, energy, and money have been invested in many countries. Meanwhile, mutant strains of ASFV have been emerging through mutations and recombinations in the genome in order to survive in different environments during the ASFV epidemic process, which hinder the early diagnosis of ASF (Sun et al., 2021; Zhang et al., 2021). Infected pigs have developed atypical clinical symptoms, such as reduced mortality, highly concealed with the long-term prevalence and the spread of ASF in China, which bring challenges to its prevention and control. Therefore, reliable early diagnosis technology and epidemiological monitoring are essential for preventing, controlling, and purifying ASF.
This study constructed a real-time recombinase-aid amplification (RAA) assay for the rapid, on-site, and primary detection of ASFV genomic DNA. Thirty samples were detected, including 12 artificially spiked samples of ASFV, 2 samples of foot-and-mouth disease virus (FMDV), 1 sample of classical swine fever virus (CSFV), 1 sample of porcine reproductive and respiratory syndrome virus (PRRSV), and 14 ASFV-free samples, which were confirmed by the real-time quantitative PCR (qPCR) previously reported (Gallina et al., 2006). The results showed that the sensitivity of the real-time RAA assay was at 103 copies per reaction at 95% probability in 9 min at 39°C. The method was universally specific for three strains of ASFV, and there was no cross-reaction with other pathogens, including FMDV, CSFV, PRRSV, porcine circovirus 2 (PCV2), pseudorabies virus (PRV), and porcine parvovirus (PPV). The coefficient of variation (CV) of repetitive experiments was 0%, and the coincidence rate was 100% compared to real-time qPCR. The real-time RAA assay was then evaluated on 123 field samples compared to the real-time qPCR assay. Fifty-one samples were determined to be positive by the real-time RAA assay, and 52 samples were positive by the real-time qPCR assay (CT values ranging from 20 to 30). The clinical coincidence rate of the real-time RAA assay was 98% compared to the real-time qPCR assay.
Our results demonstrated that the real-time RAA assay, equipped with portable instruments, provided a specific and sensitive platform for the rapid and reliable detection of the ASFV genome. The advantages of this method included the following: the extraction of DNA can be performed in a short time on-site, the template is directly used, a small battery-powered instrument is easily available, and the on-site detection process is finished within an hour. All these suggest that this approach could be used to detect different genotypes of ASFV and play a vital role in the surveillance and the control of ASF.
In this study, 30 samples were used, as described below: the p72 gene of three strains of ASFV [E70 strain (Spanish), Anhui XCGQ strain, and Georgia 2007/1 strain] was synthesized by the Wuhan GeneCreate Biological Engineering Co., Ltd., and then mixed with blood (n = 1), serum (n = 2), and lymphatic nodes (n = 1), respectively, and 12 artificially spiked samples. The other samples were preserved in our laboratory: FMDV (FMDV/O, FMDV/A), CSFV, and PRRSV, one sample of each virus. Fourteen ASFV-free samples of the liver (n = 1), muscles (n = 2), lymphatic tissues (n = 5), serum (n = 3), and blood (n = 3) were collected from healthy swine, and these samples were confirmed using the real-time qPCR previously reported (Gallina et al., 2006). One hundred twenty-three field samples were preserved in our laboratory, including blood (n = 15), heart (n = 10), liver (n = 13), spleen (n = 9), lung (n = 18), kidney (n = 14), inguinal lymph nodes (n = 13), mesenteric lymph nodes (n = 14), and hepatic and gastric lymph nodes (n = 17).
For liquid samples, 40 μl blood or serum was transferred into a 1.5-ml centrifuge tube and added with 100 μl lysis buffer B1, mixed fully, and then added with 200 μl lysis buffer B2. For tissue samples, 5–10 mg tissues was lysed with 100 μl lysis buffer B1, manually ground into pieces with a tissue grinder, then added with 200 μl lysis buffer B2, and vortexed to blend completely. Supernatant of 100–150 μl was transferred into another clean centrifuge tube after centrifuging at 12,000 rpm for 2 min and stored at −80°C until use.
Real-time RAA-specific primers and probes were designed according to the p72 gene sequence of the ASFV-SY18 strain (GenBank: MH713612.1) separated in 2018 in China. Meanwhile, the p72 gene sequence of the ASFV-SY18 strain was aligned with homologous sequences of the other ASFV strains (GenBank: LR536725.1, MK333181.1, MK333180.1, MH910496.1, MH910495.1, MH713612.1, MK128995.1, MH681419.1, LS478113.1, KJ195685.1, FR682468.1, AY578707.1, AY578702.1, KX354450.1, KM102979.1, KP055815.1, U18466.2, AM712240.1, EF121428.1, L76727.1, and EF121429.1) to determine the conserved regions of the p72 gene. Primers and probes were designed aiming to detect different genotypes of ASFV. All primers and probes were synthesized by Sangon Biotech (Shanghai, China). The probes were synthesized with an inverse arrangement of fluorophore [6-carboxyfluorescein (FAM)], quencher [black hole quencher 1 (BHQ-1)], spacer [tetrahydrofuran spacer (THF)], and block elongation [phosphate(P)]. Information on the primers and probes is listed in Table 1.
Table 1. Primers and probes used for the real-time recombinase-aid amplification (RAA) assay in this study.
The ASFV p72 gene segments (887 bp) were synthesized by Sangon Biotech (Shanghai, China) based on the ASFV genomic sequence (GenBank: MH713612.1) and were inserted into a pUC57 vector to generate standard plasmid, designated as pASFV/RAA. The pASFV/RAA plasmid was extracted using the DNeasy Blood & Tissue Kit (50) (Qiagen, Germantown, MD, United States), and the DNA concentration was measured by Nanovue (GE Life Sciences, Chicago, IL, United States). The DNA copy number were calculated using the following equation (Xia et al., 2014): DNA copy number = (M × 6.02 × 1023 × 10–9)/(n × 660). The DNA standard was then aliquoted and stored at −80°C until use.
The RAA reactions were performed in a 50-μl volume, including a centrifuge tube of RAA enzyme dry powder, reaction A of RAA 45.5 μl, DNA template 2 μl, and reaction B of RAA 2.5 μl. The detailed protocol is as follows: 45.5 μl RAA reaction A was transferred into the tube with RAA enzyme dry powder and overturned to mix thoroughly. Of the DNA template, 2 μl was added into a centrifuge tube, and then 2.5 μl RAA reaction B was added, the tube lip covered and inverted upside down five to six times to mix the reaction system thoroughly, and then centrifuged for 5–10 s. The assay was performed on a portable fluorescence quantitative PCR instrument (Suzhou Yarui Biotechnology Co., Ltd., Suzhou, China) for 1 cycle at 39°C for 40 s and 40 cycles at 39°C for 30 s. The reaction was completed within 20 min. The sample was positive if the logarithmic amplification curve revealed a Ct value ≤35; it was judged as a suspicious sample if 3539 or no value. For interpretation, a positive (+) result is when the ASFV genome DNA was detected in the sample and negative (−) when the ASFV genome DNA was not detected.
The primers and probes in the real-time qPCR assay were based on a previous report: forward primer, 5′-CCT CGGCGAGCGCTTTATCAC-3′; reverse primer, 5′-GGAAACT CATTCACCAAATCCTT-3′; probe, 5′-CGATGCAAGCTTTAT-3′ (Zsak et al., 2005). All primers and probes were synthesized by Sangon Biotech (Shanghai, China). The assay was performed in a LightCycler 480 II real-time fluorescent quantitative PCR instrument (Roche, Indianapolis, IN, United States) according to the a previously described method (Gallina et al., 2006). The reactions were conducted according to the manufacturer’s instructions in the one-step PrimeScript RT-PCR Kit (TaKaRa, Shiga, Japan). The total reaction in a 20-μl reaction volume contained 10 μl of 2 × one-step RT-PCR buffer III, 0.4 μl of TaKaRa Ex Taq HS, 0.4 μl of PrimeScript RT Enzyme Mix II, 0.4 μl of PCR forward primer, 0.4 μl of PCR reverse primer, 0.8 μl of probes, 2 μl of DNA template, and 5.6 μl RNase-free ddH2O. Cycling proceeded at 42°C for 5 min and 95°C for 10 s, followed by 40 cycles of 95°C for 5 s and 60°C for 20 s. A melting curve analysis was performed using specific melting temperatures to verify the uniqueness of the amplified product. The data were analyzed using the LC480 System software.
Data were provided as the mean and standard deviation (SD). Statistical analysis was performed using PRISM 8.0.2 software (GraphPad Software, San Diego, CA, United States).
To determine the most efficient primer pair for the real-time RAA assay, three forward primers (ASFV Fe1–Fe3) and reverse primers (ASFV Re1–Re3) based on the p72 gene of ASFV were selected (Table 1). Nine different combinations of the primers (i.e., Fe1/Re1, Fe1/Re2, Fe1/Re3, Fe2/Re1, Fe2/Re2 Fe2/Re3, Fe3/Re1, Fe3/Re2, and Fe3/Re3) were detected with the ASFV Pe probe on 104 genome copies of standard DNA. The results showed that the primer pair ASFV Fe2/Re2 yielded the highest amplification efficiency (Figure 1). Therefore, this primer pair was employed to validate the real-time RAA assay.
Figure 1. Optimal primer and probe combinations for the real-time recombinase-aid amplification (RAA) assay. Three forward primers (ASFV Fe1–Fe3), three reverse primers (ASFV Re1–Re3), and one probe (ASFV Pe) were used to select the best combination. NC, negative control.
Serial dilution of the pASFV/RAA plasmid was performed, ranging from 101–106 genome copies per reaction, and then tested using the real-time RAA assay to determine the detection limit. Every run was repeated eight times. The threshold time was plotted against log (detected molecules), and a semi-log regression was calculated using PRISM 8.0.2 software (GraphPad Software). As shown in Figure 2, the dynamic detection range of the assay spans six logs ranging from 6 to 1 log copy per reaction, with the corresponding threshold time ranging from 5 min at 106 copies per reaction to 9 min at 103 copies per reaction. These results indicate that the real-time RAA assay has a wide dynamic range for detecting ASFV genome DNA (Figures 2A,B).
Figure 2. Sensitivity of the real-time recombinase-aid amplification (RAA) assay. (A) Amplification curve of the real-time RAA assay over time using a dilution range of 106–101 copies/reaction of pASFV/RAA. (B) Reproducibility of the real-time RAA assay. The threshold time is represented as the mean ± standard deviation (SD). The standard regression line was generated based on three datasets. NC, negative control.
To further evaluate the specificity of the real-time RAA assay, ASFV was evaluated among other detections of swine disease pathogens, including CSFV, PCV2, PRRSV, PRV, PPV, and FMDV. The results showed that no cross-reaction was observed with other viruses, and ASFV strains could be detected by the developed real-time RAA assay (Table 2).
Thirty samples were collected to test the performance of the real-time RAA assay, including 12 artificially spiked-samples of ASFV, 2 FMDV samples (a FMDV/O cellular sample and a FMDV/A lymphatic sample), one CSFV sample, one PRRSV sample and 14 ASFV-free samples. Thirty samples were detected by the real-time RAA assay with three repetitions. The results showed that the CV of repetitive experiments was 0% in the intra- and inter-assays, suggesting that the repeatability was good (Tables 3, 4). Meanwhile, 30 samples were used to perform coincidence testing by the real-time RAA assay. The results showed that the coincidence rate was 100% compared to real-time qPCR (Table 5). Taken together, the real-time RAA assay was specific for detecting the ASFV genome, and the sensitivity reached 103 copies per reaction. The CV of repetitive experiments was 0% in the inter- and intra-assays, and the coincidence rate of the real-time RAA assay was 100% compared to the real-time qPCR assay, suggesting that this approach could be used to detect different genotypes of ASFV and play a vital role in its control.
Table 3. Results of repetitive experiments with the real-time recombinase-aid amplification (RAA) assay.
Table 4. Statistical results of repeatability with the real-time recombinase-aid amplification (RAA) assay.
Table 5. Coincidence rate between the real-time recombinase-aid amplification (RAA) assay and real-time quantitative PCR (qPCR).
To explore the clinical performance of the real-time RAA assay, 123 field samples preserved in our laboratory were used, including blood, heart, liver, spleen, lung, kidney, inguinal lymph nodes, mesenteric lymph nodes, and hepatic and gastric lymph nodes. The performance of the real-time RAA assay was compared to that of the real-time qPCR assay. Fifty-one samples were determined to be positive by the real-time RAA assay, while 52 samples were positive by the real-time qPCR assay (CT values ranging from 20 to 30). The clinical coincidence rate of the real-time RAA assay was 98% compared to the real-time qPCR assay (Table 6).
Table 6. Comparison of the real-time recombinase-aid amplification (RAA) assay with the real-time quantitative PCR (qPCR) assay on clinical samples.
African swine fever is a deadly infectious disease that leads to an acute hemorrhagic fever in domestic and wild pigs, with a mortality rate of up to 100%. Presently, it poses a major concern for the global swine industry due to trans-continent transmission of ASF from Africa to Europe and Asia, particularly its introduction and dramatic spread in 2018 in China, which has half of the world’s pig population (Dixon et al., 2019). At present, the characteristics of attenuated strain infection have been emerging, such as the epidemic trend slowing down, low mortality of infection in pigs, and the clinical symptoms not being obvious. ASFV variant strains have been found in different regions in China since 2020. The genome sequences of isolated strains vary, including nucleotide mutations, insertions, deletions, or substitutions. Among them, the mutation frequency of the CD2v gene was higher than in other genes, leading to the loss of the red blood cell adsorption characteristics of some strains, weakened virulence, and minor clinical symptoms in infected animals, which bring challenges to ASF prevention and control (Gao et al., 2018; Song et al., 2021). Hence, the development of a rapid diagnostic and accurate detection technology for ASFV is urgent.
Most diagnostic methods based on qPCR require a long sample preparation time, analysis, and expensive instrument, which are not suitable for rapid detection on-site. Serological detection techniques, such as ELISA antibody testing, are suitable for population testing. However, the production of antibodies is delayed. It is difficult to screen pigs through antibody testing in the incubation period. Meanwhile, there are certain deficiencies in the sensitivity and accuracy. Hence, it is essential to develop an early detection and diagnostic method that is sensitive, specific, and suitable on-site with the persistent spread of ASF. This study developed a real-time RAA assay to detect ASFV genomic DNA. The results of 12 artificially spiked samples of ASFV showed that the sensitivity was 103 per reaction, the specificity was 100% compared to real-time qPCR, and no cross-reaction with other pathogens. For the 123 field samples evaluated using the clinical coincidence rate of the real-time RAA assay was 98% compared to the real-time qPCR assay. The entire process was completed within an hour, coupled with a commercial lysis buffer for DNA extraction and a portable detection device suitable for early detection and diagnosis on-site. It has been shown that, among the developed simple and rapid detection methods for ASFV, the loop-mediated isothermal amplification (LAMP) assay can determine at least 330 copies in 75 min (James et al., 2010), polymerase cross-linking spiral reaction (PCLSR) can reach up to 720 copies/μl within 45 min (Wozniakowski et al., 2017), and cross-priming amplification (CPA) can detect 200 copies within 60 min (Gao et al., 2018). The chimeric DNA/LNA-based biosensor processed up to 40 samples (one sample at a time, per analysis of about 5 min), and the limit of detection was 178 copies/μl (Biagetti et al., 2018). The pen-side molecular diagnostic UPL (Universal ProbeLibrary) assay based on the qPCR technique required at least 35 min (Liu L. et al., 2019). CRISPR-Cas12a for ASFV detection was developed, and the sensitivity was about 10 times higher (1.16 copies/μl) than that of the commercial quantitative PCR kit or the OIE-recommended qPCR, and the entire process was finished within 2 h. However, this method has a certain limit for on-site detection (Wang et al., 2020). The sensitivity of the time-resolved fluorescence immunoassay (TRFIA) for ASFV antigen detection was 0.015 ng/ml. The protocol time was only 45 min, which is suitable for the laboratory diagnosis of ASFV infection (Chen et al., 2021). Together, the above-mentioned methods are time-consuming and unsuitable for rapid detection on-site.
However, the recombinase-based detection method shortens the reaction time from 45–120 min for LAMP, PCLSR, CPA, and PCR to 10–30 min and maintains the detection limit of qPCR. Recombinase polymerase amplification (RPA) is an isothermal nucleic acid amplification technology used to detect nucleic acid instead of PCR without an expensive thermocycler, which has been successfully applied for the detection of pathogens, such as Ebola virus, FMDV, avian influenza virus (AIV), and Brucella (Zhai et al., 2020). Similarly, an RAA assay combined with a portable instrument detected as few as 10 copies per reaction within 20 min, and DNA extraction was performed via a commercial kit (Wang et al., 2021). A directly visualized recombinase polymerase amplification–SYBR Green I method was developed with a detection limit of 103 copies/μl of ASFV in 15 min at 35°C, which can be used as a robust tool for the detection of ASFV genomic DNA (Zhang et al., 2020). PRA combined with lateral flow dipstick (LFD) was developed. This assay was accomplished within 10 min at 36–44°C, and the limit of detection was 102 copies per reaction, providing an efficient method for ASFV detection (Zhai et al., 2020). The combination of RPA and RAA yielded 93.4 and 53.6 copies per reaction within 16 min, respectively. However, DNA extraction was performed using an instrument, and the samples required pretreatment (Fan et al., 2020).
In recent years, the RAA/RPA method has been applied for the detection of many pathogens, such as Vibrio harveyi, PCV2, enterovirus 71 (EV71), and coxsackievirus A16 (CA16) (Wang et al., 2017; Li et al., 2019; Pang et al., 2019). More and more detection and diagnostic methods for ASFV are emerging with the persistent infection of ASFV and the non-classical symptoms. RAA/RPA seems to have more potential as an early diagnostic method for ASFV because its limit detection is the same as that of the OIE-recommend qPCR. The entire process is completed on-site within a short time via a portable testing device. Hence, it still requires extensive validation and optimization to provide a rapid and reliable strategy for early detection, such as for disposal, movement control, disinfection, and contamination to the environment.
Altogether, our data demonstrated that the real-time RAA, coupled with a portable detection device and a commercial lysis buffer for DNA extraction, can be a sensitive, specific, rapid, and reliable tool for ASFV genomic DNA detection on-site, promoting the control and surveillance of ASF in the future.
The raw data supporting the conclusions of this article will be made available by the authors, without undue reservation.
YY, ZDZ, YML, YJL, and YSW designed the experiments and wrote and revised the manuscript. YSW performed the experiments. YY and XQ analyzed the data. YR, YSW, ML, ZXZ, and RZ prepared and performed experiments on the clinical samples. All authors contributed to the article and approved the submitted version.
The study was supported by the Southwest Minzu University Research Startup Fund (2021115 and 16011211013), Gansu Province Science and Technology Planning Project (20YF3WA008), Special Projects of the Natural Science Foundation of China (31941002), Self-selected topics for basic scientific research business expenses of Lanzhou Veterinary Research Institute (1610312021013), and Key tasks of Lanzhou Veterinary Research Institute (CAAS-ASTIP-JBGS-20210401).
The authors declare that the research was conducted in the absence of any commercial or financial relationships that could be construed as a potential conflict of interest.
All claims expressed in this article are solely those of the authors and do not necessarily represent those of their affiliated organizations, or those of the publisher, the editors and the reviewers. Any product that may be evaluated in this article, or claim that may be made by its manufacturer, is not guaranteed or endorsed by the publisher.
Bastos, A. D., Penrith, M. L., Cruciere, C., Edrich, J. L., Hutchings, G., Roger, F., et al. (2003). Genotyping field strains of African swine fever virus by partial p72 gene characterisation. Arch. Virol. 148, 693–706. doi: 10.1007/s00705-002-0946-8
Biagetti, M., Cuccioloni, M., Bonfili, L., Cecarini, V., Sebastiani, C., Curcio, L., et al. (2018). Chimeric DNA/LNA-based biosensor for the rapid detection of African swine fever virus. Talanta 184, 35–41. doi: 10.1016/j.talanta.2018.02.095
Blome, S., Gabriel, C., and Beer, M. (2013). Pathogenesis of African swine fever in domestic pigs and European wild boar. Virus Res. 173, 122–130. doi: 10.1016/j.virusres.2012.10.026
Boshoff, C. I., Bastos, A. D. S., Gerber, L. J., and Vosloo, W. (2007). Genetic characterisation of African swine fever viruses from outbreaks in southern Africa (1973–1999). Vet. Microbiol. 121, 45–55. doi: 10.1016/j.vetmic.2006.11.007
Chen, C., Lai, H., Liang, H., He, Y., Guo, G., and Li, L. (2021). A new method for detection African swine fever virus: time-resolved fluorescence immunoassay. J. Fluoresc. 31, 1291–1296. doi: 10.1007/s10895-021-02754-9
Dixon, L. K., Sun, H., and Roberts, H. (2019). African swine fever. Antiviral Res. 165, 34–41. doi: 10.1016/j.antiviral.2019.02.018
Fan, X., Li, L., Zhao, Y., Liu, Y., Liu, C., Wang, Q., et al. (2020). Clinical validation of two recombinase-based isothermal amplification assays (RPA/RAA) for the rapid detection of African swine fever virus. Front. Microbiol. 11:1696. doi: 10.3389/fmicb.2020.01696
Galindo, I., and Alonso, C. (2017). African swine fever virus: a review. Viruses 9:103. doi: 10.3390/v9050103
Gallina, L., Dal Pozzo, F., Mc Innes, C. J., Cardeti, G., Guercio, A., Battilani, M., et al. (2006). A real time PCR assay for the detection and quantification of orf virus. J. Virol. Methods 134, 140–145. doi: 10.1016/j.jviromet.2005.12.014
Gao, Y., Meng, X. Y., Zhang, H. W., Luo, Y. Z., Sun, Y., Li, Y. F., et al. (2018). Cross-priming amplification combined with immunochromatographic strip for rapid on-site detection of African swine fever virus. Sens. Actuators B Chem. 274, 304–309. doi: 10.1016/j.snb.2018.07.164
Hakizimana, J. N., Kamwendo, G., Chulu, J. L. C., Kamana, O., Nauwynck, H. J., and Misinzo, G. (2020). Genetic profile of African swine fever virus responsible for the 2019 outbreak in northern Malawi. BMC Vet. Res. 16:316. doi: 10.1186/s12917-020-02536-8
James, H. E., Ebert, K., McGonigle, R., Reid, S. M., Boonham, N., Tomlinson, J. A., et al. (2010). Detection of African swine fever virus by loop-mediated isothermal amplification. J. Virol. Methods 164, 68–74. doi: 10.1016/j.jviromet.2009.11.034
Li, X. N., Shen, X. X., Li, M. H., Qi, J. J., Wang, R. H., Duan, Q. X., et al. (2019). Applicability of duplex real time and lateral flow strip reverse-transcription recombinase aided amplification assays for the detection of enterovirus 71 and coxsackievirus A16. Virol. J. 16:166. doi: 10.1186/s12985-019-1264-z
Liu, Q., Ma, B., Qian, N., Zhang, F., Tan, X., Lei, J., et al. (2019). Structure of the African swine fever virus major capsid protein p72. Cell Res. 29, 953–955. doi: 10.1038/s41422-019-0232-x
Liu, L., Atim, S., LeBlanc, N., Rauh, R., Esau, M., Chenais, E., et al. (2019). Overcoming the challenges of pen-side molecular diagnosis of African swine fever to support outbreak investigations under field conditions. Transbound. Emerg. Dis. 66, 908–914. doi: 10.1111/tbed.13103
Pang, J., Wang, Q., Fei, Y., Zhu, P., Qiao, L., Huang, H., et al. (2019). A real-time recombinase polymerase amplification assay for the rapid detection of Vibrio harveyi. Mol. Cell. Probes. 44, 8–13. doi: 10.1016/j.mcp.2019.01.001
Revilla, Y., Perez-Nunez, D., and Richt, J. A. (2018). African swine fever virus biology and vaccine approaches. Adv. Virus Res. 100, 41–74. doi: 10.1016/bs.aivir.2017.10.002
Song, H., Zhang, L., Zhang, J. E., Qiu, H. J., and Luo, Y. Z. (2021). Advances in epidemiology and diagnosis of African swine fever. Chin. J. Vet. Sci. 136, 535–539.
Sun, E., Zhang, Z., Wang, Z., He, X., Zhang, X., Wang, L., et al. (2021). Emergence and prevalence of naturally occurring lower virulent African swine fever viruses in domestic pigs in China in 2020. Sci. China Life Sci. 64, 752–765. doi: 10.1007/s11427-021-1904-4
Wang, J., Wang, J., Liu, L., and Yuan, W. (2017). Development of a real-time recombinase polymerase amplification assay for rapid and sensitive detection of porcine circovirus 2. Arch. Virol. 162, 2293–2296. doi: 10.1007/s00705-017-3368-3
Wang, X., He, S., Zhao, N., Liu, X., Cao, Y., Zhang, G., et al. (2020). Development and clinical application of a novel CRISPR-Cas12a based assay for the detection of African swine fever virus. BMC Microbiol. 20:282. doi: 10.1186/s12866-020-01966-6
Wang, Z., Li, P., Lin, X., Jia, H., Jiang, Y., Wang, X., et al. (2021). Application of portable real-time recombinase-aided amplification (rt-RAA) assay in the clinical diagnosis of ASFV and prospective DIVA diagnosis. Appl. Microbiol. Biotechnol. 105, 3249–3264. doi: 10.1007/s00253-021-11196-z
Wozniakowski, G., Fraczyk, M., Kowalczyk, A., Pomorska-Mol, M., Niemczuk, K., and Pejsak, Z. (2017). Polymerase cross-linking spiral reaction (PCLSR) for detection of African swine fever virus (ASFV) in pigs and wild boars. Sci. Rep. 7:42903. doi: 10.1038/srep42903
Xia, X., Yu, Y., Weidmann, M., Pan, Y., Yan, S., and Wang, Y. (2014). Rapid detection of shrimp white spot syndrome virus by real time, isothermal recombinase polymerase amplification assay. PLoS One 9:e104667. doi: 10.1371/journal.pone.0104667
Zhai, Y., Ma, P., Fu, X., Zhang, L., Cui, P., Li, H., et al. (2020). A recombinase polymerase amplification combined with lateral flow dipstick for rapid and specific detection of African swine fever virus. J. Virol. Methods 285:113885. doi: 10.1016/j.jviromet.2020.113885
Zhang, S., Sun, A., Wan, B., Du, Y., Wu, Y., Zhang, A., et al. (2020). Development of a directly visualized recombinase polymerase amplification-SYBR green I method for the rapid detection of African swine fever virus. Front. Microbiol. 11:602709. doi: 10.3389/fmicb.2020.602709
Zhang, Y. Y., Zhang, J. Y., Yang, J. J., Yang, J. M., Han, X., Mi, L. J., et al. (2021). Identification of a natural variant of African swine fever virus in China. Chin. J. Vet. Sci. 41, 199–207. doi: 10.16303/j.cnki.1005-4545.2021.02.01
Keywords: African swine fever virus, real-time recombinase-aid amplification assay, nucleic acid detection, qPCR, portable instrument
Citation: Wu Y, Yang Y, Ru Y, Qin X, Li M, Zhang Z, Zhang R, Li Y, Zhang Z and Li Y (2022) The Development of a Real-Time Recombinase-Aid Amplification Assay for Rapid Detection of African Swine Fever Virus. Front. Microbiol. 13:846770. doi: 10.3389/fmicb.2022.846770
Received: 31 December 2021; Accepted: 24 January 2022;
Published: 17 March 2022.
Edited by:
Chunfu Zheng, University of Calgary, CanadaReviewed by:
Xiaole Qi, Harbin Veterinary Research Institute (CAAS), ChinaCopyright © 2022 Wu, Yang, Ru, Qin, Li, Zhang, Zhang, Li, Zhang and Li. This is an open-access article distributed under the terms of the Creative Commons Attribution License (CC BY). The use, distribution or reproduction in other forums is permitted, provided the original author(s) and the copyright owner(s) are credited and that the original publication in this journal is cited, in accordance with accepted academic practice. No use, distribution or reproduction is permitted which does not comply with these terms.
*Correspondence: Zhidong Zhang, Wmhhbmd6aGlkb25nQHN3dW4uZWR1LmNu; Yanmin Li, bGl5YW5taW5Ac3d1bi5lZHUuY24=
†These authors have contributed equally to this work
Disclaimer: All claims expressed in this article are solely those of the authors and do not necessarily represent those of their affiliated organizations, or those of the publisher, the editors and the reviewers. Any product that may be evaluated in this article or claim that may be made by its manufacturer is not guaranteed or endorsed by the publisher.
Research integrity at Frontiers
Learn more about the work of our research integrity team to safeguard the quality of each article we publish.