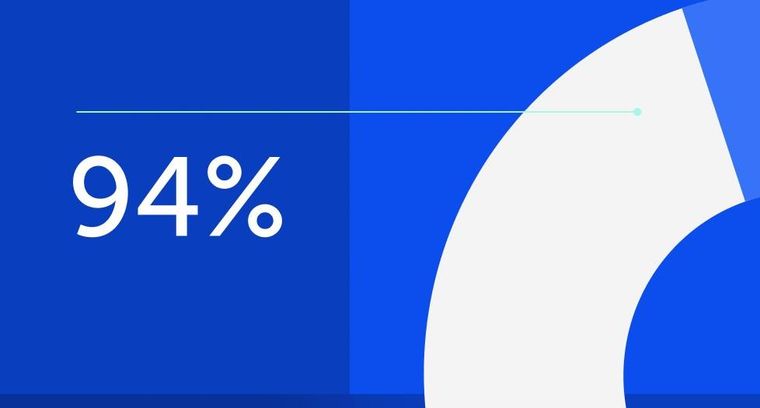
94% of researchers rate our articles as excellent or good
Learn more about the work of our research integrity team to safeguard the quality of each article we publish.
Find out more
ORIGINAL RESEARCH article
Front. Microbiol., 11 March 2022
Sec. Microbial Symbioses
Volume 13 - 2022 | https://doi.org/10.3389/fmicb.2022.845104
This article is part of the Research TopicInsights in Microbial Symbioses: 2021View all 7 articles
Wild rice (Oryza granulata) is a natural resource pool containing abundant unknown endophytic fungi species. There are few reports on the endophytic fungi in wild rice. Here, one isolate recovered from wild rice roots was identified as a new species Pseudophialophora oryzae sp. nov based on the molecular phylogeny and morphological characteristics. Fluorescent protein-expressing P. oryzae was used to monitor the fungal colonization pattern. Hyphae invaded the epidermis to the inner cortex but not into the root stele. The inoculation of P. oryzae promoted the rice growth, with the growth parameters of chlorophyll content, shoot height, root length, fresh shoot weight, fresh root weight and dry weight increasing by 24.10, 35.32, 19.35, 90.00, 33.3, and 79.17%, respectively. P. oryzae induced up-regulation of nitrate transporter OsPTR9 and potassium transporter OsHAK16 by 7.28 ± 0.84 and 2.57 ± 0.80 folds, promoting nitrogen and potassium elements absorption. In addition, P. oryzae also conferred a systemic resistance against rice blast, showing a 72.65 and 75.63% control rate in sterile plates and potting conditions. This systemic resistance was mediated by the strongly up-regulated expression of resistance-related genes NAC, OsSAUR2, OsWRKY71, EL5, and PR1α. Since P. oryzae can promote rice growth, biomass and induce systemic disease resistance, it can be further developed as a new biogenic agent for agricultural production, providing a new approach for biocontrol of rice blast.
Endophytic fungi have been found colonizing all plant species and grow symptomatically in host plant tissues (Carroll, 1988). Endophytic fungi confer benefits to the host plants by promoting growth, enhancing resistance to biotic and abiotic stresses (Sieber, 2002), and improving the host’s ecological adaptability (Schulz and Boyle, 2005; Bertolazi et al., 2019; Domka et al., 2019; Vergara et al., 2019; White et al., 2019). Endophytic fungi promote plant growth and development by increasing nutrient intake of nutrient elements by the host plants (Rana et al., 2020). Phytohormones play as messengers to control plant growth and development (Aly et al., 2010). Certain endophytes synthesize phytohormones, such as indole-3-acetic acid (IAA), gibberellins (GAs), and cytokinins, to promote host plant growth (You et al., 2013; Khan A. L. et al., 2014; Khan A. R. et al., 2014). In addition, endophytic fungi also play essential roles on improving plant disease resistance. Harpophora oryzae isolated from the wild rice roots possessed biocontrol potential to rice blast (Yuan et al., 2010; Su et al., 2013).
Magnaporthales is an order of Sordariomycetes, Ascomycota (Zhang et al., 2011; Luo et al., 2015). About 50% of these species are pathogens of monocotyledons, such as rice, maize and wheat (Kirk et al., 2008; Luo and Zhang, 2013). Pseudophialophora is a newly established genus in Magnaporthaceae of Magnaporthales (Luo et al., 2014). This study isolated and identified a new species, endophytic fungus P. oryzae sp. nov, from the wild rice roots based on phylogenetic and molecular analysis. We monitored the colonization pattern of P. oryzae in rice roots by transferring the GFP fluorescence label, then detected the biomass of P. oryzae in vivo by real-time PCR. The effect of P. oryzae on promoting plant nutrient absorption and disease resistance was further investigated. This work provides a scientific basis for P. oryzae as biological hormones, biological control agents and biological fertilizers.
Wild rice (Oryza granulata) samples were collected from Xishuangbanna, Yunnan province, southwest of China, in November 2019. The isolation method of endophytic fungi referred to Yuan’s method (Yuan et al., 2010). Briefly, the healthy rice roots were gently rinsed with tap water, then immersed in 75% ethanol for 30 s and 1% sodium hypochlorite for 10 min. Subsequently, the roots were rinsed with sterile distilled water three times and cut into approximately 5 mm long segments. The segments were then transferred into a malt extract agar (MEA) medium (2% malt extract, 2% agar). The plates were incubated at 25°C in darkness. Fungal cultures were isolated and purified, saved on potato dextrose agar (PDA) slope (Yuan et al., 2010).
Fungal DNA was extracted by DNA extraction method (Chi et al., 2009). Six genes, internal transcribed spacer (ITS), large subunit (LSU) and small subunit (SSU) of ribosomal RNA genes, DNA replication licensing factor (MCM7), the largest subunit of RNA polymerase II (RPB1), and translation elongation factor 1-α (TEF1-α) genes, were amplified for identification (Zhang et al., 2011; Luo and Zhang, 2013). Primers are listed in Supplementary Table 1. PCR amplification refers to the method of Zhang et al. (2011). PCR products were sequenced by ABI3730 (Tsingke company, Beijing), and the sequencing results were compared with the BLAST sequence on the national center for biotechnology information (NCBI) website. All reference strain names used for phylogenetic analysis and isolate numbers, sources, hosts, and GenBank accession numbers were listed in Table 1 (Luo and Zhang, 2013; Luo et al., 2014). The partial sequences of strain P-B313 were submitted to the GenBank and obtained GenBank accession numbers (Table 1). Sequences of each gene were aligned with Clustal X 2.1 (Thompson et al., 1997) and manually corrected with Genedoc (Yuan et al., 2010). A six-gene dataset was generated by connecting the individual sequence alignments. JModel Test 2.1.7 (Posada, 2008) was used to calculate the best-fit nucleotide substitution models by computing likelihood scores and calculating AIC. Cryphonectria parasitica was chosen as the outgroup taxon. Bayesian inference (BI) trees were constructed in MrBayes v3.2.6 (Ronquist et al., 2012), using the optimal nucleotide substitution model. A total of 100,000 trees were produced. The latter 37,500 trees were selected to calculate the posterior probability values of each branch in the consensus tree. Maximum-likelihood (ML) analysis with the selected optimal model was executed in IQ-Tree (Nguyen et al., 2015). Branch support was evaluated by 1000 bootstraps replicates.
Table 1. Species name, isolate ID, source, host, and GenBank accession numbers of the fungi used in this study.
Strain P-B313 was cultured in 150 mL potato dextrose broth (PDB) at 25°C 150 rpm for 3 days. The mycelia and conidia were then collected and observed under a microscope (Carl Zeiss Inc., Germany).
P-B313 fungal plug (5 mm × 5 mm) was fixed into 2.5% glutaraldehyde solution at 4°C overnight. Then the samples were rinsed with 0.1 M phosphate buffer (pH = 7) three times (15 min each time), fixed in 1% OsO4 for 2 h at 25°C, washed with phosphate buffer three times and dehydrated in a graded ethanol series. The samples were dried on HCP-2 critical point dryer (Hitachi, Japan) and coated. Finally, the samples were observed under SU-8010 scanning electron microscope (SEM) (Hitachi, Japan) (Liu X. H. et al., 2007).
The strain P-B313 was cultured in PDB for 3 days. And the conidia suspension with a concentration of 1 × 106 spores/mL was collected. Agrobacterium tumefaciens strains containing PKD5-GFP vector with sulfonylureas resistance gene were mixed with P-B313 conidia suspension in equal volume (Lu et al., 2014). The transformants were screened on a defined complex medium (DCM) containing sulfonylurea (Dai et al., 2021). The fluorescence was detected by LSM880 confocal laser scanning microscope (Carl Zeiss Inc., Germany).
Rice seeds of blast-susceptible rice cultivar CO-39 (Oryza sativa) were surface-sterilized in 70% ethanol for 5 min, in 1.0% sodium hypochlorite solution for 20 min rinsed repeatedly using sterile water. Rice seeds were then planted in half-strength Murashige and Skoog medium (Murashige and Skoog, 1962) for 3 days, then transferred into tissue culture bottles (8 cm in width, 50 cm in height) containing half-strength Murashige and Skoog in which 10 seedlings were inoculated. We then inoculated three fresh mycelium plugs (diameter 8 mm, 7-day-old) in each tissue culture vessel. Blank agar blocks were used as control.
After 14 days of co-culture with GFP-tagged strain P-B313, the roots of the symbionts were collected and observed under an LSM880 confocal laser scanning microscope (Carl Zeiss Inc., Germany).
The fungus/plant DNA ratio (FPDR) was used to detect fungal infection in rice roots. The degree of fungal infection was determined by 2–Δ Ct (Kenneth and Thomas, 2002), where ΔCt was the difference threshold value between strain P-B313 Tef-1α gene and rice Actin gene (Deshmukh et al., 2006; Deshmukh and Kogel, 2007). The specific primers were designed to be consistent with the tef-1α gene amplification primers. A total of 100 mg of root samples were collected at 5, 10, 15, and 20 days after inoculation (d.a.i.), respectively, according to Maciá-Vicente et al. (2009). The DNA was extracted using the nuclear plant genomic DNA kit (Tiangen, Beijing). The real-time PCR was performed in a total volume of 25 μL, including 10 ng of DNA, 12.5 μL of 2x SYBR Premix Ex Taq™ (Takara Bio Inc., Shiga, Japan), 1.25 μL of specific primer TEF1-F/R (or Actin-F/R for the rice Actin gene; Supplementary Table 1) and 10.25 μL of ddH2O. Melting curve analysis was performed. Ct values were measured by using the Realplex software 2.2.10.84.
Strain P-B313 was cultured in 150 mL PDB at 25°C 150 rpm for 3 days. The mycelium suspension was then inoculated into sterilized barley grains (150 mL/200 g) and fermented at 25°C for 15 days. The germinated rice seeds were planted into pots containing fermented fungal fertilizer (75 g fertilizer, 30 seeds per pot). The controls were rice seeds inoculated with sterile barley grains. After 14 days of co-culture, the growth parameters, such as the chlorophyll content, shoot length, root length, shoot fresh weight, fresh root weight, and dry weight, were determined. A total of 30 rice plants were measured in the control and treatment groups, respectively. The length of the longest root was measured.
The pathogen Magnaporthe oryzae Guy11 was cultured in a complete medium (CM) 10 days. Then the spores were collected and prepared into suspension with a concentration of 5 × 104 spores/mL. The rice leaves were sprayed with spore suspension and incubated in the dark at 22°C for 2 days, at 25°C for 4 days (light 16 h/darkness 8 h). The lesion area rate and disease index were calculated. The disease index was investigated according to the Standard Evaluation System for Rice (SES) of the International Rice Research Institute (IRRI 2002) (Supplementary Table 2). The disease equation is as follows: disease index = Σ(diseased level leaf number × representative value) / (total leaf number × heavy disease representative value) × 100% (Li et al., 2020).
The rice leaves and roots were collected separately and dried to constant weight under −80°C, then ground into dry powder. A total of 0.5 g of dry powder sample was placed in the digestion tank with 5 mL concentrated nitric acid and 1 mL hydrogen peroxide, shake well and let it stand for 1 min before digestion. After digestion, the acid was heated on an electric stove. And after cooling, use 2% nitric acid to make the volume 200 mL. Finally, phosphorus (P), potassium (K), magnesium (Mg), and iron (Fe) were determined by ICP-OES (IRIS Intrepid II XSP, Thermo, United States). The nitrogen (N) content is determined by Kjeldahl method (Stafilov et al., 2020).
After co-culture of strain P-B313 with rice for 14 days, rice plants were collected. Total rice RNA was extracted using TRIzol (Invitrogen, United States), followed by PrimeScript™ RT reagent Kit with gDNA Eraser (Perfect Real Time) (TaKaRa, Japan) kit for reverse transcription. The rice nutrition absorption-related genes OsPTR9, OsAMT3;2, OsMRS2-8, OsPT4, OsHAK16, OsIRO2, and OsYSL15 and rice disease resistance-related genes NAC, AOS, OsSAUR2, OsWRKY71, POX1, POX2, EL5, ERF4, PR1α, and PR1b were measured by quantitative analysis. The real-time PCR was performed in a total volume of 20 μL, including template cDNA (five times diluted) 1 μL, 10 μL of 2x SYBR Premix Ex Taq™ (Takara, Japan), 1 μL of specific primer (Supplementary Table 1) and 7 μL of ddH2O. Reaction conditions: 95°C for 5 min, 40 cycles (95°C for 10 s, 60°C for 15 s), and the dissolution curve was set. The relative expression quantity of gene expression was calculated by 2–ΔΔCt (Schmittgen and Livak, 2008).
Data were statistically analyzed by SPSS 16.0 version software (SPSS Inc., United States), expressed as mean ± standard deviation (SD). Graphs were created using GraphPad Prism 8.
The morphology of the colony, hyphae and conidia were observed. Strain P-B313 grew slowly on PDA medium, and the colony diameter reached 4 cm after growing at 25°C for 7 days. Aerial mycelia were white, prostrating on the medium surface. Mycelia were 0.5–4.0 μm in width, with a septum. Conidiophores were solitary, no branching. Conidia were elliptic or dumbbell-shaped, 11–15 × 3.5–6.5 μm (Figure 1).
Figure 1. Morphological characteristics of P. oryzae. (A) Fungal colony on PDA after 7 days at 25°C. (B) Mycelia morphology under the optical microscope. Bar, 5 μm. (C) Conidia morphology under an optical microscope. Bar, 5 μm. (D) Scanning electronic micrographs of mycelia and conidia. Bar, 2 μm.
We first blasted the similarity of the ITS sequence of the strain P-B313 on the NCBI website. The results showed that the identity between strain P-B313 and Pseudophialophora sp. (MK808146) was 99.4%. We conducted a phylogenetic analysis of strain P-B313 with the other related genus in Magnaporthaceae. It was found that there were 580 nucleotides in the ITS alignment, 869 in LSU, 1,032 in SSU, 926 in TEF1, 559 in MCM7, and 769 in RPB1. The 6-gene dataset involved 4,735 characters, including 925 parsimony informative, 722 variable and parsimony uninformative, and 3,088 constant. Calculated by jModel Test2.1.7, TN + F + R4 and TrN + I + G were selected as the optimal BI and ML analysis models. The two trees’ topological structures are similar using phylogenetic trees constructed by BI and ML methods. Only the BI tree is shown in Figure 2. Strain P-B313 belongs to the Pseudophialophora genus from the phylogenetic tree, but it exists in a separate clade independent of Pseudophialophora panicorum (Luo et al., 2014). In addition, the strain morphology and mycelium morphology of strain P-B313 and P. panicorum were quite different (Luo et al., 2014). Based on the molecular phylogeny and morphological, biological, and ecological characteristics, strain P-B313 was defined as a new species P. oryzae sp. nov (Collection Number: CCTCC M 2021504).
Figure 2. Phylogenetic tree of six genes combined. The tree was inferred from the combined ITS, SSU, LSU, TEF1, MCM7, and RPB1 sequence datasets. The topology of the tree is the result of BI method. The value on the branch is the BI posterior probability (BIPP)/ML bootstrap proportion (MLBP). The Bar indicates 0.04 base substitution sites.
After five generations, intense green fluorescence was found to be uniformly distributed in the hyphae and conidiophores (Figure 3). The GFP-expressed transformant was selected as a candidate for further root inoculation.
Figure 3. Laser scanning confocal microscopy of the GFP-expressed P. oryzae transformant. Hyphae and conidia showed constitutive GFP expression. Bar, 10 μm.
The colonization pattern was monitored using GFP-labeled P. oryzae. Transversely, the fungus entered the root epidermis and then invaded the inner cortical layer, finally colonized in the inner cortical layer. No hyphae approached the central part of the roots. Concomitantly, abundant hyphae preferred to colonize in the epidermis and outer cortex (Figure 4A).
Figure 4. Colonization pattern of P. oryzae in rice roots. (A) GFP-tagged hyphae gradually extended from the epidermis to the endodermis in a root cross-section and longitudinal section. Bar, 5 μm. (B) Relative amounts of fungal DNA in rice roots at different time points (5, 10, 15, and 20 d.a.i.). A fungal colonization curve plotted with Mean ± SD is shown.
The FPDR was measured simultaneously to assess fungal growth and the respective plant response. It was shown that an early moderate increase in the FPDR from 1.20 ± 0.18 to 4.90 ± 2.43 occurred within 10 d.a.i., followed by a significant increase to 22.85 ± 9.51 at 20 d.a.i. (Figure 4B).
Pseudophialophora oryzae and rice were co-cultivated to investigate whether P. oryzae promotes rice growth. It was founded that the P. oryzae inoculated rice seedlings grew better and stronger than the control plants (Figures 5A,B), exhibiting higher chlorophyll content, shoot height, root length, fresh shoot weight, fresh root weight, and plant dry weight by 24.10, 35.32,19.35, 90.00, 33.3, and 79.17%, respectively (Figures 5C–H). These results indicated that P. oryzae possessed a positive capacity for plant growth.
Figure 5. Effect of P. oryzae on the rice growth in pots. (A,B) The comparison of P. oryzae-treated plants with control in pots. (C–H) The comparison of P. oryzae-treated plants with non-treated control on the growth parameters includes the chlorophyll content, shoot height, root length, fresh shoot weight, fresh root weight, and plant dry weight. All the above bar charts were plotted with Mean ± SD. Independent-samples t-test analyzed data. The symbols * and ** indicate significant differences at P < 0.05 and P < 0.01, respectively.
We then investigated whether P. oryzae confers resistance to rice against rice blast under both plate and pot conditions. It was shown that the disease of the control rice plants grown in plates was serious, forming large circular or oval brown spots, disease spots densely covered (Figure 6A). The lesion area rate was 36.23%, and the disease index was 80.95% (Figures 6B,C). In contrast, the disease of rice plants inoculated with P. oryzae was relatively mild (Figure 6A), with a 9.91% lesion area rate (Figure 6B). The leaf area of the disease spot was small, accompanied by a few necrotic spots, and the disease index was only 25.92% (Figure 6C). The control effect of P. oryzae on rice blast reached 72.65%. Similarly, the disease resistance tests for potted plants were consistent with those for plates (Figure 6D). The lesion area rate of control and treatment was 53.13 and 12.95% (Figure 6E), respectively, and the disease index was 91.54and 28.04% (Figure 6F). The control effect of P. oryzae on rice blast reached 75.63% in pots. In conclusion, root colonization of P. oryzae can induce systemic disease resistance of hosts and has a positive control effect on rice blast.
Figure 6. Effect of P. oryzae on resistance against rice blast. (A,D) The severity of devastating symptoms on the leaves of P. oryzae-inoculated rice compared to control in plate and pot experiments, respectively. (B,E) An AxioVision image analyzer evaluated the lesion area. Bar charts were plotted with Mean ± SD. (C,F) According to the disease classification, the disease index of P. oryzae-infected and control-infected rice was calculated. Bar charts were plotted with Mean ± SD from 30 plants, respectively. Independent-samples t-test analyzed data. The symbols * and ** indicate significant differences at P < 0.05 and P < 0.01, respectively.
Through the analysis of the nutrient element contents in the shoots and roots of rice plants, it was found that after inoculation with P. oryzae, the contents of N and K in the shoot tissues of rice plants increased significantly, which increased by 15.28 and 3.88% compared with the control group, respectively (Figure 7A). There was no significant change in P, Mg, and Fe content. Similarly, the contents of elements such as N, K, and Mg in the roots of the treatment group also increased significantly, increasing by 12.35, 3.29, and 0.36%, respectively (Figure 7B). There was no significant change in P and Fe content. Therefore, the root colonization of P. oryzae can effectively promote the absorption of nutrient elements in rice roots and increase the content of nutrient elements in the tissues.
Figure 7. The effect of P. oryzae on nutrient content in rice seedling tissues. (A) The nutrient content in shoots. (B) The nutrient content in roots. Independent-samples t-test analyzed data. The symbols * and ** indicate significant differences at P < 0.05 and P < 0.01, respectively.
We analyzed the expression levels of N, P, K, Fe, Mg, and other key genes for nutrient absorption and resistance-related genes. The results showed that the root colonization of P. oryzae significantly up-regulated the expression of peptide transporter OsPTR9 and potassium transporter OsHAK16, which were 7.28 ± 0.84 times and 2.57 ± 0.80 times higher than that of the control group, respectively. Genes such as OsAMT3;2 and OsMRS2-8 were significantly down-regulated. It can be seen that after P. oryzae infects and colonizes rice roots, it can significantly up-regulate the expression of genes related to N and K element absorption, thereby promoting nutrient element absorption (Table 2).
In addition, we found that the root colonization of P. oryzae significantly up-regulated the expression of NAC, OsSAUR2, OsWRKY71, EL5, and PR1α genes, which were 3.04 ± 0.72, 10.37 ± 0.34, 1.98 ± 0.13, 2.10 ± 0.35, and 1.46 ± 0.17 times of the control group, respectively. Compared with the control group, AOS, POX2, and PR1b were significantly down-regulated by 0.36 ± 0.05, 0.39 ± 0.24, and 0.38 ± 0.16 times. However, the expression levels of POX1 and ERF4 were not significantly changed. In conclusion, P. oryzae can induce up-regulated expression of some genes representing plant defense response and improve the host systemic disease resistance (Table 3).
Plant roots provide excellent habitats and nutrients for endophytic fungi to help them survive. Endophytic fungi, in turn, protect plants from biotic and abiotic stresses (Verma et al., 2009; Lahrmann et al., 2013; Mitter et al., 2013). Endophytic fungal communities play an important role in adapting wild rice to poor environments. Our study firstly isolated P. oryzae from the wild rice roots. There are few reports of Pseudophialophora genus, besides Pseudophialophora sp. isolated from the grassroots by Luo et al. (2014, 2015). The six-genes phylogeny showed that P. oryzae was defined as a singleton in the genus, while P. panicorum clustered in another subclade. Morphologically, P. oryzae is significantly different from P. panicorum (Luo et al., 2014). A new species P. oryzae sp. nov was proposed for the first time. And P. oryzae was beneficial for rice growth and blast resistance (Figure 8).
Figure 8. Schematic representations of rice colonized by P. oryzae. P. oryzae promoted the absorption of N and K elements, promoted rice growth, and enhanced the resistance to rice blast. The white dots represent P. oryzae.
The colonization pattern of endophytic fungi is essential for understanding the symbiosis between endophytes and host plants. We found that P. oryzae hyphae invaded the root epidermis into the cortex and reached the endodermis but did not approach the stele. This colonization pattern was similar to dark septate endophytes (DSEs) and soil-inhabiting fungi (Maciá-Vicente et al., 2009). Differently, DSEs formed fungal structures, including hyphopodia and microsclerotia (Su et al., 2013), while P. oryzae did not form such structures during infection. The fungal proliferation pattern of DSE H. oryzae in rice increased firstly and then stabilized (Su et al., 2013). However, the fungal proliferation pattern of P. oryzae kept increasing within 20 days, neither causing any disease symptoms.
Endophytes promote plant growth (Rigobelo and Baron, 2021), which is mainly regulated by the levels of plant hormones (Khalmuratova et al., 2021) or promoting plants to obtain essential nutrients (Rigobelo and Baron, 2021). Endophytes can secrete growth-promoting substances such as auxin, cytokinin, gibberellin (Khan et al., 2012), and secondary metabolites (Peters et al., 1998) to regulate hormone levels and promote plant growth and development. Colonization of Anteaglonium in blueberry roots changed the metabolism of plant hormones and flavonoids, stimulating blueberries’ growth (Wu et al., 2020). Alternaria tenuissima and Fusarium tricinctum synthesized auxin and promoted the growth of host plants (Chand et al., 2020). Endophytes also promote nutrient uptake, often including N, P, and K elements critical for plant development (Tan and Zou, 2001). Xylaria regalis from cones of Thuja plicata could significantly increase the N content of red pepper and thus promote the growth of pepper (Adnan et al., 2018). Piriformospora indica improved the accumulation of N and K to improve tomato growth (Ghorbani et al., 2019). In addition, genes related to nutrient absorption also played important roles. OsPTR9 is a member of the peptide transporter PTR gene family. Overexpression of OsPTR9 could increase the lateral root density of rice, increase the contact area between root and nutrients, fix nitrogen in the atmosphere, promote the absorption of ammonium and the growth of rice (Fang et al., 2013). OsHAK16 is a member of HAK/KUP/KT family and is essential for K absorption (Okada et al., 2008). Overexpression of OsHAK16 significantly increased K content in rice and improved the stress resistance of rice (Fang et al., 2013). Our results showed that the colonization of P. oryzae in the rice roots led to the up-regulation of the expression of OsPTR9 and OsHAK16, which increased the accumulation of N and K in rice and promoted the growth of rice. In addition to enhancing nutrient absorption, whether P. oryzae produces hormones or other secretions to promote the growth of the host is still unknown. Therefore, it is necessary to study further the interaction mechanism between P. oryzae and rice symbionts.
Endophytes can live in host tissues without causing and adverse symptoms. They can induce plant immune response and improve host disease resistance by regulating genes expression and signal network related to rice defense response (Tsuda and Somssich, 2015). In the defense response of rice, pathogenesis-related (PR) genes are the key genes to induce systemic disease resistance (Asai et al., 2002; Lee et al., 2004; Djamei et al., 2007). NAC is one plant-specific transcription factor, which plays an important role in coping with biological and abiotic stresses (Kim et al., 2012; Lv et al., 2016). Several proteins with NAC domain enhanced resistance to Pseudomonas syringae infection in tomatoes (Mysore et al., 2002). OsSAUR2 is an auxin-responsive gene in plants, which has been shown to regulate auxin synthesis and transport, inhibit auxin activity and promote plant immune resistance (Ding et al., 2008; Kant et al., 2009). EL5 is an N-acetylchitooligosaccharide elicitor response gene in rice, which acts as an E3 ubiquitin ligase and positively regulates plant immune response (Takai et al., 2002). These reports were consistent with our results that up-regulated expression of PR1α, NAC, OsSAUR2, and EL5 can enhance the systemic disease resistance of rice after P. oryzae inoculated rice roots. In addition, salicylic acid (SA) (Janda et al., 2020), jasmonic acid (JA) (Barna et al., 2012; Li et al., 2021) and ethylene (ET) (Wang et al., 2019) also play important roles in inducing resistance (Glazebrook, 2005; McDowell et al., 2005; Flors et al., 2008). AOS (Gfeller et al., 2010; Xiao et al., 2019) and ERF4 (Yang et al., 2005) are key genes of JA biosynthesis pathway and ethylene pathway, respectively. Their down-regulated expression indicated that systemic resistance induced by P. oryzae was independent of JA and ET signaling pathways. OsWRKY71 is associated with the SA signaling pathway that regulates the resistance of rice and other gramineous crops to a variety of diseases (Liu X. et al., 2007). The expression of OsWRKY71 gene was up-regulated by the inoculation of P. oryzae in rice. Therefore, the systemic resistance of P. oryzae to M. oryzae infection may be mediated by SA signaling pathway. Together, our results indicated that P. oryzae could induce systemic disease resistance in rice by regulating genes related to rice defense response.
In conclusion, we isolated an endophytic fungus P-B313 from wild rice and defined it as a new species P. oryzae by phylogenetic analysis of six-genes. After co-culture with rice, the colonization pattern of P. oryzae was that hyphae invaded from the epidermis to the inner cortex but not into the stele. P. oryzae can also promote nitrogen and potassium elements absorption in rice, significantly promote rice growth, and enhance the systemic resistance against rice blast. It can be further developed as a new biogenic agent for agricultural production, providing a new approach for the biocontrol of rice blast.
The datasets presented in this study can be found in online repositories. The names of the repository/repositories and accession number(s) can be found in the article/Supplementary Material.
J-NZ and Z-ZS contributed to experimental design. J-NZ, Y-JY, M-DD, and Y-LZ contributed to experiments. J-NZ, X-JL, and LW contributed to data analysis and scripts. F-CL, X-HL, and Z-ZS supplied experimental conditions. J-NZ, Y-JY, Z-ZS, and F-CL wrote the manuscript. All authors contributed to the article and approved the submitted version.
This work was supported by the Provincial Key Research and Development Plan of Zhejiang, China (Grant Numbers: 2019C02010 and 2021C02010) and Zhejiang Science and Technology Major Program on Agricultural New Variety Breeding 2021C02064.
The authors declare that the research was conducted in the absence of any commercial or financial relationships that could be construed as a potential conflict of interest.
All claims expressed in this article are solely those of the authors and do not necessarily represent those of their affiliated organizations, or those of the publisher, the editors and the reviewers. Any product that may be evaluated in this article, or claim that may be made by its manufacturer, is not guaranteed or endorsed by the publisher.
The Supplementary Material for this article can be found online at: https://www.frontiersin.org/articles/10.3389/fmicb.2022.845104/full#supplementary-material
Adnan, M., Alshammari, E., Ashraf, S. A., Patel, K., and Lad, K. (2018). Physiological and molecular characterization of biosurfactant producing endophytic fungi Xylaria regalis from the cones of Thuja plicata as a potent plant growth promoter with its potential application. Biomed. Res. Int. 2018:7362148. doi: 10.1155/2018/7362148
Aly, A. H., Debbab, A., Kjer, J., and Chaidir, C. (2010). Fungal endophytes from higher plants: a prolific source of phytochemicals and other bioactive natural products. Fungal Divers. 41, 1–16. doi: 10.1007/s13225-010-0034-4
Asai, T., Tena, G., Plotnikova, J., Willmann, M. R., Chiu, W. L., Gomez-Gomez, L., et al. (2002). MAP kinase signaling cascade in Arabidopsis innate immunity. Nature 415, 977–983. doi: 10.1038/415977a
Barna, B., Fodor, J., Harrach, B. D., Pogany, M., and Kiraly, Z. (2012). The janus face of reactive oxygen species in resistance and susceptibility of plants to necrotrophic and biotrophic pathogens. Plant Physiol. Biochem. 59, 37–43. doi: 10.1016/j.plaphy.2012.01014
Bertolazi, A. A., de Souza, S. B., Ruas, K. F., Campostrini, E., and de Rezende, C. E. (2019). Inoculation with Piriformospora indica is more efficient in wild-type rice than in transgenic rice over-expressing the vacuolar H (+)-ppase. Front. Microbiol. 10:1087. doi: 10.3389/fmicb.2019.01087
Carroll, G. (1988). Fungal Endophytes in Stems and Leaves: from Latent Pathogen to Mutualistic Symbiont. Ecology 69, 2–9. doi: 10.2307/1943154
Chand, K., Shah, S., Sharma, J., Paudel, M. R., and Pant, B. (2020). Isolation, characterization, and plant growth-promoting activities of endophytic fungi from a wild orchid Vanda cristata. Plant Signal. Behav. 15:1744294. doi: 10.1080/15592324.2020.1744294
Chi, M. H., Park, S. Y., and Lee, Y. H. (2009). A Quick and Safe Method for Fungal DNA Extraction. Plant Pathol. J. 25, 108–111. doi: 10.5423/PPJ.2009.25.1.108
Dai, M. D., Li, Y., Sun, L. X., Lin, F. C., and Liu, X. H. (2021). Isolation and functional analysis of effector proteins of Magnaporthe oryzae. Methods Mol. Biol. 2356, 199–209. doi: 10.1007/978-1-0716-1613-0_16
Deshmukh, S. D., and Kogel, K. H. (2007). Piriformospora indica protects barley from root rot caused by Fusarium graminearum. J. Plant Dis. Prot. 114, 263–268. doi: 10.1007/BF03356227
Deshmukh, S., Huckelhoven, R., Schafer, P., Imani, J., and Sharma, M. (2006). The root endophytic fungus Piriformospora indica requires host cell death for proliferation during mutualistic symbiosis with barley. Proc. Natl. Acad. Sci. U.S.A. 103, 18450–18457. doi: 10.1073/pnas.0605697103
Ding, X., Cao, Y., Huang, L., Zhao, J., and Xu, C. (2008). Activation of the indole-3-acetic acid-amido synthetase gh3-8 suppresses expansin expression and promotes salicylate- and jasmonate-independent basal immunity in rice. Plant Cell 20, 228–240. doi: 10.1105/tpc.107.055657
Djamei, A., Pitzschke, A., Nakagami, H., Rajh, I., and Hirt, H. (2007). Trojan horse strategy in Agrobacterium transformation: abusing MAPK defense signaling. Science 318, 453–456. doi: 10.1126/science.1148110
Domka, A. M., Rozpaadek, P., and Turnau, K. (2019). Are fungal endophytes merely mycorrhizal copycats? Front. Microbiol. 10:371. doi: 10.3389/fmicb.2019.00371
Fang, Z., Xia, K., Yang, X., Grotemeyer, M. S., Meier, S., Rentsch, D., et al. (2013). Altered expression of the PTR/NRT1 homologue OsPTR9 affects nitrogen utilization efficiency, growth and grain yield in rice. Plant Biotechnol. J. 11, 446–458. doi: 10.1111/pbi.12031
Flors, V., Ton, J., van Doorn, R., Jakab, G., Garcia-Agustin, P., and Mauch-Mani, B. (2008). Interplay between JA, SA and ABA signalling during basal and induced resistance against Pseudomonas syringae and Alternaria brassicicola. Plant J. 54, 81–92. doi: 10.1111/j.1365-313X.2007
Gfeller, A., Dubugnon, L., Liechti, R., and Farmer, E. E. (2010). Jasmonate biochemical pathway. Sci. Signal 3:cm3. doi: 10.1126/scisignal.3109cm3
Ghorbani, A., Omran, V., Razavi, S. M., Pirdashti, H., and Ranjbar, M. (2019). Piriformospora indica confers salinity tolerance on tomato (Lycopersicon esculentum mill.) Through amelioration of nutrient accumulation, K+/Na+ homeostasis and water status. Plant Cell Rep. 38, 1151–1163. doi: 10.1007/s00299-019-02434-w
Glazebrook, J. (2005). Contrasting mechanisms of defense against biotrophic and necrotrophic pathogens. Ann. Rev. Phytopathol. 43, 205–227. doi: 10.1146/annurev.phyto.43.040204.135923
Janda, T., Szalai, G., and Pal, M. (2020). Salicylic acid signalling in plants. Int. J. Mol. Sci. 21:2655. doi: 10.3390/ijms21072655
Kant, S., Bi, Y. M., Zhu, T., and Rothstein, S. J. (2009). SAUR39, a small auxin-up RNA gene, acts as a negative regulator of auxin synthesis and transport in rice. Plant Physiol. 151, 691–701. doi: 10.1104/pp.109.143875
Kenneth, J. L., and Thomas, D. S. (2002). Analysis of relative gene expression data using real-time quantitative pcr and the 2–ΔΔCt method. Methods 25, 402–408. doi: 10.1006/meth.2001.1262
Khalmuratova, I., Choi, D. H., Yoon, H. J., Yoon, T. M., and Kim, J. G. (2021). Diversity and plant growth promotion of fungal endophytes in five halophytes from the buan salt marsh. J. Microbiol. Biotechnol. 31, 408–418. doi: 10.4014/jmb.2012.12041
Khan, A. L., Waqas, M., Hussain, J., Harrasi, A. A., Rawah, A. A., Adnan, M., et al. (2014). Endophytes Aspergillus caespitosus LK12 and Phoma sp. LK13 of Moringa peregrina produce gibberellins and improve rice plant growth. J. Plant Interact. 9, 731–737. doi: 10.1080/17429145.2014.917384
Khan, A. R., Ullah, I., Khan, A. L., Hong, S. J., Waqas, M., Kwak, Y., et al. (2014). Phytostabilization and physicochemical responses of Korean ecotype Solanum nigrum L. to cadmium contamination. Water Air Soil Pollut. 225:2147.
Khan, S. A., Hamayun, M., Khan, A. L., Lee, I. J., and Kim, J. G. (2012). Isolation of plant growth promoting endophytic fungi from dicots inhabiting coastal sand dunes of Korea. Pak. J. Bot. 44, 1453–1460. doi: 10.1105/tpc.112.240861
Kim, H. S., Park, H. C., Kim, K. E., Jung, M. S., and Han, H. J. (2012). A NAC transcription factor and SNI1 cooperatively suppress basal pathogen resistance in Arabidopsis thaliana. Nucl. Acids Res. 40, 9182–9192. doi: 10.1093/nar/gks683
Kirk, P. M., Cannon, P. F., and Stalpers, J. A. (2008). Dictionary of the Fungi, 10th Edn. Wallingford: CAB International.
Lahrmann, U., Ding, Y., Banhara, A., Rath, M., Hajirezaei, M. R., Döhle, S., et al. (2013). Host-related metabolic cues affect colonization strategies of a root endophyte. Proc. Natl. Acad. Sci. U.S.A. 110, 13965–13970. doi: 10.1073/pnas.1301653110
Lee, D. E., Lee, I. J., Han, O., Baik, M. G., Han, S. S., Back, K., et al. (2004). Pathogen resistance of transgenic rice plants expressing mitogen-activated protein kinase 1, MK1, from Capsicum annuum. Mol. Cells 29, 81–85.
Li, H. R., Xiang, H. M., Zhong, J. W., Ren, X. Q., Wei, H., Zhang, J. E., et al. (2020). Acid rain increases impact of rice blast on crop health via inhibition of resistance enzymes. Plants 13:881. doi: 10.3390/plants9070881
Liu, X. H., Lu, J. P., Zhang, L., Dong, B., Min, H., and Lin, F. C. (2007). Involvement of a Magnaporthe grisea serine/threonine kinase gene, MgATG1, in appressorium turgor and pathogenesis. Eukaryot. Cell 6, 997–1005. doi: 10.1128/EC.00011-07
Li, Z., Wei, Y., Cao, Z., Jiang, S., Chen, Y., Shao, X., et al. (2021). The jasmonic acid signaling pathway is associated with terpinen-4-ol-induced disease resistance against Botrytis cinerea in strawberry fruit. J. Agric. Food Chem. 69, 10678–10687. doi: 10.1021/acs.jafc.1c04608
Liu, X., Bai, X., Wang, X., and Chu, C. (2007). OsWRKY71, a rice transcription factor, is involved in rice defense response. J. Plant Physiol. 164, 969–979. doi: 10.1016/j.jplph.2006.07.006
Lu, J., Cao, H., Zhang, L., Huang, P., and Lin, F. (2014). Systematic analysis of Zn2cys6 transcription factors required for development and pathogenicity by high-throughput gene knockout in the rice blast fungus. PLoS Pathog. 10:e1004432. doi: 10.1371/journal.ppat.1004432
Luo, J., and Zhang, N. (2013). Magnaporthiopsis, a new genus in Magnaporthaceae (Ascomycota). Mycologia 105, 1019–1029. doi: 10.3852/12-359
Luo, J., Walsh, E., and Zhang, N. (2014). Four new species in Magnaporthaceae from grass roots in New Jersey pine barrens. Mycologia 106, 580–588. doi: 10.3852/13-306
Luo, J., Walsh, E., Blystone, D., and Zhang, N. (2015). Five new Pseudophialophora species from grass roots in the oligotrophic pine barrens ecosystem. Fungal Biol. 119, 1205–1215. doi: 10.1016/j.funbio.2015.08.016
Lv, Z., Wang, S., Zhang, F., Chen, L., Hao, X., Pan, Q., et al. (2016). Overexpression of a novel NAC domain-containing transcription factor gene (aaNAC1) enhances the content of artemisinin and increases tolerance to drought and Botrytis cinerea in artemisia annua. Plant Cell Physiol. 57, 1961–1971. doi: 10.1093/pcp/pcw118
Maciá-Vicente, J. G., Jansson, H. B., Talbot, N. J., and Lopez-Llorca, L. V. (2009). Real-time PCR quantification and live-cell imaging of endophytic colonization of barley (Hordeum vulgare) roots by Fusarium equiseti and Pochonia chlamydosporia. New Phytol. 182, 213–228. doi: 10.1111/j.1469-8137.2008.02743.x
McDowell, J. M., Williams, S. G., Funderburg, N. T., Eulgem, T., and Dangl, J. L. (2005). Genetic analysis of developmentally regulated resistance to downy mildew (Hyaloperonospora parasitica) in Arabidopsis thaliana. Mol. Plant Microb. Interact. 18, 1226–1234. doi: 10.1094/MPMI-18-1226
Mitter, B., Weilharter, A., Chain, P. S. G., Trognitz, F., and Sessitsch, A. (2013). Genome analysis, ecology, and plant growth promotion of the endophyte Burkholderia phytofirmans strain PsJN. Mol. Microb. Ecol. Rhizosphere 2, 865–874. doi: 10.1002/9781118297674.ch81
Murashige, T., and Skoog, F. (1962). A revised medium for rapid growth and bio assays with tobacco tissue cultures. Physiol. Plant 15, 473–497. doi: 10.1111/j.1399-3054.1962.tb08052.x
Mysore, K. S., Crasta, O. R., Tuori, R. P., Folkerts, O., Swirsky, P. B., Martin, G. B., et al. (2002). Comprehensive transcript profiling of pto- and prf-mediated host defense responses to infection by Pseudomonas syringae pv. Tomato Plant J. 32, 299–315. doi: 10.1046/j.1365-313x.2002.01424.x
Nguyen, L. T., Schmidt, H. A., von Haeseler, A., and Minh, B. Q. (2015). Iq-tree: a fast and effective stochastic algorithm for estimating maximum-likelihood phylogenies. Mol. Biol. Evol. 32, 268–274. doi: 10.1093/molbev/msu300
Okada, T., Nakayama, H., Shinmyo, A., and Yoshida, K. (2008). Expression of OsHAK genes encoding potassium ion transporters in rice. Plant Biotechnol. 25, 241–245. doi: 10.5511/plantbiotechnology.25.241
Peters, S., Draeger, S., Aust, H., and Schulz, B. (1998). Interactions in dual cultures of endophytic fungi with host and nonhost plant calli. Mycologia 90, 360–367. doi: 10.1080/00275514.1998.12026919
Posada, D. (2008). Jmodeltest: phylogenetic model averaging. Mol. Biol. Evol. 25, 1253–1256. doi: 10.1093/molbev/msn083
Rana, K. L., Kour, D., Kaur, T., Devi, R., and Yadav, A. N. (2020). Endophytic microbes: biodiversity, plant growth-promoting mechanisms and potential applications for agricultural sustainability. Antonie Van Leeuwenhoek 113, 1075–1107. doi: 10.1007/s10482-020-01429-y
Rigobelo, E. C., and Baron, N. C. (2021). Endophytic fungi: a tool for plant growth promotion and sustainable agriculture. Mycology 1–17. doi: 10.1080/21501203.2021.1945699
Ronquist, F., Teslenko, M., van der Mark, P., Ayres, D. L., and Darling, A. (2012). Mrbayes 3.2: efficient bayesian phylogenetic inference and model choice across a large model space. Syst. Biol. 61, 539–542. doi: 10.1093/sysbio/sys029
Schmittgen, T. D., and Livak, K. J. (2008). Analyzing real-time PCR data by the comparative CT method. Nat. Protoc. 3, 1101–1108. doi: 10.1038/nprot.2008.73
Schulz, B., and Boyle, C. (2005). The endophytic continuum. Mycol. Res. 109, 661–686. doi: 10.1017/s095375620500273x
Stafilov, T., Špirić, Z., Glad, M., Barandovski, L., Bačeva Andonovska, K., Šajn, R., et al. (2020). Study of nitrogen pollution in the Republic of North Macedonia by moss biomonitoring and Kjeldahl method. J. Environ. Sci. Health A Tox Hazard Subst. Environ. Eng. 55, 759–764. doi: 10.1080/10934529
Su, Z. Z., Mao, L. J., Li, N., Feng, X. X., Yuan, Z. L., Wang, L. W., et al. (2013). Evidence for biotrophic lifestyle and biocontrol potential of dark septate endophyte Harpophora oryzae to rice blast disease. PLoS One 8:e61332. doi: 10.1371/journal.pone.0061332
Takai, R., Matsuda, N., Nakano, A., Hasegawa, K., and Akimoto, C. (2002). El5, a rice N-acetylchitooligosaccharide elicitor-responsive Ring-H2 finger protein, is a ubiquitin ligase which functions in vitro in co-operation with an elicitor-responsive ubiquitin-conjugating enzyme, OsUBC5b. Plant J. 30, 447–455. doi: 10.1046/j.1365-313x.2002.01299.x
Tan, R. X., and Zou, W. X. (2001). Endophytes: a rich source of functional metabolites. Nat. Prod. Rep. 18, 448–459. doi: 10.1039/b100918o
Thompson, J. D., Gibson, T. J., Plewniak, F., Jeanmougin, F., and Higgins, D. G. (1997). The clustal_x windows interface: flexible strategies for multiple sequence alignment aided by quality analysis tools. Nucl. Acids Res. 25, 4876–4882. doi: 10.1093/nar/25.24.4876
Tsuda, K., and Somssich, I. E. (2015). Transcriptional networks in plant immunity. New Phytol. 206, 932–947. doi: 10.1111/nph.13286
Vergara, C., Araujo, K., Sperandio, M., Santos, L. A., Urquiaga, S., Zilli, J., et al. (2019). Dark septate endophytic fungi increase the activity of proton pumps, efficiency of 15N recovery from ammonium sulphate, N content, and micronutrient levels in rice plants. Braz. J. Microbiol. 50, 825–838. doi: 10.1007/s42770-019-00092-4
Verma, V. C., Kharwar, R. N., and Strobel, G. A. (2009). Chemical and functional diversity of natural products from plant associated endophytic fungi. Nat. Prod. Commun. 4, 1511–1532
Wang, Q., Chen, X., Chai, X., Xue, D., Zheng, W., Shi, Y., et al. (2019). The involvement of jasmonic acid, ethylene, and salicylic acid in the signaling pathway of Clonostachys rosea-induced resistance to gray mold disease in tomato. Phytopathology 109, 1102–1114. doi: 10.1094/PHYTO-01-19-0025-R
White, J. F., Kingsley, K. L., Zhang, Q., Verma, R., Obi, N., Verma, S. K., et al. (2019). Review: endophytic microbes and their potential applications in crop management. Pest Manag. Sci. 75, 2558–2565. doi: 10.1002/ps.5527
Wu, F. L., Li, Y., Tian, W., Sun, Y., Chen, F., Wang, L., et al. (2020). A novel dark septate fungal endophyte positively affected blueberry growth and changed the expression of plant genes involved in phytohormone and flavonoid biosynthesis. Tree Physiol. 40, 1080–1094. doi: 10.1093/treephys/tpaa047
Xiao, M., Liu, R., Ruan, Y., and Liu, C. (2019). Sodium chloride primes JA-independent defense against Spodoptera litura (Fabricius) larvae in Arabidopsis thaliana. Plant Signal. Behav. 14:1607466. doi: 10.1080/15592324.2019.1607466
Yang, Z., Tian, L., Latoszek-Green, M., Brown, D., and Wu, K. (2005). Arabidopsis ERF4 is a transcriptional repressor capable of modulating ethylene and abscisic acid responses. Plant Mol. Biol. 58, 585–596. doi: 10.1007/s11103-005-7294-5
You, Y. H., Yoon, H., Kang, S. M., Woo, J. R., Choo, Y. S., Lee, I. J., et al. (2013). Cadophora malorum Cs-8-1 as a new fungal strain producing gibberellins isolated from Calystegia soldanella. J. Basic Microbiol. 53, 630–634. doi: 10.1002/jobm.201200002
Yuan, Z. L., Lin, F. C., Zhang, C. L., and Kubicek, C. P. (2010). A new species of Harpophora (Magnaporthaceae) recovered from healthy wild rice (Oryza granulata) roots, representing a novel member of a beneficial dark septate endophyte. FEMS Microbiol. Lett. 307, 94–101. doi: 10.1111/j.1574-6968.2010.01963.x
Keywords: endophytic fungi, Pseudophialophora, symbiosis, growth promotion, disease resistance
Citation: Zhu J-N, Yu Y-J, Dai M-D, Zeng Y-L, Lu X-J, Wang L, Liu X-H, Su Z-Z and Lin F-C (2022) A New Species in Pseudophialophora From Wild Rice and Beneficial Potential. Front. Microbiol. 13:845104. doi: 10.3389/fmicb.2022.845104
Received: 29 December 2021; Accepted: 26 January 2022;
Published: 11 March 2022.
Edited by:
Zhiyong Li, Shanghai Jiao Tong University, ChinaReviewed by:
Laith Khalil Tawfeeq Al-Ani, Universiti Sains Malaysia, MalaysiaCopyright © 2022 Zhu, Yu, Dai, Zeng, Lu, Wang, Liu, Su and Lin. This is an open-access article distributed under the terms of the Creative Commons Attribution License (CC BY). The use, distribution or reproduction in other forums is permitted, provided the original author(s) and the copyright owner(s) are credited and that the original publication in this journal is cited, in accordance with accepted academic practice. No use, distribution or reproduction is permitted which does not comply with these terms.
*Correspondence: Zhen-Zhu Su, enpzdUB6anUuZWR1LmNu; Fu-Cheng Lin, ZnVjaGVuZ2xpbkB6anUuZWR1LmNu
Disclaimer: All claims expressed in this article are solely those of the authors and do not necessarily represent those of their affiliated organizations, or those of the publisher, the editors and the reviewers. Any product that may be evaluated in this article or claim that may be made by its manufacturer is not guaranteed or endorsed by the publisher.
Research integrity at Frontiers
Learn more about the work of our research integrity team to safeguard the quality of each article we publish.