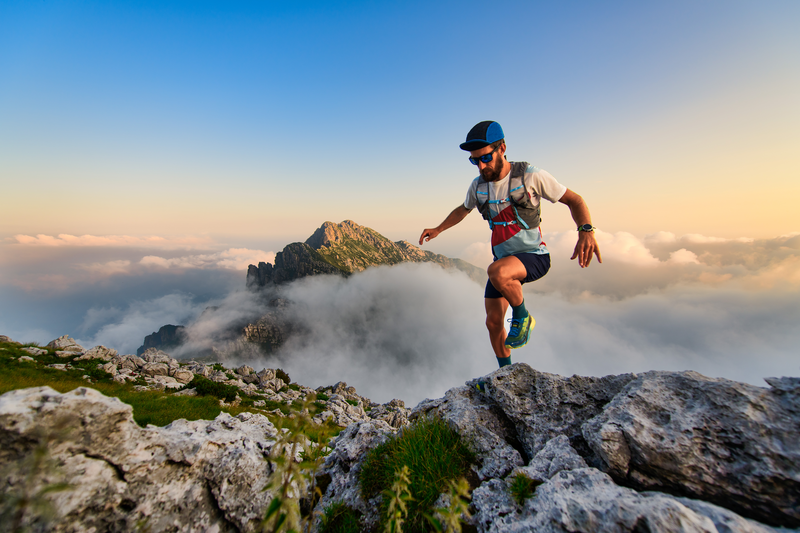
95% of researchers rate our articles as excellent or good
Learn more about the work of our research integrity team to safeguard the quality of each article we publish.
Find out more
ORIGINAL RESEARCH article
Front. Microbiol. , 01 April 2022
Sec. Virology
Volume 13 - 2022 | https://doi.org/10.3389/fmicb.2022.838047
This article is part of the Research Topic Community Series in Plant Viruses: Molecular Plant Virus Epidemiology and its Management, Volume III View all 6 articles
Two distinct emaraviruses, Pigeonpea sterility mosaic virus-I (PPSMV-I) and Pigeonpea sterility mosaic virus-II (PPSMV-II) were found to be associated with sterility mosaic disease (SMD) of pigeonpea [Cajanus cajan (L.) Millsp.]. The host range of both these viruses and their vector are narrow, confined to Nicotiana benthamiana identified through mechanical transmission, and to Phaseolus vulgaris cvs. Top Crop, Kintoki, and Bountiful (F: Fabaceae) through mite transmission. A weed host Chrozophora rottleri (F: Euphorbiaceae) was also infected and tested positive for both the viruses in RT-PCR. Among the wild Cajanus species tested, Cajanus platycarpus accessions 15661, 15668, and 15671, and Cajanus scarabaeoides accessions 15683, 15686, and 15922 were infected by both the viruses and mite vector suggesting possible sources of SMD inoculum. Though accession 15666 of C. platycarpus, 15696 of C. scarabaeoides, and 15639 of Cajanus lanceolatus were infected by both the viruses, no mite infestation was observed on them. Phylogenetic analysis of nucleotide sequences of RNA-1 and RNA-2 of PPSMV-I and PPSMV-II isolates in southern India revealed significant divergence especially PPSMV-II, which is closely related to the Fig mosaic virus (FMV) than PPSMV-I. In multilocation testing of pigeonpea genotypes for their broad-based resistance to SMD for two consecutive years, genotypes ICPL-16086 and ICPL-16087 showed resistance reaction (<10% incidence) in all three locations studied. Overall, the present study gives a clear idea about the host range of PPSMV-I and PPSMV-II, their molecular relationship, and sources of resistance. This information is critical for the development of reliable diagnostic tools and improved disease management strategies.
Sterility mosaic disease (SMD) is one of the important production constraints of pigeonpea cultivation in the Indian subcontinent (Mitra, 1931; Reddy et al., 1998). SMD is caused by two distinct emaraviruses, Pigeonpea sterility mosaic virus-I (PPSMV-I) and Pigeonpea sterility mosaic virus-II (PPSMV-II; Elbeaino et al., 2014, 2015; Kumar et al., 2017; Patil et al., 2017; Sayiprathap et al., 2020) and transmitted by an eriophyid mite Aceria cajani Channabasavanna (Acari: Arthropoda) in a semi-persistence manner (Kulkarni et al., 2002). SMD symptoms include yellow mosaic or chlorotic ring spots, reduced leaf size, stunting, excessive vegetative growth, and partial-to-complete cessation of flowering (sterility). Generally, the nature and severity of symptoms depend on the host genotype and stage of infection (Jones et al., 2004; Patil and Kumar, 2015).
Though SMD was first reported in 1931 from the Bihar state of India (Mitra, 1931), its etiology remains a mystery for several decades until Kumar et al. (1999; 2000; 2001a) unfolded the causal agent for SMD of pigeonpea as Pigeonpea sterility mosaic virus (PPSMV, later renamed as PPSMV-I), a putative RNA virus of negative orientation. Complete genome sequences of PPSMV-I was reported to contain four to five RNA segments (RNA-1 to RNA-5) and PPSMV-II with six RNA segments (RNA-1 to RNA-6) have been published recently (Elbeaino et al., 2015; Kumar et al., 2017; Patil et al., 2017). Based on the genome organization and morphological features, both the viruses were taxonomically included in the genus Emaravirus in the recently created family Fimoviridae of order Bunyavirales (Elbeaino et al., 2018). Preliminary field observations suggest that PPSMV-I was associated with chlorotic ring spots and line patterns, whereas PPSMV-II induces leaf mosaic, stunting, and sterility symptoms. A more severe form of SMD was shown by plants with mixed infection of both the viruses and more frequently occurs in nature (Elbeaino et al., 2015; Patil et al., 2017). The vector, eriophyid mite, is host specific with a narrow host range confined to pigeonpea and few of its wild relatives (Kumar et al., 2003). It is the sole vector responsible for the transmission of SMD in pigeonpea (Seth, 1962; Reddy et al., 1998; Kulkarni et al., 2002; Jones et al., 2004; Kumar et al., 2004; Patil and Kumar, 2015). Though few alternative hosts of PPSMV have been reported, these hosts are not congenial for vector multiplication (Kumar et al., 2002a; Kulkarni et al., 2003a). The source of resistance to SMD was first reported in a pigeonpea landrace, “Sabour 2E” in India (Alam, 1933). Subsequently, several disease-resistant and tolerant lines were identified. Efforts on identifying the sources of resistance to SMD were initiated at ICRISAT in 1975. Over 13,015 pigeonpea accessions from the global pigeonpea germplasm collection at ICRISAT were screened for SMD, and 326 resistant and 97 tolerant lines were reported (Nene, 1995). Recently, 28 pigeonpea genotypes have been identified as resistant to SMD from a preliminary screening of 976 pigeonpea accessions evaluated at eight different geographical locations in India (Sharma et al., 2015).
Studies on the host ranges of PPSMV-I and PPSMV-II help us develop better management strategies; however, there is little knowledge about the host range of PPSMV and its vector. In a previous study, when there was no information about the two distinct emaraviruses associated with SMD, an attempt was made to study the natural and experimental host range for PPSMV and concluded that a couple of Chrozophora rottleri weed plants tested positive for PPSMV (Kulkarni et al., 2003a). A thorough understanding of the genetic variability of emaraviruses associated with SMD of pigeonpea is essential for the development of reliable and robust diagnostic tools (Kallinen et al., 2009; Walia et al., 2009; Dong et al., 2016; Stewart, 2016). Host-plant resistance is the most viable, realistic, and cost-effective option for the management of any viral disease. However, developing stable resistant varieties of pigeonpea is complicated due to the genetic flexibility of the pathogen, which is affected by location-specific environments (Nene et al., 1989; Amin et al., 1993; Sharma and Pande, 2011; Sharma et al., 2012, 2015). By keeping the above fact in view, we studied a wide range of crop and weed species, including wild Cajanus accessions, to identify the host range of PPSMV. The SMD samples collected from different geographical locations in southern India were analyzed for their molecular relationship between PPSMV-I and PPSMV-II. Furthermore, several pigeonpea genotypes were screened at three distinct geographical locations for two consecutive years in order to identify their broad-based resistance to SMD.
Pigeonpea sterility mosaic virus culture was maintained on susceptible pigeonpea cultivar ICP 8863 (Maruti) in a glasshouse at 27 ± 1°C with 70–80% relative humidity. The leaf stapling technique (Nene and Reddy, 1976) was used to inoculate 12- to 15-day-old healthy pigeonpea seedlings.
Sterility mosaic disease-infected young leaf tissue was ground in 0.05 M phosphate buffer (1:10 w/v) containing 0.1% β-mercaptoethanol (β-ME) using mortar and pestle on an ice bucket, filtered through a muslin cloth, and inoculated immediately onto a test plant at the two- to three-leaf stage by dusting celite (abrasive) (Sigma-Aldrich) with the forefinger. The inoculated leaves were then slowly rinsed with distilled water and kept in a vector-proof glasshouse at 27 ± 1°C.
Leaf-stapling method of transmission of PPSMV was followed as per the protocol described by Nene and Reddy (1976). Young SMD-infected leaflets collected in a moist cloth bag were observed for mite infestation under a binocular microscope to ensure a minimum of 10 mites per leaf. The mite-infested leaflets were then stapled onto test plants at the two- to three-leaf stage in such a way that the undersurface of the diseased leaflet comes in contact with both surfaces of the leaf of the test plant to anchor mites for transfer and their feeding results in PPSMV transmission onto the test plant.
Polyclonal antibodies to PPSMV were developed at ICRISAT, Hyderabad, and were used to detect the virus in plant tissues by direct antigen coating (DAC)-ELISA as per the protocol suggested by Kumar et al. (2003). To minimize the non-specific reactions to host plant antigen, polyclonal antisera were cross-absorbed in healthy pigeonpea (cv. ICP 8863) leaf sap at 10 mg/ml in phosphate-buffered saline containing 0.2% ovalbumin and 2% PVP, at 37°C for 45 min. DAC-ELISA was performed by grinding a leaf sample in carbonate buffer, pH 9.6 (1:10, w/v), and the extract was added to wells of MaxiSorp ELISA plates (Nunc, Thermo Fisher Scientific, Denmark). The cross-absorbed polyclonal antiserum was used at 1:3,000 dilution. Alkaline phosphatase (ALP)-labeled goat anti-rabbit IgG (Sigma) was used at 1:4,000 dilution for detecting the immobilized antigen–antibody complex, and p-nitrophenylphosphate (Sigma) (0.5 mg/ml in 10% diethanolamine buffer, pH 9.8) was added as the substrate. The plate was observed for color changes and recorded as weak positive for light yellow and strongly positive for deep yellow.
Briefly, 100 mg of leaf tissue was ground in liquid nitrogen to a fine powder. Total RNA was extracted using the QIAGEN RNeasy plant mini kit by following the manufacturer’s instructions. The RNA quantity and quality were assessed using a spectrophotometer (NanoDrop 8000, Thermo Fisher Scientific) and stored in a refrigerator at −20°C. RT-PCR was performed as per the protocol suggested by Elbeaino et al. (2015). Total RNA (500 ng) was randomly reverse transcribed by adding 4 μl of 5× M-MuLV buffer (New England Biolabs, Ipswich, MA, United States), 0.5 μl of 10 mM dNTPs, 2 μl of 10 mM DTT, 250 ng of random primer, and 200 U of M-MuLV reverse transcriptase (New England Biolabs, Ipswich, MA, United States) in a final volume of 20 μl for 1 h at 39°C followed by inactivation of the enzyme at 65°C for 20 min. Synthetic oligonucleotide primers (Table 1) were used to amplify RNA segments PPSMV-I and PPSMV-II. Random-primed cDNA (2 μl) was added to 5× Taq polymerase buffer (New England Biolabs, Ipswich, MA, United States) containing MgCl2 to a final concentration of 1 mM, 0.2 mM dNTPs, 0.2 μM of each specific primer, and 1 U of Taq DNA polymerase in a final volume of 25 μl. The PCR mixture tube was incubated by 1 cycle of denaturation at 94°C for 4 min, followed by 35 cycles at 92°C for 30 s, 45–65°C for 30 s, and 72°C for 30 s. The final extension was at 72°C for 7 min. The amplification products were resolved in a 1.2% TBE agarose gel, visualized, and documented by a gel–doc system (Major Science image analyzer).
Seeds of 11 Nicotiana species obtained from the Central Tobacco Research Institute (CTRI), Rajamahendravaram, Andhra Pradesh, India, were used in the study along with five herbaceous plants, such as two pigeonpea cultivars (ICP-8863 and ICP-2376), Phaseolus vulgaris, Vigna unguiculata c-152, and Chenopodium album, and were grown in 8-inch pots and inoculated mechanically with SMD-infected sap and kept inside a glasshouse at 27 ± 1°C. In another set, seeds of 24 accessions of 12 Cajanus species obtained from the gene bank of ICRISAT, India, were scarified by slicing the seed coat with a scalpel blade, treated with thiram at 30 mg/10 g of seeds, and were sown in 8-inch pots along with 16 cultivated species intercropped with pigeonpea and 46 weed species raised to the two- to three-leaf stage and inoculated by leaf stapling method and kept in the glasshouse at 27 ± 1°C with 70–80% relative humidity. The test plants were monitored regularly for symptom appearance and tested for the infection of PPSMV by DAC-ELISA. The ELISA-positive samples were tested further for the infection of PPSMV-I and/or PPSMV-II using oligonucleotide primers corresponding to the RNA-3 segment through RT-PCR.
Pigeonpea leaves exhibiting typical SMD symptoms were collected during a roving survey conducted in 2017/2018 from different geographical locations covering Andhra Pradesh, Karnataka, Tamil Nadu, and Telangana states in southern India. The collected samples were placed in ziplock plastic bags and transported in cold packs to ICRISAT, Hyderabad, snap frozen in liquid nitrogen, and stored in a −80°C freezer.
Total RNA was extracted using the QIAGEN RNeasy plant mini kit by following the manufacturer’s instructions. RT-PCR was performed using oligonucleotide primers (Table 1) to amplify partial RNA-1 and RNA-2 of PPSMV-I and PPSMV-II. Amplicons were purified and sequenced by Sanger’s dideoxy chain-termination method [ABI 3730 (48 capillaries) electrophoresis]. Later, the nucleotide homology searches were done with the BLASTN sequence analysis of the NCBI1. Multiple alignments were performed using MUSCLE (Edgar, 2004), and the phylogenetic tree was constructed by MEGA X (Kumar et al., 2018) employing maximum-likelihood (ML) criterion using the neighbor-joining method, to examine the molecular relationship between and among the isolates of PPSMV-I and PPSMV-II in southern India.
Twenty pigeonpea advanced breeding lines along with SMD-susceptible genotype, ICP 8863 (Maruti), were obtained from the Pigeonpea Breeding Unit, ICRISAT, Patancheru, India. All these genotypes were evaluated for their reaction to SMD at three geographical locations, such as Bengaluru (13°04′48″N 77°34′14″E, altitude-914 m), Coimbatore (11°01′24″N 76°55′45″E, altitude-431 m), and Patancheru (17°30′35″N 78°16′31″E, altitude-547 m), in southern India for two consecutive years (rainy season 2017/2018 and 2018/2019). Plants were raised to the two- to three-leaf stage and inoculated by following the leaf stapling method as described earlier.
The test genotypes were regularly monitored for the symptom expression, SMD incidence was recorded, and percent disease incidence was calculated using the formula:
Based on the SMD incidence, test genotypes were categorized as resistant (≤10.0% incidence), moderately resistant (10.1–20.0% incidence), susceptible (20.1–40.0% incidence), and highly susceptible (>40.0% incidence) (Sharma et al., 2015).
Of the 11 Nicotiana species (Table 2) and five herbaceous plants tested by mechanical sap inoculation, only Nicotiana benthamiana was found positive for PPSMV infection. Symptoms appeared after 35–40 days of post-inoculation (dpi) as chlorotic spots, deformation of leaves, and stunted growth, while in the advanced stage (65–70 dpi), the symptoms were systemic and appeared on young leaves as yellow mosaic and crinkled leaves (Figure 1). However, the PPSMV was not mechanically transmitted onto the pigeonpea. The ELISA-positive samples when tested in RT-PCR were found infected with both the viruses (PPSMV-I and PPSMV-II) (Figure 2).
Table 2. Reaction of Nicotiana species to Pigeonpea sterility mosaic virus (PPSMV) by sap inoculation transmission.
Figure 1. Experimental host range of Pigeonpea sterility mosaic virus (PPSMV). Healthy Nicotiana benthamiana (A), sap inoculated PPSMV affected N. benthamiana exhibiting yellow mosaic symptoms (B).
Figure 2. Resolution of reverse transcription-polymerase chain reaction (RT-PCR) products of RNA-3 segment of Pigeonpea sterility mosaic virus I (PPSMV-I) (A) and Pigeonpea sterility mosaic virus II (PPSMV-II) (B) in 1.2% agarose gels. Standard 100-bp DNA marker (lane M), pigeonpea ICP 8863 (lane 1), pigeonpea ICP 2376 (lane 2), Nicotiana benthamiana (lane 3), N. clevelandii (lane 4), Macroptilium atropurpureum (lane 5), Phaseolus vulgaris cv. Top Crop (lane 6), P. v. cv. Bountiful (lane 7), P. v. cv. Kintoki (lane 8), Chrozophora rottleri (lane 9), Hibiscus panduriformis (lane 10).
When 16 cultivated crop species and 46 weed species were tested (Table 3) by leaf stapling method inoculation, Phaseolus vulgaris cvs. Top Crop, Bountiful, and Kintoki (F: Fabaceae) were infected with PPSMV but not supported for mite multiplication. The symptoms appeared as stunting, reduced leaf size, and mild crinkling. A weed host, Chrozophora rottleri (F: Euphorbiaceae) was also tested positive for PPSMV infection; however, there were no visual symptoms of virus infection or mites. A member of Fabaceae, Macroptilium atropurpureum, was found infested with a few mites but tested negative for PPSMV. Similar to the Nicotiana species, all the ELISA-positive samples when tested in RT-PCR were found infected with both the viruses (Figure 2). Though P. vulgaris cvs. Top Crop, Bountiful, Kintoki, and C. rottleri were found infected with the virus, they have not supported mite multiplication, and therefore, were not considered to be a potential source of inoculum for SMD.
Among the 24 accessions of 12 wild Cajanus species tested for PPSMV infection and mite infestation (Table 4), the accessions 15661, 15668, and 15671 of Cajanus platycarpus and 15683, 15686, and 15922 of C. scarabaeoides were found positive for both the virus and mite vector. So, in nature, these can act as potential sources of SMD inoculum multiplication, whereas accession 15666 of C. platycarpus, 15696 of C. scarabaeoides, and 15639 of C. lanceolatus were infected with the virus though no mites were observed on them. While accessions 15614 and 15620 of Candida albicans and 15874 of Cajanus cinereus supported mite multiplication, no infection of PPSMV was found. The rest of the Cajanus spp. accessions were neither supportive of mite multiplication nor PPSMV infection (Figure 3). The accessions that are tested positive in ELISA were confirmed for the infection of both the viruses in RT-PCR (Figure 4).
Figure 3. Typical sterility mosaic disease (SMD) symptoms on pigeonpea as yellow mosaic (A), chlorotic spots (B), mite-inoculated PPSMV infected wild Cajanus accessions exhibiting SMD symptoms (C–F).
Figure 4. RT-PCR amplified product of RNA-3 segment of PPSMV-I (A) and PPSMV-II (B) from wild Cajanus accessions in 1.2% agarose gels. Standard 100-bp DNA marker (lane M), Cajanus cajan (lane 1), C. cinereus 15874 (lane 2), C. lanceolatus 15639 (lane 3), C. marmoratus 15651 (lane 4), C. scarabaeoides 15696 (lane 5), C. scarabaeoides 15711 (lane 6), C. scarabaeoides 15922 (lane 7), C. scarabaeoides 15683 (lane 8), C. scarabaeoides 15686 (lane 9), C. platycarpus 15666 (lane 10), C. platycarpus 15668 (lane 11), C. platycarpus 15671 (lane 12), C. platycarpus 15664 (lane 13), C. platycarpus 15661 (lane 14), C. sericeus 15760 (lane 15), C. sericeus 15762 (lane 16), C. mollis 15658 (lane 17), C. reticulatus 15675 (lane 18), C. acutifolius 15603 (lane 19), C. acutifolius 15611 (lane 20), C. albicans 15614 (lane 21), C. albicans 15620 (lane 22), C. crassus 15767 (lane 23), C. crassus 15768 (lane 24), and C. confertiflorus 15674 (lane 25).
The oligonucleotide primers targeted for RNA-1 and RNA-2 of PPSMV-I and PPSMV-II resulted in amplicons of distinct sizes (Figure 5). The nucleotide sequences of RNA-1 when subjected to phylogenetic analysis along with available corresponding sequences, the isolates of PPSMV-I and PPSMV-II, formed two separate and distinct clusters (Figure 6). The isolates Coimbatore-A BRS1117, Coimbatore-B BRS1117, Bengaluru BRS1117, Raichur BRS1117, Patancheru BRS1117, and Chevella BRS1117 of PPSMV-II clustered into a distinct subcluster, and the isolate Tandur BRS1117 distinctly separated. The RNA-1 sequence of Fig mosaic virus (FMV) also clustered with PPSMV-II isolates, whereas the RNA-1 of PPSMV-I isolates Gulbarga BRS1117 and Bengaluru BRS1117 clustered together, and the isolates Tirupati BRS1117, Vamban BRS1117, Patancheru BRS1117, and Tandur BRS1117 clustered in another subcluster. Similarly, distinct clusters were formed when the RNA-2 sequences of PPSMV-I and PPSMV-II were subjected to the phylogenetic analysis in which the RNA-2 sequence of PPSMV-I isolate Coimbatore BRS1117 and PPSMV-II isolate Gulbarga BRS1117 were distinctly separated from the rest of the isolates. The RNA-2 sequence of FMV is also clustered with PPSMV-II isolates. The RNA-1 and RNA-2 sequences of PPSMV-I and PPSMV-II isolates were phylogenetically closer. However, RNA-1 and RNA-2 sequences of PPSMV-II isolates exhibited a close relationship with FMV than the PPSMV-I.
Figure 5. Resolution of RT-PCR products of RNA-1 and RNA-2 segments of PPSMV-I (A) and PPSMV-II (B) in 1.2% agarose gels. Standard 100-bp DNA marker (lane M), healthy pigeonpea leaf sample (lane H), lanes 1–7 are the SMD-affected pigeonpea samples from different geographical locations in southern India that were analyzed.
Figure 6. Phylogenetic analysis of nucleotide sequences of RNA-1 (A) and RNA-2 (B) of PPSMV-I and PPSMV-II along with available corresponding sequences. The Gene Bank accession numbers are given in parenthesis. The scale bar represents 0.2 and 0.5 substitutions per nucleotide position for RNA-1 and RNA-2 sequences, respectively. Symbols ▲ and ▼ represents virus isolates analyzed in the present study.
Screening of pigeonpea genotypes to SMD in different geographic locations revealed considerable variations in response to SMD infection (Table 5). Susceptible check (ICP 8863) showed a highly susceptible reaction (>40% incidence) in all three locations. The susceptible test genotypes exhibited typical SMD symptoms between 15 and 18 days after postinoculation (dpi). Among the test entries, ICPL-16086 and ICPL-16087 showed resistance reactions (<10% incidence) in all three locations, whereas genotypes ICPL-16078 and ICPL-16079 showed resistance reaction at the Bengaluru and Coimbatore locations, while at the Patancheru location showed moderate (10.1–20.0% incidence) and susceptible (20.1–40% incidence) reactions, respectively. The genotypes ICPL-16072, ICPL-16077, and ICPL-16083 expressed resistant reaction at the Coimbatore and Patancheru locations, but highly susceptible reaction at the Bengaluru location. ICPL-16050 and ICPL-16052 exhibited resistant reaction in Coimbatore, while they exhibited highly susceptible reaction at both the Bengaluru and Coimbatore locations.
Table 5. Screening of pigeonpea advanced breeding lines for their reaction to sterility mosaic disease (SMD) at different geographic locations during the rainy seasons of 2017/2018 and 2018/2019.
Our study described the host range of PPSMV-I and PPSMV-II, the molecular relationship between them, and the source of resistance to SMD. The host range of PPSMV is narrow and confined to Nicotiana benthamiana through sap inoculation. The evidence of susceptibility of N. benthamiana to a wide range of plant viruses has been provided by Yang et al. (2004) as it has been linked to a naturally occurring mutation in an RNA-dependent RNA polymerase (RdRp) gene in the genome. The present study followed Kumar et al. (2002a; 2002b) and Manjunatha et al. (2018) who successfully transmitted PPSMV onto N. benthamiana and Nicotiana clevelandii by sap inoculation, but not on to the pigeonpea or any herbaceous hosts. Through mite inoculation, both the viruses can be successfully transmitted onto Phaseolus vulgaris cvs. Top Crop, Bountiful, Kintoki (F: Fabaceae) and Chrozophora rottleri (F: Euphorbiaceae), and was confirmed by the reports of Kulkarni et al. (2003a) and Kumar et al. (2004). However, there were contradicting observations of mite infestation on Hibiscus panduriformis, as in our study, neither the mite infestation nor PPSMV infection was found in both the field-collected samples as well as in artificially inoculated plants of H. panduriformis. Among the 24 accessions of 12 wild Cajanus species tested for the PPSMV infection, the accessions 15661, 15668, and 15671 of C. platycarpus and 15683, 15686, and 15922 of C. scarabaeoides were positive for both the viruses and supported mite multiplication, confirming earlier reports of Kulkarni et al. (2003b) and Kumar et al. (2005) that they can harbor the virus and vector and act as potential sources of inoculum in the field.
The study of the diversity of PPSMV-I and PPSMV-II associated with SMD of pigeonpea showed that these two emaraviruses are widespread across southern India. Analysis of sequence identity among the isolates of PPSMV-I and PPSMV-II indicated the presence of significant sequence variability. The RNA-1 and RNA-2 sequences of PPSMV-II isolates exhibited a close relationship with FMV than with PPSMV-I, and it is convincing and in agreement with the previous reports of Elbeaino et al. (2015); Kumar et al. (2017), Patil et al. (2017), and Sayiprathap et al. (2020) suggesting that these two emaraviruses infecting pigeonpea have followed two independent evolutionary paths. Patil et al. (2017) reported the prevalence of the PPSMV-II in the Coimbatore and Bengaluru locations. However, when we analyzed samples from different geographical locations in southern India, there is an existence of both the viruses in the Coimbatore and Bengaluru locations. This development of mixed infection over the years is possibly due to the spread of the virus to these locations by its mite vector, A. cajani. In nature, mites are the only means of transfer of SMD causal agent to pigeonpea and not through seed, pollen, or soil (Seth, 1962; Reddy et al., 1998; Kulkarni et al., 2002; Jones et al., 2004; Kumar et al., 2004; Patil and Kumar, 2015).
Host-plant resistance is the most viable and cost-effective option for the management of any viral disease. Though several researchers identified resistant sources to SMD in the past, most of their studies involved evaluation in one location with one isolate/strain (Nene, 1995). In contrast, our efforts led to the identification of two resistant genotypes such as ICPL-16086 and ICPL-16087 with broad-based resistance to SMD. In the present study, we also found high susceptibility of pigeonpea genotypes at the Bengaluru location, so the Bengaluru isolate could be considered as the severe strain in causing SMD in pigeonpea, and this was confirmed in an earlier report too (Nene et al., 1989). The variation in the disease reaction in different locations, attributed to different eriophyid mite vectors, was ruled out previously as Kumar et al. (2001b) reported that there is only one biotype present in India, which is transferring SMD to pigeonpea. So, the possible variation in our study is mainly due to virus variants, as Reddy et al. (1993) identified five distinct virus variants in India with different levels of virulence. There are conflicting reports about the genetics of resistance to SMD claiming both resistance and susceptibility to being dominant. Nevertheless, in most cases, susceptibility was shown to be dominant, and resistance is controlled by recessive genes (Singh and Vishwadhar, 2003). The resistance to SMD has been reported to be controlled by a single recessive gene (Srinivas et al., 1997) with oligogenic nature (Sharma et al., 1984; Gnanesh et al., 2011).
The datasets presented in this study can be found in online repositories. The names of the repository/repositories and accession number(s) can be found in the article/online.
BS conceptualized the work in discussion with AP and HS, executed the work in the field and glasshouse, and wrote the initial draft of the manuscript. VP, KJ, KS, and MS provided necessary inputs in designing the work plan. HR, ER, and LK provided all necessary support in the execution of the work in various locations. All authors contributed to the article and approved the submitted version.
This work was supported by the CGIAR Research Program on Grain Legumes and Dryland Cereals (CRP-GLDC).
The authors declare that the research was conducted in the absence of any commercial or financial relationships that could be construed as a potential conflict of interest.
All claims expressed in this article are solely those of the authors and do not necessarily represent those of their affiliated organizations, or those of the publisher, the editors and the reviewers. Any product that may be evaluated in this article, or claim that may be made by its manufacturer, is not guaranteed or endorsed by the publisher.
This work has been undertaken as part of the CGIAR Research Program—Grain Legumes and Dryland Cereals (CRP-GLDC) of ICRISAT. We thank the Central Tobacco Research Institute (CTRI), Rajamahendravaram, Andhra Pradesh, India, for providing the Nicotiana species seed material. We are also thankful to C. V. Sameer Kumar and Anupama Hingane for providing the seeds of pigeonpea advanced breeding lines, and Vijay Krishna Kumar Kotamraju of the Acharya N. G. Ranga Agricultural University for critical reading of this manuscript and suggestions to improve it. BS is thankful to the University Grants Commission (UGC) for providing research fellowships (Junior Research Fellowship and Senior Research Fellowship).
Alam, M. (1933). “Arhar sterility,” in Proceedings of the 20th Annual Meeting of the Indian Science Congress, Poona, Vol. 43, 15–16.
Amin, K. S., Reddy, M. V., Nene, Y. L., Raju, T. N., Shukla, P., Zote, K. K., et al. (1993). Multi-location evaluation of pigeonpea (Cajanus cajan) for broad-based resistance to sterility-mosaic disease in India. Indian J. Agric. Sci. 63, 542–546.
Dong, L., Lemmety, L., Latvala, S., Samuilova, O., and Valkonen, J. P. T. (2016). Occurrence of genetic diversity of Raspberry leaf blotch virus (RLBV) infecting cultivated and wild Rubus species in Finland. Ann. Appl. Biol. 168, 122–132. doi: 10.1111/aab.12247
Edgar, R. C. (2004). MUSCLE: multiple sequence alignment with high accuracy and high throughput. Nucleic Acids Res. 32, 1792–1797. doi: 10.1093/nar/gkh340
Elbeaino, T., Digiaro, M., Mielke-Ehret, N., Muehlbach, H., Martelli, G. P., and ICTV report consortium (2018). ICTV virus taxonomy profile: Fimoviridae. J. Gen. Virol. 99, 1478–1479. doi: 10.1099/jgv.0.001143
Elbeaino, T., Digiaro, M., Uppala, M., and Sudini, H. (2014). Deep sequencing of Pigeonpea sterility mosaic virus discloses five RNA segments related to Emaraviruses. Virus Res. 188, 27–31. doi: 10.1016/j.virusres.2014.03.022
Elbeaino, T., Digiaro, M., Uppala, M., and Sudini, H. (2015). Deep sequencing of dsRNAs recovered from mosaic-diseased pigeonpea reveals the presence of a novel Emaravirus, Pigeonpea sterility mosaic virus 2. Virus Res. 160, 2019–2029. doi: 10.1007/s00705-015-2479-y
Gnanesh, B. N., Ganapathy, K. N., Ajay, B. C., and Byre Gowda, M. (2011). Inheritance of sterility mosaic disease resistance to Bangalore and Patancheru isolates in pigeonpea (Cajanus cajan (L.) Millsp.). Electron. J. Plant Breed. 2, 218–223.
Jones, A. T., Kumar, P. L., Saxena, K. B., Kulkarni, N. K., Muniyappa, V., and Waliyar, F. (2004). Sterility mosaic disease- the Green Plague of pigeonpea, advances in understanding the etiology, transmission and control of a major virus disease. Plant Dis. 88, 436–445. doi: 10.1094/pdis.2004.88.5.436
Kallinen, A. K., Lindberg, I. L., Tagume, A. K., and Valkonen, J. P. (2009). Detection, distribution, and genetic variability of European mountain ash ring-spot associated virus. Phytopathology 99, 344–352. doi: 10.1094/PHYTO-99-4-0344
Kulkarni, N. K., Kumar, P. L., Muniyappa, V., Jones, A. T., and Reddy, D. V. R. (2002). Transmission of Pigeonpea sterility mosaic virus by the eriophyid mite, Aceria cajani (Acari: Arthropoda). Plant Dis. 86, 1297–1302. doi: 10.1094/PDIS.2002.86.12.1297
Kulkarni, N. K., Kumar, P. L., Muniyappa, V., Jones, A. T., and Reddy, D. V. R. (2003a). Studies on the natural and experimental host range of Pigeonpea sterility mosaic virus. J. Mycol. Plant Pathol. 33, 141–145.
Kulkarni, N. K., Reddy, A. S., Kumar, P. L., Vijaynarasimha, J., Rangaswamy, K. T., Muniyappa, V., et al. (2003b). Broad-based resistance to pigeonpea sterility mosaic disease in the accessions of Cajanus scarabaeoides. Indian J. Plant Prot. 31, 6–11.
Kumar, P. L., Jones, A. T., and Reddy, D. V. R. (2002a). Mechanical transmission of Pigeonpea sterility mosaic virus. J. Mycol. Plant Pathol. 32, 88–89.
Kumar, P. L., Duncan, G. H., Roberts, I. M., Jones, A. T., and Reddy, D. V. R. (2002b). Cytopathology of Pigeonpea sterility mosaic virus in pigeonpea and Nicotiana benthamiana: similarities with those of eriophyid mite-borne agents of undefined aetiology. Ann. Appl. Biol. 140, 87–96. doi: 10.1111/j.1744-7348.2002.tb00160.x
Kumar, P. L., Jones, A. T., Sreenivasulu, P., Fenton, B., and Reddy, D. V. R. (2001a). Characterization of a virus from pigeonpea with affinities to species in the genus Aureusvirus, Family Tombusviridae. Plant Dis. 85, 208–215. doi: 10.1094/PDIS.2001.85.2.208
Kumar, P. L., Fenton, B., Duncan, G., Jones, A. T., Sreenivasulu, P., and Reddy, D. V. R. (2001b). Assessment of variation in Aceria cajani (Acari: Eriophyidae) using analysis of nuclear rDNA ITS regions and scanning electron microscopy: implications for the variability observed in host plant resistance to pigeonpea sterility mosaic disease. Ann. Appl. Biol. 139, 61–73. doi: 10.1111/j.1744-7348.2001.tb00131.x
Kumar, P. L., Jones, A. T., and Reddy, D. V. R. (2003). A novel mite-transmitted virus with a divided RNA genome closely associated with pigeonpea sterility mosaic disease. Phytopathology 93, 71–81. doi: 10.1094/PHYTO.2003.93.1.71
Kumar, P. L., Jones, A. T., and Waliyar, F. (2004). Biology, etiology and management of pigeonpea sterility mosaic disease. Annu. Rev. Plant Pathol. 3, 1–24.
Kumar, P. L., Jones, A. T., Sreenivasulu, P., and Reddy, D. V. R. (2000). Breakthrough in the identification of the causal virus of pigeonpea sterility mosaic disease. J. Mycol. Plant Pathol. 30:249.
Kumar, P. L., Jones, A. T., Sreenivasulu, P., and Reddy, D. V. R. (1999). “Unfolding the mystery of the causal agent of pigeonpea sterility mosaic disease,” in Proceedings of the International Conference on Life Sciences in Next Millennium, 11-14 December 1999, (Hyderabad: University of Hyderabad, India), 65–69.
Kumar, P. L., Latha, T. K. S., Kulkarni, N. K., Raghavendra, N., Saxena, K. B., Waliyar, F., et al. (2005). Broad-based resistance to pigeonpea sterility mosaic disease in wild relatives of pigeonpea (Cajanus: Phaseoleae). Ann. Appl. Biol. 146, 371–379. doi: 10.1111/J.1744-7348.2005.040091.X
Kumar, S., Stecher, G., Li, M., Knyaz, C., and Tamura, K. (2018). MEGA X: molecular evolutionary genetic analysis across computing platforms. Mol. Biol. Evol. 35, 1547–1549. doi: 10.1093/molbev/msy096
Kumar, S., Subbarao, B. L., and Vipin, H. (2017). Molecular characterization of Emaraviruses associated with pigeonpea sterility mosaic disease. Nature Sci. Rep. 7:11831. doi: 10.1038/s41598-017-11958-8
Manjunatha, L., Ramappa, H. K., and Rangaswamy, K. T. (2018). Role of leaf morphology in defense against sterility mosaic disease of pigeonpea. J. Environ. Biol. 39, 298–305. doi: 10.22438/jeb/39/3/MRN-389
Mitra, M. (1931). “Report of the imperial mycologist. Scientific report of the agricultural research institute, Pusa, New Delhi, India. “Mosaic of pigeonpea”,” in Plant Diseases of International Importance: Diseases of Cereals and Pulses, Vol. 19, eds U. S. Singh, A. N. Mukhopadhyay, J. Kumar, and H. S. Chaube (New Jersey, NJ: Prentice Hall), 58–71.
Nene, Y. L. (1995). Sterility mosaic of pigeonpea: the challenge continues. Indian J. Mycol. Plant Pathol. 25, 1–11.
Nene, Y. L., and Reddy, M. V. (1976). A new technique to screen pigeonpea for resistance to sterility mosaic. Trop. Grain Legume Bull. 5, 23–24.
Nene, Y. L., Reddy, M. V., Beniwal, S. P. S., Mahmood, M., Zote, K. K., Singh, R. N., et al. (1989). Multilocational testing of pigeonpea for broad-based resistance sterility mosaic in India. Indian Phytopathol. 42, 444–448.
Patil, B. P., and Kumar, P. L. (2015). Pigeonpea sterility mosaic virus: a legume infecting Emaravirus from South Asia. Mol. Plant Pathol. 16, 775–786. doi: 10.1111/mpp.12238
Patil, B. P., Meenakshi, D., and Ritesh, M. (2017). Variability of Emaravirus species associated with sterility mosaic disease of pigeonpea in India provides evidence of segment reassortment. Viruses 9:183. doi: 10.3390/v9070183
Reddy, M. V., Raju, T. N., and Lenne, J. M. (1998). “Disease of pigeonpea,” in The Pathology of Food and Pasture Legumes, eds D. J. Allen and J. M. Lenne (Patancheru: CABI and ICRISAT).
Reddy, M. V., Raju, T. N., Ghanekar, A. M., Amin, K. S., Arjunan, G., Nene, Y. L., et al. (1993). Variability in sterility mosaic pathogen of pigeonpea in India. Indian Phytopathol. 46, 206–212. doi: 10.1007/s00122-020-03702-0
Sayiprathap, B. R., Patibanda, A. K., Kumari, V. P., Jayalalitha, K., Rao, S. V., Sharma, M., et al. (2020). Prevalence of sterility mosaic disease (SMD) and variability in Pigeonpea sterility mosaic virus (PPSMV) in southern-India. Indian Phytopathol. 73, 741–750. doi: 10.1007/s42360-020-00246-7
Seth, M. L. (1962). Transmission of pigeonpea sterility by an eriophyid mite. Indian Phytopathol. 15, 225–227.
Sharma, D., Gupta, S., Ral, G. S., and Reddy, V. (1984). Inheritance of resistance to sterility mosaic disease in pigeonpea. Indian J. Genet. Plant Breed. 44, 84–90.
Sharma, M., and Pande, S. (2011). New sources of resistance to Fusarium wilt, sterility mosaic disease and Phytophthora blight in vegetable pigeonpea germplasm. Indian J. Plant Prot. 39, 288–293.
Sharma, M., Rathore, A., Mangala, U. N., Ghosh, R., Sharma, S., Upadhyay, H., et al. (2012). New source of resistance to fusarium wilt and sterility mosaic disease in a mini-core collection of pigeonpea germplasm. Eur. J. Plant Pathol. 133, 707–714. doi: 10.1007/s10658-012-9949-9
Sharma, M., Telangre, T., Gosh, R., and Pande, S. (2015). Multi-environment field testing to identify broad, stable resistance to sterility mosaic disease of pigeonpea. J. Gen. Plant Pathol. 81, 249–259. doi: 10.1007/s10327-015-0585-z
Singh, I. P., and Vishwadhar, D. R. P. (2003). Inheritance of resistance to sterility mosaic in pigeonpea (Cajanus cajan). Indian J. Agric. Sci. 73, 414–417.
Srinivas, T., Reddy, M. V., Jain, K. C., and Reddy, M. S. S. (1997). Studies on inheritance of resistance and allelic relationship for strain of pigeonpea sterility mosaic pathogen. Ann. Appl. Biol. 130, 105–110. doi: 10.1111/j.1744-7348.1997.tb05786.x
Stewart, L. R. (2016). Sequence diversity of wheat mosaic virus isolates. Virus Res. 213, 299–303. doi: 10.1016/j.virusres.2015.11.013
Walia, J. J., Salem, N. M., and Falk, B. W. (2009). Partial sequence and survey analysis identify a multipartite, negative-sense RNA virus associated with Fig mosaic. Plant Dis. 93, 4–10. doi: 10.1094/PDIS-93-1-0004
Keywords: Cajanus cajan, SMD, PPSMV-I, PPSMV-II, host-range, host-plant resistance (HPR)
Citation: Sayiprathap BR, Patibanda AK, Prasanna Kumari V, Jayalalitha K, Ramappa HK, Rajeswari E, Karthiba L, Saratbabu K, Sharma M and Sudini HK (2022) Salient Findings on Host Range, Resistance Screening, and Molecular Studies on Sterility Mosaic Disease of Pigeonpea Induced by Pigeonpea sterility mosaic viruses (PPSMV-I and PPSMV-II). Front. Microbiol. 13:838047. doi: 10.3389/fmicb.2022.838047
Received: 17 December 2021; Accepted: 24 January 2022;
Published: 01 April 2022.
Edited by:
Rajarshi Kumar Gaur, Deen Dayal Upadhyay Gorakhpur University, IndiaReviewed by:
Juan Jose Lopez-Moya, Centre for Research in Agricultural Genomics (CSIC), SpainCopyright © 2022 Sayiprathap, Patibanda, Prasanna Kumari, Jayalalitha, Ramappa, Rajeswari, Karthiba, Saratbabu, Sharma and Sudini. This is an open-access article distributed under the terms of the Creative Commons Attribution License (CC BY). The use, distribution or reproduction in other forums is permitted, provided the original author(s) and the copyright owner(s) are credited and that the original publication in this journal is cited, in accordance with accepted academic practice. No use, distribution or reproduction is permitted which does not comply with these terms.
*Correspondence: H. K. Sudini, aC5zdWRpbmlAY2dpYXIub3Jn
Disclaimer: All claims expressed in this article are solely those of the authors and do not necessarily represent those of their affiliated organizations, or those of the publisher, the editors and the reviewers. Any product that may be evaluated in this article or claim that may be made by its manufacturer is not guaranteed or endorsed by the publisher.
Research integrity at Frontiers
Learn more about the work of our research integrity team to safeguard the quality of each article we publish.