- 1Department of Biochemistry and Biotechnology, Sardar Bhagwan Singh University, Dehradun, India
- 2M/s Xcel Life Sciences, Fremont, CA, United States
Background: Mushrooms exist as an integral and vital component of the ecosystem and are very precious fungi. Mushrooms have been traditionally used in herbal medicines for many centuries.
Scope and Approach: There are a variety of medicinal mushrooms mentioned in the current work such as Agaricus, Amanita, Calocybe, Cantharellus, Cordyceps, Coprinus, Cortinarius, Ganoderma, Grifola, Huitlacoche, Hydnum, Lentinus, Morchella, Pleurotus, Rigidoporus, Tremella, Trametes sp., etc., which play a vital role in various diseases because of several metabolic components and nutritional values. Medicinal mushrooms can be identified morphologically on the basis of their size, color (white, black, yellow, brown, cream, pink and purple-brown, etc.), chemical reactions, consistency of the stalk and cap, mode of attachment of the gills to the stalk, and spore color and mass, and further identified at a molecular level by Internal Transcribed Spacer (ITS) regions of gene sequencing. There are also other methods that have recently begun to be used for the identification of mushrooms such as high-pressure liquid chromatography (HPLC), nuclear magnetic resonance spectroscopy (NMR), microscopy, thin-layer chromatography (TLC), DNA sequencing, gas chromatography-mass spectrometry (GC-MS), chemical finger printing, ultra-performance liquid chromatography (UPLC), fourier transform infrared spectroscopy (FTIR), liquid chromatography quadrupole time-of-flight mass spectrometry (LCMS-TOF) and high-performance thin-layer chromatography (HPTLC). Lately, the matrix-assisted laser desorption ionization-time of flight mass spectrometry (MALDI-TOF MS) technique is also used for the identification of fungi.
Key Finding and Conclusion: Medicinal mushrooms possess various biological activities like anti-oxidant, anti-cancer, anti-inflammatory, anti-aging, anti-tumor, anti-viral, anti-parasitic, anti-microbial, hepatoprotective, anti-HIV, anti-diabetic, and many others that will be mentioned in this article. This manuscript will provide future direction, action mechanisms, applications, and the recent collective information of medicinal mushrooms. In addition to many unknown metabolites and patented active metabolites are also included.
Highlights
- This manuscript highlights the medicinal mushroom species and their active metabolites.
- Detail of active metabolite and action mechanisms are mentioned.
- Recent technologies used for the identification of mushroom species are described.
- Active metabolite roles in various diseases are included.
- Unknown active metabolites and patented metabolites are also incorporated.
Introduction
Human beings have cultivated and consumed mushrooms as a kind of macro fungi for centuries, due to their attractive characteristics such as their ease of cultivation, and their multiple functional activities (Roupas et al., 2012; Kalac, 2016; Phat et al., 2016; Roncero-Ramos and Delgado-Andrade, 2017). Macro fungi are economically essential because of their importance in medicine, biocontrol, food, and the biological, chemical, and other industries. These macro fungi differ in their uses as medicines and food and several other species form mycorrhizal associations and function as decomposers (Meena et al., 2020).
Fungi that belong to several taxonomic groups which produce conspicuous sporocarps are called macro fungi – these include truffles, gilled fungi, stink fungi, jelly fungi, birds’ nest, coral fungi, puffballs, and bracket fungi (Enow, 2013). These mushrooms live as saprophytes. In the decomposition process, all types of mushrooms are essential due to their ability to degrade cellulose as well as other polymers. Large fungi form large fruitifications that can be visible without the help of a microscope and these include Ascomycota and Basidiomycota having large spore-bearing structures (Al-Thani, 2010). In nature, mushrooms are widespread as well as the earliest form of fungi known to human beings (Okhuoya et al., 2010; Meena et al., 2020).
Mushrooms are seasonal macro fungi mainly found in the rainy season or when the snow melts, and form macroscopic fruiting bodies. Mushrooms can be leathery or woody, fleshy, or sub-fleshy. They bear their fertile surface on the lining of the tubes or on the lamellae and open out by means of pores. Polypores and Boletes are the tube-bearing poroid membranes whereas Agarics are the lamellate members (Deshmukh, 2004). Humans have consumed wild mushrooms from ancient times, with likely considering them a delicacy and also due to their pleasing flavor (Das, 2010). Edible mushrooms are considered to be devoid of undesirable effects and have medicinal values (Barros et al., 2008; Martinez-Medina et al., 2021).
Mushrooms are rich in essential nutrients and have rich nutritional values with high contents dietary of fibers, significant contents of vitamins (B1, B2, B12, C, D, and E), mineral substances, trace elements, a high quality of proteins including important content of essential amino acids. They may however be limited in the cystine, methionine, and sulfur-containing amino acids, carbohydrates, and fats but with excellent important fatty acids content, low or no calories and cholesterol, and antioxidants, and are known as host defense potentiators (Okwulchie and Odunze, 2004; Agrahar- Murugkar and Subbulakshmi, 2005; Heleno et al., 2010; Wani et al., 2010; Niazi and Ghafoor, 2021).
Different mushrooms species are rich in ash (7–17%), fiber (16–20%), protein (30–48%), fat (1–4%), and carbohydrate (125–40%), etc. (Manikandan, 2011). Mushrooms also have bioactive nutraceuticals such as polysaccharides (Ruthes et al., 2016), lectins (Singh et al., 2014), phenolic compounds (Heleno et al., 2015a), glycoproteins (Kumar, 2015), ergosterols (Barreira et al., 2014), unsaturated fatty acids (Tel-Cayan et al., 2017), and tocopherols (Khatua et al., 2013). These mushrooms also contain four influential nutrients like ergothioneine, glutathione, vitamin D, and selenium which serve to alleviate oxidative tension as well as an antioxidant property (CNN Health, 2018).
There are various bioactive compounds found in cultured broth, mycelium, and fruiting bodies such as volatile oils, flavonoids, alkaloids, ascorbic and organic acids, fats, polysaccharides, tocopherols, glycosides, minerals, proteins, carotenoids, terpenoids, lectins, enzymes, phenolics, and folates (Venturella et al., 2021). Figures 1–5 represents the structures of active metabolites of mushroom. Of which, polysaccharides are extremely important for modern medicine. Moreover, β-glucan is not a versatile and well-known metabolite, having a wide spectrum of biological activity (Chang and Wasser, 2012; Patel and Goyal, 2012; Finimundy et al., 2013; Kiss et al., 2021). Mushrooms also have a high percentage of water (93–95%) and also contain valuable minerals like copper, phosphorus, iron, potassium, and calcium. Due to their high protein and low-calorie values, they are recommended to heart patients and their essential amino acids are required by adults to keep healthy (Koyyalamudi et al., 2009).
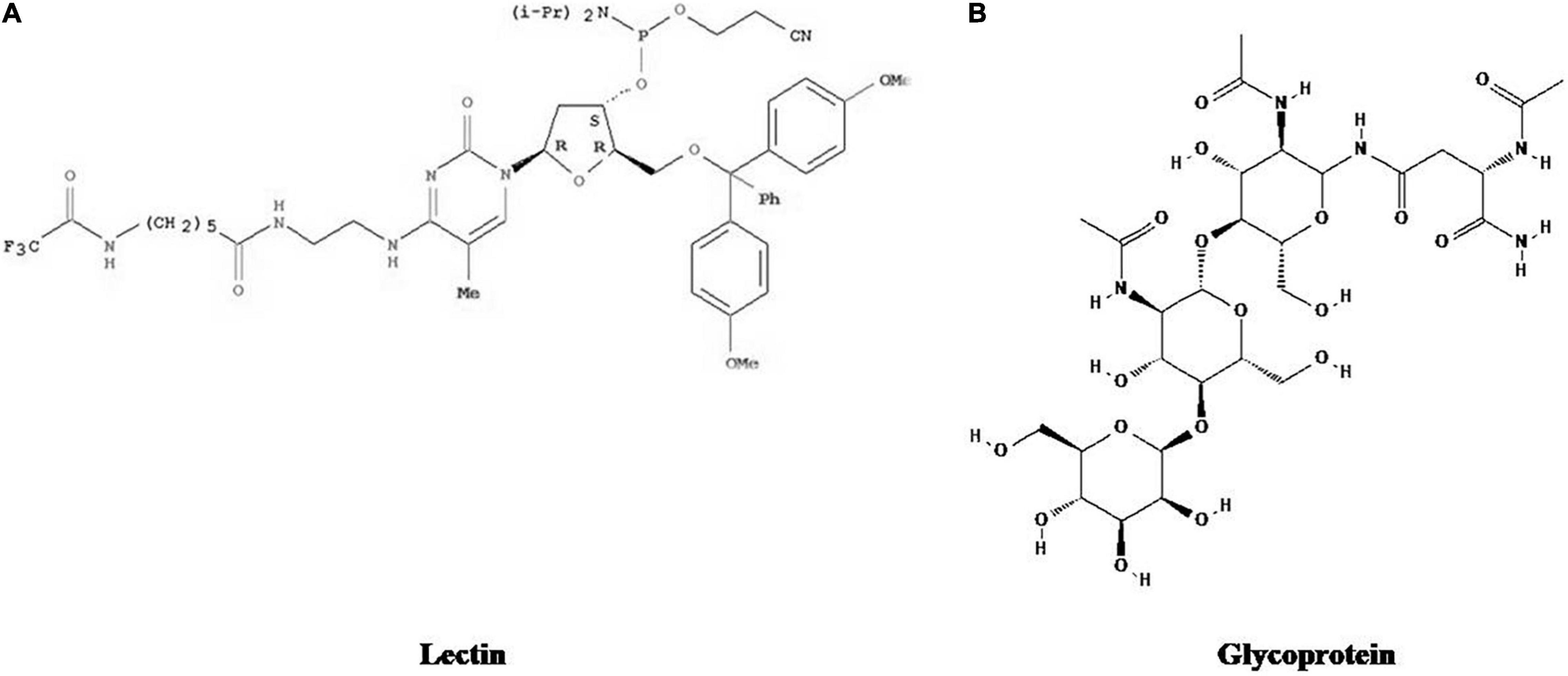
Figure 1. Structures of active metabolites of mushroom: (A) Lectins (Butt and Khan, 2019) (B) Glycoprotein (National Center for Biotechnology Information [NCBI], 2022b).
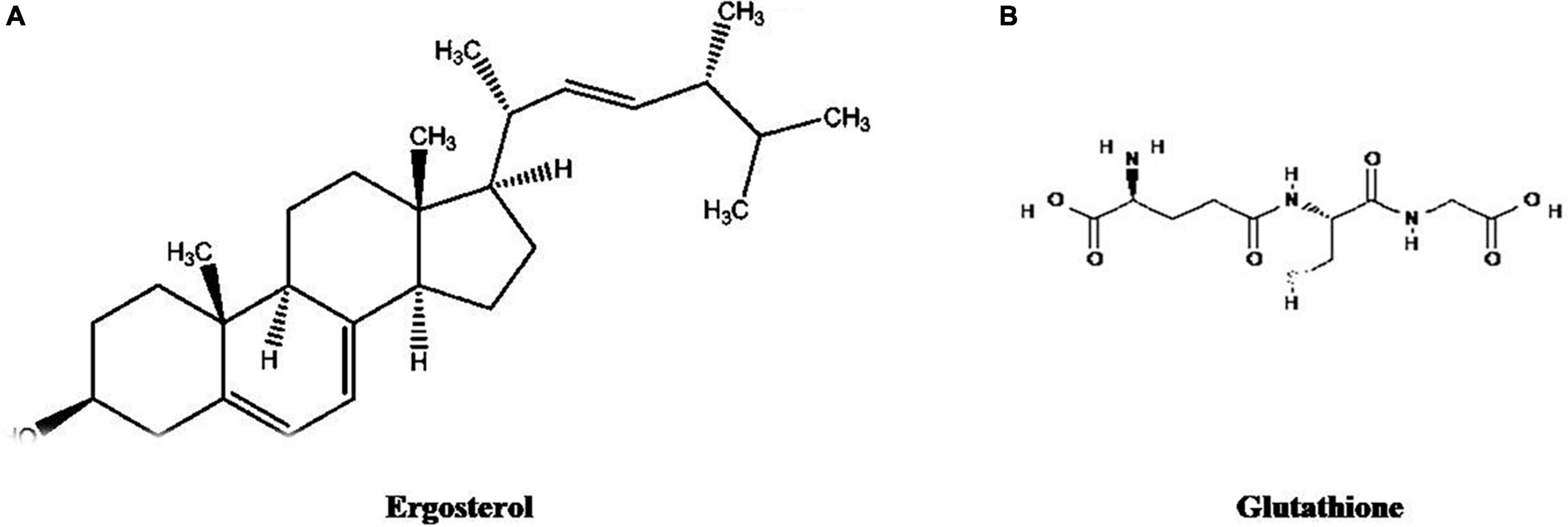
Figure 2. Structures of active metabolites of mushroom: (A) Ergosterol (Shao et al., 2010) (B) Glutathione (National Center for Biotechnology Information [NCBI], 2022a).
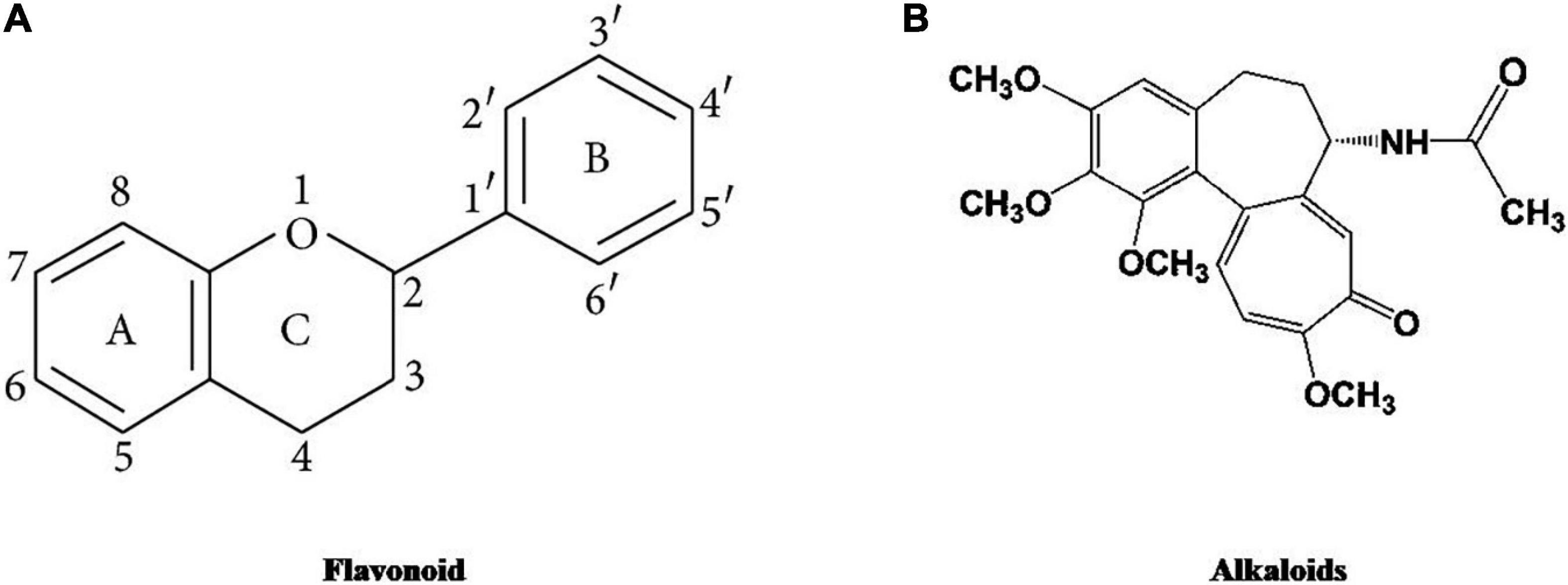
Figure 3. Structures of active metabolites of mushroom: (A) Flavonoid (Kumar and Pandey, 2013) (B) Alkaloids (International Union of Pure and Applied Chemistry, 1995).
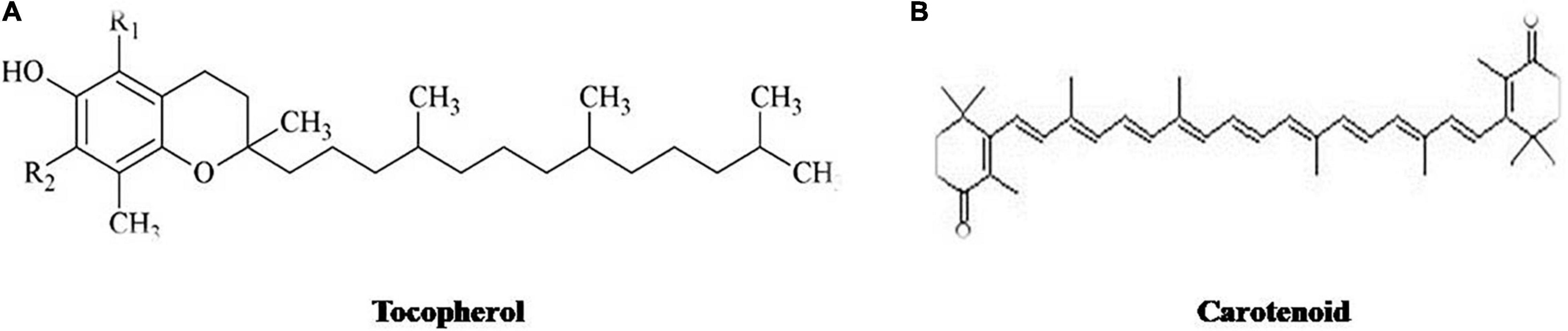
Figure 4. Structures of active metabolites of mushroom: (A) Tocopherol (Bauerova et al., 2019) (B) Carotenoid (Eldahshan and Singab, 2013).
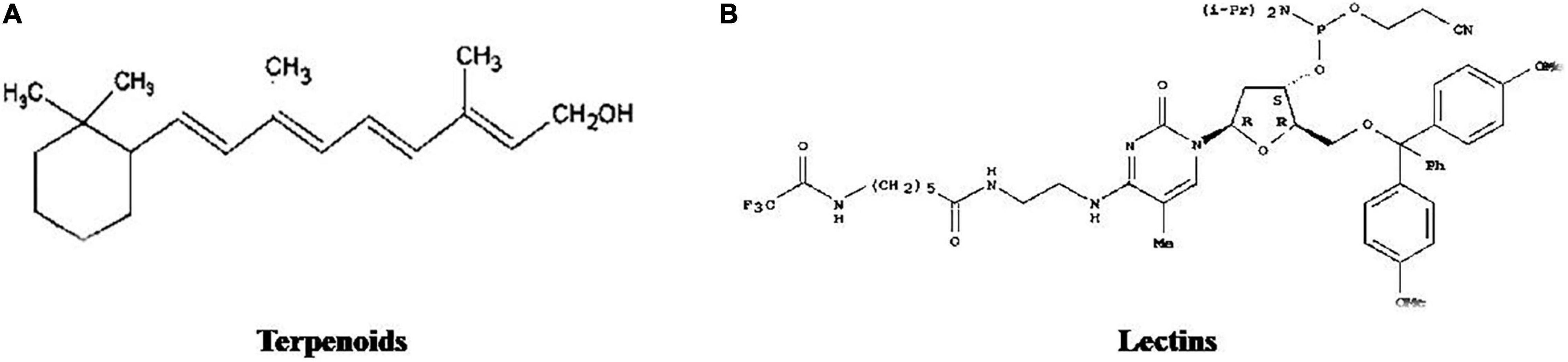
Figure 5. Structures of active metabolites of mushroom: (A) Terpenoids (Yadava et al., 2014) (B) Lectins (Butt and Khan, 2019).
Mushroom has a high concentration of tryptophan and lysine as compared to cysteine and methionine. They are also a good source of ascorbic acid and pantothenic acid as well as an excellent source of nicotinic acid and riboflavin (Ukpebor et al., 2007; Seo and Choi, 2021). Mushroom is also an ideal food for persons who wants to reduce excess fat as well as for diabetic patients due to the absence of starch. The wide applications of mushrooms are represented in in Figure 6.
This manuscript deals with medicinal mushrooms and their nutraceutical as well as bioactive compounds. There are various active metabolites such as polysaccharides, proteins, and peptides, terpenes, phenolic compounds, polyunsaturated fatty acids, carbohydrates and lipids are mentioned in this manuscript. There are several other unknown metabolites that are present in mushroom and can be beneficial for human health. This manuscript also deals with the medicinal mushrooms that are found in India and other countries and also mentions various identification techniques. Furthermore, omic approaches and the current status of each medicinal mushroom propagation have been mentioned.
Nutraceuticals and Bioactive Compounds
For thousands of years, mushrooms have been used in folk medicines. Some of them are nutraceuticals whereas others can produce bioactive compounds (Elmastas et al., 2007; Ribeiro et al., 2007). Huge numbers of mushroom species are not only edible and nutritious, but also possess toxic and medicinal qualities. Mushrooms are not only used as healthy vegetables that are rich in proteins but also a source of biologically active compounds having medicinal values. Uses such as hepatoprotective agents, immune-potentiating, complementary medicine or dietary supplements for anticancer and antiviral purposes (Olawale and Muhammed, 2017).
In recent years, there have been many studies focusing on the therapeutic and health benefits of the specific bioactive nutraceuticals of mushrooms such as antitumor, antibacterial, antioxidant, antiallergic, immunomodulating, cardiovascular protector, detoxification, antiviral, antifungal, antiparasitic, immune function enhancement, antioxidant, anti-inflammation, hepatoprotective, hypolipidemic, antithrombotic, antidiabetic, hypotensive, antiproliferative, anti-HIV, hypo-cholesterolemic, anticancer, cytotoxic, and anticoagulant activities (Wasser and Weis, 1999; Lindequist et al., 2005a; Ajith and Janardhanan, 2007; Yu et al., 2009; Zhang et al., 2011; Chang and Wasser, 2012; Finimundy et al., 2013; Rathore et al., 2017). Additionally, mushrooms also have the ability to attenuate the health hazards induced by obesity and the impaired functions caused by aging (Yahaya et al., 2014; Sayeed et al., 2015; Thangthaeng et al., 2015; Ghosh, 2016; Persson, 2016) (Table 1).
Mushrooms can cure wounds, stress, rheumatoid arthritis, asthma, diabetes, diaphoretic, liver disease, epilepsy, skin diseases, heart ailments, insomnia, allergies, cholesterol reduction, cholera besides intermittent fever, cold, gall bladder diseases, diarrhea, dysentery, anesthesia and also used as vermicides (Bahl, 1983). It can also cure heart, cancer, and nervous problems.
Clinically essential as well as well recognized drugs of medicinal mushroom and also the used drugs such as progesterone, morphine, aspirin, vinblastine, digitoxin, taxol, cortisone, vincristine as well as various others derived directly or indirectly from the higher plants. Patented products of active metabolites are mentioned in Table 2. Dasgupta and Acharya (2019) and Azeem et al. (2020) demonstrated that the poisonous mushroom Neonothopanus nimbi has aurisin A and aurisin K, which are effective against the Mycobacterium tuberculosis as well as Plasmodium falciparum (Ajith and Janardhanan, 2007). The important effect of pharmacology as well as the physiological properties of mushrooms are the regulation of biorhythm, cure of several diseases, bioregulation (immune enhancement), improvement as well as prevention from life-threatening diseases including heart diseases, cancer, and cerebral stroke, and also the maintenance of homeostasis (Wasser and Weis, 1999).
The production of secondary metabolites as the families of compounds having the same biosynthetic pathway such as non-ribosomal terpenoids or peptides. Anke (2020) proposed a theory for the existence of secondary metabolism. Consequently, secondary metabolism plays a vital role in the development of new chemical tools and also for the interaction with a changing environment during evolution (Anke, 2020). Mushroom species are divided into the edible mushrooms with representatives of Agaricus bisporus, Auricularia auricula, Pleurotus ostreatus, Lentinula edodes, and Flammulina velutipes, and medicinal mushrooms such as Poria cocos, Ganoderma lucidum, and Cordyceps sinensis.
Another, Psychedelic mushrooms—also known as magic mushrooms—are not the conventional type of mushroom, their extract delivering psychedelic effects which other mushrooms seldom do. Nevertheless, these magic mushrooms are not usually utilized in the fermented mushroom pills as well as the mushroom products manufactured by mushroom manufacturers. The US Food and Drug Administration (FDA) has banned magic mushrooms. No organic certified mushroom product has been eligible to contain magic mushrooms as an ingredient under the USDA (United States Department of Agriculture) (Dasgupta, 2019).
Globally, China was the first country to cultivate mushrooms and has the highest total production of mushrooms, followed by other countries such as Poland, the United States, The Netherlands, and Italy. Societies like Mexico, the ancient Greeks, Chinese, Romans, and Egyptians appreciated and treated mushrooms as medicines for a very long time (Feeney et al., 2014). Mushroom plays an important role in the food industry, biodegradation, in medicine, and in human welfare in general (Ozturk et al., 2003). These edible mushrooms are either harvested and cultivated under suitable conditions with rigorous quality control on palatability, shape, size, and tenderness, or collected directly from nature (Rahi and Malik, 2016). According to the derivation of mushroom names, edible mushrooms are categorized according to their morphology, texture, taste, habitat, and growth habits (Oso, 1975). Beside these edible mushrooms, there are also some non-edible or poisonous mushrooms like Celtis zenkeri, Coprinus africans, Phallus industiatus, Coprinus ephemerus, Phallus aurantiacus, Phallus rubicundus, and Mutinus bambustnus, etc.
Mushroom as Medicine
An alternative source of new antimicrobial compounds might also be mushrooms, especially secondary metabolites which induce benzoic acid derivatives, quinolones, steroids, terpenes, anthraquinones, etc., but also some primary metabolites such as proteins, oxalic acid, and peptides (Alves et al., 2012); Zhong and Xiao (2009) demonstrated that the higher mushroom produces an excess of the secondary functional metabolites mentioned above but are not limited to phenolic compounds, terpenes, polyketides, and steroids due to their metabolism (Zhong and Xiao, 2009). There are various mushrooms whose secondary metabolites possess drug-like structures (i.e., Lipinski’s Rule of five compliant such as log P, number of hydrogen bond donors, molecular weight as well as the number of hydrogen bond acceptors) moreover might be considered as a major natural inspiration for drug discovery purposes (Leeson, 2012). Lipinski’s rule of five binding mechanisms works effectively using molecular docking studies. Mushrooms also represent a source of polysaccharides having immune-stimulating as well as anti-cancer properties. Globally, Agaricus bisporus is the most cultivated mushroom followed by Flammulina velutipes, Lentinus edodes, and Pleurotus sp. The production of mushrooms is continuously growing and globally China is the biggest producer (Chang and Miles, 2008; Aida et al., 2009; Patel and Goyal, 2012). Although, wild mushrooms are becoming extremely critical for their sensory and pharmacological characteristics (Ergomul et al., 2013).
In various parts of the world, mushrooms occur from arctic to tropic levels. There are some species that mainly occur in geographically restricted areas whereas others exist in widely geographically separated areas. Nevertheless, there are many species that seem to show a preference for a definite type of habitat. Mushrooms exist in swamps, or are primarily found in upland wooded areas and also in open areas like pasture, gardens, and lawns like Pleurotus tuber-regium, whereas others are found on wood (Lignicolous) like Lentinus edodes, on dung (Corpophilous) like Coprinus lagopus, and on dead leaves (Follicolous) or litter like Tricholoma bayensis and Cortinarius melliolens (Pant et al., 2021). There are also a few mushrooms that grow on the nasidiocarps of other mushrooms and are known as Fungicolus. Mushrooms are mainly found on wastes like composting materials, sawdust, and garbage (Gbolagade, 2006).
During the pandemic of SARs-CoV-2 the uptake of mushrooms has been increased because the regular intake of natural products such as Grifola frondosa, Inonotus obliquus, and Lentinula edodes with effective anti-inflammatory and antiviral peculiarities reduced the effect of SARs-CoV-2 (Negi et al., 2020; Shahzad et al., 2020; Sarangi et al., 2021).
Mushroom also contain various types of non-digestible carbohydrates such as raffinose, chitin, oligosaccharides, β-glucans, and also resistant starch (Manzi et al., 2001; Dikeman et al., 2005) but according to Samsudin and Abdullah, mushrooms have both digestible carbohydrates such as glucose, mannitol, glycogen, and trehalose as well as non-digestible carbohydrates such as mannans, β-glucans and chitin (Samsudin and Abdullah, 2019). Mineral composition of Agaricus bisporus and Agaricus bitorquis are identified by Saiqa et al. (2008) and also identified that these compositions are low in concentration of cobalt, chromium, lead, zinc, manganese, chromium, nickel, and copper whereas enriched with essential minerals including lithium, sodium, and potassium. Both of these compositions exhibit a high quality of carbohydrates, proteins, and lipids (Saiqa et al., 2008).
There are some mushrooms such as Trametes versicolor, Schizophyllum commune, Flammulina velutipes, Ganoderma lucidum, Phellinus linteus, Lentinus edodes, Cordyceps sinensis, Inonotus obliquus, and Grifola frondosa that possesses inhibitory effects toward cancer as well their immuneceuticals actions, mainly via the elevation of the immune system of the host. The activation of NK cells, macrophages, dendritic cells, T-cells, and the production of cytokines are involved in this process (Wasser, 2010). According to Hetland et al. (2011) Agaricus blazei is plentiful in the β-glucan and polysaccharides and has been disclosed to have antiallergic, anti-infection, asthmatic, and antitumor properties in mouse models, in inflammatory bowel disease patients, it cojoined with anti-inflammatory impact. These impacts are because of innate immune cells like dendritic cells, monocytes, enhancement of skewed Th1/Th2 balance, NK cells, and inflammation.
Medicinal Mushroom in India
According to Rai et al. (2005) there are approximately 14,000 species known, out of which 2,000 species are safe for human consumption and 650 species have medicinal properties (Rai et al., 2005). In India and other developing countries that have a rich biodiversity of mushrooms, they are a boon for progress in the field of medicine, unemployment, and food due to various medicinal and nutraceutical mushrooms which are beneficial for the development of human health as minerals, drugs, food, and medicine (Rai et al., 2005; Sheena et al., 2005; Wani et al., 2010).
Currently, 35 species of mushrooms have been cultivated commercially whereas 20 species have been cultivated on an industrial scale. Mushroom diversity varies from typical Agaricus mushrooms with a stalk and umbrella-shaped top to the polypores, puffballs (Lycoperdon), Earthstars (Geastrum), and Stink Horns (Phalloides).
Active Metabolite From Mushroom
Polysaccharides
Mushroom polysaccharides are exploited and developed as a functional food substance such as ganoderan from Ganoderma lucidum, pleuran from Pleurotus species, lentinan from Lentinus edodes, calocyban from Calocybe indica, schizophyllan from Schizophyllum commune most of which are the representatives of D-glucans having common (1→3) or (1→6) β-linked glucose backbones (Villares et al., 2012; Badalyan, 2014). Commonly found monosaccharides in mushroom polysaccharides are mannitol, mannose, glucose, trehalose, rhamnose, xylose, galactose, arabinose, xylose, fucose, fructose, etc. (Valverde et al., 2015). Polysaccharides are one of the most common and potent compounds derived from mushrooms and exhibit various human-beneficial activities such as anti-inflammatory, antitumor, and immunomodulatory activities (Pant et al., 2020). The common functioning mechanisms of various mushroom polysaccharides that involves the activation of the above three activities are the activation of neutrophil, cytotoxic, cytokines (interferons, interleukins, and colony-stimulating factors), natural killer cells, monocytes, dendritic cells, and macrophages (Wasser, 2011). The health benefits of mushroom polysaccharides via the regulation of gastrointestinal and gut microbiota function (Prajapati et al., 2021). Mushroom polysaccharides as dietary fibers display an interaction process with colonized microbiota gut in gastrointestinal tracts that might change several variations of gut microbiota which impacted the health level of the host (Kong et al., 2016). Polysaccharides that obtained from the mushrooms might be degraded by the gut microbiota and some bacterial groups absorb them as an energy source that displays stimulation effects on the production of beneficial compounds such as short chains fatty acids like butyrate, valerate acid, acetate, and propionate and their propagation (Zhu et al., 2016; Ma et al., 2017). During the process of fermentation, the extracts of Ganoderma lucidum mostly composed of polysaccharides and have the ability to increase the abundance of Bifidobacteria (Yamin et al., 2012); Zhang et al. (2017) demonstrated that the Ganoderan is extracted from the Ganoderma sp. and having β-(1→3)-D-glucans with β-(1→6)-D-glucopyranosyl branches and the molecular weight is around 1.2 × 106 Da to 4.4 × 106 Da (Üstün et al., 2018; Ziaja-Sołtys et al., 2020). Additionally, due to the daily supplementation of Ganoderma lucidum polysaccharide strain, the abundance of bacteria like Roseburia, Lachnospiraceae, and Lactobacillus with health benefits to host enhanced significantly (Li et al., 2016). Colonized bacteria of the human colon utilized the β-glucans obtained from the mushroom and displays the potential to improve the health of humans by selectively changing the abundances of bacteria such as lactic acid bacteria and bifidobacterial (Wong et al., 2005). High molecular weight polysaccharides greater than 300 KDa that isolated from the extracts of water of Ganoderma lucidum mycelium which produce anti-obesity as well as microbiota-modulating effects like reduced endotoxin-bearing proteobacteria level as well as the ratio of Firmicutes/Bacteroidetes, decreased bodyweight, insulin resistance, and inflammation in mice fed a high-fat diet (Chang et al., 2015). The composition of the gut microbiota might be regulated by Inonotus obliquus polysaccharides as well as in mice having chronic pancreatitis (Hu et al., 2017).
Lentinan, from Schizophyllum sp. also has a β-(1→3)-glucan with a β-glucopyranosyl group linked by β-(1→6) linkage and molecular weight around 450 kDa (Pandya et al., 2019). Pleuran is a water- as well as alkali-soluble polysaccharide that is extracted from the Pleurotus sp. and has β-(1→3/1→6)-D-glucan or α-(1→3)-D-glucan structurally (Baeva et al., 2019). Grifolan extracted from the Grifola sp. of mushroom with gel-forming β-(1→3)-D-glucan. Molecular weight is around 770 kDa to 1650 kDa (Giavasis, 2014).
Kristiansen (2015) polysaccharide-K or PSK is extracted from the Trametes sp. with protein-bound β-glucan and having β-(1→4)-glucan with lateral β-(1→6)- glucopyranoside chains. It has a molecular weight of around 94 kDa (Zong et al., 2012). Another proteoglucan extracted from Trametes sp. is polysaccharide-protein complex. Polyporus polysaccharide is extracted from Polyporus sp. of mushroom with (1→3)- β-glucan backbone and (1→6)-β-glucopyranose side chain. Its molecular weight is around 1.6 × 105 Da (Zong et al., 2012). Polysaccharides that extracted from the Agaricus sp. have several structural variants like an acidic β-(1→6)/α-(1→3)-glucan, an acidic β-(1→6)/α-(1→4)-glucan and β-(1→6)/β-(1→3)- glucan with very broad molecular weight starting from 380 kDa to 10,000 kDa (Giavasis, 2014).
It has been found that the polysaccharide extracted from edible mushrooms such as Auricularia (jelly ears fungi) has a β-(1→3)-D-glucan that is linked with two residues of β-(1→6)-D-glucosyl for every three main chain glucose moiety. It has a molecular weight of around 2.1 × 103kDa (Miao et al., 2020). Polysaccharides that extracted from Cordyceps sp. having α-(1→4)- D-glucan linked with the branches of α-(1→6)-D-glucan with a molecular weight of around 1180 kDa (Zong et al., 2012).
Proteins and Peptides
In mushrooms, proteins and peptides are the important bioactive nutraceuticals having various health benefits like some enzyme inhibition activities, increased in the absorption and digestion of exogenous nutritional ingredients and the immune function modulation to help the host defending the invasion of pathogens as well as the inhibition activities of few enzymes (Valverde et al., 2015). It has been found that the proteins and peptides in mushrooms have pharmaceutical potential such as ribonucleases, lectins, ribosome-inactivating proteins, laccases, and fungal immunomodulatory proteins (Xu et al., 2011). Lectins are the glycol or non-immune proteins that binds with the carbohydrates of cell surfaces and possess important activities like antiviral, antitumor, antifungal, immunomodulatory properties, antibacterial, etc. (Singh et al., 2014) whereas fungal immunomodulatory proteins are used as adjuvants for the treatment of tumors because of their important activity in suppressing the invasion as well as metastasis of tumor cells (Lin et al., 2010).
Ribosome inactivating proteins are able to inhibit the fungal proliferation as well as HIV-1 reverse transcriptase activity (Puri et al., 2012). Sanchez observed anti-tumor and immunomodulatory effects on breast cancer MCF7 cells, hepatoma HepG2 cells, human leukemic T cells (Sanchez, 2017). Further, there were also anti-viral studies observed on the reverse transcriptase activity on the immunodeficiency virus (HIV-1) which showed inhibitory function. Usually, edible mushrooms possess between 19 to 39% of protein by dry weight while being a part of a complex network of fungal cells (Reddy, 2016).
Dahima et al. (2020) demonstrated that the pleurostrin extracted from the Pleurotus ostreatus is a 7 kDa anti-fungal peptide. It has also been found that the cordymin extracted from the mushroom Cordyceps militaris and Cordyceps sinensis is an anti-inflammatory peptide having a molecular weight of around 10,906 kDa. (Elkhateeb and Daba, 2019; Ashraf et al., 2020). Xylose-specific lectins extracted from Xylaria hypoxylon with a molecular weight of around 28.8 kDa show anti-tumor as well as anti-mitogenic activities (Büttner et al., 2019). Laccases that are isolated from Pleurotus ostreatus and Pleurotus eryngii display an anti-viral property (Wang and Ng, 2006; Ilyicheva et al., 2020); Lynn (2017) has also been found that there are several FIPS that have been isolated successfully from different mushrooms like Fip-gts from Ganoderma tsugae, Fip-fve from F. velutipes, and Fip-vvo from Volvariella volvacea. According to Chang et al. (2010) and Zhao et al. (2020) Fip-fve has been applied successfully for tumor immunotherapy. A 9 kDa RIP Marmorin with anti-tumor properties has been isolated from the Hypsizygus marmoreus (Wong et al., 2018).
Terpenes
Terpenes that are found from the mushrooms are regarded as volatile unsaturated hydrocarbon clusters that are categorized as mono, sesqui, di, and triterpenoids (Duru and Cayan, 2015). There are various types of sesquiterpenoids that are obtained from the mushrooms such as drimane, aristolane, sterpurane, cuparene, lactarane, spiro, bisabolane, fomannosane, and nordasinane. According to Duru and Cayan, mostly the diterpenoid mushrooms were detected as cyathane type while the triterpenoid compounds were detected as lanostane type that isolated from the mushrooms (Duru and Cayan, 2015). Various fungal sesquiterpenic molecules are effective against Leishmania mainly, Eimeria tenella, L. donovani, L. infantum, Acanthamoeba castellanii, Trypanosoma brucei, T. cruzi, Neospora caninum, T. gondii, and other parasites (Lenzi et al., 2018). Lentinus species can yield various sesquiterpenes. Lenzi et al. (2018) and Kumari et al. (2019) demonstrated that the sesquiterpenes panepoxydone and hypnophilin might be isolated from the ethyl acetate extracts of mushroom Lentinus strigosus whereas some of the sesquiterpenes such as dihydrohypnophilin, panepoxydione, and panepoxydone were isolated from the ethyl acetate extracts of fungus Lentinus conatus. According to Breitmaier (2006), Linalool is the monoterpene derivative with anti-bacterial activity. Sesquiterpenes are mainly composed of three isoprene units with general molecular formula C15H24. They are mainly produced by some fungi and plants. Geosmin is the common sesquiterpene produced by actinomycetes. Additionally, these mushroom terpenes have been associated with various health benefits such as antiviral (Asakawa et al., 2014), anticholinesterase (Dundar et al., 2015), anticancer (Song et al., 2013; Klaus et al., 2017), antioxidant (Boonsong et al., 2016; Kumari et al., 2021), antimalaria (Ozturk et al., 2015), anti-inflammatory (E1 Enshasy and Hatti-Kaul, 2013; Elsayed et al., 2014) activities. These multiple sesqui, di, and triterpenoids were obtained from various species of mushrooms like Ganoderma species, F. velutipes and Pleurotus. There are two main terpenes such as Flammulinolides and Flammulinol that are isolated from F. velutipes having cytotoxicity against the three tumor cell lines KB, HeLa, and HepG2 while terpenes that isolated from the Pleurotus species revealed very essential bioactivities like two sesquiterpenoids as well as five monoterpenoids having anti-inflammatory effects.
Lee et al. (2011) found that n-butyl ganoderate H as well as methyl ganoderate A acetonide from G. lucidum owes the ability of anti-acetylcholinesterase effect for the Alzheimer’s and related neurodegenerative diseases.
Phenolic Compounds
The phenolic compounds of mushrooms which are regarded as aromatic hydroxylated compounds with one or more hydroxyl groups as well as aromatic rings mainly comprise tannins, phenolic acids, oxidized polyphenols, hydroxybenzoic acids, lignans, flavonoids, stilbenes, and hydroxycinnamic acids (D’Archivio et al., 2010).
Additionally, these compounds provide protection against various degenerative disorders like cardiovascular diseases, cancer, brain dysfunction, and aging (Finimundy et al., 2013). They also have an excellent antioxidant capacity as well as antiviral, anti-inflammatory, antiatherogenic, cardioprotective, anticancer, antimicrobial, antithrombotic, antiallergenic and vasodilator effects (Balasundram et al., 2006; Ferreira et al., 2009; Heleno et al., 2012a). From the edible mushroom such as Phellinus baumii Pilat belongs to Hymenochaetaceae family, three phenolic compounds have been found that have the ability to inhibit LPS-stimulated nitric oxide (NO) production in RAW264.7 cells (Lee et al., 2017).
From the mushroom Phellinus species, the phenolic compound Hispidin has been derived which possesses anti-inflammatory activities via suppressing the ROS mediated NF-κB pathway in the macrophage cells of mouse (Shao et al., 2015); Chang et al. (2011) isolated phenolic compounds from the mushroom Phellinus linteus showed the anti-inflammatory mechanisms of decreasing the level of MDA in the edemapaw by enhancing the effect of SOD, GPx, and GRx in the liver, further the suppression of TNF-α and NO. Next, Patel and Goyal (2012) extracted hispolon from Phellinus species which induce epidermoid and gastric cancer cell apoptosis, and regardless of p53 status, hispolon inhibited bladder cancer and breast cell growth. According to Lai et al. (2010) the dichloromethane extracted from Ganoderma lucidum composed of phenolics, flavonoids, alkaloids, and terpenoids and also displayed anti-human papillomavirus 16 (HPV 16) E6 oncoprotein effect. When epidermoid cervical carcinoma (CaSki) cells are treated with the crude dichloromethane extracts, HPV 16 E6 production gets suppressed. In eight types of edible mushrooms such as Pleurotus ostreatus, Cantharellus cibarius, Agaricus bisporus, Lactarius deliciosus, Boletus edulis, Craterellus cornucopioides, Hygrophorus marzuolus, and Calocybe gambosa the contents of total flavonoid and total phenolic has been evaluated.
Polyunsaturated Fatty Acids
Ergosterol is one of the major sterols produced by mushrooms and has shown important antioxidant properties (Guillamon et al., 2010). It also plays an important role in the prevention of cardiovascular diseases (Kalac, 2013). They are detected as tocopherols and considered as effective as well as novel natural antioxidants and have major biological activities for the protection against microbial and cardiovascular diseases and degenerative malfunctions (Heleno et al., 2012a, 2015b; Jaworska et al., 2015). Linoleic acid participates in various physiological functions such as decreasing the inflammatory level via inhibiting the production of NO and also suppressing the expression of pro-inflammatory cytokines like TNF-α, IL-6, IL-1β, and NOS2 in RAW 264.7 cells (Saiki et al., 2017). The decreasing impact on the Alzheimer’s disease risk correlates with its inhibition effects against the acetylcholinesterase (AChE) and butyrylcholinesterase (BChE) (Ozturk et al., 2014).
Carbohydrates
Xylose, maltose, rhamnose, trehalose, mannitol, arabinose, fructose, sucrose, fucose, glucose, and mannose are the properties of carbohydrates that have been quantified in various mushrooms (Zaidman et al., 2005; Zhang et al., 2007; Ferreira et al., 2009; Heleno et al., 2012b). The antitumor polysaccharides which are isolated from mushrooms can be acidic or neutral in nature and have strong antitumor action. Moreover, they also differ in their chemical structures. Glycans which extend from homopolymers to highly complex heteropolymers and exhibit the antitumoral effect. Due to the activation of the immune system, mushroom polysaccharides have antitumor action. Wasser (2002) and Zhang et al. (2007) observed that mushroom polysaccharides cannot kill the tumor cells directly. These compounds prevent the body from stress, moreover, they can produce half the reduction in tumor size and extend the survival time of tumor-bearing mice.
According to De Groot and Hellingwerf (2001), McIntosh et al. (2005), Schmid et al. (2001)β-glucans are the main polysaccharides that are found in mushrooms whereas 50% of the fungal cell wall mass is founded by β-glucans. For the industry, it is very essential due to their excretion into the cell growth medium and making their recovery. Its chemical characterization and purification are very simple. These β- glucans are highly responsible for the antioxidant, anticholesterolemic, anticancer, neuroprotective, immunomodulating activities of several edible mushrooms (Falch et al., 2000; Ishibashi et al., 2001; Kataoka et al., 2002; Khan et al., 2013). They are also essential in protection from cancer as well as infectious diseases and also recovered the aid patients from radiotherapy and chemotherapy (Manzi et al., 2001; Chen and Seviour, 2007). Consequently, reinforcing as well as activating the host immune system is the best strategy for inhibiting the cancer cells’ growth (Daba and Ezeronye, 2003; Finimundy et al., 2013).
Lipids
Mostly the edible mushrooms contained polyunsaturated fatty acids and help to decrease the serum cholesterol level. Tocopherols are the natural antioxidant compounds found in the lipidic fraction as they act as free radical scavenging peroxyl components produced from several reactions. These antioxidants are protective against cardiovascular diseases, degenerative malfunctions, and cancer. Linoleic acid, an essential fatty acid to humans, decreases triglyceride levels, arthritis, cardiovascular diseases, and blood pressure (Hensley et al., 2004; Ferreira et al., 2009; Heleno et al., 2012a; Reis et al., 2012).
Unknown Unidentified Metabolite
Many researchers have identified some unknown metabolites such as unknown (1), unknown (2), unknown (3), unknown (4), unknown (5), unknown (6), unknown (7), unknown (8), unknown (9), unknown (10), unknown (11), unknown (12), unknown (13), unknown (14), unknown (15), unknown (16) (Satria et al., 2019) and also some other metabolites such as ganoderenic acid A, B, C, D, F, K; ganoderic acid A, B, C2, H, I, K; 12-acetoxy-7-hydroxy-3,11, 15-trioxolanost-8,16,24-trien-26-oic acid; 12-acetoxy-3-hydroxy-7,11,15-trioxolanost-8,16,24-trien-26-oic acid; 3,12,20- trihydroxy-7,11,15-trioxolanost-8,16,24-trien-26-oic acid; 12- hydroxy-3,7,11,15,23-pentaoxo-lanost-8-en-26-oic acid; 3,12- dihydroxy-4,4,14-trimethyl-7,11,15-trioxolanost-8,9,20,22-en- 26-oic acid; 12-acetoxy-7-hydroxy-3,11, 15-trioxolanost-8, 20-dien-26-oic acid; ganolucidic acid A; 3,7-dihydroxy-11,15, 23-trioxolanost-8,16-dien-26-oic acid; 7,12-dihydroxy-3,11,15, 23-pentaoxo-lanost-8,20-dien-26-oic acid; 12-acetoxy-3-hydroxy-7,11,15,23-tetraoxolanost-8,20-dien-26-oic acid and 12-acetoxy-3,7,11,15,23-pentaoxo-lanost-8,20 -dien-26-oic acid (Satria et al., 2019). There are various primary and secondary metabolites that are present in mushrooms such as proteins, lipids, carbohydrates, polyunsaturated fatty acids, alkaloids, terpenes, triterpene, sesquiterpenes, flavonoid, flavanone, saponins, alkaloids, polysaccharides, anthraquinone, tannins, steroids, glycoproteins, polyketides, phenolic compounds, melanin, β-glucan, dietary fibers, lectins, and many other compounds. Some of them are mentioned above but still, there are several other unknown metabolites present in the mushroom which do not report yet. There remains a need to find out other important bioactive components that can be essential/beneficial for human health.
Small Molecules
Valko et al. (2007) found that mushrooms have small molecules such as organic germanium, catechols, quinones, steroids, selenium, amines, sesquiterpenes, cerebrosides, and triacylglycerols. Lindequist et al. (2005b) demonstrated that the Hericium sp. have various small molecules that include compounds such as aldehyde derivative of 4-chloro-3,5-dimethoxybenzaldehyde and an erinacerin V alkaloid having a molar mass of 257 g/mol as well as an aldehyde derivative of 4-hydroxychroman having a molar mass of 206 g/mol. 5- hydroxy-6-(1-hydroxyethyl)isobenzofuran-1 (3H)-one, erinacine, 2-chloro-1,3-dimethoxy-5-methylbenzene, 4-chloro-3,5-dimethoxybenzoic acid (4-chloro-3,5-dimethoxyphenyl) methanol, Molecules 2021, 26, 251 18 of 24 3, 6-bis(hydroxymethyl)-2-methyl-4H-pyran-4-one, etc.
Stamets (2000a) and Zhang et al. (2016) demonstrated that the North American mushrooms have several other small molecules such as hexadecanoic acid, orenalline from Cortinarius armillatus, pentadecanoic acid, octadecanoic acid from Pleurotus djamor, 2E, 4E- octadecadienoic acid. Except for the orenalline, there are various compounds that have not been assessed for toxicity as well as bioactivity. Nephrotoxin is considered to evaluate the toxicity of orenalline (Gadgil, 2005); Lallawmsanga et al. (2016) has been found that the coral mushroom Ramaria cystidiophora (Southwestern British Columbia, Canada) has four new compounds that belong to butanolide groups called ramariolides A-D. It has been found that Ramariolides A has antimicrobial activities against Mycobacterium tuberculosis as well as Mycobacterium smegmatis (Lallawmsanga et al., 2016).
Deng et al. (2009) found that syringic acid and syringaldehyde are the two molecules having a molar mass of 198 and 182 g/mol correspondingly from the ethanol extract of E. Granulates fruiting bodies. Both the compounds have possible toxicity that evaluated on macrophage HL-60 cells and Raw 264.7 cells. Deng et al. (2009) demonstrated that both syringic acid as well as syringaldehyde has no effect on HL-60 as well as on Raw 264.7 cells viability of up to 31.25 and 25 μg/mL correspondingly. According to Mothana et al. (2003) and Maia et al. (2015) found that Confluentin (326 g/mol), grifolin (328 g/mol), and neogrifolin (328 g/mol) have antibacterial as well as growth-inhibitory activities when isolated from the extracted ethanol of the fruiting bodies of A. Flettii. Whereas J. hirtus have A lanostane-type triterpene 3, 11 – dioxdanosta- 8,24(Z)-diene-26-oic acid having molar mass (468 g/mol) and this triterpene inhibits the growth of gram-positive bacteria such as Enterococcus faecalis as well as Bacillus cereus (Maia et al., 2015).
Overholts (1939) demonstrated that the fungus U. criterium that is found in the North American cup has three new bisnaphthalene subclass compounds known as urnucratins A-C, and that this urnucratin A is active against the Streptococcus pyogenes, methicillin-resistant Staphylococcus aureus, and vancomycin-resistant Enterococcus faecium. Masuda et al. (2008) found that a fungus F. pinicola in North America has a new lanostane triterpenoid such as 25-dien-21-oic acid as well as 3-oxo-24-methyl-5α-lanost-8 with activity against the Bacillus cereus. In this study, Masuda et al. (2008) also demonstrate one known ergostane steroid and four known lanostane triterpenoids having anti-bacterial activities.
Medicinal Mushroom Identification
Morphologically mushrooms can be characterized/identified on the basis of their size, color (white, black, yellow, brown, cream, pink and purple-brown, etc.), consistency of the stalk and cap, chemical tests or reactions, spore color in mass, mode of attachment of the gills to the stalk (Carluccio, 2003; Fuhrer, 2005). Mushrooms can also be identified on the molecular level by Internal Transcribed Spacer (ITS) regions of gene sequencing. This ITS region is one of the most frequently used molecular markers for species delimitation in fungi. This ITS region is used to identify and compare the fungi that are present within the sample from genus to species level as it has a conserved sequence (Carlson et al., 2014).
There are various other techniques that are used for the identifications of mushroom such as microscopy, thin-layer chromatography, nuclear magnetic resonance spectroscopy, DNA sequencing, high-pressure liquid chromatography, chemical fingerprinting, ultra-performance liquid chromatography, high-performance thin-layer chromatography, liquid chromatography quadrupole time-of-flight mass spectrometry, gas chromatography-mass spectrometry, and fourier transform infrared spectroscopy (Toh Choon et al., 2012; Awadasseid et al., 2014; Hennicke et al., 2016).
Recently matrix-assisted laser desorption ionization-time of flight mass spectrometry (MALDI-TOF MS) technique has been developed rapidly which is widely used in clinical laboratories for the identification of fungus. This MALDI-TOF MS technique has been possessed as a promising tool for the identification of fungus because of its rapid performance, cost-effectiveness, and high throughput (Cassagne et al., 2016). Represents the identification and phytochemical analysis in Figure 7.
Potential Medicinal Mushrooms
Coriolus versicolor
Coriolus versicolor is one of the dried fruiting body or mycelia of Coriolus versicolor. This Coriolus versicolor is visible as a fan-shaped mushroom having a wavy margin and colored concentric zones. This obligate aerobe is commonly found year-round on dead logs, branches, stumps, and tree trunks. This fungus occurs all over the wooded temperate zones of North America, Asia, and Europe and is the most common shelf fungus in the Northern Hemisphere. This mushroom belongs to the family Basidiomycotina. This Coriolus versicolor has many different names such as Boletus versicolor, Polystictus versicolor, Trametes versicolor, Agaricus versicolor, Poria versicolor, Yun-zhi (China), i.e., cloud-like mushroom probably due to its wavy surface that covered with fluff, Kawaratake (Japan) which means mushroom by the riverbank, Turkey-tail (North-America) as it fan-shaped zoned having shades of white, gray and brown in color on the upper surface and on dead logs, it grows in overlapping clusters and Polyporus versicolor.
This Coriolus versicolor fruiting body has brackets 3 to 5 cm across which are flattened, tough, thin, and semi-circular whereas young brackets are flexible. Mostly these brackets occur in tiers and spread along the branches. The upper surface of the Coriolus versicolor fruiting body is velvety and attractively marked having concentric zones of several colors, i.e., black, gray, yellow, greenish, and brown. Mostly the margin of the fruiting body is wavy. The mushroom has white spores which are cylindrical (4–6 μm × 2–2.5 μm) and oblong. The spores and fruiting bodies do not form in agitated submerged culture and grow as pelleted or dispersed mycelium. More than 120 strains of Coriolus versicolor have been recorded (Soothill and Fairhurst, 1977). The fruiting body of Coriolus versicolor is harvested for its medicinal and nutritional values just like all other mushrooms. This fungus is known to have potential pharmacologically active secondary metabolites which belong to small molecular weight compounds in addition to the minerals and major macromolecules, i.e., carbohydrates, lipids, and proteins (Habtemariam, 2020). In European origin, the phenolic composition of Coriolus versicolor fruiting body about thirty-eight phenolic compounds have been identified by HPLC-MS/MS- based study belonging to the hydroxycinnamic acids and flavonoid such as flavonols, bioflavonoids, isoflavonoids, flavones (Janjusevic et al., 2017).
The most common species within the genus is Trametes versicolor which is commonly known as Turkey tail and has been reported on 295 woody plant species including angiosperms and conifers (Grand and Vernia, 2002). Trametes versicolor belongs to the domain ‘eukaryota’ and kingdom ‘fungi’ and has phylum and subphylum, i.e., Basidiomycota and Agaricomycotina whereas belonging to the class Agaricomycetes and subclass Agaricomycetidae. It has order polyporales and belongs to the family polyporaceae and species Trametes versicolor. This Trametes versicolor is commonly widespread across Ireland and Britain and this Turkey tail fungus occurs all over the mainland of Europe from northern Scandinavia right down to the Mediterranean region., This wood-rooting fungus is also found in Asia and North America.
In 1753, Carl Linnaeus originally described Trametes versicolor and gave it the binomial name Boletus versicolor and further, it was as recently as 1939 that this species renamed as Trametes versicolor by Czech mycologist Singer (1975). Trametes versicolor is one of the cosmopolitan genera of white-rot polypores and the most familiar genera of polypores, its species-level taxonomy is unsettled. These species are available in about all forest ecosystems and are also found often on many genera of hardwoods in every part of northern temperate forests. Apart from their noteworthy role in the ecosystem’s recyclers and decomposers, the mushrooms have various biological activities including antibacterial, anticancer, insecticidal, antioxidant, anti-proliferative, anticoagulant, antifungal, antidiabetic, hepatoprotective, antiparasitic, antiviral, and anti-inflammatory properties (Atomachi, 1988). More than one hundred and twenty strains of Coriolus versicolor have been recorded in the Compendium of Chinese Materia Medica. This Coriolus versicolor has cold properties and is slightly sweet in taste, exerting its effects through the spleen and liver according to the theory of traditional Chinese medicine practices (Wasser, 2010). Coriolus versicolor is also useful for strengthening the physique, removing toxins, dispelling heat, increasing the host’s immune function, and enhancing the energy and spirit. Coriolus versicolor is often used for several types of cancers, upper respiratory, digestive, and urinary tract infections and chronic hepatitis in the clinical practices of traditional Chinese medicine. Picture of Coriolus versicolor shown in Figure 8Aa.
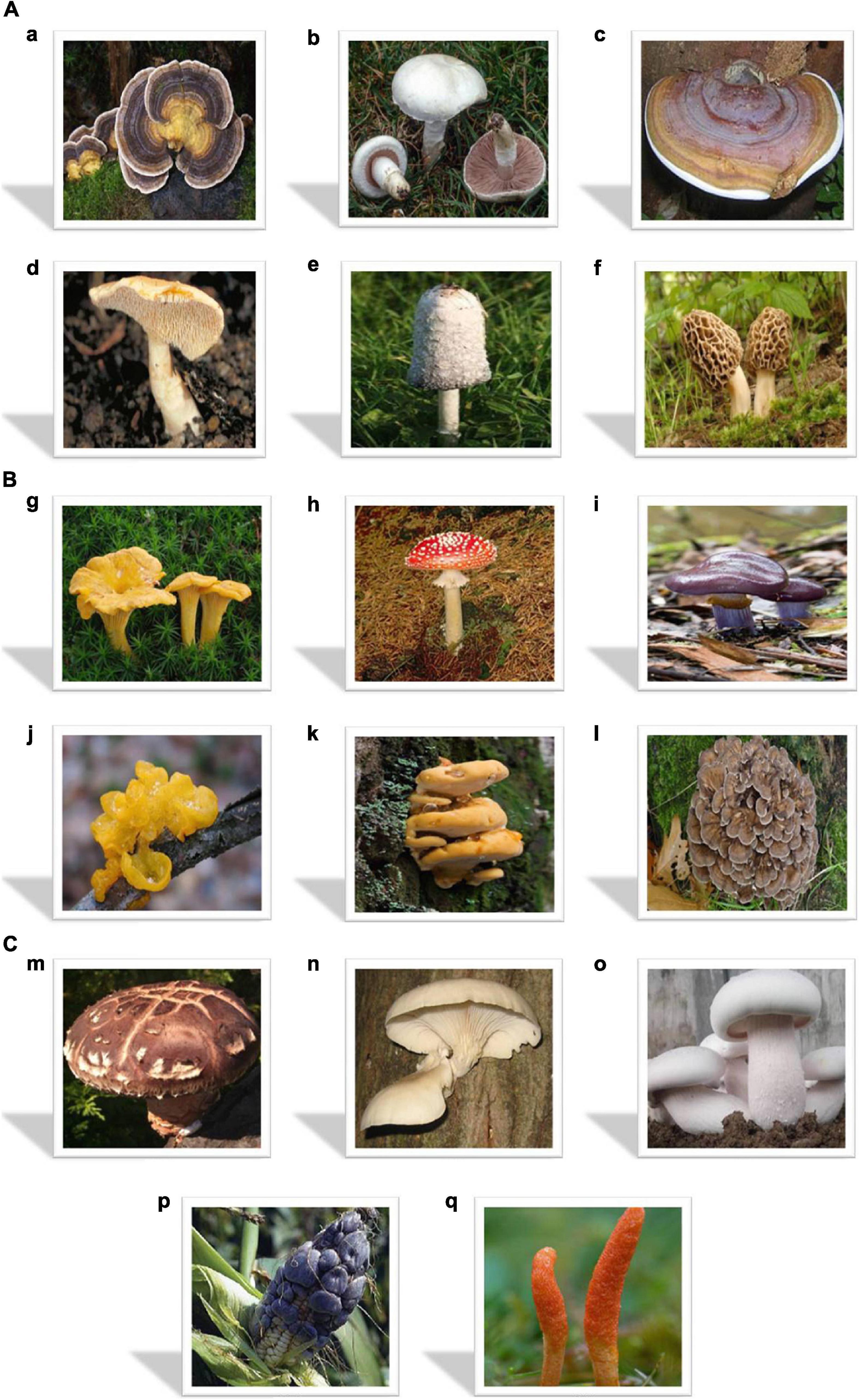
Figure 8. (A) Representation of Medicinal Mushrooms (a) Coriolus versicolor (Paul et al., 2008) (b) Agaricus sp. (Glamočlija et al., 2015) (c) Ganoderma sp. (Hong and Jung, 2004) (d) Hydnum sp. (Paul et al., 2008) (e) Coprinus sp. (Coprinus Pers, 2015) (f) Morchella sp. (Raman et al., 2018). (B) Representation of Medicinal Mushrooms (g) Cantharellus sp. (Pilz et al., 2003) (h) Amanita sp. (Tulloss, 2015) (i) Cortinarium sp. (Cortinarius (Pers.) Gray, 2020) (j) Tremella sp. (Rea, 1922) (k) Rigidoporus sp. (Rigidoporus, 1905) (l) Grifola sp. (Cetto, 2008). (C) Representation of Medicinal Mushrooms (m) Lentinus sp. (Wells, 2008) (n) Pleurotus sp. (Chang and Miles, 2004) (o) Calocybe sp. (Kirk et al., 2008) (p) Huitlacoche sp. (Spraker, 2013) (q) Cordyceps sp. (Pat O’Reilly, 2016).
Agaricus sp.
Agaricus campestris is frequently known as meadow or field mushroom. Agaricus campestris has pileus 3 to 6 cm wide. This Agaricus campestris is hemispherical to convex in nature and becomes planar at maturity. Agaricus campestris when young are pure white in color and become yellowish at the center with age and sometimes depressed at the center. Its surface is smooth, dry, and silky fibrillose whereas sometimes it is a few grayish to brown fibrils or fibrillose over the cap surface. Its complete margin is expanded beyond the gills frequently hung with veil remnants. On exposure, Agaricus campestris has firm, thick, reddening, and white flesh. It has a mild taste and a fungal odor. It has close Lamellae and it is pinkish white in button then bright pink and then becomes chocolate brown. The length of its stipe is 3–7 cm long and 0.5–1 cm diameter. It has been found that at base it is attenuated or bulbous and centric. It is white to whitish in color and has a smooth surface above the veil. It often has few fibrils below which are stuffed or hollow, silky, and the context is white slight reddening on exposure. At its best it has an annulus of white color which has a thin and skirt-like appearance. The print of its spore is chocolate brown. The spore is 5.5–7 μm × 3.5–5 μm and elliptical.
Agaricus mushroom is a fungus that is used for ongoing liver disease, cancer, digestive problems, high cholesterol, type 2 diabetes, arteriosclerosis, bloodstream disorders, heart disease, osteoporosis, and stomach ulcers. This mushroom is also used to boost up the immune system as well as for physical and emotional stress. In Agaricus campestris, the presence of insulin-releasing, anti-hyperglycemic as well as insulin-like activities has been reported. It also has been found that the lectins that isolated from the Agaricus campestris enhance the insulin that releases by isolated ret islets of Langerhans (Ewart et al., 1975; Ahmad et al., 1984a,b). It is also used in the treatment of diabetes (Gray and Flatt, 1998; Usman et al., 2021). Picture of Agaricus sp. shown in Figure 8Ab.
Ganoderma sp.
Ganoderma species is one of the facultative parasites that live as saprobe by roots of trees, feeding off as well as rooting stumps. They are pathogenic and wood-decaying fungus that causes stem and root rot in the perennial crops as well as butt root (Coetzee et al., 2015). The species of Ganoderma also plays a vital role in the breakdown of woody plants for the mobilization of nutrients. It is also useful in bioremediation due to the lignocellulose decomposing enzyme’s mechanism and also useful in bioenergy production (Coetzee et al., 2015; Bijalwan et al., 2020).
The fruiting body of Ganoderma contains polysaccharides that are useful in the inhibition of tumor growth. One of the major constituents is the glucans present in the cell wall of fungi. Ganoderma enhances the bodily resistance against tumor growth and also the immunity function. It also induces interferon production as well as kills the cells of the tumor within the body (Jong and Birmingham, 1992). It is also used in the treatment and prevention of diabetes, infections, cancer, immune system disorders, hepatoprotection and bacterostasis, etc. (Deepalakshmi and Mirunalini, 2011). Studies have shown that the regular consumption of Ganoderma has been linked to prolonged life, relaxed body, and alleviation of the symptoms of aging (Yuan et al., 2018).
Ganoderma lucidum have a 3 to 23 cm wide pileus. It is kidney-shaped and elongated. At maturity, it is more or less fan-shaped and becomes red to reddish-brown when gets mature. When it is young, it often has zones of bright yellow and white toward the margin. It has up to 2 cm deep tubes as well as 0.1 cm of pores. It is whitish in color, usually bruising brown. It has 3 to 12 long stipes 1 to 2 cm thick. It is cylindrical, dark red to black with varnished crust, has a brown print spore, smooth and twisted in nature (Vishwakarma et al., 2011).
Ganoderma lucidum is widely used in the treatment of bronchitis, gastric ulcer, hepatopathy, asthma, insomnia, chronic hepatitis, nephritis, arthritis and hypertension, weakness, fatigue, and cough (Halpern and Miller, 2002). It is also used in India in the treatment of joint pain (Harsh et al., 1993). It also has some very significant properties like hepatoprotective, anti-cancer, anti-atherosclerosis, very strong immunomodulating effects, anti-oxidant, anti-HIV, nephroprotective, anti-tumor, anti-hepatotoxic, cardiovascular, and respiratory properties (Chang, 1995; Stamets, 2000b). It is also found out that the substances extracted from the mushrooms can decrease the level of blood pressure, blood sugar level, inhibition of platelet aggregation as well as blood cholesterol, etc. (Ajith and Janardhanan, 2007). A picture of Ganoderma is shown in Figure 8Ac.
Hydnum sp.
Hydnum is the genus of fungi that belongs to the Hydnaceae family and are significant for their unusual spore-bearing structures of teeth other than gills. Hydnum repandum and Hydnum rufescens are the two best-known edible mushrooms. Hydnum repandum has a pileus of 3 to 10 cm in diameter. It is convex and becomes nearly a plane having a central depression. Its surface is smooth to slightly scaly, dry, cream to buff-orange, or planer. In the beginning, its margin is inrolled and at maturity it becomes lobed to undulating and bruising to orange-brown. Hydnum repandum is fleshy white and often discolors to yellowish when it is bruised or exposed. Its taste and odor are peppery or mild (Vishwakarma et al., 2011).
Commonly, Hydnum repandum is known as hedgehog mushroom or wood hedgehog. Repandiol compound extracted from the Hydnum repandum shows cytotoxic activity against a variety of tumor cell types, mainly colon adenocarcinoma cells (Takahashi et al., 1992). Chloroform extracted from this mushroom has some mild antibiotic activities against Staphylococcus aureus, Bacillus subtilis, Enterobacter aerogenes, and Staphylococcus epidermidis while extract of ethanol has mild activity only against the Bacillus subtilis. Picture of Hydnum is shown in Figure 8Ad.
Coprinus sp.
Coprinus is one of the tiny mushroom genera which forms fungi that consists of Coprinus comatus. This Coprinus comatus is 4 to 10 cm broad, oval, and cylindrical in nature and elongated as conical or bell-shaped as well as becomes revolute or torn at the margin. Its surface turns white to pinkish toward the margin and then becomes blackish. It has a smooth center as well as ochraceous cream, pale or buff fulvous at first, and has shaggy white to pale brownish scales often darkest at the tips. It has decurved margins that inroll at maturity. It has no or slightly pleasant taste with a mild odor (Vishwakarma et al., 2011).
Commonly, this fungus is known as shaggy ink cap, shaggy mane, or lawyer’s wig. This fungus has various bioactive functions such as its consumption helps to regulate the blood glucose level, hypoglycemic and has antitumor, antioxidative, hypolipidemic, antibacterial as well as immunomodulation effects (Bailey et al., 1984; Fan et al., 2006; Wei and Van Griensven, 2008). Picture of Coprinus is shown in Figure 8Ae.
Morchella sp.
The genus Morchella belongs to the family Morchellaceae. They have about 80 various species; one of them is Morchella esculenta that has a 3 to 5 cm diameter of pileus and is 4 to 8 cm long. This mushroom species is subglobose to ovoid and pale brownish cream and pale brown to grayish brown in color. Its surface is covered with irregularly interwoven pits of several colors or rounded pits. Its edge is thick, rounded and yellow or white in color. Its stipe is 3 to 6 cm long, having a 2 to 4 cm diameter. At base, it is bulbous, centric, or attenuated. Firstly, it appears as white in color then with age it becomes ochraceous. Just beneath the pileus, it has wrinkled as well as grooved scurfy tufts as well as it is also brittle and hollow. It has white and thin flesh with a yellow spore print. Spores are 11.5–14 μm × 19.5–23 μm ellipsoid (Vishwakarma et al., 2011).
Morchella esculenta is one of the edible morel mushrooms commonly known as Guchhi. This mushroom is used in food, medicines, and also health care. Morchella esculenta shows anti-inflammatory as well as antitumor activity against both ascites as well as solid tumors of ethanolic extracts whereas methanolic extracts prepared from its mycelia show a high content of total phenols as well as high antioxidant activity (Mau et al., 2004; Nitha et al., 2007). A picture of Morchella is shown in Figure 8Af.
Cantharellus sp.
Cantharellus is the genus of edible mushrooms that are locally known as golden Chanterelle or Chanterelle, which refers to the species Cantharellus cibarius. This Cantharellus mushroom belongs to the family Cantharellaceae.
The species Cantharellus cibarius has a pileus of 3 to 10 cm in diameter. At first, it appears as convex with an inrolled margin and then often becomes funnel-shaped along with wavy-margins. It is pale yellow to egg-yolk yellow to almost orange in color having some small appressed fibers. It is mildly peppery in taste as well as having a fruity odor. Underneath its smooth cap on the lower surface, it has gill-like ridges which run almost all the way down its stipe that tapers down seamlessly from the cap. It has a 3 to 5 cm long stipe 1 to 2 cm thick in diameter. It is thin, solid, and smooth concolorous the pileus. It has pale yellow to creamy white spore prints. Spores are 7–10 μm × 4–6 μm smooth and ellipsoid (Vishwakarma et al., 2011). This species of mushroom has excellent antihyperglycemic, antioxidant, wound healing, antimicrobial, iron-chelation, cytotoxicity, anti-hypoxic, anti-inflammatory activities (Kumari et al., 2011). Picture of Cantharellus shown in Figure 8Bg.
Amanita sp.
Amanita genus has around 600 species of agarics and it belongs to the family Amanitaceae. The edible species of mushrooms are Amanita rubescens, Amanita fulva, Amanita calyptrate, Amanita jacksonii, Amanita vaginata, Amanita caesarea whereas inedible mushroom species are Amanita citrina, Amanita volvata, Amanita atkinsoniana, Amanita albocreata, Amanita excelsa, Amanita sinicoflava, Amanita longipes, Amanita onusta, Amanita franchetii, Amanita flavorubescens, Amanita rhopalopus, Amanita silvicola, Amanita spreta, etc. (Phillips, 2010). There are some poisonous species, some lethally so, of Amanita such as Amanita farinose, Amanita brunnescens, Amanita pantherina, Amanita cokeri, Amanita ceciliae, Amanita crenulata, Amanita muscaria, Amanita porphyria, Amanita frostiana, etc., Amanita ocreata, Amanita smithiana, Amanita abrupta, Amanita subjunguillea, Amanita virosa, Amanita proxima, Amanita exitialis, Amanita arocheae, Amanita magnivelaris, Amanita bisporigera, Amanita phalloides, Amanita smithiana, and Amanita verna respectively (Zeitlmayr, 1976; Surayot et al., 2021).
The species Amanita vaginata has a pileus 9–10 cm × 3–5 cm in size and brown in color. Their cap shapes are usually convex and have smooth as well as round edges. It has been found that its cap has a fleshy brown color. Hymenophores are not present on the underside. In this mushroom, regular-shaped white colored gills present. It has a brown color stipe with 5 to 7 cm and 2 to 3 cm length and width correspondingly. Light brown color, single welled, round to oval shaped spore present having 7–8 μm × 5–6 μm size (Meena et al., 2020).
Amanita species have various biological activities like antitumor, pesticidal, cytotoxic, antioxidant, anticancer, antibacterial, acetylcholinesterase, esterolytic, antiviral, activity, anti-larvicidal, antifungal, anti-inflammatory properties (Sevindik et al., 2019). Picture of Amanita shown in Figure 8Bh.
Cortinarius sp.
Cortinarius is one of the worldwide distributed genera of mushrooms that belongs to the family Cortinariaceae (Cortinarius (Pers.) Gray, 2020). It is supposed to be the major genus of agarics that contains over 2,000 widespread species (Kirk et al., 2008). The species Cortinarius corrugates having a pileus 22–23 cm × 8–9 cm in size and ash colored. This mushroom is ovate in shape with a grooved edge. There are hymenophores beneath the cap whereas on the underside of the cap regular-shaped gills are present. The stipe of the mushroom appears as milky white, on the upper part of the stipe an anal or black ring is present while on the lower part the volva is absent (Meena et al., 2020). Picture of Cortinarius shown in Figure 8Bi.
Tremella sp.
The mushroom genus Tremella belongs to the family Tremellaceae. All species of Tremella are the parasites of other fungi and mostly produce as anamorphic yeast states when producing Basidiocarps. Tremella is colloquially classed among the gelainous or jelly fungi. Tremella fuciformis and Tremella aurantialba are the two species that are commercially cultivated for food (Zhang et al., 2009). Tremella fuciformis is locally known as silver ear fungus or snow fungus. It is tasteless in nature and has a gelatinous texture (Shu-Ting and Philip, 2004). Tremella fuciformis may have some medicinal important activities like fighting cancer, combating obesity, anti-aging, lowering cholesterol, protecting nerves, and acting as an anti-inflammatory. Picture of Tremella shown in Figure 8Bj.
Rigidoporus sp.
Rigidoporus is a genus of the fungi that belong to the family Meripilaceae. This fungi forms flattened mycelia of white color which is 1 to 2 mm thick and grows on as well as adheres to the surface of root bark. The species Rigidoporus microporus is 20 cm wide, faintly velvety, leathery in nature. It has a broadly attached shelf as well as imbricate with the substrate. Its color changes from orange to brown or red and later becomes faded (Liyanage, 1997). In the absence of any woody substrates, these rigidoporus grow fast and spread several meters via the soil (Oghenekaro et al., 2014). Rigidoporous have several biological properties like mitogenic activity, anti-hepatitis B surface antigen effect, plasma clotting activity, activation of alternative pathway complement, and tumor suppressive effects (Chang et al., 2011). Picture of Rigidoporus shown in Figure 8Bk.
Grifola sp.
The genus Grifola belongs to the family Meripilaceae. The species Grifola frondosa is locally called hen of the woods. This mushroom grows in clusters with a grayish to brown cap which appears as curled or spoon-shaped along with wavy margins of 2 to 7 cm wide at the base of the trees, especially oaks. It is 60 cm in size. Each cap bears 1 to 3 pores per mm at the under-surface with the tubes which are rarely deeper than 3 mm. Milky white stipe has a branchy structure and it becomes tough as it matures (Yeh et al., 2011).
Grifola frondosa have some biological activities such as antitumor (Masuda et al., 2006; Cui et al., 2013), anti-inflammation (Han and Cui, 2012; Su et al., 2020), immunomodulation (Adachi et al., 1994), antivirus (Gu et al., 2007; Zhao et al., 2016), antidiabetic (Postemsky and Curvetto, 2015), immune-enhancing, anti-hypertensive, hypoglycemia (Chen and Seviour, 2007) and antioxidation (Yeh et al., 2011). It also contributes to metabolic disorders treatment like non-alcoholic fatty liver disease. It also has potential in the treatment of hyperlipidemia as well as hyperglycemia. Picture of Grifola shown in Figure 8Bl.
Lentinus sp.
The genus Lentinus belongs to the family Polyporaceae and it is mainly spread in the subtropical regions (Kirk et al., 2008). The species of Lentinus have extracellular enzymes, moreover, it acts as wood-decaying basidiomycetes, gregarious on fallen wood of a wide variety of deciduous trees like chestnut, cotton, hornbeam, beech, mulberry, ironwood, shii, oak, maple, chinquapin, sweetgum, and alder in a moist or warm climate (Bisen et al., 2010). Medicinally, Lentinus edodes is used for the diseases such as fungal infection, bronchial inflammation, hyperlipidemia, hepatitis, cancer, depressed immune function, heart disease, infectious disease, flu and cold, environmental allergies, urinary inconsistencies, hypertension, diabetes, etc. (Bisen et al., 2010). Picture of Lentinus shown in Figure 8Cm.
Pleurotus sp.
Pleurotus is the genus of gilled mushroom and belongs to the family pleurotaceae. The species Pleurotus ostreatus also known as abalone or tree or oyster mushroom and is an edible mushroom (Chang and Miles, 2004). This mushroom is also used for mycoremediation and attacks and kills nematodes as well as microbes. This mushroom is mostly cultivated on straw and other media. This mushroom has the bitter-sweet aroma of benzaldehyde (Stamets, 2000b). This species of mushroom mainly grows on wood in shelflike clusters. This mushroom is of a larger size, and has white gills that run down a stubby. It has no stem. It has a white to lilac pattern of spores. This Pleurotus ostreatus has an oyster shaped, fan and broad cap spanning 5 to 25 cms. It is tan to dark-brown or white to gray in color. When it is young, its margin is enrolled and then becomes smooth and lobed or wavy. Because of its stipe arrangements, its flesh is firm, white, and of different thicknesses. The gills are white to cream in appearance (Philips and Roger, 2006). This mushroom has some biological effects due to the presence of some nutritional compositions including anti-cholestrolic, anticancer, antiviral, anti-diabetic, antioxidant, eye health, antibacterial and antiarthritic, etc. (Deepalakshmi and Mirunalini, 2014). Picture of Pleurotus shown in Figure 8Cn.
Calocybe sp.
The genus Calocybe is the fungus that belongs to the family Lyophyllaceae. The species Calocybe indica is locally known as a milky-white mushroom that is rich in ascorbic acid, lipids, riboflavin, amino acids, pyridoxine, vitamins, biotin, low fat, nicotinic acid, proteins, minerals (arsenic, zinc, potassium, manganese, calcium, phosphorus, magnesium, iron and sodium), fibers. According to Mandal et al. (2012) this mushroom has antioxidant properties and stimulated immune activation of thymocytes, splenocytes, and macrophages.
It helps in reducing the triglycerides and total plasma cholesterol level and consequently decreases the chance of cardiovascular, artery, and atherosclerosis-related disorders (Alam et al., 2008).
It also possesses biological activities regarding neurodegenerative diseases, anti-carcinogenesis, anti-aging, anti-obesity, cardiovascular disease, anti-infection, preventing physical injury, anti-tumor also helps to reduce the risk of breast cancer (Tsukamoto et al., 2003; Sharma et al., 2013). Picture of Calocybe shown in Figure 8Co.
Huitlacoche sp.
Huitlacoche is a heterothallic fungus that belongs to the family Ustilaginaceae. This fungus is also known as Mexican truffle, corn mushroom, or corn smut. This mushroom has great importance for the human diet (Valverde et al., 2012). This mushroom contains some bioactive compounds such as proteins, amino acids, glutamic acid, lysine, serine, aspartic acid, glycine, total carbohydrates, arabinose, mannose, galactose, xylose, glucitol, mannitol, glycerol, etc. Out of these, it has been found that xylose, galactose, mannose, and arabinose are present in lower proportions. It also contains heteroglycans, dietary fiber, and homoglycans, etc. (Valverde et al., 2012).
Huitlacoche mushrooms possess antitumoral, antimutagenic, immunomodulating, antiatherogenic, hyperlipidemic, hypo- glycemic, anti-inflammatory as well as various other health-promoting activities (Valdez-Morales et al., 2010). Picture of Huitlacoche shown in Figure 8Cp.
Cordyceps sp.
Cordyceps are the fungus that mainly lives on certain caterpillars and are usually found in high mountain regions (Kindzel’skii et al., 1995). The species Cordyceps sinensis comprises cordycepic acid and cordycepin substances. This mushroom has some therapeutic applications such as stabilization of blood sugar metabolism, effects of enhanced utilization of oxygen, and production of ATP. This mushroom protects the organs from kidney, liver, and heart diseases. Cordyceps sinensis also has a sedative effect on the central nervous system of the body (Zhou et al., 2009).
This Cordyceps mushroom also has immunomodulating effects and also possesses anticancer activities, especially skin and lung cancer. It also shrinks the size of tumors (Kindzel’skii et al., 1995). Picture of Cordyceps shown in Figure 8Cq.
Omics Approaches in Mushroom Research
There are several omics approaches in this post-genome era such as transcriptomics, metabonomics, genomics, and proteomics along with the bioinformatics that plas a vital role to assess the teratogenicity, nephrotoxicity, and genotoxicity of medicinal mushrooms. To predict the toxicity of chemicals, many genomes expression analyses have been performed in the form of metabolites, mRNA, and protein profiles that will have the same type of metabonomics, transcriptomics, and proteomics in a biological system such as toxic fingerprinting. Stierum et al. (2005), Xu et al. (2009), and Villeneuve and Garcia-Reyero (2011) demonstrated by in silico genome or comparative genomic analysis and elucidation of the inhibitory effect of toxic compounds, whether singly or in a mixture, can be used to discover the proteins or genes as an effective biomarker for the given toxicity (Stierum et al., 2005; Xu et al., 2009; Villeneuve and Garcia-Reyero, 2011).
Aardema and MacGregor suggested that the detailed study on metabonomics, transcriptomics, and proteomics profiles can be helpful in the exploration of the potential toxicity of any undesired mushroom compounds as well as their toxic outcomes (Aardema and MacGregor, 2002). Correspondingly, in the prediction of possible differential responses of several organisms that have various genomic compositions to several allergic as well as toxic compounds, toxicogenomics might also be helpful for several edible and non-edible mushrooms through in vitro as well as in vivo toxicity assessment.
Current Status in the Mushroom Propagation
Coriolus versicolor
Among all the mushrooms, Coriolus versicolor or Trametes versicolor is the best-studied and most potent medicinal mushroom. Coriolus versicolor has been well known for its anti-viral, anti-cancerous properties. This mushroom is mainly found in sub-tropical, tropicalm, and temperate zones and is highly adaptive. It has been found that the mycelial growth of medicinal mushroom Coriolus versicolor can be cultivated in the laboratory (Veena and Pandey, 2012).
Agaricus sp.
Globally, Agaricus medicinal mushroom holds fourth place in commercial mushroom production and is commercially grown in at least 90 countries with more than 4 million tons estimated annual production (Sonnenberg et al., 2011; Royse, 2014). The cultivation of mycelial growth of medicinal mushroom Agaricus sp. can be cultivated in laboratories (Golmwen et al., 2019).
Ganoderma sp.
The production of fruiting bodies of Ganoderma sp. is a long-term process that takes several months for the first fruiting body to even appear, depending upon the substrate as well as species. It has also been found that in submerged conditions, the growth of pure culture in liquid culture medium permits the acceleration of the growth due to which biomass can be brought to yield in several days (Asser et al., 2000).
It has been reported that the cultivation of medicinal mushroom Ganoderma sp. is possible in various labs in India. The mycelial of this medicinal mushroom grows well in potato dextrose medium at pH 5 and 25°C, followed by Kirk medium and glucose, malt extract as well as Kirk medium and molasses (Nasreen et al., 2005). Rai, Veena and Pandey, and Mishra and Singh, suggested that currently, medicinal mushrooms are gaining importance in the country (Rai et al., 2005; Veena and Pandey, 2012; Singh et al., 2014).
Hydnum sp.
The cultivation of mycelial growth of medicinal mushroom Hydnum sp. can be cultivated in the laboratory. It has been found that the most suitable carbon and nitrogen sources for the mycelial growth of Hydnum sp. are glucose and mannitol (carbon sources) as well as Ca (NO3)2 (nitrogen source) whereas the poorest mycelial growth is found to be in xylose and sucrose (carbon sources) and (NH4)2HPO4 and NH4NO3 (nitrogen sources) (Peksen et al., 2013). According to Peksen et al. (2013), Peat and peat (vermiculite mixtures) are the best media that can be used for the production of vegetative inoculum of Hydnum sp. (Peksen et al., 2013).
Coprinus sp.
It has been seen that the culture of medicinal mushroom Coprinus sp. can be cultivated in laboratories (Luo et al., 2004).
Morchella sp.
The biomass production of medicinal mushroom Morchella sp. can be done in laboratories by using Malt extract agar medium (MEA). It has also been found that the mixture of peat, sawdust, and chestnut crust (domestic waste); peat; peat, rice hulls; peat, wheat; peat, potato crust (domestic waste); peat, sawdust and peat, rice hulls, sawdust can be used as additional material (Eliuz and Goksen, 2017).
Cantharellus sp.
The cultivation of mycelial growth of medicinal mushroom Cantharellus sp. can be done in laboratories. It has been found that Murashige and Skoog (MS) medium can be used for the growth along with nitrogen (peptone, beef extract, ammonium citrate, potassium nitrate ammonium nitrate, yeast extract, sodium nitrate, and ammonium sulfate) and carbon (xylose, galactose, glucose, sucrose, and fructose) sources (Deshaware et al., 2021).
Amanita sp.
The cultivation of mycelial growth of medicinal mushroom Amanita sp. can be done in the laboratory and has also been found that the species in subgenus Lepidella are more easily to isolate and grow in the culture as compared to the species of subgenus Amanitopsis (Zhang et al., 2005).
Cortinarius sp.
Globally it has been found that the Cortinarius sp. is one of the largest genera of medicinal mushrooms. Although the genus Cortinarius is very diverse and it is very easy to figure out the species of Cortinarius from other species of mushroom because of the presence of diagnostic macroscopic characteristics (Itoo et al., 2015).
The cultivation of medicinal mushroom Cortinarius sp. can be achieved easily in a laboratory (Matheny et al., 2006).
Tremella sp.
The mycelial growth of medicinal mushroom Tremella sp. can be cultured in laboratories. It has also been found that this mycelial growth of Tremella sp. can be grown on potato dextrose agar along with cycloheximide (Jang et al., 2014).
Rigidoporus sp.
The cultivation of mycelial growth of medicinal mushroom Rigidoporus sp. can be achieved in laboratories. It has also been found that the mycelial growth of Rigidoporus sp. can be grown in potato dextrose agar medium and is amended with chloramphenicol and streptomycin sulfate for the suppression of bacterial growth (Go et al., 2021).
Grifola sp.
The mycelial growth of medicinal mushroom Grifola sp. can be cultivated in labs. It has been demonstrated that the production of mycelial growth of Grifola sp. can occur in a basal nutrient medium (peptone, sucrose, CaCl2.2H2O, yeast extract, MnSO4.H2O, NH4NO3, KNO3, KPO4H2, H3BO3, KI, Na2MoO4.2H2O, ZnSO4.7H2O, MgSO4.7H2O) supplemented with amino acids (inositol, thiamine, pyridoxine, nicotinic acid); vitamins; ascorbic acid (Cicarelli, Argentina, Santa Fé); folic acid (alanine, riboflavin, phenylalanine and tryptophan) (Postemsky and Curvetto, 2015).
Lentinus sp.
The cultivation of mycelial growth of Lentinus sp. works in the laboratory (Mossebo, 2002). It has been found that Lentinus sp. evidenced the growth of mycelia and found the high density in potato dextrose agar whereas a lesser density of mycelia was found in rice bran extract agar (Castillo et al., 2017).
Pleurotus sp.
The fruiting bodies of medicinal mushroom pleurotus sp. can be produced in laboratories (Shnyreva et al., 2017). It has been found that the mycelial growth of pleurotus sp. can be done on potato dextrose agar (Silva et al., 2013).
Calocybe sp.
The cultivation of the medicinal mushroom Calocybe sp. can work in laboratories. For the culture of medicinal mushroom Calocybe sp., potato dextrose agar medium can be used (Singh et al., 2017).
Huitlacoche sp.
The mycelial growth of medicinal mushroom Huitlacoche sp. can be grown in laboratories (Montiel et al., 2015).
Cordyceps sp.
The cultivation of the mycelial growth of medicinal mushroom Cordyceps sp. can be grown in culture in laboratories (Ghatnur et al., 2015).
Conclusion
Mushrooms are a valuable fungus that occurs as an essential component of the ecosystem. Globally, mushrooms have been well known for their nutritional and therapeutic values. They are an economically, biotechnologically, and nutritionally valued group of organisms because of their importance in medicines, biocontrol, food, and the biological, chemical, cosmeceutical, and various other industries. Medicinal mushrooms have various bioactive (primary as well as secondary) metabolites that have been explored but there are still many indigenous mushrooms and their metabolites are unknown, therefore, it is necessary for researchers to discover many other unknown metabolites which are not discovered yet. Medicinal mushrooms also possess several essential biological effects that are beneficial for human health. Genomics, proteomics, and metabolomics can play a major role in improving the active metabolic component of mushroom research.
Author Contributions
SKK created the manuscript idea and structure. AB and SKK wrote the manuscript. MS, VM, and SM took suggestions and discussions continuously for the improvement of the manuscript. All authors had gone through the manuscript and revised it before submission.
Conflict of Interest
The authors declare that the research was conducted in the absence of any commercial or financial relationships that could be construed as a potential conflict of interest.
Publisher’s Note
All claims expressed in this article are solely those of the authors and do not necessarily represent those of their affiliated organizations, or those of the publisher, the editors and the reviewers. Any product that may be evaluated in this article, or claim that may be made by its manufacturer, is not guaranteed or endorsed by the publisher.
Acknowledgments
The authors are grateful to M/s Xcel Life Science, California, United States, for their financial support of this work (Grant No. SBSU/XcelLs/2021/4/P1). The authors are also thankful to Gaurav Deep Singh, Chancellor, Sardar Bhagwan Singh University, Balawala, Dehradun, Uttarakhand, India, for providing facility, space, and resources to conduct this work successfully.
Abbreviations
ITS, Internal Transcribed Spacer; HPLC, high-pressure liquid chromatography; NMR, nuclear magnetic resonance spectroscopy microscopy; TLC, thin-layer chromatography; GC-MS, gas chromatography-mass spectrometry; UPLC, ultra-performance liquid chromatography; FTIR, fourier transform infrared spectroscopy; LCMS-TOF, liquid chromatography quadrupole time-of-flight mass spectrometry; HPTLC, high-performance thin-layer chromatography; MALDI-TOF MS, matrix-assisted laser desorption ionization-time of flight mass spectrometry; USDA, United States Department of Agriculture; NO, nitric oxide; HPV 16, anti-human papillomavirus 16; AChE, acetylcholinesterase; BChE, butyrylcholinesterase.
References
Aardema, M. J., and MacGregor, J. T. (2002). Toxicology and genetic toxicology in the new era of toxicogenomics: impact of “omics” technologies. Mutat. Res. 499, 13–25. doi: 10.1016/s0027-5107(01)00292-5
Adachi, Y., Okazaki, M., Ohno, N., and Yadomae, T. (1994). Enhancement of cytokine production by macrophages stimulated with (1→3)-βD-glucan, grifolan (GRN), isolated from Grifola frondosa. Biol. Pharm. Bull. 17, 1554–1560. doi: 10.1248/bpb.17.1554
Agrahar- Murugkar, D., and Subbulakshmi, G. (2005). Nutritional value of edible wild mushrooms collected from the khasi hills Meghalaya. Food Chem. 89, 599–603. doi: 10.1016/j.foodchem.2004.03.042
Ahmad, N., Bansal, A. K., and Kidwai, J. R. (1984a). Effects of PHA-B fraction of Agaricus bisporus lectin on insulin release and 45Ca2+ uptake by islets of Langerhans in vitro. Acta Diabetol. 21, 63–70. doi: 10.1007/BF02624765
Ahmad, N., Khan, M. M., Rastogi, A. K., and Kidwai, J. R. (1984b). Effect of age on Agaricus bisporus PHA-B stimulated insulin release and 45Ca2+ uptake in vitro by islets of Langerhans. Acta Diabetol. 21, 349–355. doi: 10.1007/bf02582088
Aida, F. M. N. A., Shuhaimi, M., Yazid, M., and Maaruf, A. G. (2009). Mushroom as a potential source of prebiotics: a review. Trends Food Sci. Technol. 20, 567–575. doi: 10.1016/j.tifs.2009.07.007
Ajith, T. A., and Janardhanan, K. K. (2007). Indian medicinal mushrooms as a source of antioxidant and antitumor agents. J. Clin. Biochem. Nutr. 40, 157–162. doi: 10.3164/jcbn.40.157
Alam, N., Amin, R., Khan, A., Ara, I., Shim, M. J., Lee, M. W., et al. (2008). Nutritional Analysis of Cultivated Mushrooms in Bangladesh – Pleurotus ostreatus, Pleurotus sajor-caju, Pleurotus florida and Calocybe indica. Mycobiology 36, 228–232. doi: 10.4489/MYCO.2008.36.4.228
Al-Thani, R. F. (2010). Survey of macro fungi (including Truffles) in Qatar. Atlas J. Biol. 1, 26–29. doi: 10.5147/ajb.v1i2.5
Alves, M., Ferreira, I. F. R., Dias, J., Teixeira, V., Martins, A., and Pintado, M. (2012). A review on antimicrobial activity of mushroom (Basidiomycetes) extracts and isolated compound. Planta Med. 78, 1707–1718. doi: 10.1055/s-0032-1315370
Anke, T. (2020). Secondary metabolites from mushrooms. J. Antibiot. 73, 655–656. doi: 10.1038/s41429-020-0358-6
Asakawa, Y., Nagashima, F., Hashimoto, T., Toyota, M., Ludwiczuk, A., Komala, I., et al. (2014). Pungent and bitter, cytotoxic and antiviral terpenoids from some bryophytes and inedible fungi. Nat. Prod. Commun. 9, 409–417.
Ashraf, S. A., Abd Elmoneim, O. E., Siddiqui, A. J., Patel, M., Awadelkareem, A. M., Snoussi, M., et al. (2020). Cordycepin for Health and Wellbeing: a Potent Bioactive Metabolite of an Entomopathogenic Medicinal Fungus Cordyceps with Its Nutraceutical and Therapeutic Potential. Molecules 25:2735. doi: 10.3390/molecules25122735
Asser, S. P., Nevo, E., Sokolov, D., Reshetnikov, T., and Tismenetsky, M. (2000). Dietary supplements from medicinal mushrooms: diversity of types and variety of regulations. Int. J. Med. Mush. 2, 1–19. doi: 10.1615/intjmedmushr.v3.i2-3.160
Atomachi, R. (1988). Antitumor glycan polysaccharides manufacture by Coriolus versicolor. Japanese Patent JP 63:087.
Awadasseid, A., Hou, J., Gamallat, Y., Xueqi, S., Eugene, K. D., Hago, A. M., et al. (2014). Purification, characterization and antitumor activity of a novel glucan from the fruiting bodies of Coriolus versicolor. PLoS One 12:e0171270. doi: 10.1371/journal.pone.0171270
Azeem, U., Hakeem, K. R., and Ali, M. (2020). “Bioactive Constituents and Pharmacological Activities,” in Fungi for Human Health, eds U. Azeem, K. R. Hakeem, and M. Ali (Cham: Springer), 59–95. doi: 10.1007/978-3-030-58756-7_7
Badalyan, S. N. (2014). “Potential of mushroom bioactive molecules to develop healthcare biotech products,” in Proceedings of the 8th International Conference on mushroom biology and mushroom products (ICMBMP8), (New Delhi: Yugantar Prakashan Pvt. Ltd), 373–378.
Baeva, E., Bleha, R., Lavrova, E., Sushytskyi, L., þopíková, J., Jablonsky, I., et al. (2019). Polysaccharides from basidiocarps of cultivating mushroom Pleurotusostreatus: isolation and structural characterization. Molecules 24:2740. doi: 10.3390/molecules24152740
Bahl, N. (1983). “Medicinal value of edible fungi,” in Proceeding of the International conference on science and cultivation technology of edible fungi. Indian mushroom science. II, ed. T. N. Kaul (Jammu: Regional Research Laboratory), 203–209. doi: 10.1201/9780203492086.ch11
Bailey, C. J., Turner, S. L., Jakeman, K. J., and Hayes, W. A. (1984). Effect of Coprinus comatus on plasma glucose concentrations in mice. Planta Med. 50, 525–526. doi: 10.1055/s-2007-969791
Balasundram, N., Sundram, K., and Samman, S. (2006). Phenolic compounds in plants and agri-industrial by-products: antioxidant activity, occurrence and potential uses. Food Chem. 99, 191–203. doi: 10.1016/j.foodchem.2005.07.042
Barreira, J. C., Oliveira, M. B. P., and Ferreira, I. C. (2014). Development of a novel methodology for the analysis of ergosterol in mushrooms. Food Anal. Method 7, 217–223. doi: 10.1007/s12161-013-9621-9
Barros, L., Correia, D. M., Ferreira, I. C. F. R., Baptista, P., and Santos, B. C. (2008). Optimization of the determination of tocopherols in Agaricus sp. Edible mushrooms by a normal phase liquid chromatographic method. Food Chem. 110, 1046–1050. doi: 10.1016/j.foodchem.2008.03.016
Bauerova, K., Kucharska, J., Ponist, S., Slovak, L., Svik, L., Jakus, V., et al. (2019). “The Role of Endogenous Antioxidants in the Treatment of Experimental Arthritis,” in a book-Antioxidant, ed. E. Shalaby (London, UK: Intech), doi: 10.5772/intechopen.85568
Beelman, R. B., Dubost, N. J., Peterson, D. G., and Hausman, M. (2007). Phytonutrient Compositions from Mushrooms or Filamentous Fungi Comprising Ergothioneine for Treatment of Neurodegenerative Diseases and Radiation Damage Database: CAPLUS. WO 2007106859 A2 20070920. Cham: Springer International Publishing AG.
Berglund, K. A., Rova, U., and Enman, J. (2011). Method for producing eritadenine in liquid phase fermentation. US 8053217.
Bijalwan, A., Bahuguna, K., Vasishth, A., Singh, A., Chaudhary, S., Tyagi, A., et al. (2020). Insights of medicinal mushroom (Ganoderma lucidum): prospects and potential in India. Biodivers. Int. J. 4, 202–209.
Bisen, P. S., Baghel, R. K., Sanodiya, B. S., Thakur, G. S., and Prasad, G. D. K. (2010). S. Lentinus edodse: a macrofungus with pharmacological activities. Curr. Med. Chem. 17, 2419–2430. doi: 10.2174/092986710791698495
Boonsong, S., Klaypradit, W., and Wilaipun, P. (2016). Antioxidant activities of extracts from five edible mushrooms using different extractants. Agric. Nat. Res. 50, 89–97. doi: 10.1016/j.anres.2015.07.002
Breitmaier, E. (2006). Terpenes: Flavors, Fragrances, Pharmaca, Pheromones. Hoboken: John Wiley & Sons.
Butt, A. H., and Khan, Y. D. (2019). CanLect-Pred: a Cancer Therapeutics Tool for Prediction of Target Cancer lectins Using Experiential Annotated Proteomic Sequences. IEEE Access 8, 9520–9531. doi: 10.1109/ACCESS.2019.2962002
Büttner, E., Liers, C., Hofrichter, M., Gebauer, A. M., and Kellner, H. (2019). Draft Genome Sequence of Xylaria hypoxylon DSM 108379, a Ubiquitous Fungus on Hardwood. Microbiol. Resour. Announc. 8, 9–11. doi: 10.1128/MRA.00845-19
Cadet, J. L., and Bolla, K. (2007). “Environmental toxins and disorders of the nervous system,” in Neurology and Clinical Neuroscience, eds A. H. V. Schapira, E. Byrne, S. DiMauro, R. S. J. Frackowiak, R. T. Johnson, Y. Mizuno, et al. (Philadelphia, PA: Mosby), 1477–1488. doi: 10.1016/B978-0-323-03354-1.50115-2
Carlson, A., Justo, A., and Hibbett, D. S. (2014). Species delimitation in Trametes: a comparison of ITS, RPB1, RPB2 and TEF1 gene phylogenies. Mycol. Soc. Am. 106, 735–745. doi: 10.3852/13-275
Carluccio, A. (2003). Complete mushroom book. London: Quadrille Publishing. P 224. Chine Becom es world’s: Biggest Edible mushroom producer. Available Online at: https://www.Allbusiness.com/company-activities-management/product-management/7665410-1.htm.retriened (accessed October 27, 2011).
Cassagne, C., Normand, A. C., L’Ollivier, C., Ranque, S., and Piarroux, R. (2016). Performance of MALDI-TOF MS platforms for fungal identification. Mycoses 59, 678–690. doi: 10.1111/myc.12506
Castillo, T. A., Pereira, J. R. G., Alves, J. M. A., and Teixeira, M. F. S. (2017). Mycelial Growth and Antimicrobial Activity of Species of Genus Lentinus (Agaricomycetes) from Brazil. Int. J. Med. Mush. 19, 1135–1143. doi: 10.1615/IntJMedMushrooms.2017024708
Chang, C. J., Lin, C. S., Lu, C. C., Martel, J., Ko, Y. F., Ojcius, D. M., et al. (2015). Ganoderma lucidum reduces obesity in mice by modulating the composition of the gut microbiota. Nat. Commun. 6:7489.
Chang, H. H., Hsieh, K. Y., Yeh, C. H., Tu, Y. P., and Sheu, F. (2010). Oral administration of an Enoki mushroom protein FVE activates innate and adaptive immunity and induces antitumor activity against murine hepatocellular carcinoma. Int. Immunopharmacol. 10, 239–246. doi: 10.1016/j.intimp.2009.10.017
Chang, H. Y., Sheu, M. J., Yang, C. H., Lu, T. C., Chang, Y. S., Peng, W. H., et al. (2011). Analgesic effects and the mechanisms of anti-inflammation of hispolon in mice. Evid. Based Complement. Alternat. Med. 2011:478246. doi: 10.1093/ecam/nep027
Chang, S. T. (1995). “Ganoderma- the leader production and technology of mushroom nutraceuticals,” in Recent Advances in Ganoderma lucidum Research, eds B. K. Kim and Y. S. Kim (Seoul, Korea: The Pharmaceutical Society of Korea), 43–52.
Chang, S. T., and Miles, P. G. (2004). “Pleurotus – A Mushroom of Broad Adaptability,” in Mushrooms: cultivation, nutritional value, medicinal effect, and environmental impact, 2nd Edn, eds P. G. Miles and S.-T. Chang (Boca Raton: CRC Press), 315–325. doi: 10.1201/9780203492086.ch16
Chang, S. T., and Miles, P. G. (2008). Mushrooms: Cultivation, Nutritional value, medicinal effect and environmental impact, 2nd Edn. Boca Raton, Fla, USA: CRC Press.
Chang, S. T., and Wasser, S. P. (2012). The role of culinary-medicinal mushrooms on human welfare with a pyramid model for human health. Int. J. Med. Mush. 14, 95–134. doi: 10.1615/intjmedmushr.v14.i2.10
Chen, J., and Seviour, R. (2007). Medicinal importance of fungal β (1→3), (1→6)-glucans. Mycol. Res. 111, 635–652. doi: 10.1016/j.mycres.2007.02.011
Cheng, J. J., Lur, H. S., Huang, N. K., Chen, H. P., Lin, C. Y., and Lu, M. K. (2009). Exploring the potential of biopharmaceutical production by Rigidoporus ulmarius: cultivation, chemistry, and bioactivity studies. Process Biochem. 44, 1237–1244. doi: 10.1016/j.procbio.2009.06.018
Chung, C. K., and Tong, S. K. (2005). U.S. Patent No. 6,908,614. Washington, DC: U.S. Patent and Trademark Office.
Chung, C. K., and Tong, S. K. (2006). U.S. Patent No. 7,087,233. Washington, DC: U.S. Patent and Trademark Office.
CNN Health (2018). The humble mushroom is the newest super-food. Available Online at: https://edition.cnn.com/2018/04/25/health/mushroo,s-food-partner/index.html (accessed April 25, 2018).
Coetzee, M. P., Marincowitz, S., Muthelo, V. G., and Wingfield, M. J. (2015). Ganoderma species, including new taxa associated with root rot of the iconic Jacaranda mimosifolia in Pretoria. South Africa. IMA Fungus 6, 249–256. doi: 10.5598/imafungus.2015.06.01.16
Cui, F., Zan, X., Li, Y., Yang, Y., Sun, W., Zhou, Q., et al. (2013). Purification and partial characterization of a novel anti-tumor glycoprotein from cultured mycelia of Grifolafrondosa. Int. J. Biol. Macromol. 62, 684–690. doi: 10.1016/j.ijbiomac.2013.10.025
D’Archivio, M., Filesi, C., Vari, R., Scazzocchio, B., and Masella, R. (2010). Bioavailability of the polyphenols: status and controversies. Int. J. Mol. Sci. 11, 1321–1342. doi: 10.3390/ijms11041321
Daba, A. S., and Ezeronye, O. U. (2003). Minireview. Anti-cancer effect of polysaccharides isolated from higher basidiomycetes mushrooms. Afr. J. Biotechnol. 2, 272–278.
Dahima, V., Doshi, A., and Singh, H. (2020). Screening of Antifungal Activity of Pleurotus pulmonarius, Pleurotus florida and Shizophyllum commune. Int. J. Curr. Microbiol. Appl. Sci. 9, 997–1004. doi: 10.20546/ijcmas.2020.904.118
Das, K. (2010). Diversity and Conservation of wild mushrooms in Sikkim with special reference to Barsey Rhododendron Sanctuary. NeB1O 1, 1–13. doi: 10.1155/2021/8856988
Dasgupta, A. (2019). “Abuse of Magic Mushroom, Poyote Cactus, LSD, Khat and Volatiles,” in the book- Critical issues in alcohol and drugs of abuse testing, ed. A. Dasgupta (Amsterdam: Elsevier), 477–494. doi: 10.1016/b978-0-12-815607-0.00033-2
Dasgupta, A., and Acharya, K. (2019). Mushrooms: an emerging resource for therapeutic terpenoids. 3 Biotech 9:369. doi: 10.1007/s13205-019-1906-2
De Groot, F. M. P., and Hellingwerf, K. (2001). Molecular organization of the cell wall of Candida albicans. Med. Mycol. 39, 1–8. doi: 10.1080/744118876
Deepalakshmi, K., and Mirunalini, S. (2011). Therapeutic properties and current medical usage of medicinal mushroom: Ganoderma lucidum. Int. J. Pharm. Sci. Res. 2, 1922–1929.
Deepalakshmi, K., and Mirunalini, S. (2014). Pleurotus ostreatus: an oyster mushroom with nutritional and medicinal properties. J. Biochem. Technol. 52, 718–726.
Deng, G., Lin, H., and Seidman, A. (2009). A. phase I/II trial of polysaccharide extracts from Grifola frondosa (Maitake mushroom) in breast cancer patients: immunological effects. J. Cancer Res. Clin. Oncol. 135, 1215–1221. doi: 10.1007/s00432-009-0562-z
Deshaware, S., Marathe, S. J., Bedade, D., Deska, J., and Shamekh, S. (2021). Investigation on mycelial growth requirements of Cantharellus cibarius under laboratory conditions. Arch. Microbiol. 203, 1539–1545. doi: 10.1007/s00203-020-02142-0
Deshmukh, S. K. (2004). “Biodiversity of Tropical Basidiomycetes as Sources of Novel Secondary Metabolites,” in Microbiology and Biotechnology for Sustainable Development, ed. P. C. Jain (New Delhi: CBS Publishers and Distributors), 121–140.
Dikeman, C. L., Bauer, L. L., Flickinger, E. A., and Fahey, G. C. (2005). Effects of stage of maturity and cooking on the chemical composition of select mushroom varieties. J. Agric. Food Chem. 53, 1130–1138. doi: 10.1021/jf048541l
Dissanayake, A. A., Mills, G. L., Bonito, G., Rennick, B., and Nair, M. G. (2021). Chemical composition and anti-inflammatory and antioxidant activities of extracts from cultivated morel mushrooms, species of genus Morchella (Ascomycota). Int. J. Med. Mushrooms 23, 73–83. doi: 10.1615/IntJMedMushrooms.2021039297
Dundar, A., Okumus, V., Ozdemir, S., Celik, K. S., Boga, M., Özçağli, E., et al. (2015). Antioxidant, antimicrobial, cytotoxic and anticholinesterase activities of seven mushroom species with their phenolic acid composition. J. Hortic. 2, 1–6.
Duru, M. E., and Cayan, G. T. (2015). Biologically active terpenoids from mushroom origin: a review. Rec. Nat. Prod. 9, 456–483.
E1 Enshasy, H. A., and Hatti-Kaul, R. (2013). Mushroom immunomodulators: unique molecules with unlimited applications. Trends Biotechnol. 31, 668–677. doi: 10.1016/j.tibtech.2013.09.003
Eliuz, E. A. E., and Goksen, G. (2017). Morel Culture Study Part I: Morchela sp. mycelial growth and nutrient-primed mycelia. J. Appl. Biol. Biotechnol. 5, 066–069. doi: 10.7324/jabb.2017.50111
Elkhateeb, W. A., and Daba, G. M. (2019). Thomas PW and Wen TC: medicinal mushrooms as a new source of natural therapeutic bioactive compounds. Egypt. Pharm. J. 18, 88–101.
Elmastas, M., Isildaak, O., Turkekul, I., and Temur, N. (2007). Determination of antioxidant activity and antioxidant compounds in wild edible mushrooms. J. Food Comp. Anal. 20, 337–345. doi: 10.1016/j.jfca.2006.07.003
Elsayed, E. A., E1 Enshasy, H., Wadaan, M. A., and Aziz, R. (2014). Mushrooms: a potential natural source of anti-inflammatory compounds for medical applications. Mediat. Inflamm. 2014:805841. doi: 10.1155/2014/805841
Enow, E. (2013). Diversity and distribution of macro fungi (mushrooms) in the Mount Cameroon Region. J. Ecol. Nat. Environ. 5, 318–334. doi: 10.5897/jene2013.0397
Ergomul, P. G., Akata, I., Kalyoncu, F., and Ergonul, B. (2013). Fatty acid compositions of six wild edible mushrooms species. Sci. World J. 2013:163964 doi: 10.1155/2013/163964
Ewart, R. B. L., Kornfeld, S., and Kipnis, D. M. (1975). Effect of lectins on hormone release from isolated rat islets of Langerhans. Diabetes 24, 705–714. doi: 10.2337/diab.24.8.705
Falch, B. H., Espevik, T., Ryan, L., and Stokke, B. T. (2000). The cytokine stimulating activity of (1→3)-β-D-glucans is dependent on the triple helix conformation. Carbohydr. Res. 329, 587–596. doi: 10.1016/s0008-6215(00)00222-6
Fan, J. M., Zhang, J. S., Tang, Q. J., Liu, Y. F., Zhang, A. Q., and Pan, Y. J. (2006). Structural elucidation of a neutral fucogalactan from the mycelium of Coprinus comatus. Carbohydr. Res. 341, 1130–1134. doi: 10.1016/j.carres.2006.03.039
Feeney, M. J., Dwyer, J., Hater-lewis, C. M., Milner, J. A., Noakes, M., Rowe, S., et al. (2014). Mushroom and health summit proceedings. J. Nutr. 144, 11285–11365. doi: 10.3945/jn.114.190728
Ferreira, I. C. F. R., Barros, L., and Abreu, R. M. V. (2009). Antioxidants in wild mushrooms. Curr. Med. Chem. 16, 1543–1560. doi: 10.2174/092986709787909587
Finimundy, T. C., Gambato, G., Fontana, R., Camassola, M., Salvador, M., Moura, S., et al. (2013). Aqueous extracts of Lentinula edodes and Pleurotus sajor-caju exhibit high antioxidant capability and promising in vitro antitumor activity. Nutr. Res. 33, 76–84. doi: 10.1016/j.nutres.2012.11.005
Gadgil, P. D. (2005). Fungi on trees and shrubs in New Zealand Fungi of New Zealand, Fungal Diversity Research Series 16. Hong Kong: Fungal Diversity, 437–458.
Gbolagade, S. J. (2006). Bacteria associated with compost used for cultivation of Nigerian edible mushroom Pleurotustuber-regium (Fr) singer and Lentinus Squarrouslus Berk. Afr. J. Biotechnol. 5, 338–342.
Ghatnur, S. M., Parvatam, G., and Balaraman, M. (2015). Culture Conditions for Production of Biomass, Adenosine, and Cordycepin from Cordyceps sinensis CS1197: optimization by Desirability Function Method. Pharmacogn. Mag. 11, S448–S456. doi: 10.4103/0973-1296.168946
Ghosh, K. (2016). A review: edible mushrooms as source of dietary fiber and its health effects. J. Phy. Sci. 21, 129–137.
Giavasis, I. (2014). Polysaccharides from medicinal mushrooms for potential use as nutraceuticals. Polysaccharides 1, 171–176.
Glamočlija, J., Stojković, D., Nikolić, M., Ćirić, A., Reis, F. S., Barros, L., et al. (2015). A comparative study on edible Agaricus mushrooms as functional foods. Food Funct. 6, 1900–1910. doi: 10.1039/c4fo01135j
Go, W. Z., Chin, K. L., Hong, P. S., Wong, M. Y., Luqman, C. A., Surendran, A., et al. (2021). Virulence of Rigidoporus microporus isolates causing White Root Rot Disease on Rubber Trees (Hevea brasiliensis) in Malaysia. Plants 10:2123. doi: 10.3390/plants10102123
Golmwen, S. A., Gberikon, G. M., and Ogbonna, I. O. (2019). Cultivation of edible mushrooms (Agaricus bisporus) in the laboratory and determination of proximate and mineral compositions of cultivated mushrooms with other protein sources. Int. J. Recent Sci. Res. 10, 30514–30516.
Grand, L. F., and Vernia, C. S. (2002). New Taxa and hosts of poroid wood-decay fungi in North Carolina. Castanea 67, 193–200.
Gray, A. M., and Flatt, P. R. (1998). Insulin-releasing and insulin-like activity of Agaricus campestris (mushroom). J. Endocrinol. 157, 259–266. doi: 10.1677/joe.0.1570259
Grothe, T., Stadler, M. Köpcke, B., Roemer, E., Bitzer, J., and Wabnitz, P. (2012). Terpenoid spiro Ketal Compounds with LXR Agonists Activity, Their use and Formulations with them. EP2468253 A1. Dortmund: World International Property Organization (WIPO).
Gu, C. Q., Li, J. W., Chao, F., Jin, M., Wang, X. W., and Shen, Z. Q. (2007). Isolation, identification and function of a novel anti-HSV-1 protein from Grifola frondosa. Antivir. Res. 75, 250–257. doi: 10.1016/j.antiviral.2007.03.011
Guillamon, E., Garcia-Lafuente, A., Lozano, M., D’Arrigo, M., Rostagno, M. A., Villares, A., et al. (2010). Edible mushrooms: role in the prevention of cardiovascular diseases. Fitoterapia 81, 715–723. doi: 10.1016/j.fitote.2010.06.005
Habtemariam, S. (2020). Trametes versicolor (Synn. Coriolus versicolor) polysaccharides in cancer therapy: targets and efficacy. Biomedicines
Han, C., and Cui, B. (2012). Pharmacological and pharmacokinetic studies with agaricoglycerides, extracted from Grifola frondosa, in animal models of pain and inflammation. Inflammation 35, 1269–1275. doi: 10.1007/s10753-012-9438-5
Harsh, N. S. K., Rai, B. K., and Tiwari, D. P. (1993). Use of Ganoderma lucidum in folk medicine. J. Trop. Biodivers. 1, 324–326.
Heleno, S. A., Barros, L., Martins, A., Morales, P., Fernández-Ruiz, V., Glamoclija, J., et al. (2015a). Nutritional value, bioactive compounds, antimicrobial activity and bio accessibility studies with wild edible mushrooms. LWT-Food Sci. Technol. 63, 799–806. doi: 10.1016/j.lwt.2015.04.028
Heleno, S. A., Martins, A., Queiroz, M. J. R., and Ferreira, I. C. (2015b). Bioactivity of phenolic acids: metabolites versus parent compounds: a review. Food Chem. 173, 501–513. doi: 10.1016/j.foodchem.2014.10.057
Heleno, S. A., Barros, L., Martins, A., Queiroz, M. J., Santos-Buelga, C., Ferreira, I. C., et al. (2012a). Phenolic, polysaccharidic and lipidic fractions of mushrooms from North-eastern Portugal: chemical compounds with antioxidant properties. J. Agric. Food Chem. 60, 4634–4640. doi: 10.1021/jf300739m
Heleno, S. A., Barros, L., Martins, A., Queiroz, M. J. R. P., Santos-Buelga, C., and Ferreira, I. C. F. R. (2012b). Portugal: chemical compounds with antioxidant properties. J. Agric. Food Chem. 60, 4634–4640.
Heleno, S. A., Barros, L., Sousa, M. J., Martins, A., and Ferreira, I. C. F. R. (2010). Tocopherol’s composition of portuguese wild mushrooms with antioxidant capacity. Food Chem. 119, 1443–1450. doi: 10.1016/j.foodchem.2009.09.025
Hennicke, F., Cheikh-Ali, Z., Liebisch, T., Macia-Vicente, J. G., Bode, H. B., and Piepenbring, M. (2016). Distinguishing commercially grown Ganoderma lucidum from Ganoderma lingzhi from Europe and East Asia on the basis of morphology, molecular phylogeny, and triterpenic acid profiles. Phytochemistry 127, 29–37. doi: 10.1016/j.phytochem.2016.03.012
Hensley, K., Benaksas, E. J., Bolli, R., Comp, P., Grammas, P., Hamdheydari, L., et al. (2004). New perspectives on vitamin E: γ-tocopherol and carboxyethylhydroxychroman metabolites in biology and medicine. Free Radic. Biol. Med. 36, 1–15. doi: 10.1016/j.freeradbiomed.2003.10.009
Hetland, G., Johnson, E., Lyberg, T., and Kvalheim, G. (2011). The mushroom Agaricusblazei Murill elicits medicinal effects on tumor, infection, allergy and inflammation through Its modulation of innate immunity and amelioration of Th1/Th2 imbalance and inflammation. Adv. Pharmacol. Sci. 2011:157015. doi: 10.1155/2011/157015
Hobbs, C. R. (2004). Medicinal value of Turkey tail fungus Trametes versicolor (L.:Fr.) pilat (Aphyllophoromycetideae). A literature review. Int. J. Med. Mushrooms 6, 195–218.
Hong, S. G., and Jung, H. S. (2004). Phylogenetic analysis of Ganoderma based on nearly complete mitochondrial small-subunit ribosomal DNA sequences. Mycologia 96, 742–755. doi: 10.1080/15572536.2005.11832922
Hu, Y., Teng, C., Yu, S., Wang, X., Liang, J., Bai, X., et al. (2017). Inonotus obliquus polysaccharide regulates gut microbiota of chronic pancreatitis in mice. AMB Exp. 7:39. doi: 10.1186/s13568-017-0341-1
Ilyicheva, T. N., Teplyakova, T. V., Svyatchenko, S. V., Asbaganov, S. V., Zmitrovich, I. V., and Vlasenko, A. V. (2020). Antiviral activity of total polysaccharide fraction of water and ethanol extracts of Pleurotus pulmonarius against the influenza A virus. Curr. Res. Environ. Appl. Mycol. 10, 224–235. doi: 10.5943/cream/10/1/22
International Union of Pure and Applied Chemistry (1995). alkaloids”. Compendium of Chemical Terminology. Internet edition. Research Triangle Park: International Union of Pure and Applied Chemistry
Ishibashi, K. I., Miura, N. N., Adachi, Y., Ohno, N., and Yadomae, T. (2001). Relationship between solubility of grifolan, a fungal 1,3-β-D-glucan, and production of tumor necrosis factor by macrophages in vitro. Biosci. Biotechnol. Biochem. 65, 1993–2000. doi: 10.1271/bbb.65.1993
Itoo, Z. A., Reshi, Z. A., Basharat, Q., Majeed, S. T., and Andrabi, K. I. (2015). Identification and characterization of ectomycorrhizal Cortinarius species (Agaricales, Basidiomycetes) from temperate Kashmir Himalaya, India, by ITS barcoding. Adv. Mol. Biol. 2015:507684. doi: 10.1155/2015/507684
Jang, Y. J., Li, C. H., Li, X., Wen, Z. Q., Huang, Q. H., Zhang, Q. H., et al. (2014). Streptomyces Tremellae sp. Nov., isolated from a culture of the mushroom Tremella fuciformis. Int. J. Syst. Evol. Microbiol. 66, 5028–5033. doi: 10.1099/ijsem.0.001464
Janjusevic, L., Karaman, M., Sibul, F., Tommonaro, G., Iodice, C., JAakovljevic, D. M., et al. (2017). The lignicolous fungus Trametes versicolor (L.) Lloyd (1920): a promising natural source of antiradical and AChE inhibitory agents. J. Enzyme Inhib. Med. Chem. 32, 355–362. doi: 10.1080/14756366.2016.1252759
Jaworska, G., Pogon, K., Bernas, E., and Duda-Chodak, A. (2015). Nutraceuticals and antioxidant activity of prepared for consumption commercial mushrooms Agaricus bisporus and Pleurotus ostreatus. J. Food Qual. 38, 111–122. doi: 10.1111/jfq.12132
Jong, S. C., and Birmingham, J. M. (1992). Medicinal Benefits of the Mushroom Ganoderma. Adv. Appl. Microbiol. 37, 101–134. doi: 10.1016/s0065-2164(08)70253-3
Kalac, P. (2013). A review of chemical composition and nutritional value of wild-growing and cultivated mushrooms. J. Sci. Food Agric. 93, 209–218. doi: 10.1002/jsfa.5960
Kalac, P. (2016). Edible mushrooms: Chemical composition and Nutritional value. Cambridge, Massachusetts: Academic Press.
Kataoka, K., Muta, T., Yamazaki, S., and Takeshige, K. (2002). Activation of macrophages by linear (1→3)-β-D-glucans. Implications for the recognition of fungi by innate immunity. J. Biol. Chem. 277, 36825–36831. doi: 10.1074/jbc.M206756200
Khan, M. A., Tania, M., Liu, R., and Rahman, M. M. (2013). Hericiumerinaceus: an edible mushroom with medicinal values. J. Complement. Integr. Med. 10, 253–258.
Khatua, S., Paul, S., and Acharya, K. (2013). Mushroom as the potential source of new generation of antioxidant: a review. Res. J. Pharm. Technol. 6, 496–505.
Kindzel’skii, L. P., Zlochevskaia, L. L., Tsyganok, T. V., and Shabaeva, M. M. (1995). The effect of natural immunomodulators on the lymphocytic natural killer activity in patients with malignant lymphoproliferative diseases. Lik. Sprava 1–2, 146–148.
Kirk, P. M., Cannon, P. F., Minter, D. W., and Stalpers, J. A. (2008). Dictionary of the Fungi (10th ed.). Wallingford, UK: CAB International.
Kiss, A., Grunvaid, P., Ladanyi, M., Papp, V., Papp, I., Nemedi, E., et al. (2021). Heat treatment of Reishi medicinal mushroom (Ganoderma lingzhi) basidiocarp enhanced its β- glucan solubility, antioxidant capacity and lactogenic properties. Foods 10:2015. doi: 10.3390/foods10092015
Klaus, A., Kozarski, M., Vunduk, J., Petrović, P., and Nikšić, M. (2017). Antibacterial and antifungal potential of wild basidiomycete mushroom Ganoderma applanatum. Lek. Sirovine 36, 37–46. doi: 10.5937/leksir1636037k
Ko, Y. F., Martel, J., Liau, J. C., Chang, I. T., Jian, W. T., Mei-Feng, L. I. N., et al. (2017). U.S. Patent No. 9,758,595. Washington, DC: U.S. Patent and Trademark Office.
Kong, Q., Dong, S., Gao, J., and Jiang, C. (2016). In vitro fermentation of sulphated polysaccharides from E. prolifera and L. japonica by human fecal microbiota. Int. J. Biol. Macromol. 91, 867–871. doi: 10.1016/j.ijbiomac.2016.06.036
Koyyalamudi, S. R., Jeong, S. C., Song, C. H., Cho, K. Y., and Pang, G. (2009). Vitamin D2 for nation and bioavailability from Agaricus bisporus button mushrooms treated with ultraviolet irradiation. J. Agric. Food Chem. 57, 3351–3355. doi: 10.1021/JF8039089
Kozarski, M., Klaus, A., Jakovljevic, D., Todorovic, N., Vunduk, J., Petrović, P., et al. (2015). Molecules antioxidants of edible mushrooms. Molecules 20, 19489–19525. doi: 10.3390/molecules201019489
Kristiansen, B. (2015). U.S. Patent No. 9,072,776. Washington, DC: U.S. Patent and Trademark Office.
Kumar, K. (2015). Role of edible mushrooms as functional foods-a review. South Asian. J. Food Technol. Environ. 1, 211–218. doi: 10.46370/sajfte.2015.v01i03and04.02
Kumar, S., and Pandey, A. K. (2013). Chemistry and Biological Activities of Flavonoids: an Overview. Sci. World J. 2013:162750. doi: 10.1155/2013/162750
Kumari, D., Reddy, M. S., and Upadhyay, R. C. (2011). Antioxidant activity of three species of wild mushroom genus Cantharellus collected from North-Western Himalaya, India. Int. J. Agric. Biol. 13, 415–418.
Kumari, P., Choudhary, S. K., and Kumar, A. (2019). “In-vitro study of antifungal activity of Lentinus edodes mushroom extract against Alternaria triticina,” in Biotechnology and Biological Sciences: Proceedings of the 3rd International Conference of Biotechnology and Biological Sciences (BIOSPECTRUM 2019), eds R. Sen, S. Mukherjee, R. Paul, and R. Narula (Boca Raton: CRC Press), Vol. 392, 8–10.
Kumari, S., Karn, S. S., and Singh, R. K. (2021). Plant as factories for antioxidant production. Int. J. Sci. Innov. Res. 9, 1–5.
Lai, L. K., Abidin, N. Z., Abdullah, N., and Sabaratnam, V. (2010). Anti-human papillomavirus (HPV) 16 E6 activity of Ling Zhi or Reishi medicinal mushroom, Ganoderma lucidum (W. Curt.: Fr.) P. Karst. (Aphyllophoromycetideae) extracts. Int. J. Med. Mush. 12, 279–286. doi: 10.1615/intjmedmushr.v12.i3.70
Lallawmsanga, Passari, A. K., Mishra, V. K., Leo, V. V., Singh, B. P., Meyyappan, G. V., et al. (2016). Anti-microbial potential, identification and phylogenetic affiliation of wild mushrooms from two sub-tropical semi-evergreen Indian forest ecosystems. PLoS One 11:e0166368. doi: 10.1371/journal.pone.0166368
Lee, I., Ahn, B., Choi, J., Hattori, M., Min, B., Bae, K., et al. (2011). Selective cholinesterase inhibition by lanostane triterpenes from fruiting bodies of Ganoderma lucidum. Bioorgan. Med. Chem. Lett. 21, 6603–6607. doi: 10.1016/j.bmcl.2011.04.042
Lee, S., Lee, D., Jang, T. S., Kang, K. S., Nam, J. W., Lee, H. J., et al. (2017). Anti-inflammatory phenolic metabolites from the edible fungus Phellinus baumii in LPS-Stimulated RAW 264.7 cells. Molecules 22:1583. doi: 10.3390/molecules22101583
Lenzi, J., Costa, T. M., Alberton, M. D., Goulart, J. A., and Tavares, L. B. (2018). Medicinal fungi: a source of antiparasitic secondary metabolites. Appl. Microbiol. Biotechnol.. 102, 5791–5810. doi: 10.1007/s00253-018-9048-8
Li, K., Zhuo, C., Teng, C., Yu, S., Wang, X., Hu, Y., et al. (2016). Effects of Ganoderma lucidum polysaccharides on chronic pancreatitis and intestinal microbiota in mice. Int. J. Biol. Macromol. 93, 904–912. doi: 10.1016/j.ijbiomac.2016.09.029
Lin, C. H., Sheu, G. T., Lin, Y. W., Yeh, C. S., Huang, Y. H., Lai, Y. C., et al. (2010). A new immunomodulatory protein from Ganoderma microsporum inhibits epidermal growth factor mediated migration and invasion in A549 lung cancer cells. Process Biochem. 45, 1537–1542. doi: 10.1016/j.procbio.2010.06.006
Lindequist, U., Niedermeyer, T. H. J., and Julich, W. D. (2005a). The pharmacological potential of mushrooms. Evid. Based Complement. Alternat. Med. 2, 285–299. doi: 10.1093/ecam/neh107
Lindequist, U., Timo, H. J., Niedermeyer, Y., and Julich, W. D. (2005b). pharmacological potential of mushroom. Evid. Based Complement. Alternat. Med. 2, 285–299.
Liu, Z., Cang, L., Chen, C., and Zhou, G. (2008). Method for Preparing Gold Needle Mushroom Canned Food for Decreasing Blood Sugar and Adjusting Blood Fat Database: CAPLUS. CN 101292726 A 20081029. Cham: SpringerNature.
Liyanage, A. D. S. (1997). “Rubber,” in Soil Borne Diseases of Tropical Crops, eds R. J. Hillocks and J. M. Walter (Wallingford, UK: CAB International), 331–347. doi: 10.1002/9781119769354.ch6
Luo, H., Mo, M., Huang, X., Li, X., and Zhang, K. (2004). Coprinus comatus: a basidiomycetes fungus forms novel spiny structures and infects nematode. Mycologia 96, 1218–1225. doi: 10.2307/3762137
Lynn, V. (2017). Mass Spectrometry for the Detection of Endogenous Steroids and Steroid Abuse in (Race) Horses and Human Athletes. Mass Spectrom. 7, 229–252. doi: 10.1002/dta.2109
Ma, G., Kimatu, B. M., Zhao, L., Yang, W., Pei, F., Hu, Q., et al. (2017). In vivo fermentation of a Pleurotuseryngii polysaccharide and its effects on fecal microbiota composition and immune response. Food Funct. 8, 1810–1812. doi: 10.1039/c7fo00341b
Mahajna, J. A., Yassin, M., and Wasser, S. P. (2007). U.S. Patent No. 7,258,862. Washington, DC: U.S. Patent and Trademark Office.
Maia, T. M., Lopes, S. T., Almeida, J. M. G., Rosa, L. H., Sampaio, J. P., Goncalves, P., et al. (2015). Evolution of mating systems in basidiomycetes and the genetic architecture underlying mating-type determination in the yeast Leucosporidiumscottii. Genetics 201, 75–89. doi: 10.1534/genetics.115.177717
Mandal, E. K., Maity, K., Maity, S., Gantait, S. K., Behera, B., Maiti, T. K., et al. (2012). Chemical analysis of an immune-stimulating (1→4)-, (1→6)-branched glucan from an edible mushroom, Calocybe indica. Carbohydr. Res. 347, 172–177. doi: 10.1016/j.carres.2011.10.040
Manikandan, K. (2011). “Nutritional and medicinal values of mushrooms,” in Mushrooms cultivation, marketing and consumption, eds M. Singh, B. Vijay, S. Kamal, and G. C. Wakchaure (Solan: Directorate of mushroom research), 11–14. doi: 10.1615/intjmedmushrooms.v18.i10.40
Manzi, P., Aguzzi, A., and Pizzoferrato, L. (2001). Nutritional value of mushrooms widely consumed in Italy. Food Chem. 73, 321–325. doi: 10.1016/s0308-8146(00)00304-6
Martinez-Medina, A. G., Chavez-Gonzalez, M. L., Verma, D. K., Barragan, L. A. P., and Martinez-Hernandez, J. L. (2021). Bio-functional components in mushrooms, a health opportunity: ergothionine and Huitlacohe as recent trends. J. Funct. Foods 77:104326. doi: 10.1016/j.jff.2020.104326
Masuda, Y., Kodama, N., and Nanba, H. (2006). Macrophage J774. 1 cell is activated by MZ-Fraction (Klasma-MZ) polysaccharide in Grifola frondosa. Mycoscience 47, 360–366. doi: 10.1007/s10267-006-0315-7
Masuda, Y., Murata, Y., Hayashi, M., and Nanba, H. (2008). Inhibitory effect of MD fraction on tumor metastasis: involvement of NK cell activation and suppression of intercellular adhesion molecule (ICAM)-1 expression in lung vascular endothelial cells. Biol. Pharm. Bull. 31, 1104–1124. doi: 10.1248/bpb.31.1104
Matheny, P. B., Curtis, J. M., and Hofstetter, V. (2006). Major clades of Agaricales: a multilocus phylogenetic overview. Mycologia 98, 982–995. doi: 10.3852/mycologia.98.6.982
Mau, J. L., Chang, C. N., Huang, S. J., and Chen, C. C. (2004). Antioxidant properties of methanolic extract from Grifola frondose, Morchella esculenta and Termitomyces albuminosus mycelia. Food Chem. 87, 111–118. doi: 10.1016/j.foodchem.2003.10.026
McIntosh, M., Stone, B. A., and Stanisich, V. A. (2005). Curdlan and other bacterial (1→3)-β-D-glucans. Appl. Microbiol. Biotechnol. 68, 163–173. doi: 10.1007/s00253-005-1959-5
Meena, B., Sivakumar, V., and Praneetha, S. (2020). Prospects of biodiversity and distribution of mushroom fungi in India. GSC Biol. Pharm. Sci. 13, 078–085.
Miao, J., Regenstein, J. M., Qiu, J., Zhang, J., Zhang, X., Li, H., et al. (2020). Isolation, structural characterization and bioactivities of polysaccharides and its derivatives from Auricularia-A review. Int. J. Biol. Macromol. 150, 102–113. doi: 10.1016/j.ijbiomac.2020.02.054
Montiel, M. J., Romero-Maldonado, A., Monreal-Escalante, E., Becerra-Flora, A., Korban, S. S., Rosales-Mendoza, S., et al. (2015). The Corn Smut (Huitlacoche) as a New Platform for Oral Vaccines. PLoS One 10:e0133535. doi: 10.1371/journal.pone.0133535
Mossebo, D. C. (2002). Growth of wood-inhabiting Lentinus species from Cameroon in laboratory culture. Mycologist 16, 168–171.
Mothana, R. A. A., Awadh, N. A. A., Jansen, R., and Lindequist, U. (2003). Antiviral lanostanoid triterpenes from the fungus Ganoderma freifferi. Int. J. Med. Mush. 74, 177–180. doi: 10.1016/s0367-326x(02)00305-2
Nasreen, Z., Kausari, T., Nadeem, M., and Bajwa, R. (2005). Study of different growth parameters in Ganoderma lucidum. Micol. Aplicada Int. 17:5.
National Center for Biotechnology Information [NCBI] (2022b). PubChem Compound Summary for CID 439212. Glycoprotein Available Online at: https://pubchem.ncbi.nlm.nih.gov/compound/Glycoprotein (accessed August 08, 2005).
National Center for Biotechnology Information [NCBI] (2022a). PubChem Compound Summary for CID 124886. Glutathione Available Online at: https://pubchem.ncbi.nlm.nih.gov/compound/Glutathione (accessed June 08, 2005).
Negi, R., Karn, S. S., and Singh, R. K. (2020). An outlook of Pandemic disease: COVID 19. Int. J. Sci. Innov. Res. 8, 15–23.
Niazi, A. R., and Ghafoor, A. (2021). Different ways to exploit mushrooms: a review. All Life 14, 450–460. doi: 10.1128/AAMCol.2Nov.2007
Nitha, B., Meera, C. R., and Janardhanan, K. K. (2007). Anti-inflammatory and antitumor activities of cultured mycelium of morel mushroom, Morchella esculenta. Curr. Sci. 92, 235–239. doi: 10.1615/intjmedmushrooms.v18.i3.60
O’Gorman, A., Barry-Ryan, C., and Frias, J. M. (2011). Evaluation and identification of markers of damage in mushrooms (Agaricus bisporus) postharvest using a GC/MS metabolic profiling approach. Metabolomics 8, 120–132. doi: 10.1007/s11306-011-0294-3
Oghenekaro, A. O., Miettinen, O., Omorusi, V. I., Evueh, G. A., Farid, M. A., Gazis, R., et al. (2014). Molecular phylogeny of Rigidoporus microporus isolates associated with white rot disease of rubber trees (Heveabrasiliensis). Fungal Biol. 18, 495–506. doi: 10.1016/j.funbio.2014.04.001
Okhuoya, J., Akpaja, E., Osemwegie, O., Oghenekaro, A., and Ihayere, C. (2010). Nigerian mushrooms: underutilized non-wood forest resources. J. Appl. Sci. Environ. Manage. 14, 43–54.
Okwulchie, I. C., and Odunze, E. I. (2004). Evaluation of the nutritional value of some tropical edible mushrooms. J. Sustain. Agric. Environ. 6, 157–162.
Olawale, A. F., and Muhammed, N. O. M. (2017). “Fungi: a review on mushrooms,” in Proceeding on Ist International Conference on Science and Education Sustainable Development 8th-9th October, Knowledge Glob. Dev. (Akure: Federal University of Technology), 18–31.
Oso, B. A. (1975). Mushrooms and Yoruba people of Nigeria. Mycologia 67, 311–319. doi: 10.2307/3758423
Overholts, L. O. (1939). Geographical distribution of some American Polyporaceae. Mycologia 13, 629–652. doi: 10.2307/3754336
Ozturk, C., Kasik, G., Dogan, H. H., and Aktas, S. (2003). Macro fungi of Alanya District. Turk. J. Bot. 27, 303–312.
Ozturk, M., Tel, G., Ozturk, F. A., and Duru, M. E. (2014). The cooking effect on two edible mushrooms in Anatolia: fatty acid composition, total bioactive compounds, antioxidant and anticholinesterase activities. Rec. Nat. Prod. 8, 189–194.
Ozturk, M., Tel-Cayan, G., Muhammad, A., Terzioğlu, P., and Duru, M. E. (2015). Mushrooms: a source of exciting bioactive compounds. Stud. Nat. Prod. Chem. 45, 363–456.
Palacios, I., Lozano, M., Moro, C., Arrigo, M. D., Rostagno, M. A., Martinez, J. A., et al. (2011). Antioxidant properties of phenolic compounds occurring in edible mushrooms. Food Chem. 128, 674–678.
Paliya, B. S., Verma, S., and Chaudhary, H. S. (2014). Major bioactive metabolites of the medicinal mushroom: Ganoderma lucidum. Int. J. Pharm. Res. 6, 12–24.
Pandya, U., Dhuldhaj, U., and Sahay, N. S. (2019). Bioactive mushroom polysaccharides as antitumor: an overview. Nat. Prod. Res. 33, 2668–2680. doi: 10.1080/14786419.2018.1466129
Pant, C., Karn, S. K., and Singh, R. K. (2020). Herbs as immunomodulators and increase the immunity against viral infection. Int. J. Sci. Innov. Res. 8, 1–5.
Pant, C., Karn, S. K., and Singh, R. K. (2021). Nano-medicine or Nano-drug in Viral Infections. Int. J. Sci. Innov. Res. 9, 5–10.
Patel, S., and Goyal, A. (2012). Recent developments in mushrooms as anticancer therapeutics: a review. 3 Biotech 2, 1–15. doi: 10.1007/s13205-011-0036-2
Paul, K. M., Cannon, P. F., Minter, D. W., and Stalpers, J. A. (2008). Dictionary of the Fungi. Wallingford: CABI.
Peksen, A., Kibar, B., and Yakupoglu, G. (2013). Favourable culture conditions for mycelial growth of Hydnum repandum, a medicinal mushroom. Afr. J. Tradit. Complement. Altern. Med. 10, 431–434. doi: 10.4314/ajtcam.v10i6.4
Phat, C., Moon, B., and Lee, C. (2016). Evaluation of umami taste in mushroom extracts by chemical analysis, sensory evaluation, and an electronic tongue system. Food Chem. 192, 1068–1077. doi: 10.1016/j.foodchem.2015.07.113
Philips, N., and Roger, F. (2006). Mushrooms, 6th Edn. London, United Kingdom: Macmillan Publishers, 266.
Pilz, D., Norvell, L., Danell, E., and Molina, R. (2003). Ecology and management of commercially harvested Chanterelle mushrooms. Gen. Tech. Rep. PNW-GTR-576. Portland, OR: Department of Agriculture, Forest Service, Pacific Northwest Research Station.
Pišlar, A. Sabotič, J., Šlenc, J., Brzin, J., and Kos, J. (2016). Cytotoxic L-amino-acid oxidases from Amanita phalloides and Clitocybegeotropa induce caspase-dependent apoptosis. Cell Death Discov. 2:16021. doi: 10.1038/cddiscovery.2016.21
Postemsky, P. D., and Curvetto, N. R. (2015). Submerged culture of Grifola gargal and G. sordulenta (Higher Basidiomycetes) from Argentina as a source of Mycelia with antioxidant activity. Int. J. Med. Mush. 17, 65–76. doi: 10.1615/intjmedmushrooms.v17.i1.70
Prajapati, D., Bhatt, A., Gupte, S., and Gupte, A. (2021). Mushroom secondary metabolites: chemistry and therapeutic applications. IJPSR 12, 5677–5689.
Puri, M., Kaur, I., Perugini, M. A., and Gupta, R. C. (2012). Ribosome-inactivating proteins: current status and biomedical applications. Drug Discov. Today 17, 774–783. doi: 10.1016/j.drudis.2012.03.007
Rahi, D. K., and Malik, D. (2016). Diversity of mushrooms and their metabolites of nutraceutical and therapeutic significance. J. Mycol. 2016:7654123. doi: 10.1155/2016/7654123
Rai, M., Tidke, G., and Wasser, S. P. (2005). Therapeutic potential of mushrooms. Nat. Prod. Rad. 4, 246–257.
Rakhee, N. K. S., Singh, V. K., Sharma, S., Sharma, R. K., Deswal, R., Bhargava, K., et al. (2016). Phytochemical and proteomic analysis of a high-altitude medicinal mushroom Cordyceps sinensis. J. Proteins Proteom. 3, 187–197.
Raman, V. K., Saini, M., Sharma, A., and Parashar, B. (2018). Morchella esculenta: a herbal boon to pharmacology. Int. J. Dev. Res. 8, 19660–19665.
Rathore, H., Prasad, S., and Sharma, S. (2017). Mushroom nutraceuticals for improved nutrition and better human health: a review. PharmaNutrition 5, 35–46. doi: 10.1016/j.phanu.2017.02.001
Rea, C. (1922). British Basidiomycetaceae. A handbook of the larger British fungi. Cambridge: Cambridge University Press, 799.
Reddy, S. M. (2016). “Diversity and applications of mushrooms,” in Plant biology and biotechnology, eds B. Bahadur, M. Venkat Rajam, I. Sahijram, and K. V. Krishnamurthy (Heidelberg: Springer), 231–261. doi: 10.1615/intjmedmushrooms.v17.i11.10
Reis, F. S., Barros, L., Martins, A., and Ferreira, I. C. F. R. (2012). Chemical composition and nutritional value of the most widely appreciated cultivated mushrooms: an inter-species comparative study. Food Chem. Toxicol. 50, 191–197. doi: 10.1016/j.fct.2011.10.056
Resurreccion, N. G. U., Shen, C. C., and Ragasa, C. Y. (2016). Chemical constituents of Lentinus edodes. Der Pharm. Lett. 8, 117–120.
Ribeiro, B., Valentao, P., Baptista, P., Seabra, R. M., and Andrade, P. B. (2007). Phenolic compounds, organic acids profiles and antioxidative properties of beef steak fungus (Fistulina hepatica). Food Chem. Toxicol. 45, 1805–1813. doi: 10.1016/j.fct.2007.03.015
Roncero-Ramos, I., and Delgado-Andrade, C. (2017). The beneficial role of edible mushrooms in human health. Curr. Opin. Food Sci. 14, 122–128. doi: 10.1016/j.cofs.2017.04.002
Roupas, P., Keogh, J., Noakes, M., and Taylor, P. (2012). The role of edible mushrooms in health: evaluation of the evidence. J. Funct. Foods 4, 687–709. doi: 10.1016/j.jff.2012.05.003
Royse, D. J. (2014). “A global perspective on the high five: Agaricus, Pleurotus, Lentinula, Auricularia & Flammulina,” in Proceedings of the 8th International Conference on Mushroom Biology and Mushroom Products (ICMBMP8), 19-22 (2014), ed. M. Singh (New Delhi: World Society of Mushroom Biology and Mushroom Products ICAR-Directorate of Mushroom Research Mushroom Society of India), 1–6.
Ruthes, A. C., Smiderle, F. R., and Iacomini, M. (2016). Mushroom heteropolysaccharides: a review on their sources, structure and biological effects. Carbohydr. Polym. 136, 358–375. doi: 10.1016/j.carbpol.2015.08.061
Saiki, P., Kawano, Y., Van Griensven, L. J., and Miyazaki, K. (2017). The anti-inflammatory effect of Agaricus brasiliensis is partly due to its linoleic acid content. Food Funct. 8, 4150–4158. doi: 10.1039/c7fo01172e
Saiqa, S., Haq, N. B., Muhammad, A. H., Muhammad, A. H., and Ali, M. A. (2008). Studies on chemical composition and nutritive evaluation of wild edible mushrooms. Iran. J. Chem. Chem. Eng. 27, 151–154.
Samsudin, N. I. P., and Abdullah, N. (2019). Edible mushrooms from Malaysia: a literature review on their nutritional and medicinal properties. Int. Food Res. J. 26, 11–31.
Sanchez, C. (2017). “Bio-actives from mushroom and their application,” in Food bioactives, ed. M. Puri (New York City: Springer International Publishing AG), 23–57. doi: 10.2174/1389557522666220113122117
Sanodiya, B. S., Thakur, G. S., Baghel, R. K., Prasad, G. B., and Bisen, P. S. (2009). Ganoderma lucidum: a potent pharmacological macrofungus. Curr. Pharm. Biotechnol. 10, 717–742. doi: 10.2174/138920109789978757
Sarangi, M., Padhi, S., Dheeman, S., Karn, S. K., and Patel, L. D. (2021). Diagnosis, prevention and treatment of COVID-19: a review. Expert Rev. Anti-Infect. Ther. 20, 243–266. doi: 10.1080/14787210.2021.1944103
Satria, D., Tamrakar, S., Suhara, H., Kaneko, S., and Shimizu, K. (2019). Mass Spectrometry-based untargeted metabolomics and α-glucosidase inhibitory activity of Lingzhi (Ganoderma lingzhi) during the developmental stages. Molecules 24:2044. doi: 10.3390/molecules24112044
Sayeed, M. A., Banu, A., Khatus, K., Khanam, P., Begum, T., Mahtab, H., et al. (2015). Effect of edible mushroom (Pleurotus ostreatus) on type-2 diabetics. Ibrahim Med. Coll. J. 8, 6–11. doi: 10.3329/imcj.v8i1.22982
Schmid, F., Stone, B. A., McDougall, B. M., Bacic, A., Martin, K. L., Brownlee, R. T., et al. (2001). Structure of epiglucan, a highly side-chain/branched (1/3;1/6)-β-glucan from the micro fungus Epicoccum nigrum Ehrenb. Ex Schlecht. Carbohydr. Res. 331, 163–171. doi: 10.1016/s0008-6215(01)00023-4
Seo, D. J., and Choi, C. (2021). Antiviral bioactive compounds of mushrooms and their antiviral mechanisms: a Review. Viruses 13:350. doi: 10.3390/v13020350
Sevindik, M., Bal, C., Baba, H., Akgul, H., and Selamoglu, Z. (2019). “Biological activity potentials of Amanita species,” in Proceeding at International Conference Eurosia Mycology Congress, 4th-6th September (Konya: Selçuk University).
Shahzad, F., Anderson, D., and Najafzadeh, M. (2020). The antiviral, anti-inflammatory effects of natural medicinal herbs and mushrooms and SARs-CoV-2 infection. Nutrients 12, 2573–2586. doi: 10.3390/nu12092573
Shao, H. J., Jeong, J. B., Kim, K. J., and Lee, S. H. (2015). Anti-inflammatory activity of mushroom-derived hispidin through blocking of NF-κB activation. J. Sci. Food Agric. 95, 2482–2486. doi: 10.1002/jsfa.6978
Shao, S., Hernandez, M., Kramer, J. K. G., Rinker, D. L., and Tsao, R. (2010). Ergosterol Profiles, Fatty Acid Composition, and Antioxidant Activities of Button Mushrooms as Affected by Tissue Part and Developmental Stage. J. Agric. Food Chem. 58, 11616–11625. doi: 10.1021/jf102285b
Sharma, S. K., Lall, A. M., Sharma, M., and Reishi, M. (2013). Response of organic supplementation on yield and nutritional parameters of Calocybe indica. Int. J. Plant Res. 26, 36–39. doi: 10.5958/j.2229-4473.26.1.005
Sheena, N., Lakshmi, B., and Janardhanan, K. K. (2005). Therapeutic potential of Ganoderma lucidum (Fr.) P. Karst. Nat. Prod. Rad. 4, 382–386.
Shnyreva, A., Kozhevnikova, E. Y., Barkov, A. V., and Shnyreva, A. V. (2017). Solid-State Cultivation of Edible Oyster Mushrooms, Pleurotus spp. under Laboratory Conditions. Adv. Microbiol. 07, 125–136. doi: 10.4236/aim.2017.72010
Shu-Ting, C., and Philip, G. M. (2004). Tremella- increased production by a mixed culture technique. Mushrooms: cultivation, nutritional value, medicinal effect and environmental impact, 2nd Edn. Boca Raton: CRC Press.
Silva, M. D. C. S. D., Nunes, M. D., Luz, J. M. R. D., and Kasuya, M. C. M. (2013). Mycelial Growth of Pleurotus Spp. in Se-Enriched Culture Media. Adv. Microbiol. 3, 11–18. doi: 10.4236/aim.2013.38a003
Singh, S. S., Wang, H., Chan, Y. S., Pan, W., Dan, X., Yin, C. M., et al. (2014). Lectins from edible mushrooms. Molecules 20, 446–469. doi: 10.3390/molecules20010446
Singh, V., Kumar, P., Kumar, S., and Kumar, K. (2017). Yield performance of collected wild milky mushroom (Calocybe sp.). Plant Arch. 17, 181–186.
Song, F. Q., Liu, Y., Kong, X. S., Chang, W., and Song, G. (2013). Progress on understanding the anticancer mechanisms of medicinal mushroom: inonotus obliquus. Asian Pac. J. Cancer Prev. 14, 1571–1578. doi: 10.7314/apjcp.2013.14.3.1571
Sonnenberg, A. S. M., Baars, J. J. P., Hendrickx, P. M., Lavrijssen, B., Gao, W., Weijn, A., et al. (2011). “Breeding and strains protection in the button mushroom Agaricus bisporus,” in Proceedings of the 7th International Conference on Mushroom Biology and Mushroom Products (ICMBMP7), eds J. M. Savoie, M. Foulongme-Oriol, M. Largeteau, and G. Barroso (Arcachon, France: World Society of Mushroom Biology and Mushroom Products), 4–7.
Spraker, J. (2013). UW-Madison Plant Pathology, Huitlacoche. Wisconsin: University of Wisconsin-Madison.
Stamets, P. (2000a). Growing Gourmet and Medicinal Mushrooms. Berkeley and Toronto: Ten speed Press, 339.
Stamets, P. (2000b). “Growth parameters for gourmet and medicinal mushroom species,” in Growing Gourmet and Medicinal Mushrooms, 3rd Edn, ed. P. Stamets (Berkeley, California. USA: Ten Speed Press), 308–315.
Stamets, P. E. (2018). Antiviral Activity from Medicinal Mushrooms and Their Active Constituents. Patent No.: US 9, 931, 316 B2. Shelton, WA: United States Patent Stamets.
Stierum, R., Heijne, W., Kienhuis, A., van Ommen, B., and Groten, J. (2005). Toxicogenomics concepts and applications to study hepatic effects of food additives and chemicals. Toxicol. Appl. Pharmacol. 207, 179–188. doi: 10.1016/j.taap.2005.01.050
Stilinović, N., Čapo, I., Vukmirović, S., Rašković, A., Tomas, A., Popović, M., et al. Chemical composition, nutritional profile and invivo antioxidant properties of the cultivated mushroom Coprinus comatus. R. Soc. Open Sci. 7:200900. doi: 10.1098/rsos.200900
Su, C. H., Lu, M. K., Lu, T. J., Lai, M. N., and Ng, L. T. (2020). A (1→6)-Branched (1→4)-β-D-Glucan from Grifola frondosa Inhibits Lipopolysaccharide-Induced Cytokine Production in RAW264. 7 Macrophages by Binding to TLR2 Rather than Dectin-1 or CR3 Receptors. J. Nat. Prod. 83, 231–242. doi: 10.1021/acs.jnatprod.9b00584
Surayot, U., Wangtueai, S., You, S. G., Palanisamy, S., Krusong, W., Brennan, C. S., et al. (2021). Extraction, Structural Characterisation, and Immunomodulatory Properties of Edible Amanita hemibapha subspecies javanica (Corner and Bas) Mucilage Polysaccharide as a Potential of Functional Food. J. Fungi. 7:683. doi: 10.3390/jof7090683
Takahashi, A., Endo, T., and Nozoe, S. (1992). Repandiol, A new cytotoxic diepoxide from the mushrooms Hydnum repandum and H. repandum varalbum. Chem. Pharam. Bull. 40, 3181–3184. doi: 10.1248/cpb.40.3181
Takeshi, I., Hiroshi, H., Satoshi, I., and Aya, K. (2012). Mushroom Extracts from Agaricus, Hericiumerinaceum, and Hypsizigusmarmoreus as Insulin Secretion Stimulators and Health Foods for Prevention and Therapy of Diabetes Mellitus. JP 2012077004A. Cham: SpringerNature.
Tel-Cayan, T., Ozturk, G., Duru, M. M. E., and Turkoğlu, A. (2017). Fatty acid profiles in wild mushroom species from Anatolia. Chem. Nat. Compd. 53, 351–353. doi: 10.1007/s10600-017-1986-3
Thangthaeng, N., Miller, M. G., Gomes, S. M., and Shukitt-Hale, B. (2015). Daily supplementation with mushroom (Agaricus bisporus) improves balance and working memory in aged rats. Nutr. Res. 35, 1079–1084. doi: 10.1016/j.nutres.2015.09.012
Toh Choon, R. L., Sariah, M., and Siti Mariam, M. N. (2012). Ergosterol from the soilborne fungus Ganoderma boninense. J. Basic Microbiol. 52, 608–612. doi: 10.1002/jobm.201100308
Tsukamoto, S., Macabalang, A. G., Nakatani, K., Obara, Y., Nakahata, N., and Ohta, T. (2003). Tricholomalides A-C, new neurotrophic diterpenes from the mushroom Tricholoma sp. J. Nat. Prod. 66, 1578–1581. doi: 10.1021/np030140x
Tubic, J., Grujicic, D., Jakovljevic, M. R., Rankovic, B., Kosanic, M., Stanojkovic, T., et al. (2009). Investigation of biological activities and secondary metabolites of Hydnum repandum acetone extract. Farmacia 67, 174–183.
Tulloss, R. E. (2015). “[mutable text]. Amanita hesleri,” in Studies in the Amanitaceae, eds R. E. Tulloss and Z. L. Yang. Available online at: http://www.amanitaceae.org?Amanita+hesleri (accessed March 13, 2015).
Ukpebor, J. E., Akpaja, E. O., Ukpebon, E. E., Eghareuba, O., and Efedue, E. (2007). Effect of the edible mushroom, Pleurotus tuber-regium on the cyanide level and nutritional contents of rubber seed cake. Pak. J. Nutr. 6, 534–537. doi: 10.3923/pjn.2007.534.537
Usman, M., Murtaza, G., and Ditta, A. (2021). Nutritional, medicinal and cosmetic value of bioactive compounds in button mushroom (Agaricus bisporus): a review. Appl. Sci. 11:5943. doi: 10.3390/app11135943
Üstün, N. S., and Bulam, S.Pekşen, A. (2018). “The use of mushrooms and their extracts and compounds in functional foods and nutraceuticals,” in Proceedings of the International Technological Sciences and Design Symposium, 27-29 June 2018, Giresun.
Valdez-Morales, M., Barry, K., Fahey, G. C. Jr., Domínguez, J., de Mejia, E. G., Valverde, M. E., et al. (2010). Effect of maize genotype, developmental stage, and cooking process on the nutraceutical potential of huitlacoche (Ustilagomyadis). Food Chem. 119, 689–697. doi: 10.1016/j.foodchem.2009.07.015
Valko, M., Leibfritz, D., Moncol, J., Cronin, M. T., Mazur, M., and Telser, J. (2007). Free radicals and antioxidants in normal physiological functions and human diseases. Int. J. Biochem. Cell Biol. 39, 44–84. doi: 10.1016/j.biocel.2006.07.001
Valverde, M. E., Hernandez-Perez, T., and Paredes-Lopez, O. (2012). “Huitlacoche- a 21st century culinary delight originated in the Aztec times,” in Hispanic Foods: Chemistry and Bioactive compounds, eds M. H. Tunick and E. Gonzalez de Mejia (Washington, DC, USA: American Chemical Society), 1109.
Valverde, M. E., Hernandez-Perez, T., and Paredes-Lopez, O. (2015). Edible mushrooms: improving human health and promoting quality life. Int. J. Microbiol. 2015:376387. doi: 10.1155/2015/376387
Veena, S. S., and Pandey, M. (2012). Physiological and cultivation requirements of Trametes versicolor, a medicinal mushroom to diversify Indian mushroom industry. Indian J. Agric. Sci. 82, 672–675.
Venturella, G., Ferraro, V., Cirlincione, F., and Gargano, M. L. (2021). Medicinal Mushrooms: bioactive compounds, use and clinical trials. Int. J. Mol. Sci. 22:634. doi: 10.3390/ijms22020634
Villares, A., Mateo-Vivaracho, L., and Guillamon, E. (2012). Structural features and healthy properties of polysaccharides occurring in mushrooms. Agriculture 2, 452–471. doi: 10.3390/agriculture2040452
Villeneuve, D. L., and Garcia-Reyero, N. (2011). Vision and strategy: predictive ecotoxicology in the 21st century. Environ. Toxicol. Chem. 30, 1–8. doi: 10.1002/etc.396
Vishwakarma, M. P., Bhatt, R. P., and Gairola, S. (2011). Some medicinal mushrooms of Garhwal Himalaya, Uttarakhand, India. Int. J. Med. Aromat. Plants 1, 33–40. doi: 10.1016/j.sjbs.2021.03.030
Wang, H., and Ng, T. B. (2006). Ganodermin, an antifungal protein from fruiting bodies of the medicinal mushroom Ganoderma lucidum. Peptides 27, 27–30. doi: 10.1016/j.peptides.2005.06.009
Wani, B. A., Bodha, R. H., and Wani, A. H. (2010). Nutritional and medicinal importance of mushrooms. J. Med. Plants Res. 4, 2598–2604.
Wasser, K. S. (2002). Medicinal mushrooms as a source of antitumor and immunomodulating polysaccharides. Appl. Microbiol. Biotechnol. 60, 258–274. doi: 10.1007/s00253-002-1076-7
Wasser, S. P. (2010). Medicinal mushroom science: history, current status, future trends, and unsolved problems. Int. J. Med. Mush. 12, 1–16. doi: 10.1615/intjmedmushr.v12.i1.10
Wasser, S. P. (2011). Current findings, future trends and unsolved problems in studies of medicinal mushrooms. Appl. Microbiol. Biotechnol. 89, 1323–1332. doi: 10.1007/s00253-010-3067-4
Wasser, S. P., and Reshetnikov, S. V. (2002). U.S. Patent No. 6,383,799. Washington, DC: U.S. Patent and Trademark Office.
Wasser, S. P., and Weis, A. L. (1999). Medicinal properties of substances occurring in higher basidiomycetes mushrooms: current perspective (review). Int. J. Med. Mush. 1, 31–62. doi: 10.1615/intjmedmushrooms.v1.i1.30
Wei, S., and Van Griensven, L. J. L. D. (2008). Pro- and antioxidative properties of medicinal mushroom extracts. Int. J. Med. Mush. 10, 315–324. doi: 10.1615/intjmedmushr.v10.i4.30
Wells, J. C. (2008). Longman Pronunciation Dictionary, 3rd Edn. Harlow Essex, United Kingdom: Longman.
Wong, J. H., Sze, S. C., Ng, T. B., Cheung, R. C., Tam, C., Zhang, K. Y., et al. (2018). Apoptosis and anti-cancer drug discovery: the power of medicinal fungi and plants. Curr. Med. Chem. 25, 5613–5630. doi: 10.2174/0929867324666170720165005
Wong, K. H., Wong, K. Y., Kwan, H. S., and Cheung, P. (2005). Dietary fibers from mushroom sclerotia: 3. In vitro fermentability using human fecal microflora. J. Agric. Food Chem. 53, 9407–9412. doi: 10.1021/jf051080z
Xu, E. Y., Schaefer, W. H., and Xu, Q. (2009). Metabolomics in pharmaceutical research and development: metabolites, mechanisms and pathways. Curr. Opin. Drug Discov. Dev. 12, 40–52.
Xu, X., Yan, H., Chen, J., and Zhang, X. (2011). Bioactive proteins from mushrooms. Biotechnol. Adv. 29, 667–674. doi: 10.1016/j.biotechadv.2011.05.003
Yadava, N., Yadava, R., and Goyal, A. (2014). Chemistry of Terpenoids. Int. J. Pharm. Sci. Rev. Res. 27, 272–278. doi: 10.1080/14786419.2019.1669029
Yahaya, N. F. M., Rahman, M. A., and Abdullah, N. (2014). Therapeutic potential of mushrooms in preventing and ameliorating hypertension. Trends. Food Sci. Tech. 39, 104–115. doi: 10.1016/j.tifs.2014.06.002
Yamin, S., Shuhaimi, M., Arbakariya, A., Fatimah, A. B., Khalilah, A. K., Anas, O., et al. (2012). Effect of Ganoderma lucidum polysaccharides on the growth of Bifidobacterium spp. as assessed using real-time PCR. Int. Food J. 19, 1199–1205.
Yang, M., Belwal, T., Devkota, H. P., Li, L., and Luo, Z. (2019). Trends of utilizing mushroom poly-saccharides (MPs) as potent nutraceutical components in food and medicine: a comprehensive review. Trends Food Sci. Technol. 92, 94–110.
Yeh, J. Y., Hsieh, L. H., Wu, K. T., and Tsai, C. F. (2011). Antioxidant properties and antioxidant compounds of various extracts from the edible basidiomycetes Grifola frondose (Maitake). Molecules 16, 3197–3211. doi: 10.3390/molecules16043197
Yu, S., Weaver, V., Martin, K., and Cantorna, M. T. (2009). The effects of whole mushrooms during inflammation. BMC Immunol. 10:12. doi: 10.1186/1471-2172-10-12
Yuan, Y., Wang, Y. J., Sun, G. P., Wang, Y. R., Cao, L. J., Shen, Y. M., et al. (2018). Archaeological evidence suggests earlier use of Ganoderma in Neolithic China. Chin. Sci. Bull. 63, 1180–1188. doi: 10.1360/n972018-00188
Zaidman, B. Z., Yassin, M., Mahajna, J., and Wasser, S. P. (2005). Medicinal mushroom modulators of molecular targets as cancer therapeutics. Appl. Microbiol. Biotechnol. 67, 453–468. doi: 10.1007/s00253-004-1787-z
Zhang, J. J., Li, Y., Zhou, T., Xu, D. P., Zhang, P., Li, S., et al. (2016). Bioactivities and Health Benefits of Mushrooms Mainly from China. Molecules 21:938. doi: 10.3390/molecules21070938
Zhang, L., Fan, C., Liu, S., Zang, Z., and Jiao, L. (2011). Chemical composition and antitumor activity of polysaccharide from Inonotus obliquus. J. Med. Plants Res. 5, 1251–1260.
Zhang, L., Li, C. G., Liang, H. L., and Reddy, N. (2017). Bioactive mushroom polysaccharides: immunoceuticals to anticancer agents. J. Nutraceuticals Food Sci. 2, 6–10.
Zhang, M., Cui, S. W., Cheung, P. C. K., and Wang, Q. (2007). Antitumor polysaccharides from mushrooms: a review on their isolation process, structural characteristics and antitumor activity. Trends Food Sci. Technol. 18, 4–19. doi: 10.1016/j.tifs.2006.07.013
Zhang, M., Huang, J., Xie, X., and Holman, C. D. (2009). Dietary intakes of mushrooms and green tea combine to reduce the risk of breast cancer in Chinese women. Int. J. Cancer 124, 1404–1408. doi: 10.1002/ijc.24047
Zhang, P., Chen, Z. H., Chen, J. S., Hu, J. S., Wei, B. Y., Zhang, Z. G., et al. (2005). Production and characterization of Amanitin toxins from a pure culture of Amanita exitialis. FEMS Microbiol. Lett. 252, 223–228. doi: 10.1016/j.femsle.2005.08.049
Zhao, C., Gao, L., Wang, C., Liu, B., Jin, Y., and Xing, Z. (2016). Structural characterization and antiviral activity of a novel heteropolysaccharide isolated from Grifola frondosa against enterovirus 71. Carbohydr. Polym. 144, 382–389. doi: 10.1016/j.carbpol.2015.12.005
Zhao, S., Gao, Q., Rong, C., Wang, S., Zhao, Z., Liu, Y., et al. (2020). Immunomodulatory Effects of Edible and Medicinal Mushrooms and Their Bioactive Immunoregulatory Products. J. Fungi 6:269. doi: 10.3390/jof6040269
Zhong, J. J., and Xiao, J. H. (2009). Secondary metabolites from higher fungi: discovery, bioactivity and bioproduction. Adv. Biochem. Eng. Biotechnol. 113, 79–150. doi: 10.1007/10_2008_26
Zhong, J., Liu, R., and Li, Y. (2011). Ganoderic Acid T Amide Derivative TLTO-A and Synthetic Method and Application Thereof Database: CAPLUS. CN 102219822 A 20111019. Cham: SpringerNature.
Zhou, X., Gong, Z., Su, Y., Lin, J., and Tang, K. (2009). Cordyceps fungi: natural products, pharmacological functions and developmental products. J. Pharm. Pharmacol. 61, 279–291. doi: 10.1211/jpp/61.03.0002
Zhu, K., Nie, X., Tan, S. P., Li, C., Gong, D. M., and Xie, M. Y. (2016). A polysaccharide from Gnodermaatrum improves liver function in type 2 diabetic rats via antioxidant action and short-chain fatty acids excretion. J. Agric. Food Chem. 64, 1938–1944. doi: 10.1021/acs.jafc.5b06103
Zhuang, C., Kawagishi, H., and Preuss, H. G. (2007). U.S. Patent No. 7,214,778. Washington, DC: U.S. Patent and Trademark Office.
Zhuang, C., Kawagishi, H., Zhang, L., and Anzai, H. (2009). Anti-Dementia Substance from Hericium erinaceum and Method of Extraction Database: CAPLUS. US 20090274720 A1 20091105. Paramus, NJ.
Ziaja-Sołtys, M., Radzki, W., Nowak, J., Topolska, J., Jabłoñska-Ryś, E., Sławiñska, A., et al. (2020). Processed Fruiting Bodies of Lentinus edodes as a Source of Biologically Active Polysaccharides. Appl. Sci. 10, 470–481. doi: 10.3390/app10020470
Keywords: mushroom, metabolites, medicine, ITS sequence, β-glucan, terpenoids, unknown metabolites, polyketides etc
Citation: Bhambri A, Srivastava M, Mahale VG, Mahale S and Karn SK (2022) Mushrooms as Potential Sources of Active Metabolites and Medicines. Front. Microbiol. 13:837266. doi: 10.3389/fmicb.2022.837266
Received: 16 December 2021; Accepted: 15 February 2022;
Published: 26 April 2022.
Edited by:
Mukesh Kumar Awasthi, Northwest A&F University, ChinaReviewed by:
Surendra Sarsaiya, Zunyi Medical University, ChinaVivek K. Chaturvedi, Banaras Hindu University, India
Copyright © 2022 Bhambri, Srivastava, Mahale, Mahale and Karn. This is an open-access article distributed under the terms of the Creative Commons Attribution License (CC BY). The use, distribution or reproduction in other forums is permitted, provided the original author(s) and the copyright owner(s) are credited and that the original publication in this journal is cited, in accordance with accepted academic practice. No use, distribution or reproduction is permitted which does not comply with these terms.
*Correspondence: Santosh Kumar Karn, c2FudG9zaGthcm5AZ21haWwuY29t, c2FudG9zaGthcm4uc2JzdUBnbWFpbC5jb20=, orcid.org/0000-0002-9803-4182