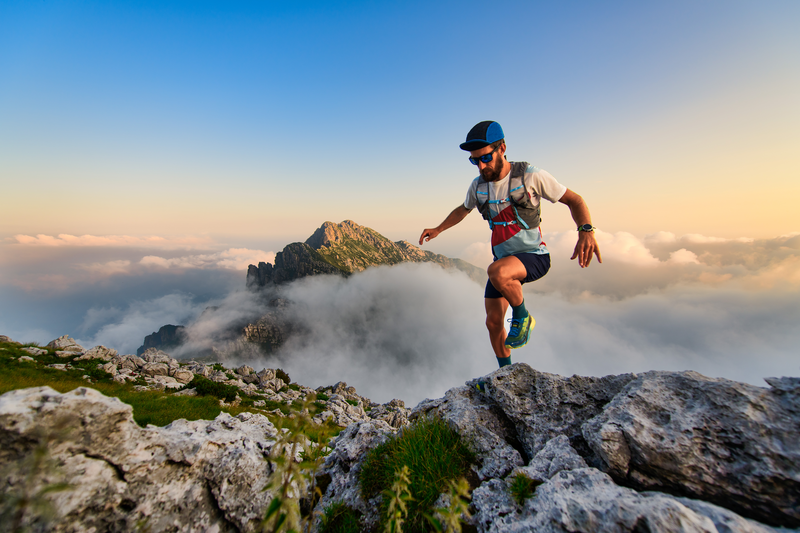
94% of researchers rate our articles as excellent or good
Learn more about the work of our research integrity team to safeguard the quality of each article we publish.
Find out more
REVIEW article
Front. Microbiol. , 09 March 2022
Sec. Antimicrobials, Resistance and Chemotherapy
Volume 13 - 2022 | https://doi.org/10.3389/fmicb.2022.835390
Microsporidia are obligate intracellular, spore-forming parasitic fungi which are grouped with the Cryptomycota. They are both opportunistic pathogens in humans and emerging veterinary pathogens. In humans, they cause chronic diarrhea in immune-compromised patients and infection is associated with increased mortality. Besides their role in pébrine in sericulture, which was described in 1865, the prevalence and severity of microsporidiosis in beekeeping and aquaculture has increased markedly in recent decades. Therapy for these pathogens in medicine, veterinary, and agriculture has become a recent focus of attention. Currently, there are only a few commercially available antimicrosporidial drugs. New therapeutic agents are needed for these infections and this is an active area of investigation. In this article we provide a comprehensive summary of the current as well as several promising new agents for the treatment of microsporidiosis including: albendazole, fumagillin, nikkomycin, orlistat, synthetic polyamines, and quinolones. Therapeutic targets which could be utilized for the design of new drugs are also discussed including: tubulin, type 2 methionine aminopeptidase, polyamines, chitin synthases, topoisomerase IV, triosephosphate isomerase, and lipase. We also summarize reports on the utility of complementary and alternative medicine strategies including herbal extracts, propolis, and probiotics. This review should help facilitate drug development for combating microsporidiosis.
Microsporidia are a diverse group of obligate intracellular pathogens phylogenetically related to the Cryptomycota, they form a basal branch on the phylogenic tree of fungi (James et al., 2013). The host range of microsporidia extends from protists to vertebrates (James et al., 2013). These organisms have several adaptations to intracellular life including having mitochondrial remnants termed mitosomes (Williams et al., 2002), small ribosomes with fewer component proteins and rRNA with reduced domains (Peyretaillade et al., 1998), an unstacked type of Golgi apparatus, and a lack of peroxisomes (Beznoussenko et al., 2007; Vavra and Lukes, 2013). All microsporidia have a dormant extracellular spore stage with a limiting spore wall and the spore contains a specialized invasion organelle (the polar tube) and the infective sporoplasm. Upon appropriate environmental stimulation, the polar tube extrudes (germinates) allowing the microsporidia to inject the sporoplasm into host cells and start to proliferate intracellularly (Weidner, 1976).
Horizontal transmission of these infectious spores primarily relies on the fecal-oral route. Another route of transmission is through contact of spores with eye, mucosa, or broken skin. Several microsporidia can also be vertically transmitted to the offspring of infected animals. Depending on the species of microsporidia some can switch hosts across disparate taxa, while other species are relatively host specific (Solter and Maddox, 1998; Andreadis et al., 2012). Symptoms due to infection can vary from asymptomatic carriage to death. Many microsporidia alter host cell biology and infection can result in the development of xenomas, juvenilization of the host, and metabolic changes in infected cells. Microsporidia have been classified by the National Institutes of Allergy and Infectious Diseases (NIAID) and the Centers for Disease Control and Prevention (CDC) as Category B biodefense priority pathogens.1
Microsporidiosis is usually self-limiting or asymptomatic in the general human population, but more severe in immune deficient patients. The importance of microsporidia in humans came into focus with the emergence of the acquired immune deficiency syndrome (AIDS). In humans severe infection has been seen in patients with AIDS, patients following organ transplantation, and in patients taking immune suppressive drugs or immune modulatory antibodies (Han and Weiss, 2017; Dumond et al., 2021; Dumortier et al., 2021). Most commonly infection results in a diarrheal syndrome; however, patients may also suffer from encephalitis, ocular infection, sinusitis, myositis, or disseminated infection (Weber et al., 1994; Weiss and Keohane, 2010; Ziad et al., 2021). Among the approximately 1,500 microsporidian species, 17 species can infect humans. Enterocytozoon bieneusi is the most frequently reported species causing gastrointestinal disease in immune-deficient individuals, followed by Encephalitozoon intestinalis (Garcia-Torres et al., 2018; Li et al., 2019). Less common human-infecting microsporidian species include Anncaliia, Vittaforma, Trachipleistophora, and Pleistophora (Shadduck et al., 1990; Field et al., 1996; Cali and Takvorian, 2003; Cali et al., 2010; Boileau et al., 2016; Anderson et al., 2019). Livestock animals are the most frequently identified reservoir hosts, transmission can occur from livestock excreta and wastewater that contain spores or via spore-contaminated livestock products (Li et al., 2020; Ruan et al., 2021). Some zoonotic microsporidia transmission cases have been caused by infections in companion animals, such as cats and dogs, which may become potential public health risks (Cama et al., 2007; Karim et al., 2014; Wan et al., 2016; Ruan et al., 2021). Wild animals are also potential pathogen reservoirs, like monkeys, foxes, raccoons, beavers, and dolphins (Sulaiman et al., 2003; Desoubeaux et al., 2018; Zhao et al., 2021). Several outbreaks of microsporidiosis in healthy individuals have also been reported (Kwok et al., 2013; Lam et al., 2013; Wang et al., 2018). It is likely that microsporidiosis is a common infection but is self-limited or asymptomatic in healthy hosts. To this end, microsporidia have been identified in up to 20% of children with diarrhea in underdeveloped countries (Gumbo et al., 1999).
Microsporidiosis caused by Nosema bombycis in silkworm, i.e., pébrine, is one of the most deadly diseases seen in the sericulture industry. Transmission of this infection can occur both horizontally and vertically, causing heavy losses or even total crop failure. Historically pébrine caused a collapse of the French and Italian silk industry in the mid-19th century, until Pasture was able to develop preventative methods and save the silkworm industry (Bhat et al., 2009; Hukuhara, 2011). This disease continues to be an important concern for silkworm growers and scientists in sericulture practicing countries around the world and remains a major threat to sericulture.
Honey bees are key pollinators of both wild plant communities and agricultural crops, they are important to the environment as well as the food supply (Calderone, 2012). Nosema ceranae and Nosema apis are major causes of microsporidiosis in honey bees (Higes et al., 2008; Fries, 2010). N. ceranae is now the predominant microsporidium species seen in the western honey bee (Apis mellifera), which is the most important bee species for honey production and animal-mediated pollination (Williams et al., 2014). While the antimicrosporidian drug fumagillin continues to be effective against N. apis, it has not been as effective for infections due to N. ceranae (Williams et al., 2008). N. apis and N. ceranae have recently been redefined as Vairimorpha apis and Vairimorpha ceranae based on a molecular phylogenetics analysis of the Nosema and Vairimorpha clades (Tokarev et al., 2020). For the purposes of this review the Nosema Genus will still be used.
Shrimp aquaculture is an important long-term sustainable industry for many developing countries (Flegel, 1997). Microsporidiosis is the most common and harmful eukaryotic pathogen to shrimp, and directly threatens shrimp aquaculture (Morado, 2011; Tang et al., 2015). Enterocytozoon hepatopenaei (EHP) and Agmasoma penaei are well-known species which cause economic losses in shrimp aquaculture. A. penaei can infect muscle and connective tissues of giant tiger shrimp Penaeus monodon and pacific white shrimp Litopenaeus vannamei. Infected shrimp are called “cotton shrimp” or “milk shrimp” due to the whitish or milky appearance seen on various parts of the body (Pasharawipas and Flegel, 1994; Prasertsri et al., 2009). Infected shrimp are not able to be sold leading to economic losses. EHP infects the hepatopancreas of shrimp damaging the functions of this critical organ and causing slow or stunted growth in infected shrimp (Thitamadee et al., 2016). In addition, EHP infection increase the susceptibility of shrimp to acute hepatopancreatic necrosis disease caused by Vibrio (Aranguren et al., 2017). EHP has been widely found in Asia and other parts of the world, severely impacting aquaculture production.
Various types of medicinal regimes have been used to treat microsporidiosis, the results of therapy have ranged from disappointing to promising. Previous reviews have summarized the drugs and therapeutic targets for the treatment of microsporidiosis in humans (Conteas et al., 2000; Han and Weiss, 2018). The objective of this review is to summarize recent studies on new drugs and targets for the treatment of these infections in both vertebrates and invertebrates.
As microsporidia were once considered “primitive” protozoa, many anti-protozoan drugs have been tested as therapeutic agents. The majority of these anti-protozoan drugs have not been effective for the treatment of microsporidiosis. The widely used anti-protozoan and anti-bacterial agent trimethoprim-sulfamethoxazole has minimal activity against either Ent. bieneusi or Enc. intestinalis (Conteas et al., 2000). While metronidazole was shown in vitro to inhibit the germination of Enc. intestinalis, clinical studies demonstrated it was ineffective against microsporidiosis (Canning and Hollister, 1990; Orenstein, 1991; He et al., 1996). Studies on the effectiveness of paromomycin have also been disappointing, and sequence data has shown that microsporidia lack the rRNA binding site for this drug (Beauvais et al., 1994). The target of many antifungal drugs is the ergosterol biosynthesis pathway, however microsporidia lack this typical fungal pathway, thus amphotericin B has not been effective (Beauvais et al., 1994; Albrecht et al., 1995). Atovaquone has also been ineffective for therapy, consistent with the absence of mitochondria in these pathogens. Itraconazole has demonstrated some microsporidian growth inhibition in vitro; however it has not been effective as a solitary agent in clinical microsporidiosis. Itraconazole has been used in combination with other drugs (e.g., fumagillin or albendazole) in several clinical cases. Current recommended therapeutic options, which directly inhibit microsporidian infections are quite limited, include only two structural classes of drugs: benzimidazoles and terpenes.
Benzimidazole compounds share a bicyclic compound consisting of the fusion of benzene and imidazole (Figure 1). They exhibit various types of bioactivity, including anti-inflammatory, anti-hypertensive, anti-bacterial, anti-parasitic, and anti-fungal effects (Labanauskas et al., 2004; Navarrete-Vazquez et al., 2010; Hosamani and Shingalapur, 2011). Benzimidazoles are well-known therapeutic agents with broad-spectrum anthelmintic ability and also activity against fungi (in commercial applications where they inhibit mold growth), they act mainly through inhibition of the microtubule assembly (Lacey, 1990). Albendazole (1) has a broad spectrum of activity against helminths with minimal host side effects. It has been licensed for human use in various parts of the world (Horton, 2000). Albendazole has been shown to control microsporidiosis caused by Encephalitozoon spp. and the β-tubulin sequences of these Encephalitozoonidae confirms the presence of amino acid residues associated with sensitivity to benzimidazoles (Li et al., 1996; Didier, 1997). There are numerous case reports demonstrating the efficacy of albendazole for microsporidiosis caused by Encephalitozoon spp., including diarrhea caused by Enc. intestinalis, chronic disseminated infection caused by Enc. hellem and disseminated infection caused by Enc. cuniculi involving the central nervous system (Lecuit et al., 1994; Dore et al., 1995; Weber et al., 1997). In immunocompetent children with diarrhea caused by microsporidium, albendazole therapy seems effective in reducing microsporidial excretion in feces (Tremoulet et al., 2004). Albendazole is also effective against myositis caused by Trachipleistophora hominis, Anncaliia algerae, and Anncaliia vesicularum (Field et al., 1996; Cali et al., 1998; Watts et al., 2014; Sutrave et al., 2018). An in vitro assay demonstrated that albendazole was less effective against Vittaforma corneae than against Enc. intestinalis or Enc. cuniculi (Didier, 1997; Franzen and Salzberger, 2008). Studies of patients with diarrhea caused by Ent. bieneusi who were treated with albendazole proved that the effect of albendazole is quite limited (Dieterich et al., 1994; Leder et al., 1998). This is consistent with the presence of amino acids associated with albendazole resistance in the β-tubulins sequences of Enterocytozoon and Vittaforma (Akiyoshi et al., 2007; Franzen and Salzberger, 2008). There are also some albendazole resistance variations in different genotypes of Encephalitozoon (Kotkova et al., 2017). Some research suggests that Enc. cuniculi genotype III show elevated resistance to albendazole treatment in immune deficient and immune competent mice (Sak et al., 2020). Based on NIH’s guidelines, albendazole is recommended for treatment of intestinal and disseminated microsporidiosis caused by microsporidia other than Enc. bieneusi and V. corneae.
Benomyl (2) and carbendazim (3) are another type of benzimidazoles that are wildly used as fungicide for fungal diseases in field crops (Figure 1). They are also considered as medicinal regimes for microsporidiosis in insects. Many studies suggest that benomyl and its derivative compounds are effective in controlling microsporidiosis in insects such as Nosema heliothidis in Heliothis zea and Nosema kingi in Drosophila willistoni (Armstrong, 1976; Brooks et al., 1978). Ultrastructural evidence suggested that carbendazim causes elongation, vacuolation, and depletion of cytoplasmic contents of the of N. bombycis in meront and spore stages (Jyothi et al., 2005). In China, carbendazim formulations have been registered and commercially marketed to control N. bombycis in silkworm seed production. However, due to its hepatotoxicity and toxic effects in animal reproduction (Barnes et al., 1983; Sakr et al., 2004), benomyl and carbendazim have not been used for the treatment of mammalian microsporidiosis.
Fumagillin (4), ovalicin (5), and their analogs are terpenes share unique structures with a cyclohexane framework, two epoxides and three or four contiguous stereogenic centers situated on the cyclohexane ring (Figure 1). Originally isolated from the fungus Aspergillus fumigatus, fumagillin was initially utilized as a treatment for amebiasis (McCowen et al., 1951; Killough et al., 1952). As more effective drugs for amebiasis were developed, it was abandoned. Researchers subsequently discovered that fumagillin had both antimicrosporidial and anti-angiogenesis activities (Lefkove et al., 2007). Fumagillin selectively and covalently binds to methionine aminopeptidase type 2 (MetAP2), however, it does not inhibit methionine aminopeptidase type 1 (MetAP1) (Sin et al., 1997). As removal of an N-terminal methionine is often essential for protein function and post-translational modification, inhibition of methionine aminopeptidase can affect eukaryotic cell survival. Mammalian cells possess both MetAP1 and MetAP2 while genome data from Enc. cuniculi and other microsporidian indicate that microsporidia lack MetAP1; hence fumagillin could selectively inhibit the growth of microsporidia in mammalian cells. MetAP1 can compensate for the inhibition of MetAP2 by fumagillin for the eukaryotic host cells, but inhibition of MetAP2 in microsporidia inhibits an essential enzyme in the pathogens. Unlike albendazole, fumagillin has been shown to be effective against Ent. bieneusi. Intestinal infection patients with dosages of 60 mg/day exhibited completely clearing of Ent. bieneusi (Molina et al., 2000; Molina et al., 2002). In a pediatric case of digestive microsporidiosis in a liver-kidney transplant child, treatment with fumagillin alone successfully eliminated the parasite (Desoubeaux et al., 2013). Fumagillin and a semisynthetic analog TNP-470 (6) are also effective against Enc. cuniculi, Enc. hellem, Enc. intestinalis, and V. corneae (Diesenhouse et al., 1993; Didier, 1997; Coyle et al., 1998). In a case of disseminated A. algerae infection, after failure of albendazole-based therapy, combined use of albendazole and fumagillin treatment resulted in clinical response and patient survival (Boileau et al., 2016). Oral treatment with fumagillin or TNP470 was also shown to control Loma salmonae or Nucleospora salmonis infection in the Chinook salmon (Oncorhynchus tshawytscha) (Higgins et al., 1998). Fumagilin-B soluble powder, a water soluble preparation containing bicyclohexylammonium fumagillin, is widely used to control N. apis in infected honeybees around the world. There are concerns about the toxicity of fumagillin and, consequentially, many countries outside of the Americas (including the European Union) have banned fumagillin for agricultural use (European Commission, 2010). In humans, treated for microsporidiosis, fumagillin has caused reversible thrombocytopenia as well as aseptic meningitis (Molina et al., 1997; Audemard et al., 2012).
Nitazoxanide (7) was developed and commercialized as an antiprotozoal drug against a broad range of parasites including protozoa, nematodes, cestodes, and trematodes (Rossignol and Maisonneuve, 1984; Cabello et al., 1997; Doumbo et al., 1997). Subsequently, it was also identified as a broad-spectrum antiviral drug, e.g., clinical trials found that oral administration of nitazoxanide reduced the duration of clinical symptoms caused by influenza (Haffizulla et al., 2014). An in vitro assay of nitazoxanide demonstrated that it had moderate activity in inhibiting the growth of Enc. intestinalis and V. corneae (Didier et al., 1998). Two case reports demonstrated that nitazoxanide therapy treatment successfully inhibited microsporidiosis in patients (Bicart-See et al., 2000; Saffo and Mirza, 2019). While the mechanism of action of nitazoxanide is not fully known, the most accepted nitazoxanide target is pyruvate: ferredoxin; however, microsporidia lack pyruvate:ferredoxin oxidoreductase and use pyruvate dehydrogenase in its place (Keeling, 2001; Hoffman et al., 2007). Nitazoxanide also has other targets and a possible nitazoxanide target in microsporidia is protein disulfide isomerase (Muller et al., 2008). In general, more trials are required to evaluate the possible role of nitazoxanide in treating microsporidiosis.
Commonly known as putrescine, spermidine and spermine, polyamines are low molecular weight organic chemicals with more than two amino groups. Polyamines play an important role in cell growth and differentiation by binding to nucleic acids through electrostatic forces and hydrogen bonding interactions. Due to their important roles in cellular physiology the intracellular concentrations of polyamines are strictly controlled through uptake, synthesis, interconversion, and degradation (Feuerstein et al., 1991; Casero, and Pegg, 1993; Marton and Pegg, 1995; Bacchi et al., 2003). Polyamine concentrations are elevated in tumors compared to normal tissue and, therefore, many researchers have focused on the development of polyamine analogs as anti-cancer therapeutic agents (Hayes et al., 2014). Enc. cuniculi has polyamine pathways that include synthesis and back-conversion (Bacchi et al., 2001; Ma et al., 2020). Isotope label studies indicate that the intracellular polyamine levels of pre-emergent Enc. cuniculi spores are dependent on uptake and interconversion rather than synthesis (Yarlett and Bacchi, 1988), indicating that synthetic polyamines targeting interconversion enzymes like spermidine spermine, N-acetyl transferase and polyamine oxidase are potential therapeutic agents for microsporidiosis. N′, N″-bis (ethyl) norspermine (BE-3-3-3) (8), a polyamine analogue that induces spermidine spermine N-acetyl transferase and down-regulates polyamine metabolism, inhibited Enc. cuniculi growth in RK-13 cells (Coyle et al., 1996; Figure 1). In vivo therapeutic studies in immunosuppressed mice demonstrated that synthetic polyamine analogs SL-11144 (9) and SL-11158 (10) are able to cure microsporidiosis due to Enc. cuniculi (Bacchi et al., 2002; Figure 1). In a SCID mouse model, the synthetic polyamine analogues were shown to have superior efficiency against Ent. bieneusi to that of fumagillin (Feng et al., 2009). Due to the reliance on uptake rather than synthesis of polyamines, screening, or developing new polyamine analogs appears to be a useful future direction for the development of new therapeutic agents for the treatment of microsporidiosis.
Lipids are vital metabolites for multiplication and differentiation in eukaryotes. The formation of membranous structures is highly reliant on the amount of available lipids. Orlistat (11) is a derivative of lipstatin (isolated from Streptomyces toxytricini) which can irreversibly and efficiently block pancreatic and gastric lipase (Figure 1). It is minimally absorbed when given orally and its effect is due to inhibition of lipases in the gastrointestinal tract. Orlistat was approved in 1999 by the FDA (United States) for obesity management (Derosa and Maffioli, 2012). Interestingly, orlistat has also been shown to have activity against Giardia intestinalis (duodenalis), Trypanosoma brucei, and Mycobacterium tuberculosis (Parker et al., 2009; Yang et al., 2012; Hahn et al., 2013). In mice infected with either Ent. bieneusi or Enc. intestinalis, orlistat treatment was shown to decrease the amount of spores seen in stool and the intestinal spore load (Abou-El-Naga et al., 2019). The effect seen was similar to that observed with fumagillin or albendazole treatment. Orlistat may affect these pathogens directly by inhibiting their lipid metabolizing enzymes or indirectly by affecting the lipid supply through inhibition of host enzymes (McClendon et al., 2009; Hahn et al., 2013). Genome analysis of microsporidia indicates that microsporidia lack many genes required for the biosynthesis of many important metabolites including lipids (Nakjang et al., 2013), to this end the antagonistic effect of orlistat on growth may be due to inhibition of the breakdown of lipids by the host, limiting the available lipid precursors for uptake by the microsporidia in the gut. Microsporidia have been shown to have an intact phospholipid metabolic pathway for synthesizing membrane phospholipids and this pathway is different from that of other eukaryotes (i.e., host cells). As phospholipids account for 60% of the total lipids in microsporidia and phosphatidic acid has been proved to be a limiting host metabolite for the proliferation of Tubulinosema ratisbonensis in Drosophila (El Alaoui et al., 2001a,b; Franchet et al., 2019), their phospholipid biosynthesis pathway may also be a promising therapeutic target.
Chitin is a carbohydrate polymer which provides high resistance to the environment and confers structural rigidity to the spore wall of microsporidia. Chitin is synthesized by a large family of chitin synthase enzymes which can be clustered into seven discernable classes (Roncero, 2002). As chitin is not found in mammalian cells and is an essential component of the microsporidian spores, chitin synthase is a promising target for antimicrosporidial drug development. Nikkomycin and polyoxin are peptide-nucleoside antibiotic which block chitin synthesis and fungal growth (Figure 1). They have structural similarity to UDP-N-acetylglucosamine, a ubiquitous and essential metabolite for chitin synthesis, which allows them to competitively inhibit chitin synthases that use UDP-N-acetylglucosamine to synthesize linear chitin molecules (Groll et al., 1998). Nikkomycin Z (12) inhibited the growth of Enc. hellem in fetal bovine lung fibroblast cells and the infectivity of any spores that developed in drug-treated cultures (Bigliardi et al., 2000). Polyoxin D (13) and nikkomycin Z were shown to reduce the number of parasitic foci in Enc. cuniculi infected monkey kidney cells (Sobottka et al., 2002). The non-specific chitin synthase inhibitor lufenuron was shown to inhibit the in vitro growth of Enc. intestinalis and V. corneae (Didier et al., 1998). Fungi have different classes of chitin synthases with distinct functions. For example, Saccharomyces cerevisiae has three chitin synthases with distinct functions in cell wall expansion, septum formation, and budding, while filamentous fungi generally have seven or eight distinct chitin synthases (Brosson et al., 2005). This property hindered the application of existing chitin synthase inhibitors, such as polyoxin or nikkomycin, because they usually inhibit one class of chitin synthases, but show less efficiency against other classes of these enzymes. In contrast, microsporidia possess a single type of class IV chitin synthase (Brosson et al., 2005). Drugs that are specifically targeting this type of chitin synthase may be sufficient to inhibit the growth of microsporidia. Microsporidia are obligate intracellular pathogens, unlike many fungi, and thus transportation across the plasma membrane may be the major factor influencing the effectiveness of hydrophilic compounds like nikkomycin and polyoxin.
Quinolones contain a bicyclic core structure related to the compound 4-quinolone. These drugs target type II topoisomerases, DNA gyrases, and type IV DNA topoisomerases of pathogens. They are broad-spectrum synthetic antibiotics which are widely used for the treatment of bacterial infections (Hooper, 2000; Andersson and MacGowan, 2003; Liu and Mulholland, 2005). Fluoroquinolones were derived from quinolones by modifying their structure with fluorine and have the same mechanism of action as quinolones. Genome sequence data demonstrates that V. corneae has a gene with high level of identity with DNA topoisomerase IV C subunit, an enzyme previously identified only from prokaryotes (Mittleider et al., 2002). Therefore, researchers have examined the efficacy of (fluoro) quinolones for inhibition of the growth of V. corneae (Didier et al., 2005; Figure 1). Their results indicate that gatifloxacin (14), lomefloxacin (15), moxifloxacin (16), and nalidixic acid (17) could inhibit V. corneae growth in vitro and in vivo, and that gatifloxacin, lomefloxacin, norfloxacin (18), and ofloxacin (19) prolonged survival of V. corneae-infected mice. Interestingly, gatifloxacin, lomefloxacin, moxifloxacin, and nalidixic acid could also inhibit Enc. intestinalis which does not have an identified type IV topoisomerase in its genome, indicating that quinolones may interact with other targets in addition to classic type IV topoisomerases.
Porphyrins are ubiquitous aromatic heterocyclic compounds in nature, they participate in many important biochemical processes in living organisms, such as oxygen transport and photosynthesis. Traditionally used against cancer, some porphyrins show inhibitory effect against bacteria, viruses, fungi, and protozoa (Jori et al., 2006; Allison and Moghissi, 2013). The synthetic amphiphilic protoporphyrin derivatives TMePyP (20) and PPIX(Asp)2 (21) prevented N. ceranae spore development in A. mellifera (Ptaszynska et al., 2018; Figure 1). Protoporphyrin derivatives with other amino acid moieties, PPIX[Lys-TFA]2 (22) and PPIX[Lys-Lys]2 (23), were also shown to reduce spore loads in infected honey bees (Buczek et al., 2020). In general, in response to light (or radiation) porphyrins react with oxygen to produce cytotoxic reactive oxygen species such as superoxide, hydrogen peroxide, or hydroxyl radicals, and these highly reactive radicals react with organic substrates to produce cytotoxicity (Castano et al., 2004). However, the mechanism of inactivation of microsporidia is currently not known, as light or radiation does not appear to be involved in the ability of porphyrins to inhibit microsporidia.
The mechanism by which microsporidia invade host cells is unique. Under appropriate environmental conditions a spore germinates shooting out its polar tube and transferring its sporoplasm into a host cell through the extruded polar tube (Han et al., 2017; Jaroenlak et al., 2020). This is a critical process in the life cycle of these organisms and enzymes or factors involved in spore germination are potential therapeutic drug targets. The exact mechanism of spore germination and polar tube discharge is not well-understood, and conditions that activate spores vary widely among species, including pH condition, cations, and calcium. Experimental evidence using Glugea hertwigi has suggested that calcium flux plays an important role in the initiation of spore discharge (Weidner and Byrd, 1982). Displacement of calcium from the polaroplast membrane is thought to trigger polaroplast contraction or combine with the polaroplast matrix to cause polaroplast swelling providing the propulsive force for germination (Weidner, 1982; Weidner and Byrd, 1982). Both calcium antagonists (verapamil and lanthanum) and calmodulin inhibitors (trifluroperazine and chlorpromazine) have been demonstrated to prevent spore germination in Spraguea lophii (Pleshinger and Weidner, 1985). The calcium channel blocker nifedipine inhibited the germination of Enc. hellem and Enc. intestinalis spores in vitro (Leitch et al., 1993; He et al., 1996). However, no in vivo data exists regarding the use of calcium channel blockers in microsporidiosis.
Based on the osmotic pressure theory of spore germination, water flow across the spore wall and plasma membrane which is accompanied by swelling of the polaroplasts and posterior vacuole after germination, results in quickly increased osmotic pressure and the corresponding eversion of the polar tube and subsequent expulsion of the sporoplasm (Frixione et al., 1997). To this end, aquaporins are also a potential target for inhibiting spore germination, because they are critical proteins for water flow across membranes (Ghosh et al., 2006a,b). The aquaporin inhibitor HgCl2 has been shown to be effective in inhibiting A. algerae spore germination (Frixione et al., 1997). However, HgCl2 is highly toxic and has significant health risks, therefore, while a proof of principle for aquaporin inhibition as a target for inhibition of germination, it is not a useful drug for the treatment of microsporidiosis.
Triosephosphate isomerase is a ubiquitous enzyme which catalyzes the interconversion between triose phosphate isomers dihydroxyacetone phosphate and D-glyceraldehyde-3-phosphate. As the process triosephosphate isomerase catalyzes is essential for the glycolytic pathway and many parasites require this enzyme for efficient energy production, several triosephosphate isomerase inhibitors have been studied in various parasitic infections. A selective inhibitor of Trypanosoma cruzi triosephosphate isomerase dramatically reduced parasites in the blood of experimentally infected mice and greatly enhanced their survival rate (Aguilera et al., 2016). Selective Fasciola hepatica triosephosphate isomerase inactivators could kill the juvenile form of F. hepatica in low concentration and showed low host toxicity (Ferraro et al., 2020). In addition to its effect on energy production, inhibition of triosephosphate isomerase results in accumulation of dihydroxyacetone phosphate or D-glyceraldehyde 3-phosphate, which can be cytotoxic (Han and Weiss, 2018). It has been shown that thiol-reactive compounds like sulbutiamine, rabeprazole, and omeprazole can effectively inhibit the triosephosphate isomerase of Enc. intestinalis (Garcia-Torres et al., 2018). As these drugs do not significantly inactivate human triosephosphate isomerase (Garcia-Torres et al., 2016), they may be considered as new potential drugs for treating microsporidiosis.
Analysis of the available sequenced microsporidian genomes on MicrosporidiaDB.org suggests that they have obtained several genes via horizontal gene transfer events, such as the ADP/ATP translocase gene family transferred from Chlamydiae and glutamate-ammonia ligase from an unknown prokaryotic source (Pombert et al., 2012). Thymidine kinase is a ley enzyme in the nucleic acid salvage pathway, it catalyzes the phosphorylation of thymidine to thymidine monophosphate. Thymidine kinase is thought to have been lost in the fungal lineage shortly after it diverged from animals (Alexander et al., 2014). However, two independent horizontal transfer events of thymidine kinase have occurred in microsporidia, including transfers of bacterial thymidine kinase genes into several microsporidia taxa and transfer of a putative viral-like thymidine kinase into Nematocida parisii (Alexander et al., 2016). Several prodrugs specifically target thymidine kinase, like 5-fluoro-2-deoxyuridine and acyclovir, and these are widely applied to treat simplex virus and several types of cancer cells (Furman and Barry, 1988; Simmons, 2002). Heterologous expression of a microsporidian thymidine kinase in S. cerevisiae can convert 5-fluoro-2-deoxyuridine into fluorodeoxyuridine monophosphate, completely inhibited the growth of the transgenic S. cerevisiae (Alexander et al., 2016). It is reasonable to postulate that the microsporidia that have thymidine kinase in their genomes maybe susceptible to such thymidine kinase prodrugs.
Most complementary and alternative medicine strategies for the treatment of microsporidiosis focus on the treatment of nosemosis in honey bees. This is because while fumagillin is the only veterinary regiment recommended by the World Organization for Animal Health for treating nosemosis in honey bees it is no longer licensed in the European Union. As a result, there is a need for alternatives to control this disease and several “natural” products have been sold as veterinary treatments for nosemosis such as Nosestat® and Vitafeed Gold®. Many of these alternative strategies have now been proven by controlled studies to be ineffective (Botias et al., 2013). More research is clearly needed to develop reliable and effective alternative strategies for the treatment of nosemosis in bees. Experience with these alternative strategies for treating nosemosis in bees should also provide new therapeutic ideas for the treatment of microsporidiosis in human or other animals.
Various plants have been used in traditional herbal remedies around the world and are reported to have numerous pharmacological activities, however, the mechanisms underlying these reported effects and the active substance in the various plant extracts is often unknown. Historically, plants have been sources of pharmacologically active compounds against many types of pathogens and plant extracts have been evaluated and used for the treatment of infectious diseases caused by bacteria, fungi, protozoa, and viruses (Ali-Shtayeh and Abu Ghdeib, 1999; Yoshida et al., 2005; Thembo et al., 2010). Such studies have resulted in new therapeutic agents such as the use of artemisinin, an antimalarial compound derived from Artemisia carvifolia. Various plant extracts have been evaluated as therapeutic agents for microsporidiosis. Ethanolic extracts of Laurus nobilis were demonstrated to inhibit N. ceranae development in A. mellifera at a concentration of 1% in syrup (Porrini et al., 2011). Decoction extracts from the Chinese herb Andrographis paniculata significantly inhibited proliferation of N. ceranae and improved the survival rate of infected bees in a dose-dependent manner (Chen et al., 2021). In addition, extracts of Aster scaber and Artemisia dubia were also demonstrated to have anti-nosemosis activity and this activity was increased when extracts from both plants were used together (Kim et al., 2016). A study that examined methanolic extracts from leaves used in traditional medicine in Indonesian found that several Indonesian plants were able to inhibit microsporidia, the extract from Diospyros sumatrana was shown in vitro to inhibit Enc. cuniculi and its activity was similar to that of albendazole against Enc. cuniculi (Sak et al., 2017). Sulfated polysaccharides extracted from several algae strains significantly reduced the parasite load of N. ceranae and improved the survival rate of infected bees (Roussel et al., 2015). Given these observations with various plant extracts that inhibit the proliferation of microsporidia, further work now needs to focus on the purification and structural identification of the key antimicrosporidial compounds in these extracts.
Propolis is a hard resinous hive product collected by honeybee workers from the juices of various plants. The chemical composition of propolis is quite complicated, including aldehydes, polysaccharides, ketones, terpenes, steroids, amino acids, hydrocarbons, and several other compounds (Bankova et al., 1983; Marcucci, 1995). Propolis exhibits anti-bacteria, anti-virus, and anti-fungus activity. Several studies have examined if propolis can control microsporidiosis. Ethanol extracts of propolis obtained from propolis structures of the stingless bee Trigona apicalis significantly reduced the N. ceranae infection rate and bee mortality rate (Bankova et al., 1983). In addition, this propolis extract treatment increased the trehalose levels and hypopharyngeal gland protein content in treated bees compared to an untreated control group (Suwannapong et al., 2018). Dichloromethane extract of propolis from Upstate New York greatly reduced N. ceranae spore loads in a dose-dependent manner (Burnham et al., 2020). A study in Italy that evaluated an ethanol extract of propolis from the honey bee A. mellifera demonstrated that treatment decreased the spore load of N. ceranae-infected worker bees (Mura et al., 2020). In addition, the food consumption and longevity of the propolis treated group increased. High performance liquid chromatography analysis revealed that there are many organic compounds belonging to flavones, flavonols, and simple phenols in the propolis extract and the active antimicrosporidial compounds were not identified. More research is needed on the application of propolis for the control of microsporidiosis, including the purification and identification of the active compounds in the ethanol extracts as well as studies that examine the activity of these propolis extracts against other microsporidia such as N. bombycis and Encephalitozoon spp.
Probiotics have been used in both vertebrates and invertebrates to modulate and maintain intestinal health, and they have also been utilized as adjunctive therapy in the treatment of gastrointestinal infections (Silva et al., 2020). Lactobacillus kunkeei strains isolated from the gut of bees have shown a potential beneficial effect of decreasing N. ceranae infections (Arredondo et al., 2018). Enterococcus faecium also significantly reduced N. ceranae load in infected bees (Borges et al., 2021). The protective effect of probiotics could be due to metabolites produced by bacteria, such as bacteriocin and surfactin (Porrini et al., 2010; Mossallam et al., 2014). Unfortunately, other studies have demonstrated that probiotics are ineffective for the prevention and treatment of microsporidiosis. Several commercial probiotics and prebiotics have been demonstrated in controlled trials to be ineffective in the treatment and prevention of nosemosis in N. ceranae (Ptaszynska et al., 2016). A randomized placebo-controlled study in AIDS patients with non-infectious diarrhea or gastrointestinal symptoms caused by microsporidia indicated that there were no significant differences in patients treated with Lactobacillus rhamnosus compared to a placebo treatment group (Salminen et al., 2004). The role of the microbiome in infectious disease therapy and pathogenesis is a rapidly evolving area of investigation. Future research on the isolation, clinical efficacy, and antagonistic mechanisms of various probiotics is clearly needed for the development of this therapeutic approach.
Microsporidia have become not only important emerging human pathogens in immune competent and immune-compromised individuals, but are also major threats to industries such as sericulture, apiculture, and aquaculture. The known therapeutic targets for microsporidiosis mentioned are summarized in Figure 2. The currently approved drugs for the treatment of microsporidiosis are albendazole and fumagillin, which target β-tubulin and MetAP2, respectively. Fumagillin has a broader antimicrosporidial activity compared to albendazole as it can inhibit Encephalitozoon spp., V. corneae, and Ent. bieneusi, while albendazole has limited activity against V. corneae and Ent. bieneusi. Apart from these two well studied targets, more therapeutic targets are still required for microsporidiosis drug development. Chitin is a crucial component of the spore wall. Microsporidia have been shown to have a single chitin synthase gene in their genomes, making this an excellent potential therapeutic target as vertebrates do not have this biochemical pathway. Drugs that target the polyamine pathway have been shown to inhibit microsporidia in vitro and to be effective in experimental murine microsporidiosis. These polyamine analogues are promising therapeutic agents that deserve further study. Some studies have demonstrated that fluoroquinolones can inhibit microsporidia, however, microsporidia lack type IV topoisomerase which is the known target of fluoroquinolones. Studies on the target of these drugs in microsporidia are clearly needed to further the development of fluoroquinolones as therapeutic agents against microsporidia. The effect of orlistat on microsporidia in experimental murine models is intriguing. This observation suggests that drugs that target lipid metabolism could be useful therapeutic agents for microsporidiosis as well as illustrates that non-absorbed drugs in the gastrointestinal tract can still have activity against microsporidia. Several of the previously mentioned chemicals or their derivatives are already in wide use for other purposes. Investigators are also screening FDA-approved drug libraries to repurpose them for microsporidiosis. This approach holds promise to accelerate development as these repurposed drugs do not require as much of an investment as novel molecules. Complementary and alternative medicine strategies for microsporidiosis need to be carefully evaluated for efficacy as illustrated by controlled studies that demonstrated that several widely used natural treatments for nosematosis were ineffective. Extracts from medicinal plants have been demonstrated to have antimicrosporidial activity and the active component in these extracts need to be identified in order to further develop these therapies. Microsporidia have reduced genomes with corresponding reductions in anabolism and catabolism providing opportunities for the development of agents that target critical enzymes in these streamlined pathways. Microsporidia also have a unique mechanism of invasion requiring spore germination and extrusion of a unique organelle, the polar tube, for successful infection transmission. Screening compound libraries to identify compounds that interfere with this unique invasion process could be a fruitful avenue of investigation for the identification of new therapeutic agents.
Figure 2. ∣ Mechanism of action of therapy and therapeutic targets for microsporidiosis. The names of therapeutic targets are presented in bold. The mode of action of orlistat and nitazoxanide are to be determined and are not shown in this figure.
JW, LW, and ZZ conceptualized and wrote the draft of the manuscript. ZF collected and prepared data about alternative medicine strategies. All the authors revised and approved the final manuscript.
This manuscript was funded by grants National Natural Science Foundation of China (Grant Nos. 31702185 and 31770159), Natural Science Foundation of Chongqing, China (Grant Nos. cstc2019jcyj-msxmX0511 and cstc2018jcyj-yszxX0012), and the National Institutes of Allergy and Infectious Diseases-USA (Grant No. R01AI124753).
The authors declare that the research was conducted in the absence of any commercial or financial relationships that could be construed as a potential conflict of interest.
All claims expressed in this article are solely those of the authors and do not necessarily represent those of their affiliated organizations, or those of the publisher, the editors and the reviewers. Any product that may be evaluated in this article, or claim that may be made by its manufacturer, is not guaranteed or endorsed by the publisher.
We are thankful to all the researchers whose studies have been reviewed in this manuscript. We deeply thank Xianzhi Meng for reviewing the scientific aspects of the manuscript.
A. algerae, Anncaliia algerae; A. penaei, Agmasoma penaei; AIDS, acquired immune deficiency syndrome; A. mellifera, Apis mellifera; Enc. cuniculi, Encephalitozoon cuniculi; Enc. hellem, Encephalitozoon hellem; Enc. intestinalis, Encephalitozoon intestinalis; Ent. bieneusi, Enterocytozoon bieneusi; EHP, Enterocytozoon hepatopenaei; F. hepatica, Fasciola hepatica; MetAP1, methionine aminopeptidase type 1; MetAP2, methionine aminopeptidase type 2; N. apis, Nosema apis; N. bombycis, Nosema bombycis; N. ceranae, Nosema ceranae; S. cerevisiae, Saccharomyces cerevisiae; V. corneae, Vittaforma corneae.
Abou-El-Naga, I. F., Said, D. E., Gaafar, M. R., Ahmed, S. M., and El-Deeb, S. A. (2019). A new scope for orlistat: effect of approved anti-obesity drug against experimental microsporidiosis. Med. Mycol. 57, 181–195. doi: 10.1093/mmy/myy005
Aguilera, E., Varela, J., Birriel, E., Serna, E., Torres, S., Yaluff, G., et al. (2016). Potent and selective inhibitors of Trypanosoma cruzi triosephosphate isomerase with concomitant inhibition of cruzipain: inhibition of parasite growth through multitarget activity. ChemMedChem 11, 1328–1338. doi: 10.1002/cmdc.201500385
Akiyoshi, D. E., Weiss, L. M., Feng, X., Williams, B. A., Keeling, P. J., Zhang, Q., et al. (2007). Analysis of the beta-tubulin genes from Enterocytozoon bieneusi isolates from a human and rhesus macaque. J. Eukaryot. Microbiol. 54, 38–41. doi: 10.1111/j.1550-7408.2006.00140.x
Albrecht, H., Stellbrink, H. J., and Sobottka, I. (1995). Failure of itraconazole to prevent Enterocytozoon bieneusi infection. Genitourin. Med. 71, 325–326. doi: 10.1136/sti.71.5.325
Alexander, W. G., Doering, D. T., and Hittinger, C. T. (2014). High-efficiency genome editing and allele replacement in prototrophic and wild strains of Saccharomyces. Genetics 198, 859–866. doi: 10.1534/genetics.114.170118
Alexander, W. G., Wisecaver, J. H., Rokas, A., and Hittinger, C. T. (2016). Horizontally acquired genes in early-diverging pathogenic fungi enable the use of host nucleosides and nucleotides. Proc. Natl. Acad. Sci. U.S.A 113, 4116–4121. doi: 10.1073/pnas.1517242113
Ali-Shtayeh, M. S., and Abu Ghdeib, S. I. (1999). Antifungal activity of plant extracts against dermatophytes. Mycoses 42, 665–672. doi: 10.1046/j.1439-0507.1999.00499.x
Allison, R. R., and Moghissi, K. (2013). Photodynamic Therapy (PDT): PDT Mechanisms. Clin. Endosc. 46, 24–29. doi: 10.5946/ce.2013.46.1.24
Anderson, N. W., Muehlenbachs, A., Arif, S., Bruminhent, J., Deziel, P. J., Razonable, R. R., et al. (2019). A fatal case of disseminated microsporidiosis due to Anncaliia algerae in a renal and pancreas allograft recipient. Open Forum. Infect. Dis. 6:ofz285. doi: 10.1093/ofid/ofz285
Andersson, M. I., and MacGowan, A. P. (2003). Development of the quinolones. J. Antimicrob. Chemother. 51(Suppl. 1), 1–11. doi: 10.1093/jac/dkg212
Andreadis, T. G., Simakova, A. V., Vossbrinck, C. R., Shepard, J. J., and Yurchenko, Y. A. (2012). Ultrastructural characterization and comparative phylogenetic analysis of new microsporidia from Siberian mosquitoes: evidence for coevolution and host switching. J. Invertebr. Pathol. 109, 59–75. doi: 10.1016/j.jip.2011.09.011
Aranguren, L. F., Han, J. E., and Tang, K. F. J. (2017). Enterocytozoon hepatopenaei (EHP) is a risk factor for acute hepatopancreatic necrosis disease (AHPND) and septic hepatopancreatic necrosis (SHPN) in the Pacific white shrimp Penaeus vannamei. Aquaculture 471, 37–42. doi: 10.1016/j.aquaculture.2016.12.038
Armstrong, E. (1976). Fumidil-B and Benomyl: chemical control of Nosema kingi in Drosophila willistoni. J. Invertebr. Pathol. 27, 363–366. doi: 10.1016/0022-2011(76)90097-5
Arredondo, D., Castelli, L., Porrini, M. P., Garrido, P. M., Eguaras, M. J., Zunino, P., et al. (2018). Lactobacillus kunkeei strains decreased the infection by honey bee pathogens Paenibacillus larvae and Nosema ceranae. Benef. Microbes 9, 279–290. doi: 10.3920/Bm2017.0075
Audemard, A., Le Bellec, M. L., Carluer, L., Dargere, S., Verdon, R., Castrale, C., et al. (2012). Fumagillin-induced aseptic meningoencephalitis in a kidney transplant recipient with microsporidiosis. Transpl. Infect. Dis. 14, E147–E149. doi: 10.1111/tid.12010
Bacchi, C. J., Lane, S., Weiss, L. M., Yarlett, N., Takvorian, P., Cali, A., et al. (2001). Polyamine synthesis and interconversion by the microsporidian Encephalitozoon cuniculi. J. Eukaryot. Microbiol. 48, 374–381. doi: 10.1111/j.1550-7408.2001.tb00327.x
Bacchi, C. J., Weiss, L. M., Lane, S., Frydman, B., Valasinas, A., Reddy, V., et al. (2002). Novel synthetic polyamines are effective in the treatment of experimental microsporidiosis, an opportunistic AIDS-Associated infection. Antimicrob. Agents Chemother. 46, 55–61. doi: 10.1128/Aac.46.1.55-61.2002
Bacchi, C. J., Yarlett, N., and Weiss, L. M. (2003). Polyamine metabolism in the Microsporidia. Biochem. Soc. Trans. 31, 420–423. doi: 10.1042/bst0310420
Bankova, V. S., Popov, S. S., and Marekov, N. L. (1983). A study on flavonoids of propolis. J. Nat. Prod. 46, 471–474. doi: 10.1021/np50028a007
Barnes, T. B., Verlangieri, A. J., and Wilson, M. C. (1983). Reproductive toxicity of methyl-1-(butylcarbamoyl)-2-benzimidazole carbamate (benomyl) in male Wistar rats. Toxicology 28, 103–115. doi: 10.1016/0300-483x(83)90110-5
Beauvais, B., Sarfati, C., Challier, S., and Derouin, F. (1994). In vitro model to assess effect of antimicrobial agents on Encephalitozoon cuniculi. Antimicrob. Agents Chemother. 38, 2440–2448. doi: 10.1128/aac.38.10.2440
Beznoussenko, G. V., Dolgikh, V. V., Seliverstova, E. V., Semenov, P. B., Tokarev, Y. S., Trucco, A., et al. (2007). Analogs of the Golgi complex in microsporidia: structure and avesicular mechanisms of function. J. Cell Sci. 120(Pt 7), 1288–1298. doi: 10.1242/jcs.03402
Bhat, S. A., Bashir, I., and Kamili, A. S. (2009). Microsporidiosis of silkworm, Bombyx mori L. (Lepidoptera-bombycidae): a review. Afr. J. Agr. Res. 4, 1519–1523. doi: 10.5897/AJAR.9000490
Bicart-See, A., Massip, P., Linas, M. D., and Datry, A. (2000). Successful treatment with nitazoxanide of Enterocytozoon bieneusi microsporidiosis in a patient with AIDS. Antimicrob. Agents Chemother. 44, 167–168. doi: 10.1128/Aac.44.1.167-168.2000
Bigliardi, E., Bernuzzi, A. M., Corona, S., Gatti, S., Scaglia, M., and Sacchi, L. (2000). In vitro efficacy of nikkomycin Z against the human isolate of the microsporidian species Encephalitozoon hellem. Antimicrob. Agents Chemother. 44, 3012–3016. doi: 10.1128/aac.44.11.3012-3016.2000
Boileau, M., Ferreira, J., Ahmad, I., Lavallee, C., Qvarnstrom, Y., and Dufresne, S. F. (2016). Successful treatment of disseminated Anncaliia algerae microsporidial infection with combination fumagillin and albendazole. Open Forum. Infect. Dis. 3:ofw158. doi: 10.1093/ofid/ofw158
Borges, D., Guzman-Novoa, E., and Goodwin, P. H. (2021). Effects of prebiotics and probiotics on honey bees (Apis mellifera) infected with the microsporidian parasite Nosema ceranae. Microorganisms 9:481. doi: 10.3390/microorganisms9030481
Botias, C., Martin-Hernandez, R., Meana, A., and Higes, M. (2013). Screening alternative therapies to control Nosemosis type C in honey bee (Apis mellifera iberiensis) colonies. Res. Vet. Sci. 95, 1041–1045. doi: 10.1016/j.rvsc.2013.09.012
Brooks, W. M., Cranford, J. D., and Pearce, L. W. (1978). Benomyl: effectiveness against the microsporidian Nosema heliothidis in the corn earworm, Heliothis zea. J. Invertebr. Pathol. 31, 239–245. doi: 10.1016/0022-2011(78)90013-7
Brosson, D., Kuhn, L., Prensier, G., Vivares, C. P., and Texier, C. (2005). The putative chitin deacetylase of Encephalitozoon cuniculi: a surface protein implicated in microsporidian spore-wall formation. FEMS Microbiol. Lett. 247, 81–90. doi: 10.1016/j.femsle.2005.04.031
Buczek, K., Trytek, M., Derylo, K., Borsuk, G., Rybicka-Jasinska, K., Gryko, D., et al. (2020). Bioactivity studies of porphyrinoids against microsporidia isolated from honeybees. Sci. Rep. 10:11553. doi: 10.1038/s41598-020-68420-5
Burnham, A. J., De Jong, E., Jones, J. A., and Lehman, H. K. (2020). North American propolis extracts from Upstate New York decrease Nosema ceranae(Microsporidia) spore levels in honey bees (Apis mellifera). Front. Microbiol. 11:1719. doi: 10.3389/fmicb.2020.01719
Cabello, R. R., Guerrero, L. R., Garcia, M. D. M., and Cruz, A. G. (1997). Nitazoxanide for the treatment of intestinal protozoan and helminthic infections in Mexico. T. Roy. Soc. Trop. Med. H. 91, 701–703. doi: 10.1016/S0035-9203(97)90531-9
Calderone, N. W. (2012). Insect pollinated crops, insect pollinators and US agriculture: trend analysis of aggregate data for the period 1992-2009. PLoS One 7:e37235. doi: 10.1371/journal.pone.0037235
Cali, A., Neafie, R., Weiss, L. M., Ghosh, K., Vergara, R. B., Gupta, R., et al. (2010). Human vocal cord infection with the Microsporidium Anncaliia algerae. J. Eukaryot. Microbiol. 57, 562–567. doi: 10.1111/j.1550-7408.2010.00510.x
Cali, A., and Takvorian, P. M. (2003). Ultrastructure and development of Pleistophora ronneafiei n. sp., a microsporidium (Protista) in the skeletal muscle of an immune-compromised individual. J. Eukaryot. Microbiol. 50, 77–85. doi: 10.1111/j.1550-7408.2003.tb00237.x
Cali, A., Takvorian, P. M., Lewin, S., Rendel, M., Sian, C. S., Wittner, M., et al. (1998). Brachiola vesicularum, n. g., n. sp., a new microsporidium associated with AIDS and myositis. J. Eukaryot. Microbiol. 45, 240–251. doi: 10.1111/j.1550-7408.1998.tb04532.x
Cama, V. A., Pearson, J., Cabrera, L., Pacheco, L., Gilman, R., Meyer, S., et al. (2007). Transmission of Enterocytozoon bieneusi between a child and guinea pigs. J. Clin. Microbiol. 45, 2708–2710. doi: 10.1128/JCM.00725-07
Canning, E. U., and Hollister, W. S. (1990). Enterocytozoon bieneusi (Microspora) - prevalence and pathogenicity in AIDS patients. Trans. R. Soc. Trop. Med. Hyg. 84, 181–186. doi: 10.1016/0035-9203(90)90247-C
Casero, R. A., and Pegg, A. E. (1993). Spermidine/spermine N1-acetyltransferase–the turning point in polyamine metabolism. FASEB J. 7, 653–661. doi: 10.1096/fasebj.7.8.8500690
Castano, A. P., Demidova, T. N., and Hamblin, M. R. (2004). Mechanisms in photodynamic therapy: part one-photosensitizers, photochemistry and cellular localization. Photodiagnosis Photodyn. Ther. 1, 279–293. doi: 10.1016/S1572-1000(05)00007-4
Chen, X., Wang, S., Xu, Y., Gong, H., Wu, Y., Chen, Y., et al. (2021). Protective potential of Chinese herbal extracts against microsporidian Nosema ceranae, an emergent pathogen of western honey bees, Apis mellifera L. J. Asia Pac. Entomol. 24, 502–512. doi: 10.1016/j.aspen.2019.08.006
Conteas, C. N., Berlin, O. G. W., Ash, L. R., and Pruthi, J. S. (2000). Therapy for human gastrointestinal microsporidiosis. Am. J. Trop. Med. Hyg. 63, 121–127. doi: 10.4269/ajtmh.2000.63.121
Coyle, C., Bacchi, C., Yarlett, N., Tanowitz, H. B., Wittner, M., and Weiss, L. M. (1996). Polyamine metabolism as a therapeutic target for microsporidia. J. Eukaryot. Microbiol. 43, S96–S96. doi: 10.1111/j.1550-7408.1996.tb05020.x
Coyle, C., Kent, M., Tanowitz, H. B., Wittner, M., and Weiss, L. M. (1998). TNP-470 is an effective antimicrosporidial agent. J. Infect. Dis. 177, 515–518. doi: 10.1086/517390
Derosa, G., and Maffioli, P. (2012). Anti-obesity drugs: a review about their effects and their safety. Expert Opin. Drug Saf. 11, 459–471. doi: 10.4093/dmj.2012.36.1.13
Desoubeaux, G., Maakaroun-Vermesse, Z., Lier, C., Bailly, E., Morio, F., Labarthe, F., et al. (2013). Successful treatment with fumagillin of the first pediatric case of digestive microsporidiosis in a liver-kidney transplant. Transpl. Infect. Dis. 15, E250–E259. doi: 10.1111/tid.12158
Desoubeaux, G., Peschke, R., Le-Bert, C., Fravel, V., Soto, J., Jensen, E. D., et al. (2018). Seroprevalence survey for microsporidia in common bottlenose dolphin (Tursiops truncatus): example of a Quantitative Approach Based on Immunoblotting. J. Wildl. Dis. 54, 870–873. doi: 10.7589/2017-11-287
Didier, E. S. (1997). Effects of albendazole, fumagillin, and TNP-470 on microsporidial replication in vitro. Antimicrob. Agents Chemother. 41, 1541–1546. doi: 10.1128/AAC.41.7.1541
Didier, E. S., Bowers, L., Stovall, M. E., Kuebler, D., Mittleider, D., Brindley, P. J., et al. (2005). Antimicrosporidial activity of (fluoro)quinolones in vitro and in vivo. Folia Parasit. 52, 173–181. doi: 10.14411/fp.2005.022
Didier, E. S., Maddry, J. A., Kwong, C. D., Green, L. C., Snowden, K. F., and Shadduck, J. A. (1998). Screening of compounds for antimicrosporidial activity in vitro. Folia Parasitol. 45, 129–139. doi: 10.1006/expr.1998.4215
Diesenhouse, M. C., Wilson, L. A., Corrent, G. F., Visvesvara, G. S., Grossniklaus, H. E., and Bryan, R. T. (1993). Treatment of microsporidial keratoconjunctivitis with topical fumagillin. Am. J. Ophthalmol. 115, 293–298. doi: 10.1016/s0002-9394(14)73578-0
Dieterich, D. T., Lew, E. A., Kotler, D. P., Poles, M. A., and Orenstein, J. M. (1994). Treatment with albendazole for intestinal disease due to Enterocytozoon bieneusi in patients with AIDS. J. Infect. Dis. 169, 178–183. doi: 10.1093/infdis/169.1.178
Dore, G. J., Marriott, D. J., Hing, M. C., Harkness, J. L., and Field, A. S. (1995). Disseminated microsporidiosis due to Septata intestinalis in nine patients infected with the human immunodeficiency virus response to therapy with albendazole. Clin. Infect. Dis. 21, 70–76. doi: 10.1093/clinids/21.1.70
Doumbo, O., Rossignol, J. F., Pichard, E., Traore, H. A., Dembele, M., Diakite, M., et al. (1997). Nitazoxanide in the treatment of cryptosporidial diarrhea and other intestinal parasitic infections associated with acquired immunodeficiency syndrome in tropical Africa. Am. J. Trop. Med. Hyg. 56, 637–639. doi: 10.4269/ajtmh.1997.56.637
Dumond, C., Aulagnon, F., Etienne, I., Heng, A. E., Bougnoux, M. E., Favennec, L., et al. (2021). Epidemiological and clinical study of microsporidiosis in French kidney transplant recipients from 2005 to 2019: trans-spore registry. Transpl. Infect. Dis. 23:e13708. doi: 10.1111/tid.13708
Dumortier, J., Radenne, S., Kamar, N., Conti, F., Abergel, A., Coilly, A., et al. (2021). Microsporidiosis after liver transplantation: a French nationwide retrospective study. Transpl. Infect. Dis. 23:e13665. doi: 10.1111/tid.13665
El Alaoui, H., Bata, J., Bauchart, D., Dore, J. C., and Vivares, C. P. (2001a). Lipids of three microsporidian species and multivariate analysis of the host-parasite relationship. J. Parasitol. 87, 554–559. doi: 10.1645/0022-3395(2001)087[0554:LOTMSA]2.0.CO;2
El Alaoui, H., Bata, J., Peyret, P., and Vivares, C. P. (2001b). Encephalitozoon cuniculi (Microspora): characterization of a phospholipid metabolic pathway potentially linked to therapeutics. Exp. Parasitol. 98, 171–179. doi: 10.1006/expr.2001.4635
European Commission (2010). Commission regulation (EU) No 37/2010 of 22 December 2009 on pharmacologically active substances and their classification regarding maximum residue limits in foodstuffs of animal origin. Offl. J. Eur. Union 1–72.
Feng, X., Reddy, V. K., Mayanja-Kizza, H., Weiss, L. M., Marton, L. J., and Tzipori, S. (2009). Therapeutic evaluation of polyamine analogue drug candidates against Enterocytozoon bieneusi in a SCID mouse model. Antimicrob. Agents Chemother. 53, 2417–2423. doi: 10.1128/AAC.01113-08
Ferraro, F., Corvo, I., Bergalli, L., Ilarraz, A., Cabrera, M., Gil, J., et al. (2020). Novel and selective inactivators of triosephosphate isomerase with anti-trematode activity. Sci. Rep. 10:2587. doi: 10.1038/s41598-020-59460-y
Feuerstein, B. G., Williams, L. D., Basu, H. S., andMarton, L. J. (1991). Implications and concepts of polyamine-nucleic acid interactions. J. Cell. Biochem. 46, 37–47. doi: 10.1002/jcb.240460107
Field, A. S., Marriott, D. J., Milliken, S. T., Brew, B. J., Canning, E. U., Kench, J. G., et al. (1996). Myositis associated with a newly described microsporidian, Trachipleistophora hominis, in a patient with AIDS. J. Clin. Microbiol. 34, 2803–2811. doi: 10.1128/JCM.34.11.2803-2811.1996
Flegel, T. W. (1997). Major viral diseases of the black tiger prawn (Penaeus monodon) in Thailand. World J. Microb. Biot. 13, 433–442. doi: 10.1023/A:1018580301578
Franchet, A., Niehus, S., Caravelle, G., and Ferrandon, D. (2019). Phosphatidic acid as a limiting host metabolite for the proliferation of the microsporidium Tubulinosema ratisbonensis in Drosophila files. Nat. Microbiol. 4, 645–655. doi: 10.1038/s41564-018-0344-y
Franzen, C., and Salzberger, B. (2008). Analysis of the beta-tubulin gene from Vittaforma corneae suggests benzimidazole resistance. Antimicrob. Agents Chemother. 52, 790–793. doi: 10.1128/AAC.00928-07
Fries, I. (2010). Nosema ceranae in European honey bees (Apis mellifera). J. Invertebr. Pathol. 103, S73–S79. doi: 10.1016/j.jip.2009.06.017
Frixione, E., Ruiz, L., Cerbon, J., and Undeen, A. H. (1997). Germination of Nosema algerae (Microspora) spores: conditional inhibition by D2O, ethanol and Hg2+ suggests dependence of water influx upon membrane hydration and specific transmembrane pathways. J. Eukaryot. Microbiol. 44, 109–116. doi: 10.1111/j.1550-7408.1997.tb05946.x
Furman, P. A., and Barry, D. W. (1988). Spectrum of antiviral activity and mechanism of action of zidovudine - An overview. Am. J. Med. 85, 176–181.
Garcia-Torres, I., De la Mora-De la Mora, I., Hernandez-Alcantara, G., Molina-Ortiz, D., Caballero-Salazar, S., Olivos-Garcia, A., et al. (2018). First characterization of a microsporidial triosephosphate isomerase and the biochemical mechanisms of its inactivation to propose a new druggable target. Sci. Rep. 8:8591. doi: 10.1038/s41598-018-26845-z
Garcia-Torres, I., de la Mora-de la Mora, I., Marcial-Quino, J., Gomez-Manzo, S., Vanoye-Carlo, A., Navarrete-Vazquez, G., et al. (2016). Proton pump inhibitors drastically modify triosephosphate isomerase from Giardia lamblia at functional and structural levels, providing molecular leads in the design of new antigiardiasic drugs. Biochim. Biophys. Acta 1860, 97–107. doi: 10.1016/j.bbagen.2015.10.021
Ghosh, K., Cappiello, C. D., McBride, S. M., Occi, J. L., Cali, A., Takvorian, P. M., et al. (2006a). Functional characterization of a putative aquaporin from Encephalitozoon cuniculi, a microsporidia pathogenic to humans. Int. J. Parasitol. 36, 57–62. doi: 10.1016/j.ijpara.2005.08.013
Ghosh, K., Takvorian, P. M., McBride, S. M., Cali, A., and Weiss, L. M. (2006b). Heterologous expression of an Encephalitozoon cuniculi aquaporin in Xenopus oocytes. J. Eukaryot. Microbiol. 53(Suppl. 1) 72–73. doi: 10.1111/j.1550-7408.2006.00178.x
Groll, A. H., Piscitelli, S. C., and Walsh, T. J. (1998). Clinical pharmacology of systemic antifungal agents: a comprehensive review of agents in clinical use, current investigational compounds, and putative targets for antifungal drug development. Adv. Pharmacol. 44, 343–500. doi: 10.1016/s1054-3589(08)60129-5
Gumbo, T., Sarbah, S., Gangaidzo, I. T., Ortega, Y., Sterling, C. R., Carville, A., et al. (1999). Intestinal parasites in patients with diarrhea and human immunodeficiency virus infection in Zimbabwe. AIDS 13, 819–821. doi: 10.1097/00002030-199905070-00011
Haffizulla, J., Hartman, A., Hoppers, M., Resnick, H., Samudrala, S., Ginocchio, C., et al. (2014). Effect of nitazoxanide in adults and adolescents with acute uncomplicated influenza: a double-blind, randomised, placebo-controlled, phase 2b/3 trial. Lancet Infect. Dis. 14, 609–618. doi: 10.1016/S1473-3099(14)70717-0
Hahn, J., Seeber, F., Kolodziej, H., Ignatius, R., Laue, M., Aebischer, T., et al. (2013). High sensitivity of Giardia duodenalis to tetrahydrolipstatin (orlistat) in vitro. PLoS One 8:e71597. doi: 10.1371/journal.pone.0071597
Han, B., Polonais, V., Sugi, T., Yakubu, R., Takvorian, P. M., Cali, A., et al. (2017). The role of microsporidian polar tube protein 4 (PTP4) in host cell infection. PLoS Pathog. 13:e1006341. doi: 10.1371/journal.ppat.1006341
Han, B., and Weiss, L. M. (2017). Microsporidia: obligate intracellular pathogens within the fungal kingdom. Microbiol. Spectr. 5:FUNK–0018–2016. doi: 10.1128/microbiolspec.FUNK-0018-2016
Han, B., and Weiss, L. M. (2018). Therapeutic targets for the treatment of microsporidiosis in humans. Expert Opin. Ther .Targets. 22, 903–915. doi: 10.1080/14728222.2018.1538360
Hayes, C. S., Burns, M. R., and Gilmour, S. K. (2014). Polyamine blockade promotes antitumor immunity. Oncoimmunology 3:e27360. doi: 10.4161/onci.27360
He, Q., Leitch, G. J., Visvesvara, G. S., and Wallace, S. (1996). Effects of nifedipine, metronidazole, and nitric oxide donors on spore germination and cell culture infection of the microsporidia Encephalitozoon hellem and Encephalitozoon intestinalis. Antimicrob. Agents Chemother. 40, 179–185. doi: 10.1097/00001813-199601000-00018
Higes, M., Martinhernandez, R., Botias, C., Bailon, E. G., Gonzalezporto, A. V., Barrios, L., et al. (2008). How natural infection by Nosema ceranae causes honeybee colony collapse. Environ. Microbiol. 10, 2659–2669. doi: 10.1111/j.1462-2920.2008.01687.x
Higgins, M. J., Kent, M. L., Moran, J. D., Weiss, L. M., and Dawe, S. C. (1998). Efficacy of the fumagillin analog TNP-470 for Nucleospora salmonis and Loma salmonae infections in chinook salmon Oncorhynchus tshawytscha. Dis. Aquat. Organ. 34, 45–49. doi: 10.3354/dao034045
Hoffman, P. S., Sisson, G., Croxen, M. A., Welch, K., Harman, W. D., CremadeS, N., et al. (2007). Antiparasitic drug nitazoxanide inhibits the pyruvate oxidoreductases of Helicobacter pylori, selected anaerobic bacteria and parasites, and Campylobacter jejuni. Antimicrob. Agents Chemother. 51, 868–876. doi: 10.1128/Aac.01159-06
Hooper, D. C. (2000). Mechanisms of action and resistance of older and newer fluoroquinolones. Clin. Infect. Dis. 31(Suppl. 2), S24–S28. doi: 10.1086/314056
Horton, J. (2000). Albendazole: a review of anthelmintic efficacy and safety in humans. Parasitology 121, S113–S132. doi: 10.1017/s0031182000007290
Hosamani, K. M., and Shingalapur, R. V. (2011). Synthesis of 2-mercaptobenzimidazole derivatives as potential anti-microbial and cytotoxic agents. Arch. Pharm. 344, 311–319. doi: 10.1002/ardp.200900291
James, T. Y., Pelin, A., Bonen, L., Ahrendt, S., Sain, D., Corradi, N., et al. (2013). Shared signatures of parasitism and phylogenomics unite Cryptomycota and microsporidia. Curr. Biol. 23, 1548–1553. doi: 10.1016/j.cub.2013.06.057
Jaroenlak, P., Cammer, M., Davydov, A., Sall, J., Usmani, M., Liang, F. X., et al. (2020). 3-Dimensional organization and dynamics of the microsporidian polar tube invasion machinery. PLoS Pathog. 16:e1008738. doi: 10.1371/journal.ppat.1008738
Jori, G., Fabris, C., Soncin, M., Ferro, S., Coppellotti, O., Dei, D., et al. (2006). Photodynamic therapy in the treatment of microbial infections: basic principles and perspective applications. Lasers Surg. Med. 38, 468–481. doi: 10.1002/lsm.20361
Jyothi, N. B., Patil, C. S., and Dass, C. M. S. (2005). Action of carbendazim on the development of Nosema bombycis Naegeli in silkworm Bombyx mori L. J. Appl. Entomol. 129, 205–210. doi: 10.1111/j.1439-0418.2005.00922.x
Karim, M. R., Dong, H., Yu, F., Jian, F., Zhang, L., Wang, R., et al. (2014). Genetic diversity in Enterocytozoon bieneusi isolates from dogs and cats in China: host specificity and public health implications. J. Clin. Microbiol. 52, 3297–3302. doi: 10.1128/JCM.01352-14
Killough, J. H., Magill, G. B., and Smith, R. C. (1952). The treatment of amebiasis with fumagillin. Science 115, 71–72. doi: 10.1126/science.115.2977.71
Kim, J. H., Park, J. K., and Lee, J. K. (2016). Evaluation of antimicrosporidian activity of plant extracts on Nosema ceranae. J. Apic. Res. 60, 167–178. doi: 10.1515/jas-2016-0027
Kotkova, M., Sak, B., Hlaskova, L., and Kvac, M. (2017). The course of infection caused by Encephalitozoon cuniculi genotype III in immunocompetent and immunodeficient mice. Exp. Parasitol. 182, 16–21. doi: 10.1016/j.exppara.2017.09.022
Kwok, A. K., Tong, J. M., Tang, B. S., Poon, R. W., Li, W. W., and Yuen, K. Y. (2013). Outbreak of microsporidial keratoconjunctivitis with rugby sport due to soil exposure. Eye (Lond) 27, 747–754. doi: 10.1038/eye.2013.55
Labanauskas, L. K., Brukštus, A. B., Gaidelis, P. G., Buchinskaite, V. A., and Daukšas, V. K. (2004). Synthesis and antiinflammatory activity of some new 1-acyl derivatives of 2-methylthio-5,6-diethoxybenzimidazole. Pharm. Chem. J. 34, 353–355. doi: 10.1016/j.bmcl.2011.08.061
Lacey, E. (1990). Mode of action of benzimidazoles. Parasitol. Today 6, 112–125. doi: 10.1016/0169-4758(90)90227-u
Lam, T. S., Wong, M. H., and Chuang, S. K. (2013). Microsporidial keratoconjunctivitis outbreak among athletes from Hong Kong who visited Singapore, 2012. Emerg. Infect. Dis. 19, 516–517. doi: 10.3201/eid1903.121150
Lecuit, M., Oksenhendler, E., and Sarfati, C. (1994). Use of albendazole for disseminated microsporidian infection in a patient with AIDS. Clin. Infect. Dis. 19, 332–333. doi: 10.1093/clinids/19.2.332
Leder, K., Ryan, N., Spelman, D., and Crowe, S. M. (1998). Microsporidial disease in HIV-infected patients: a report of 42 patients and review of the literature. Scand. J. Infect. Dis. 30, 331–338. doi: 10.1080/00365549850160594
Lefkove, B., Govindarajan, B., and Arbiser, J. L. (2007). Fumagillin: an anti-infective as a parent molecule for novel angiogenesis inhibitors. Expert Rev. Anti. Infect. Ther. 5, 573–579. doi: 10.1586/14787210.5.4.573
Leitch, G. J., He, Q., Wallace, S., and Visvesvara, G. S. (1993). Inhibition of the spore polar filament extrusion of the microsporidium, Encephalitozoon hellem, isolated from an AIDS patient. J. Eukaryot. Microbiol. 40, 711–717. doi: 10.1111/j.1550-7408.1993.tb04463.x
Li, J., Katiyar, S. K., Hamelin, A., Visvesvara, G. S., and Edlind, T. D. (1996). Tubulin genes from AIDS-associated microsporidia and implications for phylogeny and benzimidazole sensitivity. Mol. Biochem. Parasitol. 78, 289–295. doi: 10.1016/s0166-6851(96)02628-x
Li, W., Feng, Y., and Santin, M. (2019). Host specificity of Enterocytozoon bieneusi and public health implications. Trends Parasitol. 35, 436–451. doi: 10.1016/j.pt.2019.04.004
Li, W., Feng, Y., and Xiao, L. (2020). Diagnosis and molecular typing of Enterocytozoon bieneusi: the significant role of domestic animals in transmission of human microsporidiosis. Res. Vet. Sci. 133, 251–261. doi: 10.1016/j.rvsc.2020.09.030
Liu, H., and Mulholland, S. G. (2005). Appropriate antibiotic treatment of genitourinary infections in hospitalized patients. Am. J. Med. 118, 14–20. doi: 10.1016/j.amjmed.2005.05.009
Ma, J., Li, Y. G., Li, L. R., Yue, K. X., Liu, H. F., Wang, J. J., et al. (2020). A polyamine-based dinitro-naphthalimide conjugate as substrates for polyamine transporters preferentially accumulates in cancer cells and minimizes side effects in vitro and in vivo. Front. Chem. 8:166. doi: 10.3389/fchem.2020.00166
Marcucci, M. C. (1995). Propolis: chemical composition, biological properties and therapeutic activity. Apidologie 26, 83–99. doi: 10.1051/apido:19950202
Marton, L. J., and Pegg, A. E. (1995). Polyamines as targets for therapeutic intervention. Annu. Rev. Pharmacol. 35, 55–91. doi: 10.1146/annurev.pa.35.040195.000415
McCowen, C. M., Callender, M. E., and Lawlis, J. F. Jr. (1951). Fumagillin (H-3), a new antibiotic with amebicidal properties. Science 113, 202–203. doi: 10.1126/science.113.2930.202
McClendon, K. S., Riche, D. M., and Uwaifo, G. I. (2009). Orlistat: current status in clinical therapeutics. Expert Opin. Drug Saf. 8, 727–744. doi: 10.1517/14740330903321485
Mittleider, D., Green, L. C., Mann, V. H., Michael, S. F., Didier, E. S., and Brindley, P. J. (2002). Sequence survey of the genome of the opportunistic microsporidian pathogen, Vittaforma corneae. J. Eukaryot. Microbiol. 49, 393–401. doi: 10.1111/j.1550-7408.2002.tb00218.x
Molina, J. M., Goguel, J., Sarfati, C., Chastang, C., DesportesLivage, I., Michiels, J. F., et al. (1997). Potential efficacy of fumagillin in intestinal microsporidiosis due to Enterocytozoon bieneusi in patients with HIV infection: results of a drug screening study. AIDS 11, 1603–1610. doi: 10.1097/00002030-199713000-00009
Molina, J. M., Goguel, J., Sarfati, C., Michiels, J. F., Desportes-Livage, I., Balkan, S., et al. (2000). Trial of oral fumagillin for the treatment of intestinal microsporidiosis in patients with HIV infection. ANRS 054 Study Group. Agence Nationale de Recherche sur le SIDA. AIDS 14, 1341–1348. doi: 10.1097/00002030-200007070-00006
Molina, J. M., Tourneur, M., Sarfati, C., Chevret, S., de Gouvello, A., Gobert, J. G., et al. (2002). Fumagillin treatment of intestinal microsporidiosis. New Engl. J. Med. 346, 1963–1969. doi: 10.1056/NEJMoa012924
Morado, J. F. (2011). Protistan diseases of commercially important crabs: a review. J. Invertebr. Pathol. 106, 27–53. doi: 10.1016/j.jip.2010.09.014
Mossallam, S. F., Amer, E. I., and Diab, R. G. (2014). Potentiated anti-microsporidial activity of Lactobacillus acidophilus CH1 bacteriocin using gold nanoparticles. Exp. Parasitol. 144, 14–21. doi: 10.1016/j.exppara.2014.06.002
Muller, J., Naguleswaran, A., Muller, N., and Hemphill, A. (2008). Neospora caninum: functional inhibition of protein disulfide isomerase by the broad-spectrum anti-parasitic drug nitazoxanide and other thiazolides. Exp. Parasitol. 118, 80–88. doi: 10.1016/j.exppara.2007.06.008
Mura, A., Pusceddu, M., Theodorou, P., Angioni, A., Floris, I., Paxton, R. J., et al. (2020). Propolis consumption reduces Nosema ceranae infection of european honey bees (Apis mellifera). Insects 11:124. doi: 10.3390/insects11020124
Nakjang, S., Williams, T. A., Heinz, E., Watson, A. K., Foster, P. G., Sendra, K. M., et al. (2013). Reduction and expansion in microsporidian genome evolution: new insights from comparative genomics. Genome Biol. Evol. 5, 2285–2303. doi: 10.1093/gbe/evt184
Navarrete-Vazquez, G., Hidalgo-Figueroa, S., Torres-Piedra, M., Vergara-Galicia, J., Rivera-Leyva, J. C., Estrada-Soto, S., et al. (2010). Synthesis, vasorelaxant activity and antihypertensive effect of benzo[d]imidazole derivatives. Bioorgan. Med. Chem. 18, 3985–3991. doi: 10.1016/j.bmc.2010.04.027
Orenstein, J. M. (1991). Microsporidiosis in the acquired immunodeficiency syndrome. J. Parasitol. 77, 843–864. doi: 10.2307/3282733
Parker, S. K., Barkley, R. M., Rino, J. G., and Vasil, M. L. (2009). Mycobacterium tuberculosis Rv3802c encodes a phospholipase/thioesterase and is inhibited by the antimycobacterial agent tetrahydrolipstatin. PLoS One 4:e4281. doi: 10.1371/journal.pone.0004281
Pasharawipas, T., and Flegel, T. W. (1994). A specific DNA probe to identify the intermediate host of a common microsporidian parasite of Penaeus merguiensis and P. monodon. Asian Fish. Sci. 7, 157–167.
Peyretaillade, E., Biderre, C., Peyret, P., Duffieux, F., Metenier, G., Gouy, M., et al. (1998). Microsporidian Encephalitozoon cuniculi, a unicellular eukaryote with an unusual chromosomal dispersion of ribosomal genes and a LSU rRNA reduced to the universal core. Nucleic Acids Res. 26, 3513–3520. doi: 10.1093/nar/26.15.3513
Pleshinger, J., and Weidner, E. (1985). The microsporidian spore invasion tube. IV. Discharge activation begins with pH-triggered Ca2+ influx. J. Cell Biol. 100, 1834–1838. doi: 10.1083/jcb.100.6.1834
Pombert, J. F., Selman, M., Burki, F., Bardell, F. T., Farinelli, L., Solter, L. F., et al. (2012). Gain and loss of multiple functionally related, horizontally transferred genes in the reduced genomes of two microsporidian parasites. Proc. Natl. Acad. Sci. U.S.A. 109, 12638–12643. doi: 10.1073/pnas.1205020109
Porrini, M. P., Audisio, M. C., Sabate, D. C., Ibarguren, C., Medici, S. K., Sarlo, E. G., et al. (2010). Effect of bacterial metabolites on microsporidian Nosema ceranae and on its host Apis mellifera. Parasitol. Res. 107, 381–388. doi: 10.1007/s00436-010-1875-1
Porrini, M. P., Fernández, N. J., Garrido, P. M., Gende, L. B., Medici, S. K., and Eguaras, M. J. (2011). In vivo evaluation of antiparasitic activity of plant extracts on Nosema ceranae (Microsporidia). Apidologie 42, 700–707. doi: 10.1007/s13592-011-0076-y
Prasertsri, S., Limsuwan, C., and Chuchird, N. (2009). Effects of microsporidian (Thelohania) infection on the growth and histopathological changes in pond-reared pacific white shrimp (Litopenaeus vannamei). Kasetsart J. Nat. Sci. 43, 680–688.
Ptaszynska, A. A., Borsuk, G., Zdybicka-Barabas, A., Cytrynska, M., and Malek, W. (2016). Are commercial probiotics and prebiotics effective in the treatment and prevention of honeybee nosemosis C? Parasitol. Res. 115, 397–406. doi: 10.1007/s00436-015-4761-z
Ptaszynska, A. A., Trytek, M., Borsuk, G., Buczek, K., Rybicka-Jasinska, K., and Gryko, D. (2018). Porphyrins inactivate Nosema spp. microsporidia. Sci. Rep. 8:5523. doi: 10.1038/s41598-018-23678-8
Roncero, C. (2002). The genetic complexity of chitin synthesis in fungi. Curr. Genet. 41, 367–378. doi: 10.1007/s00294-002-0318-7
Rossignol, J. F., and Maisonneuve, H. (1984). Nitazoxanide in the treatment of Taenia saginata and Hymenolepis nana infections. Am. J. Trop. Med. Hyg. 33, 511–512. doi: 10.4269/ajtmh.1984.33.511
Roussel, M., Villay, A., Delbac, F., Michaud, P., Laroche, C., Roriza, D., et al. (2015). Antimicrosporidian activity of sulphated polysaccharides from algae and their potential to control honeybee nosemosis. Carbohydr. Polym. 133, 213–220. doi: 10.1016/j.carbpol.2015.07.022
Ruan, Y. F., Xu, X. F., He, Q., Li, L., Guo, J. R., Bao, J. L., et al. (2021). The largest meta-analysis on the global prevalence of microsporidia in mammals, avian and water provides insights into the epidemic features of these ubiquitous pathogens. Parasite. Vector. 14:186. doi: 10.1186/s13071-021-04700-x
Saffo, Z., and Mirza, N. (2019). Successful treatment of Enterocytozoon bieneusi gastrointestinal infection with nitazoxanide in a immunocompetent patient. IDCases 18:e00586. doi: 10.1016/j.idcr.2019.e00586
Sak, B., Brdickova, K., Holubova, N., Kvetonova, D., Hlaskova, L., and Kvac, M. (2020). Encephalitozoon cuniculi genotype III evinces a resistance to albendazole treatment in both immunodeficient and immunocompetent mice. Antimicrob. Agents Chemother. 64, e58–e20. doi: 10.1128/AAC.00058-20
Sak, B., Jandova, A., Dolezal, K., Kvac, M., Kvetonova, D., Hlaskova, L., et al. (2017). Effects of selected Indonesian plant extracts on E. cuniculi infection in vivo. Exp. Parasitol. 181, 94–101. doi: 10.1016/j.exppara.2017.07.014
Sakr, A. S., Samei, A. H. A., and Soliman, E. M. (2004). Exploring hepatotoxicity of benomyl: histological and histochemical study on albino rats. J. Med. Sci. 4, 77–83. doi: 10.3923/jms.2004.77.83
Salminen, M. K., Tynkkynen, S., Rautelin, H., Poussa, T., Saxelin, M., Ristola, M., et al. (2004). The efficacy and safety of probiotic Lactobacillus rhamnosus GG on prolonged, noninfectious diarrhea in HIV patients on antiretroviral therapy: a randomized, placebo-controlled, crossover study. HIV Clin. Trials 5, 183–191. doi: 10.1310/6f83-N39q-9ppp-Lmvv
Shadduck, J. A., Meccoli, R. A., Davis, R., and Font, R. L. (1990). Isolation of a microsporidian from a human patient. J. Infect. Dis. 162, 773–776. doi: 10.1093/infdis/162.3.773
Silva, D. R., Sardi, J. D. O., Pitangui, N. D., Roque, S. M., da Silva, A. C. B., and Rosalen, P. L. (2020). Probiotics as an alternative antimicrobial therapy: current reality and future directions. J. Funct. Foods 73:104080. doi: 10.1016/j.jff.2020.104080
Simmons, A. (2002). Clinical manifestations and treatment considerations of herpes simplex virus infection. J. Infect. Dis. 186, S71–S77. doi: 10.1086/342967
Sin, N., Meng, L., Wang, M. Q., Wen, J. J., Bornmann, W. G., and Crews, C. M. (1997). The anti-angiogenic agent fumagillin covalently binds and inhibits the methionine aminopeptidase, MetAP-2. Proc. Natl. Acad. Sci. U.S.A 94, 6099–6103. doi: 10.1073/pnas.94.12.6099
Sobottka, I., Bartscht, K., Schafer, P., Weitzel, T., Schottelius, J., Kock, N., et al. (2002). In vitro activity of polyoxin D and nikkomycin Z against Encephalitozoon cuniculi. Parasitol. Res. 88, 451–453. doi: 10.1007/s00436-002-0592-9
Solter, L. F., and Maddox, J. V. (1998). Physiological host specificity of Microsporidia as an indicator of ecological host specificity. J. Invertebr. Pathol. 71, 207–216. doi: 10.1006/jipa.1997.4740
Sulaiman, I. M., Fayer, R., Lal, A. A., Trout, J. M., Schaefer, F. W., and Xiao, L. H. (2003). Molecular characterization of microsporidia indicates that wild mammals harbor host-adapted Enterocytozoon spp. as well as human-pathogenic Enterocytozoon bieneusi. Appl. Environ. Microbiol. 69, 4495–4501. doi: 10.1128/Aem.69.8.4495-4501.2003
Sutrave, G., Maundrell, A., Keighley, C., Jennings, Z., Brammah, S., Wang, M. X., et al. (2018). Anncaliia algerae microsporidial myositis, New South Wales, Australia. Emerg. Infect. Dis. 24, 1528–1531. doi: 10.3201/eid2408.172002
Suwannapong, G., Maksong, S., Phainchajoen, M., Benbow, M. E., and Mayack, C. (2018). Survival and health improvement of Nosema infected Apis florea (Hymenoptera: apidae) bees after treatment with propolis extract. J. Asia Pac. Entomol. 21, 437–444. doi: 10.1016/j.aspen.2018.02.006
Tang, K. F. J., Pantoja, C. R., Redman, R. M., Han, J. E., Tran, L. H., and Lightner, D. V. (2015). Development of in situ hybridization and PCR assays for the detection of Enterocytozoon hepatopenaei (EHP), a microsporidian parasite infecting penaeid shrimp. J. Invertebr. Pathol. 130, 37–41. doi: 10.1016/j.jip.2015.06.009
Thembo, K. M., Vismer, H. F., Nyazema, N. Z., Gelderblom, W. C., and Katerere, D. R. (2010). Antifungal activity of four weedy plant extracts against selected mycotoxigenic fungi. J. Appl. Microbiol. 109, 1479–1486. doi: 10.1111/j.1365-2672.2010.04776.x
Thitamadee, S., Prachumwat, A., Srisala, J., Jaroenlak, P., Salachan, P. V., Sritunyalucksana, K., et al. (2016). Review of current disease threats for cultivated penaeid shrimp in Asia. Aquaculture 452, 69–87. doi: 10.1016/j.aquaculture.2015.10.028
Tokarev, Y. S., Huang, W. F., Solter, L. F., Malysh, J. M., Becnel, J. J., and Vossbrinck, C. R. (2020). A formal redefinition of the genera Nosema and Vairimorpha (Microsporidia: nosematidae) and reassignment of species based on molecular phylogenetics. J. Invertebr. Pathol. 169:107279. doi: 10.1016/j.jip.2019.107279
Tremoulet, A. H., Avila-Aguero, M. L., Paris, M. M., Canas-Coto, A., Ulloa-Gutierrez, R., and Faingezicht, I. (2004). Albendazole therapy for microsporidium diarrhea in immunocompetent Costa Rican children. Pediatr. Infect. Dis. J. 23, 915–918. doi: 10.1097/01.inf.0000141724.06556.f9
Vavra, J., and Lukes, J. (2013). Microsporidia and ‘the art of living together’. Adv. Parasitol. 82, 253–319. doi: 10.1016/B978-0-12-407706-5.00004-6
Wan, Q., Lin, Y. C., Mao, Y. X., Yang, Y. Q., Li, Q., Zhang, S. W., et al. (2016). High prevalence and widespread distribution of zoonotic Enterocytozoon bieneusi genotypes in swine in northeast China: implications for public health. J. Eukaryot. Microbiol. 63, 162–170. doi: 10.1111/jeu.12264
Wang, W. Y., Chu, H. S., Lin, P. C., Lee, T. F., Kuo, K. T., Hsueh, P. R., et al. (2018). Outbreak of microsporidial keratoconjunctivitis associated with water contamination in swimming pools in Taiwan. Am. J. Ophthalmol. 194, 101–109. doi: 10.1016/j.ajo.2018.07.019
Watts, M. R., Chan, R. C. F., Cheong, E. Y. L., Brammah, S., Clezy, K. R., Tong, C. W., et al. (2014). Anncaliia algerae microsporidial myositis. Emerg. Infect. Dis. 20, 185–191. doi: 10.3201/eid2002.131126
Weber, R., Bryan, R. T., Schwartz, D. A., and Owen, R. L. (1994). Human microsporidial infections. Clin. Microbiol. Rev. 7, 426–461. doi: 10.1128/CMR.7.4.426
Weber, R., Deplazes, P., Flepp, M., Mathis, A., Baumann, R., Sauer, B., et al. (1997). Cerebral microsporidiosis due to Encephalitozoon cuniculi in a patient with human immunodeficiency virus infection. New. Engl. J. Med. 336, 474–478. doi: 10.1056/NEJM199702133360704
Weidner, E. (1976). The microsporidian spore invasion tube. The ultrastructure, isolation, and characterization of the protein comprising the tube. J. Cell Biol. 71, 23–34. doi: 10.1083/jcb.71.1.23
Weidner, E. (1982). The microsporidian spore invasion tube. III. Tube extrusion and assembly. J. Cell Biol. 93, 976–979. doi: 10.1083/jcb.93.3.976
Weidner, E., and Byrd, W. (1982). The microsporidian spore invasion tube. II. Role of calcium in the activation of invasion tube discharge. J. Cell Biol. 93, 970–975. doi: 10.1083/jcb.93.3.970
Weiss, L. M., and Keohane, E. M. (2010). Microsporidia at the Turn of the Millenium: Raleigh 1999. J. Eukaryot. Microbiol. 46, 3S–5S. doi: 10.1111/j.1550-7408.1999.tb06055.x
Williams, B. A., Hirt, R. P., Lucocq, J. M., and Embley, T. M. (2002). A mitochondrial remnant in the microsporidian Trachipleistophora hominis. Nature 418, 865–869. doi: 10.1038/nature00949
Williams, G. R., Sampson, M. A., Shutler, D., and Rogers, R. E. L. (2008). Does fumagillin control the recently detected invasive parasite Nosema ceranae in western honey bees (Apis mellifera)? J. Invertebr. Pathol. 99, 342–344. doi: 10.1016/j.jip.2008.04.005
Williams, G. R., Shutler, D., Burgher-MacLellan, K. L., and Rogers, R. E. (2014). Infra-population and community dynamics of the parasites Nosema apis and Nosema ceranae, and consequences for honey bee (Apis mellifera) hosts. PLoS One 9:e99465. doi: 10.1371/journal.pone.0099465
Yang, P. Y., Wang, M., Liu, K., Ngai, M. H., Sheriff, O., Lear, M. J., et al. (2012). Parasite-based screening and proteome profiling reveal orlistat, an FDA-approved drug, as a potential anti Trypanosoma brucei agent. Chemistry 18, 8403–8413. doi: 10.1002/chem.201200482
Yarlett, N., and Bacchi, C. J. (1988). Effect of DL-alpha-difluoromethylornithine on polyamine synthesis and interconversion in Trichomonas vaginalis grown in a semi-defined medium. Mol. Biochem. Parasitol. 31, 1–10. doi: 10.1016/0166-6851(88)90139-9
Yoshida, M., Fuchigami, M., Nagao, T., Okabe, H., Matsunaga, K., Takata, J., et al. (2005). Antiproliferative constituents from Umbelliferae plants VII. Active triterpenes and rosmarinic acid from Centella asiatica. Biol. Pharm. Bull. 28, 173–175. doi: 10.1248/bpb.28.173
Zhao, W., Zhou, H. H., Ren, G. X., Qiang, Y., Huang, H. C., Lu, G., et al. (2021). Occurrence and potentially zoonotic genotypes of Enterocytozoon bieneusi in wild rhesus macaques (Macaca mulatta) living in Nanwan Monkey Island, Hainan, China: a public health concern. BMC Vet. Res. 17:213. doi: 10.1186/s12917-021-02916-8
Keywords: microsporidia, microsporidiosis, therapeutic targets, fumagillin, chitin synthase
Citation: Wei J, Fei Z, Pan G, Weiss LM and Zhou Z (2022) Current Therapy and Therapeutic Targets for Microsporidiosis. Front. Microbiol. 13:835390. doi: 10.3389/fmicb.2022.835390
Received: 14 December 2021; Accepted: 14 February 2022;
Published: 09 March 2022.
Edited by:
Hesham M. Al-Mekhlafi, Jazan University, Saudi ArabiaReviewed by:
Francoise Botterel, Assistance Publique - Hôpitaux de Paris, Université Paris Est Creteil, FranceCopyright © 2022 Wei, Fei, Pan, Weiss and Zhou. This is an open-access article distributed under the terms of the Creative Commons Attribution License (CC BY). The use, distribution or reproduction in other forums is permitted, provided the original author(s) and the copyright owner(s) are credited and that the original publication in this journal is cited, in accordance with accepted academic practice. No use, distribution or reproduction is permitted which does not comply with these terms.
*Correspondence: Zeyang Zhou, enl6aG91QHN3dS5lZHUuY24=; Louis M. Weiss, bG91aXMud2Vpc3NAZWluc3RlaW5tZWQuZWR1
Disclaimer: All claims expressed in this article are solely those of the authors and do not necessarily represent those of their affiliated organizations, or those of the publisher, the editors and the reviewers. Any product that may be evaluated in this article or claim that may be made by its manufacturer is not guaranteed or endorsed by the publisher.
Research integrity at Frontiers
Learn more about the work of our research integrity team to safeguard the quality of each article we publish.