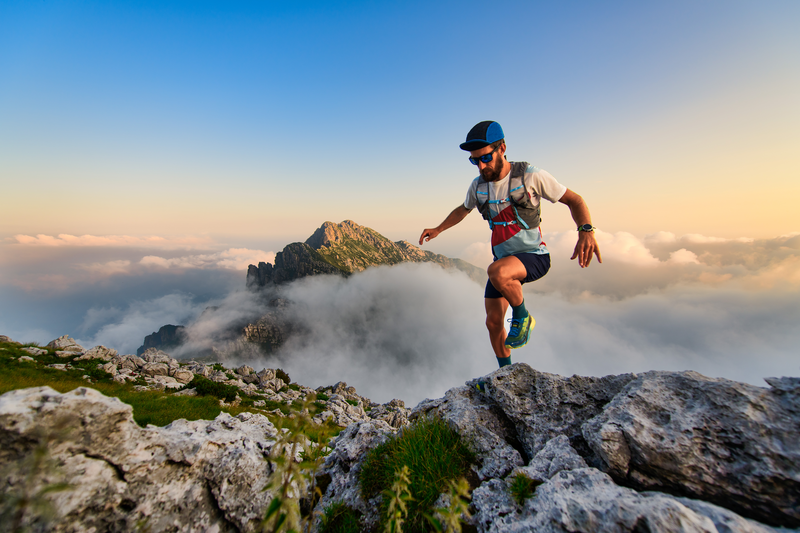
94% of researchers rate our articles as excellent or good
Learn more about the work of our research integrity team to safeguard the quality of each article we publish.
Find out more
ORIGINAL RESEARCH article
Front. Microbiol. , 21 March 2022
Sec. Infectious Agents and Disease
Volume 13 - 2022 | https://doi.org/10.3389/fmicb.2022.833520
This article is part of the Research Topic In Vivo and In Vitro Control of Infectious Parasitic Diseases View all 5 articles
Surra is a parasitic disease caused by the eukaryotic, unicellular hemoprotozoan, Trypanosoma evansi, which affects the development of animal production and is widespread among both domestic and wild animals. As such, in this research, we studied the antiparasitic activity and the ameliorative impact of Eucalyptus camaldulensis leaf extracts (ELE) against T. evansi-induced brain injury and spleen immune response in mice. As a result, we found that ELE decreased the amount of trypanosomes in the blood and improved the weight loss caused by infection. In addition, ELE reduced the parasite-induced brain and spleen histopathological damage. The parasite affected the levels of dopamine and serotonin, but after treatment with ELE, their concentrations significantly decreased to 154 ± 7 and 258 ± 11 μg/g, respectively. We clearly observed the antioxidant activity of ELE because of its ability to increase the induced change in the brain’s total antioxidant capacity and the nitric oxide level. The histopathological changes in the spleen also improved after ELE application. Based on our results, we concluded that ELE possesses antitrypanosomal antioxidant and protective effects in the brains of mice infected with T. evansi. Additional phytochemical screening and molecular studies are required to understand the mechanism underlying the effect of ELE.
Surra is a widespread hemoprotozoan disease caused by the infection of domestic and wild animals with the single-cellular eukaryote Trypanosoma evansi (Sazmand et al., 2022). Trypanosomosis poses a barrier to the development of animal production in many tropical regions of the world. The disease pattern generated by T. evansi is similar to that of T. brucei gambiense, which causes sleep sickness in humans. The pathogenicity of T. evansi varies across strains and animal species (De Menezes et al., 2004). The tsetse fly is considered the vector transmitting trypanosomes to the host, and trypanosomosis leads to the appearance of severe weakness and anemia, and the impairment of the nervous system (Desquesnes et al., 2013; Dkhil et al., 2021a). Human infection with T. evansi can also occur (Joshi et al., 2005; Powar et al., 2006). Symptoms of trypanosomosis can vary with animal species (Degneh et al., 2017). The increased parasite levels in the blood of the host disturb the level of neurotransmitters. Dkhil et al. (2021a) reported increased dopamine and serotonin levels in the brains of mice infected with T. evansi. In addition, epinephrine has been reported to be increased during T. congolense and T. lewisi infections (Sanchez, 1973; Kalu and Haruna, 1985).
Due to parasite resistance to currently used antitrypanosomal medications, scientists are looking for effective natural-resource-based therapies, such as natural products, to combat the infection.
Eucalyptus camaldulensis is a species of Eucalyptus that belongs to the Myrtaceae family and is known for its biologically active chemicals, such as alkaloids and flavonoids (Ghareeb et al., 2018). Extracts from this plant were used to treat animals suffering from malaria (Anigboro et al., 2020) and animal trypanosomosis (Kabiru et al., 2013), and it was reported to possess antioxidant properties (Shakibaie et al., 2021). In vitro studies carried out by Habila et al. (2010) showed that E. camaldulensis oil was effective against T. evansi.
To the best our knowledge, no reports have been published on the brain-protective effect and spleen-immune modulation of E. camaldulensis during T. evansi infection. As such, during trypanosomosis, we examined antioxidant activity in the brain as well as the status of neurotransmitters in mice. We also examined any morphological and histological alterations in the spleen.
We collected E. camaldulensis leaves from Qassim, Saudi Arabia. The samples were authenticated by a specialist from the herbarium of Helwan University. The leaves were cleaned, dried, and powdered. We macerated the obtained powder (100 g) by mixing it at 4°C for 24 h in 70% methanol. We filtered the obtained extract and then dried it using a vacuum evaporator (Lubbad et al., 2015). We used distilled water to dissolve the residue.
For ELE analysis, we used a Nicolet 6700 Fourier-transform infrared spectroscopy (FT-IR) optical spectrometer from Thermo Scientific (Waltham, MA, United States). We mixed the powder of the extract (10 mg) with 100 mg of potassium bromide powder (1:99 wt%) to obtain a translucent sample disk that we then loaded into an FTIR spectroscope with a scan range of 400–4000 cm–1. The chemical bonds in a molecule can be determined by interpreting the infrared absorption spectra (Pakkirisamy et al., 2017).
Total flavonoid content was quantified using an aluminum chloride colorimetric assay, and total phenolic content was calculated using the Folin-Ciocalteu technique described by Lin and Tang (2007). The flavonoids in ELE were estimated using a calibration curve of quercetin, a standard flavonoid. A gallic acid standard curve was used to determine the phenolic concentration.
We bred 25 C57Bl/6 female mice (11 ± 2 weeks old) under specific-pathogen-free conditions. They were housed in plastic cages under standard conditions of illumination with a 12-h light/dark cycle at 25 ± 1°C. Animals received a balanced diet and water ad libitum.
We maintained T. evansi in mice by weekly passage with infected blood. The mice were infected intraperitoneally with 103 trypanosomes of T. evansi (Dkhil et al., 2019). We calculated the mean number of trypanosomes/5 fields.
The animals in the first group received only water by oral gavage, whereas the second group received ELE for 4 days (100 mg/kg). Three groups of animals (third to fifth) were infected and, 1 h later, the fourth group received 100 mg/kg ELE (Anigboro et al., 2020). The fifth group was treated with 1 mg/kg Cymelarsan (Merial, Lyon, France) (Hagos et al., 2010). We sampled the brains of the mice on day 4 post-infection after killing the animals by CO2 asphyxiation.
We dissected the brains of the mice, which were then washed twice in ice-cold 50 mM Tris-HCl to remove any blood. Subsequently, we carefully divided longitudinally each brain into two halves: we used the first half for histopathological analysis, and we weighed and immediately homogenized the second half in an ice-cold medium containing 50 mM Tris-HCl (pH 7.4) to yield a 10% (w/v) homogenate. We centrifuged the supernatants obtained from the homogenates at 1,000 × g for 10 min at 4°C to determine the concentrations of nitric oxide (NO), dopamine, and serotonin. We calculated body and spleen weights on day 4 p.i. We determined spleen index as described by Dkhil (2009) by calculating the ratio of spleen weight per milligram of mouse to the mouse weight per gram. The project was approved (approval No., HU2021/Z/AD/1121-01) by the Department of Zoology, Faculty of Science, Helwan University’s Committee of Research Ethics for Laboratory Animal Care.
We fixed the brains and spleens of infected and non-infected mice in 10% formalin. After fixation, the specimens were dehydrated, embedded in wax, and sectioned into 5-μm-thick slices. Finally, we stained the sections with hematoxylin and eosin and then examined them. Spleen histology was semiquantified as described by Giamarellos-Bourboulis et al. (2006).
According to Tsakiris et al. (2004), we prepared the brain homogenate to measure the total antioxidant capacity (TAC) using commercial kits (Biodiagnostic, Egypt) by the colorimetric method described in Koracevic et al. (2001). Additionally, we estimated the NO level according to Green et al. (1982).
We weighed the brain and measured the levels of dopamine and serotonin as described by Ciarlone (1978).
We evaluated multiple variable comparisons using one-way ANOVA, and the data are presented as the mean standard error of the mean. We compared the significance between the classes using Duncan’s test. Statistical significance was set at p ≤ 0.05.
The FT-IR spectra for ELE are shown in Figure 1. We extracted Table 1 from the IR spectrum table.1 Our analysis of ELE using FTIR showed strong peaks at 1,712.46, 1,615.19, 1,514.50, 1,225.05, 1,042.56, 871.80, 766.97, and 603.51 cm–1. Medium peaks appeared at 3,399.50, 2,930.61, 1,450.66, and 819.64 cm–1. The expected classes of compounds were aliphatic primary amines, alkanes, carboxylic acids, α,β-unsaturated ketones, nitro compounds, sulfonamides, alkyl aryl ethers, anhydrides, and halo compounds (Table 1).
Figure 1. Phytochemical screening by FTIR spectroscopic analysis of Eucalyptus camaldulensis leaf extracts.
Eucalyptus camaldulensis leaf extracts has a total flavonoid and phenol content of 3.2 QE/g and 29.8 GAE/mg, respectively.
Eucalyptus camaldulensis leaf extracts (100 mg/kg) reduced the number of trypanosomes to approximately 57.2% (Table 2) on day 5 post-infection, whereas animals treated with 200 and 300 mg/kg ELE died on day 4 due to infection (Table 2). The parasite decreased the weight of the mice, but ELE increased their weight after treatment (Figure 2).
Table 2. Effect of Eucalyptus camaldulensis on the survival and trypanosomes number per 5 fields on day 5 post-infection.
Figure 2. Effect of E. camaldulensis extract on body weight of mice infected with T. evansi. *p < 0.01, significance against the control group. #p < 0.01, significance against the infected group.
Trypanosoma evansi infection generated significant neurohistopathological alterations in Purkinje cells, including inflammation, bleeding, and structural abnormalities. In addition, infection reduced the number of cells in the Purkinje layer (Figure 3). Because of the parasite, dopamine and serotonin levels were much higher, but after treatment with ELE, the levels of these neurotransmitters significantly decreased (Figures 4, 5).
Figure 3. E. camaldulensis induced change in spleen index of mice infected with T. evansi. *p ≤ 0.01, significance against the control group. #p ≤ 0.01, significance against the infected group.
Figure 4. E. camaldulensis improves the brain histopathology after infection with T. evansi. Normal structure of control cerebellum (A) and ELE-treated (B) mice. (C) Infected cerebellum of mice with injured Purkinje cells and dilated sinusoids between the molecular layers. (D) ELE-treated infected cerebellum with improved structure. (E) Drug-treated cerebellum with improved structure. Scale bar = 25 μm.
Figure 5. Change in brain dopamine of mice infected with T. evansi and treated with E. camaldulensis. *, significance against control group at p ≤ 0.01. #, significance against infected group at p ≤ 0.01.
We found that the infection decreased the TAC, but treatment with ELE ameliorated this decrease (Figure 6). Moreover, the level of NO decreased after treatment of the infected mice with ELE (Figure 7).
Figure 6. E. camaldulensis induced change in the level of Serotonin of mice infected with T. evansi. *, significance against control group at p ≤ 0.01. #, significance against infected group at p ≤ 0.01.
Figure 7. Effect of E. camaldulensis on brain total antioxidant capacity of mice infected with T. evansi. *, significance against control group at p ≤ 0.01. #, significance against infected group at p ≤ 0.01.
The infection caused marked changes in the spleen. Splenomegaly was pronounced when the spleen index increased approximately twofold (Figure 8). Our examination of spleen sections showed that the architecture of the spleen’s red and white pulps changed after infection (Figure 9). ELE treatment improved the induced histopathological changes. This was observed through the calculated spleen histology score, which indicated an ameliorative effect similar to that of the reference drug (Figure 10).
Figure 8. Brain nitric oxide level after treatment of T. evansi-infected mice with E. camaldulensis. *, significance against control group at p ≤ 0.01. #, significance against infected group at p ≤ 0.01.
Figure 9. Spleen histology of mice. Normal structure of control spleen (A) and ELE-treated (B) mice. (C) Infected spleen of mice with dilated and fused white and red pulps and increased apoptotic bodies. (D) ELE-treated infected spleen with improved structure. (E) Drug-treated spleen with improved structure. Scale bar = 50 μm.
Figure 10. Spleen histology score of mice. *, significance against control group at p ≤ 0.01. #, significance against infected group at p ≤ 0.01.
Trypanosomosis continues to be a serious health issue in both humans and animals, characterized by a complicated relationship between the host’s responses and the parasite’s actions, particularly the parasite’s generation of oxidative stress. This oxidative damage caused by parasite activity is considered to be the result of the physiological, histological, and biochemical changes that occur during infection.
Eucalyptus camaldulensis leaf extracts contains phenolic and flavonoid active compounds capable of suppressing the number of trypanosomes in mouse blood. Similar compound classes present in other plants, such as Indigofera oblongifolia, were found to have antitrypanosomal effects (Dkhil et al., 2019). Due to the presence of active phenolic compounds, E. camaldulensis shows significant antibacterial and antischistosomal activity (Ghareeb et al., 2018). The histopathological effects on the brain were induced by the rapid penetration of the trypanosome and its metabolites (Biswas et al., 2010), causing inflammation (Berlin et al., 2009). Few investigations on cerebral trypanosomosis have been conducted in Surra; however, in our prior work, we described the behavioral and histological effects of T. evansi in mice (Dkhil et al., 2021a). In addition to the histopathological effects on the brain, T. evansi causes liver and spleen injury (Biswas et al., 2010). In this study, splenomegaly with histopathological damage was pronounced, especially in the disorganization of white and red pulps.
Due to the enrichment of ELE with active compounds and the antioxidant properties of these compounds, both brain and spleen histology improved after treatment of T. evansi-infected mice.
In this study, we found that brain dopamine and serotonin levels increased after infection. The disturbance in dopamine and serotonin due to infection was also investigated by Amole et al. (1989) after infection with T. brucei brucei, which was attributed to the production of reactive oxygen species, which cause cellular damage and lead to the pathogenesis of the disease (Gupta et al., 2009).
Nitric oxide is measured for assessing immunological responses and oxidative stress levels (Chung et al., 2022). Activated macrophages create NO, which is harmful to a host of diseases, including Trypanosoma (Gazzinelli et al., 1992). Dkhil et al. (2021b) reported brain oxidative damage due to an increase in NO levels in mice infected with T. evansi. Increased NO production was reported in the brains of T. brucei-infected mice, suggesting that NO and its derivatives’ cytotoxicity may cause brain abnormalities (Keita et al., 2000). In our study, we found that ELE acted as an antioxidant agent that reduced oxidative damage in the brain (Nasr et al., 2019).
The spleen is the body’s largest secondary lymphoid organ, and it performs a variety of immunological processes in addition to its hematopoiesis and erythrocyte clearing functions. In this study, the increased spleen index and splenomegaly were evident during infection due to splenic cell hyperplasia and disease progression (Dkhil et al., 2019). Both spleen white and red pulps increase in size due to the response of macrophage activation, which occurs in the presence of the parasite. Furthermore, trypanosomes release toxins that disrupt organs and cause cell injury (Bal et al., 2012). This has an impact on the structure and function of the spleen. Similar improvements to those produced by ELE treatment after infection with T. evansi in the spleen in this study were reported in mice treated with I. oblongifolia extract (Dkhil et al., 2019).
The spleen is a key site for T-cell activation and B-cell differentiation. These activities are controlled in the brain (Whalley, 2020). Herr et al. (2017) reported that T-cell activation could be mediated by serotonin. In addition, Matt and Gaskill (2020) reported that dopamine plays an important role in immune function regulation. In this study, the neurotransmitters dopamine and serotonin increased after infection, leading to spleen cell activation. Because of the presence of phytoactive substances, ELE was able to regulate these alterations. We concluded that ELE has antitrypanosomal and immune-neuroprotective activities against T. evansi infection. However, further investigation into the molecular mechanisms underlying the neuroprotective and spleen response capabilities of E. camaldulensis is required.
The raw data supporting the conclusions of this article will be made available by the authors, without undue reservation.
The animal study was reviewed and approved (Approval No., HU2021/Z/AD/1121-01) by the Department of Zoology, Faculty of Science, Helwan University’s Committee of Research Ethics for Laboratory Animal Care. Written informed consent was obtained from the owners for the participation of their animals in this study.
MD, EA-S, RA-G, and SA-Q designed the study. MD, EA-S, RA-G, and FT carried out the experiments and analyzed the data. All authors wrote and revised the manuscript.
This study was supported by Princess Nourah Bint Abdulrahman University Research Supporting Project number (PNURSP2022R96), Princess Nourah Bint Abdulrahman University, Riyadh, Saudi Arabia, and was also supported by Researchers Supporting Project (RSP2021/03), King Saud University, Riyadh, Saudi Arabia.
The authors declare that the research was conducted in the absence of any commercial or financial relationships that could be construed as a potential conflict of interest.
All claims expressed in this article are solely those of the authors and do not necessarily represent those of their affiliated organizations, or those of the publisher, the editors and the reviewers. Any product that may be evaluated in this article, or claim that may be made by its manufacturer, is not guaranteed or endorsed by the publisher.
Amole, B., Sharpless, N., Wittner, M., and Tanowitz, H. B. (1989). Neurochemical measurements in the brains of mice infected with Trypanosoma brucei Witter (TREU 667). Ann. Trop. Med. Parasitol. 83, 225–232.
Anigboro, A. A., Avwioroko, O. J., and Cholu, C. O. (2020). Phytochemical Constituents, Antimalarial Efficacy, and protective effect of Eucalyptus camaldulensis aqueous leaf extract in Plasmodium berghei-infected mce. Prev. Nutr. Food Sci. 25, 58–64. doi: 10.3746/pnf.2020.25.1.58
Bal, M. S., Singla, L. D., Kumar, H., Vasudev, A., Gupta, K., and Juyal, P. D. (2012). Pathological studies on experimental Trypanosoma evansi infection in Swiss albino mice. J. Parasite. Dis. 36, 260–264. doi: 10.1007/s12639-012-0120-5
Berlin, D., Loeb, E., and Baneth, G. (2009). Disseminated central nervous system disease caused by Trypanosoma evansi in a horse. Vet. Parasitol. 161, 316–319. doi: 10.1016/j.vetpar.2009.01.030
Biswas, D., Choudhury, A., and Misra, K. K. (2010). Histopathology of Trypanosoma (Trypanozoon) evansi infection in bandicoot rat. II Brain and choroid plexus. Proc. Zool. Soc. 63, 27–37. doi: 10.1007/s12595-010-0004-6
Chung, C. W., Liao, B. W., Huang, S. W., Chiou, S. J., Chang, C. H., Lin, S. J., et al. (2022). Magnetic Responsive Release of Nitric Oxide from an MOF-Derived Fe3O4@PLGA Microsphere for the Treatment of Bacteria-Infected Cutaneous Wound. ACS Appl. Mater. Interfaces 5, 6343–6357. doi: 10.1021/acsami.1c20802
Ciarlone, A. E. (1978). Further modification of a fluorometric method for analyzing brain amines. Microchem. J. 23, 9-12. doi: 10.1016/0026-265X(78)90034-6
De Menezes, V. T., Queiroz, A. O., Gomes, M. A., Marques, M. A., and Jansen, A. M. (2004). Trypanosoma evansi in inbred and Swiss−Webster mice: distinct aspects of pathogenesis. Parasitol. Res. 94, 193–200. doi: 10.1007/s00436-004-1207-4
Degneh, E., Shibeshi, W., Terefe, G., Asres, K., and Ashenafi, H. (2017). Bovine trypanosomosis: changes in parasitemia and packed cell volume in dry and wet seasons at Gidami District, Oromia Regional State, western Ethiopia. Acta Vet. Scand. 59:59. doi: 10.1186/s13028-017-0327-7
Desquesnes, M., Holzmuller, P., Lai, D. H., Dargantes, A., Lun, Z. R., and Jittaplapong, S. (2013). Trypanosoma evansi and surra: a review and perspectives on origin, history, distribution, taxonomy, morphology, hosts, and pathogenic effects. BioMed. Res. Int. 2013:194176. doi: 10.1155/2013/194176
Dkhil, M. A., Thagfan, F. A., Al-Shaebi, E. M., Maodaa, S. N., Abdel-Gaber, R., Hafiz, T. A., and Al-Quraishy, S. (2021a). Brain oxidative status and behavioral response of mice infected with Trypanosoma evansi. J. King Saud. Univ. Sci. 33:101544. doi: 10.1016/j.jksus.2021.101544
Dkhil, M. A., Al-Shaebi, E. M., Alazzouni, A. S., Al-Quraishy, S., and Khalil, M. (2021b). Murine liver response to Allium sativum treatment during infection induced-trypanosomiasis. Saudi J. Biol. Sci. 28, 3270–3274. doi: 10.1016/j.sjbs.2021.02.067
Dkhil, M. A. (2009). Apoptotic changes induced in mice splenic tissue due to malaria infection. J. Microbiol. Immunol. Infect. 42, 13–18.
Dkhil, M. A., Hafiz, T. A., Thagfan, F. A., Al-Shaebi, E. M., Mubaraki, M. A., Khalil, M., et al. (2019). Indigofera oblongifolia protects against trypanosomiasis-inducedspleen injury. J. Infect. Public Health 12, 660–665. doi: 10.1016/j.jiph.2019.03.005
Gazzinelli, R. T., Oswald, I. P., Hienry, S., James, S. L., and Sher, A. (1992). The microbicidal activity of interferon-y-treated macrophages against Trypanosoma cruzi involves an L-arginine-dependent, nitrogen oxide-mediated mechanism inhibitable by interleukin-10 and transforming growth factor-beta. Eur. J. Immunol. 22, 2501–2506. doi: 10.1002/eji.1830221006
Ghareeb, M. A., Habib, M. R., Mossalem, H. S., and Abdel-Aziz, M. S. (2018). Phytochemical analysis of Eucalyptus camaldulensis leaves extracts and testing its antimicrobial and schistosomicidal activities. Bull. Natl. Res. Cent. 42, 1–9. doi: 10.1186/s42269-018-0017-2
Giamarellos-Bourboulis, E. J., Tziortzioti, V., Koutoukas, P., Baziaka, F., Raftogiannis, M., Antonopoulou, A., et al. (2006). Clarithromycin is an effective immunomodulator in experimental pyelonephritis caused by pan-resistant Klebsiella pneumoniae. J. Antimicrob. Chemother. 57, 937–944.
Green, L. C., Wagner, D. A., Glogowski, J., Skipper, P. L., and Wishnok, J. S. (1982). Tannenbaum, S.R. Analysis of nitrate, nitrite, and [15n]nitrate in biological fluids. Anal. Biochem. 126, 131–138. doi: 10.1016/0003-2697(82)90118-x
Gupta, S., Wen, J., and Garg, N. J. (2009). Oxidative stress in Chagas disease. Interdiscip. Perspect. Infect. Dis. 2009:190354. doi: 10.1155/2009/190354
Habila, N., Agbaji, A. S., Ladan, Z., Bello, I. A., Haruna, E., Dakare, M. A., et al. (2010). Evaluation of In Vitro Activity of Essential Oils against Trypanosoma brucei Astrolabe and Trypanosoma evansi. J. Parasito. Res. 2010:534601. doi: 10.1155/2010/534601
Hagos, A., Goddeeris, B. M., Yilkal, K., Alemu, T., Fikru, R., Yacob, H. T., et al. (2010). Efficacy of Cymelarsan and Diminasan against Trypanosoma equiperdum infections in mice and horses. Vet. Parasitol. 171, 200–206. doi: 10.1016/j.vetpar.2010.03.041
Herr, N., Bode, C., and Duerschmied, D. (2017). The effects of serotonin in immune cells. Front. Cardiovasc. Med. 4:48. doi: 10.3389/fcvm.2017.00048
Joshi, P. P., Shegokar, V. R., Power, R. M., Herder, S., Katti, R., Salkar, H. R., et al. (2005). Human trypanosomiasis caused by Trypanosoma evansi in India: the first case report. Am. J Trop. Med. Hyg. 73, 491–495. doi: 10.4269/ajtmh.2005.73.491
Kabiru, Y. A., Ogbadoyi, E. O., Okogun, J. I., Gbodi, T. A., and Makun, H. A. (2013). Anti-trypanosomal potential of Eucalyptus camaldulensis. Br. J. Pharmacol. Toxicol. 4, 25–32. doi: 10.19026/bjpt.4.5374
Kalu, A. U., and Haruna, E. (1985). Effects of vasopressor drugs on number of Trypanosoma congolense in ruminant blood. Vet. Parasitol. 17, 287–294. doi: 10.1016/0304-4017(85)90019-6
Keita, M., Vincendeau, P., Buguet, A., Cespuglio, R., Vallat, J. M., Dumas, M., et al. (2000). Inducible nitric oxide synthase and nitrotyrosine in the central nervous system of mice chronically infected with Trypanosoma brucei nitro tyrosine. Exp. Parasitol. 95, 19–27. doi: 10.1006/expr.2000.4505
Koracevic, D., Koracevic, G., Djordjevic, V., Andrejevic, S., and Cosic, V. (2001). Method for the measurement of antioxidant activity in human fluids. J. Clin. Pathol. 54, 356–361. doi: 10.1136/jcp.54.5.356
Lin, J. Y., and Tang, C. Y. (2007). Determination of total phenolic and flavonoid contents in selected fruits and vegetables, as well as their stimulatory effects on mouse splenocyte proliferation. Food Chem. 101, 140–147. doi: 10.1016/j.foodchem.2006.01.014
Lubbad, M. Y., Al-Quraishy, S., and Dkhil, M. A. (2015). Antimalarial and antioxidant activities of Indigofera oblongifolia on Plasmodium chabaudi-induced spleen tissue injury in mice. Parasitol. Res. 114, 3431–3438. doi: 10.1007/s00436-015-4568-y
Matt, S. M., and Gaskill, P. J. (2020). Where Is dopamine and how do immune cells see it?: Dopamine-mediated immune cell function in health and disease. J. Neuroimmune. Pharmacol. 15, 114–164. doi: 10.1007/s11481-019-09851-4
Nasr, A., Khan, T. S., Huang, S. P., Wen, B., Shao, J. W., and Zhu, G. P. (2019). Comparison among five eucalyptus species based on their leaf contents of some primary and secondary metabolites. Curr. Pharm. Biotechnol. 20, 573–587. doi: 10.2174/1389201020666190610100122
Pakkirisamy, M., Kalakandan, S. K., and Ravichandran, K. (2017). Phytochemical Screening, GC-MS, FT-IR Analysis of Methanolic Extract of Curcuma caesia Roxb (Black Turmeric). Pharmacog. J. 9, 952–956. doi: 10.5530/pj.2017.6.149
Powar, R. M., Shegokar, V. R., Joshi, P. P., Dani, V. S., Tankhiwale, N. S., Truc, P., et al. (2006). A rare case of human trypanosomiasis caused by Trypanosoma evansi. Indian J. Med. Microbiol. 24, 72–74. doi: 10.4103/0255-0857.19904
Sanchez, G. (1973). Physiological and antigenic changes in Trypanosoma lewisi mediated by epinephrine. Comp. Gen. Pharmacol. 41, 327–332. doi: 10.1016/0010-4035(73)90044-x
Sazmand, A., Desquesnes, M., and Otranto, D. (2022). Trypanosoma evansi. Trends Parasitol. [Online ahead of print] doi: 10.1016/j.pt.2021.12.010
Shakibaie, M., Torabi-Shamsabad, R., Forootanfar, H., Amiri-Moghadam, P., Amirheidari, B., Adeli-Sardou, M., et al. (2021). Rapid microwave-assisted biosynthesis of platinum nanoparticles and evaluation of their antioxidant properties and cytotoxic effects against MCF-7 and A549 cell lines. 3 Biotech 11:511. doi: 10.1007/s13205-021-03007-z
Tsakiris, S., Schulpis, K. H., Marinou, K., and Behrakis, P. (2004). Protective effect of lcysteine and glutathione on the modulated suckling rat brain Plasmodium berghei ANKA after chronic exposure. Parasitol. Res. 108, 807–814.
Keywords: Surra, Trypanosoma, brain, spleen, Eucalyptus camaldulensis
Citation: Dkhil MA, Al-Shaebi EM, Abdel-Gaber R, Alkhudhayri A, Thagfan FA and Al-Quraishy S (2022) Treatment of Trypanosoma evansi-Infected Mice With Eucalyptus camaldulensis Led to a Change in Brain Response and Spleen Immunomodulation. Front. Microbiol. 13:833520. doi: 10.3389/fmicb.2022.833520
Received: 11 December 2021; Accepted: 31 January 2022;
Published: 21 March 2022.
Edited by:
Sónia Silva, University of Minho, PortugalReviewed by:
Lachhman Das Singla, Guru Angad Dev Veterinary and Animal Sciences University, IndiaCopyright © 2022 Dkhil, Al-Shaebi, Abdel-Gaber, Alkhudhayri, Thagfan and Al-Quraishy. This is an open-access article distributed under the terms of the Creative Commons Attribution License (CC BY). The use, distribution or reproduction in other forums is permitted, provided the original author(s) and the copyright owner(s) are credited and that the original publication in this journal is cited, in accordance with accepted academic practice. No use, distribution or reproduction is permitted which does not comply with these terms.
*Correspondence: Mohamed A. Dkhil, bW9oYW1lZGRraGlsQHlhaG9vLmNvbQ==
Disclaimer: All claims expressed in this article are solely those of the authors and do not necessarily represent those of their affiliated organizations, or those of the publisher, the editors and the reviewers. Any product that may be evaluated in this article or claim that may be made by its manufacturer is not guaranteed or endorsed by the publisher.
Research integrity at Frontiers
Learn more about the work of our research integrity team to safeguard the quality of each article we publish.