- 1Servicio de Nutrición y Bienestar Animal, Departament de Ciència Animal i Dels Aliments, Universitat Autònoma de Barcelona, Bellaterra, Spain
- 2Departamento de Producción Animal, Facultad de Medicina Veterinaria y Zootecnia, Universidad Agraria del Ecuador (UAE), Guayaquil, Ecuador
- 3Laboratorios Ordesa SL, Parc Científic de Barcelona, Barcelona, Spain
The use of bifidobacteria as probiotics has proven to be beneficial in gastroenteric infections. Furthermore, prebiotics such as inulin can enhance the survival and growth of these bacteria. Two trials were performed to evaluate the effects of the administration of Bifidobacterium longum subsp. infantis CECT 7210 and oligofructose-enriched inulin against Salmonella enterica serovar Typhimurium or enterotoxigenic Escherichia coli (ETEC) F4. A total of 72 (Salmonella trial) and 96 (ETEC F4 trial) weaned piglets were used in a 2 × 2 design (with or without synbiotic, inoculated or not with the pathogen). After adaptation, animals were orally inoculated. Performance and clinical signs were evaluated. On days 4 and 8 (Salmonella trial) and 3 and 7 (ETEC F4 trial) post-inoculation (PI), one animal per pen was euthanized. Blood, digestive content and tissue samples were collected and microbiological counts, fermentation products, serum inflammatory markers and ileum histomorphometry analysis were performed. Both challenges had an impact on faecal consistency (p < 0.001), including the faecal shedding of Salmonella and increased numbers of enterobacteria and coliforms. The synbiotic administration did not have any effect on pathogen loads but induced changes in the fermentation profile, such as increased valeric acid in both trials as well as decreased acetic acid, except for Salmonella-challenged animals. The effect on propionate varied among trials, increasing in challenged synbiotic-treated pigs and decreasing in non-challenged ones in the Salmonella trial (P interaction = 0.013), while the opposed occurred in the ETEC F4 trial (P interaction = 0.013). The administration of the synbiotic increased intraepithelial lymphocytes (IEL; p = 0.039) on day 8 PI in the Salmonella trial and a similar trend occurred in non-challenged pigs in the ETEC F4 trial (P interaction = 0.086). The results did not provide evidence of reduced pathogen load with the synbiotic, although a modulation in fermentative activity could be identified depending on the challenge. Consistent increases were found in IEL, suggesting that this synbiotic combination has some immunomodulatory properties.
Introduction
Acute enteritis is a pathology that consists of a loss of faecal consistency and/or increased stool frequency, with a duration of less than 7 days. In the vast majority of cases, it is precipitated by an infectious agent, this being a frequent cause of global childhood mortality and morbidity, especially in the developing world (Thapar and Sanderson, 2004). The list of organisms that can provoke acute infectious enteritis includes viruses, bacteria and parasites. As many as 70% of cases are generated by a virus (principally rotavirus), while a non-negligible 20% are caused by a bacterial infection (Koletzko and Osterrieder, 2009). Among these bacteria, Salmonella and Escherichia coli can often be isolated, with a total of 25,708 (20, 385 Salmonella; 5, 323 E. coli) cases reported to the National Outbreak Reporting System in the United States between 2015 and 2018 (CDC, 2021). These infections commonly have their origin in food contamination, although the infection can also be produced via contact with infected animals (Conrad et al., 2017). New-born and young children are particularly susceptible to infections because their immune systems are not mature and they are not fully immunocompetent. An important form of protection is provided by the mother through passive IgG transplacental transfer, as well as in the milk (Simon et al., 2015) as it has been demonstrated that breastfeeding diminishes the incidence and severity of infectious diarrhoea (Farthing et al., 2013; Hartman et al., 2019).
Probiotics are live beneficial microorganisms that, when administered in infant formulas, can help reduce the number of episodes and duration of diarrhoea associated with acute infections (Szajewska and Mrukowicz, 2001). Nonetheless, this effect is strain-dependent and different outcomes have been reported in the literature (Skórka et al., 2017). Bifidobacterium longum subsp. infantis CECT 7210 is a bifidobacteria isolated from infant faeces that when given as a supplement to healthy children, has been associated with a reduction in diarrhoea events (Escribano et al., 2018). Moreover, by the use of different animal models, previous results have evidenced the ability of this strain to provide protection against rotavirus infection using murine models (Moreno-Muñoz et al., 2011) and reduce Salmonella shedding in oral challenged piglets (Barba-Vidal et al., 2017). Prebiotics such as inulin or its derivatives can potentially benefit the survival and multiplication of bifidobacteria (Vandeputte et al., 2017) and combat enteric pathogens (Tran et al., 2018).
Therefore, it is hypothesised that the synbiotic administration of an advantageous Bifidobacterium strain with these prebiotics will result in an improved outcome regarding digestive bacterial illness. The aim of this work is to determine the efficacy of a synbiotic combination of B. longum subsp. infantis CECT 7210 and inulin enriched with oligofructose against Salmonella Typhimurium and enterotoxigenic E. coli F4, using weaned piglets as an animal model.
Materials and Methods
Two different experiments were executed to evaluate the efficacy of the synbiotic combination against an oral challenge with either Salmonella enterica serovar Typhimurium (Salmonella trial) or enterotoxigenic E. coli F4 (ETEC F4 trial). Both experiments were performed at the Experimental Unit of the Universitat Autònoma de Barcelona (UAB) and received prior approval (Permit No. CEEAH: 4026 DMAH: 10118) from the Animal and Human Experimental Ethical Committee of this institution and its competent authorities. The treatment, management, housing, husbandry and slaughtering conditions conformed to European Union Guidelines (Directive 2010/63/EU, European Commission, 2010). All efforts were made to minimise animal suffering.
Animals, Housing and Experimental Design
These trials were carried out as biosafety Level 2 procedures and all personnel involved received appropriate training. A total of 168 male piglets were distributed between the two trials as follows: 72 (Landrace × Large White) × Pietrain of 24 (± 4) days of age weighing 7.70 (± 0.15) kg for the Salmonella trial and 96 (Landrace × Large White) × Pietrain piglets of 21 (± 4) days of age weighing 4.98 (± 0.07) kg for the ETEC F4 trial. All animals came from high-sanitary-status farms and mothers that were serologically negative to Salmonella in the Salmonella trial or were not vaccinated against E. coli in the ETEC F4 trial.
Piglets were transported to the experimental unit located at the Autonomous University of Barcelona, comprising three boxes (Salmonella trial) and four boxes (ETEC F4 trial) of eight pens each (24 and 32 pens, respectively, with three animals per pen). Each 2m2 pen was separated by a solid fence that prevented any contact between animals of different pens. Each pen had a feeder and water nipple to provide feed and water ad libitum. All weaning rooms were equipped with an automatic heater and forced ventilation and each pen had an individual heating light.
At arrival, the animals were distributed according to their initial body weight (BW) in order to ensure a homogeneous average body weight between treatment groups. Trials consisted of a factorial design 2 × 2 that included two treatments (control vs. synbiotic) and challenged or not with the pathogen (yes vs. no), resulting in a total of four experimental groups: control non-inoculated animals, NC; non-inoculated animals receiving synbiotic treatment, NS; control inoculated animals, IC; and inoculated animals receiving synbiotic treatment, IS. In the case of the first trial, the design was unbalanced, as piglets in two of the three rooms were challenged while the third room remained non-challenged. In contrast, for the second trial, we utilised four rooms and therefore had a balanced design. In each room, synbiotic treatment was distributed within four pens on one side of the room, with the four control pens on the other side of the room separated by a corridor to prevent contact between animals. Each experimental group had eight replicates, except for the non-challenged groups in the Salmonella trial, which had four replicates instead.
Probiotic Strains, Prebiotic and Diets
In both trials, the tested probiotic was B. longum subsp. infantis CECT 7210 strain, supplied by Ordesa SL in a lyophilized form and containing 5 × 1010 colony-forming units (cfu) per gram of product in a maltodextrin carrier. The estimated dosage during the procedures was the same for both trials (1 × 109 cfu per piglet and day). In the Salmonella trial, the animals received the probiotic orally each morning using disposable syringes without a needle. To this end, the lyophilized bacteria were re-suspended in 2 ml of phosphate-buffered saline (PBS) no more than 1 h prior to administration. The control groups were administered the same amount of sterile PBS as a placebo. In the ETEC F4 trial, the piglets received the probiotic mixed in their feed with the aim of simplifying daily work and minimise the manipulation and the stress of the animals. Each day, the lyophilized probiotic was thoroughly mixed manually with fresh feed, with the dose adjusted considering the average feed intake (1 g of lyophilized probiotic per 1,000 g of feed). The stability of the probiotic into the feed and feeders was previously assessed in a viability test to ensure an accurate dosage of the product per day.
The prebiotic consisted of a mixture of oligofructose (FOS) and inulin (Orafti® Synergy1, Beneo; Mannheim, Germany) that was administered through the feed (5%) in both trials.
Pre-starter diets were formulated in concordance with the nutrient requirement standards for pigs (NRC, 2012) and given in a mash form. In the Salmonella trial, both experimental diets were manufactured from the same single basal batch (with the same formula) being the prebiotic subsequently mixed with half of the batch. In the ETEC F4 trial, potential amino acid dilution in the synbiotic diet due to the incorporation of the prebiotic was compensated by the addition of synthetic amino acids with different adjusted formulae for each experimental diet (synbiotic diet included as: 0.5 g L-valine, 0.9 g L-lysine HCL, 1.2 g DL-methionine, 0.5 g L-threonine and 0.2 g L-tryptophan per kg of feed); in this way, we could guarantee the same level of the most limiting amino acids in both experimental diets. Details of the ingredient and chemical composition are given in Table 1.
Salmonella and ETEC Strains
In the first trial, the bacterial strain used for the oral challenge was a Salmonella enterica serovar Typhimurium var. monophasic (formula: 4,5,12:i:-, resistance profile: ACSSuT-Ge, Fagotype: U302) that had been isolated from a salmonellosis outbreak of fattening pigs in Spain, provided by the Infectious Diseases Laboratory (Ref. 301/99) of the UAB. Preparation of the oral inoculum consisted of 24-h incubation at 37°C in buffered peptone water (BPW; Oxoid; Hampshire, United Kingdom) and diluted (1:10) with sterile PBS (Sigma-Aldrich; Madrid, Spain). The final concentration of the inoculum was 1 × 109 cfu/ml. Inoculum concentrations were determined prior to the inoculation by McFarland standards and were doubly plated in Tryptic Soy Agar (Liofilche; Italy) on the same day in order that they could be checked by manual plate counting.
In the second trial, the bacterial strain of enterotoxigenic E. coli F4 used was isolated from the faeces of 14-week-old pigs and provided by the Infectious Diseases Laboratory (Ref. 30/14) of the UAB. This strain presented the following virulence factors: F4ab, F4ac, LT, STb and EAST1 and was negative for K99, F6, F18, F41, STa, VT1 and VT2 y EAE. The oral inoculum was prepared via 12-h overnight incubation at 37°C in Brain Heart Infusion broth (Oxoid; Hampshire, England) with slow agitation (250 rpm) in an orbital incubator. The culture was directly given to the animals with a final concentration of 1·109 cfu/ml. Inoculum concentrations were also determined before the inoculation by McFarland standards and were plated in Luria Agar (LA; made in-house: tryptase, yeast extract, NaCl, agar and Oxoid; Hampshire, UK) the same day for manual plate counting.
Experimental Procedure
Both experiments lasted 15 days. One animal from each pen was euthanized on days 4 and 8 post-inoculation (PI) in the Salmonella trial and on days 3 and 7 PI in the ETEC F4 trial.
After an adaptation period of 7 days in the Salmonella trial and 8 days in the ETEC F4 trial, an inoculum containing the pathogenic bacteria culture was given to the challenged groups orally: in the first trial, this was one 2 ml dose (2 × 109 cfu) of Salmonella Typhimurium, whereas in the second trial, it was one 6 ml dose (6 × 109 cfu) of ETEC F4. The same amount of sterile broth was administered to the non-challenged piglets. In order to ensure that the animals’ stomachs were full at the time of the oral challenge, pigs were starved for a period of 12 h and feed was reintroduced 30 min before inoculation.
From the challenge onwards, the animals’ clinical signs were checked daily to evaluate their post-inoculation status (i.e. dehydration, anorexia, apathy, general behaviour and faecal score), always by the same person. Faecal score was measured using a scale whereby 1 = solid and cloddy, 2 = soft with shape, 3 = very soft or viscous liquid and 4 = watery or with blood. Rectal temperature was assessed using a digital thermometer (Accuvet, Sanchung City, Taiwan) on days 1, 2 and 3 PI in the Salmonella trial and days 1 and 2 PI in the ETEC F4 trial.
The animals’ performance was also monitored as: individual body weight (BW) was registered on arrival and on days 0, 4 and 8 PI (0, 3 and 7 PI in the ETEC F4 trial) and feed intake was determined on days 0, 4 and 8 PI in the Salmonella trial, whereas in the ETEC F4 trial, feed intake was registered daily, concurring with the regular feed replacement aimed at maintaining probiotic viability. The average daily gain (ADG), average daily feed intake (ADFI) and the gain:feed ratio (G:F) were calculated by pen. The mortality rate was also registered and no antibiotic treatment was given to the animals in any of the experiments.
For microbiological analysis, faecal samples were collected aseptically after spontaneous defecation or by digital stimulation at arrival on the day of the inoculation (0 PI): in the Salmonella trial, this was from the animal with the highest initial BW in each pen (N = 24), whereas in the ETEC F4 trial, faecal samples were obtained from the animal with the medium BW in each pen (N = 32). Furthermore (and just for the Salmonella trial), additional faecal samples were taken on days 1, 3 and 7 PI from the same animal.
On days 4 and 8 PI (3 and 7 PI in the ETEC F4 trial), one pig per pen was euthanized. Slight differences in sampling days between trials were due to logistic reasons. On day 4 PI, the animal selected was the one with the medium initial BW, while on day 8 PI, it was the heaviest piglet in each pen. The animals were euthanized and sequentially sampled during the morning of each day (between 8:00 and 13:00 h). Before injecting the euthanasia drug, 10 ml sample blood was taken from each animal via venepuncture of the cranial cava vein using 10 ml blood collection tubes without anticoagulant (Aquisel; Madrid, Spain). Immediately after blood sampling, pigs were intravenously administered a lethal dose injection of sodium pentobarbital (140 mg/kg BW; Euthasol, Le Vet B.V.; Oudewater, Netherlands). Once dead, the animals were bled, the abdomen opened and the gastrointestinal tract extracted.
A faecal sample from the rectum was used for traditional microbiology in the ETEC F4 trial, whereas a caecal sample was obtained for microbiology in the Salmonella trial. They were kept on ice and analysed within 4 hours.
In both experiments, the digesta of the ileum and the proximal colon were collected and homogenised prior to pH determination with a pH meter calibrated on each day of use (Crison 52–32 electrode, Net Interlab; Barcelona, Spain) and the digesta score was registered on a scale as follows: 1 = liquid; 2 = liquid with some formed material; 3 = thick; and 4 = semi-solid. Subsamples of the ileal and colonic digesta were preserved for different analyses. One aliquot of colonic content was kept at −80°C for ETEC F4 (ETEC F4 trial) and probiotic quantification by qPCR. A set of ileal and colonic digesta samples were conserved frozen at −20°C in H2SO4 solution (3 ml of content plus 3 ml of 0.2 N H2SO4) for ammonia (NH3) determination and an additional set (~10 g) was also frozen (−20°C) for future analysis of short-chain fatty acids (SCFA) and lactic acid.
In the ETEC F4 trial, to determine the number of enterobacteria, coliforms and ETEC F4 attached to the intestinal mucosa, 5 cm sections of distal ileum were collected from each animal, washed thoroughly with sterile PBS, opened longitudinally and scraped with a microscopy glass slide to obtain the mucosa scraping.
For the histological study, 1 cm sections from the ileum were removed, opened longitudinally, thoroughly and carefully washed with 4% formaldehyde solution (Panreac; Castellar del Vallès, Spain) and fixed by immersion in the same solution.
Blood samples were centrifuged (3,000 × g for 15 min at 4°C) after clotting and the serum obtained was stored at −20°C.
Analytical Procedures
Feed Analysis
Chemical analyses of the diets, including dry matter (DM), ash, crude protein and diethyl ether extract, were performed according to Association of Official Agricultural Chemists standard procedures (AOAC, 1995). Neutral detergent fibre and acid-detergent fibre were determined according to the method of Van Soest et al. (1991).
Microbiological Analysis
For the microbiological analysis of Salmonella, samples were transferred to buffered peptone water solution in a concentration of 1:10. The quantitative analysis was performed by seeding serial dilutions of the samples 10−2, 10−4 and 10−6 in Xylose-Lactose-Tergitol-4 plates (XLT-4; Merck; Madrid, Spain). For the qualitative analysis, samples were incubated in BPW (37°C, 24 h), transferring 100 μl of the culture to 10 ml of Rappaport-Vassiliadis for a second incubation (42°C, 48 h) to finally seed them in XLT4 plates to observe H2S positive colonies.
For the enterobacteria and coliform counts, samples were serially diluted in Lactate Ringer Solution (Sigma-Aldrich; Madrid, Spain) and proper dilutions seeded in MacConkey agar (Oxoid; Madrid, Spain) and eosin methylene blue agar (Scharlab; Barcelona, Spain). Plaques were incubated for 24 h at 37°C and colonies were manually counted.
The presence of ETEC F4 in the colonic digesta and ileal scrapings was determined by real-time PCR. To extract the DNA from these samples, the commercial QIAmp DNA stool minikit (Qiagen; West Sussex, United Kingdom) was utilised. Afterwards, several aliquots of DNA eluted in Qiagen buffer AE (total volume; 200 μl) were stored frozen at −80°C. A qPCR targeting the gene coding the F4 fimbria of ETEC F4 using the SYBR green dye was performed according to the procedure described by Hermes et al. (2013). To express the results, the animals were distributed across five levels according to the number of gene copies per gram of fresh matter that they showed when qPCR was performed. Ranges were defined as follows: negative = under 4 logarithmic units of gene copies per gram of fresh matter; low = 4–5.5 logarithmic units of gene copies per gram of fresh matter; medium = 5.5–7 logarithmic units of gene copies per gram of fresh matter; high = 7–8.5 logarithmic units of gene copies per gram of fresh matter; and very high = more than 8.5 logarithmic units of gene copies per gram of fresh matter.
Short-Chain Fatty Acids, Lactic Acid and Ammonia Analyses
Short-chain fatty acids and lactic acid analyses were performed using gas chromatography, after the samples had undergone acid–base treatment followed by ether extraction and derivatization with N-(tertbutyldimethylsilyl)-N-methyl-trifluoroacetamide plus 1% tert-butyldimethylchlorosilane agent, using the method of Richardson et al. (1989) that was subsequently modified by Jensen et al. (1995).
Ammonia concentrations were assessed using a gas-sensitive electrode (Hatch Co.; Colorado, United States) combined with a digital voltmeter (Crison GLP 22, Crison Instruments, SA; Barcelona, Spain), following a procedure described by Hermes et al. (2009) that was adapted from Diebold et al. (2004). Samples were diluted (1:2) in 0.16 M NaOH and, after homogenisation, were centrifuged at 1500 × g for 10 min. Once the ammonia was released, it was measured in the supernatants as a change in voltage in mV.
Serum Analysis
Serum concentrations of Tumour Necrosis Factor-α (TNF-α) were determined by Quantikine Porcine TNF-α kits (R&D Systems; Minneapolis, United States) and pig major acute-phase protein (Pig-MAP) concentration was determined by a sandwich-type enzyme-linked immunosorbent assay (ELISA; Pig MAP Kit ELISA, Pig CHAMP Pro Europe SA; Segovia, Spain) according to the manufacturer’s instructions. In the Salmonella trial, antibodies against Salmonella were also assessed using an ELISA Salmonella Herdcheck (Idexx; Hoofddorp, Netherlands), establishing the cut-off for positivity in optic density ≥ 40%.
Histological Analysis
For histological study, tissue samples were dehydrated and embedded in paraffin wax, sectioned at a thickness of 4 μm and stained with haematoxylin and eosin. The measurements of 10 different villus-crypt complexes per sample and the counting of intraepithelial lymphocytes (IEL), goblet cells (GC) and the number of mitosis of each were performed with a light microscope (BHS, Olympus; Barcelona Spain), using as a guideline the procedure described in Nofrarías et al. (2006). Briefly, the villus height and crypt depth were measured using a linear ocular micrometre (Olympus, Microplanet). The same villus and crypt columns were used to determine the number of IEL, GC and mitosis. On the basis of the cellular morphology, differences between the nuclei of enterocytes, mitotic figures, goblet cells and lymphocytes were clearly distinguishable at 400× magnification. All morphometric analysis was done by the same person, who was blinded to the treatments.
Statistical Analysis
The results are expressed as means with their standard errors unless otherwise stated. Microbiological counts were log transformed for analysis. A two-way analysis of variance (ANOVA) was used to examine the effect of the two experimental treatments and the inoculation with the following model:
where Yijk relates to each observation of the outcome variable, m is the global mean, treati is the main effect of treatment, challengej is the main effect of the oral pathogen challenge and treat*challengeij corresponds to the interaction between treatment and the challenge. Finally, eij is the experimental error term. Regarding the interaction term, it was removed from the model when found not to be significant.
The effects on the post-slaughter measurements were examined using the R v3.4 (R Core Team, 2013) lm function for two-way ANOVA, with the treatment and the challenge effects as main effects. For Salmonella and E. coli F4 prevalence values and Pig-MAP serum concentrations, data were subjected to frequency analysis using the fisher. Test function in the same package.
ADFI and daily faecal scores were also analysed using the lme4 package (Bates et al., 2015) lmer function for a generalised linear mixed model with a treatment-by-time interaction term and considering the animal as the random effect.
The experimental unit was the pen. For all analyses, significance was set at p < 0.05 and values of p ≥0.05 but ≤0.10 were considered to indicate a statistical trend. When treatment effects were established, the mean comparison was adjusted with the Tukey–Kramer test. Data are presented as means and residual standard deviation.
Results
Both experiments proceeded as expected, without any remarkable incidence.
The oral challenge with the pathogenic bacteria induced moderate clinical signs in the animals that were slightly more severe after the Salmonella challenge (Salmonella trial). In these trials, humane euthanasia of two pigs was indicated (1 IS; 1 NIS). Furthermore, two spontaneous casualties were registered in the Salmonella trial (1 IC; 1 IS) and the ETEC F4 trial (1 NIS; 1 IC).
Performance Parameters
Changes in ADG, ADFI and gain:feed ratio (G:F) with the experimental treatments are shown in Table 2.
The challenge with the pathogen caused a decrease in ADFI and ADG in the Salmonella trial (p = 0.010 and p = 0.024, respectively). The effects of the ETEC F4 challenge were milder, with only a numerical trend seen for a lower ADFI in the post-inoculation period (333.65 vs. 367.95 g for challenged and non-challenged groups, respectively, p = 0.152).
No significant changes in ADFI nor ADG were registered that might be associated with the synbiotic treatment regardless of the trial, aside from a numerical difference (p = 0.130) in the ETEC F4 trial for reduced ADG during the post-inoculation phase. Moreover, in the Salmonella trial, a reduction in the G:F ratio was seen after the adaptation period (p = 0.020).
Clinical Signs
On day 1 PI, an increment of 1°C of rectal temperature was caused by the Salmonella challenge (38.9 ± 0.13°C vs. 39.8 ± 0.18°C, p < 0.001), whereas the ETEC F4 challenge did not alter the piglets’ temperatures (39.1 ± 0.07°C vs. 39.1 ± 0.17°C, p = 0.974). No significant differences were found related to the synbiotic administration.
Figure 1 shows the evolution in faecal consistency after the oral challenge for each trial. In both trials, the challenge was able to significantly impair faecal consistency (p < 0.001) with an increase in the incidence of diarrhoea. However, the progression of the faecal score over time differed between the trials: whereas with the Salmonella challenge, the impairment of faecal consistency was registered up to the end of the trial; in the ETEC F4 trial, the faecal consistency returned to normal within 3–4 days. No significant differences were found related to the synbiotic treatment.
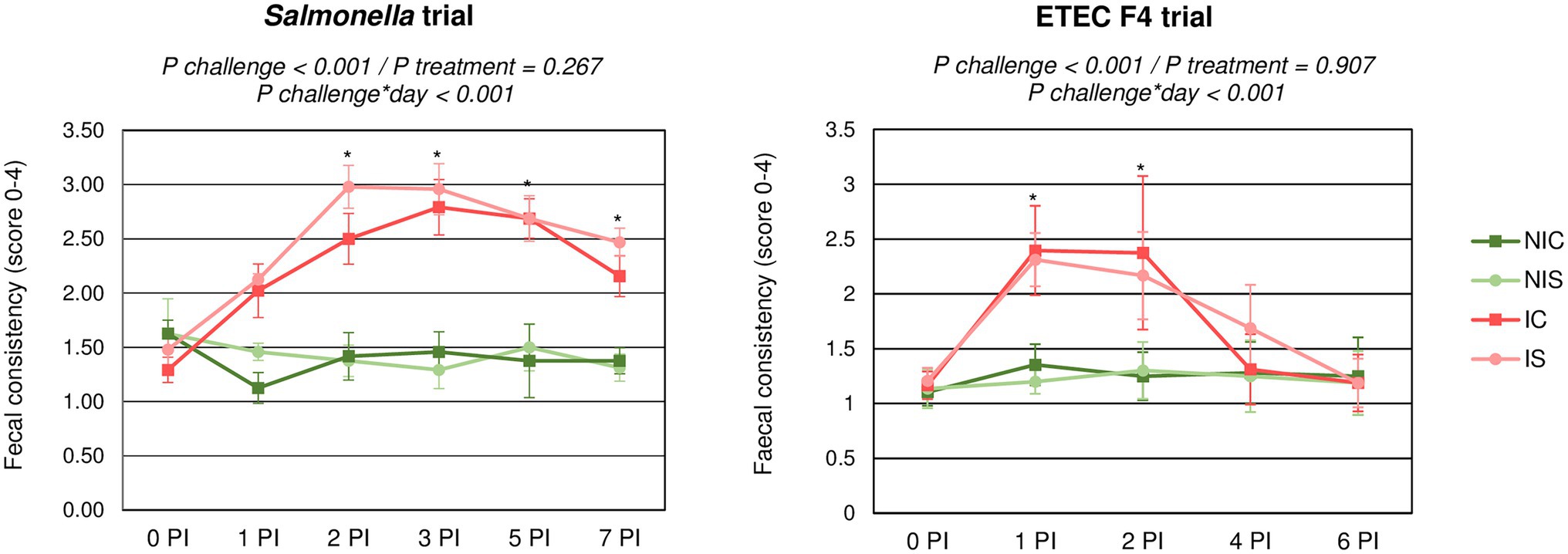
Figure 1. Evolution of average faecal scores for the different experimental groups during the post-inoculation (PI) period. IC, Inoculated animals receiving placebo; IS, Inoculated animals receiving the synbiotic; NIC, Non-inoculated animals receiving placebo; and NIS, Non-inoculated animals receiving the synbiotic. N = 8 for all groups except for non-challenged animals in Salmonella trial, N = 4. Bars correspond to standard error. *indicates statistical difference.
Regarding the consistency of the ileal and colonic digesta, the oral challenge did not instigate significant changes in any of these parameters, although some effects were observed regarding the colon digesta’s consistency following the administration of the synbiotic. In the Salmonella trial, the synbiotic treatment was associated with improved consistency at day 8 PI (3.44 vs. 2.62; p = 0.010); moreover, in the ETEC F4 trial, it caused a trend towards interaction (p = 0.061) with an improvement with supplementation in challenged animals (3.62 vs. 3.00), but a looser consistency in the non-challenged group (3.87 vs. 3.62).
Microbiological Analysis
In the Salmonella trial, the serological analysis revealed that all the animals remained seronegative throughout the study, confirming that they had not been exposed to the pathogen prior to the oral challenge. Regarding the presence of the pathogen in faeces and intestinal digesta, Figure 2 shows the evolution of Salmonella plate counts along sampling days in the challenged animals. In general terms, the non-challenged piglets remained negative during the study, with the exception of three piglets that recorded a positive result in at least one sample, albeit always at low-to-uncountable levels (< 102 cfu/g).
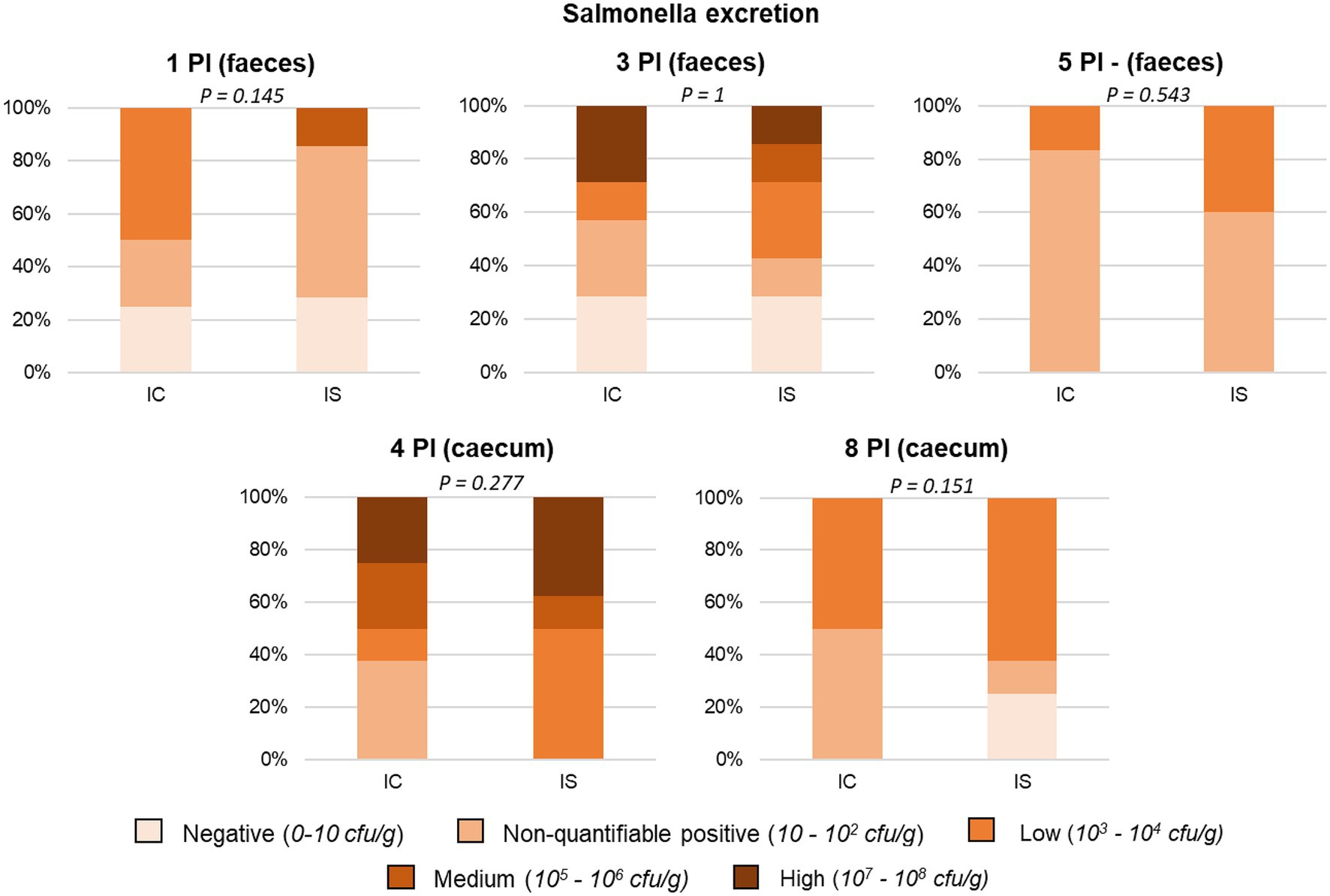
Figure 2. Percentage of animals included in the different faecal and caecal excretion levels of Salmonella. All samples were obtained from the heaviest animal in each pen, except for the caecal sample on day 4 PI, which was obtained from the anima of medium weight. IC, inoculated animals receiving placebo; IS, Inoculated animals receiving the synbiotic; and N = 8 for IC and IS. Values of p were obtained using Fisher’s Exact Test on R software.
All challenged animals were positive in at least one faecal sample and all presented Salmonella in the caecal content.
Regarding synbiotic administration, only a trend on day 1 PI was found, with the faeces revealing that the animals that received the synbiotic presented lower shedding compared to the control group (p = 0.145). Moreover, regarding the caecal digesta, 25% of piglets treated with the synbiotic turned negative to Salmonella excretion (vs. 0% control) on day 8 PI (P positive/negative excretion = 0.076).
Regarding the ETEC F4 trial, no differences between groups were registered in the plate counts of faecal enterobacteria or coliforms on the day of the piglets’ arrival. Furthermore, no changes caused by the synbiotic treatment were observed before the oral challenge, although differences could be noted related to the box (9.79, 10.69, 8.12 and 8.44 log cfu/g FM for IC, IS, NIC and NIS, respectively; p < 0.001). Table 3 shows the impact of the experimental treatments on the enterobacteria and coliform plate counts post-inoculation. The oral challenge promoted an increase in enterobacteria and coliforms either in the faeces (p < 0.013) or the ileal scrapings (p < 0.013) on day 3 PI. The effects produced by the challenge were no longer detected at day 7 PI. Moreover, no significant effects were found related to the synbiotic supplementation in any of the parameters analysed. However, a trend towards interaction in enterobacteria (p = 0.057) and coliform (p = 0.104) counts in the ileal scrapings could be identified at day 7 PI. On this day, non-challenged animals that received the synbiotic presented lower counts than the control, whereas challenged piglets exhibited the opposite effect.
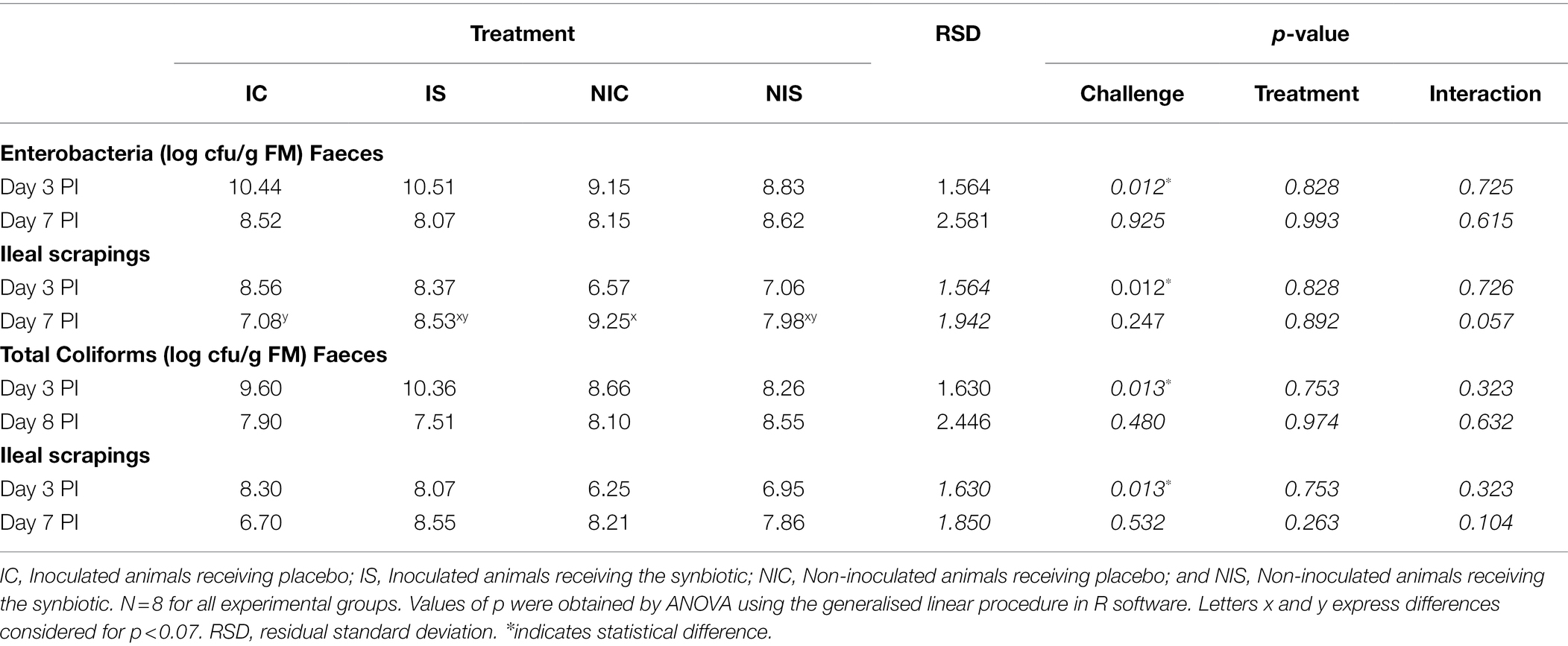
Table 3. Effects of experimental treatments on enterobacteria and coliform counts in faecal samples and ileal scrapings.
The results corresponding to qPCR targeting the coding gen of the F4 fimbria of E. coli F4 are summarised in Figure 3. Given that the pathogen could not be quantified in all animals, the data were analysed as frequencies. The figure shows the distribution of the percentage of animals within each of the five defined ranges based on the number of copies/g fresh matter found in the analysis. The effect of the oral challenge was clearly evidenced on day 3 PI through a significant increase in the percentage of animals showing large or very large numbers of copies in colonic content (p < 0.001) or ileal scrapings (p = 0.045). No significant differences were found related to the synbiotic administration.
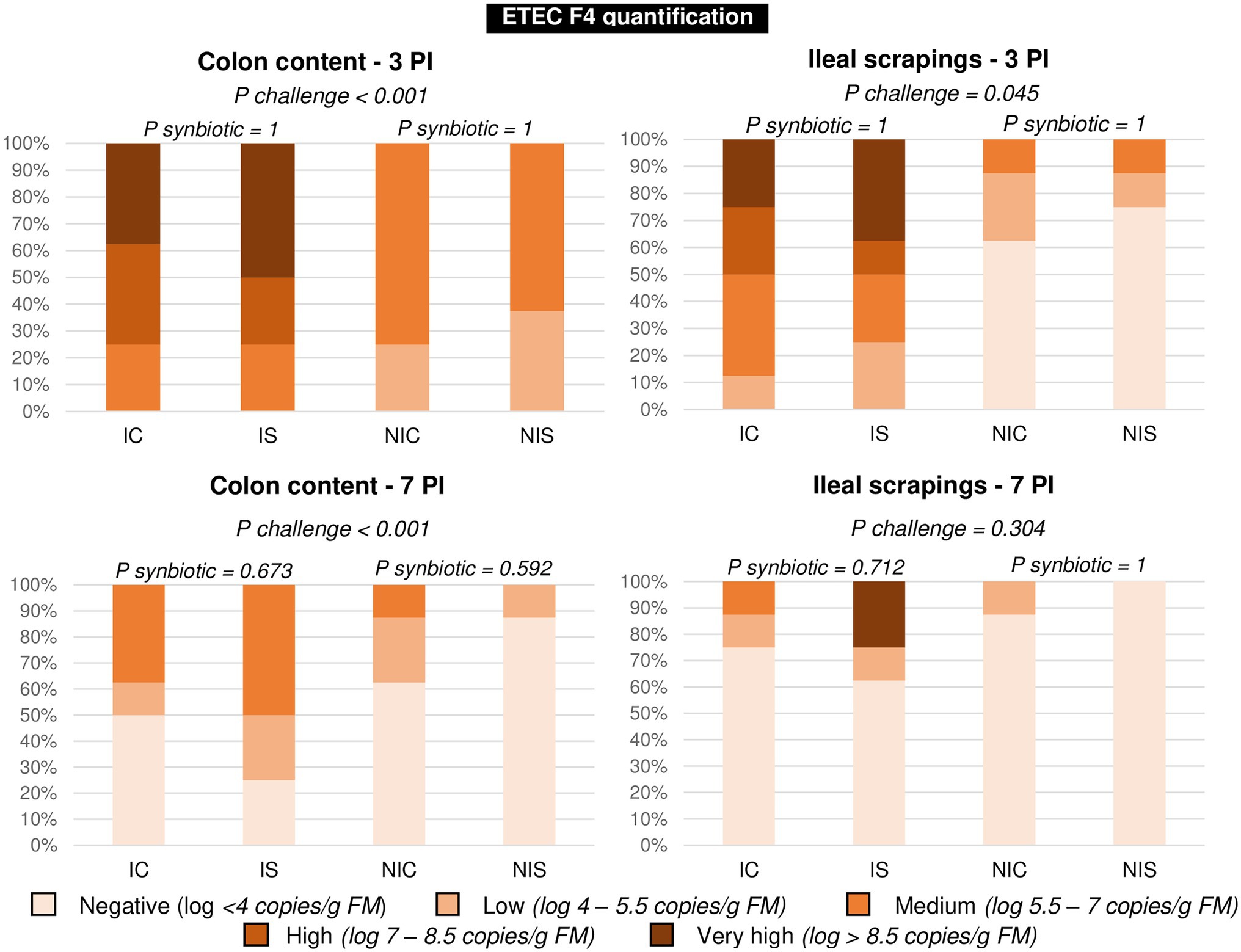
Figure 3. Percentage of animals in each presence level of ETEC F4 on days 3 and 7 post-inoculation. Different animals were sampled on day 3 PI (medium weight) and 7 PI (greatest weight). IC, inoculated animals receiving placebo; IS, Inoculated animals receiving the synbiotic; NIC, Non-inoculated animals receiving placebo; and NIS, Non-inoculated animals receiving the synbiotic. N = 8 for all experimental groups. Values of p were obtained using Fisher’s Exact Test on R software.
Intestinal Fermentation
Tables 4 and 5 show all the changes induced by the different experimental treatments in the ileal and colonic fermentation products.
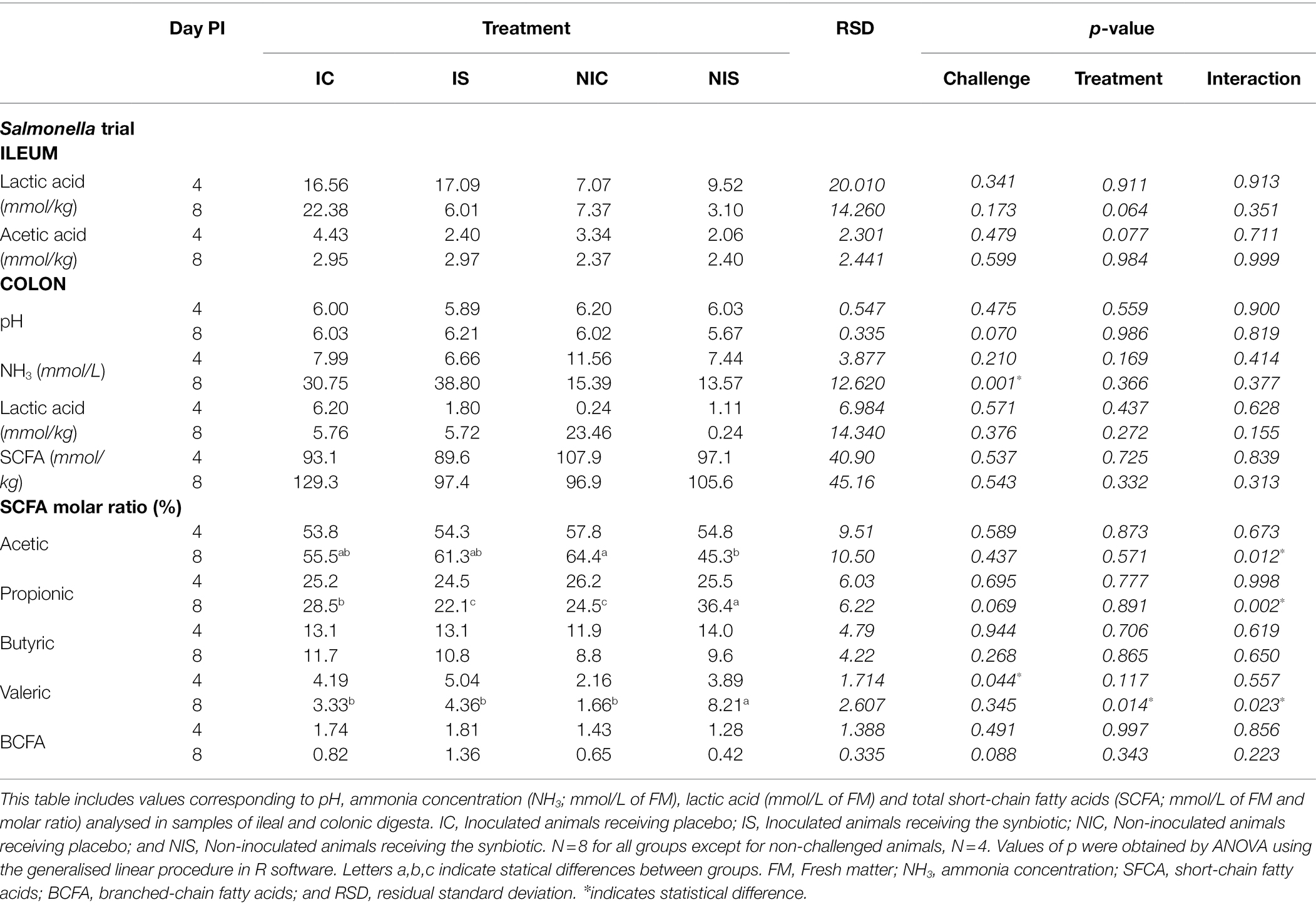
Table 4. Effects of experimental treatments on ileal and colonic fermentation in the Salmonella trial.
As displayed, the challenge with Salmonella stimulated a significant increase in ammonia levels in the colon (34.77 vs. 14.48 mmol/l; p = 0.001) on day 8 PI. It did not influence the total amount of SCFA or lactic acid, but challenged animals presented a higher molar percentage of valeric acid in the colon on day 4 PI (4.61 vs. 3.02%; p = 0.044) and also tended to have more branched-chain fatty acids on day 8 PI (1.09 vs. 0.53%; p = 0.088). Although the administration of the synbiotic did not modify the ammonia, lactic or SCFA concentrations, it promoted a higher molar percentage of valeric acid in the colon on day 8 PI (6.28 vs. 2.49%; p = 0.014) and provoked two interactions in the molar percentages of acetic (p = 0.012) and propionic acids (p = 0.002). Whereas in non-challenged piglets administration of the synbiotic mixture reduced the molar percentage of acetic acid and increased that of propionic acid, in the challenged animals, the effect was the opposite.
In the ETEC F4 trial, the effects of the challenge in terms of fermentative activity were more apparent. In the colon, the challenge was responsible for a drop in pH on day 3 PI (6.11 vs. 6.38, p = 0.035) and an increase in the total amount of SCFA on day 7 PI (129.2 vs. 111.3 mmol/kg; p = 0.026). The molar proportion of propionic was also increased on day 3 PI (24.10 vs. 21.10%; p = 0.016), especially in the synbiotic group (P interaction = 0.022).
Related to the effects of the synbiotic mixture on the fermentation parameters, an interaction was found in the ileum on day 4 PI regarding the concentration of acetic acid and lactic acid as the main products of fermentation. Acetic acid showed the greatest concentration in the non-challenged animals not receiving the synbiotic (P interaction = 0.033), whereas lactic acid presented the highest values in the non-challenged and non-supplemented animals (P interaction = 0.004). At the colonic level, no effects were found for the pH, total concentration of SCFA or lactic acid, but changes did occur in the profile of fermentation. The percentage of acetic acid fell on both days (56.05 vs. 60.65% for day 3 PI, p = 0.012 and 55.9 vs. 59.55% for day 7 PI, p = 0.029), in line with an increase in valeric acid (4.34 vs. 2.43% for day 3 PI, p = 0.010 and 4.07 vs. 2.20% for day 7 PI, p < 0.001) and a trend towards a higher butyric acid molar percentage (14.52 vs. 11.88% for day 3 PI, p = 0.093 and 14.22 vs. 12.78% for day 7 PI, p = 0.140).
Immune Response
No significant differences related to the synbiotic treatment were found in the serum levels of TNF-α or Pig-MAP. However, changes were noted associated with the pathogen inoculation. Regarding TNF-α, animals challenged with ETEC F4 presented higher concentrations than non-challenged pigs on day 7 PI (58.9, 57.2, 48.1 and 43.1 pg./ml for IC, IS, NIC and NIS, respectively; p = 0.010) and a similar pattern was found after the Salmonella challenge, although in this case, the differences did not reach statistical significance (125, 135, 124 and 101 pg./ml for IC, IS, NIC and NIS, respectively, day 8 PI; p = 0.132).
When analysing the Pig-MAP, the values did not adjust to a normal distribution and therefore the results were analysed as frequencies using Fisher’s exact test. Three range levels were defined as: high (> 2 mg/ml); borderline (1–2 mg/ml) and normal (< 1 mg/ml) according to Piñeiro et al. (2009). The results analysed in this way are shown in Figure 4. Only the Salmonella challenge was able to promote an increase in the number of animals with borderline-high levels of Pig-MAP at day 8 PI (p = 0.017), with no significant change induced by the ETEC F4 inoculation.
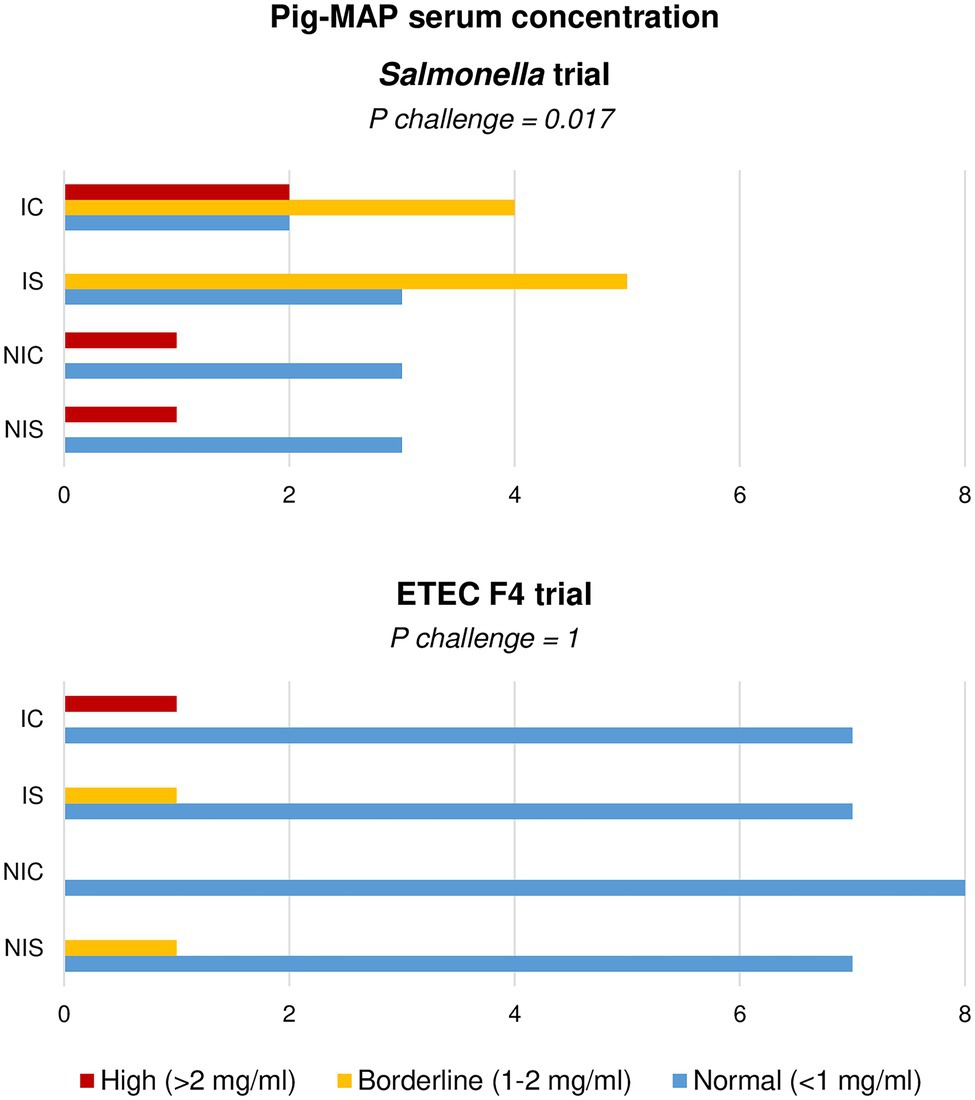
Figure 4. Effect of experimental treatments in serum levels of acute-phase protein Pig-MAP in piglets on day 8 (Salmonella trial) and 7 (ETEC F4 trial) following a pathogen oral challenge. Figure represents value frequencies between a normal (0.3–1 mg/ml) and abnormal (>2 mg/ml) range. IC, Inoculated animals receiving placebo; IS, Inoculated animals receiving the synbiotic; NIC, Non-inoculated animals receiving placebo; and NIS, Non-inoculated animals receiving the synbiotic. N = 8 for all experimental groups except for non-challenged animals in Salmonella trial, N = 4. Values of p were obtained using Fisher’s Exact Test on R software.
Intestinal Histological Structure
The effects of the experimental treatments on ileal histomorphology are summarised in Table 6. The Salmonella challenge promoted shorter villi on day 4 PI (p < 0.001) and deeper crypts on days 4 and 8 PI (p = 0.013 and p = 0.042, respectively).
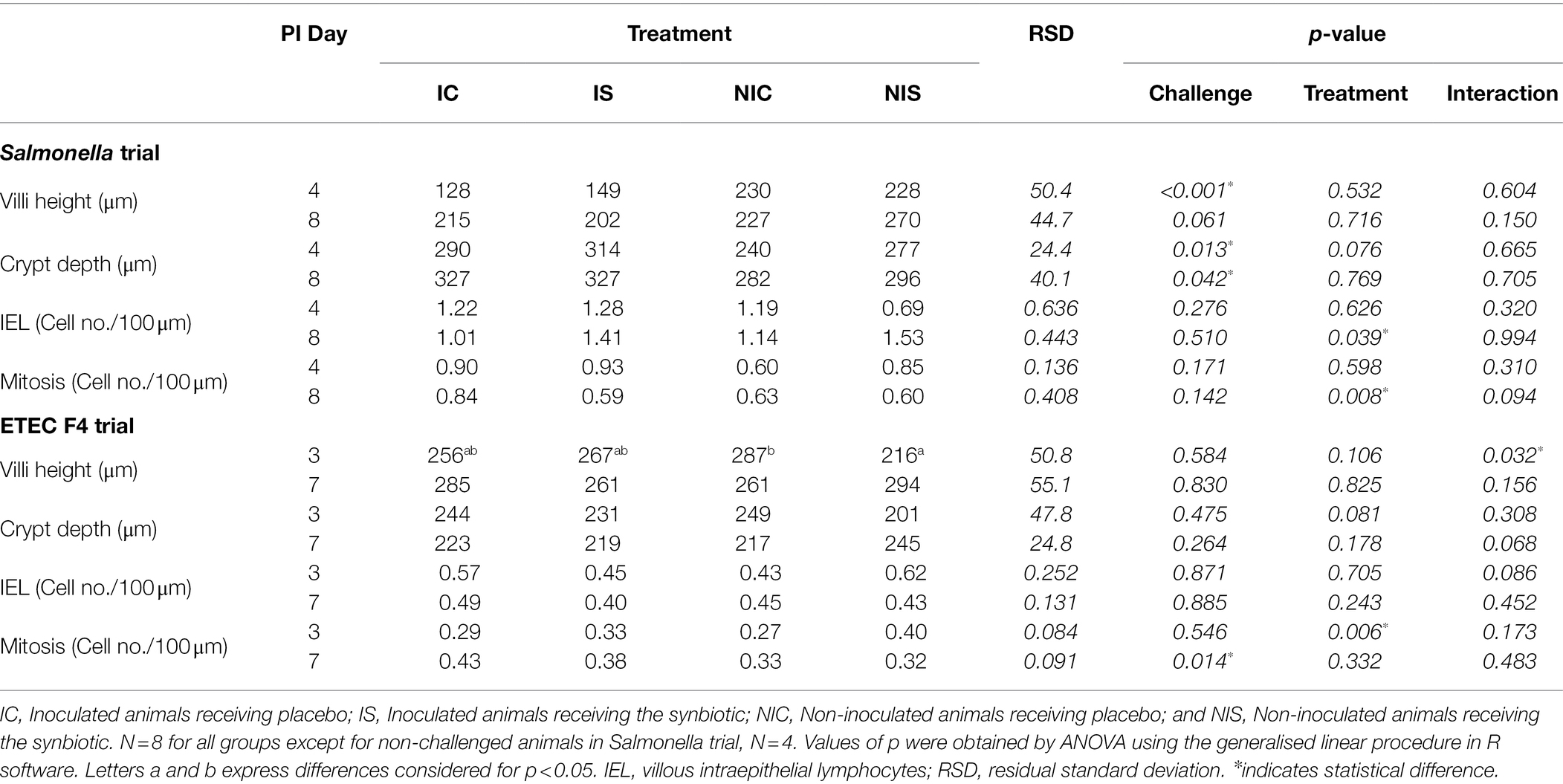
Table 6. Effects of treatments on histomorphological parameters on days 4 and 8 (Salmonella trial) and 3 and 7 (ETEC F4 trial) post-inoculation.
After the ETEC F4 challenge, no significant differences were found apart from a greater number of mitosis on day 7 PI (p = 0.014).
Regarding impact of synbiotic administration on ileal histomorphology, different outcomes were found depending on the trial. In the ETEC F4 trial, villous height was greater on day 3 PI in the animals receiving the synbiotic, but only in the non-challenge group (P interaction = 0.032). However, the opposite numerical effect was observed on the second sampling day in both trials (P interaction <0.16). Crypt depth showed an increasing trend due to the synbiotic administration on day 4 PI in the Salmonella trial (p = 0.078), but, contrarily, a decreasing one on day 3 in the ETEC F4 trial (p = 0.081). IEL presence was enhanced by the synbiotic at day 8 PI in the Salmonella trial (p = 0.039) and the same trend was observed on day 3 PI in the ETEC F4 trial, although only in the non-challenged animals (P interaction = 0.086). Mitosis were reduced by the synbiotic at day 8 PI in the Salmonella trial (p = 0.008), especially in the challenged animals (P interaction = 0.094). In contrast, in the ETEC F4 trial, mitosis increased thanks to the synbiotic at day 3 PI (p = 0.006).
Discussion
The aim of this work was to assess the potential of the combination of B. longum subsp. infantis CECT 7210 and a mixture of inulin and FOS as a synbiotic strategy to fight two common gastrointestinal pathogens: Salmonella and ETEC F4.
In our previous research (Barba-Vidal et al., 2017), the strain B. longum subsp. infantis CECT 7210 was found to have an effect against Salmonella Typhimurium and ETEC F4 colonisation in pigs with a stimulation of local immune response by increasing the number of intraepithelial lymphocytes. Furthermore, it is widely acknowledged that fructo-oligosaccharides (FOS) and inulin are selectively fermented by most strains of bifidobacteria (Wang and Gibson, 1993; Kaplan and Hutkins, 2000) due to the production of β-fructofuranosidases (Imamura et al., 1994). In fact, FOS and inulin are two of the most studied prebiotics with bifidogenic properties (Meyer and Stasse-Wolthuis, 2009). Considering these facts, we hypothesised that combining B. infantis CECT 7210 with FOS and inulin could therefore enhance its beneficial effects against pathogens, contributing to improved gut health.
With this objective, two different trials were performed to challenge animals with either Salmonella or ETEC F4. As is well known, these pathogens exhibit differences in terms of pathogenicity, mediated by distinct virulence factors and mechanisms (Boyen et al., 2008; Clements et al., 2012). Thus, the animals’ responses to the challenge and the clinical course differed depending on the pathogen. Whereas after the Salmonella challenge growth and feed intake were markedly reduced, with a clear effect on faecal score and an increase in rectal temperature, the challenge with ETEC F4 exhibited a much milder course of diarrhoea, the differences not being statistically significant for several parameters. Similar effects were observed by Barba-Vidal et al. (2017), viewing the challenge by ETEC F4 as milder than that of Salmonella Typhimurium.
The performance of the animals before the oral challenge was not modified by the administration of the synbiotic in terms of feed intake or weight gain, but in terms of gain:feed, a significant reduction in feed efficiency was seen in the Salmonella trial (0.48 vs. 0.66 for synbiotic vs. control diet, p = 0.02). We might hypothesise that this decrease in feed efficiency was the result of a dilution of the energy or limiting of amino acids in the SYN diet following the inclusion of 5% of the prebiotic. It may also have owed to changes in the transit time and digestibility of nutrients related to the inclusion of 5% of FOS/inulin (Kelly, 2009; Chen et al., 2017), being the changes that we observed in the consistency of caecal digesta at day 8 PI supported by this hypothesis. Furthermore, the reduction of gain:feed may have been due to modifications in the gut microbiota promoted by the synbiotic with an impact on host energy homeostasis (Rosenbaum et al., 2015; Ley et al., 2016). However, this is mere speculation, as we lack the evidence to support these ideas.
Clinical signs such as diarrhoea incidence were not improved by the administration of the synbiotic compound to the animals, but it is also fair to highlight that no deterioration was observed in any of the trials, verifying the safety of the probiotic strain, as proved by other authors (Moreno-Muñoz et al., 2011; Barba-Vidal et al., 2017; Rodríguez-Sorrento et al., 2020) even when it is combined with inulin and fructo-oligosaccharides.
Numerous previous studies have demonstrated that bifidobacteria, inulin and FOS can have a beneficial effect for the host, helping it to maintain a healthy gut environment. Indeed, bifidobacteria may increase the colonic intraluminal concentration of short-chain fatty acids (SCFA; Servin, 2004), which are responsible for a wide range of effects in the gastrointestinal system. Topping (1996) has described how the reduction in pH associated with an increase in SCFA might help to control the proliferation of pathogenic microorganisms. In addition, inulin and the fructo-oligosaccharides derived can produce the same effect on SCFA concentrations in vivo and in vitro (Pompei et al., 2008; Van der Beek et al., 2018; Baxter et al., 2019). In our trials, however, the concentration of SCFA in the colonic content was not enhanced by the synbiotic treatment. According to Nyman (2002), this outcome should be expected because the concentration of measured SCFA is contingent on the balance between production and absorption, and commonly SCFA produced by fermentation are rapidly absorbed or utilised by the colonic mucosa.
Regarding changes in the fermentation profile, different effects can be attributed to bifidobacteria and inulin or FOS. Several authors have described increases in the molar percentage of butyrate with probiotic bifidobacteria (Rossi et al., 2005; Belenguer et al., 2006), although the main fermentation product of bifidobacteria is acetate. Butyrogenic effects owe to a stimulation of acetate-depending, butyrate-producing colon bacteria by cross-feeding interactions that, in parallel, are required by some other bacteria that can convert lactate into butyrate, albeit only when acetate is present (De Vuyst and Leroy, 2011; Moens et al., 2016, 2017). Higher amounts of acetate and butyrate have been shown to have favourable effects on the colonic structure; for example, acetate promotes colonic epithelial proliferation and butyrate is responsible for the maintenance of mucosal integrity, reparation and colonocyte proliferation, given that it is the preferred energetic source for these cells (Topping, 1996). Inulin and FOS have been reported as exerting a bifidogenic effect in infants (Meyer and Stasse-Wolthuis, 2009; Paineau et al., 2013) as well as in modulating fermentation products in the gut. Furthermore, various in vitro studies have shown how inulin and oligofructose can increase butyrate, propionate (van de Wiele et al., 2007) and acetate (van der Beek et al., 2018) production. Differential effects may be related to the contrasting chemical structure of these compounds as well as to the specific microbial ecosystems in which they are introduced. In this regard, Rossi et al. (2005) has reported differences between inulin and FOS: whereas for inulin, the main fermentation product was butyric acid with lower amounts of acetic, lactic and propionic acids, for FOS, the main fermentation products were lactic acid and acetic acid, alongside lower amounts of butyric acid and no propionic acid. In vivo studies have also shown a variable impact on intestinal fermentation. Scholtens et al. (2006) have demonstrated increases in acetate and decreases in butyrate in the faeces of humans receiving 25–30 g/d of FOS for a period of 2 weeks, while Boets et al. (2015) have used stable isotope technology to demonstrate how in humans inulin is mainly fermented into acetate as well as to a lesser extent into butyrate and propionate.
Considering the varied findings of previous works, it was difficult to anticipate what to expect when combining bifidobacteria, inulin and FOS; moreover, the impact of the synbiotic would seem to be contingent on the trial in question. In the Salmonella trial, the synbiotic reduced the molar proportion of acetate in non-challenged animals but increased it in challenged ones. Differently, in the ETEC F4 trial, acetate was consistently reduced. These lower levels of acetate might be explained by a cross-feeding phenomena, supported in the ETEC F4 trial by the observable increasing trend of butyrate. In the same vein, the increase observed for acetate in the Salmonella-challenged animals with the synbiotic, could correspond to a more acute dysbiosis that might disturb the normal cross-feeding phenomena within bifidobacteria and colonic bacteria.
Similar kinds of interactions have been described in the literature. For instance, regarding FOS supplements to dogs, Pinna et al. (2018) found increases in the acetate:propionate ratio in low-protein diets but a decrease in high-protein diets.
Another SCFA that is rarely considered in the literature and whose concentration was augmented by the synbiotic treatment in both trials is valeric acid. This fatty acid, which is capable of inhibiting the growth of pathogenic bacteria like Clostridium difficile (McDonald et al., 2018), originates in 5-aminovalerate, which is a product of the anaerobic degradation of previously hydrolyzed protein by gut bacteria (Barker et al., 1987). Recent investigations have proved that a strain of Megasphaera elsdenii (a major inhabitant of the pig intestine) can utilise lactic acid as a fermentation substrate and convert it into valerate (Yoshikawa et al., 2018). It is feasible that a similar effect occurred in our experiments, as colonic lactic acid was augmented by the synbiotic, albeit only in the ETEC F4 trial.
Together with the inhibitory effects promoted by probiotics on enteropathogens throughout changes in the fermentation products, probiotics have also been shown to fight pathogens via other mechanisms. In particular, several species of Bifidobacterium are deemed capable of enhancing and modulating the immune response (Wagner et al., 2009; Bermudez-Brito et al., 2013; Presti et al., 2015), of releasing bacteriocins and bacteriocin-like substances (Poltavska and Kovalenko, 2012; Martinez et al., 2013) and of completing/displacing pathogens from their adhesion sites on the intestinal epithelium (Collado et al., 2005; Candela et al., 2008). The probiotic strain tested—B. longum subsp. infantis CECT 7210—has proven to be effective in reducing Salmonella loads in piglets in previous works (Barba-Vidal et al., 2017). Interestingly, the former strain administered together with L. rhamnosus HN001 was also capable of diminishing Salmonella and ETEC F4 presence as shown by our research group in the past (Rodríguez-Sorrento et al., 2020, 2021). Nevertheless, in the present trial, combining this probiotic with inulin and FOS was unable to reduce Salmonella excretion, suggesting that the combination of this Bifidobacterium strain with these prebiotics does not improve its power to fight the pathogen. Regarding the potential of B. longum subsp. infantis CECT 7210 to exclude ETEC F4, previous studies have demonstrated this strain’s ability to reduce the number of coliforms adhered to ileal mucosa in ETEC F4-challenged animals (Barba-Vidal et al., 2017), but in the present study, we were only able to find such an effect in the non-challenged animals. When challenged with the ETEC F4, the synbiotic treatment was associated with a numerical increase in attached coliforms, although not significantly. This interaction (day 7 PI; p = 0.057) may be explained by inoculated ETEC F4 potentially profiting from the supplemented inulin and FOS, considering that as stated by Rossi et al. (2005) E. coli can use these fermentable sources of carbohydrates as growth substrates, increasing its concentration when it is seeded in faecal cultures supplemented with inulin or FOS. However, despite these effects on coliforms, it is also important to remember that in both the present study and that of Barba-Vidal et al. (2017), no significant effects (nor increases or decreases) were detected in the numbers of ETEC F4 either in the digesta or in the ileal scrapings.
Although this investigation’s results do not provide evidence of the ability of the synbiotic to reduce the number of pathogens in the intestine, some insights regarding the potential positive effects can be provided. In the Salmonella trial, the reduction of villi height associated with the pathogen challenge (Olivares-Villagómez and Van Kaer, 2018) was not attenuated by the synbiotic mixture, although a decline in the number of mitosis was seen, suggesting that the amount of damaged tissue that needed to be replaced was reduced. Furthermore, the synbiotic may have also modulated the immune response at the gut level, as an increase in the number of IEL with the synbiotic was found in the Salmonella trial (p = 0.039) at day 8 PI as well as in the ETEC F4 trial, albeit only in those animals challenged with the pathogen (P interaction = 0.086). Similarly with this probiotic strain, Barba-Vidal et al. (2017) have also reported an increase in IEL in the ileum of piglets (whether challenged or not) with Salmonella of ETEC F4. A higher presence of IEL might be regarded as beneficial considering that these cells are responsible for the healing and protection of the integrity of the intestinal epithelium as well as acting as early response effectors against mucosal pathogens (Olivares-Villagómez and Van Kaer, 2018). Supporting the immunomodulatory properties of this strain, previous studies with a murine model of rotavirus infection (Moreno-Muñoz et al., 2011) have reported increases in the levels of secretory Immunoglobulin A (IgA) in the faeces. In the light of these results, increases in ileal IEL in the present study with the synbiotic could be attributed to the probiotic strain. However, a possible additional impact of the prebiotic fibres should not be discounted, considering that in the literature several works have reported the ability of inulin and FOS to enhance local immune responses (Shukla et al., 2016; Le Bourgot et al., 2017; Myhill et al., 2018).
To summarise, combining B. longum subsp. infantis CECT 7210 with inulin and FOS was not able to reduce Salmonella or coliforms loads in the gut, as has been previously reported for the single probiotic strain. Nonetheless, this synbiotic combination was able to modify the fermentative activity of the intestine with differential effects depending on the pathogen challenge, most likely disturbing the expected cross-feeding processes between the bifidobacteria and the indigenous butyrogenic colonic bacteria. The combination of this probiotic strain with inulin and FOS was also able to increase the numbers of IEL at the ileal level, suggesting certain immunomodulatory properties. A more in-depth study of the changes produced in the gut ecosystem is necessary in order to develop a greater understanding of the role of this synbiotic combination in a scenario of well-balanced or dysbiotic microbiota.
Data Availability Statement
The original contributions presented in the study are included in the article/Supplementary Material, further inquiries can be directed to the corresponding author.
Ethics Statement
The animal study was reviewed and approved by the Animal and Human Experimental Ethical Committee of the Autonomous University of Barcelona (Permit No. CEEAH: 4026; DMAH: 10118).
Author Contributions
AR-S participated in the experimental design and was responsible for the animal trial, laboratory analysis, data analysis, and writing. LC participated in the experimental design, animal trials, data analysis, and writing. PL-C participated in animal trials and data analysis. GC-O and JM-M participated in the experimental design and contributed to data analysis and writing. SM-O participated in the experimental design, animal trials, laboratory analysis, data analysis, and writing. All authors contributed to the article and approved the submitted version.
Funding
This project has been supported by CDTI (Ministerio de Ciencia e Innovación de España) and Fondo Europeo de Desarrollo Regional (FEDER; Acronym: SMARTFOODS, project number IDI-20141206).
Conflict of Interest
GC-O and JM-M were employed by Laboratorios Ordesa SL.
The remaining authors declare that the research was conducted in the absence of any commercial or financial relationships that could be construed as a potential conflict of interest.
The handling editor declared a past co-authorship with the authors.
Publisher’s Note
All claims expressed in this article are solely those of the authors and do not necessarily represent those of their affiliated organizations, or those of the publisher, the editors and the reviewers. Any product that may be evaluated in this article, or claim that may be made by its manufacturer, is not guaranteed or endorsed by the publisher.
References
AOAC (1995). International Official Methods of Analysis of AOAC International. Washington, DC: AOAC.
Barba-Vidal, E., Castillejos, L., López-Colom, P., Rivero-Urgell, M., Moreno-Muñoz, J. A., and Martín-Orúe, S. M. (2017). Evaluation of the probiotic strain Bifidobacterium longum subsp. infantis CECT 7210 capacities to improve health status and fight digestive pathogens in a piglet model. Front. Microbiol. 8:533. doi: 10.3389/fmicb.2017.00533
Barker, H. A., D'Ari, L., and Kahn, J. (1987). Enzymatic reactions in the degradation of 5-aminovalerate by clostridium aminovalericum. J. Biol. Chem. 262, 8994–9003.
Bates, D., Mächler, M., Bolker, B., and Walker, S. (2015). Fitting linear mixed-effects models using lme4. J. Stat. Softw. 67, 1–48. doi: 10.18637/jss.v067.i01
Baxter, N. T., Schmidt, A. W., Venkataraman, A., Kim, K. S., Waldron, C., and Schmidt, T. M. (2019). Dynamics of human gut microbiota and short-chain fatty acids in response to dietary interventions with three fermentable fibers. MBio 10:e02566-18. doi: 10.1128/mBio.02566-18
Belenguer, A., Duncan, S. H., Calder, A. G., Holtrop, G., Louis, P., Lobley, G. E., et al. (2006). Two routes of metabolic cross-feeding between Bifidobacterium adolescentis and butyrate-producing anaerobes from the human gut. Appl. Environ. Microbiol. 72, 3593–3599. doi: 10.1128/AEM.72.5.3593-3599.2006
Bermudez-Brito, M., Muñoz-Quezada, S., Gomez-Llorente, C., Matencio, E., Bernal, M. J., Romero, F., et al. (2013). Cell-free culture supernatant of Bifidobacterium breve CNCM I-4035 decreases pro-inflammatory cytokines in human dendritic cells challenged with Salmonella typhi through TLR activation. PLoS One 8:e59370. doi: 10.1371/journal.pone.0059370
Boets, E., Deroover, L., Houben, E., Vermeulen, K., Gomand, S. V., Delcour, J. A., et al. (2015). Quantification of in vivo colonic short chain fatty acid production from inulin. Nutrients 7, 8916–8929. doi: 10.3390/nu7115440
Boyen, F., Haesebrouck, F., Maes, D., Van Immerseel, F., Ducatelle, R., and Pasmans, F. (2008). Non-typhoidal Salmonella infections in pigs: a closer look at epidemiology, pathogenesis and control. Vet. Microbiol. 130, 1–19. doi: 10.1016/j.vetmic.2007.12.017
Candela, M., Perna, F., Carnevali, P., Vitali, B., Ciati, R., Gionchetti, P., et al. (2008). Interaction of probiotic lactobacillus and Bifidobacterium strains with human intestinal epithelial cells: adhesion properties, competition against enteropathogens and modulation of IL-8 production. Int. J. Food Microbiol. 125, 286–292. doi: 10.1016/j.ijfoodmicro.2008.04.012
Chen, L., Gao, L. X., Huang, Q. H., Zhong, R. Q., Zhang, L. L., Tang, X. F., et al. (2017). Viscous and fermentable nonstarch polysaccharides affect intestinal nutrient and energy flow and hindgut fermentation in growing pigs. J. Anim. Sci. 95, 5054–5063. doi: 10.2527/jas2017.1662
Clements, A., Young, J. C., Constantinou, N., and Frankel, G. (2012). Infection strategies of enteric pathogenic Escherichia coli. Gut Microbes 3, 71–87. doi: 10.4161/gmic.19182
Collado, M. C., Gueimonde, M., Hernández, M., Sanz, Y., and Salminen, S. (2005). Adhesion of selected Bifidobacterium strains to human intestinal mucus and the role of adhesion in enteropathogen exclusion. J. Food Prot. 68, 2672–2678. doi: 10.4315/0362-028X-68.12.2672
Conrad, C. C., Stanford, K., Narvaez-Bravo, C., Callaway, T., and McAllister, T. (2017). Farm fairs and petting zoos: a review of animal contact as a source of zoonotic enteric disease. Foodborne Pathog. Dis. 14, 59–73. doi: 10.1089/fpd.2016.2185
De Vuyst, L., and Leroy, F. (2011). Cross-feeding between bifidobacteria and butyrate-producing colon bacteria explains bifdobacterial competitiveness, butyrate production, and gas production. Int. J. Food Microbiol. 149, 73–80. doi: 10.1016/j.ijfoodmicro.2011.03.003
Diebold, G., Mosenthin, R., Piepho, H. P., and Sauer, W. C. (2004). Effect of supplementation of xylanase and phospholipase to a wheat-based diet for weanling pigs on nutrient digestibility and concentrations of microbial metabolites in ileal digesta and feces. J. Anim. Sci. 82, 2647–2656. doi: 10.2527/2004.8292647x
Escribano, J., Ferré, N., Gispert-Llaurado, M., Luque, V., Rubio-Torrents, C., Zaragoza-Jordana, M., et al. (2018). Bifidobacterium longum subsp infantis CECT7210-supplemented formula reduces diarrhea in healthy infants: a randomized controlled trial. Pediatr. Res. 83, 1120–1128. doi: 10.1038/pr.2018.34
Farthing, M., Salam, M. A., Lindberg, G., Dite, P., Khalif, I., Salazar-Lindo, E., et al. (2013). Acute diarrhea in adults and children: a global perspective. J. Clin. Gastroenterol. 47, 12–20. doi: 10.1097/MCG.0b013e31826df662
Hartman, S., Brown, E., Loomis, E., and Russell, H. A. (2019). Gastroenteritis in children. Am. Fam. Physician 99, 159–165.
Hermes, R. G., Molist, F., Pérez, J. F., Gómez de Segura, A., Ywazaki, M., Davin, R., et al. (2013). Casein glycomacropeptide in the diet may reduce Escherichia coli attachment to the intestinal mucosa and increase the intestinal lactobacilli of early weaned piglets after an enterotoxigenic E. coli F4 challenge. Br. J. Nutr. 109, 1001–1012. doi: 10.1017/S0007114512002978
Hermes, R. G., Molist, F., Ywazaki, M., Nofrarías, M., Gomez de Segura, A., Gasa, J., et al. (2009). Effect of dietary level of protein and fiber on the productive performance and health status of piglets. J. Anim. Sci. 87, 3569–3577. doi: 10.2527/jas.2008-1241
Imamura, L., Hisamitsu, K., and Kobashi, K. (1994). Purification and characterization of beta-fructofuranosidase from Bifidobacterium infantis. Biol. Pharm. Bull. 17, 596–602.
Jensen, M. T., Cox, R. P., and Jensen, B. B. (1995). Microbial production of skatole in the hind gut of pigs given different diets and its relation to skatole deposition in backfat. Anim. Sci. 61, 293–304.
Kaplan, H., and Hutkins, R. W. (2000). Fermentation of fructooligosaccharides by lactic acid bacteria and bifidobacteria. Appl. Environ. Microbiol. 66, 2682–2684.
Koletzko, S., and Osterrieder, S. (2009). Acute infectious diarrhea in children. Dtsch. Arztebl. Int. 106, 539–548. doi: 10.3238/arztebl.2009.0539
Le Bourgot, C., Le Normand, L., Formal, M., Respondek, F., Blat, S., Apper, E., et al. (2017). Maternal short-chain fructo-oligosaccharide supplementation increases intestinal cytokine secretion, goblet cell number, butyrate concentration and Lawsonia intracellularis humoral vaccine response in weaned pigs. Br. J. Nutr. 117, 83–92. doi: 10.1017/S0007114516004268
Ley, R. E., Turnbaugh, P. J., Klein, S., and Gordon, J. (2016). Microbial ecology: human gut microbes associated with obesity. Nature 444, 1027–1031. doi: 10.1038/4441022a
Martinez, F. A., Balciunas, E. M., Converti, A., Cotter, P. D., and De Souza Oliveira, R. P. (2013). Bacteriocin production by Bifidobacterium spp. Rev. Biotechnol Adv. 31, 482–488. doi: 10.1016/j.biotechadv.2013.01.010
McDonald, J. A. K., Mullish, B. H., Pechlivanis, A., Liu, Z., Brignardello, J., Kao, D., et al. (2018). Inhibiting growth of Clostridioides difficile by restoring valerate, produced by the intestinal microbiota. Gastroenterology 155, 1495–1507. doi: 10.1053/j.gastro.2018.07.014
Meyer, D., and Stasse-Wolthuis, M. (2009). The bifidogenic effect of inulin and oligofructose and its consequences for gut health. Eur. J. Clin. Nutr. 63, 1277–1289. doi: 10.1038/ejcn.2009.64
Moens, F., Verce, M., and De Vuyst, L. (2017). Lactate- and acetate-based cross-feeding interactions between selected strains of lactobacilli, bifidobacteria and colon bacteria in the presence of inulin-type fructans. Int. J. Food Microbiol. 241, 225–236. doi: 10.1016/j.ijfoodmicro.2016.10.019
Moens, F., Weckx, S., and De Vuyst, L. (2016). Bifidobacterial inulin-type fructan degradation capacity determines cross-feeding interactions between bifidobacteria and Faecalibacterium prausnitzii. Int. J. Food Microbiol. 231, 76–85. doi: 10.1016/j.ijfoodmicro.2016.05.015
Moreno-Muñoz, J. A., Chenoll, E., Bataller, E., Ramón, D., Genovés, S., Montava, R., et al. (2011). Novel probiotic Bifidobacterium longum subsp. infantis CECT 7210 strain active against rotavirus infections. Appl. Environ. Microbiol. 77, 8775–8783. doi: 10.1128/AEM.05548-11
Myhill, L. J., Stolzenbach, S., Hansen, T. V. A., Skovgaard, K., Stensvold, C. R., Andersen, L. O., et al. (2018). Mucosal barrier and Th2 immune responses are enhanced by dietary inulin in pigs infected with Trichuris suis. Front. Immunol. 9:2557. doi: 10.3389/fimmu.2018.02557
Nofrarías, M., Manzanilla, E. G., Pujols, J., Gibert, X., Majó, N., Segalés, J., et al. (2006). Effects of spray-dried porcine plasma and plant extracts on intestinal morphology and on leukocyte cell subsets of weaned pigs. J. Anim. Sci. 84, 2735–2742. doi: 10.2527/jas.2005-414
Nyman, M. (2002). Fermentation and bulking capacity of indigestible carbohydrates: the case of inulin and oligofructose. Br. J. Nutr. 87, S163–S168. doi: 10.1079/bjnbjn/2002533
Olivares-Villagómez, D., and Van Kaer, L. (2018). Intestinal intraepithelial lymphocytes: sentinels of the mucosal barrier. Trends Immunol. 39, 264–275. doi: 10.1016/j.it.2017.11.003
Paineau, D., Respondek, F., Menet, V., Sauvage, R., Bornet, F., and Wagner, A. (2013). Effects of short-chain fructooligosaccharides on faecal bifidobacteria and specific immune response in formula-fed term infants: a randomized, double-blind, placebo-controlled trial. J. Nutr. Sci. Vitaminol 60, 167–175. doi: 10.3177/jnsv.60.167
Piñeiro, C., Piñeiro, M., Morales, J., Andrés, M., Lorenzo, E., del Pozo, M., et al. (2009). Pig-MAP and haptoglobin concentration reference values in swine from commercial farms. Vet J. Inpress. 179, 78–84. doi: 10.1016/j.tvjl.2007.08.010
Pinna, C., Vecchiato, C. G., Bolduan, C., Grandi, M., Stefanelli, C., Windisch, W., et al. (2018). Influence of dietary protein and fructooligosaccharides on fecal fermentative end-products, fecal bacterial populations and apparent total tract digestibility in dogs. BMC Vet. Res. 14:106. doi: 10.1186/s12917-018-1436-x
Poltavska, O. A., and Kovalenko, N. K. (2012). Antimicrobial activity of bifidobacterial bacteriocin-like substances. Mikrobiol. Z. 74, 32–42.
Pompei, A., Cordisco, L., Raimondi, S., Amaretti, A., Pagnoni, U. M., Matteuzzi, D., et al. (2008). In vitro comparison of the prebiotic effects of two inulin-type fructans. Anaerobe 14, 280–286. doi: 10.1016/j.anaerobe.2008.07.002
Presti, I., D'Orazio, G., Labra, M., La Ferla, B., Mezzasalma, V., Bizzaro, G., et al. (2015). Evaluation of the probiotic properties of new lactobacillus and Bifidobacterium strains and their in vitro effect. Appl. Microbiol. Biotechnol. 99, 5613–5626. doi: 10.1007/s00253-015-6482-8
R Core Team (2013). R: A Language and Environment for Statistical Computing. Vienna: R Foundation for Statistical Computing. Available at: https://www.R-project.org/
Richardson, A. J., Calder, A. G., Stewart, C. S., and Smith, A. (1989). Simultaneous determination of volatile and non-volatile acidic fermentation products of anaerobes by capillary gas chromatography. Lett. Appl. Microbiol. 9, 5–8. doi: 10.1111/j.1472-65X.1989.tb00278.x
Rodríguez-Sorrento, A., Castillejos, L., López-Colom, P., Cifuentes-Orjuela, G., Rodríguez-Palmero, M., Moreno-Muñoz, J. A., et al. (2021). Effects of the Administration of Bifidobacterium longum subsp. infantis CECT 7210 and lactobacillus rhamnosus HN001 and their Synbiotic combination With Galacto-oligosaccharides Against Enterotoxigenic Escherichia coli F4 in an early weaned piglet model. Front. Microbiol. 12:642549. doi: 10.3389/fmicb.2021.642549
Rodríguez-Sorrento, A., Castillejos, L., López-Colom, P., Cifuentes-Orjuela, G., Rodríguez-Palmero, M., Moreno-Muñoz, J. A., et al. (2020). Effects of Bifidobacterium longum Subsp. infantis CECT 7210 and Lactobacillus rhamnosus HN001, combined or not with Oligofructose-enriched inulin, on weaned pigs orally challenged with Salmonella Typhimurium. Front. Microbiol. 11:2012. doi: 10.3389/fmicb.2020.02012
Rosenbaum, M., Knight, R., and Leibel, R. L. (2015). The gut microbiota in human energy homeostasis and obesity. Trends Endocrinol. Metab. 26, 493–501. doi: 10.1016/j.tem.2015.07.002
Rossi, M., Corradini, C., Amaretti, A., Nicolini, M., Pompei, A., Zanoni, S., et al. (2005). Fermentation of fructooligosaccharides and inulin by bifidobacteria: a comparative study of pure and fecal cultures. Appl. Environ. Microbiol. 71, 6150–6158. doi: 10.1128/aem.71.10.6150-6158.2005
Scholtens, P. A., Alles, M. S., Willemsen, L. E., Van den Braak, C., Bindels, J. G., Boehm, G., et al. (2006). Dietary fructo-oligosaccharides in healthy adults do not negatively affect faecal cytotoxicity: a randomised, double-blind, placebo-controlled crossover trial. Br. J. Nutr. 95, 1143–1149. doi: 10.1079/bjn20061765
Servin, A. L. (2004). Antagonistic activities of lactobacilli and bifidobacteria against microbial pathogens. FEMS Microbiol. Rev. 28, 405–440. doi: 10.1016/j.femsre.2004.01.003
Shukla, G., Bhatia, R., and Sharma, A. (2016). Prebiotic inulin supplementation modulates the immune response and restores gut morphology in giardia duodenalis-infected malnourished mice. Parasitol. Res. 115, 4189–4198. doi: 10.1007/s00436-016-5196-x
Simon, A. K., Hollander, G. A., and McMichael, A. (2015). Evolution of the immune system in humans from infancy to old age. Proc. Biol. Sci. 282:20143085. doi: 10.1098/rspb.2014.3085
Skórka, A., Pieścik-Lech, M., Kołodziej, M., and Szajewska, H. (2017). To add or not to add probiotics to infant formulae? An updated systematic review. Ben. Microbes. 8, 717–725. doi: 10.3920/BM2016.0233
Szajewska, H., and Mrukowicz, J. Z. (2001). Probiotics in the treatment and prevention of acute infectious diarrhea in infants and children: a systematic review of published randomized, double-blind, placebo-controlled trials. J. Pediatr. Gastroenterol. Nutr. 33, S17–S25. doi: 10.1097/00005176-200110002-00004
Thapar, N., and Sanderson, I. R. (2004). Diarrhoea in children: an interface between developing and developed countries. Lancet 363, 641–653. doi: 10.1016/s0140-6736(04)15599-2
Topping, D. (1996). Short chain fatty acids produced by intestinal bacteria. Asia Pacific J. Clin. Nutr. 5, 15–19.
Tran, T. H. T., Everaert, N., and Bindelle, J. (2018). Review on the effects of potential prebiotics on controlling intestinal enteropathogens Salmonella and Escherichia coli in pig production. J. Anim. Physiol. Anim. Nutr. 102, 17–32. doi: 10.1111/jpn.12666
Van de Wiele, T., Boon, N., Possemiers, S., Jacobs, H., and Verstraete, W. (2007). Inulin-type fructans of longer degree of polymerization exert more pronounced in vitro prebiotic effects. J. Appl. Microbiol. 102, 452–460. doi: 10.1111/j.1365-2672.2006.03084.x
Van der Beek, C. M., Canfora, E. E., Kip, A. M., Gorissen, S. H. M., Olde Damink, S. W. M., van Eijk, H. M., et al. (2018). The prebiotic inulin improves substrate metabolism and promotes short-chain fatty acid production in overweight to obese men. Metabolism 87, 25–35. doi: 10.1016/j.metabol.2018.06.009
Van Soest, P. J., Robertson, J. B., and Lewis, B. A. (1991). Methods for dietary fiber, neutral detergent fiber, and non-starch polysaccharides in relation to animal nutrition. J. Dairy Sci. 74, 3583–3597. doi: 10.3168/jds.S0022-0302(91)78551-2
Vandeputte, D., Falony, G., Vieira-Silva, S., Wang, J., Sailer, M., Theis, S., et al. (2017). Prebiotic inulin-type fructans induce specific changes in the human gut microbiota. Gut 66, 1968–1974. doi: 10.1136/gutjnl-2016-313271
Wagner, R. D., Johnson, S. J., and Kurniasih Rubin, D. (2009). Probiotic bacteria are antagonistic to Salmonella enterica and Campylobacter jejuni and influence host lymphocyte responses in human microbiota-associated immunodeficient and immunocompetent mice. Mol. Nutr. Food Res. 53, 377–388. doi: 10.1002/mnfr.200800101
Wang, X., and Gibson, G. R. (1993). Effects of the in vitro fermentation of oligofructose and inulin by bacteria growing in the human large intestine. Appi. Bacteriol. 75, 373–380.
Keywords: probiotic, piglet, ETEC, Salmonella, fructo oligosaccharides, prebiotic, inulin, bifidobacteria
Citation: Rodríguez-Sorrento A, Castillejos L, López-Colom P, Cifuentes-Orjuela G, Moreno-Muñoz JA and Martín-Orúe SM (2022) Assessment of the Effects of the Synbiotic Combination of Bifidobacterium longum subsp. infantis CECT 7210 and Oligofructose-Enriched Inulin Against Digestive Bacterial Infections in a Piglet Model. Front. Microbiol. 13:831737. doi: 10.3389/fmicb.2022.831737
Edited by:
Diana Luise, University of Bologna, ItalyReviewed by:
Ester Arévalo Sureda, University of Liège, BelgiumCorina-Diana Ceapa, Universidad Nacional Autónoma de México, Mexico
Copyright © 2022 Rodríguez-Sorrento, Castillejos, López-Colom, Cifuentes-Orjuela, Moreno-Muñoz and Martín-Orúe. This is an open-access article distributed under the terms of the Creative Commons Attribution License (CC BY). The use, distribution or reproduction in other forums is permitted, provided the original author(s) and the copyright owner(s) are credited and that the original publication in this journal is cited, in accordance with accepted academic practice. No use, distribution or reproduction is permitted which does not comply with these terms.
*Correspondence: Lorena Castillejos, bG9yZW5hLmNhc3RpbGxlam9zQHVhYi5jYXQ=