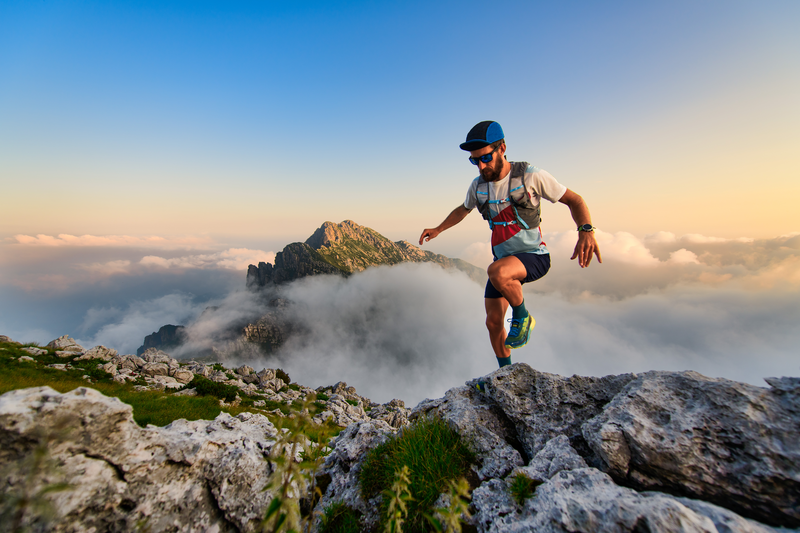
94% of researchers rate our articles as excellent or good
Learn more about the work of our research integrity team to safeguard the quality of each article we publish.
Find out more
ORIGINAL RESEARCH article
Front. Microbiol. , 30 March 2022
Sec. Evolutionary and Genomic Microbiology
Volume 13 - 2022 | https://doi.org/10.3389/fmicb.2022.828990
This article is part of the Research Topic Insights in Evolutionary and Genomic Microbiology: 2021 View all 12 articles
Genomic information for bacteria within the genus Rahnella remains limited. Rahnella sp. JZ-GX1 was previously isolated from the Pinus massoniana rhizosphere in China and shows potential as a plant growth-promoting (PGP) bacterium. In the present work, we combined the GridION Nanopore ONT and Illumina sequencing platforms to obtain the complete genome sequence of strain JZ-GX1, and the application effects of the strain in natural field environment was assessed. The whole genome of Rahnella sp. JZ-GX1 comprised a single circular chromosome (5,472,828 bp, G + C content of 53.53%) with 4,483 protein-coding sequences, 22 rRNAs, and 77 tRNAs. Based on whole genome phylogenetic and average nucleotide identity (ANI) analysis, the JZ-GX1 strain was reidentified as R. victoriana. Genes related to indole-3-acetic acid (IAA), phosphorus solubilization, nitrogen fixation, siderophores, acetoin, 1-aminocyclopropane-1-carboxylate (ACC) deaminase, gamma-aminobutyric acid (GABA) production, spermidine and volatile organic compounds (VOCs) biosynthesis were present in the genome of strain JZ-GX1. In addition, these functions were also confirmed by in vitro experiments. Importantly, compared to uninoculated control plants, Pyrus serotina, Malus spectabilis, Populus euramericana (Dode) Guinier cv. “San Martino” (I-72 poplar) and Pinus elliottii plants inoculated with strain JZ-GX1 showed increased heights and ground diameters. These findings improve our understanding of R. victoriana JZ-GX1 as a potential biofertilizer in agriculture.
According to the latest statistics of the United Nations, the global population is expected to reach 9.7 billion by 2050 (Goswami and Deka, 2020). To meet the growing demand for food, excessive use of chemical fertilizers and pesticides in agricultural production has damaged soil health and ecology (Ruzzi and Aroca, 2015). At the same time, abiotic stresses, including drought, high salinity, and toxic heavy metals are prevalent worldwide (Rosier et al., 2018; Mondal et al., 2021). It is estimated that abiotic stresses affect approximately 10 hectares of land per minute, with three hectares affected by soil salinization around the world (Ha-Tran et al., 2021). Similar to high salinity, extremely dry conditions lead to an annual harvest loss of 17% of harvests in tropical, arid and semiarid areas (Chandra et al., 2021). Trees suffer even more from the recurrence of abiotic stress owing to their long lifecycle and the global forest cover is gradually decreasing (Teshome et al., 2020). Therefore, appropriate measures need to be taken to make the whole agricultural and forestry ecosystem develop in the direction of sustainable intensification (Pretty et al., 2018).
With progress in the development of genetic tools and technology, our understanding of the “black box” of microorganisms in soil has become more transparent, and we are at a moment when rhizosphere microbial research is likely to be of great application value (Olenska et al., 2020). A large number of studies have determined the important contributions of specific rhizosphere growth-promoting bacteria to sustainable agricultural production. The plant growth-promoting rhizobacteria (PGPR) reported to date are mainly concentrated in Bacillus, Pseudomonas, Enterobacter, Burkholderia, Klebsiella, Azospirillum, and Serratia (Liu et al., 2020a; Alexandre et al., 2021; Kusale et al., 2021; Wang et al., 2021; Yasmin et al., 2021). These rhizosphere colonizing microorganisms employ a variety of mechanisms to alleviate adverse soil conditions, thus promoting plant growth. They mobilize soil nutrients by secreting organic acids, siderophores, fixed nitrogen, and dissolved phosphate (Kong et al., 2020a; Liu et al., 2020b; Mariotti et al., 2021). They can also secrete a series of substances, such as 1-aminocyclopropane-1-carboxylate (ACC deaminase), volatiles, spermidine, gamma-aminobutyric acid (GABA), and phytohormones to induce plant resistance to abiotic stress (Zhou et al., 2016b; Liu et al., 2020c; Nascimento et al., 2020; Choudhury et al., 2021). Up to now, PGPR strains have been studied in detail in agricultural crops, but their application in woody plants has not been well-explored.
Strain JZ-GX1 was screened with a high yield phytase as the index in our laboratory and was first classified as Rahnella aquatilis based on the 16S rRNA gene sequence. However, with the advancement of taxonomic research, many members of Rahnella have been gradually reported, so it is necessary to identify strain JZ-GX1 at a greater resolution. Previous studies have reported that the JZ-GX1 strain exhibited a significant growth-promoting effect on poplar and corn in greenhouse conditions (Li et al., 2013, 2021), so does it have the characteristics of plant growth-promoting in addition to the degradation of phytate? Moreover, the growth-promoting effect of this strain on woody plants in the field needs to be further studied. Therefore, the whole genome sequencing technique was used in this study to provide new insights into the molecular and genetic mechanism of Rahnella sp. JZ-GX1 in promoting plant growth. Furthermore, two ecologically important trees and two economic trees were selected for use in field experiments to further explore the growth-promoting ability of strain JZ-GX1 under natural conditions. The overarching goal was to assess this strain as a new microbial inoculant for sustainable agricultural practices.
Rahnella sp. JZ-GX1 was isolated from the rhizosphere soil of a 28-year-old Pinus massoniana in Guangxi, China, on April 8, 2011, and was deposited in the Chinese Center for Type Culture Collection (Accession No. CCTCC M2012439). This strain was routinely incubated in Luria-Bertani (LB) liquid media at 28°C with shaking for 24 h. Genomic DNA was extracted using a modified freeze–thawing method (Chen et al., 2020).
Rahnella sp. JZ-GX1 genome was sequenced by the single molecule real-time (SMRT) method at Biomarker Technology Co., Ltd. (Beijing, China). The genome assembly was created using Nanopore long reads, and Illumina paired-end sequences were used for base and indel correction. The filtered subreads was assembled using Canu v1.5 software. Finally, the Pilon software is used to further correct the assembled genome using second generation data, and the final genome with high accuracy was obtained.
The predicted gene sequences were compared with the Clusters of Orthologous Groups (COG), Kyoto Encyclopedia of Genes and Genomes (KEGG), Swiss-Prot, TrEMBL, non-redundant (Nr), and other functional databases by BLAST, and gene functional annotations were obtained (Tatusov et al., 2003). Based on the results from comparison with the Nr database (Aziz et al., 2008), the application software Blast2GO was used to annotate functions according to the GO database. HMMER software was applied for functional annotation based on the Pfam database (El-Gebali et al., 2019). In addition, the functions of genes were annotated and analyzed using COG and KEGG metabolic pathway enrichment analysis.
A phylogenetic tree was constructed by combining the genome of JZ-GX1 with a set of closely related genomes selected from all public KBase genomes using the Insert Genome Into Species Tree 2.1.10 tool.1 Relatedness was determined by alignment similarity with a selected subset of COG domains. Next, a phylogenetic tree was reconstructed using FastTree (version 2.1.10). Average Nucleotide Identity (ANI) analysis was performed between strain JZ-GX1 and other Rahnella isolates included in the phylogenetic tree using an online ANI calculator.2
The chromosome, plasmid 1 and plasmid 2 sequences are available under NCBI BioProject PRJNA720502, with accession numbers CP089919, CP089920, and CP089921, respectively.
To induce the production of indole-3-acetic acid (IAA), 250 μL of a culture of strain JZ-GX1 grown overnight in LB medium was transferred into 25 mL of TSB medium supplemented with sterile-filtered L-tryptophan (500 μg/mL). The liquid culture was grown at 28°C and 180 rpm, and cells were separated from the exhausted medium by centrifugation (10,000 × g for 15 min). The concentration of IAA in the bacterial solution was sampled and determined at 96 h after inoculation. The collected supernatant was filtered through 0.22 μm cellulose acetate filters (DISMIC®; Frisenette ApS, Knebel, Denmark). The bacterial supernatant (1 mL) was mixed with 4 mL of Salkowski’s reagent (50 mL of 35% HClO4, 1 mL of 0.5 M FeCl3) and allowed to rest for 30 min in the dark. After incubation, absorbance was measured at 530 nm (pink color) (T60 UV-VIS Spectrophotometer; PG Instruments, Leicester, United Kingdom) and quantified using the calibration curve of an IAA standard with linear regression analysis (Kang et al., 2020). The ACC deaminase activity was determined by previously described method (Penrose and Glick, 2003). The experiment was repeated two times, and each treatment was conducted in triplicate.
For siderophores production, strain was cultivated in a Chrome Azurol-S agar assay (Schwyn and Neilands, 1987), and positive results were indicated by the formation of a clear orange zone around the colonies. Burkholderia pyrrocinia JK-SH007 was used as a positive control.
Nitrogen fixation ability using N-free Ashby medium agar plates contained 5 g of glucose, 5 g of mannitol, 0.1 g of CaCl2⋅2H2O, 0.1 g of MgSO4⋅7H2O, 0.005 g of Na2MoO4⋅2H2O, 0.9 g of K2HPO4, 0.1 g of KH2PO4, 0.01 g of FeSO4⋅7H2O, 5.0 g of CaCO3, 15.0 g of agar in 1 L of distilled water, the final pH was adjusted to 7.3 (Liu et al., 2017).
The rhizosphere bacteria were assessed for potential inorganic phosphate solubilization on the National Botanical Research Institute’s phosphate (NBRIP) growth medium [per liter: 10.0 g of glucose, 5.0 g of MgCl2⋅6H2O, 0.25 g of MgSO4⋅7H2O, 0.2 g of KCl and 0.1 g of (NH4)2SO4] supplemented with Ca3(PO4)2 at a final concentration of 0.5% (Chandran et al., 2014).
Organophosphorus detection medium contained 0.1 g of Calcium phytate, 10.0 g of Glucose, 5.0 g of MgCl2, 0.2g of KCl, 0.25 g of MgSO4, 0.1 g of (NH4)2SO4, 16.0 g of agar in 1 L of distilled water, the final pH was adjusted to 7.3 (Shen et al., 2016).
Potassium dissolving ability using modified Aleksandrov medium including 5.0 g of glucose, 0.5 g of MgSO4⋅7H2O, 0.1 g of CaCO3, 0.006 g of FeCl3; 2.0 g of Ca3(PO4)2, 3.0 g of potassium aluminium silicate and 20.0 g agar in 1 L of distilled water, the final pH was adjusted to 7.2 (Etesami et al., 2017). The JZ-GX1 colonies were stabbed in triplicate on all agar plates using sterile toothpicks and positive results were expressed by the presence of solubilization zone around bacterial colony after 120 h of incubation at 28°C.
Each plate detection was composed of nine replicates, and the experiment was repeated twice.
The strain was inoculated in Methyl Red-Voges-Proskauer (MR-VP) broth (glucose 0.5 g, K2HPO4 0.2 g, water 1,000 mL, pH 7.2-7.4). After shaker culture at 30°C for 2 days, the culture medium was mixed with 40% NaOH, a small amount of creatine was added and the mixture was heated in a boiling water bath to test for a positive reaction (shown as a red color) (Rath et al., 2018). Escherichia coli DH5α was used as a negative control. Each treatment was composed of three replicates, and the experiment was repeated twice.
Genomic DNA was extracted using a modified freeze–thawing method (Chen et al., 2020). Then, polymerase chain reaction (PCR) assays were used to detect the acdS, gadB, gadD, gabT, speA, speD, G1pase, pstA, pstC, and nirB genes by designing PCR primers based on the genome sequence of JZ-GX1 strain. The primers used in the experiment are listed in Supplementary Table 1.
Arabidopsis thaliana Col-0 was used in this study. Seeds were surface sterilized and sown on 1/2 MS agar medium. The Petri dishes were positioned vertically in a growth chamber under a long photoperiod (16 h light/8 h dark) and 70% relative humidity at 22°C. Seven days later, five seedlings were transferred to a new 1/2 MS agar medium, and at the same time, 10 μL of bacterial solution was added to the other compartment, and LB medium without bacterial solution was used as the control. After the bacterial solution was homogeneously distributed in the culture medium, the Petri dish was sealed with parafilm and grew vertically in the same conditions. After 14 days, A. thaliana seedlings were removed and weighed with a 1/10,000 balance; the length of the main root of A. thaliana was measured and recorded with a Vernier calliper. Then, the seedlings were placed in a Petri dish filled with clear water so that the roots could be fully elongated, and the number of lateral roots was counted and recorded. The root hair of A. thaliana was photographed with a Zeiss stereomicroscope (Zeiss Microscope System Standard 16; Carl Zeiss Ltd.; Wetzlar, Germany) (Bhattacharyya et al., 2015; Perez-Flores et al., 2017; Li F. et al., 2020). Each treatment was composed of ten replicates, and the experiment was repeated twice.
The antifungal effect of the volatile organic compounds (VOCs) produced by Rahnella sp. JZ-GX1 was evaluated using I plates (90 mm in diameter). I plates are plastic Petri dishes with a partition in the center that divides the plate into two parts. The microorganisms grown on side thus cannot spread to the other side. However, gas exchange can proceed normally. One compartment of the I plates, containing LB agar medium, was inoculated with Rahnella sp. JZ-GX1. The other compartment of the I plates, containing PDA medium, was inoculated with pathogenic fungi using mycelial discs (6 mm diameter). I plates with pathogenic fungi on one half served as the control. The fungal plant pathogens used in this study were preserved in the Forest Pathology Laboratory of Nanjing Forestry University. All plates were sealed with Parafilm and cultured for 5–7 days at 25°C (Zhang et al., 2019c,Zhang et al., 2021). Each treatment was composed of three replicates, and the experiment was repeated twice.
The experiment was conducted at nurseries in Changzhou (31°47′N, 119°58′E) and Suqian (33°72′N, 118°68′E), Jiangsu Province. The areas have a subtropical monsoon climate, with an average annual precipitation of approximately 1,056 mm and an annual average temperature of approximately 15°C. The soil type is paddy soil. Two-year-old Pyrus serotina, Malus spectabilis, and I-72 poplar and 1-year-old Pinus elliottii were selected as indicator tree species. Three replicates were applied following in a randomized block design. Each plant was irrigated with 800 mL of bacterial liquid (including 50 mL of bacterial suspension), and seedlings that were not inoculated with JZ-GX1 were used as controls, with 90 plants per treatment. The whole experiment was carried out in open air, with drip irrigation provided when the weather was dry. After 8 months, plant height and ground diameter were recorded.
Analysis of variance (ANOVA) followed by Duncan’s multiple comparison test was performed for comparisons among all means, and Student’s t-test was performed for comparison of pairs of means with SPSS 22.0 software (IBM Inc., Armonk, NY, United States). Error bars show the standard deviation and significance was defined as p < 0.01. Graphs were generated using GraphPad Prism 8.0 (GraphPad Software, Inc., United States).
The complete genome of Rahnella sp. JZ-GX1 contained one gapless circular chromosome of 5,472,828 bp and two plasmids, with a G + C content of 53.53%. Twenty two rRNAs, and 77 tRNAs were found in the chromosome, including 0 pseudogenes, 12 CRISPR sequences, 12 gene islands, and two pre-bacteriophages (Figure 1). A total of 4,483 protein-coding genes were predicted, of which 88.85% were annotated to COG functional categories, 80.68% to GO functional categories and 68.77% to KEGG pathways. Details are presented in Supplementary Table 2.
Figure 1. The whole genome of Rahnella victoriana JZ-GX1. The genome map is composed of seven circles. From the outer circle to inner circle, each circle displays information regarding the genome of (1) forward CDS, (2) reverse CDS, (3) forward COG function classification, (4) reverse COG function classification, (5) nomenclature and locations of predictive secondary metabolite clusters, (6) G + C content, and (7) GC skew.
Phylogenetic analysis based on the whole genome further confirmed the close taxonomic relationship of strain JZ-GX1 with Rahnella victoriana (Figure 2). On the basis of ANI, the similarity between genomes of strain JZ-GX1 and other R. victoriana strains was approximately 98%; when the strain was compared with other species, the ANI threshold was less than 90% (Table 1). All the data indicated that strain JZ-GX1 belongs to R. victoriana rather than R. aquatilis, as formerly proposed.
Figure 2. Taxonomic assignment of strain JZ-GX1. Escherichia coli 042 uid161985 was used as outgroup. Bar, 0.02 substitutions per nucleotide position.
Table 1. Average nucleotide identity (ANI) values based on alignment of the whole genome of strain JZ-GX1 and the most closely related members of the genus Rahnella.
Based on the COG analysis, the identified proteins were classified into 25 functional categories. Among these proteins, in addition to the high content of COG R (general function), the content of COG E (amino acid transport and metabolism) was the highest, followed by COG K (transcription) and COG G (heredity and basic metabolism), indicating that the functional proteins encoded by the cell genome play an important role in maintaining cellular life and genetic metabolism (Figure 3A).
Figure 3. Functional categories of R. victoriana JZ-GX1. (A) Clusters of Orthologous Groups of proteins (COGs) annotation and (B) Clusters of kyoto encyclopedia of genes and genomes (KEGG) annotation.
Using gene KEGG annotations, 20 functional classes were predicted in the genome of R. victoriana JZ-GX1, which can be divided into five categories: (1) systems related to cell processes, such as cell movement (68); (2) systems related to environmental information processing, such as signal transduction (139) and transmembrane transport (340); (3) systems related to genetic information processing; (4) systems related to immunity (39); and (5) systems related to metabolism. Among them, metabolism-related systems accounted for the highest proportion of R. victoriana JZ-GX1 metabolic pathways (Figure 3B). These results showed that JZ-GX1 strain has strong genetic potential to synthesize secondary metabolites, which is consistent with the above protein annotation results.
Functional analysis of R. victoriana JZ-GX1 genome revealed the presence of several genes contributing directly to plant hormones and nutrient availability. We identified 11 genes encoding key enzymes involved in the synthesis and secretion of IAA through the IPyA (ipdC) and IAM (amiE) pathways. For the N cycle, genes encoding nodulation protein (nodN), nitrate reductase catalytic subunit (nasA), and nitrite transporter (nirC) were detected. These enzymes are involved in the denitrification process, catalyzing the conversion of nitrate to nitrite to nitric oxide, followed by the conversion of nitric oxide to nitrous oxide. Strain JZ-GX1 possessed genes encoding the synthesis of enterobactin and rhizobactin, which form the main part of catechol and hydroxamic acid type siderophores, and a ferric enterobactin transport system (fepACDG) was detected. The annotation information also revealed the presence of several gene clusters involved in mineral phosphate solubilization, including the phosphate-specific transport operon (pstAC) as well as a two-component signal transduction system for phosphate uptake consisting of phoPRH genes (Table 2).
Table 2. Genes related to plant growth-promoting activities in the Rahnella victoriana JZ-GX1 genome.
In addition to the genes that directly promote growth, many genes responsible for synthesis and transport of compatible solutes were predicted, such as Na+/H+ antiporter (NhaAB), glycine betaine transporter (opuD), proline/betaine transporter (proP), and exopolysaccharide production protein. Genes encoding acetolactate synthase (alsD and alsS) and 2,3-butanediol (bdh and budABC), which are involved in the synthesis of acetoin, a volatile molecule associated with plant growth promotion, were detected in the genome. The gene gadB responsible for GABA production, as well as gabT and gabD, encoding GABA aminotransferase and succinate-semialdehyde dehydrogenase involved in GABA degradation, were predicted. Moreover, arginine decarboxylase (speA), agmatinase (speB), S-adenosylmethionine decarboxylase proenzyme (speD) and spermidine synthase (speE), related to spermidine biosynthesis, were present in R. victoriana JZ-GX1 (Table 3).
Table 3. Genes related to plant stress resistance improving in the Rahnella victoriana JZ-GX1 genome.
To verify the accuracy of genome sequencing, we investigated the PGP characteristics of strain JZ-GX1 in vitro. The results demonstrated that R. victoriana JZ-GX1 appeared as a yellow halo on the CAS plate and Aleksandrov plate, indicating that it can secrete siderophores and dissolve potassium (Figures 4A,F). The dissolution circle method showed that JZ-GX1 has the ability to degrade insoluble organic phosphorus and inorganic phosphorus at the same time (Figures 4B,C). Moreover, it could grow on Ashby medium, which proved that it has a certain autogenous nitrogen fixation ability (Figure 4E). The VP test showed that R. victoriana JZ-GX1 could produce acetoin (Figure 4D). In addition, the IAA content and ACC deaminase activity were 9.0934 μg/mL and 17.9794 mmol α-keto/mg protein/h, respectively (Figure 4G).
Figure 4. Detection of plant growth-promoting characteristics of Rahnella victoriana JZ-GX1. (A) Siderophore production, (B) inorganic phosphorus dissolution, (C) organophosphorus dissolution, (D) acetoin production, (E) nitrogen fixation, (F) potassium solubilization, (G) IAA and ACC deaminase production, and (H) detection of key gene related to PGP traits in JZ-GX1 by PCR amplification (lane M, DNA marker; 1, acdS; 2, gadB; 3, gadD; 4, gabT; 5, speA; 6, speD; 7, G1pase; 8, pstA; 9, pstC; 10, nirB). One-way ANOVA was performed, and Duncan’s post-hoc test was applied. Different letters indicate statistically significant differences (p < 0.01) among treatments (n = 3).
We tested the JZ-GX1 strain for the presence of operons for the biosynthesis of ACC deaminase, GABA, and spermidine, the fixation of nitrogen and the dissolution of phosphorus by PCR using specific primers. A fragment of the predicted size for each of these compounds was observed in the DNA of the genome sequence, which indicates that the strain produces these compounds. Fragments of the predicted sizes for acdS (∼1,002 bp), gadB (∼1,473 bp), gadD (∼1,392 bp), gabT (∼1,269 bp), speA (∼1,983 bp), speD (∼795 bp), G1pase (∼1,302 bp), pstA (∼846 bp), pstC (∼957 bp), and nirB (∼2,550 bp) were amplified from strain JZ-GX1 DNA (Figure 4H).
To investigate the effect of VOCs produced by R. victoriana JZ-GX1 on plant growth, we performed I-plate assays as these avoid any physical contact between A. thaliana and R. victoriana JZ-GX1. Biomass production was stimulated in Arabidopsis plants exposed to R. victoriana JZ-GX1 in this manner (Figure 5A). Following exposure to R. victoriana JZ-GX1, the fresh weights of shoots and roots increased significantly by 1.71- and 1.68-fold, respectively, compared to the controls (Figures 5B,C). In addition, Arabidopsis exposed to strain JZ-GX1 VOCs showed denser root hairs (Figure 5D). The bacterial VOCs significantly inhibited primary root growth and stimulated lateral root formation at the same time (Figures 5E,F). These data indicate that VOCs from JZ-GX1 can promote plant growth and modulate rhizogenesis in A. thaliana.
Figure 5. Effect of Rahnella victoriana JZ-GX1 VOCs on plant growth and root morphology of Arabidopsis thaliana compared to the control (CK). (A) Plant phenotype, (B) shoot fresh weight, (C) root fresh weight, (D) root morphology, (E) primary root length, (F) lateral root length. The scale is 1,000 μm. Different letters indicate statistically significant differences among treatments (Student’s t-test, p < 0.01) (n = 100).
The VOCs emitted by PGPR often show an inhibitory effect on pathogens. As shown in Figure 6, strain JZ-GX1 VOCs showed significant antifungal activities against six plant pathogenic fungi, namely, Guignardia camelliae, Fusicoccus aesculin, Fusarium oxysporum, Alternaria tenuissima, Sphaeropsis sapinea, and Verticillium dahlia. The fungi not exposed to R. victoriana JZ-GX1 were covered with plates, while the hyphae of all test fungi except V. dahlia in the presence of VOCs were limited to one side of the partition plate, and the pigments of F. oxysporum and V. dahlia were inhibited.
Figure 6. The antifungal spectrum of Rahnella victoriana JZ-GX1 VOCs. Plate on the left untreated, plate on the right treated with VOCs. (A) Guignardia camelliae, (B) Fusicoccus aesculin, (C) Fusarium oxysporum, (D) Alternaria tenuissima, (E) Sphaeropsis sapinea, and (F) Verticillium dahlia.
Eight months after inoculation, the microbial agent had a marked growth-promoting effect on the height and ground diameter of I-72 poplar compared with that of the CK, with growth rates of 9.7 and 16.1%, respectively. The growth rates of plant height and ground diameter of P. elliottii inoculated with strain JZ-GX1 were 23.6 and 14.6% greater than those of uninoculated trees, respectively. The height of pear trees with root application of strain JZ-GX1 was 14% higher than that of the control trees, but the treatment had no effect on ground diameter. For M. spectabilis, the plant height after inoculation increased to varying degrees compared with the ground diameter, but the difference was not significant (Table 4). These results of this field experiment support the discovery of multiple PGP mechanisms in the genome of R. victoriana JZ-GX1.
Plant growth-promoting rhizobacteria have been demonstrated to produce the auxin IAA to enhance plant growth by controlling many physiological processes, such as cell division, vascular tissue differentiation and root elongation (Liu et al., 2019; Luziatelli et al., 2020). However, the biosynthetic pathways of IAA in bacteria are different. Five tryptophan-dependent IAA synthesis pathways have been identified in bacteria, namely, the indole-3-acetonitrile (IAN), tryptamine (TAM), indole-3-acetamide (IAM), indole-3-pyruvate (TPyA), and tryptophan side-chain oxidase (TSO) pathways, and a tryptophan-independent pathway (Zhang et al., 2019b). It has been reported that R. aquatilis ZF7 can synthesize IAA through the IPyA pathway (Yuan et al., 2020), and in this study, R. victoriana JZ-GX1 was predicted to contain two IAA production pathways (TPyA and IAM). PGPR usually interacts with plant root exudates and synthesizes IAA with the corresponding tryptophan as the precursor (Bhattacharjee et al., 2012; Liu et al., 2016). The JZ-GX1 strain has more than one IAA synthesis pathway, which indicates that it has a stronger ability to secrete IAA than the same microorganism.
Phosphorus (P) is one of the most limiting nutrients required for the growth and development of plants (Zeng et al., 2017; Li et al., 2018). Phytic acid mineralizing rhizobacteria (PMR) play an important role in promoting the dissolution of insoluble organic phosphorus in soil (Kour et al., 2021). R. victoriana JZ-GX1 was initially isolated with high phytate-degrading activity as an index, which can promote an increase in the total P content in maize (Li et al., 2013, 2021). However, the mechanism of organophosphorus dissolution has not been clearly revealed. The preliminary determination of the enzymatic properties of R. victoriana JZ-GX1 showed that it has a strong ability to hydrolyze phytate under acidic conditions (Li and Wu, 2014). In this study, we found that R. victoriana JZ-GX1 harbors two phytase-encoding genes (acid glucose-1-phosphatase and 4-phytase) but lacks appA-like phytase genes. The results of this study are consistent with previously reported enzymatic properties. Until now, there has been very little information available regarding the regulation of phytate-degrading gene expression in bacteria. The presence of phytase genes in this genome could open up opportunities for future molecular cloning and application studies of phytase from R. victoriana JZ-GX1.
In the rhizosphere, long-distance interactions through VOCs are an important method of signal transmission between bacteria and plants (Fincheira and Quiroz, 2018; Kong et al., 2021; Li et al., 2021). To date, many bacteria have been shown to stimulate plant growth by releasing volatiles. For example, B. subtilis SYST2-derived VOCs can promote tomato growth both in vitro and in pot experiments by triggering growth hormone activity (Tahir et al., 2017). Similar findings were obtained for Arabidopsis treated with volatiles from B. megaterium B55, and the combined effects of blended compounds increased leaf number and leaf surface area up to 2- and 4-fold, respectively, compared with those of the control (Meldau et al., 2012). B. amyloliquefaciens GB03 generates 3-hydroxy-2-butanone (acetoin) and 2,3-butanediol as its primary volatiles, which were shown to promote plant growth in trials on Arabidopsis (Ryu et al., 2003). The role of these two compounds in plant growth promotion was also confirmed by the addition of the pure acetoin and a mutant bacterium lacking a 2,3-butanediol biosynthesis gene (Farag et al., 2006). In our research, we also detected acetoin in the JZ-GX1 strain by gene prediction and physiological detection, and confirmed that JZ-GX1-derived VOCs could promote an increase in fresh weight and root development without contact with A. thaliana. Although the specific mechanism needs to be further explored, this is the first report on the biological activity in plant growth promotion of the VOCs produced by Rahnella sp.
When plants are faced with abiotic stress, several important metabolites, including osmotic regulators and active molecules, accumulate in large quantities (Zhou et al., 2017). Spermidine (Spd) is an important plant growth regulator and has also been identified as a protector against various abiotic stressors, such as high salinity, drought, low temperature, and iron deficiency (Zhou et al., 2016a; Li G. et al., 2020; Qian et al., 2021). Reportedly, B. subtilis STU6 induces and uses plant arginine to produce more Spd, which promotes bacterial colonization and absorption by the host, thus enhancing the iron acquisition system and cell wall iron remobilization in a NO-dependent manner (Zhou et al., 2019). Three genes of spermidine synthase (GE00638, GE00674, and GE00675) were identified in strain JZ-GX1. Furthermore, GABA plays a vital role in plant responses to multiple stressors (Al-Quraan et al., 2013). Guo et al. (2020) found that GABA can improve the tolerance of cucumber to iron deficiency in an auxin-dependent manner. In a previous study, we demonstrated the potential roles of R. victoriana JZ-GX1 in alleviating iron deficiency-induced chlorosis in C. camphora (Kong et al., 2020b). Here, strain JZ-GX1 was found to possess the genes encoding glutamate decarboxylase, which is involved in GABA production and degradation (GE04524, GE04546, and GE04695). Combined with the previous results, we further clarified the potential mechanism by which R. victoriana JZ-GX1 promotes iron uptake in plants. However, the contents of these two substances in plants should be determined, and the genes for the synthesis of these two substances by R. victoriana JZ-GX1 should be knocked out to prove this conjecture.
At present, the genus Rahnella consists of six closely related species, including R. aquatilis, R. variigena, R. victoriana, R. bruchi, R. woolbedingensis, and R. inusitata (Yuan et al., 2020). Among them, R. aquatilis has been widely reported to promote plant growth (Peng et al., 2019; Zhang et al., 2019a; Sun et al., 2020). There are few reports regarding the other species, and so far the only reports of R. victoriana was considered associated with decline symptoms on oak and hornbeam (Yousef et al., 2019). In this study, R. victoriana JZ-GX1 was proven to be a PGPR strain with a variety of beneficial features, including IAA production, phosphate solubilization, nitrogen fixation, siderophores, acetoin, ACC deaminase, GABA production, spermidine and VOCs biosynthesis. In addition, the ability of strain JZ-GX1 to promote forest growth has also been well-verified in complex field environments. Overall, the data obtained in this study provide more clues for the potential application of R. victoriana JZ-GX1 in the development of eco-friendly biofertilizers, which can promote soil fertility and crop yield.
The datasets presented in this study can be found in online repositories. The names of the repository/repositories and accession number(s) can be found at: https://www.ncbi.nlm.nih.gov/, PRJNA720502.
W-LK completed the data analysis and the first draft of the manuscript. W-LK, W-YW, and S-HZ were the finishers of the experimental research. W-YW participated in the experimental result analysis. X-QW directed experimental design, data analysis, and manuscript writing and revision. All authors read and agreed on the final version of manuscript.
This work was supported by the National Key Research and Development Program of China (2017YFD0600104), the Priority Academic Program Development of the Jiangsu Higher Education Institutions (PAPD), and the Postgraduate Research and Practice Innovation Program of Jiangsu Province (KYCX20_0872).
The authors declare that the research was conducted in the absence of any commercial or financial relationships that could be construed as a potential conflict of interest.
All claims expressed in this article are solely those of the authors and do not necessarily represent those of their affiliated organizations, or those of the publisher, the editors and the reviewers. Any product that may be evaluated in this article, or claim that may be made by its manufacturer, is not guaranteed or endorsed by the publisher.
The Supplementary Material for this article can be found online at: https://www.frontiersin.org/articles/10.3389/fmicb.2022.828990/full#supplementary-material
Alexandre, F. S., Della Flora, L. V., Henrique, I. G., Da Silva, D. C., Mercedes, A. P., Silva, A. C., et al. (2021). Arbuscular mycorrhizal fungi (Rhizophagus clarus) and rhizobacteria (Bacillus subtilis) can improve the clonal propagation and development of teak for commercial plantings. Front. Plant Sci. 12:628769. doi: 10.3389/fpls.2021.628769
Al-Quraan, N. A., Sartawe, F. A., and Qaryouti, M. M. (2013). Characterization of gamma-aminobutyric acid metabolism and oxidative damage in wheat (Triticum aestivum L.) seedlings under salt and osmotic stress. J. Plant Physiol. 170, 1003–1009. doi: 10.1016/j.jplph.2013.02.010
Aziz, R. K., Bartels, D., Best, A. A., DeJongh, M., Disz, T., Edwards, R. A., et al. (2008). The RAST server: rapid annotations using subsystems technology. BMC Genomics 9:75. doi: 10.1186/1471-2164-9-75
Bhattacharjee, R. B., Jourand, P., Chaintreuil, C., Dreyfus, B., Singh, A., and Mukhopadhyay, S. N. (2012). Indole acetic acid and ACC deaminase-producing Rhizobium leguminosarum bv. trifolii SN10 promote rice growth, and in the process undergo colonization and chemotaxis. Biol. Fertil. Soils 48, 173–182. doi: 10.1007/s00374-011-0614-9
Bhattacharyya, D., Garladinne, M., and Lee, Y. H. (2015). Volatile indole produced by rhizobacterium Proteus vulgaris JBLS202 stimulates growth of Arabidopsis thaliana through auxin, cytokinin, and brassinosteroid pathways. J. Plant Growth Regul. 34, 158–168. doi: 10.1007/s00344-014-9453-x
Chandra, P., Wunnava, A., Verma, P., Chandra, A., and Sharma, R. K. (2021). Strategies to mitigate the adverse effect of drought stress on crop plants-influences of soil bacteria: a review. Pedosphere 31, 496–509. doi: 10.1016/s1002-0160(20)60092-3
Chandran, M., Manisha, A., and Subashini, A. (2014). Production of phosphate biofertilizer using lignocellulosic waste as carrier material. Asian J. Chem. 26, 2065–2067. doi: 10.14233/ajchem.2014.15787
Chen, F., Ye, J., Chio, C., Liu, W., Shi, J., and Qin, W. (2020). A simplified quick microbial genomic DNA extraction via freeze-thawing cycles. Mol. Biol. Rep. 47, 703–709. doi: 10.1007/s11033-019-05176-w
Choudhury, A. R., Choi, J., Walitang, D. I., Trivedi, P., Lee, Y., and Sa, T. (2021). ACC deaminase and indole acetic acid producing endophytic bacterial co-inoculation improves physiological traits of red pepper (Capsicum annum L.) under salt stress. J. Plant Physiol. 267:153544. doi: 10.1016/j.jplph.2021.153544
El-Gebali, S., Mistry, J., Bateman, A., Eddy, S. R., Luciani, A., Potter, S. C., et al. (2019). The Pfam protein families database in 2019. Nucleic Acids Res. 47, D427–D432. doi: 10.1093/nar/gky995
Etesami, H., Emami, S., and Alikhani, H. A. (2017). Potassium solubilizing bacteria (KSB): mechanisms, promotion of plant growth, and future prospects - a review. J. Soil Sci. Plant Nutr. 17, 897–911. doi: 10.4067/s0718-95162017000400005
Farag, M. A., Ryu, C., Sumner, L. W., and Pare, P. W. (2006). GC-MS SPME profiling of rhizobacterial volatiles reveals prospective inducers of growth promotion and induced systemic resistance in plants. Phytochemistry 67, 2262–2268. doi: 10.1016/j.phytochem.2006.07.021
Fincheira, P., and Quiroz, A. (2018). Microbial volatiles as plant growth inducers. Microbiol. Res. 208, 63–75. doi: 10.1016/j.micres.2018.01.002
Goswami, M., and Deka, S. (2020). Plant growth-promoting rhizobacteria-alleviators of abiotic stresses in soil: a review. Pedosphere 30, 40–61. doi: 10.1016/s1002-0160(19)60839-8
Guo, Z., Du, N., Li, Y., Zheng, S., Shen, S., and Piao, F. (2020). Gamma-aminobutyric acid enhances tolerance to iron deficiency by stimulating auxin signaling in cucumber (Cucumis sativus L.). Ecotoxicol. Environ. Saf. 192:110285. doi: 10.1016/j.ecoenv.2020.110285
Ha-Tran, D. M., Nguyen, T. T. M., Hung, S., Huang, E., and Huang, C. (2021). Roles of plant growth-promoting rhizobacteria (PGPR) in stimulating salinity stress defense in plants: a review. Int. J. Mol. Sci. 22:3154. doi: 10.3390/ijms22063154
Kang, S. M., Asaf, S., Khan, A. L., Khan, A. L., Mun, B. G., Khan, M. A., et al. (2020). Complete genome sequence of Pseudomonas psychrotolerans CS51, a plant growth-promoting bacterium, under heavy metal stress conditions. Microorganisms 8:382. doi: 10.3390/microorganisms8030382
Kong, W. L., Li, P. S., Wu, X. Q., Wu, T. Y., and Sun, X. R. (2020a). Forest tree associated bacterial diffusible and volatile organic compounds against various phytopathogenic fungi. Microorganisms 8:590. doi: 10.3390/microorganisms8040590
Kong, W. L., Wu, X. Q., and Zhao, Y. J. (2020b). Effects of Rahnella aquatilis JZ-GX1 on treat chlorosis induced by iron deficiency in Cinnamomum camphora. J. Plant Growth Regul. 39, 877–887. doi: 10.1007/s00344-019-10029-8
Kong, W. L., Wang, Y. H., and Wu, X. Q. (2021). Enhanced iron uptake in plants by volatile emissions of Rahnella aquatilis JZ-GX1. Front. Plant Sci. 12:704000. doi: 10.3389/fpls.2021.704000
Kour, D., Rana, K. L., Kaur, T., Yadav, N., Yadav, A. N., Kumar, M., et al. (2021). Biodiversity, current developments and potential biotechnological applications of phosphorus-solubilizing and -mobilizing microbes: a review. Pedosphere 31, 43–75. doi: 10.1016/s1002-0160(20)60057-1
Kusale, S. P., Attar, Y. C., Sayyed, R. Z., Malek, R. A., Ilyas, N., Suriani, N. L., et al. (2021). Production of plant beneficial and antioxidants metabolites by Klebsiella variicola under salinity stress. Molecules 26:1894. doi: 10.3390/molecules26071894
Li, F., Shi, T., Tang, X., Tang, M., Gong, J., and Yi, Y. (2020). Bacillus amyloliquefaciens PDR1 from root of karst adaptive plant enhances Arabidopsis thaliana resistance to alkaline stress through modulation of plasma membrane H+-ATPase activity. Plant Physiol. Biochem. 155, 472–482. doi: 10.1016/j.plaphy.2020.08.019
Li, G., Liang, Z., Li, Y., Liao, Y., and Liu, Y. (2020b). Exogenous spermidine regulates starch synthesis and the antioxidant system to promote wheat grain filling under drought stress. Acta Physiol. Plant. 42, 1723–1734.
Li, G., Wu, X., Ye, J., Hou, L., Zhou, A., and Zhao, L. (2013). Isolation and identification of phytate-degrading rhizobacteria with activity of improving growth of poplar and Masson pine. World J. Microbiol. Biotechnol. 29, 2181–2193. doi: 10.1007/s11274-013-1384-3
Li, G. E., and Wu, X. Q. (2014). Study on enzymology characteristics of phytase secreted by efficient phytate-degrading rhizobacteria Rahnella aquatilis JZ-GX1 (in Chinese). J. Cent. S. Univ. For. Technol. 34, 90–93.
Li, G. X., Wu, X. Q., Ye, J. R., and Yang, H. (2018). Characteristics of organic acid secretion associated with the interaction between Burkholderia multivorans WS-FJ9 and poplar root system. Biomed Res. Int. 2018:9619724. doi: 10.1155/2018/9619724
Li, P. S., Kong, W. L., Wu, X. Q., and Zhang, Y. (2021). Volatile organic compounds of the plant growth-promoting rhizobacteria JZ-GX1 enhanced the tolerance of Robinia pseudoacacia to salt stress. Front. Plant Sci. 12:753332. doi: 10.3389/fpls.2021.753332
Liu, A., Zhang, P., Bai, B., Bai, F., Jin, T., and Ren, J. (2020a). Volatile organic compounds of endophytic Burkholderia pyrrocinia strain JK-SH007 promote disease resistance in poplar. Plant Dis. 104, 1610–1620. doi: 10.1094/PDIS-11-19-2366-RE
Liu, S., Tian, Y., Jia, M., Lu, X., Yue, L., Zhao, X., et al. (2020b). Induction of salt tolerance in Arabidopsis thaliana by volatiles from Bacillus amyloliquefaciens FZB42 via the jasmonic acid signaling pathway. Front. Microbiol. 11:562934. doi: 10.3389/fmicb.2020.562934
Liu, Y., Wang, Y., Kong, W., Liu, W., Xie, X., and Wu, X. (2020c). Identification, cloning and expression patterns of the genes related to phosphate solubilization in Burkholderia multivorans WS-FJ9 under different soluble phosphate levels. AMB Express 10:108. doi: 10.1186/s13568-020-01032-4
Liu, W., Chen, F., Wang, C., Fu, H., Fang, X., Ye, J., et al. (2019). Indole-3-acetic acid in Burkholderia pyrrocinia JK-SH007: enzymatic identification of the indole-3-acetamide synthesis pathway. Front. Microbiol. 10:2559. doi: 10.3389/fmicb.2019.02559
Liu, X., Li, X., Li, Y., Li, R., and Xie, Z. (2017). Plant growth promotion properties of bacterial strains isolated from the rhizosphere of the Jerusalem artichoke (Helianthus tuberosus L.) adapted to saline-alkaline soils and their effect on wheat growth. Can. J. Microbiol. 63, 228–237. doi: 10.1139/cjm-2016-0511
Liu, Y., Chen, L., Zhang, N., Li, Z., Zhang, G., Xu, Y., et al. (2016). Plant-microbe communication enhances auxin biosynthesis by a root-associated bacterium, Bacillus amyloliquefaciens SQR9. Mol. Plant Microbe Interact. 29, 324–330. doi: 10.1094/MPMI-10-15-0239-R
Luziatelli, F., Ficca, A. G., Cardarelli, M., Melini, F., Cavalieri, A., and Ruzzi, M. (2020). Genome sequencing of Pantoea agglomerans C1 provides insights into molecular and genetic mechanisms of plant growth-promotion and tolerance to heavy metals. Microorganisms 8:153. doi: 10.3390/microorganisms8020153
Mariotti, L., Scartazza, A., Curadi, M., Picciarelli, P., and Toffanin, A. (2021). Azospirillum baldaniorum Sp245 induces physiological responses to alleviate the adverse effects of drought stress in purple basil. Plants 10:1141. doi: 10.3390/plants10061141
Meldau, D. G., Long, H. H., and Baldwin, I. T. (2012). A native plant growth promoting bacterium, Bacillus sp. B55, rescues growth performance of an ethylene-insensitive plant genotype in nature. Front. Plant Sci. 3:112. doi: 10.3389/fpls.2012.00112
Mondal, S., Pramanik, K., Ghosh, S. K., Pal, P., Mondal, T., Soren, T., et al. (2021). Unraveling the role of plant growth-promoting rhizobacteria in the alleviation of arsenic phytotoxicity: a review. Microbiol. Res. 250:126809. doi: 10.1016/j.micres.2021.126809
Nascimento, F. X., Hernandez, A. G., Glick, B. R., and Rossi, M. J. (2020). Plant growth-promoting activities and genomic analysis of the stress-resistant Bacillus megaterium STB1, a bacterium of agricultural and biotechnological interest. Biotechnol. Rep. 25:e406. doi: 10.1016/j.btre.2019.e00406
Olenska, E., Malek, W., Wojcik, I., Swiecicka, I., Thijs, S., and Vangronsveld, J. (2020). Beneficial features of plant growth-promoting rhizobacteria for improving plant growth and health in challenging conditions: a methodical review. Sci. Total Environ. 743:140682–140682. doi: 10.1016/j.scitotenv.2020.140682
Peng, J., Wu, D., Liang, Y., and Guo, Y. B. (2019). Disruption of acdS gene reduces plant growth promotion activity and maize saline stress resistance by Rahnella aquatilis HX2. J. Basic Microbiol. 59, 402–411. doi: 10.1002/jobm.201800510
Penrose, D. M., and Glick, B. R. (2003). Methods for isolating and characterizing ACC deaminase-containing PGPR. Physiol. Plant. 118, 10–15. doi: 10.1034/j.1399-3054.2003.00086.x
Perez-Flores, P., Valencia-Cantero, E., Altamirano-Hernandez, J., Pelagio-Flores, R., Lopez-Bucio, J., Garcia-Juarez, P., et al. (2017). Bacillus methylotrophicus M4-96 isolated from maize (Zea mays) rhizoplane increases growth and auxin content in Arabidopsis thaliana via emission of volatiles. Protoplasma 254, 2201–2213. doi: 10.1007/s00709-017-1109-9
Pretty, J., Benton, T. G., Bharucha, Z. P., Dicks, L. C., Flora, C. B., Godfray, H. C. J., et al. (2018). Global assessment of agricultural system redesign for sustainable intensification. Nat. Sustain. 1, 441–446. doi: 10.1038/s41893-018-0114-0
Qian, R., Ma, X., Zhang, X., Hu, Q., Liu, H., and Zheng, J. (2021). Effect of exogenous spermidine on osmotic adjustment, antioxidant enzymes activity, and gene expression of Gladiolus gandavensis seedlings under salt stress. J. Plant Growth Regul. 40, 1353–1367. doi: 10.1007/s00344-020-10198-x
Rath, M., Mitchell, T. R., and Gold, S. E. (2018). Volatiles produced by Bacillus mojavensis RRC101 act as plant growth modulators and are strongly culture-dependent. Microbiol. Res. 208, 76–84. doi: 10.1016/j.micres.2017.12.014
Rosier, A., Medeiros, F. H. V., and Bais, H. P. (2018). Defining plant growth promoting rhizobacteria molecular and biochemical networks in beneficial plant-microbe interactions. Plant Soil 428, 35–55. doi: 10.1007/s11104-018-3679-5
Ruzzi, M., and Aroca, R. (2015). Plant growth-promoting rhizobacteria act as biostimulants in horticulture. Sci. Hortic. 196, 124–134. doi: 10.1016/j.scienta.2015.08.042
Ryu, C. M., Farag, M. A., Hu, C. H., Reddy, M. S., Wei, H. X., Pare, P. W., et al. (2003). Bacterial volatiles promote growth in Arabidopsis. Proc. Natl. Acad. Sci. U.S.A. 100, 4927–4932. doi: 10.1073/pnas.0730845100
Schwyn, B., and Neilands, J. B. (1987). Universal chemical assay for the detection and determination of siderophores. Anal. Biochem. 160, 47–56. doi: 10.1016/0003-2697(87)90612-9
Shen, L., Wu, X. Q., Zeng, Q. W., and Liu, H. (2016). Regulation of soluble phosphate on the ability of phytate mineralization and beta-propeller phytase gene expression of Pseudomonas fluorescens JZ-DZ1, a phytate-mineralizing rhizobacterium. Curr. Microbiol. 73, 915–923. doi: 10.1007/s00284-016-1139-0
Sun, X. W., Ma, W., Xu, Y., Jing, X. M., and Ni, H. (2020). Complete genome sequence of Rahnella aquatilis MEM40, a plant growth-promoting rhizobacterium isolated from rice rhizosphere soil, with antagonism against Magnaporthe oryzae and Fusarium graminearum. Microbiol. Resour. Announc. 9:e00651-20. doi: 10.1128/MRA.00651-20
Tahir, H. A. S., Gu, Q., Wu, H., Raza, W., Hanif, A., Wu, L., et al. (2017). Plant growth promotion by volatile organic compounds produced by Bacillus subtilis SYST2. Front. Microbiol. 8:171. doi: 10.3389/fmicb.2017.00171
Tatusov, R. L., Fedorova, N. D., Jackson, J. D., Jacobs, A. R., Kiryutin, B., Koonin, E. V., et al. (2003). The COG database: an updated version includes eukaryotes. BMC Bioinformatics 4:41. doi: 10.1186/1471-2105-4-41
Teshome, D. T., Zharare, G. E., and Naidoo, S. (2020). The threat of the combined effect of biotic and abiotic stress factors in forestry under a changing climate. Front. Plant Sci. 11:601009. doi: 10.3389/fpls.2020.601009
Wang, Y., Hou, L., Wu, X., Zhu, M., Dai, Y., and Zhao, Y. (2021). Mycorrhiza helper bacterium Bacillus pumilus HR10 the improves growth and nutritional status of Pinus thunbergii by promoting mycorrhizal proliferation. Tree Physiol. tab139. doi: 10.1093/treephys/tpab139
Yasmin, H., Bano, A., Wilson, N. L., Nosheen, A., Naz, R., Hassan, M. N., et al. (2021). Drought-tolerant Pseudomonas sp. showed differential expression of stress-responsive genes and induced drought tolerance in Arabidopsis thaliana. Physiol. Plant. 174:e13497. doi: 10.1111/ppl.13497
Yousef, M., Heshmat, R., Valiollah, B., and Sandra, D. (2019). Brenneria spp. and Rahnella victoriana associated with acute oak decline symptoms on oak and hornbeam in Iran. For. Pathol. 49:e12535. doi: 10.1111/efp.12535
Yuan, L., Li, L., Zheng, F., Shi, Y., Xie, X., Chai, A., et al. (2020). The complete genome sequence of Rahnella aquatilis ZF7 reveals potential beneficial properties and stress tolerance capabilities. Arch. Microbiol. 202, 483–499. doi: 10.1007/s00203-019-01758-1
Zeng, Q., Wu, X., Wang, J., and Ding, X. (2017). Phosphate solubilization and gene expression of phosphate-solubilizing bacterium Burkholderia multivorans WS-FJ9 under different levels of soluble phosphate. J. Microbiol. Biotechnol. 27, 844–855. doi: 10.4014/jmb.1611.11057
Zhang, L., Fan, J. Q., Feng, G., and Declerck, S. (2019a). The arbuscular mycorrhizal fungus Rhizophagus irregularis MUCL 43194 induces the gene expression of citrate synthase in the tricarboxylic acid cycle of the phosphate-solubilizing bacterium Rahnella aquatilis HX2. Mycorrhiza 29, 69–75.
Zhang, P., Jin, T., Sahu, S. K., Xu, J., Shi, Q., Liu, H., et al. (2019b). The distribution of tryptophan-dependent indole-3-acetic acid synthesis pathways in bacteria unraveled by large-scale genomic analysis. Molecules 24:1411. doi: 10.3390/molecules24071411
Zhang, Y., Li, T., Liu, Y., Li, X., Zhang, C., Feng, Z., et al. (2019c). Volatile organic compounds produced by Pseudomonas chlororaphis subsp. aureofaciens SPS-41 as biological fumigants to control Ceratocystis fimbriata in postharvest sweet potatoes. J. Agric. Food Chem. 67, 3702–3710. doi: 10.1021/acs.jafc.9b00289
Zhang, Y., Li, T., Xu, M., Guo, J., Zhang, C., Feng, Z., et al. (2021). Antifungal effect of volatile organic compounds produced by Pseudomonas chlororaphis subsp. aureofaciens SPS-41 on oxidative stress and mitochondrial dysfunction of Ceratocystis fimbriata. Pestic. Biochem. Physiol. 173:104777. doi: 10.1016/j.pestbp.2021.104777
Zhou, C., Liu, Z., Zhu, L., Ma, Z., Wang, J., and Zhu, J. (2016a). Exogenous melatonin improves plant iron deficiency tolerance via increased accumulation of polyamine-mediated nitric oxide. Int. J. Mol. Sci. 17:1777. doi: 10.3390/ijms17111777
Zhou, C., Ma, Z., Zhu, L., Xiao, X., Xie, Y., Zhu, J., et al. (2016b). Rhizobacterial strain Bacillus megaterium BOFC15 induces cellular polyamine changes that improve plant growth and drought resistance. Int. J. Mol. Sci. 17:976. doi: 10.3390/ijms17060976
Zhou, C., Zhu, L., Guo, J., Xiao, X., Ma, Z., and Wang, J. (2019). Bacillus subtilis STU6 ameliorates iron deficiency in tomato by enhancement of polyamine-mediated iron remobilization. J. Agric. Food Chem. 67, 320–330. doi: 10.1021/acs.jafc.8b05851
Keywords: complete genome sequence, Rahnella victoriana, biofertilizer, volatile organic compounds, natural field environment
Citation: Kong W-L, Wang W-Y, Zuo S-H and Wu X-Q (2022) Genome Sequencing of Rahnella victoriana JZ-GX1 Provides New Insights Into Molecular and Genetic Mechanisms of Plant Growth Promotion. Front. Microbiol. 13:828990. doi: 10.3389/fmicb.2022.828990
Received: 04 December 2021; Accepted: 07 March 2022;
Published: 30 March 2022.
Edited by:
Baolei Jia, Chung-Ang University, South KoreaReviewed by:
Vijay Kumar, Institute of Himalayan Bioresource Technology (CSIR), IndiaCopyright © 2022 Kong, Wang, Zuo and Wu. This is an open-access article distributed under the terms of the Creative Commons Attribution License (CC BY). The use, distribution or reproduction in other forums is permitted, provided the original author(s) and the copyright owner(s) are credited and that the original publication in this journal is cited, in accordance with accepted academic practice. No use, distribution or reproduction is permitted which does not comply with these terms.
*Correspondence: Xiao-Qin Wu, eHF3dUBuamZ1LmVkdS5jbg==
Disclaimer: All claims expressed in this article are solely those of the authors and do not necessarily represent those of their affiliated organizations, or those of the publisher, the editors and the reviewers. Any product that may be evaluated in this article or claim that may be made by its manufacturer is not guaranteed or endorsed by the publisher.
Research integrity at Frontiers
Learn more about the work of our research integrity team to safeguard the quality of each article we publish.