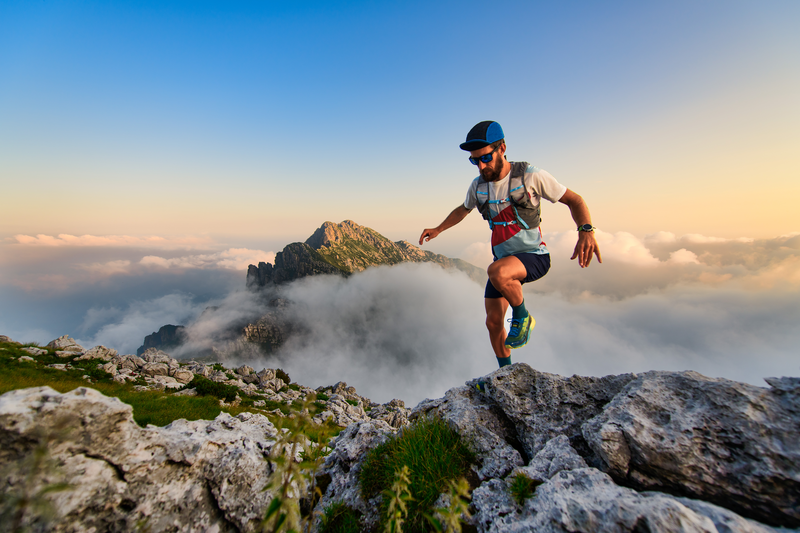
94% of researchers rate our articles as excellent or good
Learn more about the work of our research integrity team to safeguard the quality of each article we publish.
Find out more
ORIGINAL RESEARCH article
Front. Microbiol. , 10 February 2022
Sec. Antimicrobials, Resistance and Chemotherapy
Volume 13 - 2022 | https://doi.org/10.3389/fmicb.2022.826624
This article is part of the Research Topic Global Dissemination and Evolution of Epidemic Multidrug-Resistant Gram-Negative Bacterial Pathogens: Surveillance, Diagnosis and Treatment View all 11 articles
The colistin resistance gene mcr-1 is emerging as a global public health concern, altering the regulation of colistin usage globally since 2017, especially in China. However, few studies have revealed the impact of policy change on the epidemiology of mcr-positive Enterobacteriaceae (MCRPE) in patients. Here, we describe a molecular epidemiological study to investigate the MCRPE in patients in China from 2009–2019. During the surveillance period, 26,080 non-duplicated Enterobacteriaceae isolates were collected in Beijing. Colistin-resistant isolates were screened by enrichment culture supplemented with colistin, and the presence of the mcr gene was determined by PCR amplification. MCRPE isolates were then analyzed by susceptibility testing, genotyping, and risk factor analysis. Of the 26,080 isolates, mcr-1 was detected in 171 (1.1%) of 15,742 Escherichia coli isolates and 7 (0.1%) of 10,338 Klebsiella pneumoniae isolates. The prevalence of mcr-1-positive E. coli (MCRPEC) showed an increasing trend from 2009 to 2016, while a decreasing trend was observed since 2017. Multi-locus sequence typing analysis showed that MCRPEC isolates had extremely diverse genetic backgrounds, and most of these isolates were non-clonal. The prevalence of MCRPE in China remained at a low level, and even showed a declining trend over the last 3 years after the banning of colistin usage as feed additive in food animal in 2017. However, colistin permission in clinical therapy could still increase the risk of MCRPE transmission and intractable infections, active surveillance and monitoring strategies of MCRPE are recommended to prolong the clinical longevity of colistin.
Colistin is regarded as one of the last therapeutic options available to treat infections caused by carbapenem-resistant Enterobacteriaceae (CRE), which pose an increasing risk to public health. Until 2015, resistance to colistin was only associated with mutations and regular changes in chromosomal genes (Wang et al., 2018). Liu et al. (2016) described a plasmid-mediated colistin resistance gene, mcr-1, which encodes a phosphoethanolamine transferase enzyme (MCR-1) and results in the addition of phosphoethanolamine to lipid A in Enterobacteriaceae. The emergence of the mobile colistin resistance gene mcr-1 could result in bacterial isolates being resistant to all classes of antibiotics, which will compromise the available treatment options for severe infections (Zhong et al., 2018).
Enterobacteriaceae, especially Escherichia coli and Klebsiella pneumoniae, typically form part of the normal flora in healthy people, yet can induce various infections under certain conditions, such as respiratory, urinary, and bloodstream infections (Paterson, 2006; Seiffert et al., 2013). Although the use of colistin in humans has been very limited in the past, it has been implemented in veterinary medicine since the early 1980s, mainly for the prevention and treatment of Enterobacteriaceae infections (Kempf et al., 2016). In China, colistin sulfate premix has been widely used as animal feed additive since 2009, and the production has reached 30,000 tons in 2015 (Wang et al., 2020). Wang et al. (2017) speculated that the emergence of mcr-1 probably occurred first in animals, before extending to humans. Liu et al. (2016) identified the plasmid-borne colistin resistance gene, mcr-1, in Enterobacteriaceae from hospitalized humans, animals, and raw meat from China in 2015 (Wang et al., 2017). Subsequently, mcr-1-positive Enterobacteriaceae (MCRPE) have been found in inpatients, healthy humans, animals, raw meat, vegetables, and a number of environmental settings globally (Quesada et al., 2016). E. coli and K. pneumoniae are considered key reservoirs and disseminators of mcr-1. On April 30, 2017, the Chinese Ministry of Agriculture formally issued the banning of colistin as feed additives for food animals, aiming to reduce the wide spread of colistin resistance and protect the effectiveness of colistin in the clinical setting.
To date, MCRPE have been reported in numerous countries across Asia, Africa, Europe, North America, and South America (Quan et al., 2017; Saavedra et al., 2017; Wang et al., 2018; Clemente et al., 2019), indicating the rapid transfer of mcr-1 among Enterobacteriaceae (Carattoli, 2013). Colistin has been approved for human medicine by the China Food and Drug Administration since January 2017 (Shen Y. et al., 2018), there is an urgent need to assess the role of MCRPE in the treatment of infections. Herein, we investigated the prevalence, risk factors, and molecular epidemiology of MCRPE carriage among inpatients and outpatients in Beijing, the capital of China, from 2009 to 2019. The aim of our study was to better understand the clinical impact of policy change on the current epidemiological trends and characteristics of MCRPE colonization in patients over this time frame.
The aim of this epidemiological and clinical study was to investigate the prevalence of MCRPE in patients. We conducted a long-term molecular epidemiological surveillance in Beijing from 2009–2019. Beijing, the capital city of China, has a population of 21 million and widely accepts patients from throughout the country. MCRPE strains were isolated from different specimen types in patients, including blood, urine, sputum, fecal, and tissue. At least 1,000 samples were collected annually.
All samples were taken from non-duplicate patients and we screen MCRPE isolates from samples by culturing in enrichment media supplemented with 2 mg/L colistin. The isolates before 2017 were identified from the retrospective collection. Pure colonies of E. coli and K. pneumoniae were selected according to their morphology and color on an SS agar plate supplemented with 2 mg/L colistin. The surviving bacteria were identified by matrix-assisted laser desorption/ionization time of flight mass spectrometry (MALDI-TOF MS) (Bruker Daltonik GmbH, Bremen, Germany), and further confirmed by 16S rDNA sequence analysis. Ethics approval was granted by the Chinese PLA General Hospital. Individual consent forms obtained from all patients before sampling. All participants held the right to quit the study at any stage.
The presence of the colistin-resistance genes (mcr) was confirmed by Sanger sequencing (Seiffert et al., 2013). All mcr-positive strains were subjected to whole-genome sequencing (WGS). Genomic DNA extraction of isolates was performed using the Wizard Genomic DNA Purification Kit (Promega, Beijing, China) following a standard protocol, and then sequenced on the Illumina HiSeq 2500 platform (Annoroad Biotec Co.) with a 150-bp paired-end strategy. All procedures were carried out according to the manufacturer’s instructions. The sequencing data were analyzed using multiple programs. The draft assembly of the sequences was generated using SPAdes version 3.11.1. Colistin resistance gene mcr and multi-locus sequence typing (MLST) were confirmed using standalone BLAST analysis SRST2 (Shen Z. et al., 2018).
We calculated minimum inhibitory concentrations (MICs) for all MCRPE isolates against commonly used antibiotics via the broth microdilution method in accordance with the Clinical and Laboratory Standards Institute (CLSI) guidelines. Breakpoints for aminoglycosides (amikacin, gentamicin, and tobramycin), β-lactams (ampicillin and piperacillin), β-lactam/β-lactamase inhibitor combination (amoxicillin-clavulanate, ampicillin-sulbactam, and piperacillin-tazobactam), carbapenems (ertapenem, imipenem, and meropenem), cephems (cefazolin, cefepime, ceftazidime, cefotetan, and ceftriaxone), fluoroquinolones (ciprofloxacin and levofloxacin), trimethoprim-sulfamethoxazole, and nitrofurantoin were interpreted according to the annual CLSI-M100-S281 [Clinical and Laboratory Standards Institute (CLSI), 2018], while the results of antimicrobial susceptibility for colistin was interpreted in accordance with the European Committee on Antimicrobial Susceptibility Testing (EUCAST) criteria2 [European Committee on Antimicrobial Susceptibility Testing [ECAST], 2020]. The reference strains E. coli ATCC 25922 and K. pneumoniae ATCC 13883 (carbapenem-susceptible) were used as controls.
We collected clinical data from patients with or without mcr-positive isolates between 2009 and 2019. Multiple risk factors were assessed, including sex (male and female), age (<15, 15–24, 25–34, 35–44, 45–54, 55–64, and ≥ 65 years), living conditions (city or village), specimen types (blood, sputum, and urine), patient types (inpatient or outpatient), comorbidities (diabetes, hypertension, and malignant tumor), and other risk factors (operation history, mechanical ventilation, urinary catheter, drainage tube, venous catheterization, and antibiotic use in the past 3 months). Variants were collated into specifically designed databases using Microsoft Excel 2016 (Microsoft, Redmond, WA, United States). Univariate analysis was performed using the Statistical Package for the Social Sciences version 23.0 (SPSS, Chicago, IL, United States). A chi-square test was used to examine the difference in the resistance levels of the different groups, and variables with a p-value < 0.2 were considered statistically significant. Significant variables with P < 0.2 were taken into multivariable analysis. A multivariable logistic regression model using a backward stepwise process was adopted to estimate the odds ratios (ORs) and 95% confidence intervals (CIs) of the risk factors related to mcr-positive E. coli. Variables with a p-value < 0.05 were considered significant risk factors. Pooled prevalence was estimated using a metaphor package with 95% CIs to represent the resistance rates of bacteria from 2010 to 2019 (Zou et al., 2019).
For the prevalence study, a total of 26,080 non-duplicated isolates from inpatients and outpatients were collected in Beijing from 2009 to 2019, which included 15,742 E. coli and 10,338 K. pneumoniae isolates. We detected the mcr-1 in 171 (1.09, 95 CI, 0.93–1.26) E. coli (MCRPEC) and 7 (0.07, 95 CI, 0.03–0.14) K. pneumoniae (MCRPKP) isolates (Supplementary Table 1). The age, specimen type, and sex information about the MCRPEC cases and the study population are shown in Figures 1A–C, and the information of the 7 MCRPKP cases is shown in Supplementary Table 2. The majority of the patients were over 65 (41.4%), and most isolates were obtained from urine (71.1%). The distribution of age, sex, and specimen information was similar among the MCRPEC cases and total cases. During the study period, the overall percentage of mcr-1-positive isolates remained low, especially for K. pneumoniae, and we found diverse trends in the prevalence of MCRPEC (Figure 1A and Supplementary Table 1). The proportion of MCRPEC increased from 2009 (0.0%) to 2016 (1.8%) (p < 0.001), except in 2013 (0.3%), while a decreasing trend was observed since 2017 (1.6%) (Figure 1A).
Figure 1. Summary of information. (A) The age distribution of the total sample population and mcr-1-positive E. coli (MCRPEC). (B) The specimen type distribution of the total sample population and MCRPEC. (C) The sex distribution of the total sample population and MCRPEC. (D) The prevalence of mcr-1-positive Enterobacteriaceae (MCRPE) during 2009–2019. (E) The prevalence of main plasmids types in all MCRPEC during 2010–2019.
Overall, 150/171 (87.72%) MCRPEC and all 7 MCRPKP strains exhibited resistance to colistin (Supplementary Tables 3, 4). Antimicrobial susceptibility profiles of the 171 MCRPEC strains are shown in Supplementary Table 3. Among the 171 MCRPEC isolates, the MICs for colistin ranged from 0.25 to 16 μg/mL. Most MCRPEC remained susceptible to amikacin (85.4%), ertapenem (95.9%), imipenem (98.2%), piperacillin-tazobactam (91.0%), and cefotetan (96.2%). Among the 171 MCRPEC isolates, 3 (1.8%) were carbapenem-resistant, 132 (77.2%) were extended-spectrum β-lactamases (ESBL), and the proportion of ESBL strains increased from 50.0% in 2010 to 82.4% in 2019 (data not shown). We classified the MCRPEC isolates from 2010 to 2019 into inpatient and outpatient groups. The MIC50 values were similar between clinical E. coli isolates from inpatients (n = 127) and outpatients (n = 44), except for gentamicin and amikacin, which were both more than 16-fold higher in the inpatient group (Supplementary Table 5).
We collected clinical data from 171 mcr-1-positive clinical E. coli isolates and randomly selected 734 mcr-1-negative clinical E. coli isolates (62 isolates were excluded because of incomplete data, leaving 672) from 15,571 mcr-1-negative E. coli infection cases. Multiple variables were assessed to determine the risk factors associated with MCRPEC by OR analysis (Tables 1, 2). Age and living conditions were not associated with MRCPEC infection. We determined that E. coli isolated from male were more likely to be mcr-1 positive compared to those from outpatients (OR = 1.6, p < 0.02). Specimen types of E. coli were significantly associated with mcr-1 positivity; E. coli isolates from bile and drainage fluid samples had a higher proportion of MCRPEC than those from other samples. Furthermore, MCRPEC was far more prevalent among E. coli isolated from patients with malignant tumors (R = 2.3, p < 0.001).
Table 2. Multivariable logistic regression analysis of factors associated with mcr-1-positive E. coli.
In addition, we analyzed the outcome of patients in 28 days with MRCPEC or mcr-1-negative E. coli (Table 3). Significant differences were observed between the two groups; in particular, patients with MRCPEC infections were more likely to result in treatment failure.
We further evaluated the molecular characteristics of MRCPEC strains as minimum spanning trees, phylogenetic tree and heatmaps (Figures 2, 3 and Supplementary Figures 1, 2). There were 66 distinct sequence types (STs) in the 171 isolates, suggesting extreme divergence of MRCPEC strains. Molecular epidemiological analysis revealed that none of these STs were predominant, but ST410 (n = 10), ST648 (n = 9), ST156 (n = 7), and ST224 (n = 7) were more prevalent than the other STs. These four STs have been recognized as common clades of E. coli carrying mcr-1 in a previous study (Wang et al., 2017). Our results suggest that horizontal dissemination of mcr-1 in E. coli was discovered between 2014 and 2019. In addition, an ST617 E. coli isolate in 2014 and an ST3224 E. coli isolate in 2015 carried both mcr-1 and the carbapenem resistance gene blaNDM–1.
Figure 2. Genetic analysis of mcr-1- carrying plasmids. Different arrows represent particular genes having a name written underneath of each arrow. The arrows without names represent hypothetical proteins.
Figure 3. Distribution of Inc type, ARGs, heavy metal genes, and VAGs among MCRPEC isolates. The color of each box represents the percentage of the corresponding item among sequenced isolates in the corresponding year. ARGs, antibiotic resistance genes; HMGs, heavy metal genes; VAGs, virulence-associated genes.
In order to investigate the correlation between human MCRPEC and animal MCRPEC, the whole genomes of 150 animal MCRPEC, 122 animal mcr-1 negative E. coli (MCRNEC), and 137 human MCRNEC were downloaded from the published data in the National Center for Biotechnology Information (NCBI) database. All the MCRPEC and MCRNEC isolates were allocated to 142 STs, and the diversity of clinical origin was greater than isolates of animal origin (Supplementary Figure 1). Of these mcr-1-positive clades, most sequence-types were common to both animal and human origin, such as ST156, ST101, ST354, and ST48 (Figure 4 and Supplementary Figure 1). Interestingly, several ST branches were discovery only in human carriage, such as ST131 and ST95 (Supplementary Figure 1).
Figure 4. Minimum spanning tree of mcr-1-positive E. coli by MLST type and gene allele from inpatients and outpatients. Each node in the tree represents an ST, and the size of a node is proportional to the number of isolates it represents. The length of the branch is equal to the number of different alleles (calculated using seven MLST genes) between two linked nodes.
A total of 139 mcr-1 positive plasmids were identified and assigned to known Inc types, among which the most prevalence was IncI2 (n = 111, 64.9%), followed by IncX4 (n = 15, 8.8%), IncHI2 (n = 9, 5.3%), and Incp0111 (n = 4, 2.3%). IncI2 remains the dominant Inc type in MCRPEC prevalence during 2010 to 2019, while a decreasing trend was observed in the proportion of IncI2 plasmids from 2017 to 2019 (Figure 1E). The genetic environment of mcr-1 depends on its backbone structure plasmids, which vary greatly between different Inc types (Figure 2). The genetic context of mcr-1 within each plasmid type (IncI2, IncX4, IncHI2, and Incp0111) was similar to the four reported mcr-1-carrying plasmid (pHNSHP45, KX254343, MF774186, and MF455226, respectively) from four pig-derived E. coli isolates (Supplementary Figures 3–6). Notably, the ancestral mobile element, ISApl1, which previously thought to be responsible for mcr-1 transmission, was only detected in 42 (24.6%) isolates.
Antibiotic resistance genes (ARGs), heavy metal genes, and virulence-associated genes (VAGs) in the 171 MCRPEC isolates were determined by WGS. The percentages of ARGs, heavy metal genes, and VAGs for each year are shown in Figure 3. The mcr-1 gene co-existed with strA/B (aminoglycoside resistance), ampC1 (β-lactam ARG), blaCTX–M–1 and blaTEM–1D (ESBL-encoding genes), floR (florfenicol resistance), and tetA (tetracycline resistance gene) (Figure 3). espL/R/X (type III secretion system), csgB (which encodes the curli nucleator protein of E. coli), ompA (outer membrane protein A), fdec (intimin-like protein), and fimH (type I fimbriae) were more strongly associated with mcr-1 carriage than other VAGs (Figure 3). Furthermore, WGS showed that mcr-1 was mostly located on 3 Inc (incompatible) type plasmids, IncX4-type (n = 21, 12.3%), IncI2-type (n = 115, 67.3%), and IncHI2-type (n = 28, 16.4%), which is similar to a previous report (Jiang et al., 2020).
In 2009–2016, we noted a significant increase in the prevalence of MCRPEC from 2009 to 2016 (0.0% ∼ 1.8%), except in 2013, while a decreasing trend was noted after 2016 (Figure 1D). Notably, the Ministry of Agriculture of China (Article number 2428) withdrew colistin as a feed additive and growth promoter in November 2016, and this was officially enforced in April 2017 (Walsh and Wu, 2016). Colistin produced before Apr, 2017 was still allowed to use according to the data from Ministry of Agricultural. The overall production of colistin sulfate reached over 50,000 tons in 2015–2016, we believed the rising of colistin resistance may be later comparing with the sales and usage of colistin, and several studies also indicated colistin resistance reached peak around later 2016-mid 2017 (Shen et al., 2016; Wang et al., 2020). In Tu et al.’s study, the prevalence of mcr-1 (5.6%) was significantly lower than before the ban (86.4%, p < 0.01) in a large scale swine farm (Tu et al., 2021). According to previous study, the banning had a significant effect on reducing colistin resistance in both animal and humans by comparing 2016–2017 and 2018–2019 (Shen et al., 2020; Wang et al., 2020). Furthermore, the human carriage of MCRPEC also decreased from 14.3% in 2016 to 6.3% in 2019 (p < 0.0001) in hospital across 24 provincial capital cities and municipalities in China (Wang et al., 2020). In a prevalence dynamics analysis of human mcr-1 colonization from April 2011 to December 2019, a dramatic decline in human mcr-1 colonization prevalence was observed, consisting with the complete ban of colistin in animal feed (Shen et al., 2021). Above all, we suggest that the withdrawal of colistin as an animal growth promoter in China had a positive impact on MCRPEC infections in both animals and humans. However, we believed the overall colistin resistance will remain declining as long as the banning in effective. It might take a few extra years for the resistance reduced to the stage before colistin usage. In this study, the prevalence of mcr-1 decreased from 32 (1.8%) of 1,771 in 2016, to 24 (1.6%) of 1,731 (p = 0.3) in 2018 and 17 (1.0%) of 1,712 (p < 0.05) in 2019. However, the extended longitude study was necessary to further evaluate the effective of banning.
In the analysis of different factors associated with MCRPEC carriage, we observed that E. coli from inpatients were more likely to carry the colistin resistance gene mcr-1 (OR = 1.5, p < 0.05), suggesting that hospitalization may be a risk factor for colonization of mcr-1. Among the different specimen types, we observed that E. coli isolates from bile and drainage fluid were much more likely to be mcr-1 positive than from urine and blood. In addition, we observed a significant association between MCRPEC infection and comorbidities, such as diabetes, malignant tumors, and operation history (p < 0.05). Unsurprisingly, antibiotic use in the past 3 months was strongly associated with high MCRPEC occurrence.
Our MLST analysis showed that the 171 MRCPEC isolates had extremely diverse genetic backgrounds, and many were non-clonal. In this study, ST410 (n = 9) was the most prevalent sequence type among inpatients, while ST648 (n = 4) and ST117 (n = 4) were more prevalent than other types among outpatients. E. coli ST410 has been reported worldwide as an extraintestinal pathogen associated with multidrug resistance and is capable of patient-to-patient transmission, causing hospital outbreaks (Roer et al., 2018). E. coli ST648 is a predominant multidrug-resistant clone observed worldwide and is frequently associated with various β-lactamases, including ESBLs, NDM, and KPC (Mushtaq et al., 2011; Kim et al., 2012). E. coli ST117 is a highly virulent pathogenic lineage associated with extraintestinal infections in humans and poultry (Cummins et al., 2019). These findings indicate that mcr-1 has wide host adaptability in E. coli and different virulence potentials. Of all mcr-1-positive isolates, most sequence-types were common in both human and animal origin, and nucleotide sequences of four main plasmid Inc-types (IncI2, IncX4, IncHI2, and Incp0111) were similar to reported plasmids from animal origins in China, which reminds us the speculation that mcr-1 probably occurred first in animals and extended to humans (Wang et al., 2017).
The plasmid-mediated gene mcr-1 has disseminated globally and could pose severe threats to human health when transferred into CRE and extended-spectrum beta-lactamase Enterobacteriaceae (ESBL-E), leaving fewer treatment options for infections caused by multi-drug resistant strains. Among 178 mcr-1 positive Enterobacteriaceae, 161 (90.5%) isolates harbored ESBL-encoding genes (such as blaCTX–M and blaTEM) and 35 (19.7%) isolates were carbapenemases (such as NDM-1, KPC-2, and OXA-48) producer. To date, a few reports showed that 0.1–4.6% CRE strains were mcr-1 positive in clinical settings (Huang et al., 2018; Chen et al., 2019; Xiaomin et al., 2020), and one study showed that 2.40% ESBL-E strains also harbored mcr-1 (Jørgensen et al., 2017). The coexistence of transferable colistin and carbapenem resistance has become an alarming concern, calling for global monitoring and surveillance. Although mobile colistin resistance poses great difficulties in the treatment of severe infectious diseases, in this study, most mcr-1 positive isolates remained susceptible to many other antibiotics, such as carbapenems, piperacillin-tazobactam, amikacin, and cefotetan. Therefore, antimicrobials should be used in combination to defend against colistin-resistant bacteria in clinical therapy.
Our study has several limitations. One limitation was that the colistin resistance gene mcr-1 was screened in Enterobacteriaceae isolates collected retrospectively. It is possible to lose mcr-1 carrying plasmids during preservation, which may result in an underestimation of the prevalence of mcr-1. Another limitation is the partial collection of patient information. Since the colossal groups surveyed in this study, we only included 672 (4.3%) of 15,571 mcr-1 negative E. coli isolates for multivariable analysis. Even though, our data were large-scale and combined across different periods each year. For further studies, analysis of more isolates should be included to reduce bias in identifying the risk factors related to mcr-1 in patients.
In conclusion, this survey revealed a decreasing prevalence from 2016 to 2019, which is opposite to the increasing trend observed between 2009 and 2016. MCPREC isolates possessed extremely diverse genetic backgrounds in patients in the clinical setting from Beijing, China, and our findings provide evidence of the transmission of mcr-1 among patients. Moreover, the coexistence of transferable colistin resistance and carbapenem resistance poses a great threat, and effective monitoring and surveillance are necessary to control and prevent the dissemination of MCRPE.
Whole-genome sequencing data that support the findings of this study have been deposited in the NCBI database under BioProject accession number PRJNA78361.
QZ contributed significantly to perform the experiments. YL contributed significantly to perform the data analyses and wrote the manuscript. YT and YS helped to perform the experiments and manuscript preparation. SW and YZ contributed significantly to the conception of the study. All authors contributed to the article and approved the submitted version.
This work was supported by National Key Research and Development Program is supported by Ministry of Science and Technology of the People’s Republic of China (2017YFC1601502).
The authors declare that the research was conducted in the absence of any commercial or financial relationships that could be construed as a potential conflict of interest.
All claims expressed in this article are solely those of the authors and do not necessarily represent those of their affiliated organizations, or those of the publisher, the editors and the reviewers. Any product that may be evaluated in this article, or claim that may be made by its manufacturer, is not guaranteed or endorsed by the publisher.
The Supplementary Material for this article can be found online at: https://www.frontiersin.org/articles/10.3389/fmicb.2022.826624/full#supplementary-material
Carattoli, A. (2013). Plasmids and the spread of resistance. Int. J. Med. Microbiol. 303, 298–304. doi: 10.1016/j.ijmm.2013.02.001
Chen, C. W., Tang, H. J., Chen, C. C., Lu, Y. C., Chen, H. J., Su, B. A., et al. (2019). The microbiological characteristics of carbapenem-resistant Enterobacteriaceae carrying the mcr-1 gene. J. Clin. Med. 8:261. doi: 10.3390/jcm8020261
Clemente, L., Manageiro, V., Correia, I., Amaro, A., Albuquerque, T., Themudo, P., et al. (2019). Revealing mcr-1-positive ESBL-producing Escherichia coli strains among Enterobacteriaceae from food-producing animals (bovine, swine and poultry) and meat (bovine and swine), Portugal, 2010–2015. Int. J. Food Microbiol. 296, 37–42. doi: 10.1016/j.ijfoodmicro.2019.02.006
Clinical and Laboratory Standards Institute [CLSI] (2018). Performance Standards for Antimicrobial Testing: twenty-eighth Informational Supplement M100-S28. Available online at: https://community.clsi.org/media/1930/m100ed28_sample.pdf. [Accessed January 2018].
Cummins, M. L., Chowdhury, P. R., Marenda, M. S., Browning, G. F., and Djordjevic, S. P. (2019). Salmonella genomic island 1B variant found in a sequence type 117 avian pathogenic Escherichia coli isolate. Msphere 4, e00169–19. doi: 10.1128/mSphere.00169-19
European Committee on Antimicrobial Susceptibility Testing [ECAST] (2020). Breakpoint Tables for Interpretation of MICs and Zone Diameters. Available online at: http://www.eucast.org. [Accessed 1 January 2020]
Huang, B., He, Y., Ma, X., Cai, R., Zeng, J., Lu, Y., et al. (2018). Promoter variation and gene expression of mcr-1-harboring plasmids in clinical isolates of Escherichia coli and Klebsiella pneumoniae from a Chinese hospital. Antimicrob. Agents Chemother. 62, e00018–e18. doi: 10.1128/AAC.00018-18
Jiang, Y., Zhang, Y., Lu, J., Wang, Q., Cui, Y., Wang, Y., et al. (2020). Clinical relevance and plasmid dynamics of mcr-1-positive Escherichia coli in China: a multicentre case-control and molecular epidemiological study. Lancet Microbe. 1, e24–e33. doi: 10.1016/S2666-5247(20)30001-X
Jørgensen, S. B., Søraas, A., Arnesen, L. S., Leegaard, T., Sundsfjord, A., and Jenum, P. A. (2017). First environmental sample containing plasmid-mediated colistin-resistant ESBL-producing Escherichia coli detected in Norway. APMIS 125, 822–825. doi: 10.1111/apm.12720
Kempf, I., Jouy, E., and Chauvin, C. (2016). Colistin use and colistin resistance in bacteria from animals. Int. J. Antimicrob. Agents 48, 598–606. doi: 10.1016/j.ijantimicag.2016.09.016
Kim, Y. A., Qureshi, Z. A., Adams-Haduch, J. M., Park, Y. S., Shutt, K. A., and Doi, Y. (2012). Features of Infections Due to Klebsiella pneumoniae Carbapenemase–Producing Escherichia coli: emergence of Sequence Type 131. Clin. Infect. Dis. 55, 224–231. doi: 10.1093/cid/cis387
Liu, Y. Y., Wang, Y., Walsh, T. R., Yi, L. X., Zhang, R., Spencer, J., et al. (2016). Emergence of plasmid-mediated colistin resistance mechanism MCR-1 in animals and human beings in China: a microbiological and molecular biological study. Lancet Infect. Dis. 16, 161–168. doi: 10.1016/S1473-3099(15)00424-7
Mushtaq, S., Irfan, S., Sarma, J., Doumith, M., Pike, R., Pitout, J., et al. (2011). Phylogenetic diversity of Escherichia coli strains producing NDM-type carbapenemases. J. Antimicrob. Chemother. 66, 2002–2005. doi: 10.1371/journal.pone.0147740
Paterson, D. L. (2006). Resistance in gram-negative bacteria: enterobacteriaceae. Am. J. Infect. Control. 34, S20–S28. doi: 10.1016/j.amjmed.2006.03.013
Quan, J., Li, X., Chen, Y., Jiang, Y., Zhou, Z., Zhang, H., et al. (2017). Prevalence of mcr-1 in Escherichia coli and Klebsiella pneumoniae recovered from bloodstream infections in China: a multicentre longitudinal study. Lancet Infect. Dis. 17, 400–410. doi: 10.1016/S1473-3099(16)30528-X
Quesada, A., Ugarte-Ruiz, M., Iglesias, M. R., Porrero, M. C., Martínez, R., Florez-Cuadrado, D., et al. (2016). Detection of plasmid mediated colistin resistance (MCR-1) in Escherichia coli and Salmonella enterica isolated from poultry and swine in Spain. Res. Vet. Sci. 105, 134–135. doi: 10.1016/j.rvsc.2016.02.003
Roer, L., Overballe-Petersen, S., Hansen, F., Schønning, K., Wang, M., Røder, B. L., et al. (2018). Escherichia coli sequence type 410 is causing new international high-risk clones. Msphere 3, e337–18. doi: 10.1128/mSphere.00337-18
Saavedra, S. Y., Diaz, L., Wiesner, M., Correa, A., Arévalo, S. A., Reyes, J., et al. (2017). Genomic and molecular characterization of clinical isolates of Enterobacteriaceae harboring mcr-1 in Colombia, 2002 to 2016. Antimicrob. Agents Chemother. 61, e841–e17. doi: 10.1128/AAC.00841-17
Seiffert, S. N., Hilty, M., Perreten, V., and Endimiani, A. (2013). Extended-spectrum cephalosporin-resistant Gram-negative organisms in livestock: an emerging problem for human health. Drug Resist. Updates. 16, 22–45. doi: 10.1016/j.drup.2012.12.001
Shen, C., Zhong, L., Yang, Y., Doi, Y., Paterson, D., Stoesser, N., et al. (2020). Dynamics of mcr-1 prevalence and mcr-1-positive Escherichia coli after the cessation of colistin use as a feed additive for animals in China: a prospective cross-sectional and whole genome sequencing-based molecular epidemiological study. Lancet Microbe. 1, e34–e43. doi: 10.1016/S2666-5247(20)30005-7
Shen, C., Zhong, L. L., Zhong, Z., Doi, Y., Shen, J., Wang, Y., et al. (2021). Prevalence of mcr-1 in Colonized Inpatients. China, 2011-2019. Emerg. Infect. Dis. 27, 2502–2504. doi: 10.3201/eid2709.203642
Shen, Y., Zhou, H., Xu, J., Wang, Y., Zhang, Q., Walsh, T. R., et al. (2018). Anthropogenic and environmental factors associated with high incidence of mcr-1 carriage in humans across China. Nat. Microbiol. 3, 1054–1062. doi: 10.1038/s41564-018-0205-8
Shen, Z., Hu, Y., Sun, Q., Hu, F., Zhou, H., Shu, L., et al. (2018). Emerging Carriage of NDM-5 and MCR-1 in Escherichia coli From Healthy People in Multiple Regions in China: a Cross Sectional Observational Study. EClin. Med. 6, 11–20. doi: 10.1016/j.eclinm.2018.11.003
Shen, Z., Wang, Y., Shen, Y., Shen, J., and Wu, C. (2016). Early emergence of mcr-1 in Escherichia coli from food-producing animals. Lancet Infect. Dis. 16:293. doi: 10.1016/S1473-3099(16)00061-X
Xiaomin, S., Yiming, L., Yang, Y., Shen, Z., Yongning, W., and Shaolin, W. (2020). Global impact of mcr-1-positive Enterobacteriaceae bacteria on “one health”. Crit. Rev. Microbiol. 46, 565–577. doi: 10.1080/1040841X.2020.1812510
Tu, Z., Gu, J., Zhang, H., Liu, J., Shui, J., and Zhang, A. (2021). Withdrawal of colistin reduces incidence of mcr-1-harboring IncX4-type plasmids but has limited effects on unrelated antibiotic resistance. Pathogens 10:1019. doi: 10.3390/pathogens10081019
Walsh, T. R., and Wu, Y. (2016). China bans colistin as a feed additive for animals. Lancet Infect. Dis. 16:1102. doi: 10.1016/S1473-3099(16)30329-2
Wang, R., Van, D. L., Shaw, L. P., Bradley, P., Wang, Q., Wang, X., et al. (2018). The global distribution and spread of the mobilized colistin resistance gene mcr-1. Nat. Commun. 9, 1–9. doi: 10.1038/s41467-018-03205-z
Wang, Y., Tian, G. B., Zhang, R., Shen, Y., Tyrrell, J. M., Huang, X., et al. (2017). Prevalence, risk factors, outcomes, and molecular epidemiology of mcr-1-positive Enterobacteriaceae in patients and healthy adults from China: an epidemiological and clinical study. Lancet Infect. Dis. 17, 390–399. doi: 10.1016/S1473-3099(16)30527-8
Wang, Y., Xu, C., Zhang, R., Chen, Y., Shen, Y., Hu, F., et al. (2020). Changes in colistin resistance and mcr-1 abundance in Escherichia coli of animal and human origins following the ban of colistin-positive additives in China: an epidemiological comparative study. Lancet Infect. Dis. 20, 1161–1171. doi: 10.1016/S1473-3099(20)30149-3
Zhong, L. L., Phan, H. T., Shen, C., Vihta, K. D., Sheppard, A. E., Huang, X., et al. (2018). High rates of human fecal carriage of mcr-1–positive multidrug-resistant Enterobacteriaceae emerge in China in association with successful plasmid families. Clin. Infect. Dis. 66, 676–685. doi: 10.1093/cid/cix885
Keywords: colistin, mcr-1, Enterobacteriaceae, longitude study, China
Citation: Zhao Q, Li Y, Tian Y, Shen Y, Wang S and Zhang Y (2022) Clinical Impact of Colistin Banning in Food Animal on mcr-1-Positive Enterobacteriaceae in Patients From Beijing, China, 2009–2019: A Long-Term Longitudinal Observational Study. Front. Microbiol. 13:826624. doi: 10.3389/fmicb.2022.826624
Received: 01 December 2021; Accepted: 07 January 2022;
Published: 10 February 2022.
Edited by:
Guo-bao Tian, Sun Yat-sen University, ChinaCopyright © 2022 Zhao, Li, Tian, Shen, Wang and Zhang. This is an open-access article distributed under the terms of the Creative Commons Attribution License (CC BY). The use, distribution or reproduction in other forums is permitted, provided the original author(s) and the copyright owner(s) are credited and that the original publication in this journal is cited, in accordance with accepted academic practice. No use, distribution or reproduction is permitted which does not comply with these terms.
*Correspondence: Ying Zhang, Y2hlcnJ5emp1QGFsaXl1bi5jb20=; Shaolin Wang, c2hhb2xpbndhbmdAY2F1LmVkdS5jbg==
†These authors have contributed equally to this work and share first authorship
Disclaimer: All claims expressed in this article are solely those of the authors and do not necessarily represent those of their affiliated organizations, or those of the publisher, the editors and the reviewers. Any product that may be evaluated in this article or claim that may be made by its manufacturer is not guaranteed or endorsed by the publisher.
Research integrity at Frontiers
Learn more about the work of our research integrity team to safeguard the quality of each article we publish.