- 1Department of Botany, University of Balochistan, Quetta, Pakistan
- 2Department of Microbiology, University of Balochistan, Quetta, Pakistan
Berberis baluchistanica Ahrendt is a medicinal plant known to have potential for the treatment of various diseases. In the present study, the ethanolic extracts of the bark, leaves, and roots of B. baluchistanica plant were evaluated for in vitro antimicrobial, anti-leishmanial, anticancer, and anti-inflammatory activities. The antibacterial and antifungal activities were determined by agar mix and agar well diffusion method. All extracts showed potential activity against the target bacteria (Bacillus subtilis, Bacillus licheniformis, Escherichia coli, Klebsiella pneumoniae, Pseudomonas aeruginosa, Rhodococcus erythropolis, Salmonella typhi, and Staphylococcus aureus) and fungal strains (Aspergillus flavus, Aspergillus niger, and Mucor mucedo). S. aureus proved to be the most sensitive strain for each extract, with a maximum zone of inhibition for bark at 23 ± 0.12 mm, for leaves at 22 ± 0.36 mm, and for root extracts at 20.21 ± 0.06 mm). The minimum inhibitory concentration values of B. baluchistanica bark, leaves, and roots for different target bacterial strains ranged from 1.56 to 25 mg ml–1, and the minimum bactericidal concentrations were in the range of 3.12 to 25 mg ml–1, respectively. The root extract possessed potent antifungal activity against A. flavus with 83% of growth inhibition, A. niger with 80%, and M. mucedo with 73%. The bark extract was found active against M. mucedo with 86% of inhibition, followed by 70% against A. flavus and 60% against A. niger. The leaf extract showed a significant response by 83% inhibition against M. mucedo, followed by A. flavus and A. niger with 73 and 72% inhibition, respectively. In an anti-leishmanial bioassay, the inhibitory concentration (IC50) was observed for each extract against Leishmania major. The bark showed good activity (IC50 = 4.95 ± 0.36 mg/ml), followed by the roots (IC50 = 7.07 ± 0.18 mg/ml) and the leaves (IC50 = 8.25 ± 0.29 mg/ml). An evaluation of anticancer activity was done by using MTT cell assay against HeLa cell line. Upon comparing the values of each extract to the standard, it was revealed that the ethanolic bark extract showed the highest anticancer activity with IC50 = (12 ± 0.15 μg/ml), followed by the roots (14 ± 0.15 μg/ml) and the leaves (17 ± 0.21 μg/ml), respectively. The anti-inflammatory assay was undertaken by the inhibition of albumin denaturation activity, proteinase inhibitory activity, and heat-induced hemolysis activity. The IC50 value for protein denaturation of the bark was IC50 = 0.64 ± 0.25 mg/ml, followed by the roots (0.67 ± 0.21 mg/ml) and the leaves (0.73 ± 0.13 mg/ml). The proteinase inhibitory activity of the bark extract was IC50 = 0.55 ± 0.12 mg/ml, followed by the leaves (0.62 ± 0.23 mg/ml) and the roots (0.69 ± 0.15 mg/ml), respectively. For heat-induced hemolysis assay, the bark showed the lowest IC50 value (0.48 ± 0.15 mg/ml) compared to the leaves (0.52 ± 0.35 mg/ml) and the roots (0.58 ± 0.05 mg/ml) of the plant. All analyzed parts of the B. baluchistanica plant showed significant biological activities which make the plant medicinally important and a good candidate for the isolation of antimicrobial, inflammatory, and anticancer compounds. Further studies may lead us to determine the active compounds responsible for the biological activities of the plant extracts.
Introduction
The use of medicinal plants as an alternative therapy for the prevention and treatment of various infectious diseases is an ancient practice (Tareen et al., 2016). Long before mankind, the subsistence of microbes was discovered; the idea was well accepted that some medicinal plants that contain active components belonging to different phytochemical groups had antimicrobial potential (Rios and Recio, 2005). The curative properties of various medicinal plants have been known to treat human and animal diseases. It is estimated by the World Health Organization (WHO) that about 80% of the world’s population still rely on traditional medicine for their primary healthcare needs due to their easy availability (Dos Santos et al., 2021). Medicinal plants with bioactive components having antibacterial, antifungal, and antioxidant properties are used for the treatment of various infections. These natural antimicrobial agents have great medicinal potentials because of the increasing resistance to the usual antibiotics and drugs (Pervez et al., 2019).
Antibiotics are one of the most essential weapons to be used against various infections and have greatly enhanced the quality of human life. However, in the last decades, these health advantages had lost their effectiveness not only because their many contraindications but also due to the rapid increase of multidrug resistance. Medicinal plants might represent an alternative treatment in the treatment of infectious diseases (Sailaja, 2014). They can also be a possible source for new potent antibiotic molecules to which pathogenic strains are not resistant. It is necessary to explore new drugs, for the healthcare system in developing countries, which could be cheaper and affordable and with a lower chance of resistance produced by common pathogens (Banik et al., 2014). Thus, the use of traditional therapies has prompted scientists to analyze the biological potentials of medicinal plants against various infection agents and to prove their effectiveness for their safe traditional uses.
Considering the vast potentiality of plants as a source for antimicrobial drugs, a systematic analysis was carried out to screen the local flora Berberis baluchistanica Ahrendt, locally known as Zralag in Pashto, Zarch in Brahvi, and Korae in Balochi, for different biological activities. It is endemic to Balochistan and belongs to family Berberidaceae. The plant is distributed in Harboi, Kalat, and Zarghun areas of Quetta and Ziarat (Uddin et al., 2021). This medicinal plant is valued for its bark and roots, considered as non-toxic and consumed in raw form either as powder or a decoction. As it contains berberine, the plant is used for the treatment of various diseases, like cough, fever, internal injury, eye disease, kidney stone removal, wound healing, rheumatism, and other infections of human beings and livestock (Baloch et al., 2013). Recently, various secondary metabolites, like berberisinol, berberine, 8-oxoberberine, oleanolic acid, palmatine, gallic acid, phenols, carotenoids, and vitamins, were isolated and found with remarkable antioxidant, anti-leishmanial, anti-diabetic, antibacterial, and antifungal potential (Pervez et al., 2019).
The in vitro antibacterial, antifungal, anti-inflammatory, anti-leishmanial, anticancer, and detailed biological potential of the bark, leaf, and root ethanolic extracts of B. baluchistanica has not been reported to date. Therefore, the plant was tested for the development of an alternative drug line, and the present study was carried out to evaluate the antibacterial, antifungal, anti-inflammatory, anti-leishmanial, and anticancer effects of the bark, leaf, and root extracts of the plant against different microorganisms by using an in vitro assay method.
Materials and Methods
Plant Collection
Berberis baluchistanica plant was collected from the Ziarat region of Balochistan and identified by Dr. Saadullah Khan Leghari, professor at the Department of Botany, University of Balochistan Quetta. The voucher specimens were prepared, and the plant was deposited in the Herbarium, Department of Botany, University of Balochistan Quetta, Pakistan.
Sample Preparation
The bark, leaves, and roots of the plant were separated, washed off with distilled water in order to remove any kind of dust and contamination, and dried under the shade for 3 to 4 consecutive weeks at room temperature and under controlled humidity. The dried bark, leaves, and roots were separately ground into fine powder using an electrical grinder and stored in desiccators for further analysis.
Maceration Extraction of Bioactive Compounds
In maceration extraction, 200 g of fine grounded powder was used for extraction using 2 L ethanol as solvent with 1:10 ratio, following standard procedures described by Akbar et al. (2019a). The process was conducted in a dark room to avoid light exposure. The flask was occasionally shaken with specific intervals. The ethanolic mixture was filtered with Whatman filter paper no. 1. Each extract was dried with the help of a rotary evaporator and used for further analysis.
Biological Analysis
The following biological activities were performed on the bark, leaves, and roots of B. baluchistanica.
Antibacterial Activity
The antibacterial activity of B. baluchistanica bark, leaf, and root extracts was determined by using agar well diffusion method (Akbar et al., 2019a). Freshly prepared sterilized culture media were inoculated with the target bacterial strains (Bacillus subtilis, Bacillus licheniformis, Escherichia coli, Klebsiella pneumoniae, Pseudomonas aeruginosa, Rhodococcus erythropolis, Salmonella typhi, and Staphylococcus aureus) and incubated at 37°C for 24 h. The target bacterial cultures were spread over the surface of sterilized Muller Hinton Agar (Oxoid, UK) plates with the help of a sterilized cotton swab for the determination of antibacterial activity. Wells were made through 6-mm sterilized cork borers in agar plates, and extracts were added into the agar wells. Dimethyl sulfoxide (DMSO) was used as negative control, and the antimicrobial drug doxycycline was used as a positive control. The plates were incubated at 37°C for 24 h. The results were interpreted by measuring the diameter of the clear zone around the wells in millimeter (mm).
Minimum Inhibitory and Minimum Bactericidal Concentration
The minimum inhibitory concentration (MIC) and minimum bacterial concentration (MBC) of each extract were determined by the method described by Sadiq et al. (2017) with minor modifications. Briefly, 50 mg/ml stock solution of each extract was prepared in DMSO. Twofold serial dilutions of each extract were prepared in sterile nutrient broth as per the manufacturer’s instruction to obtain the concentrations of 50, 25, 12.5, 6.25, 3.12, and 1.56 mg/ml. Each bacterial inoculum of approximately 105–106 colony-forming units (CFU)/ml was introduced in each concentration of the extract and incubated at 37°C for 24 h in an incubator. The test tubes containing the extract-free nutrient broth with bacterial inoculums were used as the positive control, and the test tubes containing the extract and nutrient broth without inoculums were used as the negative control. The lowest concentration which showed no visible growth after 24 h of incubation period was considered as the MIC. The MBC determined by standard plate count method for the target bacteria by subculturing 1 ml from each dilution that had no eye-visible growth was inoculated on the surface of freshly prepared nutrient agar plates with the help of a sterile spreader and incubated at 37°C for 24 h. Each experiment was conducted in triplicates. The minimum concentration that had no visible growth on the agar plates after 24 h of incubation was measured as the MBC.
Kill Time Assay
Kill time study was used to calculate the bactericidal effects of the bark, leaves, and roots of B. baluchistanica following Akbar and Anal (2014) with slight modifications. Stock solutions (50 mg/ml) of each extract were used to prepare different dilutions by mixing with nutrient broth in clean and dried test tubes and sterilized under pressure at 121°C for 15 min in an autoclave. The kill time curves were established by inoculating the target bacterial strains B. subtilis, B. licheniformis, E. coli, K. pneumoniae, P. aeruginosa, R. erythropolis, S. typhi, and S. aureus at approximately 106CFU/ml in the broth containing each extract. Nutrient broth containing test bacteria without extract was used as the positive control. The growth and turbidity pattern of the inoculated test bacterial strains were observed at specific time intervals (0, 2, 6, 16, and 24 h) with the help of a spectrophotometer (T60 UV–Vis, PG UK) at 625 nm. The growth of the test bacterial species was also confirmed with the help of a standard plate count technique.
Antifungal Activity
The antifungal activities of the extract of B. baluchistanica was determined by agar well diffusion method following Akbar et al. (2019a). Briefly, 2 ml of each extract was dissolved in DMSO and transferred to freshly prepared 35-ml potato dextrose agar (Oxoid UK), shaken properly, and poured into petri plates. After solidification, 6-mm-diameter wells were made into the agar by a cork borer, and the fresh cultures of test fungi (A. flavus, A. niger, and M. mucedo) were poured into different wells and incubated at 37°C for 72 h. Media having organisms without extract and media alone were used as positive and negative controls, respectively. The antifungal drug fluconazole was used as the reference. The results of the growth inhibition of the target fungal species were recorded after 5 days of incubation. The growth inhibition of the target fungi was determined by measuring the linear growth in millimeter (mm) with reference to the negative control. The final results were calculated by the following equation:
In vitro Anti-leishmanial Susceptibility Assay
Parasite Culture
The promastigotes of Leishmania major were isolated from the previously isolated and preserved culture stored in the Food Microbiology and Bioprocess Laboratory, Department of Microbiology, University of Balochistan Quetta. The promastigotes were grown in NNN biphasic medium with penicillin and streptomycin.
The anti-leishmanial activity assay was done by the method previously described (Shaheen et al., 2020) but with minor modifications. The log phase of promastigotes at 1 × 106 cells/ml was used for the current assay. The ethanolic extracts of the bark, leaves, and roots of the medicinal plant B. baluchistanica were examined against L. major (promastigotes) in culture by means of a 96-well plate. The promastigotes of L. major were grown in NNN biphasic medium. Briefly, a stock solution (1 mg/ml) of the test sample was prepared in DMSO. A twofold serial dilution of each sample was carried out, and the activity was performed at different concentrations (1, 0.5, 0.25, 0.125, and 0.0625 mg/ml). Then, 10 μl of each plant dilution and 50 μl of the promastigote log-phase culture was dispensed to each well of a 96-well plate. Glucantime was used as a standard drug. Media with organism and DMSO were maintained as positive and negative controls, respectively. The titer plate was incubated for 72 h at 37°C. The assay was performed in triplicate. After incubation, 1 ml of DMSO was added to each well, and the percent mortality of the test and standard drug was confirmed by using 20 μl (5 mg/ml in phosphate buffer, pH 7.2) of NBT solution. The absorbance was measured at 630 nm by using Microplate Reader (RT- 6000), and the IC50 values of each extract with anti-leishmanial activities were calculated by the linear regression method. The percent of cell viability was calculated by using the following formula:
MTT Cell Assay
Cell Culture
HeLa cell line (cervical cancer carcinoma) was cultured in a humidified atmosphere with 5% CO2 in minimal essential medium supplemented with 10% FBS, 100 units/ml penicillin, and 100 μg/ml streptomycin at 37°C.
The cytotoxic effect of the ethanolic extracts of the bark, leaves, and roots against HeLa (cervical cancer carcinoma) was determined by a rapid colorimetric assay using MTT 3-(4, 5- dimethylthiazol-2-yl)-2, 5-diphenyltetrazolium bromide (Javed et al., 2021). Briefly, 180 μl of cell suspension (1 × 105 cell/ml) was seeded in 96-well plates and incubated at 37°C at 5% CO2 condition, treated with 100 μg/ml of each extract, and incubated for 48 h. The cell viability was evaluated by dissolving MTT in phosphate-buffered saline (PBS, pH 7.2), and 20 μl (5 mg/ml in phosphate buffer) of 0.5% 3-(4, 5-dimethylthiazol-2-yl)-2, 5-diphenyltetrazolium bromide (MTT solution) was added and incubated for 3 h. After incubation, the supernatant in each well was carefully removed, and 1 ml of DMSO was added to each well. The amount of formazan formed was determined by measuring the absorbance at 570 nm with a UV spectrophotometer. Doxorubicin (100 μg/ml) was used as the standard and DMSO was used as the negative control, respectively. A standard curve (absorbance against the number of cells) was plotted, and measurements were performed to calculate the concentration required for 50% inhibition (IC50). The percent of cell viability was calculated by using the following formula:
Assessment of in vitro Anti-inflammatory Activity
Protein Denaturation Assay
The anti-inflammatory activity of B. baluchistanica bark, leaf, and root extracts was carried out by inhibition of protein denaturation assay according to the method of Yesmin et al. (2020) with slight modifications. The reaction mixture (5 ml) consisted of 0.2 ml of 1% bovine albumin, 3.8 ml of PBS (pH 6.4), and 1 ml of each extract (1 mg/ml), was mixed, and was incubated in a water bath (37°C) for 15 min. Then, the reaction mixture was heated at 70°C for 5 min. After cooling, the absorbance was measured at 660 nm using a spectrophotometer (T60 UV-Vis. PG UK). Phosphate buffer solution was used as control. The percentage inhibition of protein denaturation was calculated by using the following formula:
where A1 = absorption of the control sample, and A2 = absorption of the test sample.
Proteinase Inhibitory Activity
To examine the in vitro anti-inflammatory action, proteinase inhibitory activity of the bark, leaf, and root extracts was performed according to the method of Gunathilake et al. (2018). The reaction solution (2 ml) consisted of 0.06 mg trypsin, 1 ml of 20 mM Tris–HCl buffer (pH 7.4), and 1 ml of each extract dilution sample. The mixture was incubated (37°C for 5 min), and then 1 ml of 0.8% (w/v) casein was added, followed by further incubation for 20 min. At the end of incubation, 2 ml of 70% perchloric acid was added to conclude the reaction. The mixture was centrifuged, and the absorbance of the supernatant was measured at 210 nm. Phosphate buffer solution was used as the control, and aspirin was used as the standard drug for the experiment. The percentage inhibition was calculated by using the following formula:
Heat-Induced Hemolysis Assay
Preparation of Erythrocyte Suspension
Erythrocyte suspension was prepared according to the method described in Yesmin et al. (2020) with some modifications. Whole human blood was collected from a healthy human. Sodium oxalate was used to prevent blood clotting. For supernatant removal, the blood was centrifuged at 2,500 rpm for 5 min. The mixture was washed with an equal volume of normal saline (0.9% NaCl), and centrifugation was done at 2,500 rpm for 5 min. The process was done three times, and the blood volume was measured and reconstituted as 10% (v/v) suspension with isotonic buffer solution (10 mM sodium phosphate buffer, pH 7.4). The composition of the buffer solution (g/L) used was NaH2PO4⋅2H2O (0.2 g), Na2HPO4 (1.15 g), and NaCl (9.0 g).
The heat-induced hemolysis activity was carried out as described by Gunathilake et al. (2018) with some modifications. Briefly, 0.05 ml of blood cell suspension and 0.05 ml of each extract were mixed with 2.95 ml phosphate buffer (pH 7.4). The mixture was incubated at 54°C for 20 min in water bath. After the incubation, the mixture was centrifuged (2,500 rpm for 3 min), and the absorbance of the supernatant was measured at 540 nm using a spectrophotometer (T60 UV–vis. PG UK). Phosphate buffer solution was used as the control, and aspirin was used as the standard drug for the experiment. The percent inhibition of hemolysis was calculated using the following equation:
where A1 = absorption of the control and A2 = absorption of the test sample.
Statistical Analysis
The results of all the measured data in each experiment were expressed as average ± standard deviations (SD). The magnitude of the means, standard curve, and standard deviations were calculated by using MS Excel 2010 Software. The inhibitory concentrations (IC50) were measured by the linear regression method. Significant group difference (p < 0.05) between means was determined by using SPSS software.
Results
Antibacterial Activity
The bark, leaf, and root extracts of the B. baluchistanica plant was tested for its antibacterial properties against different bacterial strains. The diameter of the inhibition zones of the bark extract against B. subtilis, B. licheniformis, E. coli, K. pneumonia, P. aeruginosa, R. erythropolis, S. typhi, and S. aureus was 15 ± 0.23, 18 ± 0.54, 22 ± 0.51, 13 ± 1.16, 12 ± 1.13, 16 ± 0.12, 15 ± 0.74, and 23 ± 0.36 mm, respectively. The leaf extract showed a relatively higher activity, and the zone of inhibition against B. subtilis was 16 ± 1.01 mm, B. licheniformis 17 ± 1.02 mm, E. coli 19 ± 0.47 mm, K. pneumonia 16 ± 0.61 mm, P. aeruginosa 11 ± 0.15 mm, R. erythropolis 18 ± 1.12 mm, S. typhi 10 ± 0.53 mm, and S. aureus 22 ± 0.12 mm. The calculated results for the root extracts were 12 ± 0.41, 14 ± 0.32, 23.14 ± 1.16, 7 ± 0.01, 14 ± 0.41, 15 ± 0.24, 14 ± 1.06, and 20.21 ± 0.06 mm against B. subtilis, B. licheniformis, E. coli, K. pneumonia, P. aeruginosa, R. erythropolis, S. typhi, and S. aureus (Table 1). All the extracts were found active against the selected target bacteria, except K. pneumonia that was resistant to root extracts (Figure 1).
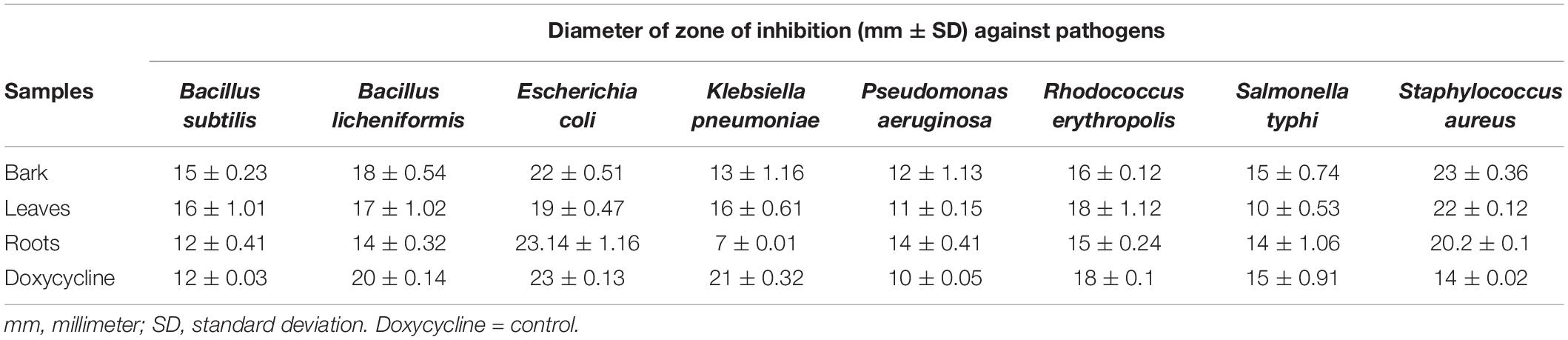
Table 1. Antibacterial activity of different parts of Berberis baluchistanica with inhibitory zones in mm as mean ± standard deviation.
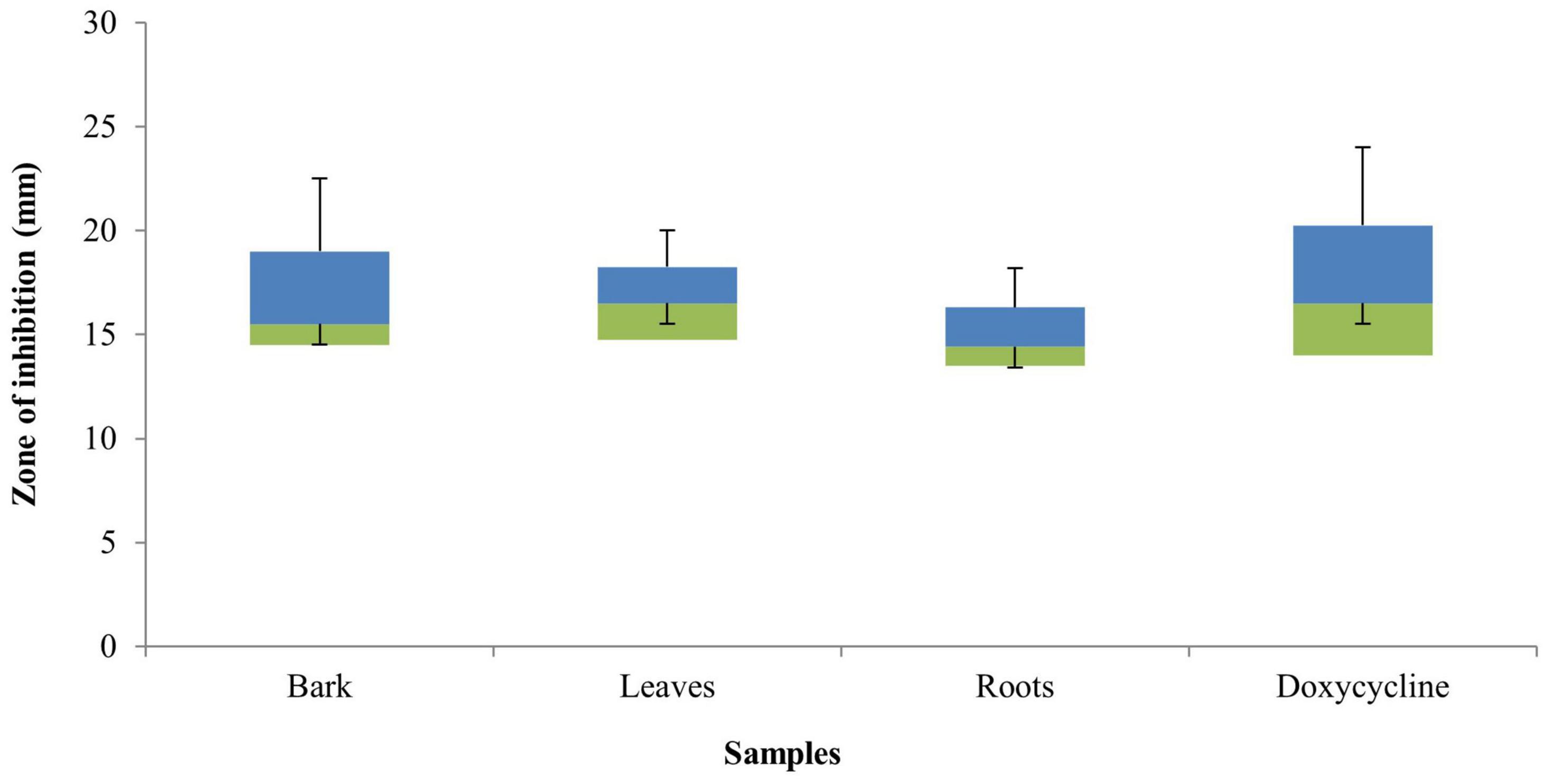
Figure 1. Box plot graph of the antibacterial activity of Berberis baluchistanica bark, leaf, and root extracts against various bacterial strains. Bars represent the standard deviation of the mean.
Minimum Inhibitory and Minimum Bactericidal Concentration
The MIC values of B. baluchistanica bark, leaves, and roots for different target test bacterial strains B. subtilis, B. licheniformis, E. coli, K. pneumoniae, P. aeruginosa, R. erythropolis, S. typhi, and S. aureus ranged from 1.56 to 25 mg ml–1 (Table 2). The MIC for the bark extract was 1.56 mg ml–1 against S. aureus and 3.12 mg ml–1 against S. typhi, while the bactericidal effect of the extract was above the MIC concentration range tested. For the leaf extract, the MIC was found as 1.56 mg ml–1 against E. coli and S. aureus, and the highest was 6.25 mg ml–1 against R. erythropolis. For the root extracts, the MIC was found to be 1.56 mg ml–1 against E. coli and S. aureus and highest against K. pneumoniae (25 mg ml–1). Based on the conducted research, it was found that the ethanolic extract of all parts strongly inhibited the growth of E. coli, S. aureus, S. typhi, and P. aeruginosa, while K. pneumoniae turned out to be the most resistant strains for root extracts (Figure 2). The MBC of the B. baluchistanica extracts were in the range of 3.12 to 25 mg ml–1. The root extracts for the bacterial strains B. subtilis and R. erythropolis were bacteriostatic, while both the leaf and root extracts were bacteriostatic for B. licheniformis (Table 2).
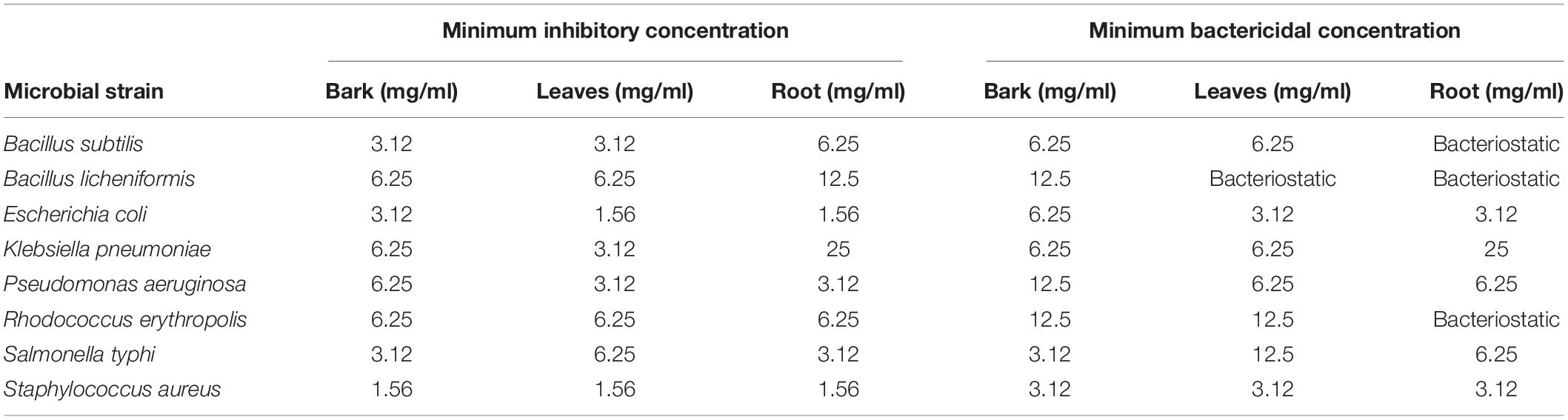
Table 2. Minimum inhibitory concentration and minimum bactericidal concentration of Berberis baluchistanica.
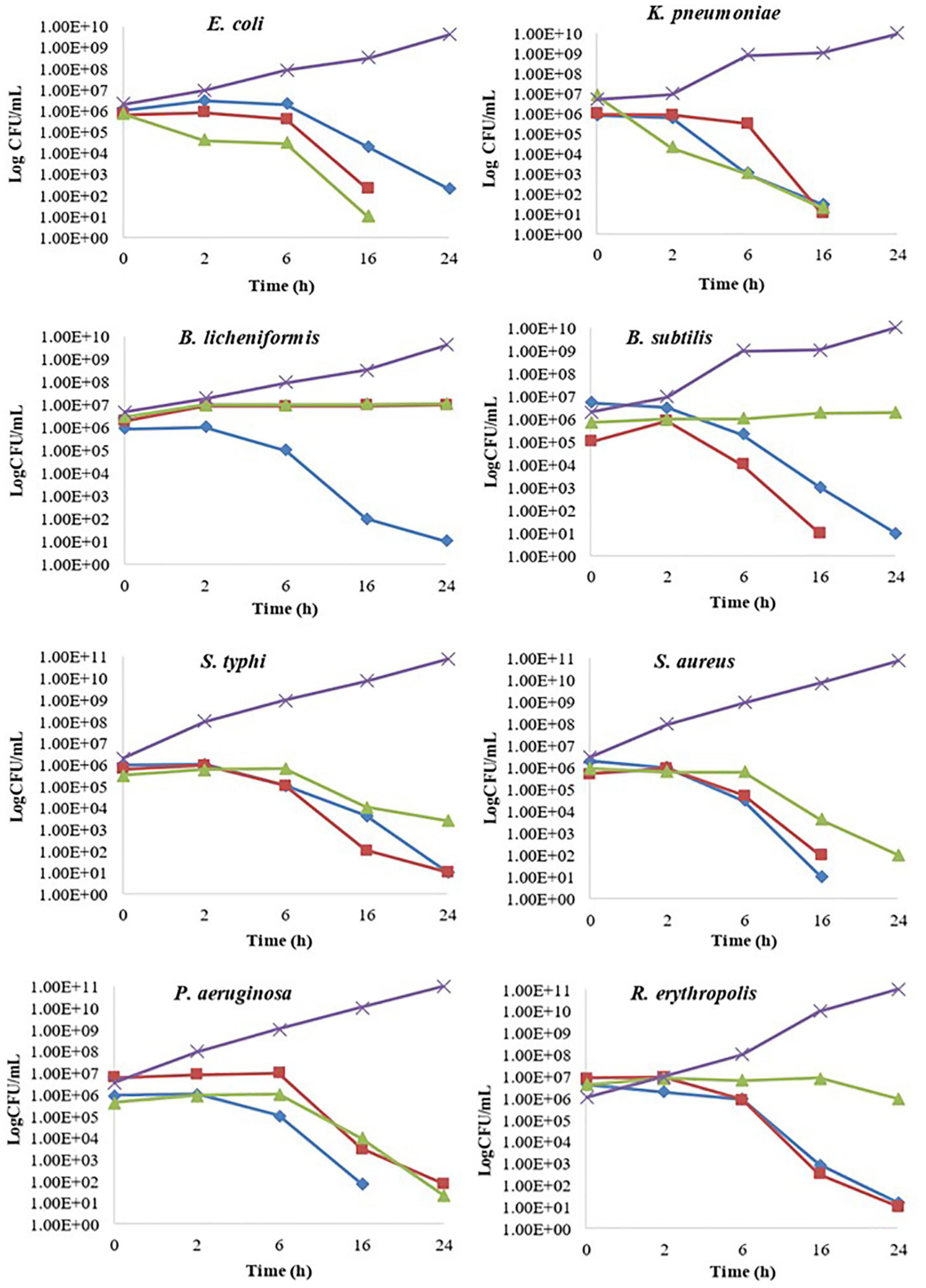
Figure 2. Kill time assay of Berberis baluchistanica bark, leaf, and root extracts against the target bacteria. In the graphs, ◆ represents bark ethanolic extract, ■ represents leaves ethanolic extract, ▲ represent roots ethanolic extract, and × refers to the positive control.
Kill Time Assay
The kill time assay for target bacterial strains B. subtilis, B. licheniformis, E. coli, K. pneumoniae, P. aeruginosa, R. erythropolis, S. typhi, and S. aureus was performed by analyzing the growth decrease in log CFU/ml with time at different concentrations of B. baluchistanica bark, leaf, and root extracts compared to the positive controls, respectively. All extracts at their MIC inhibited the growth of bacteria and showed a significant (p < 0.05) decrease in bacterial viability over a period of 24 h compared to the positive control. The extract was considered bacteriostatic at the lowest concentration that reduced the original inoculum size to 0–3 log CFU/ml and bactericidal if the inoculum size was reduced by > 3 log CFU/ml. Almost all bacteria were sensitive against each extract and showed a bactericidal effect in 24 h of interaction. The effects of bark, leaf, and root extracts on each bacterial cell viability are shown in Figure 2. B. licheniformis, when treated with bark extract, showed a decrease in viability that was more than 3 log CFU/ml, indicating a bactericidal effect at 24 h. The leaf and root extracts showed a bacteriostatic effect against B. licheniformis as the bacterial count was less than 3 log CFU/ml at 24 h, whereas in the case of B. subtilis, when treated with bark and leaf extracts, bactericidal effects were observed after 6 h of incubation, and the decrease in bacterial count was more than 3 log CFU/ml. Complete bacterial elimination was observed after 16 h, but the root extracts showed a bacteriostatic effect.
Antifungal Activity
The antifungal activity of B. baluchistanica bark leaves and root extracts was evaluated by measuring the percentage of growth inhibition potential against three filamentous fungi (A. flavus, A. niger, and M. mucedo). Comparing our results with the antifungal reference drug fluconazole, each extract was found active against all three fungi. The root extract possessed a potent antifungal activity against A. flavus with 83% growth inhibition and against A. niger with 80% but showed a relatively less effect on the growth of M. mucedo with 73% of inhibition. The bark extract was found active in a similar study against M. mucedo with 86% growth inhibition, followed by 70% against A. flavus and less inhibition against A. niger at 60%. The leaf extracts showed an active response of 83% inhibition against M. mucedo, followed by A. flavus and A. niger with 73 and 72% inhibition, respectively (Figure 3). The antifungal drug fluconazole was much potent with 98% inhibition against M. mucedo, 96% against A. niger, and 95% against A. flavus (Table 3).
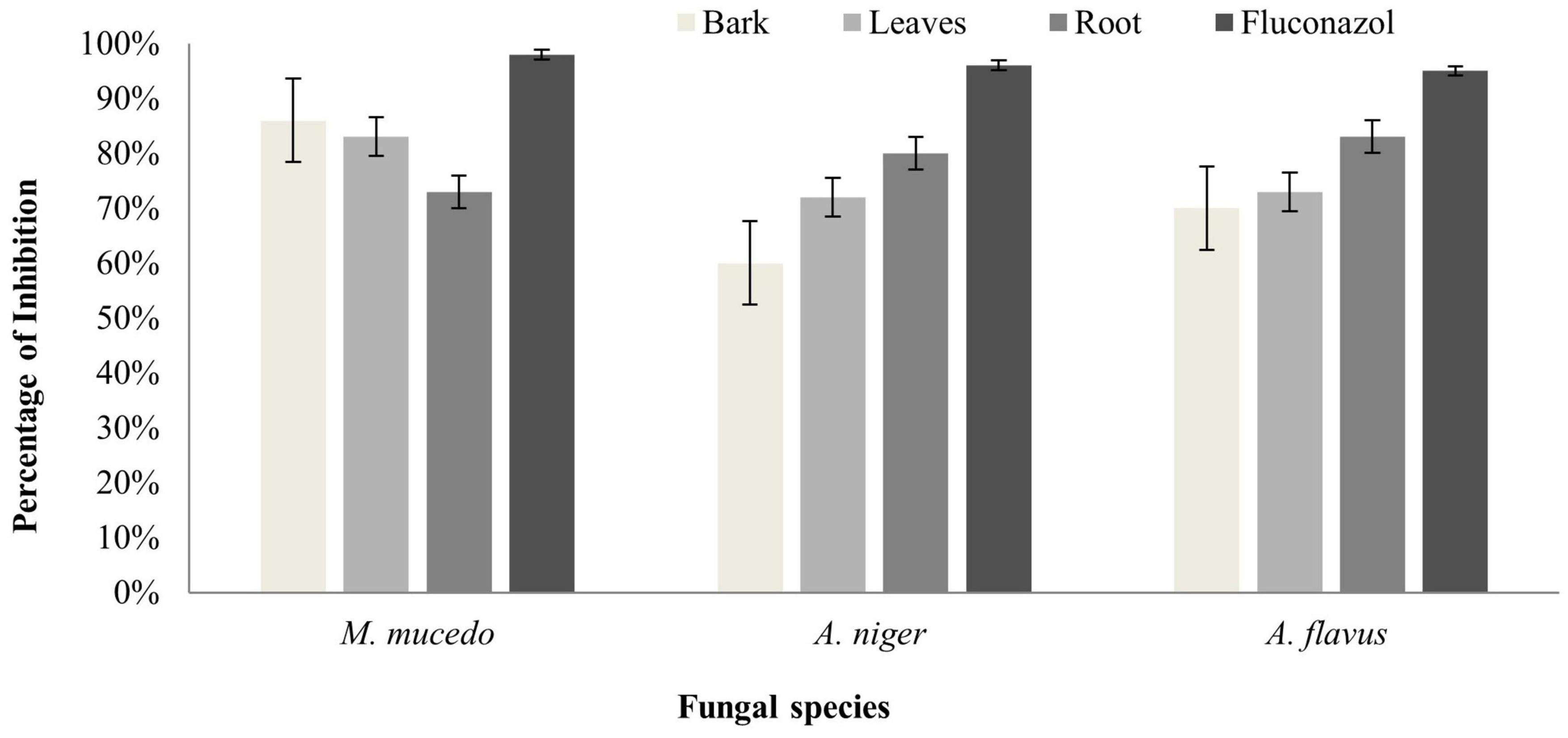
Figure 3. Antifungal activity of Berberis baluchistanica bark leaves and roots against fungal species Mucor mucedo, Aspergillus niger, and Aspergillus flavus. Bars represent the standard deviation of the mean.
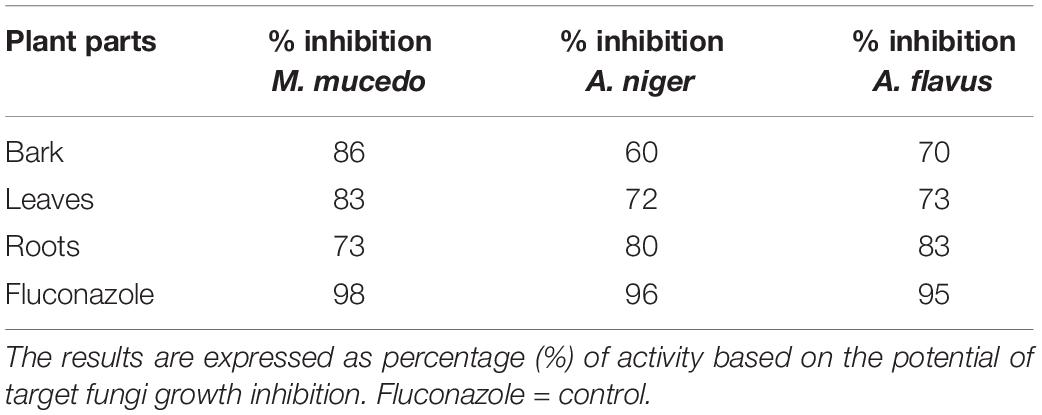
Table 3. Antifungal activity of Berberis baluchistanica bark, leaves, and roots against three fungal species.
Anti-leishmanial Assay
Anti-leishmanial assay was used for determining the anti-leishmanial activity of promastigotes (L. major). A twofold serial dilution of each sample was carried out, and the activity was performed at different concentrations (1, 0.5, 0.25, and 0.125 mg/ml). The standard drug glucantime (IC50 = 5.41 ± 0.23 cmg/ml) was used to compare the parasite inhibition with each extract. The IC50 value was observed for each extract against L. major, and the bark showed good potential (IC50 = 4.95 ± 0.36 mg/ml), followed by the roots (IC50 = 7.07 ± 0.18 mg/ml) and leaves (IC50 = 8.25 ± 0.29 mg/ml) by comparing the values of each extract with the standards (Table 4). The results revealed that, in all concentrations, the standard drug glucantime reduced the viability compared with each extract (P < 0.05) and control (P < 0.05). The order of% inhibition activity was bark > roots > leaves. The highest activity was observed at the concentration of 1 mg/ml, and the viability increased with a decrease in concentration, as presented in Figure 4.
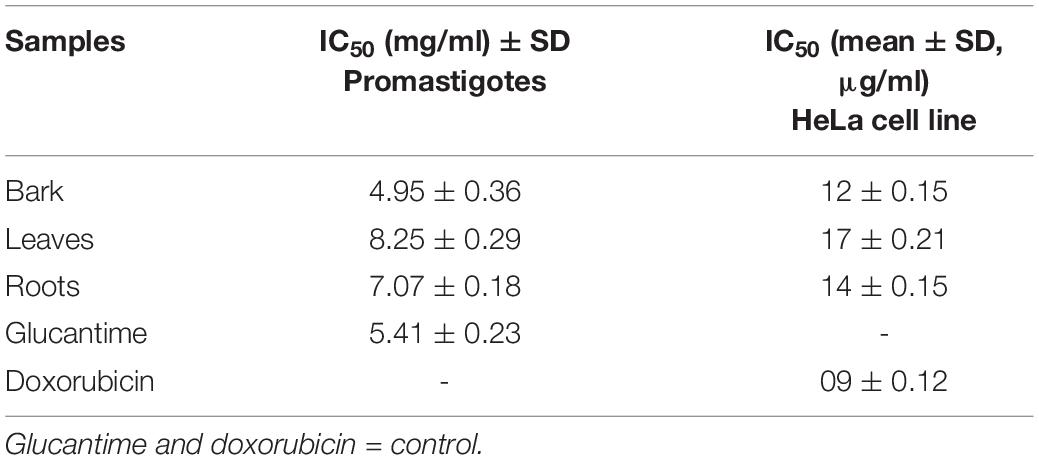
Table 4. Estimated IC50 values of bark, leaves, and roots of Berberis baluchistanica against promastigotes and HeLa cell line.
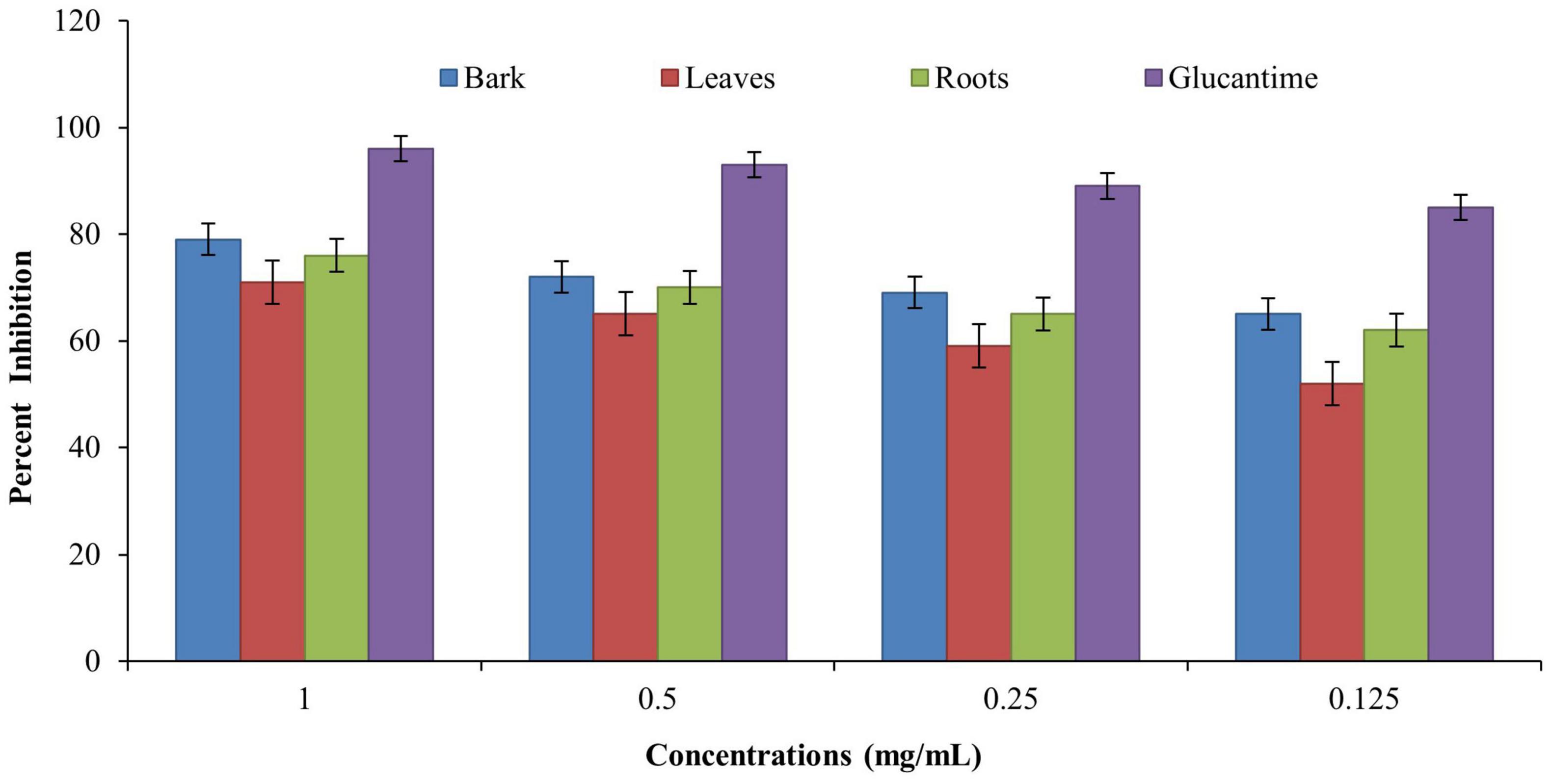
Figure 4. Anti-leishmanial activities of Berberis baluchistanica bark leaves and roots against promastigotes (Leishmania major). Bars represent the standard deviation of the mean.
MTT Cell Assay
Evaluation of the anticancer activity of the ethanolic extracts of the bark, leaves, and roots of the B. baluchistanica plant was done by using MTT cell assay against HeLa cell line. The assay was performed at 100 μg/ml for each extract, and doxorubicin was used as the standard drug to compare the inhibition with each extract. Each extract exhibited a higher anticancer (HeLa cell lines) activity and% inhibition in terms of IC50. Comparing the values to the standard (IC50 09 ± 0.12 μg/ml), it was revealed that the ethanolic bark extract showed the highest anticancer activity with lowest IC50 (12 ± 0.15 μg/ml), followed by the roots (14 ± 0.15 μg/ml) and leaves (17 ± 0.21 μg/ml), respectively, as shown in Table 4. The results revealed that, in all concentrations, the standard drug doxorubicin reduced the viability compared with each extract, and the data was significantly different from each other (P < 0.05), as shown in Figure 5.
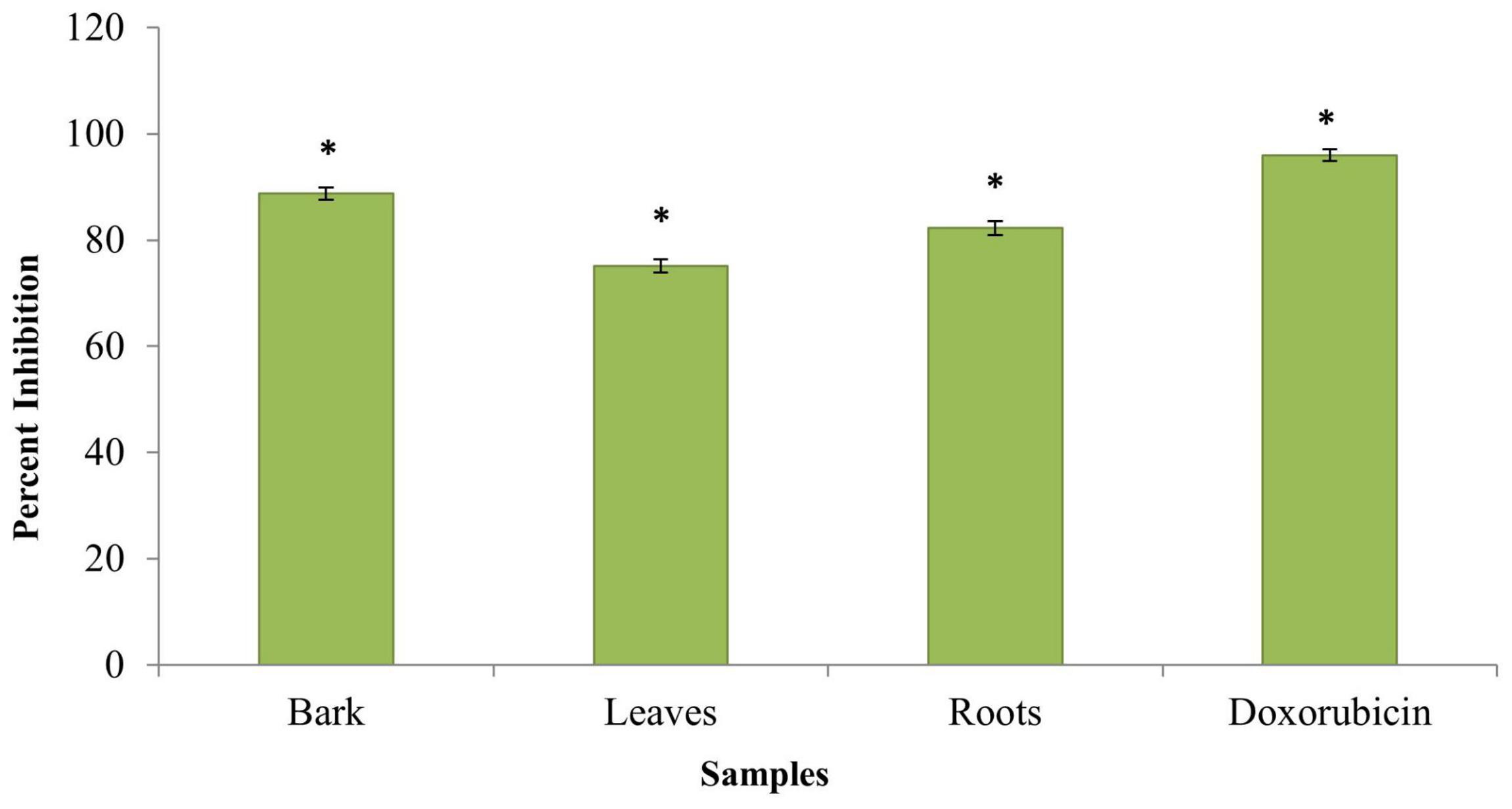
Figure 5. Anticancer activity of Berberis baluchistanica bark leaves and roots against HeLa cell line. Bars represent the standard deviation of the mean. Significant (P < 0.05) differences between groups are indicated by an asterisk.
Assessment of in vitro Anti-inflammatory Activity
Inhibition of Protein Denaturation
The ethanolic extracts of the bark, leaves, and roots were able to inhibit protein denaturation, and the inhibitions were in a concentration-dependent manner. The% inhibition of protein denaturation of all extracts was within the range from 48 to 81% at the concentration of 1–0.125 mg/ml, as shown in Figure 6A. The bark of B. baluchistanica revealed a significantly higher (p < 0.05) level of inhibition compared with those of the leaves and the roots. The IC50 value was observed for each extract, and the bark showed good potential (IC50 0.64 ± 0.25 mg/ml), followed by the roots (IC50 = 0.67 ± 0.21 mg/ml) and the leaves (IC50 = 0.73 ± 0.13 mg/ml). By comparing the values of each extract to the standard, the order of% inhibition was bark > roots > leaves. The results revealed that, in all concentrations, the standard drug aspirin (IC50 = 0.43 ± 0.10 mg/ml) showed a relatively higher inhibition compared to the extracts (Table 5).
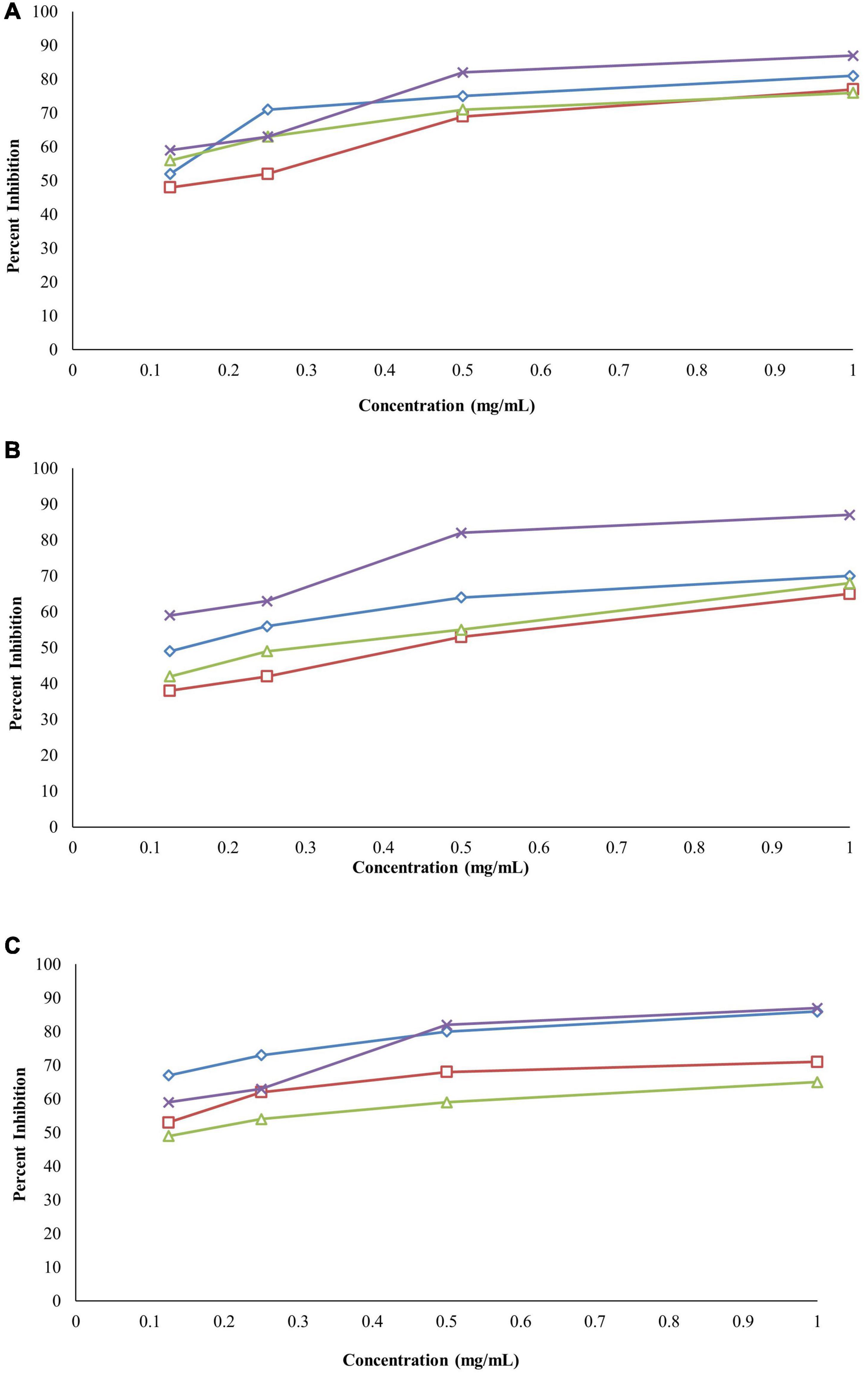
Figure 6. (A) Anti-inflammatory activity of Berberis baluchistanica bark leaves and roots by protein denaturation assay graphs. ◊ represents bark, □ represents leaves, △ represent roots, and × refers to the positive control (aspirin). (B) Anti-inflammatory activity of B. baluchistanica bark leaves and roots by proteinase inhibition assay. ◊ represents bark, □ represents leaves, △ represents roots, and × refers to the positive control (aspirin). (C) Anti-inflammatory activity of B. baluchistanica bark leaves and roots by heat-induced hemolysis assay. ◊ represents bark, □ represents leaves, △ represents roots, and × refers to the positive control (aspirin).
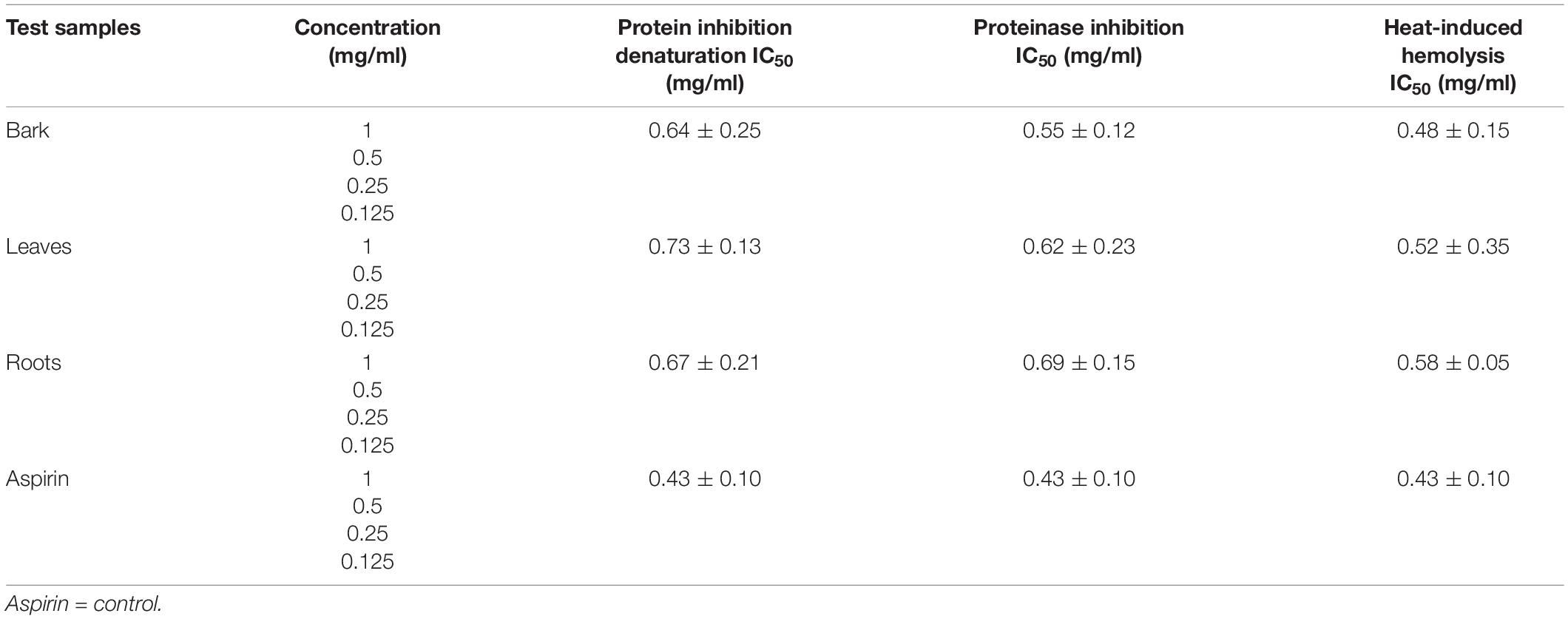
Table 5. IC50 value of in vitro anti-inflammatory assay carried out on Berberis baluchistanica bark, leaf, and root extracts.
Proteinase Inhibitory Activity
The bark, leaf, and root extracts of the B. baluchistanica plant were tested for its anti-inflammatory potential by proteinase inhibitory activity. The activity was performed at 1–0.125 mg/ml for each extract, and aspirin was used as the standard drug. Each extract exhibited good proteinase inhibition. The highest activity was observed at the concentration of 1 mg/ml, and the inhibition decreased with a decrease in concentration, as shown in Figure 6B. By comparing the obtained values of each extract to the standard drug aspirin (IC50 = 0.43 ± 0.10 mg/ml), it was revealed that ethanolic bark extract showed significantly higher (p < 0.05) levels of proteinase inhibition with lowest IC50 (0.55 ± 0.12 mg/ml), followed by the leaves (0.62 ± 0.23 mg/ml) and roots (0.69 ± 0.15 mg/ml), respectively. The order of% inhibition of extracts was bark > leaves > roots, as shown in Table 5.
Heat-Induced Hemolysis
The anti-inflammatory effect of the ethanolic extracts of the bark, leaves, and roots of the B. baluchistanica plant was carried out by heat-induced hemolysis. The percent inhibition of heat-induced hemolysis of red blood cells was analyzed at different concentrations (1–0.125 mg/ml). The ethanolic extracts of each part were able to inhibit hemolysis in a concentration-dependent manner, as presented in Figure 6C. The% inhibition of hemolysis was within the range from 49 to 86%. The bark extract showed significantly higher (p < 0.05) levels of hemolysis inhibition with the lowest IC50 value of 0.48 ± 0.15 mg/ml compared with the leaves (0.52 ± 0.35 mg/ml) and the roots (0.58 ± 0.05 mg/ml) of the plant. By comparing the% inhibition to the standard drug (aspirin, IC50 0.43 ± 0.10 mg/ml), the order of the% inhibition of extracts was aspirin > bark > leaves > roots (Table 5).
Discussion
The antibacterial activity of the B. baluchistanica bark, leaf, and root extracts was determined by using agar well diffusion method. All extracts showed effective antibacterial activity against the bacterial strains of B. subtilis, B. licheniformis, E. coli, K. pneumonia, P. aeruginosa, R. erythropolis, S. typhi, and S. aureus. The inhibition zone values for the tested agents are recorded in mm and presented in Table 1. The zones of inhibition less than 10 mm are referred to as weak; those with 10–16 mm are considered moderate, and those higher than 16 mm are active (Irshad et al., 2018). S. aureus was found to be the most sensitive strain in this study, with a maximum zone of inhibition of 23 ± 0.36 mm for the bark and 22 ± 0.12 mm for the leaves, followed by the root extracts (20.21 ± 0.06 mm). E. coli was found to be highly sensitive against root extracts with a maximum zone of inhibition at 23.14 ± 1.16 mm, at 22 ± 0.51 mm for the bark, and comparatively less sensitive to leave extracts at 19 ± 0.47 mm. The results revealed that all selected test pathogens were susceptible to each extract except the Gram-negative bacterium K. pneumonia that turned out to be the most resistant strain for the roots (7 ± 0.01 mm) and showed a minimum inhibition. In comparison with the standard drug doxycycline, the bark extract showed significant effectiveness against both Gram-positive and Gram-negative bacteria, followed by the leaf and root extracts (Figure 1). The results of the current study were in agreement with those previously reported (Uddin et al., 2021) and relatively higher than those found in the literature (Kakar et al., 2012). However, Pervez et al. (2019) evaluated the antibacterial potential of berberisinol that exhibited a significant activity against E. coli, S. aureus, and S. pyogenes, a moderate activity against B. subtilis and K. pneumoniae, and a weak activity against P. aeruginosa. The obtained results are in agreement with the recently reported antibacterial activity of the methanolic extract from the roots of B. baluchistanica against S. aureus, B. subtilis, P. aeruginosa, and E. coli with 30-, 22-, 19-, and 29-mm zones of inhibition (Idrees et al., 2021). A similar trend of interactions was observed with the previously documented results of other species of Berberis (Ilyas et al., 2021). The results suggested that each extract possesses a broad spectrum of antimicrobial potentials. The strong antibacterial effect of the obtained extracts is probably due to the presence of secondary metabolites, such as alkaloids, steroids, coumarin, saponins, and terpenoids, and a high content of phenolics and flavonoids which have been reported to be involved in the inhibition of nucleic acid biosynthesis and other metabolic processes (Iqbal et al., 2020).
The MIC values of the B. baluchistanica bark, leaves, and roots for different target bacterial strains B. subtilis, B. licheniformis, E. coli, K. pneumoniae, P. aeruginosa, R. erythropolis, S. typhi, and S. aureus ranged from 1.56 to 25 mg ml–1, and the MBC values of the B. baluchistanica extracts were in the range of 3.12 to 25 mg ml–1, respectively. The bark and leaf extracts showed the lowest MIC and MBC values compared with the root extract. The MIC values of the root extracts against multidrug resistant E. coli and S. aureus were found to be lower in the current study, while the MIC values for K. pneumoniae and P. aeruginosa were found to be relatively higher than previously reported (Kakar et al., 2012). The highest bacteriostatic effect of leaves was observed against B. licheniformis, and for root extracts it was observed against B. licheniformis, B. subtilis, and R. erythropolis. Other species of Berberis have also exhibited excellent antibacterial activities (Rafique and Salman, 2021). The hydro-alcoholic extracts of the stem and roots of B. aristata, B. chitria, and B. lyceum exhibited significant activity against E. coli, K. pneumoniae, P. aeruginosa, S. aureus, S. pneumoniae, and some other bacteria. However, none of the above-mentioned four Berberis species showed any activity against S. typhimurium except B. lyceum (Srivastava et al., 2006).
In the kill time assay, the target bacterial strains showed a decrease in viability when exposed to the extracts. During the first 2 h, there were an increased number of all the strains when treated with each sample. Later, there was a slower growth compared with the control, which clearly indicated that the extract of the bark, leaves, and roots inhibited the growth of the bacteria. The obtained results showed that the bark extract restrained the growth of E. coli cells. However, the leaf and root extracts, at a very minimal concentration, killed the cells by the end of 24 h. The effects of the bark, leaf, and root extracts on the viability of K. pneumoniae were somehow similar. The cells had a reduced target bacterial count with the passage of time, and this was completely killed at 24 h. B. licheniformis, when treated with the bark extract, showed a decrease in viability that was more than 3 log CFU/ml, indicating a bactericidal effect at 24 h. However, the leaf and root extracts showed a bacteriostatic effect as the bacterial count was less than 3 log CFU/ml at 24 h, whereas in the case of B. subtilis, when treated with the bark and leaf extracts, bactericidal effects were observed after 6 h of incubation, and the decrease in bacterial count was more than 3 log CFU/ml. Complete bacterial elimination was observed after 16 h; however, the root extracts showed a bacteriostatic effect. Complete elimination of P. aeruginosa, R. erythropolis, S. typhi, and S. aureus cells was observed after 24 h of treatment with the bark, leaf, and root extracts. The bacterial growth in each control sample was increased with the passage of time. The obtained results supported previous research that reported a complete reduction in the growth of S. typhi, E. coli, and S. aureus in the kill time assay (Sadiq et al., 2017; Akbar et al., 2019b).
The antifungal activity of the B. baluchistanica bark, leaf, and root extracts was evaluated by measuring the percentage of inhibition zone against three filamentous fungi (A. flavus, A. niger, M. mucedo). These fungal pathogens were cultured using autoclaved potato dextrose agar. Comparing our results with the antifungal drug fluconazole, each extract was found to be active against all three fungi. The root extract possessed potent antifungal activity against A. flavus with 83% of growth inhibition and against A. niger with 80% but showed a relatively less effect on the growth of M. mucedo with 73% of inhibition zone. The bark extract was found to be active in a similar study against M. mucedo with 86% of inhibition, followed by 70% against A. flavus and less inhibition against A. niger at 60%. The leaf extract showed an active response of 83% inhibition against M. mucedo, followed by A. flavus and A. niger with 73 and 72% inhibition, respectively. The antifungal drug fluconazole was much potent with 98% inhibition against M. mucedo, 96% against A. niger, and 95% against A. flavus (Table 3). Recently, Uddin et al. (2021) reported the BBS-NiONP antifungal response against three fungal species: A. alternate, A. niger, and F. oxysporum with the following manner of inhibition: A. alternata > A. niger > F. oxysporum. Previously, Pervez et al. (2019) determined the antifungal potential of the compound berberisinol against the different fungal strains of C. glabrata, A. flavus, M. canis, F. solani, C. albicans, and A. niger that showed no significant antifungal activity against all the fungal strains.
According to Khan et al. (2012), the crude methanolic extract of the roots of B. baluchistanica showed a maximum activity against C. albicans and M. canis with% inhibition of 62 and 51%, respectively, and least inhibition (11%) against A. flavus.
Leishmaniasis is an infectious parasitic disease that is caused by a Leishmania strain that causes cutaneous, mucocutaneous, and visceral diseases, especially in developing countries. The disease has not been controlled due to the absence of an effective and easily available low-cost treatment. B. baluchistanica might control the disease due to its bioactive components and strong antimicrobial potentials.
Anti-leishmanial assay was the method used for determining the leishmanicidal activity, and promastigote (L. major) species was used for investigation. A twofold serial dilution of each sample was carried out, and the activity was performed at different concentrations (1, 0.5, 0.25, and 0.125 mg/ml). The standard drug glucantime (IC50 5.41 ± 0.23 mg/ml) was used to compare the parasite inhibition with each extract. The activity was carried out under an incubation period of 48 h at 22°C. Moreover, 50% inhibitory concentration was observed for each extract against L. major, and the bark showed good potential (IC50 4.95 ± 0.36 mg/ml), followed by the roots (IC50 7.07 ± 0.18 mg/ml) and the leaves (IC50 8.25 ± 0.29 mg/ml), as derived by comparing the values of each extract to the standards. The order of% inhibition was bark > roots > leaves. The results revealed that, in all the concentrations, the standard drug glucantime reduced the viability compared with each extract (P < 0.05) and control (P < 0.05). The highest activity was observed in the concentration of 1 mg/ml, and the viability increased with a decrease in concentration. The obtained results are in agreement with recently documented data of other species of Berberis (Najim and Hassan, 2020). Previously, Baloch et al. (2013) reported the strong anti-leishmanial potential of B. baluchistanica roots.
Cancer is a disease that is explained by cells in the human body that are increasing but with failure to be controlled. About 60% of medications allowed for cancer treatment are of a natural source. The anticancer activity was not reported and published by other researchers to date, but different species of genus Berberis are reported to have in vitro cytotoxic activity against different destructive cell lines (El Fakir et al., 2021; Ilyas et al., 2021). An evaluation of the anticancer activity of the ethanolic extracts of the bark, leaves, and roots of the B. baluchistanica plant was done by using MTT cell assay against HeLa cell line. The assay was performed at 100 μg/ml for each extract, and doxorubicin was used as the standard drug with which to compare the inhibition of each extract. Comparing the values to the standard (IC50 = 09 ± 0.12 μg/ml), it was revealed that the ethanolic bark extract showed the highest anticancer activity with the lowest IC50 (12 ± 0.15 μg/ml), followed by the roots (14 ± 0.15 μg/ml) and the leaves (17 ± 0.21 μg/ml), respectively. Each extract exhibited a higher anticancer (HeLa cell lines) activity, and the% inhibitions are shown in Table 4 and Figure 5.
Assessment of in vitro Anti-inflammatory Activity
Inflammation is the response of living tissues as a result of any damage or injury. It is exemplified by pain, fever, redness, and loss of body physiological functions. Different steroidal anti-inflammatory drugs and non-steroidal anti-inflammatory drugs are being used to treat the effects of prolonged and severe inflammation, but the long-term use of these drugs may also cause adverse effects. Therefore, safe and long-term-use anti-inflammatory agents need to be discovered, and plant-derived medicines are perceived to be efficient, of low cost, and with less adverse effects. The different parts of the medicinal plant B. baluchistanica were evaluated for anti-inflammatory activity by three methods, including protein denaturation, proteinase inhibition, and heat-induced hemolysis.
Denaturation of tissue proteins is one of the main causes of inflammatory diseases. The production of auto-antigens in different inflammatory diseases may be due to protein denaturation. Chemical agents that have the potential to prevent protein denaturation would be valuable for the development of anti-inflammatory drugs (Chandra et al., 2012). Ethanolic extracts of the bark, leaves, and roots were able to inhibit protein denaturation, and the inhibitions were in a concentration-dependent manner. The% inhibition of each extract at different concentrations (1–0.125 mg/ml) on protein denaturation is shown in Figure 6A. The bark extract of B. baluchistanica revealed a significantly higher (p < 0.05) level of inhibition compared with the leaves and the roots. The IC50 was observed for each extract, and the bark showed good potential (IC50 = 0.64 ± 0.25 mg/ml), followed by the roots (IC50 = 0.67 ± 0.21 mg/ml) and the leaves (IC50 = 0.73 ± 0.13 mg/ml). By comparing the values of each extract to the standard, the order of% inhibition was bark > roots > leaves. The results revealed that, in all the concentrations, the standard drug aspirin showed a relatively higher inhibition compared with each extract.
The bark, leaf, and root extracts of the B. baluchistanica plant were tested for its anti-inflammatory potential by proteinase inhibitory activity. Each extract exhibited high proteinase inhibition, and the results are shown in Figure 6B. The% inhibitions were within the range of 38–70%. The bark extract has shown a significantly higher (p < 0.05) proteinase inhibition compared with the leaves and the roots. The order of% inhibition of proteinase activity was bark > leaves > roots. The activity was performed at 1–0.125 mg/ml for each extract, and aspirin was used as the standard drug to compare the inhibition with each extract. Comparing the values to the standard, it was revealed that the ethanolic bark extract showed the highest proteinase inhibition with the lowest IC50 (0.55 ± 0.12 mg/ml), followed by the leaves (0.62 ± 0.23 mg/ml) and the roots (0.69 ± 0.15 mg/ml), respectively. The highest activity was observed in the concentration of 1 mg/ml, and the inhibition decreased with a decrease in concentration.
The anti-inflammatory effect of the ethanolic extracts of the bark, leaves, and roots of B. baluchistanica plant was carried out by heat-induced hemolysis. The percent inhibition of heat-induced hemolysis of red blood cells was analyzed at different concentrations of 1–0.125 mg/ml. The ethanolic extracts of each part were able to inhibit hemolysis in a concentration-dependent manner. The% inhibition of hemolysis was within the range from 49 to 86%. The bark extract showed significantly higher (p < 0.05) levels of hemolysis inhibition with the lowest IC50 value (0.48 ± 0.15 mg/ml) compared with the leaves (0.52 ± 0.35 mg/ml) and the roots (0.58 ± 0.05 mg/ml) of the plant. By comparing the% inhibition with the standard drug aspirin with IC50 (0.43 ± 0.10 mg/ml), the order of the% inhibition of extracts was aspirin > bark > leaves > roots. Previously, Siddiqui et al. (2017) evaluated the anti-inflammatory property of the fruit of B. baluchistanica on mice by using acetic acid-induced writhing method and formalin method and claimed that the fruit could be used as a good anti-inflammatory drug. The obtained results are in agreement with the recently reported anti-inflammatory effect of B. baluchistanica by Pervez et al. (2020) in acetic acid-induced writhing and formalin-induced flinching behavior tests. Phytochemicals such as phenolics, flavonoids, saponins, and tannins are able to protect the protein membranes from denaturation by binding the cat ions and other molecules. According to Abate et al. (2021), the higher the concentration of these classes of compounds, the lower the inhibition concentration IC50 needed to reduce the inflammation response. Therefore, polyphenolic compounds present in ethanolic extracts of bark leaves and roots could be the possible reason of the stabilization of the lysosomal membrane by its anti-denaturation property.
Conclusion
In the perspective of the research conducted, it can be revealed that the B. baluchistanica bark, leaf, and root extracts were found to have crucial antibacterial, antifungal, anti-inflammatory, anti-leishmanial, and anticancer potentials. From the results, it can be concluded that the B. baluchistanica bark, leaf, and root extracts could be good sources of effective drugs for various infections against humans. Each part of the plant was found to be potentially active for antimicrobial and other activities, whereas the bark was found to be relatively more potent than the other parts in some biological activities. However, more investigations based on these preliminary studies are needed to explore the bioactive molecules along with the mechanisms of action which are responsible for their effectiveness.
Data Availability Statement
The raw data supporting the conclusions of this article will be made available by the authors, without undue reservation.
Author Contributions
ZG collected the data and analyzed and drafted the manuscript. AA and SL conceptualized, designed, and supervised the study. AA reviewed the draft and finalized it. All authors reviewed the final manuscript.
Conflict of Interest
The authors declare that the research was conducted in the absence of any commercial or financial relationships that could be construed as a potential conflict of interest.
Publisher’s Note
All claims expressed in this article are solely those of the authors and do not necessarily represent those of their affiliated organizations, or those of the publisher, the editors and the reviewers. Any product that may be evaluated in this article, or claim that may be made by its manufacturer, is not guaranteed or endorsed by the publisher.
References
Abate, G., Zhang, L., Pucci, M., Morbini, G., Mac Sweeney, E., Maccarinelli, G., et al. (2021). Phytochemical Analysis and Anti-Inflammatory Activity of Different Ethanolic Phyto-Extracts of Artemisia annua L. Biomolecules 11, 975. doi: 10.3390/biom11070975
Akbar, A., Ali, I., Samiullah, N. U., Khan, S. A., Rehman, Z., and Rehman, S. U. (2019a). Functional, antioxidant, antimicrobial potential and food safety applications of curcuma longa and cuminum cyminum. Pak. J. Bot 51, 1129–1135.
Akbar, A., Sadiq, M. B., Ali, I., Muhammad, N., Rehman, Z., Khan, M. N., et al. (2019b). Synthesis and antimicrobial activity of zinc oxide nanoparticles against foodborne pathogens Salmonella typhimurium and Staphylococcus aureus. Biocatalysis and agricultural biotechnology 17, 36–42. doi: 10.1016/j.bcab.2018.11.005
Akbar, A., and Anal, A. K. (2014). Zinc oxide nanoparticles loaded active packaging, a challenge study against Salmonella typhimurium and Staphylococcus aureus in ready-to-eat poultry meat. Food Control 38, 88–95. doi: 10.1016/j.foodcont.2013.09.065
Baloch, N., Nabi, S., Yasser, M., and Kahraman, A. (2013). In-vitro antileishmanial, cytotoxic, antioxidant activities and phytochemical analysis of Berberis baluchistanica roots extracts and its fractions. J Phytopharmacol 4, 282–287.
Banik, S., Ibrahim, M., Amin, M. N., Moghal, M., Majumder, M., Alam, M., et al. (2014). Determination of biological properties of Alocasia Macrorrhizos: A medicinal plant. World Journal of Pharmaceutical Research 3, 193–210.
Chandra, S., Chatterjee, P., Dey, P., and Bhattacharya, S. (2012). Evaluation of in vitro anti-inflammatory activity of coffee against the denaturation of protein. Asian Pacific Journal of Tropical Biomedicine 2, S178–S180.
Dos Santos, L. C. W., Arakaki, D. G., De Pádua Melo, E. S., and Nascimento, V. A. (2021). Health hazard assessment due to slimming medicinal plant intake. Biological Trace Elem. Res. 200, 1442–1454. doi: 10.1007/s12011-021-02732-z
El Fakir, L., Bouothmany, K., Alotaibi, A., Bourhia, M., Ullah, R., Zahoor, S., et al. (2021). Antioxidant and Understanding the Anticancer Properties in Human Prostate and Breast Cancer Cell Lines of Chemically Characterized Methanol Extract from Berberis hispanica Boiss. & Reut. Applied Sciences 11, 3510.
Gunathilake, K., Ranaweera, K., and Rupasinghe, H. (2018). Influence of boiling, steaming and frying of selected leafy vegetables on the in vitro anti-inflammation associated biological activities. Plants 7, 22. doi: 10.3390/plants7010022
Idrees, M., Saeed-Ur-Rehman, S. K. L., Ur Rehman, M., Rehman, K. U., Achakzai, A. K. K., Zareen, B., et al. (2021). Antimicrobial activity of three medicinally important plants (Berberis balochistanica, Thymus serpyllum and Salvia bucharica) of Shudden, Mazhoo Tehsil Nana Sab area, District Pishin, Balochistan-Pakistan. Pure and Applied Biology 11, 570–576.
Ilyas, S., Tabasum, R., Iftikhar, A., Nazir, M., Hussain, A., Hussain, A., et al. (2021). Effect of Berberis vulgaris L. root extract on ifosfamide-induced in vivo toxicity and in vitro cytotoxicity. Scientific Reports 11, 1708. doi: 10.1038/s41598-020-80579-5
Iqbal, J., Abbasi, B. A., Ahmad, R., Mahmoodi, M., Munir, A., Zahra, S. A., et al. (2020). Phytogenic synthesis of nickel oxide nanoparticles (NiO) using fresh leaves extract of Rhamnus triquetra (wall.) and investigation of its multiple in vitro biological potentials. Biomedicines 8, 117. doi: 10.3390/biomedicines8050117
Irshad, A., Khan, N., Farina, Y., Baloch, N., Ali, A., Mun, L. K., et al. (2018). Synthesis, spectroscopic characterization, X-ray diffraction studies and in-vitro antibacterial activities of diorganotin (IV) derivatives with N-methyl-4-bromobenzohydroxamic acid. Inorganica Chimica Acta 469, 280–287.
Javed, T., Raja, S. A., Ur Rehman, K., Khalid, S., Khalid, N., and Riaz, S. (2021). In silico bimolecular characterization of anticancer phytochemicals from Fagonia indica. Pakistan Journal of Pharmaceutical Sciences 34, 883–889.
Kakar, S. A., Tareen, R. B., Kakar, M. A., Jabeen, H., Kakar, S., Al-Kahraman, Y., et al. (2012). Screening of antibacterial activity of four medicinal plants of Balochistan-Pakistan. Pak. J. Bot 44, 245–250.
Khan, A. A., Baloch, N. U., Nabi, S., Khan, M. J., Jamali, M., and Al-Kahraman, Y. (2012). In vitro antimicrobial and insecticidal activity of methanolic extract and its fractions of Berberis baluchistanica roots. WJPR 2, 219–226.
Najim, Z. K., and Hassan, H. F. (2020). Antileishmanial Effect of Berberine and extracts of Berberis vulgaris on the Growth of Leishmanial Species. INTERNATIONAL JOURNAL OF PHARMACEUTICAL QUALITY ASSURANCE 11, 237–241.
Pervez, S., Saeed, M., Ali, M. S., Fatima, I., Khan, H., and Ullah, I. (2019). Antimicrobial and Antioxidant Potential of Berberisinol, a New Flavone from Berberis baluchistanica. Chemistry of Natural Compounds 55, 247–251. doi: 10.1007/s10600-019-02660-4
Pervez, S., Saeed, M., Khan, H., and Ghaffar, R. (2020). Antinociceptive and Anti-inflammatory like effects of Berberis baluchistanica. Current Molecular Pharmacology 14, 746–752. doi: 10.2174/1874467213666201204153045
Rafique, M., and Salman, M. (2021). Exploration of Antibacterial Activities of Berberis royleana Fractions Extracts. bioRxiv doi: 10.1101/2021.06.14.448462
Rios, J.-L., and Recio, M. C. (2005). Medicinal plants and antimicrobial activity. Journal of ethnopharmacology 100, 80–84.
Sadiq, M. B., Tarning, J., Aye Cho, T. Z., and Anal, A. K. (2017). Antibacterial activities and possible modes of action of Acacia nilotica (L.) Del. against multidrug-resistant Escherichia coli and Salmonella. Molecules 22, 47. doi: 10.3390/molecules22010047
Sailaja, I. (2014). Antifungal activity of some wild plant extracts against fungal pathogens. International journal of integrative medical sciences 1, 41–44.
Shaheen, N., Qureshi, N. A., Iqbal, A., Ashraf, A., and Fatima, H. (2020). Evaluation of Safety, Antileishmanial, and Chemistry of Ethanolic Leaves Extracts of Seven Medicinal Plants: An In-vitro Study. Open Chemistry Journal 7, 26–36.
Siddiqui, F. A., Ahmad, M., Jahan, N., and Sajid, M. I. (2017). Phytochemical screening and assessment of analgesic, Anti-inflammatory and hematological properties of the fruit of Berberis baluchistanica. Pakistan journal of pharmaceutical sciences 30, 1007–1012.
Srivastava, S. K., Rai, V., Srivastava, M., Rawat, A., and Mehrotra, S. (2006). Estimation of heavy metals in different Berberis species and its market samples. Environmental monitoring and assessment 116, 315–320. doi: 10.1007/s10661-006-7395-x
Tareen, N. M., Saeed-Ur-Rehman, M. A., Shinwari, Z. K., and Bibi, T. (2016). Ethnomedicinal utilization of wild edible vegetables in district Harnai of Balochistan Province-Pakistan. Pak. J. Bot 48, 1159–1171.
Uddin, S., Safdar, L. B., Anwar, S., Iqbal, J., Laila, S., Abbasi, B. A., et al. (2021). Green Synthesis of Nickel Oxide Nanoparticles from Berberis balochistanica Stem for Investigating Bioactivities. Molecules 26, 1548. doi: 10.3390/molecules26061548
Keywords: ethanolic extracts, antimicrobial, anti-inflammatory, anti-leishmanial, anticancer, hemolysis inhibition
Citation: Gul Z, Akbar A and Leghari SK (2022) Elucidating Therapeutic and Biological Potential of Berberis baluchistanica Ahrendt Bark, Leaf, and Root Extracts. Front. Microbiol. 13:823673. doi: 10.3389/fmicb.2022.823673
Received: 27 November 2021; Accepted: 24 January 2022;
Published: 09 March 2022.
Edited by:
Filomena Nazzaro, National Research Council (CNR), ItalyCopyright © 2022 Gul, Akbar and Leghari. This is an open-access article distributed under the terms of the Creative Commons Attribution License (CC BY). The use, distribution or reproduction in other forums is permitted, provided the original author(s) and the copyright owner(s) are credited and that the original publication in this journal is cited, in accordance with accepted academic practice. No use, distribution or reproduction is permitted which does not comply with these terms.
*Correspondence: Ali Akbar, YWxpYWtiYXIudW9iQGdtYWlsLmNvbQ==