- 1College of Plant Sciences, Jilin University, Changchun, China
- 2College of Resource and Environment, Jilin Agricultural University, Changchun, China
The plant pathogenic fungus Sclerotinia sclerotiorum can survive on a wide range of hosts and cause significant losses on crop yields. FKH, a forkhead box (FOX)-containing protein, functions to regulate transcription and signal transduction. As a transcription factor (TF) with multiple biological functions in eukaryotic organisms, little research has been done on the role of FKH protein in pathogenic fungi. SsFkh1 encodes a protein which has been predicted to contain FOX domain in S. sclerotiorum. In this study, the deletion mutant of SsFkh1 resulted in severe defects in hyphal development, virulence, and sclerotia formation. Moreover, knockout of SsFkh1 lead to gene functional enrichment in mitogen-activated protein kinase (MAPK) signaling pathway in transcriptome analysis and SsFkh1 was found to be involved in the maintenance of the cell wall integrity (CWI) and the MAPK signaling pathway. Yeast two-hybrid and bimolecular fluorescence complementation assays showed that SsFkh1 interacts with SsMkk1. In addition, we explored the conserved MAPK signaling pathway components, including Bck1, Mkk1, Pkc1, and Smk3 in S. sclerotiorum. ΔSsmkk1, ΔSspkc1, ΔSsbck1, and ΔSssmk3knockout mutant strains together with ΔSsmkk1com, ΔSspkc1com, ΔSsbck1com, and ΔSssmk3com complementation mutant strains were obtained. The results indicated that ΔSsmkk1, ΔSspkc1, ΔSsbck1, and ΔSssmk3 displayed similar phenotypes to ΔSsfkh1 in sclerotia formation, compound appressorium development, and pathogenicity. Taken together, SsFkh1 may be the downstream substrate of SsMkk1 and involved in sclerotia formation, compound appressorium development, and pathogenicity in S. sclerotiorum.
Introduction
Sclerotinia sclerotiorum is a common phytopathogen that affects a broad range of the plant species. It is one of the most damaging pathogens causing extensive damage to soybean, sunflower, and rapeseed, resulting in large amount of losses in each year (Guyon et al., 2014). Furthermore, S. sclerotiorum produces dormant melanized and long-lived multicellular structures, sclerotia, which make it difficult to defense this fungus using fungicides in the field (Zhu et al., 2013).
Sclerotia are asexual dormant structure composed of concentrated vegetative hyphal cells, which are produced by a series of plant pathogenic fungi. These structures contribute to the survival of fungi in harsh environments, such as low temperature, microbial infection, or lack of nutritional host (Smith et al., 2015). Sclerotinia sclerotiorum produce sclerotia to survive for long terms under adverse circumstances (Bolton et al., 2010). Sclerotia could germinate infectious hyphae, infecting the stems or roots of the plant directly, or to germinate apothecia, which release ascospores to air as the primary infection source in sclerotinia diseases (Guogen et al., 2017). Sclerotia development is a crucial biological process (BP) in the life of S. sclerotiorum. Environmental changes and metabolisms affect sclerotia development (Erental et al., 2008). In addition, sclerotia development was regulated by cyclic adenosine monophosphate (cAMP)-dependent protein kinase, extracellular signal-regulated kinase (ERK)-like mitogen-activated protein kinase (MAPK), transcription factor (TF) SsC6TF1 and secreted protein Sscaf1 (Fan et al., 2017). Furthermore, the melanin in sclerotia is important for S. sclerotiorum to overcome the unfavorable environment. However, the molecular regulation mechanisms of sclerotia development in S. sclerotiorum are still unknown.
The forkhead box (FOX) TF contain a wing-like helix structure in DNA-binding region, a chromatin conformational change region, a nuclear localization region, and a transcription effector region (Takatani et al., 2004). These transcription factors show functionality in normal growth and development, cell differentiation, metabolism, and other BPs (Carlsson and Mahlapuu, 2002; Arsenault et al., 2015). FOX TFs are highly conserved in fungal and animal genomes. In Saccharomyces cerevisiae, FKH1, FKH2, FHL1, and HCM1 were reported (Postnikoff et al., 2012; Arsenault et al., 2015; Pataki et al., 2017). The absence of MoFKH1 in Magnaporthe oryzae impaired conidial germination, mycelial growth, and virulence (Park et al., 2014). In Candida albicans, CaFKH2 controls the morphogenesis of fungal hyphae and pseudohyphal toxicity (Bensen et al., 2002). In this study, the deletion of SsFkh1 affects sclerotia development and pathogenicity. However, the regulation mechanism of SsFkh1 regulating the sclerotia development and infection cushion in S. sclerotiorum is unclear.
Mitogen-activated protein kinase signaling pathways are ubiquitous in eukaryotes and are involved in cellular development, differentiation, stress, and nutrition, among other processes. In S. cerevisiae, MAPK pathways have been found to be involved in the cell wall integrity (CWI) pathway (Gu et al., 2015). The CWI pathway is important for the highly controlled and polarized restructuring of the fungal cell walls during development, morphological changes, and the environmental challenges (Fuchs and Mylonakis, 2009). CWI is activated by cell surface sensors, Wsc1, Wsc2, Wsc3, Mid2, and Mtl1. In S. cerevisiae, during cell wall stress, Wsc1 activates the small G protein Rho1 through the guanosine nucleotide exchange factor Rom2. Then Rho1 activates Pkc1 (the protein kinase C), which then phosphorylates the upstream kinase of conserved MAPK cascade Bck1. Bck1, in turn, transmits the signal to the redundant pair of MAP kinase kinases Mkk1 and Mkk2, then MAPK Slt2/Mpk1 was phosphorylated and activated. Finally, Slt2/Mpk1 phosphorylates Rlm1 transcription factor and SBF complex to regulate gene expression (Levin, 2005, 2011; Jendretzki et al., 2011; Sanz et al., 2012, 2016). The CWI pathway is largely conserved in other fungi. The homologs of Bck1 and Slt2/Mpk1, MoMck1, and MoMps1 in M. oryzae, respectively, were crucial for pathogenicity and CWI (Jeon et al., 2008). Surprisingly, the functions of MAPK cascade in CWI of S. sclerotiorum are not known.
In this study, SsFkh1 regulates sclerotium and compound appressorium (infection cushion) development, and responses to cell wall sensitivity. This study revealed the involvement of SsFkh1 in maintenance of cell wall integrity mediated by MAPK kinases. We investigated the function of conserved MAPK signaling pathway components, including Bck1, Mkk1, Pkc1, and Smk3, regulate sclerotia formation, the development of the compound appressorium, and the CWI pathway in S. sclerotiorum. We also specifically elucidated the relationships between SsFkh1 and SsMkk1. SsFkh1 may be involved in cell wall integrity pathway mediated by MAPK kinases, which regulated sclerotia formation and pathogenicity in S. sclerotiorum.
Materials and Methods
Fungal Strains, Plant Materials, and Culture Conditions
Sclerotinia sclerotiorum 1980 (UF-70) wild-type strain was used for gene deletion and complementation. All strains were grown on potato dextrose agar (PDA) at 25°C. Plant materials, including cowpea, tomatoes, and Arabidopsis were cultivated at 22°C with 16 h light period (Liu et al., 2018).
Construction of Mutants and PCR Verification of Gene Deletion
All PCR and quantitative reverse transcription PCR (qRT-PCR) primer sequences used in this study are shown in Supplementary Table S1.
SsFkh1 (SS1G_07360) gene were deleted with homologous recombination. The 5′ region (~1.5 kb) and the 3′ region (~1.5 kb) of the SsFkh1 gene were amplified from the genomic DNA (gDNA) of WT S. sclerotiorum and were cloned into the pXEH vector to generate a pXEH-L-R construct containing a hygromycin phosphotransferase (hph) cassette driven by a trpC promoter (Wang et al., 2016). The successfully constructed plasmids used for PEG-mediated protoplasts transformation to improve the efficiency of homologous recombination. The hyphal tips growing on the selection medium containing 600 μg/ml hygromycin B were transferred onto PDA plates (including 100 μg/ml hygromycin B) and subsequently regenerated five more times on PDA containing 100 μg/ml hygromycin B with 5 days intervals. Hygromycin-resistant transformants obtained by protoplast transformation were verified by PCR (Rollins, 2003).
SsMkk1 (SS1G_00059), SsBck1 (SS1G_10983), SsSmk3 (SS1G_05445), and SsPkc1 (SS1G_14026) genes were deleted with CRISPR-Cas9 system. The CRISPR-Cas9-TrpC-Hyg vector was used for single guide RNA (sgRNA) construction as previously described (Hisano et al., 2015). The SsMkk1, SsBck1, SsSmk3, and SsPkc1 genes were selected as the initial mutagenesis targets, respectively. The sgRNA primers for target sites within the SsMkk1, SsBck1, SsSmk3, and SsPkc1 locus were designed using the online E-Crispr tool, respectively. CRISPR-Cas9-mediated insertion site sequences were identified by thermal asymmetrical interlaced PCR (TAIL-PCR). Each sgRNA primers (M-F/MR, B-F/BR, S-F/SR, and P-F/PR) used in TAIL-PCR was 100 μM, T4 PNK used for phosphorylating and annealing the sgRNA oligos (Phosphorylate and anneal the oligos using the following parameters: 37°C for 30 min; 95°C for 5 min; ramp down to 25°C at 5°C min−1). Dilute phosphorylated and annealed oligos 1:50 by adding 1 μl of product from the initial PCR to 49 μl of room temperature ddH2O. Cloning the sgRNA oligos into CRISPR-Cas9-TrpC-Hyg vector and transforming it into Escherichia coli DH5α (Liu and Chen, 2007). The final TAIL-PCR products were purified from an 0.8% agarose gel using the TIANgel Midi Purification Kit (TIANGEN, China, catalog no. DP209-02) and then send out for sequencing. For 3′ sequence insertion characterization, the specific primer was used for each reaction. The successfully constructed plasmids used for PEG-mediated protoplasts transformation. The screening of transformants and verification was performed as mentioned above.
Construction of Complementation Strains and PCR Verification
For genetic complementation of SsFkh1 deletion mutants, a genomic region containing the full-length fragment of SsFkh1, including upstream and downstream of the coding sequence, was cloned from the WT S. sclerotiorum gDNA. This fragment was then cloned into pD-NEO1 vector carrying the promoter trpC (Wang et al., 2016). The complementation transformants were screened on PDA medium with 100 μg/ml G418 and then verified by PCR. The vector pYf-11 was used to genetic complementation of SsMkk1, SsBck1, SsSmk3, and SsPkc1 deletion mutants. The transformants on G418 selected medium were screened by protoplast transformation and subsequently regenerated five more times on PDA containing 100 μg/ml G418 with 5 days intervals. The transformants were identified by PCR.
Phenotypic Analysis
For colony morphology, 5 mm diameter mycelial plugs cut from the edge of colonies grown for 2 days of each strain were placed on PDA medium and incubated at 25°C. After 3 days, colony diameters of each strain were measured and recorded. Each experiment included three replicates and was repeated three times. After 14 days, the number and dry weight of sclerotia were determined. GraphPad prism was used for t-tests and multiple t-tests to analyze significant differences. All analyses in this study used the same statistical methods. For quantification of compound appressoria assays, 5 mm diameter fresh mycelial plugs were inoculated on glass slides and then incubated under suitable humidity conditions at 25°C for 3 days. For morphological observation of compound appressoria, 5 mm diameter fresh mycelial plugs were placed on the glass slide and were placed in a box at 25°Cfor 24 h. The optical microscope was used to observed compound appressoria. For oxalic acid (OA) production assay, the strains were grown on PDA medium supplemented with 100 μg/ml bromophenol and pH adjusted to 7.0 using NaOH, incubated at 25°C in the dark. Color changes were recorded at 2 dpi.
Response to Stress
To investigate sensitivities to different stresses, mycelial plugs of each strain were inoculated onto PDA added with different agents, which included H2O2 (15 mM), Calcium fluoride white (CFW, 0.5 mg/ml), and Congo red (CR, 150 mg/ml). Response to stress was observed, and colony diameters were determined on day 3. Three replicates were conducted for each treatment.
RNA Extraction and Quantitative Real-Time PCR Analysis
Total RNA was extracted from hypha harvested from the strain grown on PDA plates for 2 days. Each hypha sample (0.1 g) was ground into a fine powder in liquid nitrogen, using a TransZol Up Plus RNA kit (TransGen Biotech Co., Ltd., Beijing, China). Then, the resulting product was reverse-transcribed into cDNAs (TransScript All-in-One First-Strand cDNA Synthesis SuperMix for qPCR, TransGen Biotech Co., Ltd., Beijing, China). Quantitative Real-Time PCR was used to analyze the expression levels of SsFkh1, SsMkk1, SsBck1, SsSmk3, and SsPkc1 with a PrimePro 48 real-time detection system (TECHNE, United Kingodm). The actin gene was used as an endogenous reference, and transcript levels were calculated by the 2–△△Ct method (Zhang et al., 2021). Each experiment was repeated three times.
Pathogenicity Assay
For pathogenicity assays, 5 mm diameter mycelial plugs cut from the edge of colonies grown for 2 days of WT, ΔSsfkh1, and ΔSsfkh1com strains were inoculated on cowpea leaves. The mycelial plugs cut from the edge of colonies grown for 2 days of WT, ΔSsmkk1, ΔSspkc1, ΔSsbck1, and ΔSssmk3 strains were inoculated on wounded and unwounded tomato leaves. The inoculated leaves were placed in chamber and cultivated at 25°C for 3 days.
Transcriptomic Analysis
RNA-Secq using DESeq2 (version 1.12.4), Q-value transcripts <0.05 and |log2 (fold change)| > 1 was used. The DEGs were detected and the biological information analysis of DEGs was carried out as previously reported (Hou et al., 2020). The enrichment analysis of genes expressed differentially (GO) was conducted using top GOs that assigned every DEG to a single GO keyword. In the DEGs, the GO keywords in their corrected Q-value of 0,05 were regarded as highly enriched. A global categorization method for gene function contained a database of three different ontological biological processes, cellular components (CC), and molecular functions (MF) that were employed in the analysis1 and KEGG.2
Yeast Two-Hybrid Assay
Yeast two-hybrid (Y2H) was used to determine the possible protein–protein interactions between SsFkh1 and SsMkk1. Bait plasmid was constructed by cloning the amplified cDNA fragment of SsFkh1 with primer pairs 1F/1R into the vector pGBKT7. The full-length cDNA of SsMkk1 was amplified with primer pairs 2F/2R. The resulting fragments were cloned into the vector pGADT7 to generate prey plasmids. Both bait and prey plasmids were determined by sequencing and then co-transformed into yeast strain (AH109) using a lithium acetate transformation protocol. The transformants growing on SD-Trp/Leu solid medium were isolated and determined on SD-Trp/Leu/His and SD-Trp/Leu/His/Ade solid medium. The addition of X-α-gal was used to assess the activity of α-galactosidase by the production of blue precipitate. The interaction between pGADT7-T and pGBKT7-53 were used as positive control, and between pGBKT7-Lam and pGADT7-T were used negative control, respectively (Zhu et al., 2019).
Bimolecular Fluorescence Complementation Assay
Bimolecular fluorescence complementation (BiFC) assay was performed in an Arabidopsis protoplast system to confirm protein interaction (Fan et al., 2008). SsFkh1 and SsMkk1 were fused with separate regions of yellow fluorescent protein (YFP; 2005) to generate YFP-N-SsFkh1 and YFP-C-SsMkk1 for Arabidopsis protoplast transfection (Yoo et al., 2007). The fluorescent signal and localization of fusion proteins were detected using an inverted fluorescence microscope (Eclipse Ts2R; Nikon, NY, United States; emission, 514 nm). The nucleus was visualized by DAPI (4′,6-diamidino-2-phenylindole).
Results
Deletion of SsFkh1 Affects Sclerotia Development and Pathogenicity
To investigate the underlying mechanism involving in sclerotia development, which is mediated by SsFkh1, we generated the SsFkh1 deletion mutant ΔSsfkh1 and its complement strain ΔSsfkh1com by homologous recombination. Indeed, knockout of SsFkh1 led to impaired sclerotia development, as revealed by significantly reduced sclerotial numbers and dry weight, and reduced pathogenicity on its host cowpea plant. After 14 days, compared with WT, ΔSsfkh1 mutants produced less sclerotia, and only produced 60% dry weight of sclerotia as WT (Figures 1A–C). Moreover, in previous studies, some genes were reported involved in sclerotium development, including Sssmk1 (Doehlemann et al., 2010), Sspac1 (Rollins, 2003), Ssrgb1 (Erental et al., 2007), Sssac1 (Ii and Rollins, 2007), Sspka1, and Sspka2 (Ii et al., 2004). Considering the defect of sclerotium development in ΔSsfkh1 mutant. We further profiled the expression of the various sclerotium development-associated genes mentioned above. The qRT-PCR results showed that the sclerotium-associated genes were significantly decreased expressed in ΔSsfkh1 mutant (Figure 1D). Larger lesions were caused in the WT and ΔSsfkh1com strains, but the ΔSsfkh1 mutant showed reduced virulence on cowpea and tomato leaves (Figures 1E,F). In addition, compared with WT, the number of compound appressorium was decreased, and not well developed (Figure 1G). These results indicated that the SsFkh1 may regulate the sclerotium development through sclerotium-associated genes, and affect compound appressorium development.
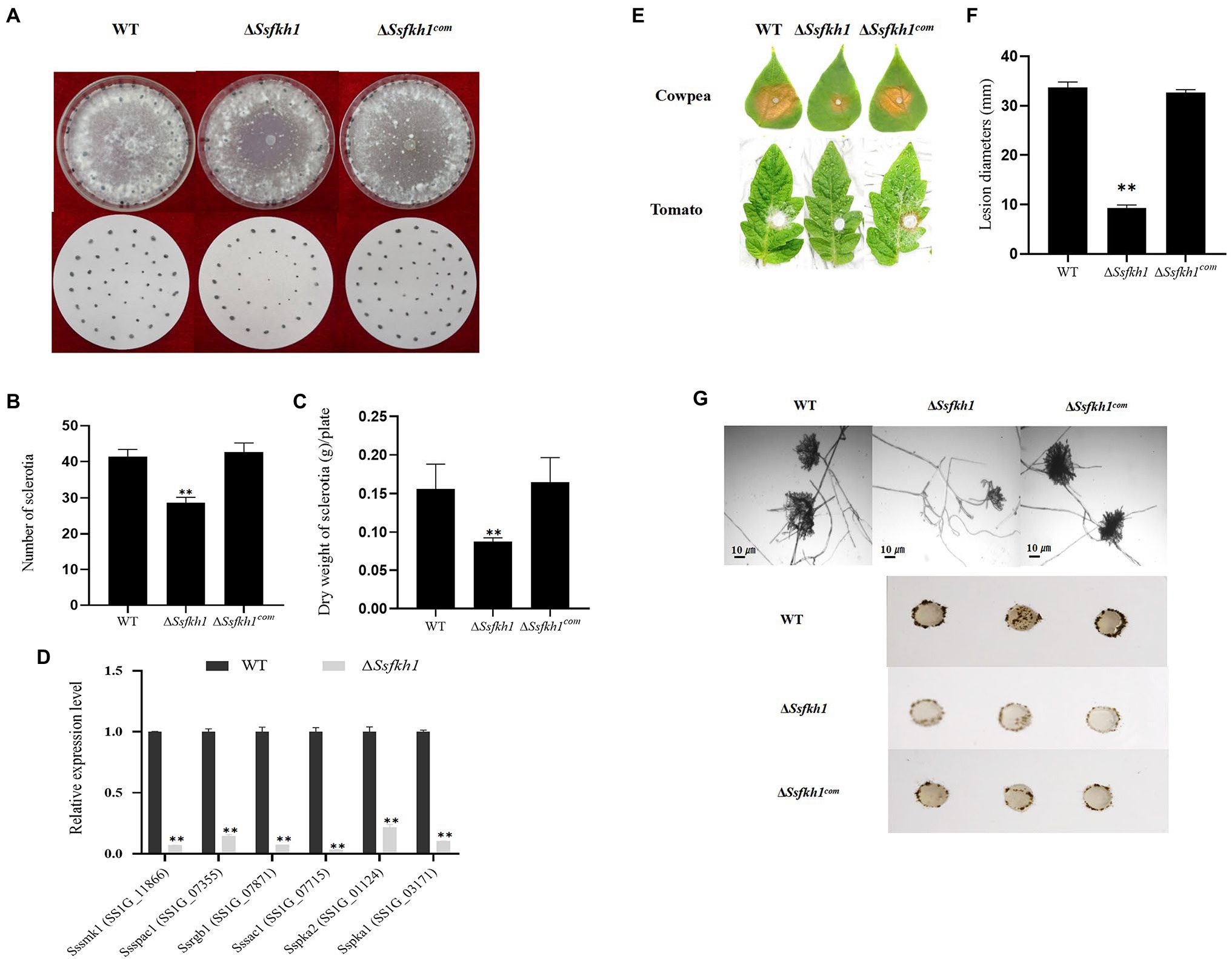
Figure 1. (A) Morphologic observation of the WT, ΔSsfkh1, and ΔSsfkh1com strains on potato dextrose agar (PDA) medium. Photographs were taken by 14 DAI. (B) Comparison of sclerotium numbers among WT, ΔSsfkh1, and ΔSsfkh1com. Sclerotia were collected from 9-cm-diameter SPA plates for statistics analysis. Number of sclerotia in WT, ΔSsfkh1, and ΔSsfkh1com strains. (C) Comparison of sclerotium dry weights among WT, ΔSsfkh1, and ΔSsfkh1com. Sclerotia were collected from 9-cm-diameter SPA plates for statistics analysis. Number of sclerotia in WT, ΔSsfkh1, and ΔSsfkh1com strains. (D) Expression profiles of sclerotium-associated genes in SsFkh1 deletion mutants. Relative expression levels of sclerotium development-associated genes were investigated in hyphal tissue in ΔSsfkh1 mutant and WT strains. The experiments were performed in triplicate [**p < 0.01 (Student’s t-test)]. Values are means ± SE. (E) Pathogenicity phenotype of WT, ΔSsfkh1, and ΔSsfkh1com strains on cowpea and tomato leaves. Pictures were taken by 2 DAI when the lesions were observed under the agar plug. (F) Lesion diameters were measured on tomato leaves daily until 3 DAI. Values on the bars followed by the same letter are not significantly different at p = 0.05. The experiment was repeated three times. (G) Compound appressoria of WT, ΔSsfkh1, and ΔSsfkh1com strains on glass sides were observed by light microscopy at 1 DAI and compound appressorium development of WT, ΔSsfkh1, and ΔSsfkh1com strains on glass sides at 3 DAI using 5 mm diameter mycelial plugs.
Effect of Loss of SsFkh1 on Adapting to Stress in Sclerotinia sclerotiorum
To evaluate the roles of SsFkh1 in adapting to stress, we compared the colony morphology of ΔSsfkh1 mutant, WT, and complemented strains on PDA with H2O2, Calcofluor white (CFW), and Congo red (CR). Compared with WT and complemented strains, ΔSsfkh1 mutant showed an obvious reduction in colony diameter under all stress conditions (Figures 2A,B). These results suggested that the SsFkh1 played an important role in cell wall integrity in S. sclerotiorum.
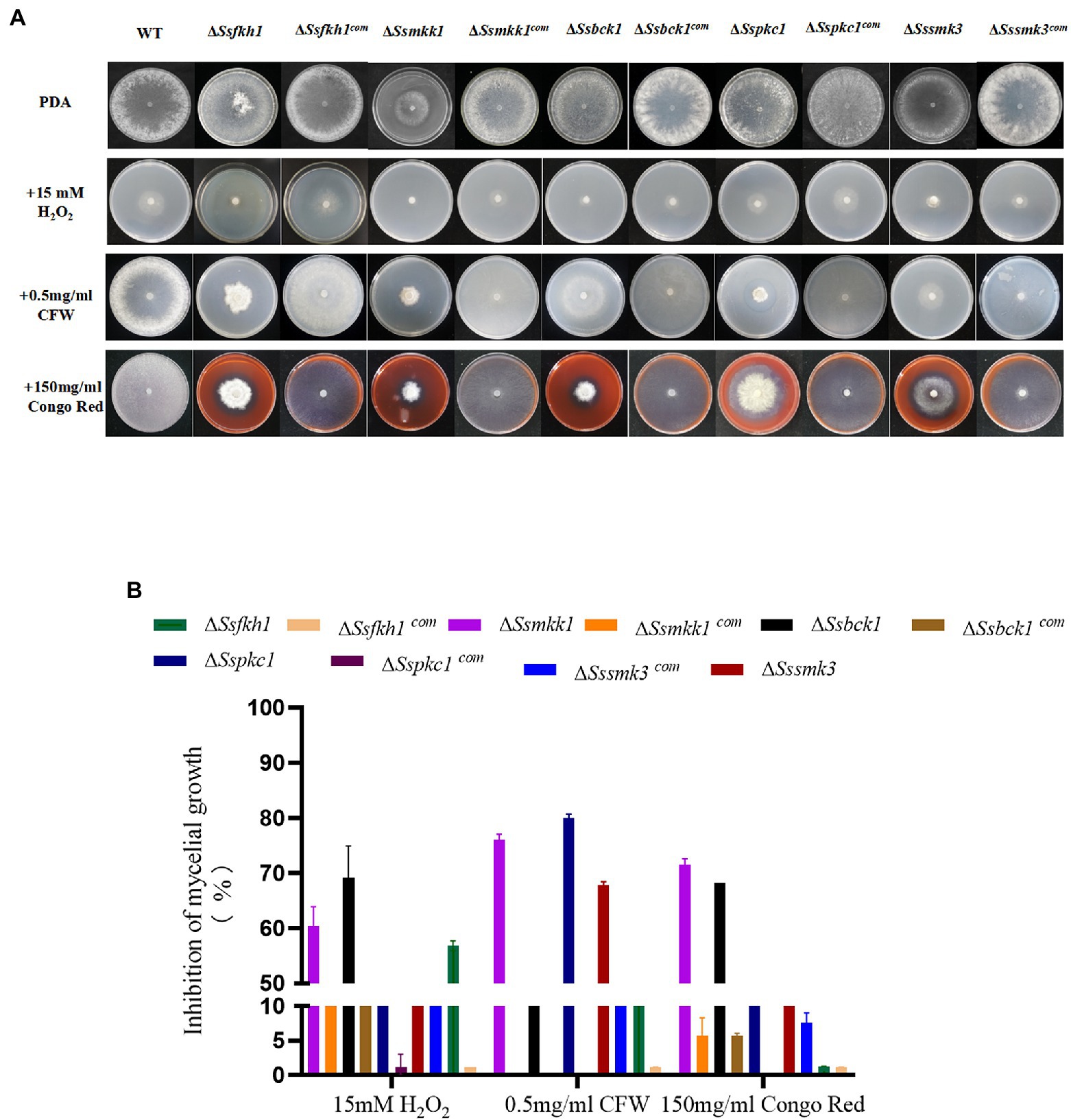
Figure 2. (A) Sensitivity of WT, ΔSsfkh1, ΔSsmkk1, ΔSspkc1, ΔSsbck1, ΔSssmk3, ΔSsfkh1com, ΔSsmkk1com, ΔSspkc1com, ΔSsbck1com, and ΔSssmk3com strains to 15 mM H2O2, 0.5 mg/ml Calcium fluoride white (CFW), 150 mg/ml Congo Red (CR) after incubation at 25°C for 3 days. Photographs were taken by 3 DAI. (B) Inhibition of mycelial growth of ΔSsfkh1, ΔSsmkk1, ΔSspkc1, ΔSsbck1, ΔSssmk3, ΔSsfkh1com, ΔSsmkk1com, ΔSspkc1com, ΔSsbck1com, and ΔSssmk3com strains. The experiment was repeated three times.
Deletion of SsFkh1 Alters Gene Expression
In order to verify the reliability of transcriptome data, some genes were randomly selected from transcriptome data for verification. Several genes obtained from RNA-seq were selected for qRT-PCR analysis including SS1G_12143, SS1G_06394, SS1G_13636, SS1G_04353, SS1G_00601, SS1G_09402, SS1G_05959, SS1G_10880, SS1G_14424, and SS1G_12905. In general, the findings of qRT-PCR were consistent with those of transcriptome analysis, thereby demonstrating that RNA-Seq data was valid and accurate (Figure 3A). The RNA-Seq data were submitted to NCBI (accession # PRJNA795029).
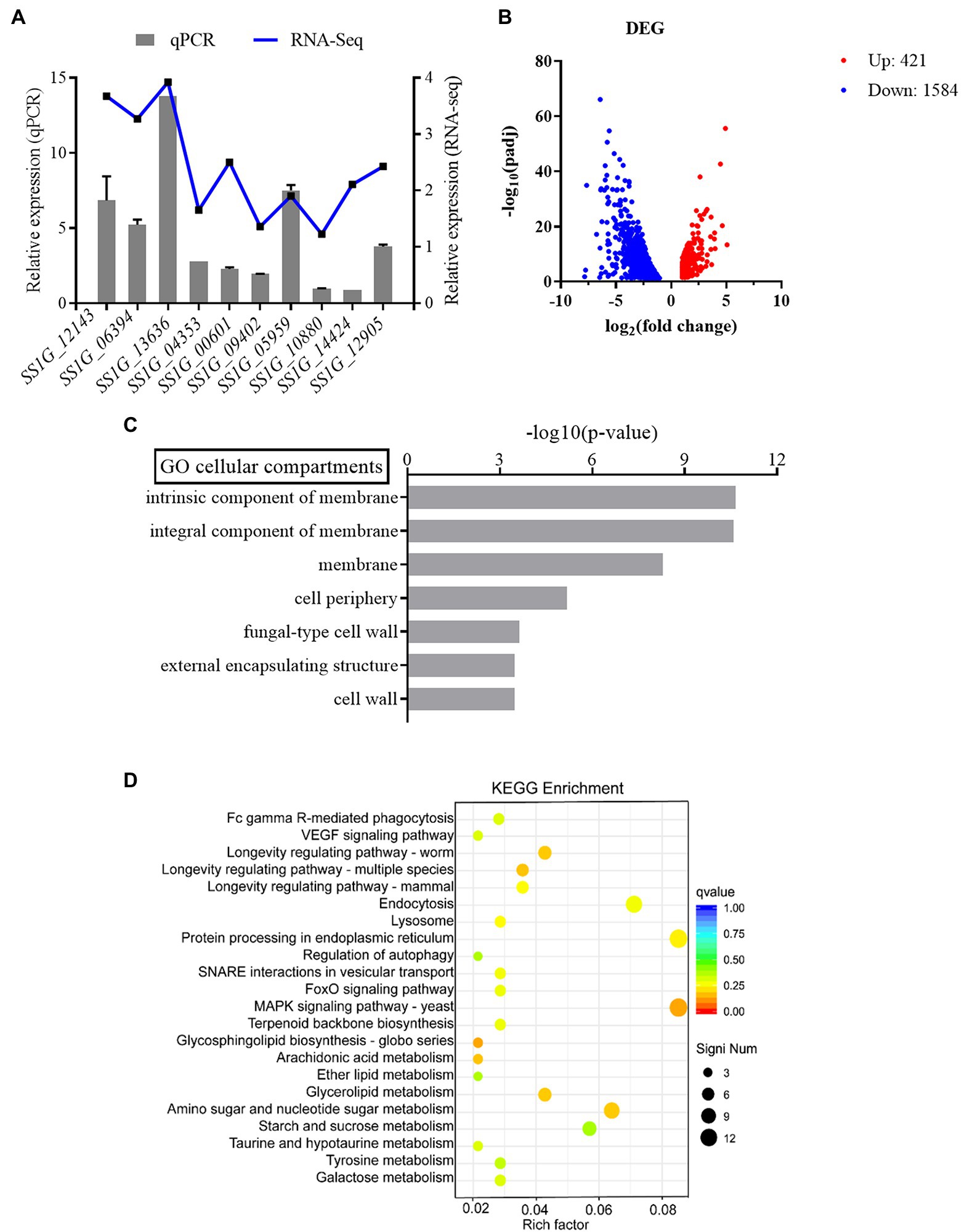
Figure 3. (A) The relative mRNA expression levels of DEGs and values of |Log2FC| (treatment group/control group) in RNA-seq. (B) Differential gene expression of WT and ΔSsfkh1. Each point in the figure represents a gene, in which red indicates an upregulation gene, blue indicates a downregulation gene. (C) Gene ontology analysis (upregulation gene). Classification of the annotated amino acid sequences. Amino acid sequences were grouped into different functional subcategories: cellular component (CC), molecular function (MF), and biological process (BF). (D) KEGG pathway enrichment analysis.
Additionally, the differences in the gene expression patterns between the treatment and control groups were analyzed using read count data from the transcriptome. In total, 2,005 differentially expressed genes (DEGs) were assigned (value of q < 0.05, |FoldChange| > 2) in ΔSsfkh1 sample (Figure 3B). To analyze the functions of the DEGs, MF, CC, and BP were determined. Some membrane-related and cell wall-related components were enriched in the cellular component, indicating that ΔSsfkh1 strains may be involved in controlling the expression of cell wall-associated genes (Figure 3C). The results of the KEGG analysis revealed that “MAPK signaling pathway” was particularly enriched (Figure 3D). These results indicated SsFkh1 may be related to cell wall and MAPK pathway.
SsFkh1 Interacts With SsMkk1
Cell wall integrity mediated by MAPK kinases is important not only for the integrity of cell wall, but also for the virulence, osmotic stress response, and development of some fungi. Based on the defects in cell wall integrity of ΔSsfkh1 mutant, we speculated SsFkh1 may interact with SsMkk1. The yeast two-hybrid assay was conducted to determine the interaction between SsFkh1 and SsMkk1. As shown in Figure 4, the SsFkh1 was interacted strongly with SsMkk1 in S. sclerotiorum (Figure 4A). In addition, a BiFC assay was used to further confirm the in vivo interaction between SsFkh1 and SsMkk1. Consistent with the result of yeast two-hybrid, BiFC also demonstrated the interaction between SsFkh1 and SsMkk1 (Figure 4B). Given the role of SsBck1 and SsSmk3 in MAPK signaling pathway, we also investigated the interaction between SsBck1 and SsSmk3 with SsFkh1; however, SsFkh1 exhibited no interaction with SsBck1 and SsSmk3 (data not shown).
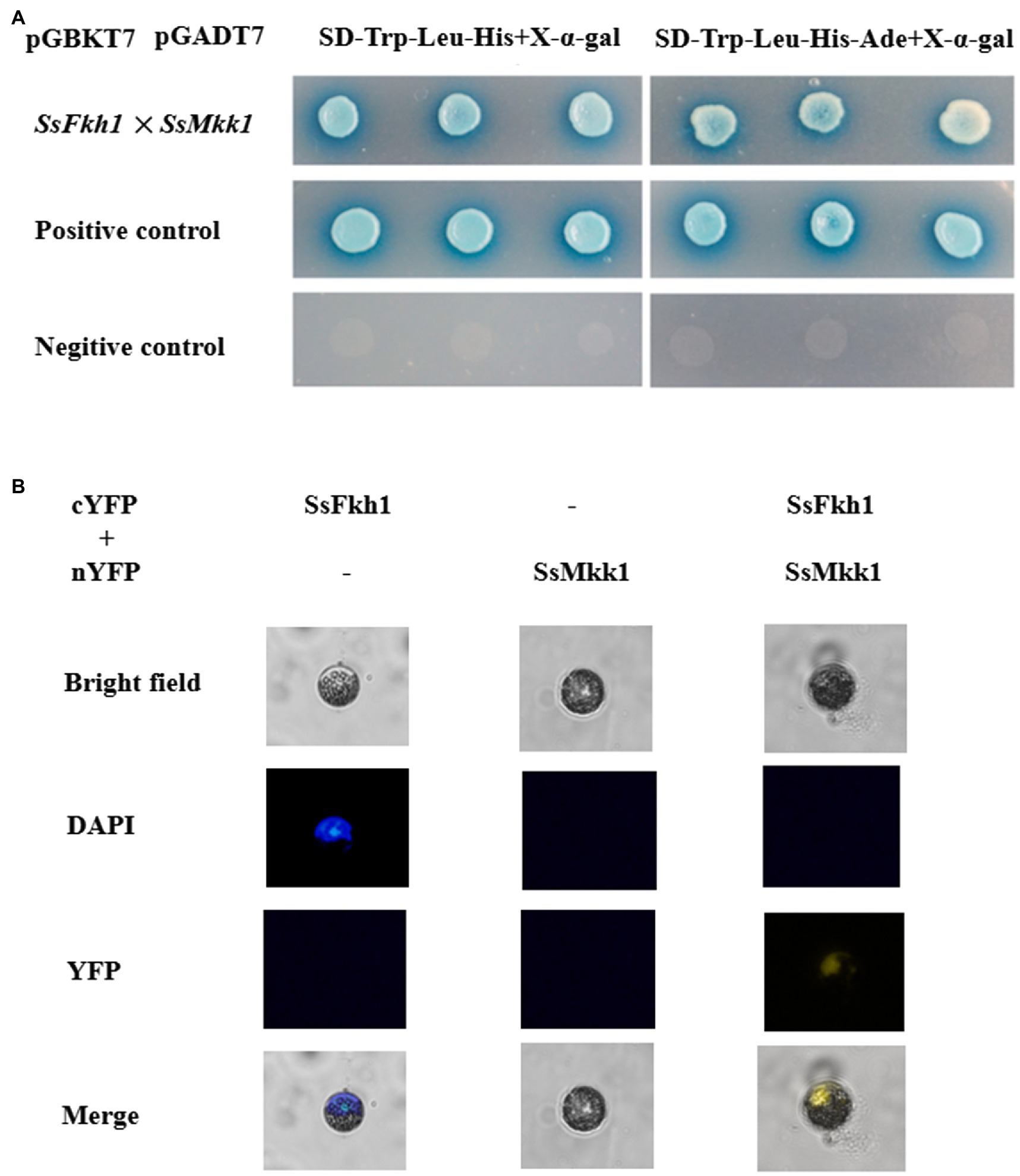
Figure 4. (A) Yeast two-hybrid (Y2H) analysis of interactions of SsFkh1 and SsMkk1. A pair of plasmids pGBKT7-53 and pGADT7 was used as a positive control. A pair of plasmids pGBKT7-Lam and pGADT7 was used as a negative control. (B) Confirmation of the interaction between SsFkh1 and SsMkk1 by bimolecular fluorescence complementation assay (BiFC) in Arabidopsis protoplast. Images were captured under a fluorescence microscope at 13 h after Arabidopsis protoplast transformation. The nucleus was visualized using DAPI (4=,6-diamidino-2-phenylindole) fluorescent dye. cYFP, C-terminal region of YFP; and nYFP, N-terminal region of YFP.
Deletion of SsMkk1, SsBck1, SsSmk3, and SsPkc1 Influence Sclerotium Development in Sclerotinia sclerotiorum
Based on the amino acid sequences of Pkc1, Bck1, Mkk1, and Smk3 from the budding yeast S. cerevisiae, SsMkk1 (SS1G_00059), SsBck1 (SS1G_10983), SsSmk3 (SS1G_05445), and SsPkc1 (SS1G_14026) were retrieved from the genome of S. sclerotiorum. SsMkk1, SsBck1, SsSmk3, and SsPkc1 are highly homologous to their counterparts from other fungal species (Supplementary Figure S1). To explore the function of SsMkk1, SsBck1, SsSmk3, and SsPkc1, the mutant strains were generated and verified by PCR (Supplementary Figure S2). In addition, to determine the function of SsMkk1, SsBck1, SsSmk3, and SsPkc1 in sclerotium development in S. sclerotiorum, the deletion mutants ΔSsmkk1, ΔSspkc1, ΔSsbck1, ΔSssmk3, and complemented strains were cultured on PDA plates. After 14 days, compared with WT, ΔSspkc1, ΔSsbck1, and ΔSssmk3 mutants produced less number of sclerotia, and only produced 58.2, 53.9, and 51.8% of dry weight of sclerotia as WT, respectively (Figures 5A,B). Even more, ΔSsmkk1 mutant completely lost the sclerotium-producing ability (Figures 5A,B). In addition, ΔSsmkk1 mutant showed a reduced growth rate on PDA and twisted hyphae morphology. Similar to ΔSsmkk1 mutant, ΔSssmk3 mutant also exhibited the twisted hyphae morphology, but no different growth rate (Figures 5A,C). These results indicated that SsMkk1 plays more essential roles in vegetative growth and sclerotia development than its upstream and downstream kinases SsBck1, SsPkc1, and SsSmk3, respectively.
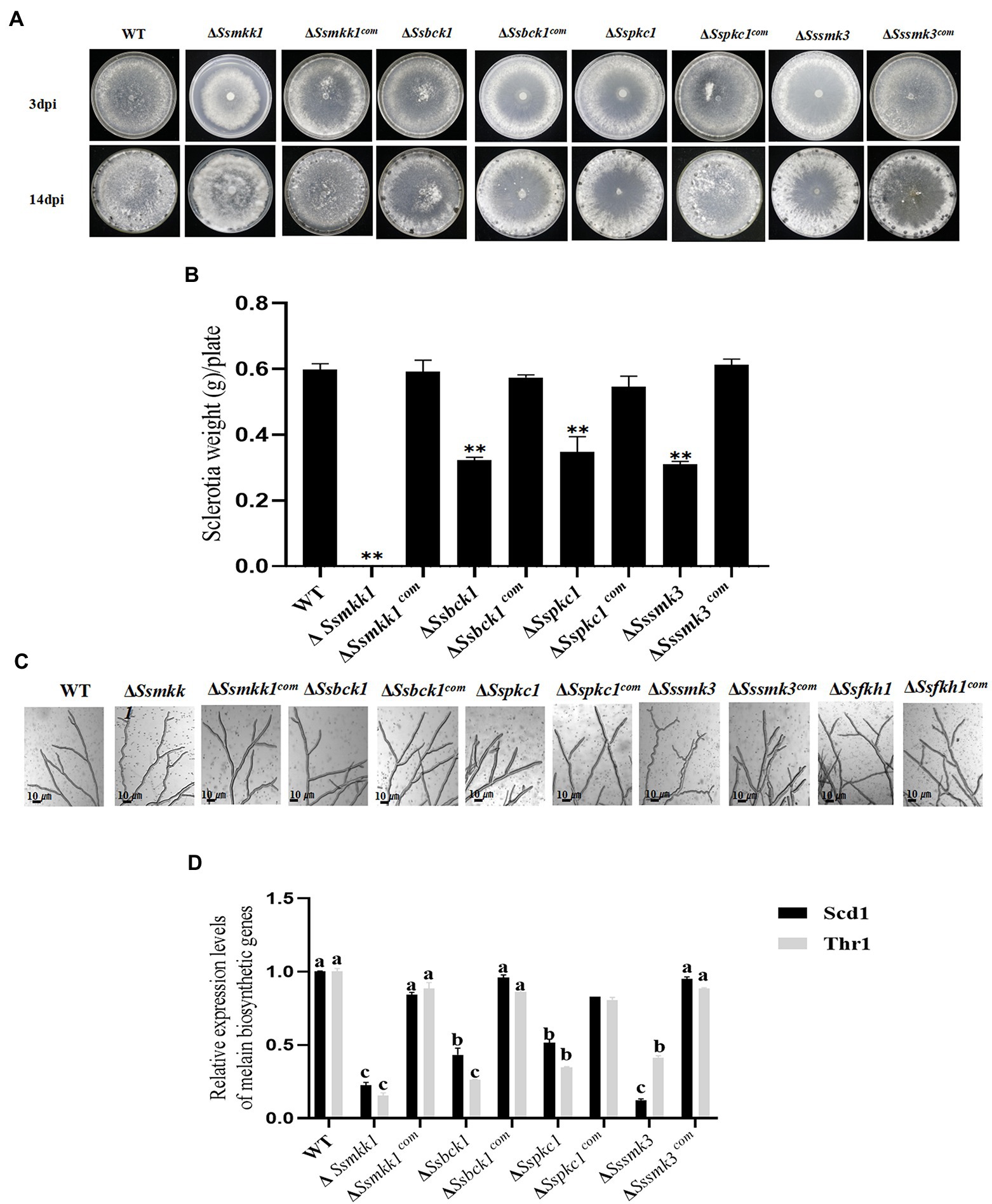
Figure 5. (A) Colony morphology of WT, ΔSsmkk1, ΔSspkc1, ΔSsbck1, ΔSssmk3, ΔSsmkk1com, ΔSspkc1com, ΔSsbck1com, and ΔSssmk3com on PDA after 3 and 14 days of incubation at 25°C. Photographs were taken by 3 DAI and 14 DAI. (B) Comparisons in sclerotia among the above strains on PDA plates after 14 days of incubation [** p < 0.01 (Student’s t-test)]. Values on the bars followed by the same letter are not significantly different at p = 0.05. (C) The observation of hyphae from WT, ΔSsmkk1, ΔSspkc1, ΔSsbck1, ΔSssmk3, ΔSsfkh1, ΔSsmkk1com, ΔSspkc1com, ΔSsbck1com, ΔSsfkh1com, and ΔSssmk3com. The photographs were taken after 16 h with a light microscope. Bar = 10 μm. (D) Comparisons of the transcript levels of two melanin biosynthesis-related genes SCD1 and THR1 among the above strains. The expression level of each gene in WT was referred to 1. Data are represented as mean values ± SE. Different letters indicate statistical significance (p < 0.05).
Colony morphology assay exhibited that ΔSsmkk1, ΔSspkc1, ΔSsbck1, and ΔSssmk3 produced less melanin in comparison with the wild type. This observation was further confirmed by determining the expression levels of two melanin biosynthesis-related genes SCD1 and THR1 in these mutants (Liang et al., 2017). Melanin accumulation is an important aspect of sclerotia formation because it increases the fungus’ resistance to harsh environmental conditions. qRT-PCR assays exhibited melanin biosynthetic gene downregulated expression in ΔSsmkk1, ΔSspkc1, ΔSsbck1, and ΔSssmk3 mutants (Figure 5D), demonstrating that MAPKs SsMkk1, SsBck1, SsSmk3, and SsPkc1 regulate melanin biosynthesis in S. sclerotiorum.
ΔSsmkk1 Exhibited Lower Virulence Than ΔSspkc1, ΔSsbck1, and ΔSssmk3
To verify the function of these four S. sclerotiorum MAPKs in pathogenicity, infection assay with wound and unwound tomato leaves was conducted. Detached tomato leaves were inoculated with mycelium plugs derived from WT, ΔSsmkk1, ΔSspkc1, ΔSsbck1, and ΔSssmk3 mutants. Typical symptoms were caused in the WT strain, but the ΔSsmkk1, ΔSspkc1, ΔSsbck1, and ΔSssmk3 mutants displayed reduced virulence on tomato leaves. Furthermore, the virulence of four mutants was rescued on wounded tomato leaves (Figures 6A,C).
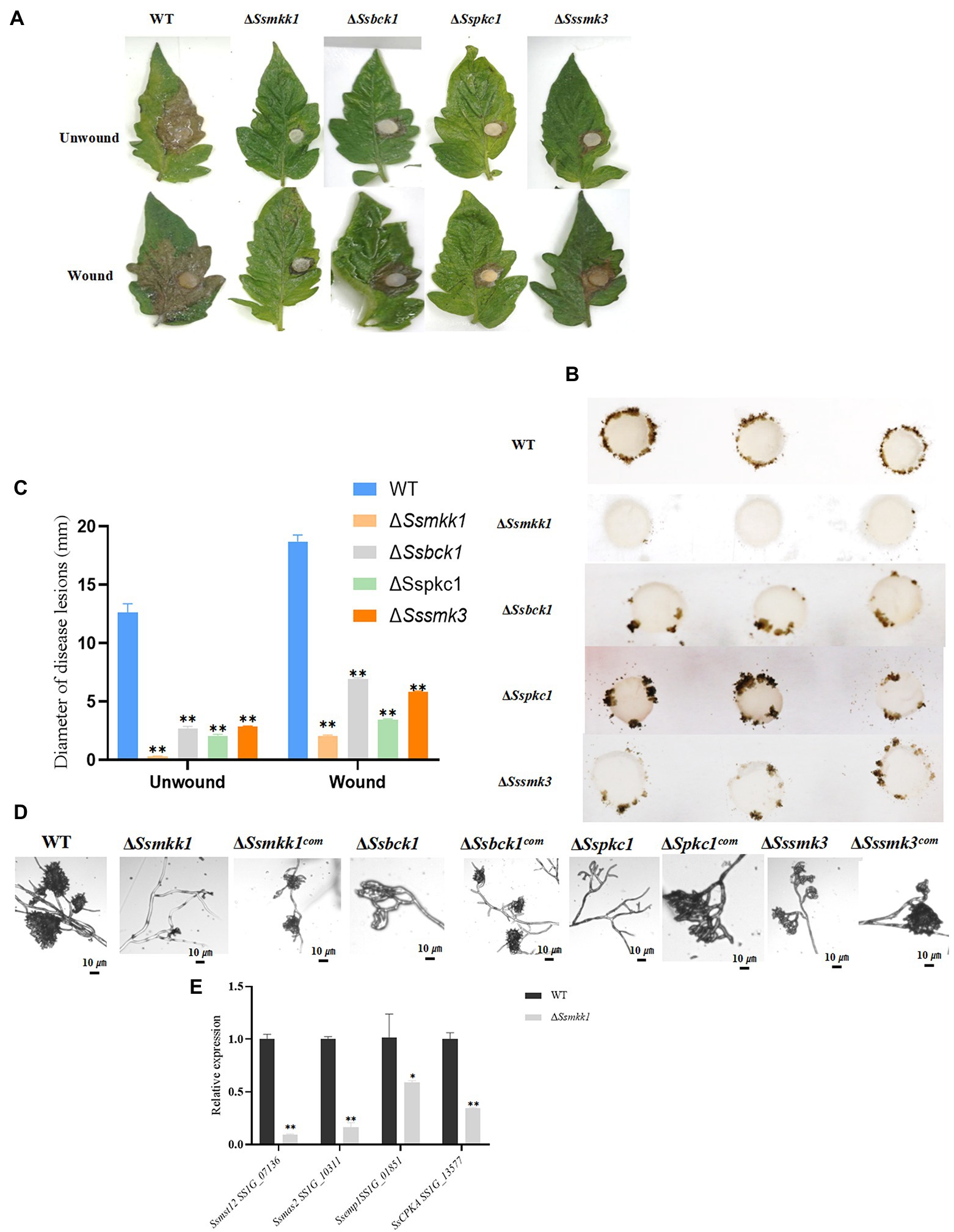
Figure 6. (A) WT, ΔSsmkk1, ΔSspkc1, ΔSsbck1, and ΔSssmk3 strains were inoculated on unwounded and wounded common tomato leaves and photographed 2 DAI after inoculation. (B) Compound appressorium development of WT, ΔSsmkk1, ΔSspkc1, ΔSsbck1, and ΔSssmk3 strains on glass sides. Compound appressoria were observed at 3 DAI using 5-mm-diameter mycelial plugs. (C) Diameters of disease lesions of WT, ΔSsmkk1, ΔSspkc1, ΔSsbck1, and ΔSssmk3 strains. Values on the bars followed by the same letter are not significantly different at p = 0.05. The experiment was repeated three times. (D) Compound appressoria of WT, ΔSsmkk1, ΔSspkc1, ΔSsbck1, ΔSssmk3, ΔSsmkk1com, ΔSspkc1com, ΔSsbck1com, and ΔSssmk3com strains were observed on parafilm after 16 h. The photographs were taken after 16 hpi with a light microscope. Bar = 10 μm. (E) Relative expression levels of compound appressorium-associated genes were investigated in hyphal tissue in WT and ΔSsmkk1 strains. The constitutively expressed Actin was used as the reference gene to standardize data [*p < 0.05; **p < 0.01 (Student’s t-test, n = 3)].
Compound appressorium formed by hyphae play an important role in S. sclerotiorum penetration into the host. The WT and mutant strains were inoculated on glass slide to induce compound appressorium. Compared with WT, the ΔSsmkk1 mutant failed to produce compound appressorium, and ΔSspkc1, ΔSsbck1, and ΔSssmk3 mutants produced less mature compound appressorium (Figures 6B,D). These results indicated that SsMkk1, SsBck1, SsSmk3, and SsPkc1 are necessary for compound appressorium development and virulence in S. sclerotiorum. Furthermore, the expression of genes involved in compound appressorium formation was analyzed in WT and ΔSsmkk1 mutant, including Ssmst12, Ssmas2, Ssemp1, and Sscpka. The expression level of all these four genes were dramatically decreased in ΔSsmkk1 mutant. These results provided a strong support to the defect in compound appressorium development of ΔSsmkk1 mutant (Figure 6E).
Disruption of SsMkk1, SsBck1, SsSmk3, and SsPkc1 Leads to Defective Cell Wall Integrity
To determine the role of SsMkk1, SsBck1, SsSmk3, and SsPkc1in the maintenance of the cell wall integrity in S. sclerotiorum, the sensitivity of ΔSsmkk1, ΔSspkc1, ΔSsbck1, and ΔSssmk3 to cell wall-damaging compounds was analyzed. Compared with WT, all the four mutants were more sensitive to CFW and CR. In addition, the ΔSsmkk1 mutant showed obvious reduction in colony diameter under H2O2 stress. ΔSsbck1 was also more sensitive to H2O2 stress (Figures 2A,B). The morphology and growth of complemented strains was restored to WT levels on all the stress media.
Discussion
We characterized the functions of SsFkh1 in S. sclerotiorum in the context of sclerotium development and pathogenicity. Moreover, CWI pathway mediated by MAPK kinases was enriched in transcriptome analysis. The conserved CWI pathway is responsible for the integrity of cell wall in many fungal species. Similar to the reports from other fungi, the MAPK mutants in the CWI pathway of S. sclerotiorum showed increased sensitivity to CR and CFW (Yun et al., 2013; Zhang et al., 2017; Valiante et al., 2020). Previous studies have shown that the three MAPKs Bck1, Mkk1, and Slt2 function clearly in the same way in S. cerevisiae, Aspergillus fumigatus, Magnaporthe oryzae, Ustilago maydis, and Ashbya gossypii. Moreover, the three MAPK mutants of Fusarium graminearum or M. oryzae showed similar morphological characters. MAPK kinase mutant (BcMkk1) exhibits more severe defects in mycelial growth, conidiation, responses to cell wall, and oxidative stresses, but has less reduced virulence than mutants of its upstream (BcBck1) and downstream (BcBmp3) kinases in Botrytis cinerea (Yin et al., 2018). In this study, when compared to the WT and complemented strains, the number of sclerotia in the ΔSspkc1, ΔSsbck1, and ΔSssmk3 mutants decreased significantly (Figure 5A). Interestingly, ΔSsmkk1 did not produce sclerotia and produced a very small number of compound appressorium. Different from BcMkk1 of B. cinerea, ΔSsmkk1 showed lower virulence than ΔSspkc1, ΔSsbck1, and ΔSssmk3 mutants (Figure 6A). Moreover, ΔSsmkk1 was more sensitive to cell wall stimulation (Figure 2). These evidences suggested that SsMkk1 may be an important kinase in MAPK pathway. In addition, transcriptome analysis showed that knockout of SsFkh1 resulted in a large enrichment of CWI pathway mediated by MAPK kinases. And yeast two-hybrid and BiFC assay confirmed the interaction between SsMkk1 and SsFkh1 (Figure 4). Therefore, we investigated the relationship between SsFkh1 and related proteins involved in MAPK pathway. However, the role of SsFkh1 which played in CWI pathway mediated by MAPK kinases remains to be further studied.
Sclerotium development include six stages and is tightly regulated by many genetic factors (Li and Rollins, 2009). Loss of the ability to produce normal sclerotia in S. sclerotiorum may disrupt the disease cycle and the ability to cause disease (Rollins, 2003; Erental et al., 2007; Li et al., 2018). In this study, we found that the ΔSsmkk1 mutant lost its ability to produce sclerotia and infection cushion and exhibited severely impaired pathogenicity compared to the WT and complemented strains (Figure 5). Similarly, after deletion of its homologous protein, MoMkk1, M. oryzae could produce less aerial hyphal, cause defective asexual development, and attenuated pathogenicity (Yang et al., 2018). Moreover, some sclerotium development-associated genes were reported. For example, the ERK like MAPK gene Smk1 was obtained from S. sclerotiorum, which showed that Smk1 was necessary for sclerotium development, and further clarified that the regulation of Smk1 on sclerotium development was through the pH-dependent signal pathway of OA accumulation (Chen et al., 2004). Previous studies have shown that sclerotium development was influenced by various environmental changes, primary metabolites, and secondary metabolites in S. sclerotiorum (Erental et al., 2008). The inability of S. sclerotiorum to form normal sclerotia might affect the disease cycle and the potential to cause illness (Zhu et al., 2019). OA plays a critical role in the infection process of S. sclerotiorum (Fagundes-Nacarath et al., 2018; Liang and Rollins, 2018). The Ssnox1 mutant has been reported to display lower oxalate production and reduced pathogenicity (Hyo-jin et al., 2011). Moreover, the knockout mutant of adenylate cyclase gene Sac1 seriously affected mycelial growth, sclerotia development, and pathogenicity in S. sclerotiorum (Rollins and Dickman, 1998). In this study, ΔSsmkk1 strains produced less OA than other knockout mutants and WT (data not shown). However, this may be related to the slow growth of ΔSsmkk1 hypha, and the amount of OA could be determined in the further experiment. In addition, melanin protects mycelia and sclerotia from some adverse environmental conditions. The genes involved in melanin biosynthesis, SCD1 encoding a scytalone dehydratase and THR1 encoding a trihydroxynaphthalene reductase, disruption of SCD1 or THR1 impaired sclerotial development in S. sclerotiorum (Liang et al., 2017). Melanin is a necessary condition for sclerotia formation. The qRT-PCR results showed that the sclerotium-associated genes were significantly decreased expressed in ΔSsmkk1, ΔSspkc1, ΔSsbck1, and ΔSssmk3 mutant strains (Figure 5D). MAPK pathway may affect sclerotia formation by regulating the expression of melanin synthesis genes. ΔSsfkh1 also showed sclerotia defects, and the downregulation of sclerotia related genes.
Compound appressoria are hyphal tip-differentiated multicellular infection structures formed by numerous plant pathogenic fungi on the host surface (Hofman and Jongebloed, 1988; Boenisch and Schäfer, 2011). Impenetrable surfaces, such as dialysis tubing, parafilm, plastics, glass, and cellophane, are frequently effective in triggering compound appressoria differentiation in S. sclerotiorum (Liu et al., 2018). Compound appressoria is essential for penetrating host cells, and the complexity of compound appressoria is related to physical resistance to penetration on the host surface (Tariq and Jeffries, 1984; Jamaux et al., 1995; Huang et al., 2016). Some genes affect the formation and development of compound appressoria. The glutathione transpeptidase gene SsGgt1 of S. sclerotiorum is necessary for the accumulation of glutathione, the development of sclerotia, and the formation of compound appressoria. There was no compound appressoria formation after knockout of the gene (Moyi and Rollins, 2010). The compound appressorium formation-related gene 1, Sscaf1, which encodes a secretory protein and involved in to infection cushions formation, plays a crucial role in host penetration (Xiao et al., 2014). In this study, ΔSsfkh1, ΔSsmkk1, ΔSspkc1, ΔSsbck1, and ΔSssmk3 mutant strains produce less compound appressorium compared with WT and complement mutants (Figures 1G, 6B). In addition, the virulence of the ΔSsfkh1, ΔSsmkk1, ΔSspkc1, ΔSsbck1, and ΔSssmk3 mutant strains were weakened (Figures 1E, 6A).
In conclusion, ΔSsmkk1, ΔSspkc1, ΔSsbck1, and ΔSssmk3 maintained the phenotype to produce a small number of sclerotia and exhibited decreased pathogenicity. These phenotypes are consistent with ΔSsfkh1. Moreover, transcriptome analysis showed that deletion of SsFkh1 resulted in a large enrichment of CWI pathway mediated by MAPK kinases. Therefore, we explored the MAPK pathway and discovered that SsFkh1 was directly interacting with SsMkk1. Furthermore, the SsBck1 and SsPkc1 phenotypes showed reduced MAPK pathway activity upstream of SsMkk1, which may indicate that there might be several MAPKKKs acting upstream of SsMkk1, although SsMkk1 appears to be the main kinase. On the one hand, SsFkh1 can control the expression of certain genes and, on the other, it may also preserve cell wall integrity. And we will get more evidence to confirm the relationship between SsFkh1 and the CWI pathway mediated by MAPK kinases in the further experiments. In a word, SsFkh1 may be participate in the CWI pathway mediated by MAPK kinases. This provides an important basis for analyzing the development mechanism of sclerotia and compound appressorium of S. sclerotiorum in the future.
Data Availability Statement
The original contributions presented in the study are included in the article/Supplementary Material, further inquiries can be directed to the corresponding author.
Author Contributions
JC and HP planned and designed the research and wrote the manuscript. XZ modified the manuscript. All authors performed the experiments and analyzed the data. All authors contributed to the article and approved the submitted version.
Funding
This work was financially supported by National Natural Science Foundation of China (31772108, 31972978), grants from Inter-governmental International Cooperation Special Project of National Key R & D Program of China (2019YFE0114200), the Thirteenth National Key R & D Program of China (2019YFD1002000), and Science and Technology Development Plan of Jilin Province (20190201025JC).
Conflict of Interest
The authors declare that the research was conducted in the absence of any commercial or financial relationships that could be construed as a potential conflict of interest.
Publisher’s Note
All claims expressed in this article are solely those of the authors and do not necessarily represent those of their affiliated organizations, or those of the publisher, the editors and the reviewers. Any product that may be evaluated in this article, or claim that may be made by its manufacturer, is not guaranteed or endorsed by the publisher.
Acknowledgments
Gang Yu analyzed the data and modified the manuscript.
Supplementary Material
The Supplementary Material for this article can be found online at: https://www.frontiersin.org/articles/10.3389/fmicb. 2022.816091/full#supplementary-material
Footnotes
References
Arsenault, H. E., Roy, J., Mapa, C. E., Cyert, M. S., and Benanti, J. A. (2015). Hcm1 integrates signals from Cdk1 and calcineurin to control cell proliferation. Mol. Biol. Cell 26, 3570–3577. doi: 10.1091/mbc.E15-07-0469
Bensen, E. S., Filler, S. G., and Berman, J. (2002). A forkhead transcription factor is important for true hyphal as well as yeast morphogenesis in Candida albicans. Eukaryot. Cell 1, 787–798. doi: 10.1128/EC.1.5.787-798.2002
Boenisch, M. J., and Schäfer, W. (2011). Fusarium graminearum forms mycotoxin producing infection structures on wheat. BMC Plant Biol. 11:110. doi: 10.1186/1471-2229-11-110
Bolton, M., Thomma, B., and Nelson, B. D. (2010). Sclerotinia sclerotiorum (Lib.) de Bary: biology and molecular traits of a cosmopolitan pathogen. Mol. Plant Pathol. 7, 1–16. doi: 10.1111/j.1364-3703.2005.00316.x
Carlsson, P., and Mahlapuu, M. (2002). Forkhead transcription factors: key players in development and metabolism. Dev. Biol. 250, 1–23. doi: 10.1006/dbio.2002.0780
Chen, C., Harel, A., Gorovoits, R., Yarden, O., and Dickman, M. B. (2004). MAPK regulation of sclerotial development in Sclerotinia sclerotiorum is linked with pH and cAMP sensing. Mol. Plant-Microbe Interact. 17, 404–413. doi: 10.1094/MPMI.2004.17.4.404
Doehlemann, G., Berndt, P., and Hahn, M. (2010). Different signalling pathways involving a Gα protein, cAMP and a MAP kinase control germination of Botrytis cinerea conidia. Mol. Microbiol. 59, 821–835. doi: 10.1111/j.1365-2958.2005.04991.x
Erental, A., Dickman, M. B., and Yarden, O. (2008). Sclerotial development in Sclerotinia sclerotiorum: awakening molecular analysis of a "dormant" structure. Fungal Biol. Rev. 22, 6–16. doi: 10.1016/j.fbr.2007.10.001
Erental, A., Harel, A., and Yarden, O. (2007). Type 2A phosphoprotein phosphatase is required for asexual development and pathogenesis of Sclerotinia sclerotiorum. Mol. Plant-Microbe Interact. 20, 944–954. doi: 10.1094/MPMI-20-8-0944
Fan, H., Yu, G., Liu, Y., Zhang, X., Liu, J., Zhang, Y., et al. (2017). An atypical forkhead-containing transcription factor SsFKH1 is involved in sclerotial formation and is essential for pathogenicity in Sclerotinia sclerotiorum. Mol. Plant Pathol. 18, 963–975. doi: 10.1111/mpp.12453
Fagundes-Nacarath, I. R. F., Debona, D., and Rodrigues, F. A. (2018). Oxalic acid-mediated biochemical and physiological changes in the common bean- Sclerotinia sclerotiorum interaction. Plant Physiol. Biochem. 129, 109–121. doi: 10.1016/j.plaphy.2018.05.028
Fan, J. Y., Cui, Z. Q., Wei, H. P., Zhang, Z. P., and Zhang, X. E. (2008). Split mCherry as a new red bimolecular fluorescence complementation system for visualizing protein-protein interactions in living cells. Biochem. Biophys. Res. Commun. 367, 47–53. doi: 10.1016/j.bbrc.2007.12.101
Fuchs, B. B., and Mylonakis, E. (2009). Our paths might cross: the role of the fungal cell wall integrity pathway in stress response and cross talk with other stress response pathways. Eukaryot. Cell 8, 1616–1625. doi: 10.1128/EC.00193-09
Gu, Q., Chen, Y., Liu, Y., Zhang, C., and Ma, Z. (2015). The transmembrane protein FgSho1 regulates fungal development and pathogenicity via the MAPK module Ste50-Ste11-Ste7 in Fusarium graminearum. New Phytol. 206, 315–328. doi: 10.1111/nph.13158
Guogen, Y., Liguang, T., Yingdi, G., Xie, J., Fu, Y., Jiang, D., et al. (2017). A cerato-platanin protein SsCP1 targets plant PR1 and contributes to virulence of Sclerotinia sclerotiorum. New Phytol. 217, 739–755. doi: 10.1111/nph.14842
Guyon, K., Balague, C., Roby, D., and Raffaele, S. (2014). Secretome analysis reveals effector candidates associated with broad host range necrotrophy in the fungal plant pathogen Sclerotinia sclerotiorum. BMC Genomics 15:336. doi: 10.1186/1471-2164-15-336
Hisano, Y., Sakuma, T., Nakade, S., Ohga, R., Ota, S., Okamoto, H., et al. (2015). Precise in-frame integration of exogenous DNA mediated by CRISPR/Cas9 system in zebrafish. Sci. Rep. 5:8841. doi: 10.1038/srep08841
Hofman, T. W., and Jongebloed, P. (1988). Infection process of Rhizoctonia solani on Solanum tuberosum and effects of granular nematicides. Neth. J. Plant Pathol. 94, 243–252. doi: 10.1007/BF01977314
Hou, J., Feng, H. Q., Chang, H. W., Liu, Y., Li, G. H., Yang, S., et al. (2020). The H3K4 demethylase Jar1 orchestrates ROS production and expression of pathogenesis-related genes to facilitate Botrytis cinerea virulence. New Phytol. 225, 930–947. doi: 10.1111/nph.16200
Huang, L., Buchenauer, H., Han, Q., Zhang, X., and Kang, Z. (2016). Ultrastructural and cytochemical studies on the infection process of Sclerotinia sclerotiorum in oilseed rape. J. Plant Dis. Protect. 115, 9–16. doi: 10.1007/BF03356233
Hyo-jin, K., Changbin, C., Mehdi, K., and Dickman, M. B. (2011). Identification and characterization of Sclerotinia sclerotiorum NADPH oxidases. Appl. Environ. Microbiol. 77, 7721–7729. doi: 10.1128/AEM.05472-11
Ii, W. M. J., Dickman, M. B., and Rollins, J. A. (2004). Characterization and functional analysis of a cAMP-dependent protein kinase A catalytic subunit gene (pka1) in Sclerotinia sclerotiorum. Physiol. Mol. Plant Pathol. 64, 155–163. doi: 10.1016/j.pmpp.2004.07.004
Ii, W. M. J., and Rollins, J. A. (2007). Deletion of the adenylate cyclase (sac1) gene affects multiple developmental pathways and pathogenicity in Sclerotinia sclerotiorum. Fungal Genet. Biol. 44, 521–530. doi: 10.1016/j.fgb.2006.11.005
Jamaux, I., Gelie, B., and Lamarque, C. (1995). Early stages of infection of rapeseed petals and leaves by Sclerotinia sclerotiorum revealed by scanning electron microscopy. Plant Pathol. 44, 22–30. doi: 10.1111/j.1365-3059.1995.tb02712.x
Jendretzki, A., Wittland, J., Wilk, S., Straede, A., and Heinisch, J. J. (2011). How do I begin? Sensing extracellular stress to maintain yeast cell wall integrity. Eur. J. Cell Biol. 90, 740–744. doi: 10.1016/j.ejcb.2011.04.006
Jeon, J., Goh, J., Yoo, S., Chi, M. H., Choi, J., Rho, H. S., et al. (2008). A putative MAP kinase kinase kinase, MCK1, is required for cell wall integrity and pathogenicity of the rice blast fungus, Magnaporthe oryzae. Mol. Plant-Microbe Interact. 21, 525–534. doi: 10.1094/MPMI-21-5-0525
Levin, D. E. (2005). Cell wall integrity signaling in Saccharomyces cerevisiae. Microbiol. Mol. Biol. Rev. 69, 262–291. doi: 10.1128/MMBR.69.2.262-291.2005
Levin, D. E. (2011). Regulation of cell wall biogenesis in Saccharomyces cerevisiae: the cell wall integrity signaling pathway. Genetics 189, 1145–1175. doi: 10.1534/genetics.111.128264
Li, J., Mu, W., Veluchamy, S., Liu, Y., Zhang, Y., Pan, H., et al. (2018). The GATA-type IVb zinc-finger transcription factor SsNsd1 regulates asexual-sexual development and appressoria formation in Sclerotinia sclerotiorum. Mol. Plant Pathol. 19, 1679–1689. doi: 10.1111/mpp.12651
Li, M., and Rollins, J. A. (2009). The development-specific protein (Ssp1) from Sclerotinia sclerotiorum is encoded by a novel gene expressed exclusively in sclerotium tissues. Mycologia 101, 34–43. doi: 10.3852/08-114
Liang, X., and Rollins, J. A. (2018). Mechanisms of broad host range necrotrophic pathogenesis in Sclerotinia sclerotiorum. Phytopathology 108, 1128–1140. doi: 10.1094/PHYTO-06-18-0197-RVW
Liang, Y., Xiong, W., Steinkellner, S., and Feng, J. (2017). Deficiency of the melanin biosynthesis genes SCD1 and THR1 affects sclerotial development and vegetative growth, but not pathogenicity, in Sclerotinia sclerotiorum. Mol. Plant Pathol. 19, 1444–1453. doi: 10.1111/mpp.12627
Liu, L., Wang, Q., Zhang, X., Liu, J., Zhang, Y., and Pan, H. (2018). Ssams2, a gene encoding GATA transcription factor, is required for appressoria formation and chromosome segregation in Sclerotinia sclerotiorum. Front. Microbiol. 9:3031. doi: 10.3389/fmicb.2018.03031
Liu, Y. G., and Chen, Y. (2007). High-efficiency thermal asymmetric interlaced PCR for amplification of unknown flanking sequences. Biotechniques 43: 649.
Moyi, L., and Rollins, J. A. (2010). The development-specific ssp1 and ssp2 genes of Sclerotinia sclerotiorum encode lectins with distinct yet compensatory regulation. Fungal Genet. Biol. 47, 531–538. doi: 10.1016/j.fgb.2010.03.008
Park, J., Kong, S., Kim, S., Kang, S., and Lee, Y. H. (2014). Roles of forkhead-box transcription factors in controlling development, pathogenicity, and stress response in Magnaporthe oryzae. Plant Pathol. J. 30, 136–150. doi: 10.5423/PPJ.OA.02.2014.0018
Pataki, E., Miklos, I., Weisman, R., and Sipiczki, M. (2017). fhl1 gene of the fission yeast regulates transcription of meiotic genes and nitrogen starvation response, downstream of the TORC1 pathway. Curr. Genet. 63, 91–101. doi: 10.1007/s00294-016-0607-1
Postnikoff, S., Malo, M. E., Wong, B., Harkness, T., and Copenhaver, G. P. (2012). The yeast forkhead transcription factors Fkh1 and Fkh2 regulate lifespan and stress response together with the anaphase-promoting complex. PLoS Genet. 8:e1002583. doi: 10.1371/journal.pgen.1002583
Rollins, J. A. (2003). The Sclerotinia sclerotiorum pac1 gene is required for sclerotial development and virulence. Mol. Plant-Microbe Interact. 16, 785–795. doi: 10.1094/MPMI.2003.16.9.785
Rollins, J. A., and Dickman, M. B. (1998). Increase in endogenous and exogenous cyclic AMP levels inhibits sclerotial development in Sclerotinia sclerotiorum. Appl. Environ. Microbiol. 64, 2539–2544. doi: 10.1128/AEM.64.7.2539-2544.1998
Sanz, A. B., García, R., Rodríguez-Pea, J. M., Nombela, C., and Arroyo, J. (2016). Cooperation between SAGA and SWI/SNF complexes is required for efficient transcriptional responses regulated by the yeast MAPK Slt2. Nucleic Acids Res. 44, 7159–7172. doi: 10.1093/nar/gkw324
Sanz, A. B., Garcia, R., Rodriguez-Pena, J. M., Diez-Muniz, S., Nombela, C., Peterson, C. L., et al. (2012). Chromatin remodeling by the SWI/SNF complex is essential for transcription mediated by the yeast cell wall integrity MAPK pathway. Mol. Biol. Cell 23, 2805–2817. doi: 10.1091/mbc.e12-04-0278
Smith, M. E., Henkel, T. W., and Rollins, J. A. (2015). How many fungi make sclerotia? Fungal Ecol. 13, 211–220. doi: 10.1016/j.funeco.2014.08.010
Takatani, T., Takahashi, K., Uozumi, Y., Matsuda, T., Ito, T., Schaffer, S. W., et al. (2004). Taurine prevents the ischemia-induced apoptosis in cultured neonatal rat cardiomyocytes through Akt/caspase-9 pathway. Biochem. Biophys. Res. Commun. 316, 484–489. doi: 10.1016/j.bbrc.2004.02.066
Tariq, V. N., and Jeffries, P. (1984). Appressorium formation by Sclerotinia sclerotiorum: scanning electron microscopy. Trans. Br. Mycol. Soc. 82, 645–651. doi: 10.1016/S0007-1536(84)80105-9
Valiante, V., Macheleidt, J., Föge, M., and Brakhage, A. A. (2020). The Aspergillus fumigatus cell wall integrity signaling pathway: drug target, compensatory pathways, and virulence. Front. Microbiol. 6:325. doi: 10.3389/fmicb.2015.00325
Wang, L., Liu, Y., Liu, J., Zhang, Y., and Pan, H. (2016). The Sclerotinia sclerotiorum FoxE2 gene is required for apothecial development. Phytopathology 106, 484–490. doi: 10.1094/PHYTO-08-15-0181-R
Xiao, X., Xie, J., Cheng, J., Li, G., and Fu, Y. (2014). Novel secretory protein Ss-Caf1 of the plant-pathogenic fungus Sclerotinia sclerotiorum is required for host penetration and normal sclerotial development. Mol. Plant-Microbe Interact. 27, 40–55. doi: 10.1094/MPMI-05-13-0145-R
Yang, J., Liu, M., Liu, X., Yin, Z., Sun, Y., Zhang, H., et al. (2018). Heat-shock proteins MoSsb1, MoSsz1, and MoZuo1 attenuate MoMkk1-mediated cell-wall integrity signaling and are important for growth and pathogenicity of Magnaporthe oryzae. Mol. Plant-Microbe Interact. 31, 1211–1221. doi: 10.1094/MPMI-02-18-0052-R
Yin, Y., Wu, S., Chaonan, C., Ma, T., Jiang, H., Matthias, H., et al. (2018). The MAPK kinase BcMkk1 suppresses oxalic acid biosynthesis via impeding phosphorylation of BcRim15 by BcSch9 in Botrytis cinerea. PLoS Pathog. 14:e1007285. doi: 10.1371/journal.ppat.1007285
Yoo, S. D., Cho, Y. H., and Sheen, J. (2007). Arabidopsis mesophyll protoplasts. Nat. Protoc. 2, 1565–1572. doi: 10.1038/nprot.2007.199
Yun, Y., Liu, Z., Zhang, J., Shim, W. B., and Ma, Z. (2013). The MAPKK FgMkk1 of Fusarium graminearum regulates vegetative differentiation, multiple stress response, and virulence via the cell wall integrity and high-osmolarity glycerol signaling pathways. Environ. Microbiol. 16, 2023–2037. doi: 10.1111/1462-2920.12334
Zhang, H., Li, Y., Lai, W., Huang, K., Li, Y., Wang, Z., et al. (2021). SsATG8 and SsNBR1 mediated-autophagy is required for fungal development, proteasomal stress response and virulence in Sclerotinia sclerotiorum. Fungal Genet. Biol. 157:103632. doi: 10.1016/j.fgb.2021.103632
Zhang, G., Sun, Z., Ren, A., Shi, L., Shi, D., Li, X., et al. (2017). The mitogen-activated protein kinase GlSlt2 regulates fungal growth, fruiting body development, cell wall integrity, oxidative stress and ganoderic acid biosynthesis in Ganoderma lucidum. Fungal Genet. Biol. 104, 6–15. doi: 10.1016/j.fgb.2017.04.004
Zhu, W., Wei, W., Fu, Y., Cheng, J., Xie, J., Li, G., et al. (2013). A secretory protein of necrotrophic fungus Sclerotinia sclerotiorum that suppresses host resistance. PLoS One 8:e53901. doi: 10.1371/journal.pone.0053901
Keywords: Sclerotinia sclerotiorum, SsFkh1, MAPK, CWI, sclerotia, pathogenicity
Citation: Cong J, Xiao K, Jiao W, Zhang C, Zhang X, Liu J, Zhang Y and Pan H (2022) The Coupling Between Cell Wall Integrity Mediated by MAPK Kinases and SsFkh1 Is Involved in Sclerotia Formation and Pathogenicity of Sclerotinia sclerotiorum. Front. Microbiol. 13:816091. doi: 10.3389/fmicb.2022.816091
Edited by:
Jesús Navas-Castillo, La Mayora Experimental Station (CSIC), SpainReviewed by:
Jiatao Xie, Huazhong Agricultural University, ChinaJiasen Cheng, Huazhong Agricultural University, China
Copyright © 2022 Cong, Xiao, Jiao, Zhang, Zhang, Liu, Zhang and Pan. This is an open-access article distributed under the terms of the Creative Commons Attribution License (CC BY). The use, distribution or reproduction in other forums is permitted, provided the original author(s) and the copyright owner(s) are credited and that the original publication in this journal is cited, in accordance with accepted academic practice. No use, distribution or reproduction is permitted which does not comply with these terms.
*Correspondence: Hongyu Pan, cGFuaG9uZ3l1QGpsdS5lZHUuY24=