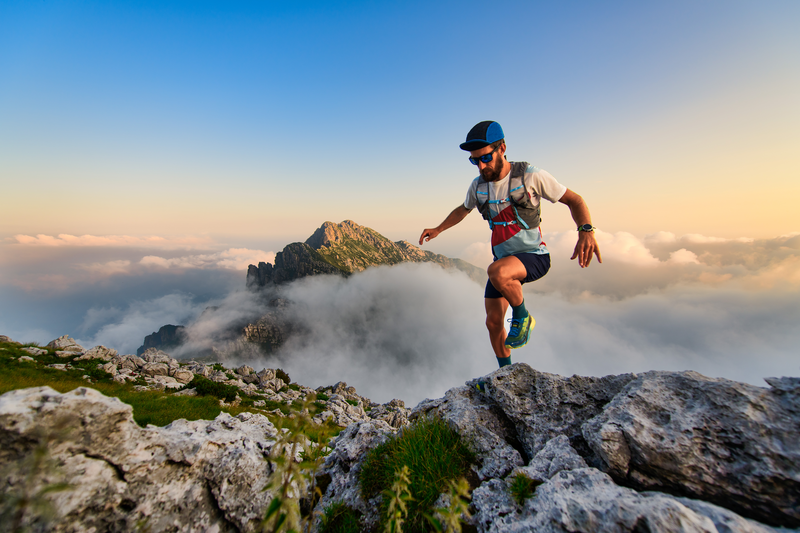
95% of researchers rate our articles as excellent or good
Learn more about the work of our research integrity team to safeguard the quality of each article we publish.
Find out more
ORIGINAL RESEARCH article
Front. Microbiol. , 04 May 2022
Sec. Food Microbiology
Volume 13 - 2022 | https://doi.org/10.3389/fmicb.2022.811784
This article is part of the Research Topic Rising Stars in Food Microbiology: 2021 View all 5 articles
Natural antimicrobials (NAM) are promising candidates for the successful control of poultry-borne bacteria, carrying potent antimicrobial activity (AMA) against a wide range of multidrug-resistant pathogens. Individual activities of carvacrol, eugenol, trans-cinnamaldehyde, oregano, and thymol, along with the combined activity of paired compounds, were examined using broth microdilution and checkerboard techniques. The characteristic interactions between the compounds were calculated using an improved method, based on combination index (CI) values. The bacteria examined herein were selected due to their known genetic resistance to at least one antibiotic. Our results indicated that thymol was most effective, exhibiting the lowest minimum inhibitory concentration (MIC) value against Salmonella pullorum, Escherichia coli, and Klebsiella pneumoniae, establishing the order of antimicrobial efficacy as: thymol > oregano > carvacrol > trans-cinnamaldehyde > eugenol. In the interaction study, the paired combination of carvacrol and thymol showed synergistic effects and was highly effective in reducing the antibiotic resistance of all the evaluated pathogens. Notably, all CI values were <1.0 in evaluations of S. pullorum, indicating the absence of antagonism between eugenol and thymol (or oregano). In K. pneumoniae, majority of CI values, which had a few concentration points, were smaller than 1.0, indicating a synergistic effect between eugenol and carvacrol (oregano or thymol), and trans-cinnamaldehyde and carvacrol. In E. coli, apart from some concentration points, some CI values were smaller than 1.0, demonstrating a synergistic effect between eugenol and carvacrol, and thymol and carvacrol (eugenol or oregano). It is therefore of great significance to investigate and illuminate the minimal effect concentration of these five components when they are used in combination as feed additives. Moreover, the improved evaluation method of this study provides a precise and extensive means to assess the synergistic effects of NAM.
The incidence of antimicrobial resistance (AMR) is increasing. Based on the World Health Organization, approximately 700,000 mortalities are attributed to drug-resistant infections each year. Without necessary action against these infections, the death rate can potentially rise to 10 million deaths annually by 2050, with AMR forcing approximately 24 million people into abject poverty by 2030 (Courtenay et al., 2019). The poultry industry is highly dependent on antibiotics in feed to manage pathogens (Van Boeckel et al., 2015); however, the increase in acquired bacterial antibiotic resistance is a concern to both the poultry industry and consumers. Public concern over the use of dietary antibiotics in consumable meat production, especially in relation to antibiotic resistance formation in bacteria, has elevated the demand for developing alternatives to antibiotics for use in farm animals. Therefore, there is a great interest in the development of natural antimicrobials (NAM), which can successfully manage multidrug-resistant pathogens and provide a worthwhile alternative to antibiotics (Wright, 2019; Yang et al., 2019; Drame et al., 2020; Naeim et al., 2020). Furthermore, this trend has emerged due to the European Union and China banning the use of antibiotics as growth promoters in livestock or other agricultural domains. The so-called antimicrobial growth promoters (AGPs) and non-AGPs, which are non-antibiotic feed supplements that focus on increasing animal development and improving their health status, could be effectively used to control salmonellosis and other diseases caused by foodborne pathogens in animal sectors (Shan et al., 2007; Aljumaah et al., 2020).
Currently, NAM have excellent potential in successfully managing pathogens, particularly due to their potent antimicrobial activity (AMA) against a wide range of bacterial pathogens (Singh et al., 2015; Aziz and Karboune, 2018; Sima et al., 2018). NAM have garnered considerable attention owing to their non-toxic nature and rising concern over the safety of synthetic chemicals, which have historically been used as food preservatives and flavor enhancers (Salamci et al., 2007). Phenolic isomers, carvacrol, and thymol are the active ingredients in oregano oil (Origanum glandulosum). Trans-cinnamaldehyde is an aromatic aldehyde present in cinnamon bark extract (Cinnamomum zeylandicum), and eugenol is a polyphenolic compound found in clove oil (Eugenia caryophillis) (Jensen, 2002; Mattson et al., 2011). All these compounds are described as “generally recognized as safe” (GRAS) by the Food and Drug Administration (Lambert et al., 2001; Venkitanarayanan et al., 2013).
Although several studies have examined the activity of NAM against numerous discrete microbes, including pathogens borne in various ways (Friedman et al., 2002; Kollanoor Johny et al., 2010; Venkitanarayanan et al., 2013; Upadhyaya et al., 2016; Sima et al., 2018; Pinkerton et al., 2019), different studies have varying antimicrobial sample sources, testing methods and bacterial strains, indices to evaluate the results (Fyfe et al., 1998; Ettayebi, 2000; Delaquis, 2002). Thus, it is necessary to adopt an effective and standardized method to evaluate and quantify the synergistic roles of natural antimicrobial combinations.
Here, the discrete activities of carvacrol, eugenol, trans-cinnamaldehyde, oregano, and thymol, and their activities in paired combinations, were examined using the broth microdilution and checkerboard techniques. The checkerboard method (Shin and Kang, 2003; Filoche et al., 2005; Si et al., 2008) combined with combination index (CI) values was used to test the antimicrobial abilities of the compounds. The CI values are commonly used for the assessment of antimicrobial properties of NAM. In addition, the synergistic effects of the components individually or along with organic acids were tested against foodborne pathogens using the fractional inhibitory concentration (FIC) and EC indices (Franzot and Casadevall, 1997; Zhou et al., 2007a,b).
The five components have strong antibacterial effects, but their application in animals is not restricted by their flavor. Herein, the efficacies of NAM against antibiotic-resistant bacteria were assessed. The aim of this research was to compare the AMA of NAM and develop a standard parameter for comparing dose-response data. Moreover, the effective concentrations of paired combinations of carvacrol, eugenol, oregano, thymol, and trans-cinnamaldehyde against poultry-borne pathogens were reduced. We also evaluated the synergistic interaction of these agents against resistant target bacteria. Furthermore, an enhanced technique was established for the evaluation and quantification of the synergistic combinations. It is well-understood that the bacterial growth-inhibition mechanisms of many NAM provide a safe, efficient, and cost-effective way of managing antibiotic resistance in the food animal production chain.
Selected NAM that are active against target bacterial pathogens were used in this study. Analytical grade trans-cinnamaldehyde (99% purity), eugenol (99% purity), carvacrol (98% purity), thymol (99% purity), geraniol (99% purity), linalool (99% purity), and citral (99% purity), as well as dimethyl sulfoxide (DMSO, 99.9%), were acquired from Sigma-Aldrich (St. Louis, MO, United States). Oregano (98% purity), mugwort (98% purity), and curcumin were obtained from RHAWN (Shanghai, China).
Escherichia coli 8G4 and Klebsiella pneumoniae 208G28, both strains derived from broiler chickens at the Institute of Quality Standards and Testing Technology for Agro-Products of the Chinese Academy of Agricultural Sciences CAAS (Beijing, China), were used for analysis. Three strains—E. coli BNCC 336435, K. pneumoniae BNCC 102997, and Salmonella pullorum BNCC 19945—were purchased from Jiangsu BeNa Culture Collection (Jiangsu, China) and used in this study. All bacteriological media were acquired from Beijing Land Bridge Technology Co., Ltd. (Beijing, China). Bacterial stock cultures were maintained at −80°C in nutrient broth (NB) medium (Beijing Land Bridge Technology Co., Ltd., Beijing, China) containing 15% glycerol. Working cultures of the poultry-borne pathogens were maintained at 4°C on nutrient agar (NA) medium (Beijing Land Bridge Technology Co., Ltd., Beijing, China). Prior to the experiment, a single colony was transferred from NA to 10.0 ml NB, followed by overnight incubation at 37°C with shaking at 150 rpm. Subsequently, 100 μl of bacterial suspension was added to 9.9 ml NB before incubation at 37°C for 12 h to acquire log phase cultures. Bacterial density was adjusted to 1 × 109 CFU/ml using the Infinite M200 PRO microplate reader (TECAN, San José, CA, United States) and diluted to an optical density (OD) of 0.1 (approximately 1 × 106 CFU/ml) at 600 nm.
Minimum inhibitory concentrations (MICs) of NAM indicating bacterial resistance were established using the broth microdilution assay, following a protocol described previously (Wiegand et al., 2008), with some alterations. Initially, we selected more than a dozen different types of NAM to inhibit poultry-borne pathogenic bacteria, after consulting the literature. Subsequently, five plant extracts (trans-cinnamaldehyde, eugenol, carvacrol, thymol, and oregano) were selected as study variables. A certain amount of each plant extract component was dissolved in DMSO and added to NB, to obtain a stock solution of approximately 2,000 μg/mL.
All natural antimicrobial solutions were prepared and filter-sterilized using 0.22 μm-pore filters (Millipore Sigma, Bedford, MA, United States) before each use. A minimum of eight concentrations in a geometric series and one control were prepared for each chemical. First, 200 μL of diluted antimicrobial solution was added to the Corning Costar® 96-well microplate (Corning Incorporated, NY, United States). Thereafter, 20μL of the prepared bacterial solution was inoculated before plate incubation at 37°C for 24 h with shaking at 150 rpm. Finally, absorbance was detected using the Infinite M200 PRO microplate reader (TECAN) at 600 nm. A minimum of three distinct experiments were performed for each compound, and the results are presented as the percentage inhibition rate (%) in relation to control. The lowest concentration of NAM required to completely inhibit the growth of the tested microorganism was designated as the MIC.
Herein, we applied the CI method to assess the interactions of the compounds, derived from the median effect principle (Chou and Talalay, 1984). The CI-isobologram equation, which is based on major biochemical and biophysical equations from the median-effect equation, was employed for the assessment of the interactions of trans-cinnamaldehyde, eugenol, carvacrol, thymol, and oregano. For the combination of the compounds, the following equation was applied:
where n(CI)x is the CI of n compounds at x% suppression, (Dx)1–n is the sum concentrations of n compounds that produce x% synergistic suppression, (Dx)1–n{([D]j/ is the proportional concentration of each n compound that produce x% synergistic suppression; and (Dm)j{fax)j/[1–(fax)j]1/mj is the concentration of discrete compound that produces x% suppression, in which Dm is the median-effect dose, fax is the fractional suppression at x% suppression, and m is the slope of the median-effect plot. The Chou-Talalay technique is often employed in drug combination investigations and offers an estimation of the drug synergistic effect by computing the CI. Accordingly, CI < 1 indicates synergistic effect, CI < 0.5 indicates highly synergistic outcome, CI = 1 indicates additive outcome, and CI > 1 indicates antagonistic effect (Chou, 2006). The types of interactions between NAM were determined using CompuSyn software 2.0.
Half-maximal inhibitory concentration (IC50) and MIC values were obtained by fitting the dose-response curve of the antibiotics using advanced biometric software, such as SPSS and Origin. One-way analysis of variance (ANOVA) and Duncan’s multiple comparison test using the Origin software were used to analyze the experimental data and marked differences (P < 0.05) between means of both groups. The CompuSyn computer program was employed for the determination of the concentration-effect curve parameters and CI values.
Table 1 summarizes the MICs of 10 NAM producing distinct effects against poultry-borne pathogenic bacteria. Generally, NAM included in the evaluation were effective against the relevant pathogenic bacteria. Growth of E. coli was inhibited by carvacrol at 241.4 μg/mL, trans-cinnamaldehyde at 519.3 μg/mL, eugenol at 502.0 μg/mL, and oregano at 241.4 μg/mL. At 198.4 μg/mL, thymol was effective against S. pullorum, E. coli, and K. pneumoniae. Furthermore, carvacrol (167.4 μg/mL), trans-cinnamaldehyde (411.2 μg/mL), eugenol (502.0 μg/mL), thymol (198.4 μg/mL), and oregano (232.2 μg/mL) inhibited the growth of S. pullorum. Additionally, thymol concentration needed to inhibit the growth of all microorganisms was lower than that of the other NAMs.
Table 1. Minimum inhibitory concentrations (MIC) values of natural antimicrobials against Poultry-borne pathogens.
In addition, the MIC values of mugwort and linalool against five bacterial strains were more than 904.6 and 860.5 μg/mL, respectively, while the MIC values of geraniol and citral were 869.4 and 878.3 μg/mL, respectively, against S. pullorum and E. coli. For K. pneumoniae, the required concentrations of geraniol and citral were more than 869.4 and 878.3 μg/mL, respectively. Due to the color and solubility characteristics of curcumin causing too much deviation in the results, no further research was conducted using this compound.
Overall, the order of antimicrobial efficacy of the NAM employed in our experiments was established, as follows: thymol > oregano > carvacrol > trans-cinnamaldehyde > eugenol > geraniol > citral > linalool > mugwort.
The growth inhibitory effects of trans-cinnamaldehyde, eugenol, carvacrol, thymol, and oregano, used alone or in combination, were tested against poultry-borne pathogenic bacteria. Here, S. pullorum is presented as an example, and results related to other poultry-borne pathogenic bacteria are shown in Figures 1–3 (Supplementary Figures 1, 2 in Supplementary Information) Dose-response, effects of trans-cinnamaldehyde, eugenol, carvacrol, thymol, and oregano, individually and in combination with other NAM, were tested against S. pullorum (Figure 1I). The ANOVA data embodied the correlation between single components and the synergistic group. All tested NAM elicited a dose-dependent response from S. pullorum, indicating that higher tested doses resulted in greater inhibition. For example, when combining thymol and trans-cinnamaldehyde, the natural antibacterial effect of thymol increased along with the dose. The inhibition rate increased significantly (p < 0.05) at concentrations between 12.4 and 198.4 μg/mL, but no significant (p > 0.05) change was observed between 24.8 and 49.6 μg/mL. Beyond 49.6 μg/mL, the growth inhibition rate of S. pullorum increased significantly (p < 0.05), but no marked elevations were seen at the highest concentrations. Thus, despite continued increases in the concentration, the inhibition rate did not notably change, perhaps due to drug accumulation having reached saturation of solubility. Significant differences were observed between all five tested concentrations of trans-cinnamaldehyde. A combination of thymol and trans-cinnamaldehyde induced no significant increase in growth inhibition rate at the two lower concentrations; however, marked elevation were observed at the four higher concentrations.
Figure 1. (A–I) Effect of single and combined of natural antimicrobials on the growth inhibition rate of Salmonella pullorum BNCC 19945. The significant differences among different concentrations or individual and combinations were analyzed using one-way ANOVA, followed by Duncan multiple comparisons (P < 0.05 represent significant differences). The differences between various concentrations and groups are marked by letters: the same letter represent no differences, while different letter indicate significant differences.
Figure 2. Effect of single and combined of natural antimicrobials on the growth inhibition rate of Klebsiella pneumoniae BNCC 102997.
Figure 3. (A–I) Effect of single and combined of natural antimicrobials on the growth inhibition rate of Klebsiella pneumoniae sample 208G28. Note: The significant differences among different concentrations or individual and combinations were analyzed using one-way ANOVA, followed by Duncan multiple comparisons (P < 0.05 represents significant differences). The differences between various concentrations and groups are marked by letters: the same letters represent no differences, while different letters indicate significant differences.
According to the CI model, the relationship between the effects of drug combinations on the fraction affected (fa) levels of poultry-borne pathogens, which are generated using CompuSyn, and the CI index, was evaluated. Furthermore, the type and degree of interaction between the combined drugs (synergistic, antagonistic, or additive) were evaluated. Concentrations used in the individual natural antimicrobial exposures and the parameters of the curve fit of the concentration–response data are presented in Supplementary InformationSupplementary Table 1. The degree of interaction between drug combinations was evaluated according to the CI index, outcomes of which are presented in Table 2.
Table 2. Dose-effect relationship parameters and mean combination index (CI) values of drug combinations for bacterial strains.
Synergistic interaction was examined by calculating the CI values of the NAM against poultry-borne pathogenic bacteria evaluated in this study; values are shown in Figures 4A–E, revealing the degree of synergistic effect. S. pullorum (Figure 4A) is presented as an example. Following exposure to the combination of eugenol and thymol (or oregano) for 24 h, a stable synergistic effect was observed between 0.05 and 0.97 fa levels. A combination of thymol and carvacrol displayed a slight antagonistic behavior between 0.05 and 0.97 fa levels. A combination of eugenol and carvacrol showed very strong synergism at the 0.05 fa level; an additive property was seen at fa levels between 0.75 and 0.97, which became synergistic with increasing degree at lower fa levels. The combination of eugenol and trans-cinnamaldehyde exhibited a very strong synergistic effect, with an fa level of 0.05, which changed to a strong synergistic effect, with the fa level rising to 0.25. An additive effect was perceived between 0.30 and 0.65 fa levels, and higher fa levels yielded antagonistic effects, which became slightly antagonistic at fa levels between 0.70 and 0.80 and moderately antagonistic from 0.85 to 0.97. The combination of trans-cinnamaldehyde and carvacrol showed very potent synergism at an fa level of 0.05, an additive effect at fa levels from 0.10 to 0.30, and an antagonistic effect between 0.35 and 0.97. In the trans-cinnamaldehyde and oregano mixture, the 0.05, 0.10, and 0.15 fa levels gave strong synergistic effects, with an additive effect observed at fa levels from 0.20 to 0.40, and higher fa levels (between 0.45 and 0.97) yielding antagonistic effects. Combined thymol and trans-cinnamaldehyde showed an additive effect between 0.05 and 0.25 fa levels, and an antagonistic effect from 0.30 to 0.97. Lastly, the combination of thymol and oregano exhibited a strong synergism between 0.05 and 0.40 fa levels, changing to an additive property with the fa level rising to 0.85; fa levels at 0.90, 0.95, and 0.97 gave antagonistic effects.
Figure 4. Evaluation of combined effects of binary natural antimicrobials combinations based on CI model. Combination index plot (fa–CI plot) for pairwise drug combinations: (A) Salmonella pullorum BNCC 19945; (B) Klebsiella pneumoniae BNCC 102997; (C) K. pneumoniae Sample 208G28; (D) Escherichia coli BNCC 336435; (E) E. coli Sample 8G4.
Based on the antibacterial effects of these combination groups, we derived several consistent conclusions. First, eugenol showed a more potent synergistic property than the other NAM, although the antibacterial property of eugenol alone was the weakest among all five compounds. The synergistic outcomes of trans-cinnamaldehyde and eugenol may be due to the action of eugenol/trans-cinnamaldehyde on enzymes or diverse proteins. Moreover, the synergistic properties of carvacrol/eugenol and thymol/eugenol may be due to thymol and carvacrol disintegrating the outer membrane of S. pullorum, enabling eugenol entry into the cytoplasm to interact with proteins. Second, thymol showed a potent antibacterial behavior against poultry-borne pathogens, while its interactive effects with other compounds were not significant. Conversely, the paired combination of carvacrol and thymol (total dose 183 μg/mL) showed synergistic effects against E. coli, S. pullorum, and K. pneumoniae. Additionally, for S. pullorum, all CI values were less than 1.0, which indicates zero antagonism between eugenol and thymol (or oregano). Given these results, the paired combinations at certain concentrations present synergistic AMA, at least against majority of the test bacteria.
In this study, we aimed to identify several synergistic NAM combinations that can inhibit the growth of poultry-borne bacterial pathogens. Eight NAM, often referred to as active aromatic compounds, can inhibit bacterial growth; these include carvacrol (Pei et al., 2009; Palaniappan and Holley, 2010; Pinheiro et al., 2017; Walsh et al., 2019), trans-cinnamaldehyde (Kollanoor Johny et al., 2010; Palaniappan and Holley, 2010; Hermans et al., 2011), thymol (Oussalah et al., 2007), oregano (Friedman et al., 2002; Aslim and Yucel, 2008; Ravishankar et al., 2008), citral (Tang et al., 2018), curcumin (Ravishankar et al., 2008; Navarro et al., 2015), eugenol (Charan Raja, 2015; Liu et al., 2015), and geraniol (Tang et al., 2018). However, data related to the associations between different NAM are limited, and an extensive investigation is needed to provide empirical data on the bactericidal effects of combinational therapies.
Here, five NAM (trans-cinnamaldehyde, eugenol, carvacrol, thymol, and oregano) were found active against tested strains of poultry-borne bacteria in vitro. We noted that thymol was more effective than the other four compounds, exhibiting the lowest MIC value (198 μg/mL) against five bacterial strains. Pei et al. (2009) used carvacrol, cinnamaldehyde, eugenol, and thymol against E. coli and observed MIC values of 9.7, 2.6, 3.0, and 2.6 mM, respectively. In the present study, the MIC values of eugenol and trans-cinnamaldehyde were comparable to those of Pei et al. (2009). Our results further agreed with those of Olasupo et al. (2003), wherein the MIC values of 1.5 and 1.0 mM, 2.5 and 3.0 mM, and 1.2 and 1.0 mM for carvacrol, eugenol, and thymol, respectively, inhibited E. coli and Salmonella typhimurium. Our data also correlates with those by Rivas et al. (2010), who demonstrated the AMA of thymol and carvacrol against verocytotoxigenic E. coli and E. coli O157:H7 at 500 μg/mL, as well as those of Helander et al. (1998) showing that thymol, cinnamaldehyde, and carvacrol inhibited E. coli and S. typhimurium at concentrations ranging from 1 to 3 mM. Miladi et al. (2017) used thymol and carvacrol against a panel of clinical and foodborne pathogens and observed MIC values ranging from 32–128 mg/L and 32–64 mg/L, respectively. However, the MICs of thymol and carvacrol in our study were slightly higher for gram-negative bacteria. The discrepancy in AMA could be attributed to testing the compounds against strains with elevated resistance to the examined antibiotics. Additionally, the mechanism of antibacterial action may vary between different bacteria (Pei et al., 2009). Oussalah et al. (2007) studied the AMA of 28 essential oils against four pathogens and showed that the majority of oils inhibited E. coli, S. typhimurium, Listeria monocytogenes, and Staphylococcus aureus. Among the bacteria tested herein, S. pullorum was most responsive to the employed NAM, and the concentrations of trans-cinnamaldehyde and carvacrol required for inhibition were less than for E. coli and K. pneumoniae. In addition, previous reports showed that NAM also demonstrated important antifungal (Aspergillus flavus) properties (López-Malo et al., 2002; Lopez-Malo et al., 2005, 2006; Garcia-Garcia et al., 2011). A. flavus exhibited higher sensitivity to thymol, eugenol, carvacrol, sodium bisulfite, and sodium benzoate (at pH 3.5) than to vanillin or citral. Moreover, liquid model systems based on the binary and ternary mixtures on Listeria innocua inactivation were used to evaluate bactericidal effect of three natural agents (carvacrol, thymol, and eugenol). Results showed that the most effective individual antimicrobial agent was carvacrol, followed by thymol and eugenol, and the most effective binary mixture was 75 mg/kg of carvacrol mixed with 62.5 mg/kg of thymol.
The AMA of thymol, oregano, trans-cinnamaldehyde, eugenol, and carvacrol exhibited comparable results, although our results concluded that the order of AMA efficacy was thymol > oregano > carvacrol > trans-cinnamaldehyde > eugenol. However, Michiels et al. (2007) reported that the AMA efficacy order against E. coli in small intestinal simulations was cinnamaldehyde > carvacrol > thymol, while Friedman et al. (2002) observed the order carvacrol > cinnamaldehyde > thymol against E. coli and Salmonella enterica, using a microplate assay. Olasupo et al. (2003) found that thymol was more effective than other essential oils, and the AMA order of natural organic compounds against E. coli and S. typhimurium was carvacrol > eugenol > cinnamic acid > diacetyl, evaluated using a checkerboard assay and the Bioscreen C instrument. These differences in AMA order observed in various studies may be attributed to the various evaluation techniques performed to assess the AMA. Moreover, several factors such as culture medium from different manufacturers, growth curve discrepancies, differences in bacterial growth phases among the different bacteria, pH, incubation temperature and time, and volume of the inoculum could affect experimental outcomes.
Previous studies have revealed the different techniques used to evaluate the synergistic behavior of NAM. Many researchers have used the checkerboard technique based on the FIC and EC indices to evaluate combined effects (Si et al., 2008). Our research used the broth microdilution assay associated with the checkerboard method based on the CI and fa indices to evaluate combined effects (Chou, 2006). Synergy is defined as the synergistic effect of two compounds that is larger than the sum of the effects of each individual compound (Kobilinsky et al., 2007). The efficacy of synergistic associations of plant-based antimicrobials are well-established (Brenes and Roura, 2010) and is based on the principle that combining active compounds augments AMA, while diminishing toxicity and amount of substance in the final formula. Pei et al. (2009) defined the synergistic effects of thymol/carvacrol, cinnamaldehyde/eugenol, thymol/eugenol, and carvacrol/eugenol. Likewise, the effective concentrations of the five components evaluated in this study could be significantly lower in combination than when used alone.
Little is known about the exact mechanism by which NAM reduce antibiotic resistance; however, it is likely due to structural alterations occurring in the resistant bacteria after antimicrobial exposure. Recently, Nowotarska et al. (2014) used model membranes to demonstrate penetration of the phenolic group of carvacrol into the lipid molecules of the bacterial membrane. Similarly, Stratakos et al. (2018) provided antimicrobial mechanistic insights on carvacrol, demonstrating its significant efficacy against clinically relevant Shiga toxin-synthesizing E. coli (STEC) and non-STEC strains. Gayan et al. (2020) examined the synergistic effect of carvacrol in combination with mild heat and showed pronounced inactivation of the heat-resistant E. coli ATCC 43888. Lambert et al. (2001) found that thymol and carvacrol are hydrophobic and susceptible to disturbing the outer membrane of gram-negative bacteria, enhancing permeability of the cytoplasmic membrane to adenosine triphosphate, releasing lipopolysaccharides, and abrogating the inhibitory effects of protective enzymes. Accordingly, several studies have reported that the carbonyl group on trans-cinnamaldehyde might bind to proteins, preventing amino acid decarboxylase activities, while the hydroxyl (-OH) group on eugenol may bind to proteins, causing structural breakdown, deactivating enzyme, ultimately stimulating cell membrane lysis and bacterial death (Hemaiswarya et al., 2008; Palaniappan and Holley, 2010; Quinto et al., 2019). We hypothesized that the mechanisms of AMA may be different, or that the combinational action may alter against various bacteria. Additional investigations are warranted to enhance the understanding of the mechanism behind the synergistic properties of NAM.
Several previous reports have indicated that NAM can protect against bacterial infection and lower pathogen virulence in vitro. However, few studies have demonstrated their effectiveness in living animal models (Abudabos et al., 2019; Liu et al., 2020). Previous studies have demonstrated the positive effects of various phytogenic feed additives in broilers (Si et al., 2006; Sengül et al., 2008; Alzawqari et al., 2016; Khan et al., 2019). Production parameters improved in birds supplemented with herbal oil, which consists of different important alkaloids (Alzawqari et al., 2016; Khan et al., 2019). The results of the present study are similar those of Sengül et al. (2008), who showed that the growth performance of Japanese quail was not negatively affected by supplementation with thyme extract. Rather, these parameters improved notably with addition of thyme extract products to their diets. In addition, Si et al. (2006) used thymol, carvacrol, and trans-cinnamaldehyde against Salmonella in pigs and found that higher concentrations were required to maintain AMA when introduced in the diet.
In summary, we highlighted the potential use of thymol and carvacrol as combined treatment for controlling poultry-borne bacterial pathogens. We further concluded that the antibacterial abilities of NAM were enhanced when used in combination, and the minimum effective concentrations could be decreased substantially. In our study, we provided empirical and comprehensive data for synergistic outcomes. Further studies should establish the mode of action of combined treatments and assess the effectiveness of these combined compounds in animal intestinal models and animals.
The activity of NAM alone and in combination against common poultry pathogens (S. pullorum, E. coli, and K. pneumoniae) was evaluated using broth dilution and checkerboard methods, and the types of interactions between two combined drugs were evaluated based on CI model. The comprehensive analysis showed that the order of the inhibitory effects of the NAM on pathogens was: thymol > oregano > carvacrol > trans-cinnamaldehyde > eugenol > geraniol > citral > linalool > mugwort. Thymol had the strongest inhibitory effect on common pathogens and showed a good antibacterial effect. The combined interaction of NAM (carvacrol, eugenol, trans-cinnamaldehyde, oregano, and thymol) was evaluated using the CI model, and the synergistic effect of the combination of carvacrol and thymol was observed to have better inhibition effect on common pathogens in vitro. Thus, the combination of thymol and carvacrol was selected for comprehensive analysis to study the effect of NAM on microbial resistance in broilers.
The original contributions presented in the study are included in the article/Supplementary Material, further inquiries can be directed to the corresponding author.
SY conceived and designed the experiments. RZ and RL performed statistical and data analysis. QL and JW contributed to the reagents and materials. XL wrote this manuscript and prepared the original draft. YC and YW provided the detection samples in this research. All authors have read and agreed to the published version of the manuscript.
This study was funded by the National Key Research and Development Program (EU: SFS-46-2017, China: 2017YFE0114400).
The authors declare that the research was conducted in the absence of any commercial or financial relationships that could be construed as a potential conflict of interest.
All claims expressed in this article are solely those of the authors and do not necessarily represent those of their affiliated organizations, or those of the publisher, the editors and the reviewers. Any product that may be evaluated in this article, or claim that may be made by its manufacturer, is not guaranteed or endorsed by the publisher.
We would like to thank SY for instructing this study.
The Supplementary Material for this article can be found online at: https://www.frontiersin.org/articles/10.3389/fmicb.2022.811784/full#supplementary-material
CI, combination index; MIC, minimum inhibitory concentrations; CLSI, Clinical and Laboratory Standards Institute; EC, effect of the combination.
Abudabos, A. M., Hussein, E. O. S., Ali, M. H., and Al-Ghadi, M. Q. (2019). The effect of some natural alternative to antibiotics on growth and changes in intestinal histology in broiler exposed to Salmonella challenge. Poult. Sci. 98, 1441–1446. doi: 10.3382/ps/pey449
Aljumaah, M. R., Alkhulaifi, M. M., and Abudabos, A. M. (2020). In vitro antibacterial efficacy of non-antibiotic growth promoters in poultry industry. J. Poult. Sci. 57, 45–54. doi: 10.2141/jpsa.0190042
Alzawqari, M. H., Al-Baddany, A. A., Al-Baadani, H. H., Alhidary, I. A., Khan, R. U., Aqil, G. M., et al. (2016). Effect of feeding dried sweet orange (Citrus sinensis) peel and lemon grass (Cymbopogon citratus) leaves on growth performance, carcass traits, serum metabolites and antioxidant status in broiler during the finisher phase. Environ. Sci. Pollut. Res. Int. 23, 17077–17082. doi: 10.1007/s11356-016-6879-7
Aslim, B., and Yucel, N. (2008). In vitro antimicrobial activity of essential oil from endemic Origanum minutiflorum on ciprofloxacin-resistant Campylobacter spp. Food Chem. 107, 602–606. doi: 10.1016/j.foodchem.2007.08.048
Aziz, M., and Karboune, S. (2018). Natural antimicrobial/antioxidant agents in meat and poultry products as well as fruits and vegetables: a review. Crit. Rev. Food Sci. Nutr. 58, 486–511. doi: 10.1080/10408398.2016.1194256
Brenes, A., and Roura, E. (2010). Essential oils in poultry nutrition: main effects and modes of action. Animal Feed Sci. Technol. 158, 1–14. doi: 10.1016/j.anifeedsci.2010.03.007
Charan Raja, M. R. (2015). Versatile and synergistic potential of eugenol: a review. Pharmaceutica Anal. Acta 6:5. doi: 10.4172/2153-2435.1000367
Chou, T. C. (2006). Theoretical basis, experimental design, and computerized simulation of synergism and antagonism in drug combination studies. Pharmacol. Rev. 58, 621–681. doi: 10.1124/pr.58.3.10
Chou, T. C., and Talalay, P. (1984). Quantitative analysis of dose-effect relationships: the combined effects of multiple drugs or enzyme inhibitors. Adv. Enzyme Regul. 22, 27–55. doi: 10.1016/0065-2571(84)90007-4
Courtenay, M., Castro-Sanchez, E., Fitzpatrick, M., Gallagher, R., Lim, R., and Morris, G. (2019). Tackling antimicrobial resistance 2019-2024 - the UK’s five-year national action plan. J. Hosp. Infect. 101, 426–427. doi: 10.1016/j.jhin.2019.02.019
Delaquis, P. (2002). Antimicrobial activity of individual and mixed fractions of dill, cilantro, coriander and eucalyptus essential oils. Int. J. Food Microbiol. 74, 101–109. doi: 10.1016/s0168-1605(01)00734-6
Drame, O., Leclair, D., Parmley, E. J., Deckert, A., Ouattara, B., Daignault, D., et al. (2020). Antimicrobial resistance of campylobacter in broiler chicken along the food chain in Canada. Foodborne Pathog Dis. 17, 512–520. doi: 10.1089/fpd.2019.2752
Ettayebi, K. (2000). Synergistic effects of nisin and thymol on antimicrobial activities in Listeria monocytogenes and Bacillus subtilis. FEMS Microbiol. Lett. 183, 191–195. doi: 10.1111/j.1574-6968.2000.tb08956.x
Filoche, S. K., Soma, K., and Sissons, C. H. (2005). Antimicrobial effects of essential oils in combination with chlorhexidine digluconate. Oral. Microbiol. Immunol. 20, 221–225. doi: 10.1111/j.1399-302X.2005.00216.x
Franzot, S. P., and Casadevall, A. (1997). Pneumocandin L-743,872 enhances the activities of amphotericin B and fluconazole against Cryptococcus neoformans in vitro. Antimicrob. Agents Chemother. 41, 331–336. doi: 10.1128/AAC.41.2.331
Friedman, M., Henika, P. R., and Mandrell, R. E. (2002). Bactericidal activities of plant essential oils and some of their isolated constituents against Campylobacter jejuni, Escherichia coli, Listeria monocytogenes, and Salmonella enterica. J. Food Prot. 65, 1545–1560. doi: 10.4315/0362-028x-65.10.1545
Fyfe, L., Armstrong, F., and Stewart, J. (1998). Inhibition of Listeria monocytogenes and Salmonella enteriditis by combinations of plant oils and derivatives of benzoic acid: the development of synergistic antimicrobial combinations1The work in this manuscript is the subject of a patent application.1. Int. J. Antimicrobial Agents 9, 195–199. doi: 10.1016/s0924-8579(97)00051-4
Garcia-Garcia, R., Lopez-Malo, A., and Palou, E. (2011). Bactericidal action of binary and ternary mixtures of carvacrol, thymol, and eugenol against Listeria innocua. J. Food Sci. 76, M95–M100. doi: 10.1111/j.1750-3841.2010.02005.x
Gayan, E., Geens, E., Berdejo, D., Garcia-Gonzalo, D., Pagan, R., Aertsen, A., et al. (2020). Combination of mild heat and plant essential oil constituents to inactivate resistant variants of Escherichia coli in buffer and in coconut water. Food Microbiol. 87:103388. doi: 10.1016/j.fm.2019.103388
Helander, I. M., Alakomi, H.-L., Latva-Kala, K., Mattila-Sandholm, T., Pol, I., Smid, E. J., et al. (1998). Characterization of the action of selected essential oil components on gram-negative bacteria. J. Agric. Food Chem. 46, 3590–3595. doi: 10.1021/jf980154m
Hemaiswarya, S., Kruthiventi, A. K., and Doble, M. (2008). Synergism between natural products and antibiotics against infectious diseases. Phytomedicine 15, 639–652. doi: 10.1016/j.phymed.2008.06.008
Hermans, D., Martel, A., van Deun, K., van Immerseel, F., Heyndrickx, M., Haesebrouck, F., et al. (2011). The cinnamon-oil ingredient trans-cinnamaldehyde fails to target Campylobacter jejuni strain KC 40 in the broiler chicken cecum despite marked in vitro activity. J. Food Prot. 74, 1729–1734. doi: 10.4315/0362-028X.JFP-10-487
Jensen, R. G. (2002). The composition of bovine milk lipids: January 1995 to December 2000. J. Dairy Sci. 85, 295–350. doi: 10.3168/jds.S0022-0302(02)74079-4
Khan, R. U., Naz, S., Nikousefat, Z., Tufarelli, V., and Laudadio, V. (2019). Thymus vulgaris: alternative to antibiotics in poultry feed. World’s Poultry Sci. J. 68, 401–408. doi: 10.1017/s0043933912000517
Kobilinsky, A., Nazer, A. I., and Dubois-Brissonnet, F. (2007). Modeling the inhibition of Salmonella typhimurium growth by combination of food antimicrobials. Int. J. Food Microbiol. 115, 95–109. doi: 10.1016/j.ijfoodmicro.2006.10.039
Kollanoor Johny, A., Darre, M. J., Donoghue, A. M., Donoghue, D. J., and Venkitanarayanan, K. (2010). Antibacterial effect of trans-cinnamaldehyde, eugenol, carvacrol, and thymol on Salmonella enteritidis and Campylobacter jejuni in chicken cecal contents in vitro. J. Appl. Poultry Res. 19, 237–244. doi: 10.3382/japr.2010-00181
Lambert, R. J., Skandamis, P. N., Coote, P. J., and Nychas, G. J. (2001). A study of the minimum inhibitory concentration and mode of action of oregano essential oil, thymol and carvacrol. J. Appl. Microbiol. 91, 453–462. doi: 10.1046/j.1365-2672.2001.01428.x
Liu, Q., Niu, H., Zhang, W., Mu, H., Sun, C., and Duan, J. (2015). Synergy among thymol, eugenol, berberine, cinnamaldehyde and streptomycin against planktonic and biofilm-associated food-borne pathogens. Lett. Appl. Microbiol. 60, 421–430. doi: 10.1111/lam.12401
Liu, S., Ahmed, S., Zhang, C., Liu, T., Shao, C., and Fang, Y. (2020). Diversity and antimicrobial activity of culturable fungi associated with sea anemone Anthopleura xanthogrammica. Electron. J. Biotechnol. 44, 41–46. doi: 10.1016/j.ejbt.2020.01.003
López-Malo, A., Alzamora, S. M., and Palou, E. (2002). Aspergillus flavus dose–response curves to selected natural and synthetic antimicrobials. Int. J. Food Microbiol. 73, 213–218. doi: 10.1016/s0168-1605(01)00639-0
Lopez-Malo, A., Maris Alzamora, S., and Palou, E. (2005). Aspergillus flavus growth in the presence of chemical preservatives and naturally occurring antimicrobial compounds. Int. J. Food Microbiol. 99, 119–128. doi: 10.1016/j.ijfoodmicro.2004.08.010
Lopez-Malo, A., Palou, E., Leon-Cruz, R., and Alzamora, S. M. (2006). Mixtures of natural and synthetic antifungal agents. Adv. Exp. Med. Biol. 571, 261–286. doi: 10.1007/0-387-28391-9_18
Mattson, T. E., Johny, A. K., Amalaradjou, M. A., More, K., Schreiber, D. T., Patel, J., et al. (2011). Inactivation of Salmonella spp. on tomatoes by plant molecules. Int. J. Food Microbiol. 144, 464–468. doi: 10.1016/j.ijfoodmicro.2010.10.035
Michiels, J., Missotten, J., Fremaut, D., De Smet, S., and Dierick, N. (2007). In vitro dose–response of carvacrol, thymol, eugenol and trans-cinnamaldehyde and interaction of combinations for the antimicrobial activity against the pig gut flora. Livestock Sci. 109, 157–160. doi: 10.1016/j.livsci.2007.01.132
Miladi, H., Zmantar, T., Kouidhi, B., Chaabouni, Y., Mahdouani, K., Bakhrouf, A., et al. (2017). Use of carvacrol, thymol, and eugenol for biofilm eradication and resistance modifying susceptibility of Salmonella enterica serovar Typhimurium strains to nalidixic acid. Microb. Pathog. 104, 56–63. doi: 10.1016/j.micpath.2017.01.012
Naeim, H., El-Hawiet, A., Abdel Rahman, R. A., Hussein, A., El Demellawy, M. A., and Embaby, A. M. (2020). Antibacterial activity of Centaurea pumilio L. root and aerial part extracts against some multidrug resistant bacteria. BMC Complement Med. Ther. 20:79. doi: 10.1186/s12906-020-2876-y
Navarro, M., Stanley, R., Cusack, A., and Sultanbawa, Y. (2015). Combinations of plant-derived compounds against Campylobacter in vitro. J. Appl. Poultry Res. 24, 352–363. doi: 10.3382/japr/pfv035
Nowotarska, S. W., Nowotarski, K. J., Friedman, M., and Situ, C. (2014). Effect of structure on the interactions between five natural antimicrobial compounds and phospholipids of bacterial cell membrane on model monolayers. Molecules 19, 7497–7515. doi: 10.3390/molecules19067497
Olasupo, N. A., Fitzgerald, D. J., Gasson, M. J., and Narbad, A. (2003). Activity of natural antimicrobial compounds against Escherichia coli and Salmonella enterica serovar Typhimurium. Lett. Appl. Microbiol. 37, 448–451. doi: 10.1046/j.1472-765x.2003.01427.x
Oussalah, M., Caillet, S., Saucier, L., and Lacroix, M. (2007). Inhibitory effects of selected plant essential oils on the growth of four pathogenic bacteria: E. coli O157:H7, Salmonella Typhimurium, Staphylococcus aureus and Listeria monocytogenes. Food Control. 18, 414–420. doi: 10.1016/j.foodcont.2005.11.009
Palaniappan, K., and Holley, R. A. (2010). Use of natural antimicrobials to increase antibiotic susceptibility of drug resistant bacteria. Int. J. Food Microbiol. 140, 164–168. doi: 10.1016/j.ijfoodmicro.2010.04.001
Pei, R. S., Zhou, F., Ji, B. P., and Xu, J. (2009). Evaluation of combined antibacterial effects of eugenol, cinnamaldehyde, thymol, and carvacrol against E. coli with an improved method. J. Food Sci. 74, M379–M383. doi: 10.1111/j.1750-3841.2009.01287.x
Pinheiro, P. F., Menini, L. A. P., Bernardes, P. C., Saraiva, S. H., Carneiro, J. W. M., Costa, A. V., et al. (2017). Semisynthetic phenol derivatives obtained from natural phenols: antimicrobial activity and molecular properties. J. Agric. Food Chem. 66, 323–330. doi: 10.1021/acs.jafc.7b04418
Pinkerton, L., Linton, M., Kelly, C., Ward, P., Gradisteanu Pircalabioru, G., Pet, I., et al. (2019). Attenuation of Vibrio parahaemolyticus virulence factors by a mixture of natural antimicrobials. Microorganisms 7:679. doi: 10.3390/microorganisms7120679
Quinto, E. J., Caro, I., Villalobos-Delgado, L. H., Mateo, J., De-Mateo-Silleras, B., and Redondo-Del-Rio, M. P. (2019). Food safety through natural antimicrobials. Antibiotics (Basel) 8:208. doi: 10.3390/antibiotics8040208
Ravishankar, S., Zhu, L., Law, B., Joens, L., and Friedman, M. (2008). Plant-derived compounds inactivate antibiotic-resistant Campylobacter jejuni strains. J. Food Prot. 71, 1145–1149. doi: 10.4315/0362-028x-71.6.1145
Rivas, L., McDonnell, M. J., Burgess, C. M., O’Brien, M., Navarro-Villa, A., Fanning, S., et al. (2010). Inhibition of verocytotoxigenic Escherichia coli in model broth and rumen systems by carvacrol and thymol. Int. J. Food Microbiol. 139, 70–78. doi: 10.1016/j.ijfoodmicro.2010.01.029
Salamci, E., Kordali, S., Kotan, R., Cakir, A., and Kaya, Y. (2007). Chemical compositions, antimicrobial and herbicidal effects of essential oils isolated from Turkish Tanacetum aucheranum and Tanacetum chiliophyllum var. chiliophyllum. Biochem. Systemat. Ecol. 35, 569–581. doi: 10.1016/j.bse.2007.03.012
Sengül, T., Yurtseven, S., Cetin, M., Kocyigit, A., and Sögüt, B. (2008). Effect of thyme (T-vulgaris) extracts on fattening performance, some blood parameters, oxidative stress and DNA damage in Japanese quails. J. Animal Feed Sci. 17, 608–620. doi: 10.22358/jafs/66689/2008
Shan, B., Cai, Y. Z., Brooks, J. D., and Corke, H. (2007). The in vitro antibacterial activity of dietary spice and medicinal herb extracts. Int. J. Food Microbiol. 117, 112–119. doi: 10.1016/j.ijfoodmicro.2007.03.003
Shin, S., and Kang, C. A. (2003). Antifungal activity of the essential oil of Agastache rugosa Kuntze and its synergism with ketoconazole. Lett. Appl. Microbiol. 36, 111–115. doi: 10.1046/j.1472-765x.2003.01271.x
Si, H., Hu, J., Liu, Z., and Zeng, Z. L. (2008). Antibacterial effect of oregano essential oil alone and in combination with antibiotics against extended-spectrum beta-lactamase-producing Escherichia coli. FEMS Immunol. Med. Microbiol. 53, 190–194. doi: 10.1111/j.1574-695X.2008.00414.x
Si, W., Gong, J., Chanas, C., Cui, S., Yu, H., Caballero, C., et al. (2006). In vitro assessment of antimicrobial activity of carvacrol, thymol and cinnamaldehyde towards Salmonella serotype Typhimurium DT104: effects of pig diets and emulsification in hydrocolloids. J. Appl. Microbiol. 101, 1282–1291. doi: 10.1111/j.1365-2672.2006.03045.x
Sima, F., Stratakos, A. C., Ward, P., Linton, M., Kelly, C., Pinkerton, L., et al. (2018). A novel natural antimicrobial can reduce the in vitro and in vivo pathogenicity of T6SS positive Campylobacter jejuni and Campylobacter coli chicken isolates. Front. Microbiol. 9:2139. doi: 10.3389/fmicb.2018.02139
Singh, M., Smith, J., and Bailey, M. (2015). Using natural antimicrobials to enhance the safety and quality of poultry - ScienceDirect. Handb. Nat. Antimicrobials Food Safety Qual. 375–401. doi: 10.1016/B978-1-78242-034-7.00018-9
Stratakos, A. C., Sima, F., Ward, P., Linton, M., Kelly, C., Pinkerton, L., et al. (2018). The in vitro effect of carvacrol, a food additive, on the pathogenicity of O157 and non-O157 Shiga-toxin producing Escherichia coli. Food Control. 84, 290–296. doi: 10.1016/j.foodcont.2017.08.014
Tang, X., Shao, Y. L., Tang, Y. J., and Zhou, W. W. (2018). Antifungal activity of essential oil compounds (Geraniol and Citral) and inhibitory mechanisms on grain pathogens (Aspergillus flavus and Aspergillus ochraceus). Molecules 23:2108. doi: 10.3390/molecules23092108
Upadhyaya, I., Yin, H. B., Surendran Nair, M., Chen, C. H., Lang, R., Darre, M. J., et al. (2016). Inactivation of Salmonella enteritidis on shell eggs by coating with phytochemicals. Poult. Sci. 95, 2106–2111. doi: 10.3382/ps/pew152
Van Boeckel, T. P., Brower, C., Gilbert, M., Grenfell, B. T., Levin, S. A., Robinson, T. P., et al. (2015). Global trends in antimicrobial use in food animals. Proc. Natl. Acad. Sci. U S A. 112, 5649–5654. doi: 10.1073/pnas.1503141112
Venkitanarayanan, K., Kollanoor-Johny, A., Darre, M. J., Donoghue, A. M., and Donoghue, D. J. (2013). Use of plant-derived antimicrobials for improving the safety of poultry products. Poult. Sci. 92, 493–501. doi: 10.3382/ps.2012-02764
Walsh, D. J., Livinghouse, T., Goeres, D. M., Mettler, M., and Stewart, P. S. (2019). Antimicrobial activity of naturally occurring phenols and derivatives against biofilm and planktonic bacteria. Front. Chem. 7:653. doi: 10.3389/fchem.2019.00653
Wiegand, I., Hilpert, K., and Hancock, R. E. (2008). Agar and broth dilution methods to determine the minimal inhibitory concentration (MIC) of antimicrobial substances. Nat. Protoc. 3, 163–175. doi: 10.1038/nprot.2007.521
Wright, G. D. (2019). Unlocking the potential of natural products in drug discovery. Microb Biotechnol. 12, 55–57. doi: 10.1111/1751-7915.13351
Yang, Y., Ashworth, A. J., Willett, C., Cook, K., Upadhyay, A., Owens, P. R., et al. (2019). Review of antibiotic resistance, ecology, dissemination, and mitigation in U.S. broiler poultry systems. Front. Microbiol. 10:2639. doi: 10.3389/fmicb.2019.02639
Zhou, F., Ji, B., Zhang, H., Jiang, H., Yang, Z., Li, J., et al. (2007a). Synergistic effect of thymol and carvacrol combined with chelators and organic acids against Salmonella Typhimurium. J. Food Prot. 70, 1704–1709. doi: 10.4315/0362-028x-70.7.1704
Keywords: natural antimicrobials, antibiotic resistance, poultry-borne bacteria, minimum inhibitory concentrations, synergistic effect, combination index
Citation: Liu X, Liu R, Zhao R, Wang J, Cheng Y, Liu Q, Wang Y and Yang S (2022) Synergistic Interaction Between Paired Combinations of Natural Antimicrobials Against Poultry-Borne Pathogens. Front. Microbiol. 13:811784. doi: 10.3389/fmicb.2022.811784
Received: 09 November 2021; Accepted: 14 April 2022;
Published: 04 May 2022.
Edited by:
David Rodriguez-Lazaro, University of Burgos, SpainReviewed by:
Guadalupe Virginia Nevárez-Moorillón, Autonomous University of Chihuahua, MexicoCopyright © 2022 Liu, Liu, Zhao, Wang, Cheng, Liu, Wang and Yang. This is an open-access article distributed under the terms of the Creative Commons Attribution License (CC BY). The use, distribution or reproduction in other forums is permitted, provided the original author(s) and the copyright owner(s) are credited and that the original publication in this journal is cited, in accordance with accepted academic practice. No use, distribution or reproduction is permitted which does not comply with these terms.
*Correspondence: Shuming Yang, eWFuZ3NodW1pbmdAY2Fhcy5jbg==
Disclaimer: All claims expressed in this article are solely those of the authors and do not necessarily represent those of their affiliated organizations, or those of the publisher, the editors and the reviewers. Any product that may be evaluated in this article or claim that may be made by its manufacturer is not guaranteed or endorsed by the publisher.
Research integrity at Frontiers
Learn more about the work of our research integrity team to safeguard the quality of each article we publish.