- 1Key Laboratory of Marine Drugs, The Ministry of Education of China, School of Medicine and Pharmacy, Ocean University of China, Qingdao, China
- 2Laboratory for Marine Drugs and Bioproducts, Qingdao National Laboratory for Marine Science and Technology, Qingdao, China
- 3Institute of Evolution and Marine Biodiversity, Ocean University of China, Qingdao, China
Three new 2,5-diketopiperazines, speramide C (1), 3,21-epi-taichunamide F (2), and 2-epi-amoenamide C (3), along with four known analogs (4–7), were obtained from the sponge-derived fungus Aspergillus sclerotiorum GDST-2013-0501 collected from the South China Sea. The chemical structures of new compounds were elucidated by analyzing NMR and MS spectroscopy data, and their absolute configurations were determined by electronic circular dichroism (ECD) calculations. Compound 1 represents the first prenylated indole alkaloid with an ethylene oxide ring at the isopentenyl side chain. Compound 4 displayed DNA topoisomerase I inhibitory activity and antibacterial activity against Staphylococcus epidermidis. The low cytotoxic or non-cytotoxic compound 4 displayed DNA topoisomerase I inhibitory activity, which could provide a starting point for the development of antitumor agents.
Introduction
2,5-diketopiperazines (2,5-DKPs) are important cyclodipeptides (piperazine-2,5-dione core) characterized by the dipeptide core derived from the “head to tail” cyclization of two amino acids (Li et al., 2009; Huang et al., 2014; Ma et al., 2016; Chekan and Moore, 2018). Structurally, these natural compounds usually arise from the oxidative condensation of several isoprene units, tryptophan, and other cyclic amino acid residues such as proline, phenylalanine, tryptophan, histidine, or leucine. Moreover, the prenylated 2,5-DKPs cyclized from tryptophan and proline (Figure 1) possessed a range of interesting structural and stereochemical features that were previously found in a variety of natural products from fungi, bacteria, marine invertebrates, plants, and mammals (Greshock et al., 2008; Li et al., 2009; Huang et al., 2014; Ma et al., 2016). It was reported that marine-derived fungi have been shown to be the rich sources of 2,5-DKP derivatives, in particular Aspergillus and Penicillium spp (Huang et al., 2014; Ma et al., 2016). Significantly, a myriad of biological activities, including antimicrobial, antitumor, antiviral, insecticidal activities, and anthroprotective effects, were displayed by members of this family (Borthwick, 2012; Ma et al., 2016; Borthwick and Costa, 2017; Chen et al., 2018).
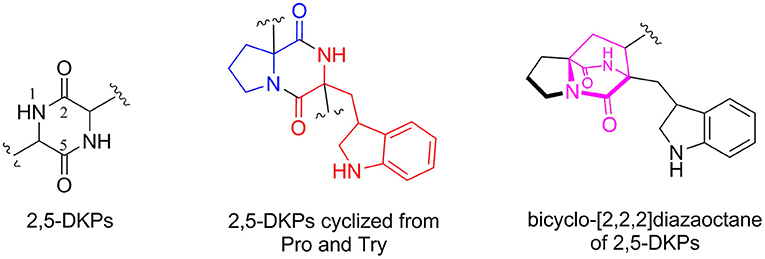
Figure 1. The core structure of 2,5-DKPs and the substructure of common 2,5-DKPs cyclized from Try and Pro.
During our ongoing search for new bioactive secondary metabolites from the marine-derived fungi Aspergillus in the South China Sea (Chen et al., 2014a,b; Liu et al., 2019; Wu et al., 2020; Chao et al., 2021), a sponge-derived fungal strain, A. sclerotiorum GDST-2013-0501, attracted our attention because the extract of the fungal culture showed antibacterial activity. Chemical investigation of the ethyl acetate extract led to the isolation of three new 2,5-DKP derivatives and four known analogs (Figure 2). Herein, we report the isolation, structure elucidation, and biological activities of these compounds.
Materials and Methods
General Experimental Procedure
Optical rotations were measured on a JASCO P-1020 digital polarimeter. UV spectra were recorded on a Beckman DU 640 spectrophotometer. ECD spectra were recorded on a Jasco J-815-150S circular dichroism spectrometer. IR spectra were recorded on a Nicolet-Nexus-470 spectrometer using KBr pellets. 1H, 13C, and 2D NMR spectra were recorded on an Agilent DD2 500 MHz NMR spectrometer (500 MHz for 1H and 125 MHz for 13C), using TMS as an internal standard. The HRESIMS and ESIMS spectra were obtained from a Micromass Q-TOF spectrometer. A semi-preparative HPLC was performed on a Hitachi L-2000 HPLC system coupled with a Hitachi L-2455 photodiode array detector. A Kromasil C18 semi-preparative HPLC column (250 mm × 10 mm, 5 μm) was used. Silica gel (Qing Dao Hai Yang Chemical Group Co.; 200–300 mesh), Sephadex LH-20 (Amersham Biosciences), and octadecylsilyl silica gel (Unicorn; 45–60 μm) were used for column chromatography (CC). Precoated silica gel GF254 plates (Yan Tai Zi Fu Chemical Group Co., Yantai, People's Republic of China) were used for analytical TLC.
Fungal Material
The fungal strain A. sclerotiorum GDST-2013-0501 was isolated from the inner part of an unidentified sponge GDST-2013-05 collected from the South China Sea, in May 2013. The fungal identification was performed by analysis of its morphological characteristics and ITS region of the rDNA. The sequence data (Supplementary Figures S13, S14) were submitted to the Genbank with accession number MT534582. The strain was deposited in the Key Laboratory of Marine Drugs, the Ministry of Education of China, School of Medicine and Pharmacy, Ocean University of China, Qingdao, PR China.
Extraction and Isolation
In total, forty 500 ml Erlenmeyer flasks with the fungal strain were cultivated in rice medium (3.6 g of natural sea salt from Yangkou saltern, China; 70 g of rice; 100 ml of H2O) for 25 days at room temperature. The ethyl acetate extracts were combined and concentrated to dryness under a vacuum to obtain an ethyl acetate extract (30.0 g). This extract was fractionated by silica gel VLC using a step gradient elution with ethyl acetate–petroleum ether (0–100%) and then with methanol–ethyl acetate (0–100%) to afford five fractions (Fr.1–Fr.5). Fr.4 was separated into four subfractions (W1–W4) by silica gel CC eluting with a step gradient of dichloromethane–methanol (50:1, v:v). W4 was further purified on an ODS column eluting with 40–60% methanol–H2O to produce 6 (8.2 mg) and two subfractions, W41 and W42. W41 was further purified by HPLC (20% methanol–H2O) to afford 3 (3.9 mg) and 4 (5.0 mg). W42 was purified by HPLC (20% methanol–H2O) to afford 1 (20.0 mg) and 2 (3.0 mg). W3 was further purified by HPLC (20% acetonitrile–H2O) to afford 5 (10 mg) and 7 (4.0 mg).
Speramide C (1): yellow powder; −29.1 (c 0.10, methanol); UV (methanol) λmax (log ε) 206 (0.74), 236 (0.46) 288 (0.19), 336 (0.13) nm; IR (KBr) νmax 3,904, 2,927, 2,361, 1,650, 1,522, 1,110 cm−1; 1H and 13C NMR data, Table 1; ESIMS m/z 482.2 [M+H]+; HRESIMS m/z 482.2291 [M+H]+ (calcd for C26H32N3O6, 482.2286).
3,21-epi-Taichunamide F (2): yellow powder; −18.3 (c 0.10, methanol); UV (methanol) λmax (log ε) 210 (2.28), 258 (0.85) nm; ECD (1.01 mM, methanol) λmax (Δε) 225 (+41.13), 242 (−8.37), 262 (+9.71), 270 (+6.78), 299 (+7.31), 340 (−3.01) nm; IR (KBr) νmax 3,821, 2,960, 2,361, 1,699, 1,539, 1,160 cm−1; 1H and 13C NMR data, Table 1; ESIMS m/z 494.3 [M+H]+; HRESIMS m/z 494.2281 [M +H]+ (calcd for C27H32N3O6, 494.2286).
2-epi-Amoenamide C (3): yellow powder; −26.4 (c 0.10, methanol); UV (methanol) λmax (log ε) 208 (3.67), 338 (3.23) nm; ECD (1.04 mM, methanol) λmax (Δε) 226 (+37.37), 240 (−19.30), 269 (+26.31), 305 (−4.15), 341 (+13.71) nm; IR (KBr) νmax 3,633, 2,922, 2,362, 1,701, 1,540, 1,383, 1,187 cm−1; 1H and 13C NMR data, Table 1; ESIMS m/z 478.1159 [M+H]+; HRESIMS m/z 478.2332 [M +H]+ (calcd for C27H32N3O5, 478.2336).
Antibacterial Activity Assays
The antibacterial activity was evaluated by the conventional broth dilution assay (Appendino et al., 2008). Three pathogenic bacterial strains, Staphyloccocus aureus, S. epidermidis, and Escherichia coli, were used, and ciprofloxacin was used as a positive control.
DNA Topo I Inhibitory Activity Assay
The Topo I inhibitory activity was measured by assessing the relaxation of supercoiled pBR322 plasmid DNA (Bogurcu et al., 2011; Xin et al., 2017). The gel was stained with GelRed, visualized under UV illumination, and then photographed with a gel imaging system. Camptothecin (CPT) was used as a positive control.
Cytotoxicity Assays
The cytotoxicity against human promyelocytic leukemia HL-60, human erythroleukemia K562 cell lines, and one human normal liver cell line HL7702 (L-02) was evaluated using the MTT method (Mosmann, 1983). The cytotoxicity against human lung carcinoma A549 cell lines was evaluated using the SRB method (Skehan et al., 1990). Adriamycin was used as a positive control.
Acetylcholinesterase Inhibitory Assays
The enzyme acetylcholinesterase (AChE) was from Electrophorus electricus (electric eel). AChE inhibitory activity was evaluated using the method described by Ellman et al. (1961). Galanthamine hydrobromide was used as a positive control.
Results
The Sponge-Derived Fungal Strain A. sclerotiorum GDST-2013-0501
The sponge-derived fungal strain, A. sclerotiorum GDST-2013-0501, was cultured with rice medium in 30 Erlenmeyer flasks at room temperature for 30 days. The fermented rice medium was extracted three times with ethyl acetate. The combined ethyl acetate layers were evaporated under reduced pressure to give the ethyl acetate extract (30 g). By repeating column chromatography (CC) over silica gel, octadecylsilyl (ODS), Sephadex LH-20, and semi-preparative HPLC, seven compounds were obtained, including speramide C (1), 3,21-epi-taichunamide F (2), and 2-epi-amoenamide C (3), together with four known compounds, notoamide F (4) (Tsukamoto et al., 2008), 6-epi-notoamide R (5) (Cai et al., 2013), notoamide G (6) (Tsukamoto et al., 2008), and epi-deoxybrevianamide E (7) (Sobolevskaya et al., 2013) (Figure 2).
Structure Elucidation
Speramide C (1), a yellow amorphous powder, was established to have a molecular formula of C26H31N3O6 by HRESIMS from the [M + H]+ ion at m/z 482.2291 (482.2286, calcd for C26H32N3O6) with 13 degrees of unsaturation. The 1H NMR data of 1 (Table 1) included the resonances of two aromatic protons (δH 6.98, d, J = 8.0 Hz; 6.04, d, J = 8.0 Hz), two olefinic protons (δH 6.85, d, J = 9.5 Hz and 5.55, d, J = 9.5 Hz), and four methyl groups (δH 1.34, s; 1.33, s; 1.30, s and 0.75, s) as well as signals attributable to five methylene and two methine groups. The 13C NMR data (Table 1) displayed 26 carbon resonances, including two amide carbonyl carbons (at δC 166.8 and 166.0), six aromatic carbons (four quaternary), two olefinic carbons, four methyl groups, five methylenes, two methines, and five sp3 quaternary carbons. The 1H and 13C NMR data suggested that compound 1 is a 2,5-DKP, structurally closely related to speramide B, which was previously isolated from the fungus A. ochraceus. Further analyses of the 1H and 13C NMR data (Supplementary Table S1) found that 1 differed from speramide B mainly at the isopentenyl side chain. The difference between these two compounds was that an ethylene oxide group (one methylene group at δC 60.8 and one methine group at δC 91.7) in 1 instead of a double bond (two olefinic carbons at δC 110.8 and 145.6) in speramide B (Supplementary Table S1) (Chang et al., 2016). The ethylene oxide group linked to the isopentenyl side chain (C-23) was assigned by the HMBC correlations from Me-25 to C-2, from Me-26 to C-25, and from H-23 to C-25, along with the COSY correlation of H2-22/H-23 (Figure 3). Therefore, the planar structure of 1 was elucidated.
The relative configurations of C-2, C-3, C-13, and C-19 of 1 were determined by NOESY correlations (Figure 3). The NOESY correlations of 3-OH/19-OH, H-13/H-12a, and Me-25/H-12a revealed these protons were cofacial. However, the relative configuration of C-23 was left unassigned due to the flexibility of the isopentenyl side chain. To define the absolute configurations of C-2, C-3, C-13, and C-19, an ECD calculation was performed at the B3LYP/6-31G(d,p) level using a time-dependent density functional theory (TD–DFT) method by the SpecDis 1.6 program. Finally, the absolute configuration of (2R,3S,13S,19R)-1 was defined on account of the good agreement between the calculated ECD curve and the experimental one (Figure 4).
3,21-Epi-taichunamide F (2), obtained as a yellow powder, was found to have the chemical formula C27H31N3O6, which indicates 14 degrees of unsaturation. The 1H NMR data of 2 (Table 1) displayed the typical pattern of a prenylated indole alkaloid skeleton with the presence of one 1,2,3,4-tetrasubstituted benzene unit (H-4, δH 7.36, J = 8.0 Hz; H-5, δH 6.88, J = 8.0 Hz), one Z-configured double bond (H-9, δH 7.76, d, J = 10.2 Hz and H-8, δH 5.93, d, J = 10.2 Hz), four methyl groups (δH 1.15, 1.30, 1.40, and 1.42), one methoxy group (δH 3.31), and four sp3-hybridized methylene groups. Detailed analysis of the 1D, 2D NMR (Table 1 and Figure 5), and MS data revealed that the planar structure of 2 is the same as that of the prenylated 2,5-DKP taichunamide F, which was previously isolated from the fungus A. taichungensis (Kagiyama et al., 2016). The most obvious differences between 2 and taichunamide F were the 1H and 13C NMR chemical shifts of 2, 12, and 23-positions (Supplemtary Table S2), indicating that their absolute configurations of them might be different.
The stereochemistry of 2 was established by NOESY experiments in conjunction with the ECD spectra and ECD calculations. The relative configuration of 2 was elucidated by a NOESY experiment (Figure 5). The NOESY correlations of 21-NH/H-23, H-23/Me-26, Me-26/3-OH, and 3-OH/12-OMe along with the interaction between H-12 and Me-25 indicated the α-orientation of 21-NH, H-23, and 3-OH, and β-orientation of H-12. The absolute configurations of the bridgehead carbons C-13 and C-19 of 2 were established by a CD exciton chirality method as reported by Williams et al. (1989). The Cotton effects (CEs) at 200–250 nm, which arises from an n–π* transition of the amide bonds, is a reliable diagnostic method to discern the absolute stereochemistry of bicyclo[2.2.2]diazaoctane diketopiperazine (Herscheid et al., 1979; Williams et al., 1989; Kato et al., 2007). The closely resembled CEs at 200−250 nm of compound 2 (Figure 6) (positive CEs: Δε +41.13 at 225 nm and negative CEs: Δε −8.37 at 242 nm) to taichunamide F indicated that the absolute configurations of bicyclo[2.2.2]-diazaoctane of 2 were 13R,19S. According to the relative configurations elucidated earlier, the absolute configuration of 2 was ascertained as 3S, 12R, 13R, 19S, and 23S, which was different from the reported taichunamide F. To further confirm the absolute configuration of 2, the calculated ECD was performed using TD–DFT at the B3LYP/6-311+G (d, p) level in methanol by the CPCM polarizable conductor calculation model. Conformational searches were carried out using the Merck Molecular Force Field (MMFF) by means of the Spartan's 10 software to acquire meaningful conformers for 3S,12R,13R,19S,23S-2. The theoretical ECD spectrum for 3R,12S,13S,19R, and 23R-2 was obtained by directly reversing the spectrum of 3S, 12R, 13R, 19S, and 23S-2. The calculated curve of 3S, 12R, 13R, 19S, and 23S-2 matched with its experimental ECD spectrum (Figure 6). As a result, we confirmed that 2 is the C-3 epimer of taichunamide F, and the absolute configuration of 2 is 3S, 12R, 13R, 19S, and 23S.
2-epi-Amoenamide C (3) was also obtained as a yellow powder and its molecular formula was determined to be C27H31N3O5 based on HRESIMS, requiring an index of hydrogen deficiency of 14. The 1H NMR data of 3 (Table 1) displayed two aromatic protons (δH 6.32, H-5, d, J = 8.3 Hz; 7.28, H-4, d, J = 8.3 Hz) and typical signals of one cis-1,2-disubstituted double bond (δH 7.25, H-9, d, J = 10.0 Hz; 5.77, H-8, d, J = 10.0 Hz). Detailed analysis of 1H NMR data indicated that 3 also belongs to the prenylated indole alkaloid. The 13C NMR (Table 1) along with its HSQC spectrum of 3 exhibited 27 carbon resonances, including three carbonyls (one ketocarbonyl carbon at δC 197.1 and two amide carbonyl carbons at δC 172.1 and 167.9) corresponding with νmax 1,701 cm−1 in the IR spectrum, six aromatic carbons, two olefinic carbons, one methoxy group, four methyl groups, four methylene groups, two methine carbons, and five sp3 quaternary carbons. Further analyses of the 1H–1H COSY and HMBC spectra of 3 confirmed the presence of a bicyclo[2.2.2]diazaoctane core including a proline (Figure 5). Moreover, these spectroscopic features of 3 were very similar to those of amoenamide C, previously isolated from the fungus Fusarium sambucinum (Zhang et al., 2019). The most obvious differences in 1H and 13C NMR spectra between 3 and aminoamide C were the chemical shifts of C-2, C-12, and C-23-positions (Supplementary Table S3), indicating that the absolute configurations of them might be different. The aforementioned data, as well as the HMBC correlations from H-12 to C-14 and C-23, from H-23 to C-2 and C-14, from NH-1 to C-24, and from H-4 to C-3 and C-9b (Figure 5), indicated the presence of an aza-spiro structure fused to the bicyclo-[2,2,2]diazaoctane system via C-13 and C-23. Therefore, the planar structure of 3 was elucidated.
The relative configuration of 3 was ascertained by the examination of its NOESY spectrum (Figure 5). The obvious NOESY correlations between 21-NH/H-23, H-23/Me-26, and 21-NH/1-NH suggested that these protons should be cofacial and α-oriented, which established the relative configurations of C-23 and the spiro-carbon C-2. Correspondingly, the NOESY correlations between Me-25/H-12 indicated that these protons should be β-oriented. Thus, the relative configurations of 3 were determined, which differed from those of aminoamide C.
In the experimental ECD spectrum of 3, the positive CEs at 226 nm (Δε +37.37) and negative CEs at 240 nm (Δε −19.30) (Figure 7) compared well with the relevant regions in that of (+)-brevianamide B (Williams et al., 1989). These results combined the relative configurations allowed the assignment of the absolute configuration of 3 as 2S, 12S, 13R, 19S, and 23S, which was a C-2 epimer of the reported aminoamide C (Zhang et al., 2019). This result was further confirmed by ECD calculation with the calculated ECD of 2S, 12S, 13R, 19S, and 23S-3 matched with that of the experimental spectrum (Figure 7).
The known compounds notoamide F (4) (Tsukamoto et al., 2008), 6-epi-notoamide R (5) (Cai et al., 2013), notoamide G (6) (Tsukamoto et al., 2008), and epi-deoxybrevianamide E (7) (Sobolevskaya et al., 2013) were identified by the comparison of their spectroscopic data, ECD and specific optical rotation data with those in the literature.
Bioassays of Compounds
All of the isolated compounds were subjected to a panel of bioassays to evaluate their potential activities. These included evaluation of antibacterial activity toward Staphyloccocus aureus, S. epidermidis, and Escherichia coli, cytotoxic activity against three human tumor cell lines, including human promyelocytic leukemia HL-60, human lung carcinoma A-549, chronic leukemia K562 cell lines and one human normal liver cell line HL7702 (L-02), DNA topoisomerase I (Topo I), and acetylcholinesterase (AChE) inhibitory activities. Compound 4 displayed moderate Topo I inhibitory activity with the MIC value of 100.0 μM (the positive control CPT with the MIC value of 40.0 μM) (Supplementary Figure S26), whereas 4 showed no significant cytotoxic activity against all of the human tumor cell lines and even the normal cell lines. The aforementioned results suggested that the low cytotoxic or non-cytotoxic compound 4 might possess Topo I inhibitory activity and deserves to be further studied for the rational drug design of antitumor agents. Besides, 4 also showed moderate antibacterial activity against S. epidermidis with the MIC value of 12.5 μM (the positive control ciprofloxacin with the MIC value of 3.13 μM). These data suggest that the antibacterial activity of 4 against S. epidermidis may be mediated through Topo I inhibition, which should be further confirmed by testing for bacterial DNA topoisomerase I activity.
Conclusion
The 2,5-DKP derivatives cyclized from tryptophan and proline could mostly be classified as prenylated indole alkaloids possessing a simple piperazine-2,5-dione core or a complicated bicyclo[2.2.2]diazaoctane ring and several isoprene units (Greshock et al., 2008). The present study revealed that the new compound 1 and the known analog 7 belong to prenylated indole alkaloids, possessing a simple piperazine-2,5-dione core and one or two isoprene units, while the new compounds 2 and 3 along with three known analogs (4–6) belong to the substructure type of bicyclo[2.2.2]diazaoctane ring-containing prenylated indole alkaloids, which usually have two or three isoprene units (Borthwick and Costa, 2017). Compound 1 is the first reported prenylated indole alkaloid with an ethylene oxide ring at the isopentenyl side chain.
Data Availability Statement
The original contributions presented in the study are included in the article/Supplementary Material, further inquiries can be directed to the corresponding author/s.
Author Contributions
C-YuW and C-LS participated in conceiving the idea and revising the manuscript. C-YiW contributed to the fermentation, extraction, isolation, and manuscript preparation. X-HL, Y-YZ, X-YN, and Y-HZ contributed to the bioactivity test. C-YiW, X-MF, and XL contributed to the data analysis, writing, revising, and proofreading of the manuscript. All the authors read and approved the final version of the manuscript.
Funding
This work was supported by the National Key Research and Development Program of China (No. 2018YFC0310900); the National Natural Science Foundation of China (Nos. 41830535 and U1706210); the Program of Open Studio for Druggability Research of Marine Natural Products, Pilot National Laboratory for Marine Science and Technology (Qingdao, China) directed by Kai-Xian Chen and Yue-Wei Guo; and the Taishan Scholars Program, China.
Conflict of Interest
The authors declare that the research was conducted in the absence of any commercial or financial relationships that could be construed as a potential conflict of interest.
Publisher's Note
All claims expressed in this article are solely those of the authors and do not necessarily represent those of their affiliated organizations, or those of the publisher, the editors and the reviewers. Any product that may be evaluated in this article, or claim that may be made by its manufacturer, is not guaranteed or endorsed by the publisher.
Supplementary Material
The Supplementary Material for this article can be found online at: https://www.frontiersin.org/articles/10.3389/fmicb.2022.808532/full#supplementary-material
1H NMR, 13C NMR, HMQC, COSY, HMBC, NOESY, and MS spectra of 1, 2, and 3; ECD calculation details of 1 and 2; and DNA Topo I inhibitory activity of the isolated compounds.
References
Appendino, G., Gibbons, S., Giana, A., Pagani, A., Grassi, G., Stavri, M., et al. (2008). Antibacterial cannabinoids from Cannabis sativa: a structure-activity study. J. Nat. Prod. 71, 1427–1430. doi: 10.1021/np8002673
Bogurcu, N., Sevimli-Gur, C., Ozmen, B., Bedir, E., and Korkmaz, K. S. (2011). ALCAPs induce mitochondrial apoptosis and activate DNA damage response by generating ROS and inhibiting topoisomerase I enzyme activity in K562 leukemia cell line. Biochem. Biophys. Res. Commun. 409, 738–744. doi: 10.1016/j.bbrc.2011.05.078
Borthwick, A. D. (2012). 2,5-Diketopiperazines: synthesis, reactions, medicinal chemistry, and bioactive natural products. Chem. Rev. 112, 3641–3716. doi: 10.1021/cr200398y
Borthwick, A. D., and Costa, N. C. D. (2017). 2,5-diketopiperazines in food and beverages: taste and bioactivity. Crit. Rev. Food Sci. 577, 18–742. doi: 10.1080/10408398.2014.911142
Cai, S. X., Luan, Y. P., Kong, X. L., Zhu, T. J., Gu, Q. Q., and Li, D. H. (2013). Isolation and photoinduced conversion of 6-epi-Stephacidins from Aspergillus taichungensis. Org. Lett. 15, 2168–2171. doi: 10.1021/ol400694h
Chang, Y. W., Yuan, C. M., Zhang, J., Liu, S., Cao, P., Hua, H. M., et al. (2016). Speramides A–B, two new prenylated indole alkaloids from the freshwater-derived fungus Aspergillus ochraceus KM007. Tetrahedron Lett. 57, 4952–4955. doi: 10.1016/j.tetlet.2016.09.071
Chao, R., Hou, X. M., Xu, W. F., Hai, Y., Wei, M. Y., Wang, C. Y., et al. (2021). Targeted isolation of asperheptatides from a coral-derived fungus using LC-MS/MS-based molecular networking and antitubercular activities of modified cinnamate derivatives. J. Nat. Prod. 84, 11–19. doi: 10.1021/acs.jnatprod.0c00804
Chekan, J. R., and Moore, B. S. (2018). Biosynthesis of the antibiotic bicyclomycin in soil and pathogenic bacteria. Biochemistry. 57, 897–898. doi: 10.1021/acs.biochem.7b01204
Chen, C., Ye, Y., Wang, R., Zhang, Y., Wu, C., Debnath, S. C., et al. (2018). Streptomyces nigra sp. nov. is a novel actinobacterium isolated from mangrove soil and exerts a potent antitumor activity in vitro. Front. Microbiol. 9, 1587. doi: 10.3389/fmicb.2018.01587
Chen, M., Shao, C. L., Fu, X. M., Kong, C. J., She, Z. G., and Wang, C. Y. (2014b). Bioactive indole alkaloids and phenyl ether derivatives from a marine-derived Aspergillus sp. Fungus.J. Nat. Prod. 77, 1601–1606.
Chen, M., Shao, C. L., Meng, H., She, Z. G., and Wang, C. Y. (2014a). Bioactive indole alkaloids and phenyl ether derivatives from a marine-derived Aspergillus sp. Fungus. J. Nat. Prod. 77, 2720–2724. doi: 10.1021/np500650t
Ellman, G. L., Courtney, K. D., Andres, V. Jr, and Featherstone, R. M. (1961). A new and rapid colorimetric determination of acetylcholinesterase activity. Biochem. Pharmacol. 7, 88–95. doi: 10.1016/0006-2952(61)90145-9
Greshock, T. J., Grubbs, A. W., Jiao, P., Wicklow, T. D., Gloer, J. B., and Williams, R. M. (2008). Isolation, structure elucidation, and biomimetic total synthesis of versicolamide B, and the isolation of antipodal (–)-Stephacidin A and (+)-Notoamide B from Aspergillus versicolor NRRL 35600. Angew. Chem. Int. Ed. 47, 3573–3577. doi: 10.1002/anie.200800106
Herscheid, J. D. M., Tijhuis, M. W., Noordik, J. H., and Ottenheijm, H. C. J. (1979). Desulfurization of epidithiodioxopiperazines. A mechanistic and chiroptical study. J. Am. Chem. Soc. 101, 1159–1162. doi: 10.1021/ja00499a018
Huang, R. M., Yi, X. X., Zhou, Y. Y., Su, X. D., Peng, Y., and Gao, C. H. (2014). An update on 2,5-diketopiperazines from marine organisms. Mar. Drugs. 12, 6213–6235. doi: 10.3390/md12126213
Kagiyama, I., Kato, H., Nehira, T., Frisvad, J. C., Sherman, D. H., Williams, R. M., et al. (2016). Taichunamides: Prenylated indole alkaloids from Aspergillus taichungensis (IBT 19404). Angew. Chem. Int. Ed. 55, 1128–1132. doi: 10.1002/anie.201509462
Kato, H., Yoshida, T., Tokue, T., Nojiri, Y., Hirata, H., Ohta, T., et al. (2007). Notoamides A–D: Prenylated indole alkaloids isolated from a marine-derived fungus, Aspergillus sp. Angew. Chem. Int. Ed. 46, 2254–2256. doi: 10.1002/anie.200604381
Li, G. Y., Yang, T., Luo, Y. G., Chen, X. Z., Fang, D. M., and Zhang, G. L. (2009). Brevianamide J, a new indole alkaloid dimer from fungus Aspergillus versicolor. Org. Lett. 11, 3714–3717. doi: 10.1021/ol901304y
Liu, L., Zheng, Y. Y., Shao, C. L., and Wang, C. Y. (2019). Metabolites from marine invertebrates and their symbiotic microorganisms: molecular diversity discovery, mining, and application. Mar. Life Sci. Tech. 1, 60–94. doi: 10.1007/s42995-019-00021-2
Ma, Y. M., Liang, X. A., Kong, Y., and Jia, B. (2016). Structural diversity and biological activities of indole diketopiperazine alkaloids from fungi. J. Agric. Food Chem. 64, 6659–6671. doi: 10.1021/acs.jafc.6b01772
Mosmann, T. (1983). Rapid colorimetric assay for cellular growth and survival: Application to proliferation and cytotoxicity assays. J. Immunol. Meth. 65, 55–63. doi: 10.1016/0022-1759(83)90303-4
Skehan, P., Storeng, R., Scudiero, D., Monks, A., McMahon, J., Vistica, D., et al. (1990). New colorimetric cytotoxicity assay for anticancer-drug screening. J. Natl. Cancer Inst. 82, 1107–1112. doi: 10.1093/jnci/82.13.1107
Sobolevskaya, M. P., Afiyatullov, S. S., Dyshlovoi, S. A., Kirichuk, N. N., Denisenko, V. A., Kim, N. Y., et al. (2013). Metabolites from the marine isolate of the fungus Aspergillus versicolor KMM 4644. Chem. Nat. Compd+. 49, 181–183. doi: 10.1007/s10600-013-0552-x
Tsukamoto, S., Kato, H., Samizo, M., Nojiri, Y., Onuki, H., Hirota, H., et al. (2008). Notoamides F-K, prenylated indole alkaloids isolated from a marine-derived Aspergillus sp. J. Nat. Prod. 71, 2064–2067. doi: 10.1021/np800471y
Williams, R. M., Kwast, E., Coffman, H., and Glinka, T. (1989). Remarkable, enantiodivergent biogenesis of brevianamide A and B. J. Am. Chem. Soc. 111, 3064–3065. doi: 10.1021/ja00190a050
Wu, J. S., Yao, G. S., Shi, X. H., Ur Rehman, S., Xu, Y., Fu, X. M., et al. (2020). Epigenetic agents trigger the production of bioactive nucleoside derivatives and bisabolane sesquiterpenes from the marine-derived fungus Aspergillus versicolor. Front. Microbiol. 11, 85. doi: 10.3389/fmicb.2020.00085
Xin, L. T., Liu, L., Shao, C. L., Yu, R. L., Chen, F. L., Yue, S. J., et al. (2017). Discovery of DNA topoisomerase I inhibitors with low-cytotoxicity based on virtual screening from natural products. Mar. Drugs 15, 217–225. doi: 10.3390/md15070217
Keywords: Aspergillus sclerotiorum, 2, 5-diketopiperazines, sponge-derived fungus, bicyclo[2.2.2]diazaoctane, DNA topoisomerase I
Citation: Wang C-Y, Liu X-H, Zheng Y-Y, Ning X-Y, Zhang Y-H, Fu X-M, Li X, Shao C-L and Wang C-Y (2022) 2,5-Diketopiperazines From a Sponge-Derived Fungus Aspergillus sclerotiorum. Front. Microbiol. 13:808532. doi: 10.3389/fmicb.2022.808532
Received: 12 November 2021; Accepted: 15 February 2022;
Published: 20 May 2022.
Edited by:
Xiaoke Hu, Yantai Institute of Coastal Zone Research (CAS), ChinaReviewed by:
Sunil Kumar Deshmukh, The Energy and Resources Institute (TERI), IndiaYihua Chen, Institute of Microbiology (CAS), China
Xiaowei Luo, Guangxi University of Chinese Medicine, China
Yonghong Liu, South China Sea Institute of Oceanology (CAS), China
Copyright © 2022 Wang, Liu, Zheng, Ning, Zhang, Fu, Li, Shao and Wang. This is an open-access article distributed under the terms of the Creative Commons Attribution License (CC BY). The use, distribution or reproduction in other forums is permitted, provided the original author(s) and the copyright owner(s) are credited and that the original publication in this journal is cited, in accordance with accepted academic practice. No use, distribution or reproduction is permitted which does not comply with these terms.
*Correspondence: Chang-Lun Shao, c2hhb2NoYW5nbHVuQG91Yy5lZHUuY24=; Chang-Yun Wang, Y2hhbmd5dW5Ab3VjLmVkdS5jbg==