- 1Department of Microbiology, College of Resources, Sichuan Agricultural University, Chengdu, China
- 2Soil and Fertilizer Institute, Sichuan Academy of Agricultural Sciences, Chengdu, China
- 3Ecosystems and Environment Research Programme, University of Helsinki, Helsinki, Finland
Truffles (Tuber spp.) are edible ectomycorrhizal fungi with high economic value. Bacteria in ectomycorrhizosphere soils are considered to be associated with the nutrient uptake of truffles and hosts. Whether Tuber spp. inoculation can affect the growth of Quercus aliena, the ectomycorrhizosphere soil, and the rhizosphere nirK and nirS-denitrifier communities at the ectomycorrhizae formation stage is still unclear. Therefore, we inoculated Q. aliena with the black truffles Tuber melanosporum and Tuber indicum, determined the physiological activity and morphological indices of Q. aliena seedlings, analyzed the physicochemical properties of ectomycorrhizosphere soils, and applied DNA sequencing to assess the nirK and nirS- denitrifier community structure in ectomycorrhizosphere soils. Peroxidase activity was higher in the seedlings inoculated with T. melanosporum than in the T. indicum inoculation and uninoculated control treatments. The available phosphorus contents were lower and nitrate contents were higher in those with truffle inoculation, and T. melanosporum treatment differed more from the control than the T. indicum treatment. The richness of the nirK-community was highest in the T. indicum treatment and lowest in the uninoculated treatment. The differences in nirK-community composition across treatments were not statistically significant, but the nirS communities were different. The nirS-type bacteria correlated with three environmental factors (pH, available phosphorus, and nitrate contents), whereas the nirK-type bacteria were only associated with the nitrate contents. Generally, this work revealed that inoculation with Tuber spp. would change a few nutrient contents and richness of nirK-type bacteria and had little effects on growth of Q. aliena seedlings in the initial stage of inoculation. The results of this study may provide in-depth insights into the relationships between Tuber spp. and hosts, which should be taken into account when developing truffle production methods.
Introduction
Tuber spp., commonly known as truffles, belong to the family Tuberaceae in the order Pezizales, phylum Ascomycota. The truffle fruiting bodies are valuable because of their specific aroma and are expensive delicacies due to the decreasing yields of wild truffles and unpredictability of cultivation (Allen and Bennett, 2021). Tuber spp. coexist and interact with their host plants through the formation of ectomycorrhizae. The major host plants of Tuber spp. include various Corylaceae species, among them, Pinus and Quercus (Geng et al., 2009; Garcia-Barreda et al., 2015; Wan et al., 2016). The growth and development of truffles and their hosts are closely associated as the truffles form a mass of mycelia in the soil surrounding the host plant roots (Suz et al., 2010a). Ectomycorrhizal fungi may improve the growth of their hosts in oligotrophic environments and under drought (Alvarez et al., 2010), such as increasing average plant height, ground diameter growth, and disease resistance (Liese et al., 2017). Quercus aliena Blume is an oak specie native to East Asia, including southwest China, which is a truffle producing region (Li et al., 2014). Q. aliena with high adaptability to dynamic environment is a suitable host species for Tuber spp. (Li et al., 2018). However, there is little information on how Tuber species affect the growth of Q. aliena.
Bacteria in ectomycorrhizosphere soils were considered to be associated with the mycelium colonization and the formation of volatile aroma compounds of truffle (Splivallo et al., 2015). The formation of ectomycorrhizae is accompanied by changes in soil microbial communities and soil properties (García-Montero et al., 2006; Barbieri et al., 2007). For example, inoculation with Tuber spp. changed the nitrogen content in ectomycorrhizosphere (Li et al., 2018; Yang et al., 2019), possibly due to the enrichment of nitrogen cycle-related bacteria in the truffle ascocarps (Barbieri et al., 2010; Vahdatzadeh et al., 2015; Monaco et al., 2021), ectomycorrhizae, and mycorrhizosphere (Beatrice et al., 2012; Le-Roux et al., 2016). Denitrification is a key nitrogen-transforming process which results in nitrogen loss in soil (Bárta et al., 2017). The taxonomic composition of denitrifying bacteria, denitrifiers correlated with the content of ammonia-nitrogen (NH4+-N) and nitrate-nitrogen (NO3–-N) that can be directly absorbed by Tuber mycelia (Montanini et al., 2002) and its hosts (Marcel et al., 2018). Pseudomonas, Bradyrhizobium, and Ensifer were the predominant bacterial genera associated with truffle (Barbieri et al., 2007; Benucci and Bonito, 2016; Deveau et al., 2016). And these genera include many denitrifiers such as Pseudomonas aeruginosa (Zhang et al., 2020), Ensifer meliloti (Chan and Mccormick, 2004; Torres et al., 2014, 2018), Bradyrhizobium japonicum (Mesa et al., 2010), Pseudomonas stutzeri (Wittorf et al., 2018), and Bradyrhizobium oligotrophicum (Cristina and Kiwamu, 2011). These carry either of the nitrite reductase genes nirK and nirS, which previous studies have indicated to be widely distributed in Tuber spp. ectomycorrhizosphere soils.
The community structure of nirK- and nirS- denitrifiers is affected by environmental factors, including availability of carbon sources and nitrate concentration (Azziz et al., 2017). However, whether the specific ectomycorrhizosphere soil properties (García-Montero et al., 2006; Barbieri et al., 2007; Li et al., 2018) reregulate the structure of nirK- and nirS-carrying bacterial communities is still unclear. In addition, the effects of the Tuber spp. inoculation on the primary nutrient in ectomycorrhizosphere soil and growth of hosts during ectomycorrhizae formation stage remain to be assessed. Therefore, we inoculated Q. aliena with the black truffles Tuber melanosporum Vittad. and Tuber indicum Vittad., determined the physiological activity and morphological indices of Q. aliena seedlings, analyzed the physicochemical properties of ectomycorrhizosphere soils, and applied DNA sequencing to assess the nirK and nirS- denitrifier community structure in ectomycorrhizosphere soils. Our aims were to define the effect of Tuber spp. inoculation on the growth of Q. aliena seedlings in the initial stage of ectomycorrhizae formation, and to elucidate the environmental factors affecting the denitrifier community structure in ectomycorrhizosphere soils.
Materials and Methods
Cultivation of Quercus aliena Seedlings
Tuber melanosporum were from France and T. indicum were from Huidong, China. Q. aliena seeds were obtained from Yunnan Academy of Forestry Sciences, China. Seeds were soaked in fresh water for approximately 20 h, sterilized in 0.3% potassium permanganate for 30 min, and rinsed with distilled water until the rinse water became colorless. River sand was sterilized for 90 min at 121°C and the seeds were germinated in damp sand for 1 month. The germinated seeds were sown in pots filled with a sterilized mixture of vermiculite, perlite, and water at a ratio of 1:1:1 (v/v/v). The pots were placed in a plastic greenhouse with a daytime temperature of 23–25°C and a night-time temperature of 16–20°C and watered with sterile water to maintain soil moisture at 25%. After 1 month, the Q. aliena seedlings were transplanted into nursery pots full with sterilized mixture. The truffles inoculum and inoculation were done as described previously (Kang et al., 2020). The seedlings were inoculated with T. melanosporum (mel.ali) or T. indicum (ind.ali), and uninoculated Q. aliena seedlings served as a control treatment (CK.ali). Sixty seedlings per treatment were grown in a greenhouse for 180 days. The moisture content in the substrate was maintained at about 50% by watering with tap water every 2–3 days. In the greenhouse, the average temperature was 22.2°C (10.7–35.6°C) and the average humidity was 78.8% (45–100%) from March to July, and 24.0°C (12.7–38.7°C) and 82.07% (39–100%) from July to November.
Morphological Identification and Morpho-Anatomy of Ectomycorrhizae and Sample Collection
Six seedlings per treatment were randomly sampled 6 months after inoculation. Three seedlings were used for ectomycorrhizal identification, and the other three were used to analyze the development of seedlings. Soil was removed from the rootlets by gentle shaking, and the bulk soil was collected to determine soil properties. The soil still adhering to the roots (<3 mm thick soil) were scraped with sterile tweezers and collected as the rhizosphere soil. Approximately 3.0 g of rhizosphere soil per seedling was stored at -80°C for microbial analysis. The roots of seedlings were rinsed with water and observed under microscope. The morpho-anatomy of the ectomycorrhizae were characterized by fixing with paraffin, followed by transverse and longitudinal slicing into 3 μm thick sections using a revolving slicer (Leica, RM2016, Wetzlar, Germany). The sections were stained with fast green for 3 min and safranin O for 0.5–2 min. The images of cross and longitudinal sections were captured using a Pannoramic 250 fast length adjustment of short reads (FLASH) digital section scanner (3DHIESTECH, Budapest, Hungary).
Determining Physiological Indices and Soil Properties
To measure the growth of the Q. aliena seedlings, the shoot height, stem diameter, root weight, crown weight, root dry weight, and crown dry weight of the seedlings were determined (Supplementary Table 1). Seedling index and root crown ratio (Moore et al., 2002) were calculated using Equations 1 and 2:
Young roots (0–5mm in diameter and 0–10cm from apical) from three seedlings per treatment were sampled for measuring physiological indices. Root activity was measured using triphenyltetrazolium chloride (TTC) staining (Zhang et al., 2013), superoxide dismutase (SOD) activity was measured using the NBT method (Fridovich, 2011), and peroxidase (POD) activity was determined using the guaiacol colorimetric method (Meloni et al., 2003).
Total nitrogen (TN), nitrate-nitrogen (NO3–-N), ammonium-nitrogen (NH4+-N), available phosphorus (AP), available potassium (AK), and organic matter (OM) contents, and pH of the soil were measured as described previously (Hill et al., 2003).
DNA Extraction and Sequencing
Deoxyribonucleic acid was extracted from three replicate 1.0 g rhizosphere soil samples per treatment using the FastDNA Spin Kit (MP Biomedicals, Santa Ana, CA, United States). The quality of extracted DNA was analyzed using gel electrophoresis. The DNA extracts were stored at −20°C.
The nirS and nirK fragments were amplified using primers nirKF (5′-TCATGGTGCTGCCGCGY- GANGG-3′), nirKR (5′-GAACTTGCCGGTKGCCCAGAC-3′) (Yan et al., 2003), nirSF (5′-TT- CRTCAAGACSCAYCCGAA-3′), and nirSR (5′-CGTTGAACTTRCCGG-3′) (Hill et al., 2003). The 20 μl PCR reactions contained 0.5 μl primer, 10 μl SYBR Green PCR master mix (Power SYBRR Green PCR master mix, Applied Biosystems, United States), and 2 μl of 5 ng μl–1 template DNA. The PCR procedure included an initial denaturation at 94°C for 4 min, 30 cycles of 15 s at 94°C, 15 s at 55°C, and 30 s at 72°C, and a final extension at 72°C for 10 min (Bárta et al., 2010). Amplified fragments in 2 μl of the PCR reaction were separated by electrophoresis in a 1.5% agarose gels that were extracted using an Axygen DNA Gel Extraction Kit (Axygen Inc., Corning, NY, United States), and quantified using a Quant-iT PicoGreen dsDNA Assay Kit and a FLx800 microplate reader (BioTek, Vermont, United States). Sequencing libraries were prepared using a TruSeq Nano DNA LT Library Prep Kit and quantified using a Promega QuantiFluor fluorescence quantification system (Promega Corporation, Madison, WI, United States). The libraries were sequenced using an Illumina MiSeq sequencer (Personalbio, Shanghai, China) and 2 × 300 bp paired-end reads were generated.
Sequence Analysis
Paired-end reads were merged using FLASH (Mago and Salzberg, 2011) and quality filtered using Quantitative Insights Into Microbial Ecology (QIIME) and Trimmomatic (Caporaso et al., 2010). The sequences were assigned to operational taxonomic units (OTUs) at 97% similarity level using Uparse software. The 30,800-73,109 nirK sequence reads per sample were classified into 1,734-3,125 OTUs per sample, and the 56,427-81,655 nirS gene sequence reads into 585-1145 OTUs (Supplementary Figure 1). Rarefaction curves were generated using QIIME (Supplementary Figure 2). The alpha diversity was evaluated by calculating five indices using QIIME, including Chao1, Shannon, Simpson ACE, and Observed species. The OTUs were assigned to taxa using the Ribosomal Database Project Classifier1 (Wang et al., 2007) based on the functional gene pipeline & repository database (Release7.3).2 The taxonomic composition was visualized using R v 3.5.0.3 The sequence data were submitted to the NCBI Sequence Read Archive (SRA) database with the accession numbers SRX6578870–SRX6578878.
Statistical Analysis
The normality of data were tested using the Shapiro-Wilk test (Supplementary Figure 3) and one-way ANOVA with Duncan’s multiple range test. Least significant difference (LSD) tests were done using Statistical Package for the Social Sciences (SPSS) version 10.0 for Windows (IBM SPSS Inc., Chicago, IL, United States). Differences were considered statistically significant at p < 0.05. After removal of singleton and unidentified OTUs, the multivariate homogeneity of dispersions was tested with 999 permutations in the R package vegan v2.5-7 in R 4.1.2 (Oksanen et al., 2020). Differences in beta-diversity were tested using permutational multivariate analysis of variance (PERMANOVA) and pairwise PERMANOVA in the R packages vegan and pairwise Adonis (Anderson, 2006) (Supplementary Figure 4). Weighted UniFrac based beta-diversity was visualized using non-metric multidimensional scaling (NMDS). We standardized soil properties and nirK- and nirS-bacterial genera using z-score, and the soil properties associated with the variation in nirK and nirS communities were analyzed using redundancy analysis (RDA) in Canoco V 4.5 (Center for Biometry, Wageningen, Netherlands).
Results
The Characteristics of Ectomycorrhizae, Seedlings, and Soil
Inoculation with T. melanosporum and T. indicum resulted in typical ectomycorrhizae on the Q. aliena seedlings (Figure 1). Two ectomycorrhizae were monopodial or binary branched and were yellowish-brown, and some ectomycorrhizae formed abundant transparent mycelium. The outer green cells in images of cross (Figures 2A,C) and longitudinal (Figures 2D,F) were the outer mantle cells, the root without Tuber spp. inoculation had no mantle cell (Figures 2B,E). Closer examination revealed uneven puzzle-like patterns on the ectomycorrhizal tips (Figures 2G,I), which were different from rectangle cell in root tips (Figure 2H). The average seedling index ranged from 7.96 to 9.48, and the root crown ratio ranged from 2.58 to 3.46 (Table 1). The SOD activity and root activity ranged from 3.23 to 4.12 U(gh)–1 FW and 21.20 to 25.13 μg TTF(gh)–1. The seedling index, root crown ratio, SOD activity, and root activity were on the same level in three treatments. The POD activity was higher in the T. melanosporum inoculated seedlings than in the not inoculated and T. indicum inoculated seedlings (p < 0.05). However, the ind.ali did not differ significantly in POD activity from CK.ali.
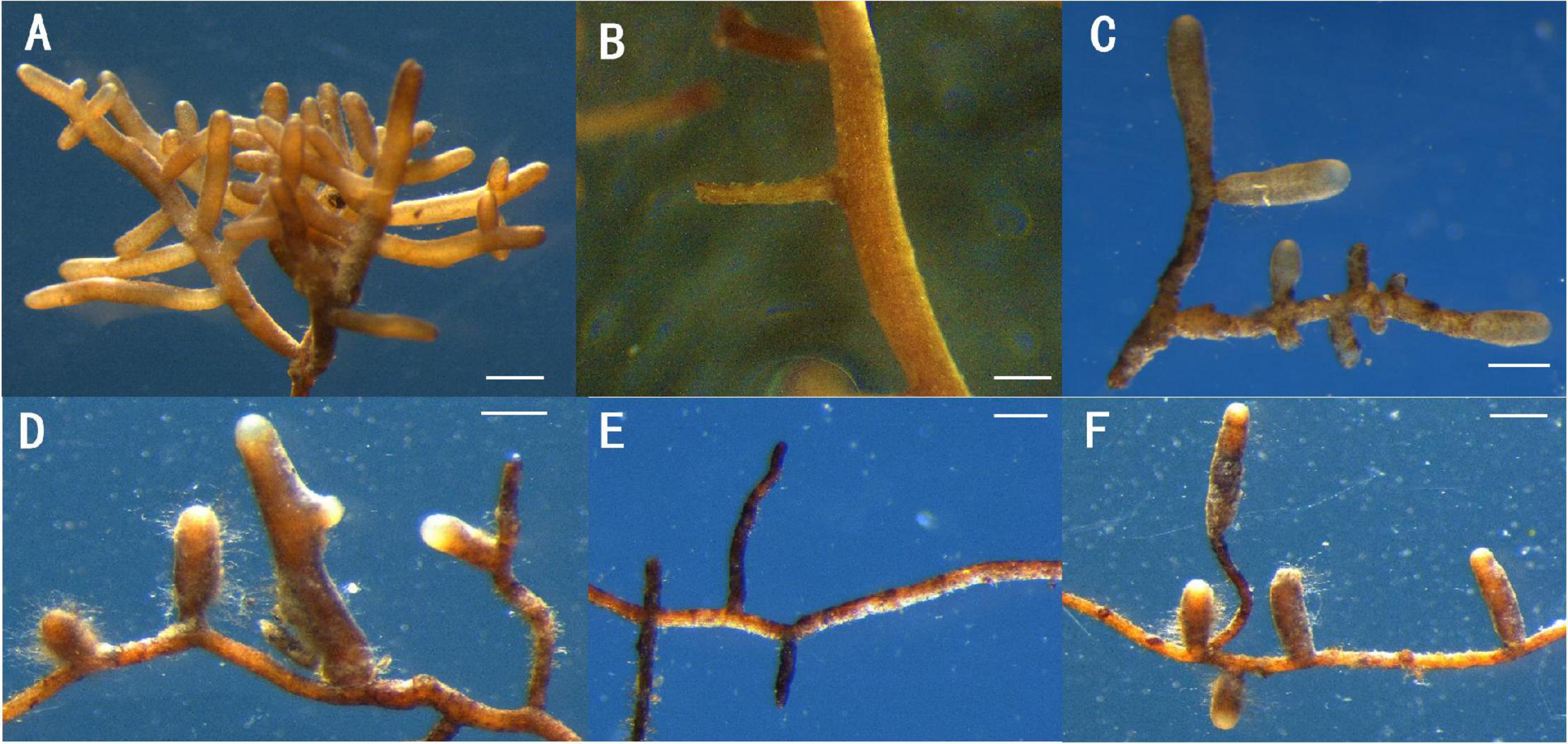
Figure 1. Two ectomycorrhizae and roots of Quercus aliena without Tuber species partner, Scale bar = 1 mm. (A,D) Ectomycorrhizae of Q. aliena seedlings with Tuber indicum; (B,E) root of Q. aliena without Tuber partner; (C,F) Ectomycorrhizae of Q. aliena seedling with Tuber melanosporum.
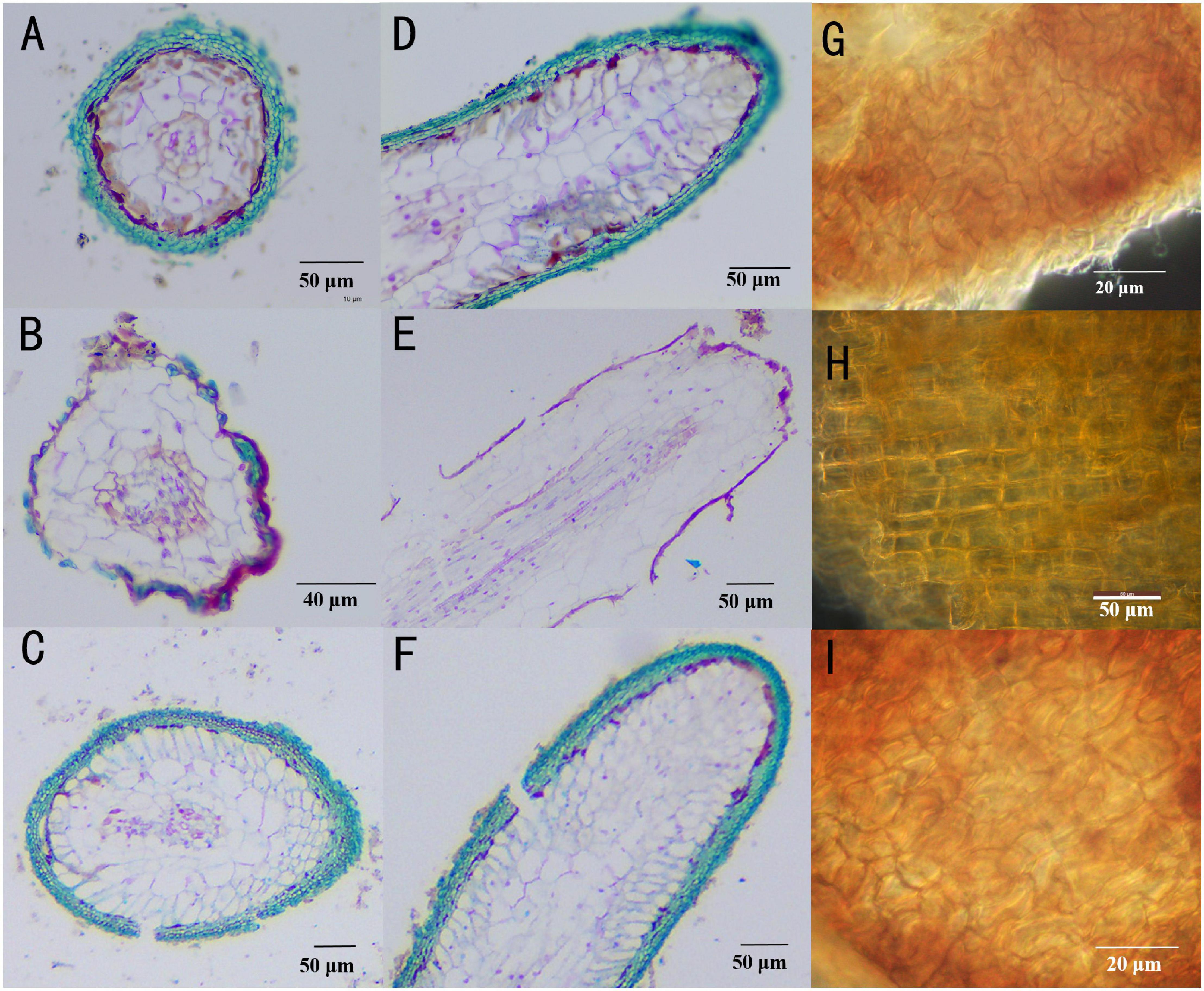
Figure 2. Structural characteristics of Quercus aliena root tips with or without Tuber partner. (A) Cross section of Tuber indicum ectomycorrhizae, Scale bar = 50 μm; (B) Q. aliena roots without Tuber partner, Scale bar = 40 μm; (C) Tuber melanosporum ectomycorrhizae, Scale bar = 50 μm; (D) longitudinal section of T. indicum ectomycorrhizae Scale bar = 50 μm; (E) Q. aliena roots without Tuber partner, Scale bar = 50 μm; (F) T. melanosporum ectomycorrhizae, Scale bar = 50 μm; (G) mantle cell of T. indicum ectomycorrhizae, Scale bar = 20 μm; (H) cell of root tips without Tuber partner, Scale bar = 50 μm. (I) mantle cell of T. melanosporum ectomycorrhizae, Scale bar = 20 μm.
The average pH, content of OM, NH4+-N content, and the AK content in the control treatment were not significantly different from T. melanosporum and T. indicum treatments (Table 2). The NO3–-N content ranged from 5.04 to 8.71 g kg–1, AP content ranged from 9.88 to 19.95 mg kg–1, and TN content from 0.82 to 0.90 g kg–1. The content of NO3–-N and AP in Tuber spp. treatments were higher in the uninoculated treatment. Furthermore, these significantly higher in T. melanosporum treatment than in the T. indicum treatment (p < 0.05). The total nitrogen content was significantly higher in the T. melanosporum treatment than in the T. indicum treatment (p < 0.05), but there was no significant difference between Tuber spp. treatments and control treatment.
The nirK- and nirS-Communities
In the rhizospheres, the diversity of the nirK-community was higher in the T. indicum treatment than in the uninoculated treatment (p < 0.05) (Table 3). The richness of the nirK-community was highest in the T. indicum treatment and lowest in the uninoculated treatment (p < 0.05). The diversity and richness of the nirS-communities were on the same level in all the treatments.
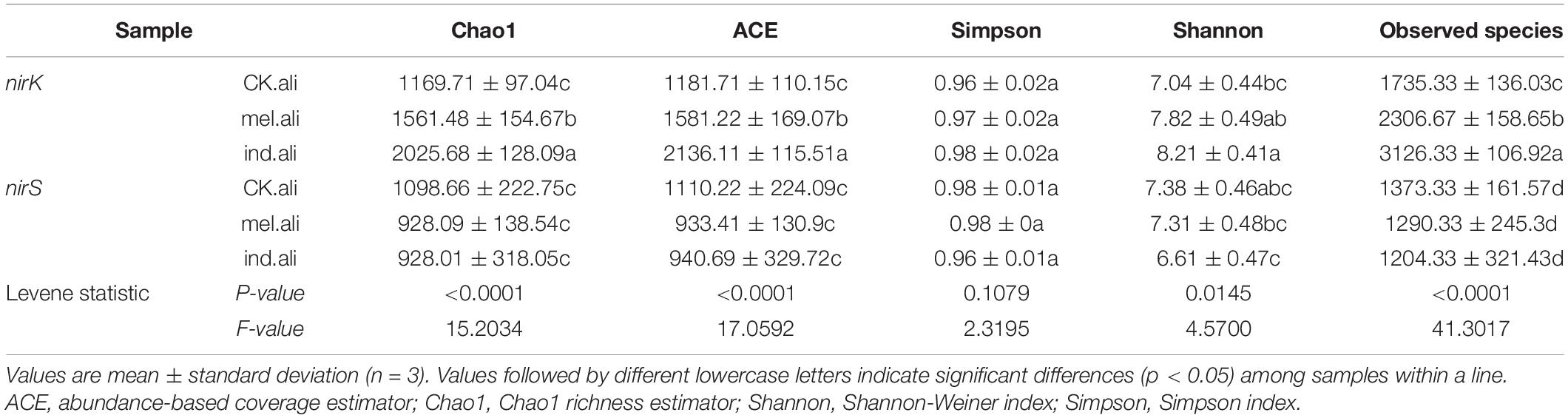
Table 3. Community richness and diversity indices of nirK- and nirS-type denitrifying bacteria in the rhizosphere soil of Quercus aliena with or without Tuber partner.
The identified nirK were mostly assigned to phyla Proteobacteria (98.1–99.9%) and Firmicutes (<0.1%), and to families Rhizobiaceae, Bradyrhizobiaceae, Alcaligenaceae, Hyphomicrobiaceae, and Rhodobacteraceae (Figure 3A). The Achromobacter, Bosea, Sinorhizobium, Rhizobium, Devosia, Ochrobactrum, Paracoccus, and Citrobacter were dominant genera in three treatments (Supplementary Table 2). Achromobacter (13%) was a predominant denitrifer in T. melanosporum treatment, while Nitratireductor, Diaphorobacter, Mesorhizobium, Bosea, and Ochrobactrum were predominant denitrifers in T. indicum treatment (Figure 4A). The identified nirS were mostly assigned to phyla Proteobacteria (97.5–82.5%), Chloroflexi (0–5.2%), and Candidate division NC10 (0–.3%), and to families Pseudomonadaceae, Chromobacteriaceae, Halomonadaceae, Burkholderiaceae, and Rhodanobacteraceae (Figure 3B). Pseudomonas, Pseudogulbenkiania, Halomonas, Cupriavidus, Magnetospirillum, Rhodanobacter, Rubrivivax, and Azoarcus were dominant nirS-type bacterial genera (Supplementary Table 2). Dechlorospirillum, Herbaspirillum, Oleispira, and Ruegeria were predominant denitrifers in T. melanosporum treatment, while Marinobacter, Rubrivivax, Cupriavidus, Hydrogenophilus, and Dinoroseobacter were predominant denitrifers in T. indicum treatment (Figure 4B).
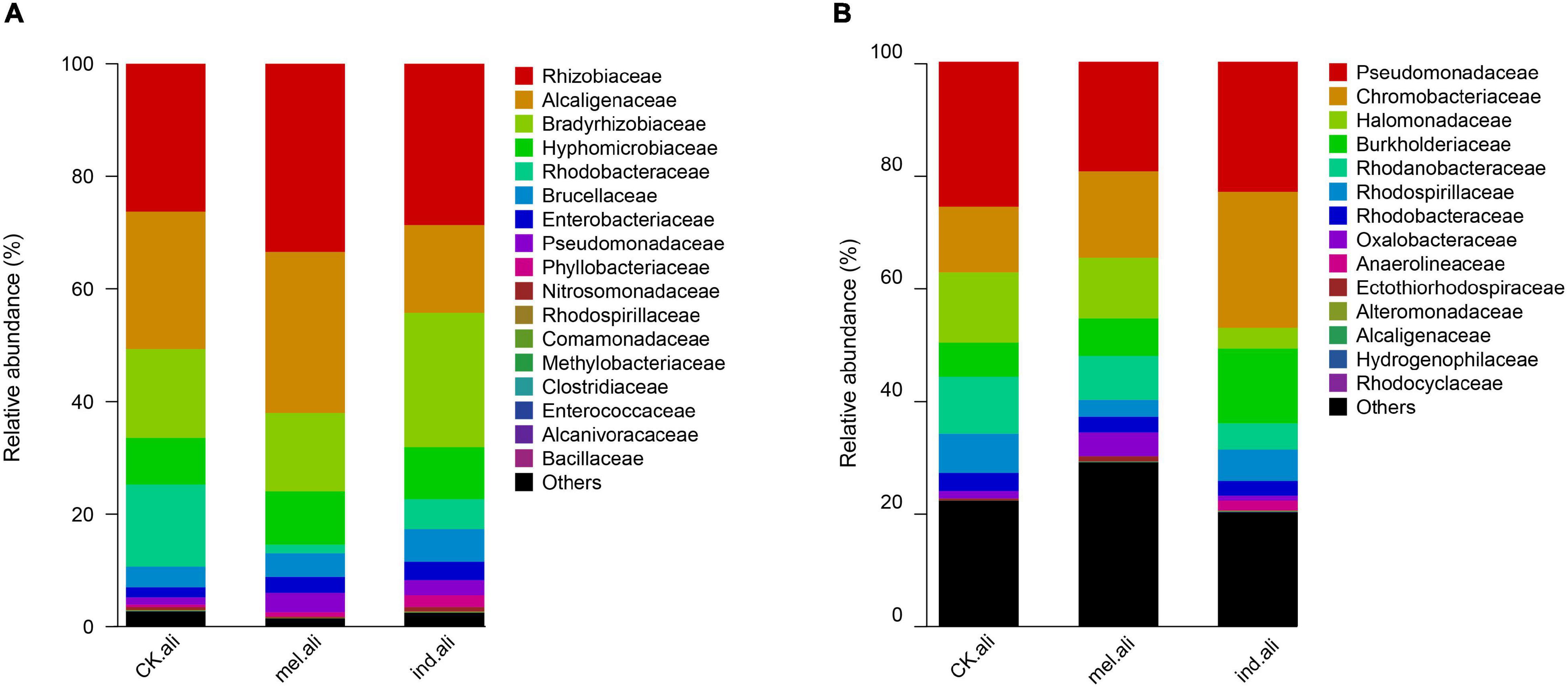
Figure 3. Family level taxonomic composition of (A) nirK- and (B) nirS-type denitrifier communities in the rhizosphere soil of Quercus aliena. CK.ali, uninoculated Q. aliena; ind.ali, Q. aliena inoculated with Tuber indicum; and mel.ali, Q. aliena inoculated with Tuber melanosporum.
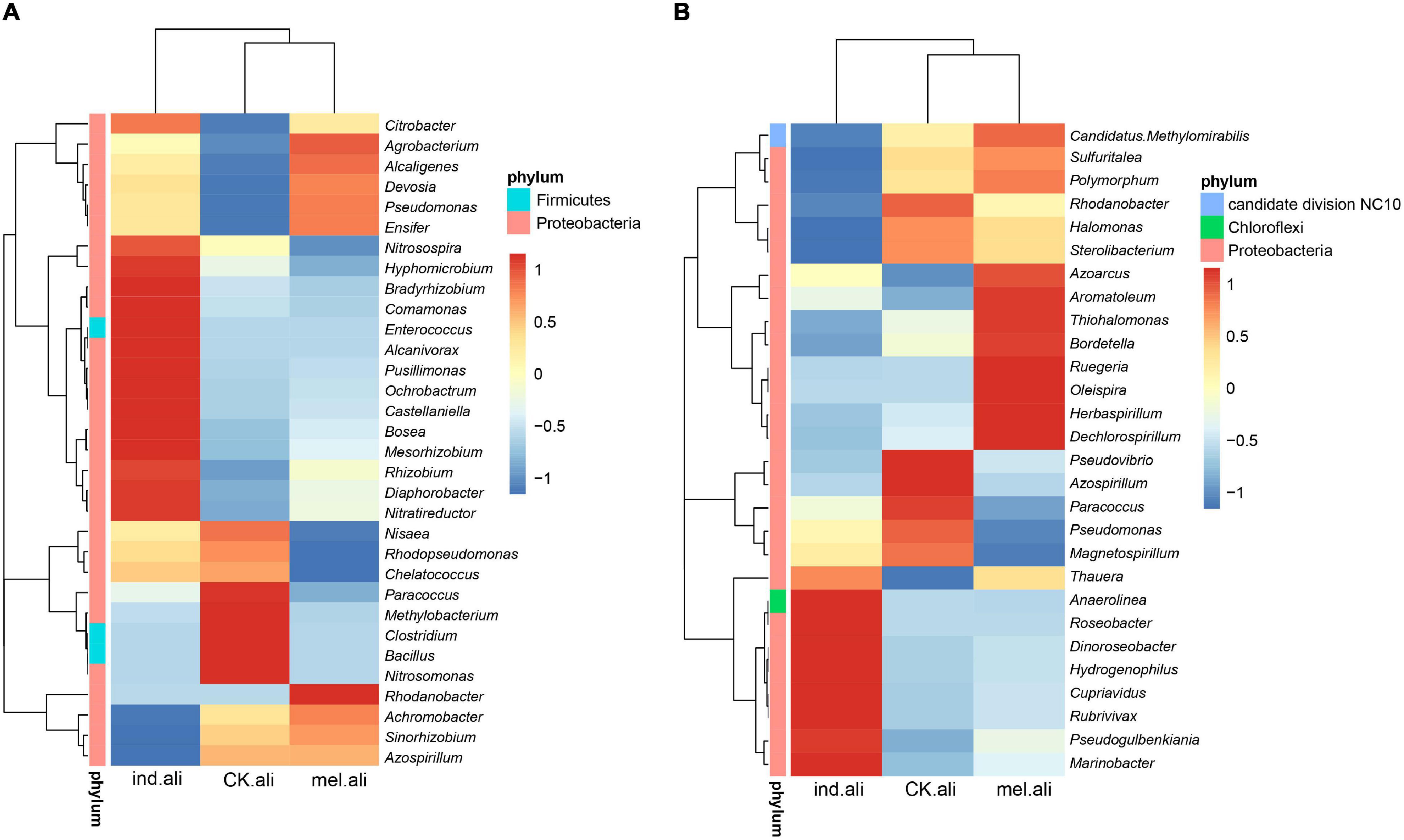
Figure 4. Heat-maps of the most abundant (A) nirK- and (B) nirS-type denitrifying bacterial genera in rhizosphere soil of Quercus aliena. CK.ali, uninoculated Q. aliena; ind.ali, Q. aliena inoculated with Tuber indicum; and mel.ali, Q. aliena inoculated with Tuber melanosporum.
In the weighted UniFrac-based NMDS, the nirK communities were not clearly separated into treatment-specific groups. The samples in T. indicum and T. melanosporum treatments were similar but different from those in the control treatment (Figure 5A). The nirS communities showed that three samples of the control treatment were collected in a group, and only two samples in T. indicum and T. melanosporum treatment were similar. The control treatment was different with Tuber spp. treatments, and T. indicum treatment was also different with T. melanosporum treatment (Figure 5B). In the redundancy analysis, the axis RDA1 accounted for 28.12% and RDA2 for 22.69% of the variation in nirK-community composition (Figure 6A). The differences in nirK-community composition were associated with differences in NO3–-N content (r2 = 0.6646, p = 0.026). RDA1 accounted for 28.90% and RDA2 for 21.71% of the variation in nirS-community composition (Figure 6B). The differences in nirS-community composition were associated with differences in pH (r2 = 0.5875, p = 0.043) and available phosphorus (r2 = 0.9228, p < 0.001) and NO3–-N (r2 = 0.6778, p = 0.017) content.
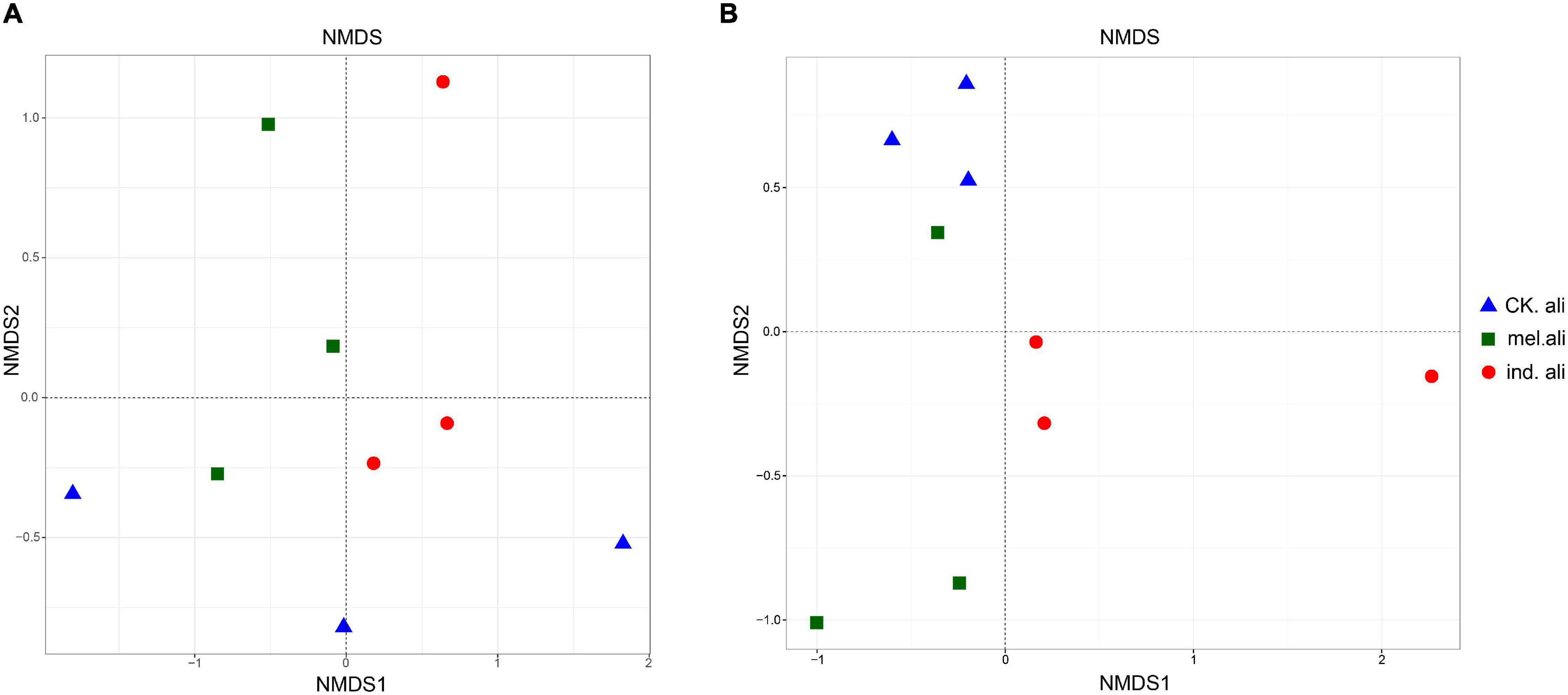
Figure 5. Non-metric multidimensional scaling (NMDS) plots of (A) nirK- and (B) nirS-type denitrifying bacterial communities in rhizosphere soil of Quercus aliena. CK.ali, rhizosphere soil of Q. aliena without Tuber partner; mel.ali, ectomycorrhizosphere soil of Q. aliena with Tuber melanosporum partner; and ind.ali, ectomycorrhizosphere soil of Q. aliena with Tuber indicum partner.
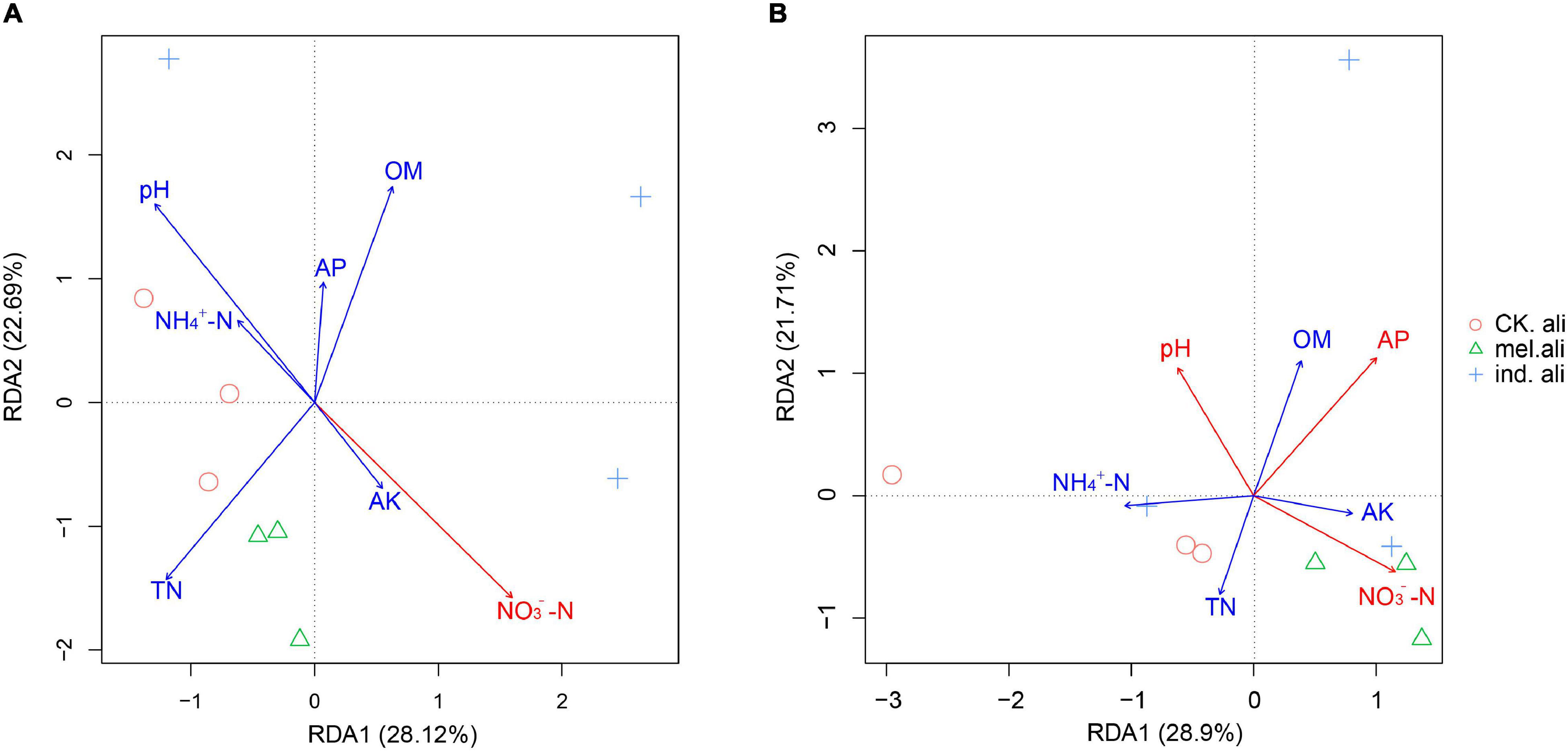
Figure 6. Redundancy analysis (RDA) between (A) nirK- and (B) nirS-type denitrifying bacterial communities and soil factors in the rhizosphere soil of Quercus aliena. CK.ali, rhizosphere soil of Q. aliena without Tuber partner; mel.ali, ectomycorrhizosphere soil of Q. aliena with Tuber melanosporum partner; and ind.ali, ectomycorrhizosphere soil of Q. aliena with Tuber indicum partner.
Discussion
We assessed how T. melanosporum and T. indicum inoculations affect the growth of Q. aliena seedlings, the properties of rhizosphere soil, and the associated nirK- and nirS-denitrifier communities in the initial stage of ectomycorrhizae formation. The morphology of T. melanosporum and T. indicum ectomycorrhizae were similar to the ectomycorrhizae of other Tuber spp. and inoculated Quercus spp. (Perez et al., 2007; Suz et al., 2010b; Li et al., 2018). Likewise, the cross and longitudinal sections of the ectomycorrhizae were similar to those of the other Tuber spp.–Quercus spp. ectomycorrhizae (Benucci et al., 2012; Deng et al., 2014; Zhang et al., 2019), and the uneven puzzle-like patterns in the root tips were similar to those of T. melanosporum– and T. indicum–Quercus spp. ectomycorrhizae (Deng et al., 2014; Huang et al., 2020).
Similar to inoculating Pinus armandii and Carya illinoinensis with Tuber spp. (Zhang et al., 2020; Huang et al., 2021), inoculating Q. aliena with T. melanosporum and T. indicum barely affected the plant parameters 6 months after inoculation. However, earlier studies showed that inoculation with Tuber spp. improved the growth of hosts during the summer drought (Domínguez Núñez et al., 2006; Morte et al., 2010). In a 2-year experiment, inoculation with Tuber spp. promoted the growth of Quercus spp. hosts in greenhouse (Wang et al., 2019). Our result probably implies that the inoculation plays a minor role only in the primary growth stages of plants grown in the favorable greenhouse environment, because 6 months are too short for the long lifespan of Q. aliena. Similar to P. armandii and C. illinoinensis inoculated with Tuber spp. and T. sinoaestivum, respectively (Zhang et al., 2020; Huang et al., 2021), the POD activity was higher in the seedlings inoculated with T. melanosporum than in the other treatments. In a previous study, POD activity was higher, and the incidence of pathogenic fungus Microdochium tabacinum was lower in soybeans inoculated with AMF fungi (Gao et al., 2018). Since the POD activity is one of the enzymatic defense mechanisms in plants (Ma et al., 2012), inoculation with T. melanosporum may possibly assist Q. aliena to confront infection.
In our study, the available phosphorus content of the ectomycorrhizosphere soils were higher in with truffle inoculation than without truffle inoculation, which was consistent with previous work (Li et al., 2017; Zhang et al., 2020). The higher concentration of phosphorus may be caused by the ectomycorrhizae (Dominguez et al., 2012) and the functional bacteria in ectomycorrhizosphere soils (Matthijs et al., 2007). Similar to previous studies (Kang et al., 2020; Siebyła and Hilszczañska, 2020), truffle inoculation did not affect the organic matter, total nitrogen, and available potassium contents, implying the T. indicum and T. melanosporum have limited influence on these properties of ectomycorrhizosphere soils. The nitrate-nitrogen contents were higher with than without truffle inoculation. Previous study showed that T. melanosporum mycelium prefer organic nitrogen than nitrate-nitrogen (Kamal, 2011), and this may be one cause of significantly more nitrate-nitrogen content in T. melanosporum ectomycorrhizosphere soils. Furthermore, PttNRT2.4A is a high affinity nitrate importer expressed in ectomycorrhizae, and the transcript levels PttNRT2.4A increased when ectomycorrhizae were exposed to low nitrate concentrations (10–100 μM) (Willmann et al., 2014). This may indicate that the nitrate-nitrogen content might be regulated by the level of nitrate transporter function gene in Tuber spp. ectomycorrhizae and the nitrate-nitrogen content in ectomycorrhizosphere soils. Furthermore, the higher total nitrogen content in T. melanosporum treatment than T. indicum treatment may due to the nitrate-nitrogen content.
Denitrifying bacteria are widely distributed in terrestrial ecosystems. Commonly, the nirK-type denitrifiers have been more abundant than nirS-type denitrifiers, e.g., in forest, paddy, and wetland soils and in the rhizosphere (Levy-Booth and Winder, 2010; Bannert et al., 2011; Hamonts et al., 2013). In agreement, the biodiversity and richness of nirK-type denitrifiers were greater than those of nirS-type denitrifiers in the rhizosphere of Q. aliena cultivated in greenhouse. In addition, the top abundant genera of nirS-type denitrifiers in rhizosphere soil of Q. aliena with Tuber spp. inoculation were the same as these in C. illinoinensis with Tuber spp. inoculation (Huang et al., 2021). Contrary to α-diversity, the β-diversity of nirS-type denitrifiers in ectomycorrhizosphere soils were different from rhizosphere soils, which may indicate that the Tuber spp. inoculation effect nirS-type denitrifier composition at species level. This needs further study with other DNA sequencing technology, such as metagenomics, to classify denitrifiers at species-level to reveal the character of denitrifiers composition in ectomycorrhizosphere soils.
The higher nirK-denitrifier richness in ectomycorrhizosphere soils was possibly associated with the higher nitrate-nitrogen content with truffle inoculation than without. Although the nitrate-nitrogen content was higher in T. melanosporum than T. indicum treatments, the nirK-denitrifier richness was lower in T. melanosporum than in T. indicum treatments. Among the nirK-denitrifiers detected in our study, Bacillus, Nitrosomonas, Nitrosospira, Alcaligenes, and Pseudomonas include many heterotrophic nitrifying–aerobic denitrifying bacterial strains, e.g., Bacillus haynesii (Huang et al., 2017), Alcaligenes faecalis (Joo et al., 2005; Zhao et al., 2012), Pseudomonas YY3 (Lang et al., 2019), Nitrosomonas europaea (Kozlowski et al., 2014), N. europaea (Chain et al., 2003), and Nitrospira inopinata (Van Kessel et al., 2015). Thus, the higher nitrate-nitrogen content in T. melanosporum ectomycorrhizosphere soils may have been partly due to nitrification activity. The nirS-type bacteria were more sensitive than nirK-type bacteria to anoxia, N limitation (Hamonts et al., 2013), and urine application (Anderson et al., 2014). In our study, the nirS-type bacteria correlated with NO3–-N and AP contents and pH, whereas the nirK-type bacteria correlated with the NO3–-N only, which may indicate that the nirS-type bacteria were more sensitive than the nirK-type bacteria to soil properties (Jones and Hallin, 2010; Azziz et al., 2017).
However, the nirK-denitrifiers were more sensitive to the inoculation. Baneras et al. (2012) concluded that the nirK-denitrifier community composition in rhizosphere soils varied with plant species, possibly due to the root exudates. Tuber spp. inoculation affected the compounds of Q. mongolica root exudates (Wang et al., 2021). In addition, ectomycorrhizae may secrete signal molecules belonging to diverse groups of organic compounds (Bouwmeester et al., 2007), and these metabolites present in the root-adhering soil were well-correlated with soil microbial denitrification activity and denitrifying bacterial community structure (Guyonnet et al., 2017; Hou et al., 2018). Compared to the uninoculated Q. aliena, the higher nirK-denitrifier diversity and richness in the rhizosphere of the inoculated seedlings might be due to difference in root exudates.
Conclusion
We inoculated T. melanosporum and T. indicum on Q. aliena seedlings, determined the characteristics of ectomycorrhizae, seedlings and rhizosphere soil, and analyzed the rhizosphere nirK- and nirS-denitrifier communities. The higher POD activity in the seedlings inoculated with T. melanosporum than in the other treatments suggested that inoculation with T. melanosporum may possibly assist Q. aliena to confront infection. The AP contents were lower and nitrate-nitrogen nitrogen (NO3–-N) contents were higher with than without truffle inoculation, and T. melanosporum treatment differed more from the control than the T. indicum treatment. The diversity and richness of nirK-denitrifier communities were improved by two truffles inoculation, and the effect of Tuber spp. inoculation on the nirS-denitrifier communities was relatively small. The effect of inoculation on the soil properties and nirK- and nirS-denitrifier communities likely depends on the Tuber spp.-host plant combination.
Data Availability Statement
The datasets presented in this study can be found in online repositories. The names of the repository/repositories and accession number(s) can be found below: https://www.ncbi.nlm.nih.gov/genbank/, PRJNA556350.
Author Contributions
ZK, XL, and YG conceived and designed the experiments. ZK, BZ, and YL performed the experiments. ZK, XL, YL, XZ, PP, and YG wrote and revised the manuscript. All authors approved the final version of the manuscript.
Funding
This work was supported by the Science and Technology Support Project in Sichuan Province (2016NYZ0040 and 2021YFYZ0026) and the Sichuan Mushroom Innovation Team.
Conflict of Interest
The authors declare that the research was conducted in the absence of any commercial or financial relationships that could be construed as a potential conflict of interest.
Publisher’s Note
All claims expressed in this article are solely those of the authors and do not necessarily represent those of their affiliated organizations, or those of the publisher, the editors and the reviewers. Any product that may be evaluated in this article, or claim that may be made by its manufacturer, is not guaranteed or endorsed by the publisher.
Acknowledgments
We would like to thank the researchers of Soil and Fertilizer Institute, Sichuan Academy of Agricultural Sciences, and Zou jie for their contribution to this study.
Supplementary Material
The Supplementary Material for this article can be found online at: https://www.frontiersin.org/articles/10.3389/fmicb.2022.792568/full#supplementary-material
Supplementary Figure 1 | Rank abundance curves for (A) nirK- and (B) nirS-type denitrifying bacterial operational taxonomic unit (OTU) diversity in rhizosphere soil of not inoculation Quercus aliena (CK.ali) and Q. aliena inoculated with Tuber melanosporum (mel.ali) or Tuber indicum (ind.ali).
Supplementary Figure 2 | Rarefaction curves of (A) nirK- and (B) nirS-OTUs in the rhizosphere of not inoculated Q. aliena (CK.ali) and Q. aliena inoculated with Tuber melanosporum (mel.ali) or Tuber indicum (ind.ali).
Supplementary Figure 3 | The Shapiro-Wilk test for normality of (A) morphological indices and (B) physiological indices of Quercus aliena seedlings, (C) soil properties, and (D) richness and diversity of nirK- and nirS-denitrifiers.
Supplementary Figure 4 | Differential abundance analysis of nirK- and nirS-OTUs in the rhizosphere of not inoculated Q. aliena (CK.ali) and Q. aliena inoculated with Tuber melanosporum (mel.ali) or Tuber indicum (ind.ali). (A) nirK-OTUs in CK vs. mel.ali, (B) nirK-OTUs in CK vs. ind.ali, (C) nirK-OTUs in ind.ali vs. mel.ali, (D) nirS-OTUs in CK vs. mel.ali, (E) nirS-OTUs in CK vs. ind.ali, and (F) nirS-OTUs in ind.ali vs. mel.ali. The x-axes show median log2 dispersion within treatments and the y-axes median log2 difference between treatments.
Footnotes
- ^ http://sourceforge.net/projects/rdp-classifier/
- ^ http://fungene.cme.msu.edu/
- ^ https://www.r-project.org/
References
Allen, K., and Bennett, J. W. (2021). Tour of truffles: aromas, aphrodisiacs, adaptogens, and more. Mycobiology 49, 201–212. doi: 10.1080/12298093.2021.1936766
Alvarez, M., Huygens, D., Olivares, E., Saavedra, I., Alberdi, M., and Valenzuela, E. (2010). Ectomycorrhizal fungi enhance nitrogen and phosphorus nutrition of Nothofagus dombeyi under drought conditions by regulating assimilative enzyme activities. Physiol. Plantarum. 136:1237.
Anderson, M. J. (2006). Distance-based tests for homogeneity of multivariate dispersions. Biometrics 62, 245–253. doi: 10.1111/j.1541-0420.2005.00440.x
Anderson, C. R., Hamonts, K., Clough, T. J., and Condron, L. M. (2014). Biochar does not affect soil N-transformations or microbial community structure under ruminant urine patches but does alter relative proportions of nitrogen cycling bacteria. Agr. Ecosyst. Environ. 191, 63–72. doi: 10.1016/j.agee.2014.02.021
Azziz, G., Monza, J., Etchebehere, C., and Irisarri, P. (2017). NirS- and nirK-type denitrifier communities are differentially affected by soil type, rice cultivar and water management. Eur. J. Soil Biol. 78, 20–28. doi: 10.1016/j.ejsobi.2016.11.003
Baneras, L., Ruiz-Rueda, O., Lopez-Flores, R., Quintana, X. D., and Hallin, S. (2012). The role of plant type and salinity in the selection for the denitrifying community structure in the rhizosphere of wetland vegetation. Int. Microbiol. 15, 89–99. doi: 10.2436/20.1501.01.162
Bannert, A., Kleineidam, K., Wissing, L., Mueller-Niggemann, C., Vogelsang, V., Welzl, G., et al. (2011). Changes in diversity and functional gene abundances of microbial communities involved in nitrogen fixation, nitrification, and denitrification in a tidal wetland versus paddy soils cultivated for different time periods. Appl. Environ. Microbiol. 77, 6109–6116. doi: 10.1128/AEM.01751-10
Barbieri, E., Ceccaroli, P., Saltarelli, R., Guidi, C., Potenza, L., Basaglia, M., et al. (2010). New evidence for nitrogen fixation within the Italian white truffle Tuber magnatum. Fungal. Biol. 114, 936–942. doi: 10.1016/j.funbio.2010.09.001
Barbieri, E., Guidi, C., and Bertaux, J. (2007). Occurrence and diversity of bacterial communities in Tuber magnatum during truffle maturation. Environ. Microbiol. 9, 2234–2246. doi: 10.1111/j.1462-2920.2007.01338.x
Bárta, J., Melichová, T., Vanìk, D., Picek, T., and Santruková, H. (2010). Effect of pH and dissolved organic matter on the abundance of nirK and nirS denitrifiers in spruce forest soil. Biogeochemistry 101, 123–132. doi: 10.1007/s10533-010-9430-9
Bárta, J., Tahovská, K., Šantrùèková, H., and Oulehle, F. (2017). Microbial communities with distinct denitrification potential in spruce and beech soils differing in nitrate leaching. Sci. Rep. 7:9738. doi: 10.1038/s41598-017-08554-1
Beatrice, B., Claudia, R., Sabrina, T., Marcella, P., Francesco, P., and Andrea, R. (2012). Comparison of ectomycorrhizal communities in natural and cultivated Tuber melanosporum truffle grounds. FEMS Microbiol. Ecol. 3:1379. doi: 10.1111/j.1574-6941.2012.01379.x
Benucci, G. M. N., and Bonito, G. M. (2016). The truffle microbiome: species and geography effects on bacteria associated with fruiting bodies of hypogeous Pezizales. Microb. Ecol. 72, 4–8. doi: 10.1007/s00248-016-0755-3
Benucci, G. M. N., Gogan-Csorbai, A., Baciarelli-Falini, L., Bencivenga, M., Di-Massimo, G., and Donnini, D. (2012). Mycorrhization of Quercus robur L., Quercus cerris L. and Corylus avellana L. seedlings with Tuber macrosporum vittad. Mycorrhiza 22, 639–646. doi: 10.1007/s00572-012-0441-3
Bouwmeester, H. J., Roux, C., Lopez-Raez, J. A., and Becard, G. (2007). Rhizosphere communication of plants, parasitic plants and AM fungi. Trends Plant Sci. 12, 224–230. doi: 10.1016/j.tplants.2007.03.009
Caporaso, J. G., Kuczynski, J., Stombaugh, J., Bittinger, K., Bushman, F. D., Costello, E. K., et al. (2010). QIIME allows analysis of high-throughput community sequencing data. Nat. Methods 2010:303. doi: 10.1038/nmeth.f.303
Chain, P., Lamerdin, J., Larimer, F., Regala, W., Lao, V., Land, M., et al. (2003). Complete genome sequence of the ammonia-oxidizing bacterium and obligate chemolithoautotroph Nitrosomonas europaea. J. Bacteriol. 185, 2759–2773. doi: 10.1128/JB.185.9.2759-2773.2003
Chan, Y. K., and Mccormick, W. A. (2004). Experimental evidence for plasmid-borne nor-nir genes in Sinorhizobium meliloti JJ1c10. Can. J. Microbiol. 50, 657–667. doi: 10.1139/w04-062
Cristina, S., and Kiwamu, M. (2011). Redundant roles of Bradyrhizobium oligotrophicum cu-type (nirK) and cd1-type (nirS) nitrite reductase genes under denitrifying conditions. FEMS Microbiol. Ecol. 5:15. doi: 10.1093/femsle/fny015
Deng, X., Yu, F., and Liu, P. (2014). Contribution to confirmed & synthesized on mycorrhizae of Tuber indicum s.l. with two dominated & subalpine broadleaf trees in southwestern China. AJPS 5, 3269–3279. doi: 10.4236/ajps.2014.521341
Deveau, A., Antony-Babu, S., Le Tacon, F., Robin, C., Frey-Klett, P., and Uroz, S. (2016). Temporal changes of bacterial communities in the Tuber melanosporum ectomycorrhizosphere during ascocarp development. Mycorrhiza 26, 389–399. doi: 10.1007/s00572-015-0679-7
Dominguez, J. A., Martin, A., Anriquez, A., and Albanesi, A. (2012). The combined effects of Pseudomonas fluorescens and Tuber melanosporum on the quality of Pinus halepensis seedlings. Mycorrhiza 22, 429–436. doi: 10.1007/s00572-011-0420-0
Domínguez Núñez, J. A., Serrano, J. S., Barreal, J. A. R., and González, J. A. S. D. O. (2006). The influence of mycorrhization with Tuber melanosporum in the afforestation of a mediterranean site with quercus ilex and quercus faginea. Forest. Ecol. Manag. 231, 226–233. doi: 10.1016/j.foreco.2006.05.052
Fridovich, I. (2011). Superoxide dismutases: anti- versus pro-oxidants? Anti Cancer. Agent. Me. 11, 175–177. doi: 10.2174/187152011795255966
Gao, P., Guo, Y., Li, Y., and Duan, T. (2018). Effects of dual inoculation of AMF and rhizobium on alfalfa (Medicago sativa) root rot caused by Microdochium tabacinum. Australas. Plant. Path. 47, 195–203. doi: 10.1007/s13313-018-0543-2
Garcia-Barreda, S., Molina-Grau, S., and Reyna, S. (2015). Reducing the infectivity and richness of ectomycorrhizal fungi in a calcareous quercus ilex forest through soil preparations for truffle plantation establishment: a bioassay study. Fungal. Biol. 119, 1137–1143. doi: 10.1016/j.funbio.2015.08.014
García-Montero, L. G., Casermeiro, M. A., Hernando, J., and Hernando, I. (2006). Soil factors that influence the fruiting of Tuber melanosporum (black truffle). Soil. Res. 44:46. doi: 10.1071/sr06046
Geng, L. Y., Wang, X. H., Yu, F. Q., Deng, X. J., Tian, X. F., Shi, X. F., et al. (2009). Mycorrhizal synthesis of Tuber indicum with two indigenous hosts, Castanea mollissima and Pinus armandii. Mycorrhiza 19, 461–467. doi: 10.1007/s00572-009-0247-0
Guyonnet, J. P., Florian, V., Guillaume, M., Labois, C., Cantarel, A. A. M., Michalet, S., et al. (2017). The effects of plant nutritional strategy on soil microbial denitrification activity through rhizosphere primary metabolites. FEMS Microbiol. Ecol. 4:22. doi: 10.1093/femsec/fix022
Hamonts, K., Clough, T. J., Stewart, A., Clinton, P. W., Richardson, A. E., Wakelin, S. A., et al. (2013). Effect of nitrogen and waterlogging on denitrifier gene abundance, community structure and activity in the rhizosphere of wheat. FEMS Microbiol. Ecol. 83, 568–584. doi: 10.1111/1574-6941.12015
Hill, T., Walsh, K. A., Harris, J. A., and Moffett, B. F. (2003). Using ecological diversity measures with bacterial communities. FEMS Microbiol. Ecol. 43, 1–11. doi: 10.1016/S0168-6496(02)00449-X
Hou, S., Ai, C., Zhou, W., Liang, G., and He, P. (2018). Structure and assembly cues for rhizospheric nirK- and nirS-type denitrifier communities in long-term fertilized soils. Soil. Biol. Biochem. 119, 32–40. doi: 10.1016/j.soilbio.2018.01.007
Huang, F., Pan, L., Lv, N., and Tang, X. (2017). Characterization of novel Bacillus strain N31 from mariculture water capable of halophilic heterotrophic nitrification-aerobic denitrification. J. Biosci. Bioeng. 124, 564–571. doi: 10.1016/j.jbiosc.2017.06.008
Huang, L. L., Guerin-Laguette, A., Wang, R., and Yu, F. Q. (2020). Characterization of Tuber indicum (pezizales, tuberaceae) mycorrhizae synthesized with four host trees exotic to China. Symbiosis 82, 215–224. doi: 10.1007/s13199-020-00715-7
Huang, Y., Zou, J., Kang, Z. J., Zhang, X. P., Penttinen, P., Zhang, X. P., et al. (2021). Effects of truffle inoculation on a nursey culture substrate environment of Carya illinoinensis seedlings. Fungal. Biol. 125:7. doi: 10.1016/j.funbio.2021.02.006
Jones, C. M., and Hallin, S. (2010). Ecological and evolutionary factors underlying global and local assembly of denitrifier communities. ISME J. 4, 633–641. doi: 10.1038/ismej.2009.152
Joo, H. S., Hirai, M., and Shoda, M. (2005). Characteristics of ammonium removal by heterotrophic nitrification-aerobic denitrification by alcaligenes faecalis No. 4. J. Biosci. Bioeng. 100, 184–191. doi: 10.1263/jbb.100.184
Kamal, S. (2011). “Effect of nutrient source and plant hormones on mycelial morphology of the black perigord truffle Tuber melanosporum,” in In Proceedings of the 7 th International Conference on Mushroom Biology and Mushroom Products (ICMBMP7).
Kang, Z., Zou, J., Huang, Y., Zhang, X., Ye, L., Zhang, B., et al. (2020). Tuber melanosporum shapes nirS-type denitrifying and ammonia-oxidizing bacterial communities in Carya illinoinensis ectomycorrhizosphere soils. PeerJ 8:e9457. doi: 10.7717/peerj.9457
Kozlowski, J. A., Price, J., and Stein, L. Y. (2014). Revision of N2O-producing pathways in the ammonia-oxidizing bacterium nitrosomonas europaea ATCC 19718. Appl. Environ. Microb. 80, 4930–4935. doi: 10.1128/AEM.01061-14
Lang, X., Li, Q., Xu, Y., Ji, M., Yan, G., and Guo, S. (2019). Aerobic denitrifiers with petroleum metabolizing ability isolated from caprolactam sewage treatment pool. Bioresour. Technol. 290:121719. doi: 10.1016/j.biortech.2019.121719
Le-Roux, C., Tournier, E., Lies, A., Duponnois, R., Mousain, D., and Prin, Y. (2016). Bacteria of the genus Rhodopseudomonas (Bradyrhizobiaceae): obligate symbionts in mycelial cultures of the black truffles Tuber melanosporum and Tuber brumale. SpringerPlus 5:2756. doi: 10.1186/s40064-016-2756-6
Levy-Booth, D. J., and Winder, R. S. (2010). Quantification of nitrogen reductase and nitrite reductase genes in soil of thinned and clear-cut douglas-fir stands by using real-time PCR. Appl. Environ. Microbiol. 76, 7116–7125. doi: 10.1128/AEM.02188-09
Li, Q., Yan, L., Ye, L., Zhou, J., Zhang, B., Peng, W., et al. (2018). Chinese black truffle (Tuber indicum) alters the ectomycorrhizosphere and endoectomycosphere microbiome and metabolic profiles of the host tree quercus aliena. Front. Microbiol. 9:2202. doi: 10.3389/fmicb.2018.02202
Li, Q., Zhao, J., Xiong, C., Li, X., Chen, Z., Li, P., et al. (2017). Tuber indicum shapes the microbial communities of ectomycorhizosphere soil and ectomycorrhizae of an indigenous tree (Pinus armandii). PLoS One 12:e0175720. doi: 10.1371/journal.pone.0175720
Li, X., Liu, C., Tang, P., Yang, M., Qing, Y., Jia, D., et al. (2014). Plant community and ecological environment in truffle producing area of Miyi, panzhihua, sichuan. Southwest China J. Agric. Sci. 27:63. doi: 10.3969/j.issn.1001-4829.2014.04.063
Liese, R., Lübbe, T., Albers, N. W., and Meier, I. C. (2017). The mycorrhizal type governs root exudation and nitrogen uptake of temperate tree species. Tree. Physiol. 2017:131. doi: 10.1093/treephys/tpx131
Ma, L., Li, Y., Yu, C., Wang, Y., Li, X., Li, N., et al. (2012). Alleviation of exogenous oligochitosan on wheat seedlings growth under salt stress. Protoplasma 249, 393–399. doi: 10.1007/s00709-011-0290-5
Mago, T., and Salzberg, S. L. (2011). FLASH: fast length adjustment of short reads to improve genome assemblies. Bioinformatics 27, 2957–2963. doi: 10.1093/bioinformatics/btr507
Marcel, M. M. K., Hannah, K. M., and Boran, K. (2018). The microbial nitrogen-cycling network. Nat. Rev. Microbiol. 2018:9. doi: 10.1038/nrmicro.2018.9
Matthijs, S., Tehrani, K. A., Laus, G., Jackson, R. W., Cooper, R. M., and Cornelis, P. (2007). Thioquinolobactin, a Pseudomonas siderophore with antifungal and anti-pythium activity. Environ. Microbiol. 9, 425–434. doi: 10.1111/j.1462-2920.2006.01154.x
Meloni, D. A., Oliva, M. A., Martinez, C. A., and Cambraia, J. (2003). Photosynthesis and activity of superoxide dismutase, peroxidase and glutathione reductase in cotton under salt stress. Environ. Exp. Bot. 49, 69–76. doi: 10.1016/S0098-8472(02)00058-8
Mesa, S., Alché, J. D. D., Bedmar, E., and Delgado, M. J. (2010). Expression of nir, nor and nos denitrification genes from Bradyrhizobium japonicum in soybean root nodules. Physiol. Plantarum. 120, 205–211. doi: 10.1111/j.0031-9317.2004.0211.x
Monaco, P., Bucci, A., Naclerio, G., and Mello, A. (2021). Heterogeneity of the white truffle Tuber magnatum in a limited geographic area of Central-Southern Italy. Environ. Microbiol. 13, 591–599. doi: 10.1111/1758-2229.12956
Montanini, B., Moretto, N., Soragni, E., Percudani, R., and Ottonello, S. (2002). A high-affinity ammonium transporter from the mycorrhizal ascomycete Tuber borchii. Fungal Genet Biol. 36, 22–34. doi: 10.1016/S1087-1845(02)00001-4
Moore, T. R., Bubier, J. L., Frolking, S. E., Lafleur, P. M., and Roulet, N. T. (2002). Plant biomass and production and CO2 exchange in an ombrotrophic bog. J. Ecol. 90, 25–36. doi: 10.1046/j.0022-0477.2001.00633.x
Morte, A., Navarro-Ródenas, A., and Nicolás, E. (2010). Physiological parameters of desert truffle mycorrhizal Helianthemun almeriense plants cultivated in orchards under water deficit conditions. Symbiosis 52, 133–139. doi: 10.1007/s13199-010-0080-4
Oksanen, J., Blanchet, F. G., Friendly, M., Kindt, R., Legendre, P., McGlinn, D., et al. (2020). Vegan: Community Ecology Package. R Package Version 2.5-7. Available online at: https://CRAN.R-project.org/package=vegan
Perez, F., Palfner, G., Brunel, N., and Santelices, R. (2007). Synthesis and establishment of Tuber melanosporum vitt. ectomycorrhizae on two nothofagus species in Chile. Mycorrhiza 17, 627–632. doi: 10.1007/s00572-007-0140-7
Siebyła, M., and Hilszczañska, D. (2020). Diversity of soil bacteria complexes associated with summer truffle (Tuber aestivum). Folia Forestalia Polonica 62, 114–127. doi: 10.2478/ffp-2020-0012
Splivallo, R., Deveau, A., Valdez, N., Kirchhoff, N., Frey-Klett, P., and Karlovsky, P. (2015). Bacteria associated with truffle-fruiting bodies contribute to truffle aroma. Environ. Microbiol. 17:12521. doi: 10.1111/1462-2920.12521
Suz, L. M., Martín, M. P., Daniel, O., Fischer, C. R., and Carlos, C. (2010a). Mycelial abundance and other factors related to truffle productivity in Tuber melanosporum–quercus ilex orchards. FEMS Microbiol. Ecol. 2010, 72–78. doi: 10.1111/j.1574-6968.2008.01213.x
Suz, L. M., Martín, M. P., Fischer, C. R., Bonet, J. A., and Colinas, C. (2010b). Can NPK fertilizers enhance seedling growth and mycorrhizal status of Tuber melanosporum-inoculated quercus ilex seedlings? Mycorrhiza 20, 349–360. doi: 10.1007/s00572-009-0289-3
Torres, M. J., Rubia, M. I., Peña, T. C. D. L., Pueyo, J. J., Bedmar, E. J., and Delgado, M. J. (2014). Genetic basis for denitrification in Ensifer meliloti. BMC Microbiol. 14:142. doi: 10.1186/1471-2180-14-142
Torres, M. J., Sergio, A., Bedmar, E. J., and Delgado, M. J. (2018). Overexpression of the periplasmic nitrate reductase supports anaerobic growth by Ensifer meliloti. Fems Microbiol. Lett. 365:7. doi: 10.1093/femsle/fny041
Vahdatzadeh, M., Deveau, A., and Splivallo, R. (2015). The role of the microbiome of truffles in aroma formation: a meta-analysis approach. Appl. Environ. Microbiol. 81, 6946–6952. doi: 10.1128/AEM.01098-15
Van Kessel, M. A. H. J., Speth, D. R., Albertsen, M., Nielsen, P. H., Op den Camp, H. J. M., Kartal, B., et al. (2015). Complete nitrification by a single microorganism. Nature 528:7583. doi: 10.1038/nature16459
Wan, S. P., Yu, F. Q., Tang, L., Wang, R., Wang, Y., Liu, P. G., et al. (2016). Ectomycorrhizae of Tuber huidongense and Tuber liyuanum with castanea mollissima and pinus armandii. Mycorrhiza 26, 249–256. doi: 10.1007/s00572-015-0663-2
Wang, Q., Garrity, G. M., Tiedje, J. M., and Cole, J. R. (2007). Naiïve bayesian classifier for rapid assignment of rRNA sequences into the new bacterial taxonomy. Appl. Environ. Microbiol. 73, 5261–5267. doi: 10.1128/AEM.00062-07
Wang, R., Guerin-Laguette, A., Butler, R., Huang, L. L., and Yu, F. Q. (2019). The european delicacy tuber melanosporum forms mycorrhizae with some indigenous chinese quercus species and promotes growth of the oak seedlings. Mycorrhiza 29, 649–661. doi: 10.1007/s00572-019-00925-y
Wang, Y., Ran, W., Lu, B., Guerin-Laguette, A., and Yu, F. Q. (2021). Mycorrhization of quercus mongolica seedlings by tuber melanosporum alters root carbon exudation and rhizosphere bacterial communities. Plant Soil 467, 391–403. doi: 10.21203/rs.3.rs-375107/v1
Willmann, A., Thomfohrde, S., Haensch, R., and Nehls, U. (2014). The poplar NRT2 gene family of high affinity nitrate importers: impact of nitrogen nutrition and ectomycorrhiza formation. Environ. Exp. Bot. 108, 79–88. doi: 10.1016/j.envexpbot.2014.02.003
Wittorf, L., Jones, C. M., Bonilla-Rosso, G., and Hallin, S. (2018). Expression of nirK and nirS genes in two strains of Pseudomonas stutzeri harbouring both types of NO-forming nitrite reductases. Res. Microbiol. 169, 343–347. doi: 10.1016/j.resmic.2018.04.010
Yan, T. F., Fields, M. W., Wu, L. Y., Tiedje, J. M., and Zhou, J. (2003). Molecular diversity and characterization of nitrite reductase gene fragments (nirK and nirS) from nitrate- and uranium-contaminated ground water. Environ. Microbiol. 5, 13–24. doi: 10.1046/j.1462-2920.2003.00393.x
Yang, M., Zou, J., Liu, C., Xiao, Y., Zhang, X., Yan, L., et al. (2019). Chinese white truffles shape the ectomycorrhizal microbial communities of Corylus avellana. Ann. Microbiol. 69, 553–565. doi: 10.1007/s13213-019-1445-4
Zhang, X., Huang, G., Bian, X., and Zhao, Q. (2013). Effects of root interaction and nitrogen fertilization on the chlorophyll content, root activity, photosynthetic characteristics of intercropped soybean and microbial quantity in the rhizosphere. Plant Soil Environ. 59, 80–88. doi: 10.1007/s11104-012-1528-5
Zhang, X., Yang, Q., Zhang, Q., Jiang, X., Wang, X., Li, Y., et al. (2020). Rapid detection of cytochrome cd1-containing nitrite reductase encoding gene nirS of denitrifying bacteria with loop-mediated isothermal amplification assay. Sci. Rep. 10:16484. doi: 10.1038/s41598-020-73304-9
Zhang, X., Ye, L., Kang, Z., Zou, J., and Li, X. (2019). Mycorrhization of Quercus acutissima with Chinese black truffle significantly altered the host physiology and root-associated microbiomes. PeerJ 7:e6421. doi: 10.7717/peerj.6421
Keywords: Tuber indicum, Tuber melanosporum, Quercus aliena, ectomycorrhizosphere, denitrifying bacteria, growth of host plants, soil properties
Citation: Kang Z, Li X, Li Y, Ye L, Zhang B, Zhang X, Penttinen P and Gu Y (2022) Black Truffles Affect Quercus aliena Physiology and Root-Associated nirK- and nirS-Type Denitrifying Bacterial Communities in the Initial Stage of Inoculation. Front. Microbiol. 13:792568. doi: 10.3389/fmicb.2022.792568
Received: 10 October 2021; Accepted: 22 March 2022;
Published: 28 April 2022.
Edited by:
Yongchun Li, Zhejiang Agriculture and Forestry University, ChinaCopyright © 2022 Kang, Li, Li, Ye, Zhang, Zhang, Penttinen and Gu. This is an open-access article distributed under the terms of the Creative Commons Attribution License (CC BY). The use, distribution or reproduction in other forums is permitted, provided the original author(s) and the copyright owner(s) are credited and that the original publication in this journal is cited, in accordance with accepted academic practice. No use, distribution or reproduction is permitted which does not comply with these terms.
*Correspondence: Yunfu Gu, Z3V5ZkBzaWNhdS5lZHUuY24=; Xiaolin Li, a2VycnlsZWVfdHdAc2luYS5jb20=
†These authors have contributed equally to this work