- 1Institute of Plant Protection, Shandong Academy of Agricultural Sciences, Jinan, China
- 2Key Laboratory of Natural Enemies Insects, Ministry of Agriculture and Rural Affairs, Jinan, China
- 3Shandong Provincial Engineering Technology Research Center on Biocontrol of Crop Diseases and Insect Pest, Jinan, China
- 4Shandong University of Traditional Chinese Medicine, Jinan, China
- 5Shandong Provincial Key Laboratory of Applied Microbiology, Ecology Institute, Qilu University of Technology (Shandong Academy of Sciences), Jinan, China
- 6Department of Plant Protection, Shandong Agricultural Technology Extension Center, Jinan, China
Continuous cropping of watermelon (Citrullus lanatus) may lead to soil degradation. As a soil conditioner, microbial agent has great potential in improving soil function and enhancing plant growth. In this study, we aimed to explore how microbial agent relieves the soil sickness of watermelon by analyzing watermelon performance, soil physicochemical properties and microbial community structures. Results suggested that microbial agent treatments significantly changed the photosynthetic efficiency of upper and lower leaves, which helped improve the growth of watermelon. The single fruit weight, fruit sugar degree and total phosphorus of soil following treatment with a mixture of Paecilomyces lilacinus DZ910 and Bacillus subtilis KC1723 (treatment D_K) were higher than those in single biofertilizer treatments and control. The soil microbial community under microbial agent treatments also changed significantly, indicating the feasibility of using microbial agents as soil remediations. The proportions of Pseudomonas and Flavobacterium, changed significantly after using microbial agents. Pseudomonas increased significantly after B. subtilis KC1723 and D_K treatments, while Flavobacterium increased significantly after using all three kinds of microbial agents compared to control. Increases in these bacteria were positively correlated with agronomic variables of watermelon. The fungi Aspergillus and Neocosmospora in the soil, which create an soil sickness of watermelon, decreased after KC1723 and D_K treatments. Meanwhile, Aspergillus and Neocosmospora were positively related to Myceliophthora incidence and negatively correlated with watermelon growth (single fruit weight and photosynthetic efficiency of upper leaves). Our microbial agent, especially D_K, represents a useful technique for alleviating soil sickness in watermelon.
Introduction
Watermelon (Citrullus lanatus) is an annual plant belonging to the family of Cucurbitaceae and is an important fruit crop grown worldwide (Guo et al., 2013). Watermelon flesh is rich in nutritional compounds, such as sugars, vitamins and other minerals that play important roles in human metabolism (Ding et al., 2021). Because of the high economic benefit, watermelon has been cultivated continuously in the same fields by farmers. Previous studies have shown that long-term continuous cropping may cause changes in soil fertility, enzyme activities, microbial communities and nematode communities (Chen et al., 2020; Zla et al., 2020).
Microbial agents, such as biopesticides and biofertilizers, are becoming widely used by people in agricultural practice, and should be expected to play an increasing role in the development of sustainable agriculture (Kowalska et al., 2020). Microorganisms are material and energy carriers in the soil and also essential indicators of soil health (Hermans et al., 2017; Mcik et al., 2020; Chen et al., 2022). Healthy growth of crops is inseparable from a healthy soil microbial community structure (Chen et al., 2022). Soil microbial community structure in agricultural production is mainly influenced by agricultural management practices (Meriles et al., 2009; Liu et al., 2020). The primary agricultural practice of continuous cropping changes the soil microbial community structure (Zhang et al., 2018), decreasing bacterial biomass and increasing fungal biomass, leading to the dominant microorganisms altered from bacteria to fungi (Hu et al., 2010; Dong et al., 2016). Therefore, we need to adjust the soil properties used for continuous cropping by adding the necessary nutrients. Many strategies have been proposed to control continuous cropping problems, including crop rotation, soil sterilization and compost addition (Everts and Himmelstein, 2015; Xu et al., 2015; Ding et al., 2021). However, these strategies have their own limitations. Crop rotation takes a long time to play a regulatory role, soil sterilization might cause environmental damage, and compost releases nutrients slowly and has a low nutrient content (Grey and Henry, 1999; Bailey and Lazarovits, 2003; Tian et al., 2009). Microbial agents are composed of a large number of beneficial microorganisms, which can improve soil structure and affect plant growth (Boraste et al., 2009; Bhardwaj et al., 2014). The application of microbial agents can improve soil biological activity and the soil properties (Shah et al., 2001; Kolodziejczyk, 2014), so it is play a crucial role in sustainable agricultural development and are an indispensable technical measure for improving the quality of cultivated land (Ge et al., 2003). Supporters of microbial agent have been proving their beneficial effect on agricultural production for a long time (Xu, 2001). Therefore, forming bacteriostatic soil by increasing beneficial bacteria should suppress soil-borne diseases (Zhang et al., 2020). According to existing findings, we hypothesized that application of our microbial agent can promote the productivity of watermelon plants and improve soil quality, such as changing soil fertility, increasing beneficial microorganisms and decreasing harmful microorganisms.
Ding et al. recently reported that the application of urban waste compost alleviates soil problems associated with the continuous cropping of watermelon (Ding et al., 2021). However, there are few articles concerning the application of microbial agents for alleviating soil degradation caused by continuous cropping of watermelon. Thus, the purpose of this study was to assess the effectiveness of Bacillus subtilis and Paecilomyces lilacinus, these two strains exhibited inhibitory activity against a variety of plant pathogens (Li et al., 2022a,b), in alleviating soil degradation caused by continuous cropping and improving watermelon yield. We analyzed and compared soil chemical characteristics, microbial community structures, photosynthetic characteristics of watermelon leaves, and watermelon fruit quality and yield. Using high-throughput sequencing technology, we analyzed changes in soil microbial community structures to explore how microbial agents affect soil quality under continuous cropping.
Materials and methods
Source of microbial agent
The microbial agent contained two main components, Paecilomyces lilacinus DZ910 and Bacillus subtilis KC1723. P. lilacinus DZ910 was isolated from Zibo, Shandong Province, China. B. subtilis KC1723 was isolated from Jinan, Shandong Province, China. P. lilacinus DZ910 and B. subtilis KC1723 strains were deposited in the China General Microbiological Culture Collection Center under preservation numbers CGMCC 40079 and CGMCC 24397, respectively.
Site descriptions and sampling
Watermelon was planted in a solar greenhouse located in Renfeng Town, Jinan City, China (37.16° N, 117.36° E) on January 13, 2022. The test site had a planting density of 36,000 plants per hm2 and has been used for growing watermelons for more than a decade. The soil is considered a fluvo-aquic type. The characteristics of the soil are as follows: pH, 7.76 ± 0.12; soil organic matter, 23.7 ± 0.6 g/kg. Ridge planting, conventional drip irrigation. All the treatments were managed in the same way.
The experiment consisted of four treatments: CK (untreated control), DZ910 (P. lilacinus DZ910), KC1723 (B. subtilis KC1723), D_K (mixture of P. lilacinus DZ910 and B. subtilis KC1723).Fungal strains were grown in PDA medium (P. lilacinus DZ910) and LB medium (B. subtilis KC1723). Conidial suspensions were prepared in sterile distilled water. Fungal conidial suspensions of P. lilacinus DZ910 (1 × 109/mL), B. subtilis KC1723 (1 × 1011/mL), and a combined suspension of DZ910 and KC1723 (1 × 108/mL + 1 × 1010/mL, respectively) were prepared and used for experiments. The fungal conidial suspension used in this study was 90 l/hm2, and diluted 10 times when used. Before transplanting, approximately 50 ml diluted suspension solution was poured into each hole. Watermelons of uniform size were used for this experiment. The daily field management of all treatments was consistent. Each treatment was replicated three times.
Photosynthetic efficiency and virulence assays
Three months after application of different microbial agents, chlorophyll content and photosynthesis parameters were measured using a portable instrument (Handy PEA, Hansatech). Three partitioned blades were tested, the lower blade (LB) was 50 cm away from the ground, the middle blade (MB) was 100 cm away, and the upper blade (UB) was 150 cm away. The experiment was repeated three times independently with 20 replicates each.
Virulence assays were performed at the same time. Ten leaves per plant were examined from 50 cm above the ground. According to the proportion of diseased area to total area, the disease index (DI) was classified into the following grades: 1, ≤5%; 3, 6–10%; 5, 11–25%; 7, 26–50%; 9, ≥50%. DI = Σ(number of disease plants×corresponding disease grade value)/(total number of plants investigated×highest value) × 100. The experiment was repeated three times independently with 15 replicates each.
Yield and fruit quality
Ten plants from each treatment were randomly assigned to assess watermelon fruit on April 19, 2022. The fruit were weighed and then used for quality determination. Fruit firmness, soluble solid content and sugar content were detected using the appropriate instruments following the corresponding operation instructions.
Determination of soil physicochemical properties
Total nitrogen (TN) contents were measured using an automatic Kjeldahl distillation titration unit (Foss, Sweden). Total phosphorus (TP) was digested with H2SO4-HClO4 (Miranda et al., 2001). Total potassium (TK) was digested with HNO3-HClO4-HF (Shen et al., 2013). Soil total organic matter (OM) was assayed according to the vitriol acid potassium dichromate oxidation method (Nelson and Sommers, 2018).
DNA extraction and sequencing of soil microbial communities
Soil total DNA was extracted from 500 mg of fresh soil using a PowerSoil® DNA Isolation Kit (MO Bio Laboratories, San Diego, CA, USA) according to the manufacturer’s protocol. The bacterial universal primer pair 338F (5′-GTACTCCTACGGGA GGCAGCA-3′) and 806R (5′-GTGGACTACHVGGGTW TCTAAT-3′) targeting the V3-V4 region of the 16S rRNA gene was used to characterize soil bacterial communities (Kim et al., 2011). The fungal universal primer pair ITS1-F (5′-CTTGGTCATTTAGAGGAAGTAA-3′) and ITS2-R (5′-TGCGTTCTTCATCGATGC-3′) targeting the internal transcribed spacer (ITS) region was used to identify soil fungal communities (Martin, 2011).
The 16S rRNA gene and ITS regions in the DNA samples were amplified by polymerase chain reaction (PCR) before sequencing. The PCR was performed in a total volume of 50 μl, containing 2 μl of template DNA, 5 μl of 10 × buffer, 2 μl of 10 μM forward primer, 2 μl of 10 μM reverse primer, 4 μl of 2.5 μM dNTPs, 0.3 μl of DNA polymerase (2.5 U μL−1), and 34.7 μl of ddH2O. Reaction conditions were 5 min at 95°C, followed by 30 cycles (for the bacterial 16S rRNA gene) or 35 cycles (for the fungal ITS region) of 30 s at 95°C, 30 s at 56°C and 40 s for elongation at 72°C, followed by a final extension at 72°C for 10 min and a hold at 10°C.
All PCR amplicons were examined using 2% (w/v) agarose gels. Purified DNA was quantified using a QuantiFluor™-ST (Promega, USA) and subsequently sequenced (PE300) using the Illumina MiSeq platform (Illumina Inc., USA) by Shanghai Majorbio Bio-pharm Technology Co., Ltd. Operational taxonomic units (OTUs) were assigned at a threshold of 97% similarity level and clustered using UPARSE (version 7.1) (Edgar, 2013). Chimeric sequences were identified and removed using UCHIME (Knight, 2011). Representative sequences of each OTU were taxonomically classified using the Silva and Unite database (Quast et al., 2012). Sequences matching the archaea, mitochondria and chloroplasts were removed and the number of sequences were rarefied to 32,650 reads per sample. The sequencing datasets supporting the current study are available in the National Center for Biotechnology Information (NCBI) under BioProject accession number PRJNA908896.
Data analysis
Data were analyzed by one-way analysis of variance (ANOVA) using SPSS 20.0 (SPSS Inc., USA). Alpha-diversity analyses, including ACE index, Chao index and Shannon index, were calculated using Mothur software (Quast et al., 2012). Taxonomic dissimilarity analysis between samples was based on the PCoA method with Bray-Curtis distances (beta diversity) (Lozupone et al., 2011). The R language vegan package (version 3.3.1) was used to draw community heat maps. The relationship between genus diversity and soil physicochemical properties was analyzed using redundancy analysis (RDA) by Canoco for Windows (ver. 4.5). The significance level for differences was set at p ≤ 0.05.
Results
Effects of microbial agents on watermelon agronomic variables
We measured chlorophyll content, photosynthetic efficiency and disease index of leaves to assess the growth of plants under different treatment (Figures 1A,B; Supplementary Figure S1a). Microbial agents had no obvious effect on chlorophyll content (Figure 1A). The photosynthetic efficiency of upper leaves (PE-up) and lower leaves (PE-low) were significantly higher under P. lilacinus (DZ910), B. subtilis (KC1723) and mixed microbial agent (D_K) treatments than in the control (CK) (p < 0.05) (Supplementary Figure S1a). The disease index of leaves was significantly lower under DZ910 and D_K treatments than under CK and KC1723 treatments (p < 0.05) (Figure 1B).
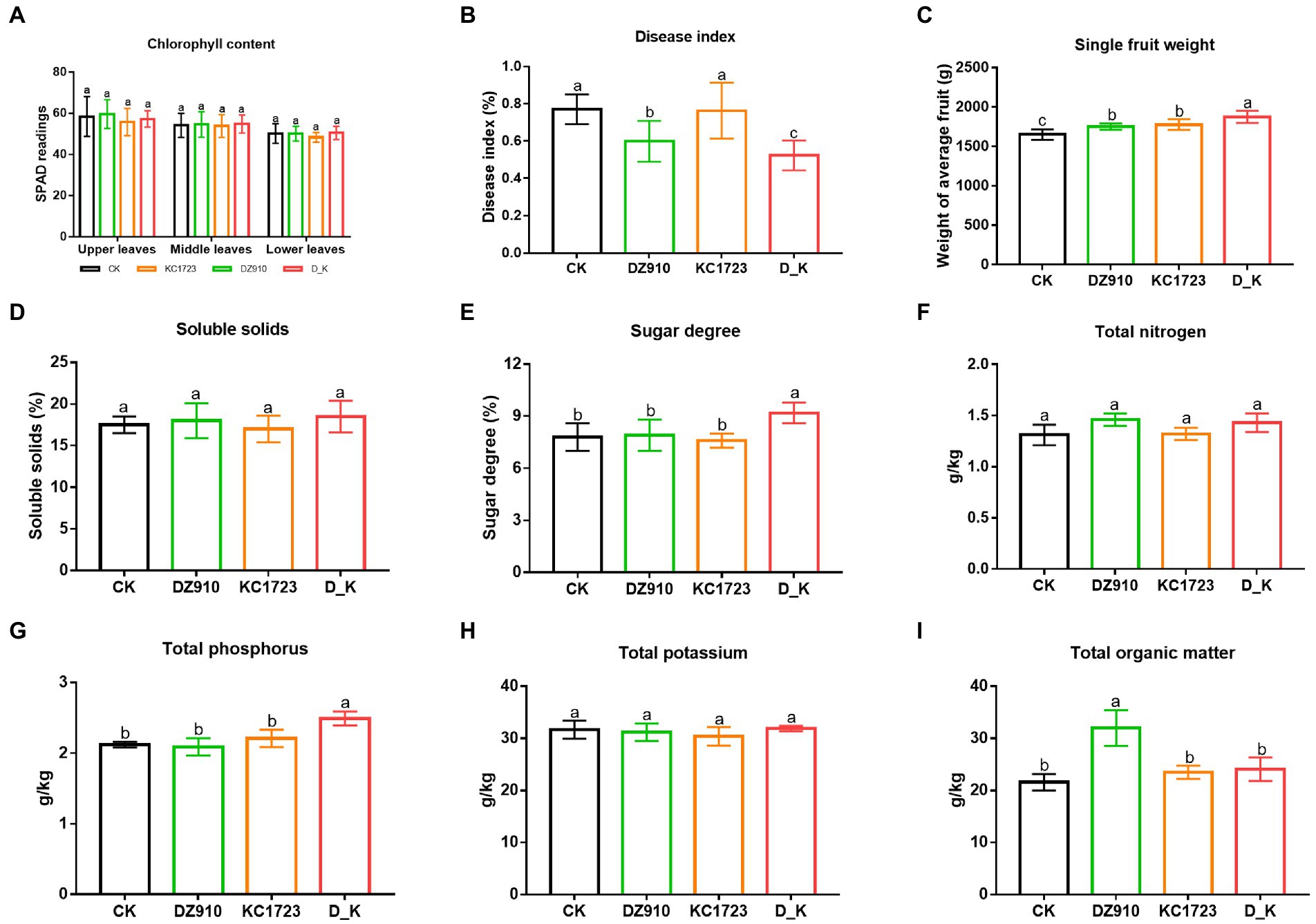
Figure 1. Changes of watermelon performance and soil physicochemical properties in different treatments. (A) chlorophyll content, (B) disease index, (C) SWF, (D) soluble solids, (E) sugar degree, (F) total nitrogen, (G) total phosphorus, (H) total potassium, and (I) total organic matter. The letters above the values indicate significant differences in different treatments (analysis of variance [ANOVA]: Duncan test; p < 0.05).
The single fruit weight (SWF), soluble solids and sugar degree were determined to assess the growth of watermelon fruit (Figures 1C–E; Supplementary Figure S1b). The D_K treatment produced the highest SWF, which was significantly higher than that in other treatments (Figure 1C; Supplementary Figure S1b). Sugar degree was significantly higher in the D_K treatment compared with the other treatments (p < 0.05) (Figure 1E). However, there were no significant differences in the soluble solids of fruit among different treatments (Figure 1D).
Changes in soil physicochemical properties
The effects of using P. lilacinus (DZ910), B. subtilis (KC1723) and the mixed microbial agent (D_K) on soil physicochemical characteristics are shown in Figure 1. Contents of TN and TK did not differ significantly between treatments (Figures 1F,G). TP in the D_K treatment was significantly higher than that in the other treatments (Figure 1H). Likewise, soil OM in the DZ910 treatment was significantly higher than that in other treatments (Figure 1I).
Soil bacterial and fungal alpha diversities
We evaluated and compared the richness and diversity of bacterial and fungal communities in the different soil samples using Chao1, Shannon and ACE indices (Figure 2). As shown in Figures 2A,D, the Chao1 estimator for the bacterial community showed no significant difference between samples, whereas a lower Chao1 estimator for the fungal community was observed in the soil treated with KC1723. The Shannon index of the bacterial community was higher in the sample under DZ910, KC1723 and D_K treatments than under CK, with the highest value in the soil treated with DZ910 (Figure 2B). However, the Shannon index for the fungal community showed a lower value in the soil under KC1723 and D_K treatment (Figure 2E). The ACE index value for the bacterial community was significantly higher under DZ910 treatment than under other treatments, while the ACE index value for the fungal community was significantly lower under KC1723 treatment than under other treatments (Figures 2C,F).
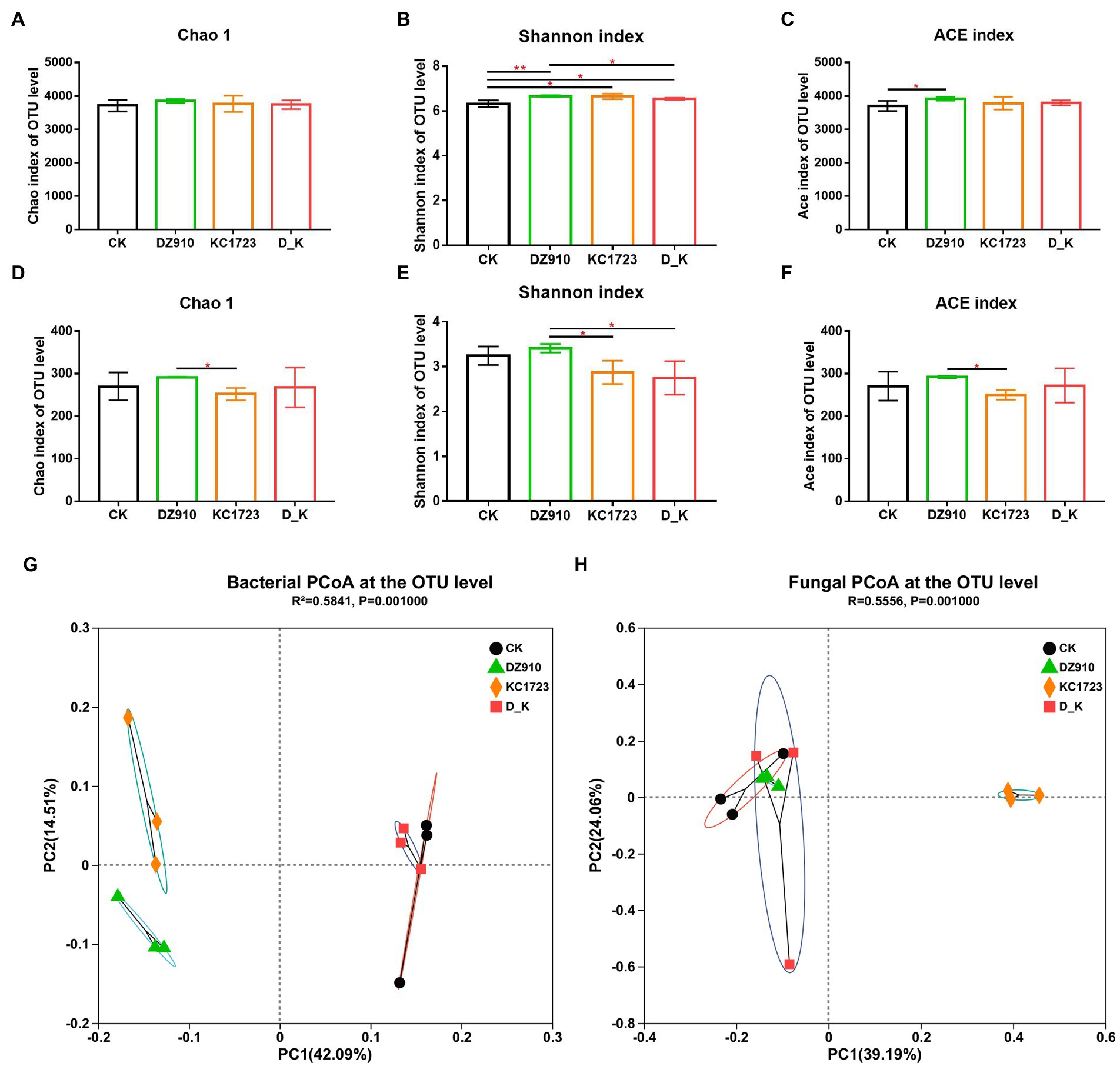
Figure 2. Changes in soil microbial alpha and beta diversities. (A–C) bacterial Chao1, Shannon and ACE indices, (D–F) fungal Chao1, Shannon and ACE indices, (G) PCoA clustering analysis of bacteria, (H) PCoA clustering analysis of fungi. Error bars indicate mean ± SE. p-values by T-test indicated significant differences, and the symbol (*) indicated that 0.01 < p ≤ 0.05, (**) indicated that 0.001 < p ≤ 0.01.
Soil bacterial and fungal beta diversities
The results of principal coordinate analysis (PCoA) of different soil samples are also shown in Figure 2. Variation in bacterial and fungal community composition was affected by 11 principal coordinate components, respectively (Figures 2G,H). In terms of bacteria, the cumulative contribution rates of the two maximally reflected differences were 42.09 and 14.51% and the cumulative explanatory variation was 56.6% (Figure 2G). The distance between CK and D_K treatments was small, and they grouped together. DZ910 and KC1723 treatments were both far away from CK and D_K treatments. In fungi, the two main coordinates extracted explained 63.25% of the variation, of which 39.19% was explained by PC1 and 24.06% by PC2 (Figure 2H). Similarly, the results showed that CK, DZ910 and D_K treatments gathered together. However, the KC1723 treatment was far away from CK, DZ910 and D_K treatments.
Microbial community structures of different treatments
The bacterial and fungal populations in the different treatments were analyzed by high-throughput sequencing. In this study, a total of 26 bacterial genera and 20 fungal genera (relative abundance >1%) were detected in the different soil samples (Supplementary Tables S1, S2). To further analyze the relative abundance of the microbial community, we found that 3 identified bacterial genera, including Dongia, Pseudomonas and Flavobacterium, and five identified fungal genera, Aspergillus, Myceliophthora, Trichoderma, Humicola and Neocosmospora, showed significant differences in the soil under different treatments (Supplementary Tables S1, S2; Figure 3). In the bacterial community, OTUs belonging to Dongia were decreased in DZ910 and KC1723 treatments, but showed no significant change in D_K compared with those in CK (Figure 3). The abundance of Pseudomonas increased significantly in KC1723 and D_K, and the abundance of Flavobacterium increased significantly in all microbial agent treatments (DZ910, KC1723 and D_K) compared with those in CK (Figure 3). Within the fungal community, the abundance of Aspergillus decreased significantly in KC1723 and the abundance of Myceliophthora and Neocosmospora decreased significantly in KC1723 and D_K compared to those in CK (Figure 3). However, the abundance of Trichoderma and Humicola increased significantly in the KC1723 treatment compared to CK (Figure 3).
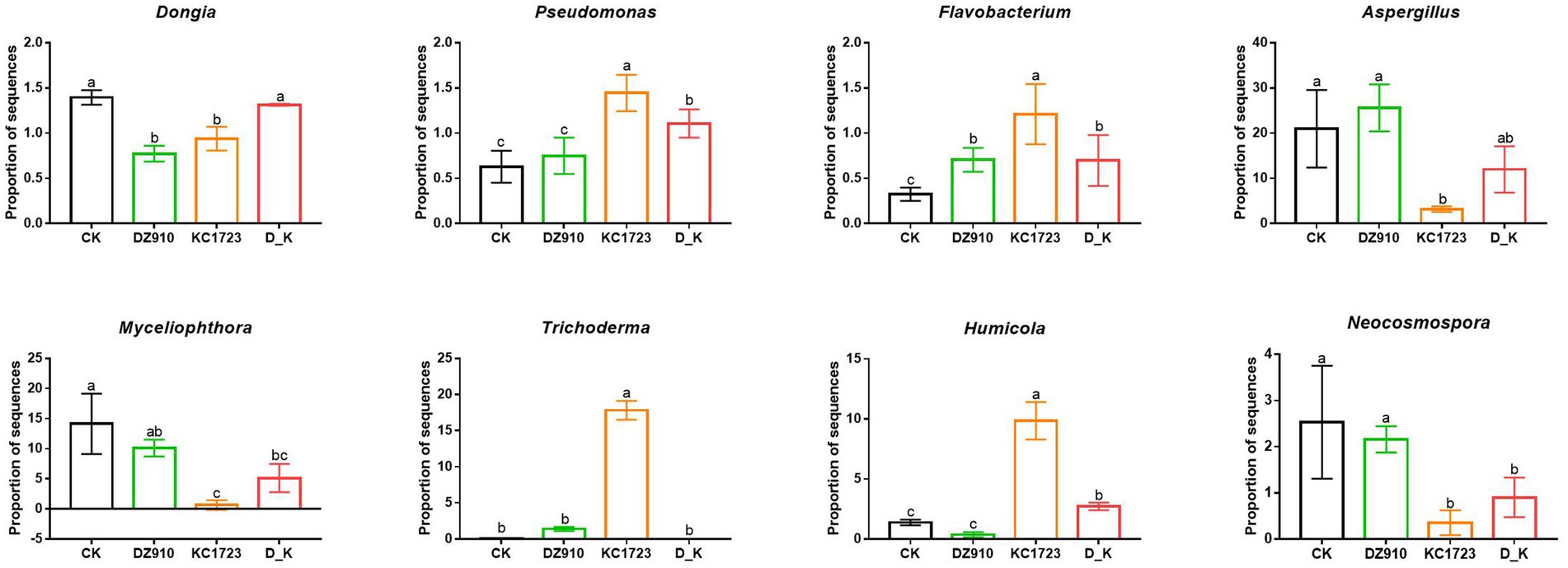
Figure 3. Effects of different microbial agents on several key microorganisms. The letters indicate significant differences among the different treatments (analysis of variance [ANOVA]: Duncan test; p < 0.05).
Relationships of microbial community with physicochemical properties
We constructed a correlation heatmap between microbial community at the genus level and physicochemical properties using Spearman’s correlation analysis (Figure 4). Among the top 50 most abundant bacterial genera, 16 genera were affected by at least one physicochemical property (Figure 4A). Nitrospira, Chryseolinea and norank_f_67–14 were significantly negatively correlated with TN. Norank_f_norank_o_norank_c_KD4-96 was significantly negatively correlated with TP. Nine genera were affected by TK, one of which was negatively affected by TK, while the other eight genera, Dongia, unclassified_k_norank_d_Bacteria, norank_f_JG30-KF-CM45, Nonomuraea, norank_f_AKYG1722, Streptomyces, Steroidobacter and norank_f_norank_o_Rokubacteriales, were positively correlated with TK. Four genera, norank_f_TRA3-20, norank_f_norank_o_Actinomarinales, MND1 and norank_f_67–14, were significantly negatively correlated with OM.
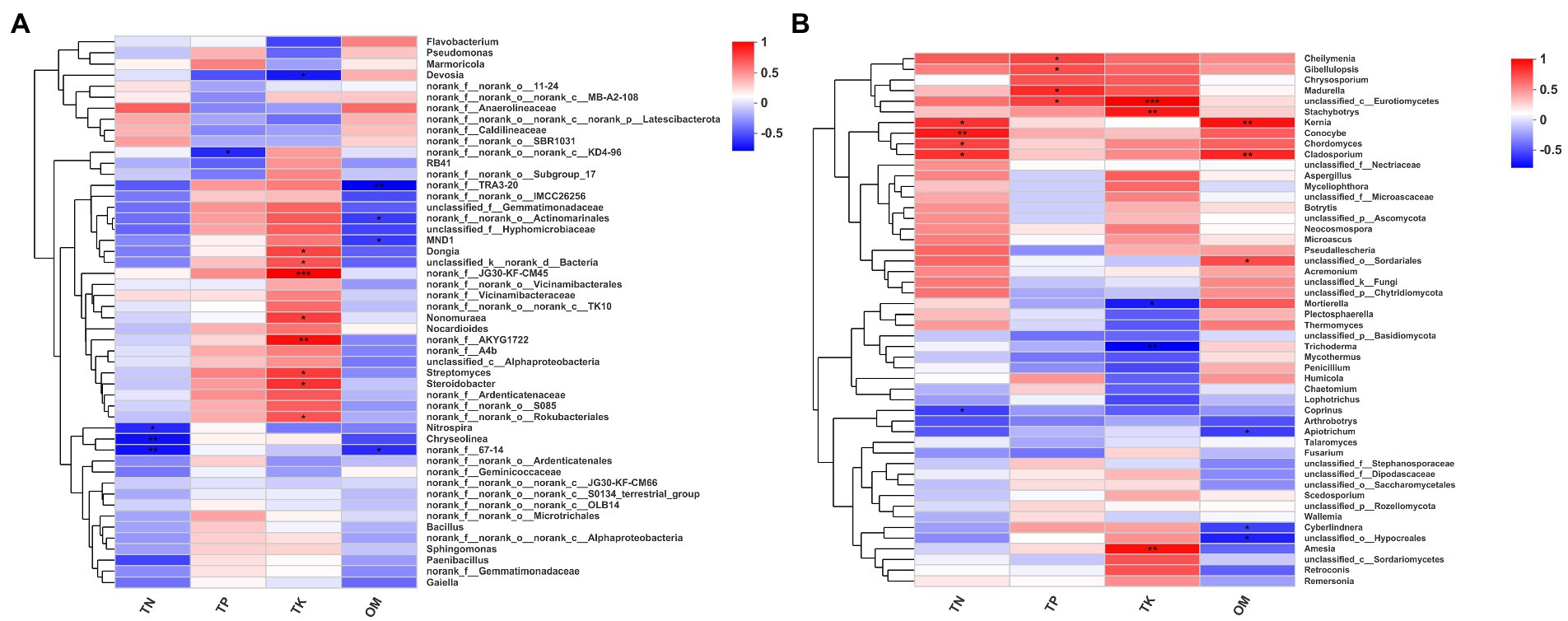
Figure 4. Correlation heat map of microbial classification and environmental factors. (A) Correlation heat map of the top fifty genera (bacteria) and environmental factors. (B) Correlation heat map of the top fifty genera (fungi) and environmental factors. The symbol (*) indicated that 0.01 < p ≤ 0.05, (**) indicated that 0.001 < p ≤ 0.01, (***) indicated that p ≤ 0.001.
In the top 50 fungal genera, 17 genera were affected by at least one physicochemical property (Figure 4B). Kernia, Conocybe, Chordomyces and Cladosporium were significantly positively correlated with TN, and Coprinus was significantly negatively correlated with TN. Cheilymenia, Gibellulopsis, Madurella and unclassified_c_Eurotiomycetes were significantly positively correlated with TP. Three genera, unclassified_c_Eurotiomycetes, Stachybotrys and Amesia, were significantly positively affected by TK, and two genera, Mortierella and Trichoderma were significantly negatively affected by TK. Six genera were affected by OM: Kernia, Cladosporium, unclassified_o_Sordariales, Apiotrichum, Cyberlindnera and unclassified_o_Hypocreales.
Network analysis of physicochemical properties, major microorganisms and agronomic variables
We conducted network analysis among physicochemical properties, major microorganisms and agronomic variables to assess their relationships. There were 22 nodes and 44 edges in the network (Figure 5). TK was the physicochemical characteristic most related to microorganisms. It was positively related to Dongia, Amesia and Retroconis, and negatively related to Trichoderma and Humicola. TN was strongly associated with Nitrospira, which was similar to the results of the correlation heatmap (Figure 5). Interestingly, two bacterial genera, Pseudomonas and Flavobacterium, were positively related to SWF, PE-up and PE-low. At the same time, a positive correlation was observed between them. The fungal genus Myceliophthora was positively related to Neocosmospora and Aspergillus, and they were all negatively related to SWF and PE-up.
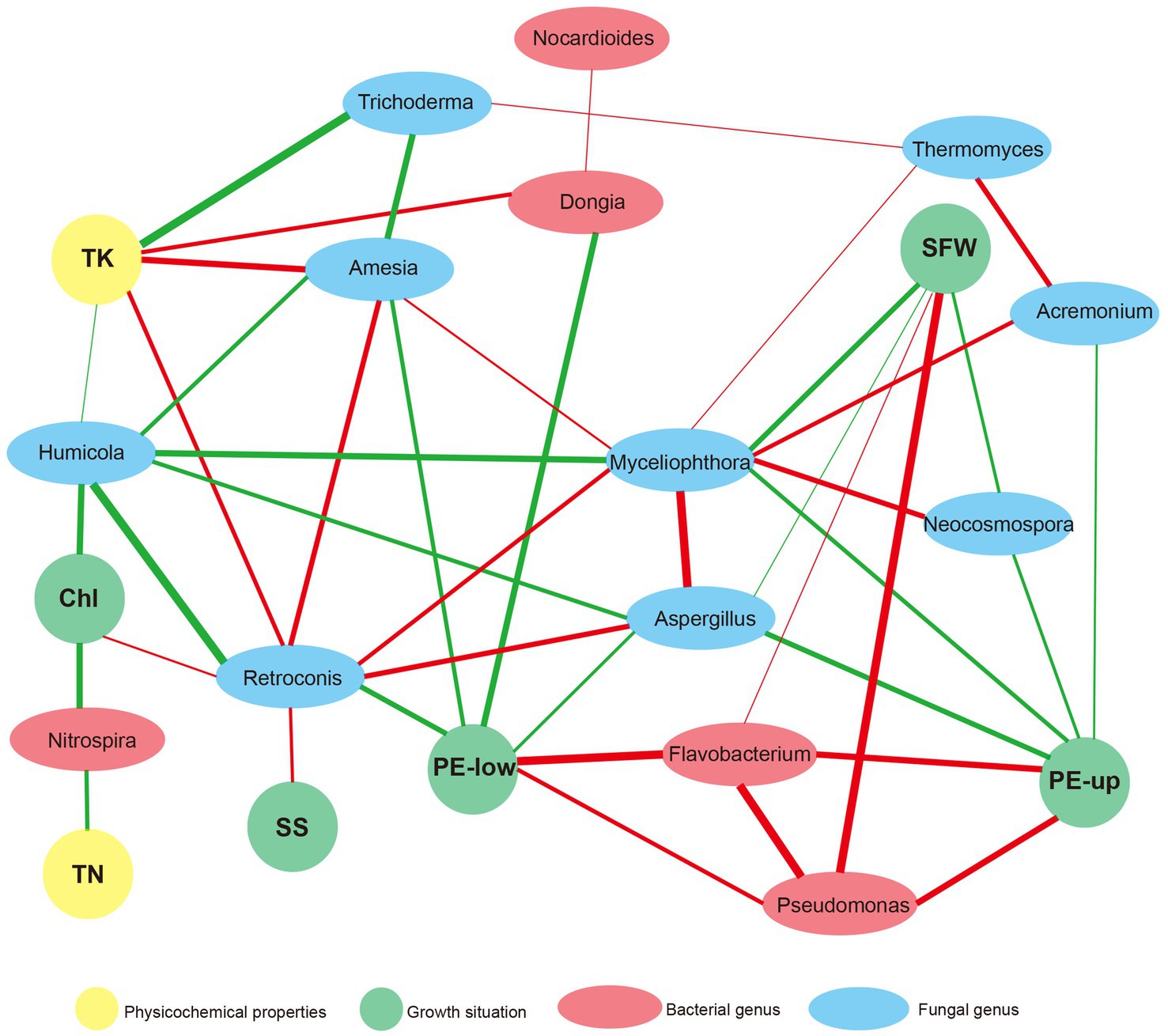
Figure 5. Network analysis based on the co-occurrence of physicochemical properties, major microorganisms and the agronomic variables. The agronomic variables, such as PE-up and PE-low leaves, SFW, sugar degree (SS) and chlorophyll content (Chl), were shown in green ellipses. The physicochemical properties, TK and TN were shown in yellow ellipses. The major bacteria, including Dongia, Pseudomonas, Flavobacterium, Nitrospira, and Nocardioides, were shown in red ellipses, and the major fungus, such as Aspergillus, Myceliophthora, Trichoderma, Humicola, Neocosmospora, Retroconis, Acremonium, Amesia and Thermomyces, were shown in gray ellipses. A connection represents a significant correlation (p < 0.05) according to Spearman’s analysis. The red lines denote positive correlations and the green lines mean negative correlations (the darker the color the stronger the correlation).
Discussion
Previous studies indicated that obstacles to continuous cropping are caused by long-term monoculture cropping leading to a reduction in soil quality and crop productivity (Qin et al., 2017; Chen et al., 2020). There are many complex factors contributing to obstacles to continuous cropping, including changes in soil physicochemical properties, increases in autotoxic substances and changes in microbial communities in soil (Li et al., 2018; Li and Liu, 2019). Application of microbial agents is one way to relieve soil sickness and increase the yield and quality of agricultural products (Mandrik et al., 2020). In this study, application of our microbial agents effectively improved the growth of watermelon and soil degradation caused by continuous cropping.
Chlorophyll content, photosynthetic efficiency and disease index help to assess the growth of crops (Cheng et al., 2012). In this study, three types of microbial agents increased the PE-up and PE-low, which helped to improve the growth of watermelon. The microbial agents are reported to promote photosynthesis, thereby increasing net photosynthetic rate (Agamy et al., 2012; Duan et al., 2021). As shown in Figures 1C,E, both SWF and sugar degree were higher under D_K treatment than under other treatments. This indicates that B. subtilis and P. lilacinus synergistically promote plant photosynthetic efficiency, resulting in increased SWF and sugar degree.
Soil physicochemical properties (TN, TP, TK and OM) are very important properties in soil ecosystems that not only reflect soil health and quality, but also act as ideal indicators for assessing the growth of crops (Lemaire et al., 2019; Chen et al., 2020). TP improved significantly after applying a mixture of P. lilacinus DZ910 and B. subtilis KC1723 (D_K) (Figures 1F–I). K fertilizer is a essential nutrient in soil for plant growth and plays vital roles in the yield and quality of agricultural crops (Song et al., 2020). In this study, application of D_K might have increased soil total phosphorous and promoted watermelon growth.
Microorganisms also play a vital role in maintaining plant health (Cardinale et al., 2006; Diacono and Montemurro, 2011). Changes in the diversity and composition of soil microorganisms has a considerable influence on soil ecosystems (Alami et al., 2021). Application of different microbial agents had a significant influence on the composition, diversity and structure of the bacterial and fungal communities in the soil (Figures 2, 3; Supplementary Tables S1, S2). Only three bacterial genera (greater than 1%), Dongia, Pseudomonas and Flavobacterium, showed significant differences under different treatments (Figure 3). Network analysis showed that Pseudomonas and Flavobacterium were positively related to the growth of watermelon (Figure 5). Application of KC1723 and D_K significantly increased the relative abundance of Pseudomonas. Previous studies found that Pseudomonas is a plant-beneficial bacteria, and its abundance is significantly depleted after continuous cropping (Rumberger, 2007; Xiong et al., 2015). At the same time, the application of microbial agents also significantly increased the relative abundance of Flavobacterium in soil. This is thought to be correlated with the capacity to degrade complex organic compounds (Kolton et al., 2016). The abundance of Flavobacterium in rhizosphere soil is positively related to fruit maturation and plant resistance (Sang and Kim, 2012; Kolton et al., 2014; Zhou et al., 2022). Therefore, Pseudomonas and Flavobacterium might be significant factors for watermelon growth. Five fungal genera (greater than 1%), Aspergillus, Myceliophthora, Trichoderma, Humicola and Neocosmospora, showed significant differences after the application of different microbial agents (Figure 3). Network analysis indicated that Aspergillus, Myceliophthora and Neocosmospora are negatively related to SWF and PE-up (Figure 5). Chen et al. found that the ratio of Aspergillus increased in soil of Panax quinquefolium following continuous cropping (Chen et al., 2012). In our study, application of KC1723 significantly decreased the abundance of Aspergillus in the soil. The mix microbial agent D_K contains KC1723, and could also reduce the content of Aspergillus in the soil. Neocosmospora is an important genus of phytopathogens, causing stem rot in many crops, such as soybean and potato (Greer et al., 2015; Riaz et al., 2022a,b). This fungus symptomatically causes stunted growth and typical grayish-black streaking on plants (Riaz et al., 2022a,b). With the spread of Neocosmospora fungus, plants gradually wilt and then die (Riaz et al., 2020). In our study, application of KC1723 and D_K effectively reduced the abundance of Neocosmospora in watermelon continuous cropping soil, which probably explains why using microbial agents, especially KC1723 and D_K, can alleviate problems with watermelon continuous cropping.
Collectively, microbial agents have great potential in continuous cropping soil remediation. In this study, microbial agents (DZ910, KC1723 and D_K) improved watermelon growth, soil physicochemical properties and microbial community structures. The D_K treatment, which combined suspensions of DZ910 and KC1723, showed the best continuous cropping soil remediation effect.
Conclusion and perspectives
This study revealed that application of microbial agents (DZ910, KC1723 and D_K) can relieve the obstacles of continuous cropping to watermelon cultivation. Agronomic variables of watermelon, such as PE-up and PE-low, SWF and sugar degree of fruit, and TP of soil under D_K treatment changed significantly. Soil microbial communities under microbial agent treatments also changed significantly, indicating the feasibility of microbial agents as soil remediations. Levels of several bacteria in the soil, such as Pseudomonas and Flavobacterium, and fungi, Aspergillus and Neocosmospora, changed significantly after using microbial agents. Besides, Pseudomonas and Flavobacterium were positively correlated with the growth of watermelon. Aspergillus and Neocosmospora were positively related to Myceliophthora and negatively correlated with watermelon growth (SWF and PE-up). Above all, our results provide a useful technique for relieving obstacles to continuous cropping of watermelon.
Data availability statement
The datasets presented in this study can be found in online repositories. The names of the repository/repositories and accession number(s) can be found below: NCBI Sequence Read Archive Project - PRJNA908896.
Author contributions
JL and XG conceived and designed the research. PC, JZ, ML, JH, AZ, ZS, and FF performed the experiments. PC, JH, ZS, and AZ collected samples and performed data acquisition. PC, JZ, and JL analyzed the data and wrote the main manuscript text. All authors contributed to the article and approved the submitted version.
Funding
This research was supported by the National Key Research and Development Program of China (no. 2021YFD1700101) and Key Technology Research and Development Program of Shandong (no. 2021CXGC010811).
Conflict of interest
The authors declare that the research was conducted in the absence of any commercial or financial relationships that could be construed as a potential conflict of interest.
Publisher’s note
All claims expressed in this article are solely those of the authors and do not necessarily represent those of their affiliated organizations, or those of the publisher, the editors and the reviewers. Any product that may be evaluated in this article, or claim that may be made by its manufacturer, is not guaranteed or endorsed by the publisher.
Supplementary material
The Supplementary material for this article can be found online at: https://www.frontiersin.org/articles/10.3389/fmicb.2022.1101975/full#supplementary-material
References
Agamy, R., Hashem, M., and Alamri, S. (2012). Effect of soil amendment with yeasts as bio-fertilizers on the growth and productivity of sugar beet. Afr. J. Agric. Res. 49, 6613–6623. doi: 10.5897/AJAR12.1989
Alami, M. M., Pang, Q., Gong, Z., Yang, T., Tu, D., Zhen, O., et al. (2021). Continuous cropping changes the composition and diversity of bacterial communities: a meta-analysis in nine different fields with different plant cultivation. Agriculture 11:1224. doi: 10.3390/agriculture11121224
Bailey, K. L., and Lazarovits, G. (2003). Suppressing soil-borne diseases with residue management and organic amendments. Soil Tillage Res. 72, 169–180. doi: 10.1016/S0167-1987(03)00086-2
Bhardwaj, D., Ansari, M., Sahoo, R., and Tuteja, N. (2014). Biofertilizers function as key player in sustainable agriculture by improving soil fertility, plant tolerance and crop productivity. Microb. Cell Factor. 13:66. doi: 10.1186/1475-2859-13-66
Boraste, A., Vamsi, K. K., Jhadav, A., Khairnar, Y., and Joshi, B. (2009). Biofertilizers: a novel tool for agriculture. Int. J. Microbiol. Res. 1, 23–31. doi: 10.9735/0975-5276.1.2.23-31
Cardinale, B. J., Srivastava, D. S., Emmett Duffy, J., Wright, J. P., Downing, A. L., Sankaran, M., et al. (2006). Effects of biodiversity on the functioning of trophic groups and ecosystems. Nature 443, 989–992. doi: 10.1038/nature05202
Chen, Y., Du, J., Li, Y., Tang, H., and Ding, X. (2022). Evolutions and managements of soil microbial community structure drove by continuous cropping. Front. Microbiol. 13:839494. doi: 10.3389/fmicb.2022.839494
Chen, P., Wang, Y. Z., Liu, Q. Z., Zhang, Y. T., and Li, W. H. (2020). Phase changes of continuous cropping obstacles in strawberry (Fragaria × ananassa Duch.) production. Appl. Soil Ecol. 155:103626. doi: 10.1016/j.apsoil.2020.103626
Chen, J., Zhang, X. S., Yang, J. X., Jiao, X. L., and Gao, W. W. (2012). Effect of continuous cropping and soil treatment on rhizosphere fungal community of Panax quinquefolium. Zhongguo Zhong Yao Za Zhi 37, 3531–3535. doi: 10.4268/cjcmm20122305
Cheng, X. H., Feng, X. X., Zhang, Z. P., Shen, M., and Wang, L. J. (2012). Effects of ‘Alstrong’ amino-acid fertilizer on photosynthetic efficiency and yield of strawberry in plastic tunnels. J. Fruit Sci. 29, 883–889. doi: 10.13925/j.cnki.gsxb.2012.05.032
Diacono, M., and Montemurro, F. (2011). “Long-term effects of organic amendments on soil fertility” in Sustainable Agriculture. eds. E. Lichtfouse, M. Hamelin, M. Navarrete, and P. Debaeke, vol. 2 (Dordrecht: Springer)
Ding, S., Zhou, D., Wei, H., Wu, S., and Xie, B. (2021). Alleviating soil degradation caused by watermelon continuous cropping obstacle: application of urban waste compost. Chemosphere 262:128387. doi: 10.1016/j.chemosphere.2020.128387
Dong, L., Xu, J., Feng, G., Li, X., and Chen, S. (2016). Soil bacterial and fungal community dynamics in relation to Panax notoginseng death rate in a continuous cropping system. Sci. Rep. 6:31802. doi: 10.1038/srep31802
Duan, W., Peng, L., Zhang, H., Han, L., and Li, Y. (2021). Microbial biofertilizers increase fruit aroma content of Fragaria × ananassa by improving photosynthetic efficiency. Alex. Eng. J. 60, 5323–5330. doi: 10.1016/j.aej.2021.04.014
Edgar, R. C. (2013). UPARSE: highly accurate OTU sequences from microbial amplicon reads. Nat. Methods 10, 996–998. doi: 10.1038/nmeth.2604
Everts, K. L., and Himmelstein, J. C. (2015). Fusarium wilt of watermelon: towards sustainable management of a re-emerging plant disease. Crop Prot. 73, 93–99. doi: 10.1016/j.cropro.2015.02.019
Ge, J. Q., Yu, X. C., and Wang, Z. H. (2003). The function of microbial fertilizer and its application prospects. Chin. J. Eco-Agric. 11, 87–88.
Greer, A., Spurlock, T., and Coker, C. (2015). First report of neocosmospora stem rot of soybean caused by Neocosmospora vasinfecta in Arkansas. Plant Dis. 99:554. doi: 10.1094/PDIS-05-14-0559-PDN
Grey, M., and Henry, C. (1999). Nutrient retention and release characteristics from municipal solid waste compost. Compost Sci. Utilizat. 7, 42–50. doi: 10.1080/1065657X.1999.10701951
Guo, S., Zhang, J., Sun, H., Salse, J., Lucas, W. J., Zhang, H., et al. (2013). The draft genome of watermelon (Citrullus lanatus) and resequencing of 20 diverse accessions. Nat. Genet. 45, 51–58. doi: 10.1038/ng.2470
Hermans, S. M., Buckley, H. L., Case, B. S., Curran-Cournane, F., Taylor, M., and Lear, G. (2017). Bacteria as emerging indicators of soil condition. Appl. Environ. Microbiol. 83, 2816–2826. doi: 10.1128/AEM.02826-16
Hu, H. Q., Li, X. S., and He, H. (2010). Characterization of an antimicrobial material from a newly isolated bacillus amyloliquefaciens from mangrove for biocontrol of capsicum bacterial wilt. Biol. Control 54, 359–365. doi: 10.1016/j.biocontrol.2010.06.015
Kim, M., Morrison, M., and Yu, Z. (2011). Evaluation of different partial 16S rRNA gene sequence regions for phylogenetic analysis of microbiomes. J. Microbiol. Methods 84, 81–87. doi: 10.1016/j.mimet.2010.10.020
Knight, R. (2011). UCHIME improves sensitivity and speed of chimera detection. Bioinformatics 27, 2194–2200. doi: 10.1093/bioinformatics/btr381
Kolodziejczyk, M. (2014). Effectiveness of nitrogen fertilization and application of microbial preparations in potato cultivation. Turkish Journal of Agriculture and Forestry 38, 299–310. doi: 10.3906/tar-1305-105
Kolton, M., Erlacher, A., Berg, G., and Cytryn, E. (2016). “The Flavobacterium genus in the plant Holobiont: ecological, physiological, and applicative insights” in Microbial Models: From Environmental to Industrial Sustainability. Microorganisms for Sustainability, 1 Edn. ed. S. Castro-Sowinski (Singapore: Springer).
Kolton, M., Frenkel, O., Elad, Y., and Cytryn, E. (2014). Potential role of Flavobacterial gliding-motility and type IX secretion system complex in root colonization and plant defense. Mol. Plant-Microbe Interact. 27, 1005–1013. doi: 10.1094/MPMI-03-14-0067-R
Kowalska, J., Tyburski, J., Matysiak, K., Tylkowski, B., and Malusá, E. (2020). Field exploitation of multiple functions of beneficial microorganisms for plant nutrition and protection: real possibility or just a Hope? Front. Microbiol. 11:1904. doi: 10.3389/fmicb.2020.01904
Lemaire, G., Sinclair, T., Sadras, V., and Bélanger, G. (2019). Allometric approach to crop nutrition and implications for crop diagnosis and phenotyping. A review. Agron. Sustain. Dev. 39:27. doi: 10.1007/s13593-019-0570-6
Li, J., Gao, X. X., Li, M., Fang, F., Sun, Z. W., and Xue, D. X. (2022a). A Bacillus subtilis strain and its application. CHN. Patent number ZL202210433671.1.
Li, J., Gao, X. X., Li, M., Sun, Z. W., Xue, D. X., and Fang, F. (2022b). A Paecilomyces lilacinus strain and its application. CHN. Patent number ZL202210433669.4.
Li, W. H., and Liu, Q. Z. (2019). Changes in fungal community and diversity in strawberry rhizosphere soil after 12 years in the greenhouse. J. Integr. Agric. 18, 677–687. doi: 10.1016/S2095-3119(18)62003-9
Li, W. H., Liu, Q. Z., and Chen, P. (2018). Effect of long-term continuous cropping of strawberry on soil bacterial community structure and diversity. J. Integr. Agric. 17, 2570–2582. doi: 10.1016/S2095-3119(18)61944-6
Liu, H., Pan, F. J., Han, X. Z., Song, F. B., and Yan-Li, X. U. (2020). A comprehensive analysis of the response of the fungal community structure to long-term continuous cropping in three typical upland crops. J. Integr. Agric. 19, 866–880. doi: 10.1016/S2095-3119(19)62630-4
Lozupone, C., Lladser, M. E., Knights, D., Stombaugh, J., and Knight, R. (2011). UniFrac: an effective distance metric for microbial community comparison. ISME J. 5, 169–172. doi: 10.1038/ismej.2010.133
Mandrik, M. N., Orlovskaya, P. I., Kislushko, P. M., and Kalamiyets, E. I. (2020). Microbial preparation for soil bioremediation and crop yield increase. Paper presented at the 2nd International Scientific Conference “Plants and Microbes: the Future of Biotechnology”, Saratov.
Martin, M. (2011). Cutadapt removes adapter sequences from high-throughput sequencing reads. EMBnet J. 17, 10–12. doi: 10.14806/ej.17.1.200
Mcik, M., Gryta, A., and Frc, M. (2020). Biofertilizers in agriculture: an overview on concepts, strategies and effects on soil microorganisms. Adv. Agron. 162, 31–87. doi: 10.1016/bs.agron.2020.02.001
Meriles, J. M., Gil, S. V., Conforto, C., Figoni, G., Lovera, E., March, G. J., et al. (2009). Soil microbial communities under different soybean cropping systems: characterization of microbial population dynamics, soil microbial activity, microbial biomass, and fatty acid profiles. Soil Tillage Res. 103, 271–281. doi: 10.1016/j.still.2008.10.008
Miranda, K. M., Espey, M. G., and Wink, D. A. (2001). A rapid, simple spectrophotometric method for simultaneous detection of nitrate and nitrite. Nitric Oxide 5, 62–71. doi: 10.1006/niox.2000.0319
Nelson, D. W., and Sommers, L. E. (2018). “Total carbon, organic carbon, and organic matter” in Methods of Soil Analysis. Part 3. Chemical Methods. eds. D. L. Sparks, A. L. Page, P. A. Helmke, R. H. Loeppert, P. N. Soltanpour, and M. A. Tabatabai, et al. (ASA and SSSA: Madison WI), 961–1010.
Qin, S., Stephen, Y., Cao, L., Zhang, J., Shi, S., Liu, Y., et al. (2017). Breaking continuous potato cropping with legumes improves soil microbial communities, enzyme activities and tuber yield. PLoS One 12:e175934:e0175934. doi: 10.1371/journal.pone.0175934
Quast, C., Pruesse, E., Yilmaz, P., Gerken, J., and Glckner, F. O. (2012). The SILVA ribosomal RNA gene database project: improved data processing and web-based tools. Nucleic Acids Res. 41, D590–D596. doi: 10.1093/nar/gks1219
Riaz, M., Akhtar, N., Khan, S., Shakeel, M., and Tahir, A. (2020). Neocosmospora rubicola: an unrecorded pathogen from Pakistan causing potato stem rot. Sarhad J. Agricult. 36, 906–912. doi: 10.17582/journal.sja/2020/36.3.906.912
Riaz, M., Akhtar, N., Msimbira, L., Antar, M., Ashraf, S., Khan, S., et al. (2022a). Neocosmospora rubicola, a stem rot disease in potato: Characterization, distribution and management. Front. Microbiol. 13:953097. doi: 10.3389/fmicb.2022.953097
Riaz, M., Mahmood, R., Antar, M., Akhtar, N., Khan, S., Anjum, M. A., et al. (2022b). A bacterial consortium and synthetic fertilizer based biocontrol approach against potato rot disease “Neocosmospora rubicola”. Front. Agron. 4:828070. doi: 10.3389/fagro.2022.828070
Rumberger, A. (2007). Microbial community development in the rhizosphere of apple trees at a replant disease site. Soil Biol. Biochem. 39, 1645–1654. doi: 10.1016/j.soilbio.2007.01.023
Sang, M. K., and Kim, K. D. (2012). The volatile-producing Flavobacterium johnsoniae strain GSE09 shows biocontrol activity against Phytophthora capsici in pepper. J. Appl. Microbiol. 113, 383–398. doi: 10.1111/j.1365-2672.2012.05330.x
Shah, S. H., Saleem, M. F., and Shahid, M. (2001). Effect of different fertilizers and effective microorganisms on growth, yield and quality of maize. Int. J. Agric. Biol. 3, 378–379.
Shen, Z., Zhong, S., Wang, Y., Wang, B., Mei, X., Li, R., et al. (2013). Induced soil microbial suppression of banana fusarium wilt disease using compost and biofertilizers to improve yield and quality. Eur. J. Soil Biol. 57, 1–8. doi: 10.1016/j.ejsobi.2013.03.006
Song, X. D., Liu, F., Wu, H. Y., Cao, Q., and Zhang, G. L. (2020). Effects of long-term K fertilization on soil available potassium in East China. Catena 188:104412. doi: 10.1016/j.catena.2019.104412
Tian, Y., Zhang, X., Liu, J., Chen, Q., and Gao, L. (2009). Microbial properties of rhizosphere soils as affected by rotation, grafting, and soil sterilization in intensive vegetable production systems. Sci. Hortic. 123, 139–147. doi: 10.1016/j.scienta.2009.08.010
Xiong, W., Li, Z., Liu, H., Xue, C., Zhang, R., Wu, H., et al. (2015). The effect of long-term continuous cropping of black pepper on soil bacterial communities as determined by 454 pyrosequencing. PLoS One 10:e136946:e0136946. doi: 10.1371/journal.pone.0136946
Xu, H. L. (2001). Effects of a microbial inoculant and organic fertilizers on the growth, photosynthesis and yield of sweet corn. J. Crop. Prod. 3, 183–214. doi: 10.1300/J144v03n01_16
Xu, W., Liu, D., Wu, F., and Liu, S. (2015). Root exudates of wheat are involved in suppression of Fusarium wilt in watermelon in watermelon-wheat companion cropping. Eur. J. Plant Pathol. 141, 209–216. doi: 10.1007/s10658-014-0528-0
Zhang, F., Huo, Y., Cobb, A. B., Luo, G., Zhou, J., Yang, G., et al. (2018). Trichoderma biofertilizer links to altered soil chemistry, altered microbial communities, and improved grassland biomass. Front. Microbiol. 9:848. doi: 10.3389/fmicb.2018.00848
Zhang, S., Jiang, Q., Liu, X., Liu, L., and Ding, W. (2020). Plant growth promoting rhizobacteria alleviate aluminum toxicity and ginger bacterial wilt in acidic continuous cropping soil. Front. Microbiol. 11:569512. doi: 10.3389/fmicb.2020.569512
Zhou, X., Zhang, X., Ma, C., Wu, F., Jin, X., Dini-Andreote, F., et al. (2022). Biochar amendment reduces cadmium uptake by stimulating cadmium-resistant PGPR in tomato rhizosphere. Chemosphere 307:136138. doi: 10.1016/j.chemosphere.2022.136138
Keywords: continuous cropping, microbial biofertilizers, soil quality, microbial community, watermelon
Citation: Chen P, Zhang J, Li M, Fang F, Hu J, Sun Z, Zhang A, Gao X and Li J (2023) Synergistic effect of Bacillus subtilis and Paecilomyces lilacinus in alleviating soil degradation and improving watermelon yield. Front. Microbiol. 13:1101975. doi: 10.3389/fmicb.2022.1101975
Edited by:
Md. Motaher Hossain, Bangabandhu Sheikh Mujibur Rahman Agricultural University, BangladeshReviewed by:
Xingang Zhou, Northeast Agricultural University, ChinaWeitao Jiang, Shandong Agricultural University, China
Copyright © 2023 Chen, Zhang, Li, Fang, Hu, Sun, Zhang, Gao and Li. This is an open-access article distributed under the terms of the Creative Commons Attribution License (CC BY). The use, distribution or reproduction in other forums is permitted, provided the original author(s) and the copyright owner(s) are credited and that the original publication in this journal is cited, in accordance with accepted academic practice. No use, distribution or reproduction is permitted which does not comply with these terms.
*Correspondence: Xingxiang Gao, xingxiang02@163.com; Jian Li,
sdlijian910@126.com
†These authors have contributed equally to this work