- College of Animal Husbandry and Veterinary Medicine, Jinzhou Medical University, Jinzhou, China
In this study, Bacillus subtilis, Clostridium butyricum and Enterococcus faecalis were made into a probiotic complex (PC). The PC was supplemented in AA+ male broilers’ diets to investigate the effects of PC on broiler growth performance, carcass traits, blood indicators, harmful gas emissions in feces and microbiota. Three hundred and sixty 1-day-old AA+ male broilers with an average initial body weight (data) were randomly divided into 3 dietary treatments of 6 replicates each, with 20 birds per replicate. The control group (T0) was fed a basal diet, while the test groups (T1 and T2) were supplemented with 0.025 and 0.05% PC in the basal diet, respectively. The trail was 42 days. The results showed that the supplementation of 0.05% PC significantly (p < 0.05) improved average daily gain (ADG) and average daily feed intake (ADFI) of broilers from 22 to 42 days and 1–42 days. Compared to the control group, the breast rate was significantly higher in T2, and the thymic index was significantly higher than that in T1 treatment (p < 0.05). The addition of PC had no significant effects on antibody potency in broiler serum (p > 0.05), but significantly increased albumin and total protein content in serum (p < 0.05). The addition of PC reduced H2S and NH3 emissions in the feces; the levels of Escherichia coli and Salmonella in the feces were significantly reduced and the levels of Lactobacillus were increased. And the most significant results were achieved when PC was added at 0.05%. Correlation analysis showed a significant positive correlation (p < 0.05) between the levels of E. coli and Salmonella and the emissions of H2S and NH3. Conclusion: Dietary supplementation with a 0.05% probiotic complex could improve the growth performance of broilers and also reduced fecal H2S and NH3 emissions, as well as fecal levels of E. coli and Salmonella, and increased levels of Lactobacillus. Thus, PC made by Bacillus subtilis, Clostridium butyricum and Enterococcus faecalis is expected to be an alternative to antibiotics. And based on the results of this trial, the recommended dose for use in on-farm production was 0.05%.
Introduction
Antibiotic promoters (AGP) had been widely used to improve growth performance and protect poultry from pathogens. However, many countries had banned or will soon ban the use of antibiotics in poultry feed due to the effects of antibiotic residues on human health (Aalaei et al., 2019). The search for alternatives to antibiotics was therefore an urgent task. Researchers were focused on finding alternatives to antibiotics that can improve growth performance and keep animals healthy (Khan et al., 2016). Green and healthy alternatives to antibiotics had been developed at this stage, such as probiotics, prebiotics, enzymes and acidifiers. Probiotics were often fed to poultry to potentially increase feed intake and nutrient absorption (Ghareeb et al., 2012). Many studies had demonstrated that probiotics can consistently induce positive effects on gastrointestinal morphology (Kim et al., 2021), nutrient absorption (Bai et al., 2017), antioxidant capacity (Shi et al., 2019), apoptosis (Wu et al., 2019), and immune response (Yahfoufi et al., 2018).
Among the many bacteria used as probiotics, the spore-forming genus Bacillus was favored by researchers and companies because its spores are resistant to harsh conditions and could be preserved for long periods of time at certain ambient temperatures (Chen et al., 2013; Wealleans et al., 2017). Bacillus subtilis was a facultative anaerobic or aerobic bacterium that can grow in the intestinal tract. In the animal intestine, Bacillus subtilis could produce substances such as antimicrobial peptides that enhance humoral and intestinal mucosal immunity (Lee et al., 2015). Most researchers had demonstrated that the addition of Bacillus subtilis to broiler diets can improve growth performance in broilers (Rhayat et al., 2017; Wealleans et al., 2017; Qiu et al., 2021; Zhang et al., 2021). Clostridium butyricum was an anaerobic bacterium that is widely found in the intestinal tract and feces of animals. Compared to Lactobacillus and Bifidobacterium, C. butyricum was more tolerant to lower pH, relatively high bile concentrations and higher temperatures (Zhang et al., 2016). Due to their probiotic properties, C. butyricum and Bacillus subtilis were widely used worldwide. Previous studies had shown that C. butyricum can significantly improve growth performance, nutrient metabolism and intestinal morphology in broilers (Takahashi et al., 2018; Huang et al., 2019; Molnár et al., 2020). Enterococcus faecalis was a lactic acid bacterium commonly found in the gastrointestinal tract of humans and animals (Cao et al., 2013). The addition of E. faecalis to the diet promoted the growth of beneficial bacteria in the gut and inhibits harmful bacteria, thus maintaining a healthy gut and enabling the animal to achieved higher growth performance (Adil and Magray, 2012; Ognik et al., 2017). Enterococcus faecalis had a strong colonization advantage over other probiotics and was therefore still widely used as a pharmaceutical and microbial additive (Al-Balawi and Morsy, 2020; Hanifeh et al., 2021; Zhang et al., 2022).
The birds gastrointestinal tract contains different microbial communities whose interactions had an important impact on the nutritional, immunological and physiological status of the host (Zhao et al., 2013). Therefore, supplementing the diet with antibiotic alternatives holds promise as a good option to improve overall gut health and immunity through the growth of specific microorganisms, thus improving the diet (Borda-Molina et al., 2018). In this regard, the addition of probiotics to the diet was seen as a promising alternative to antibiotics (Dev et al., 2020). Researchers had reported the effects of B. subtilis, C. butyricum and E. faecalis as single strains on broilers. However, based on the physiological characteristics and possible synergistic effects of these three probiotics, is it possible to speculate that their combined use in broiler diets will have a beneficial effect on broilers? Therefore, this trial investigated the supplementation of AA+ male broiler diets with a complex probiotic made from B. subtilis, C. butyricum and E. faecalis to study the effects on broiler growth performance, carcass traits, blood indicators, harmful gas emissions and fecal microbiota, and to look for possible links between fecal microbial content and apparent indicators.
Materials and methods
Ethics statement
The Animal Conservation and Utilization Committee of the JZMU approved the animal use agreement (No. JZMULL2021006).
Probiotic sources
The main components of PC are B. subtilis (2 × 108 cfu/g), C. butyricum (2 × 106 cfu/g) and E. faecalis (1 × 106 cfu/g).
Animals and experimental design
Three hundred and sixty 1-day-old AA+ male broilers with similar body weight (44.77 ± 0.25) were selected and randomly divided into 3 treatment groups of 6 replicates each, with 20 chicks in each replicate. The control group (T0) was fed a basal diet, while the test groups (T1 and T2) were supplemented with 0.025 and 0.05% probiotic complex in the basal diet, respectively. The test period was 42 days.
Animals feeding management
The base diet for the trial (Table 1) was configured with reference to the NRC (1994) version. Broilers were free to feed and drink. The test broilers house is a fully enclosed house with an automatic environmental control system to ensure optimum temperature and humidity. The broiler house has 48 pens to provide shelter for the broilers. Each pen holds ten broilers at a density of approximately 625 cm2 per broiler. Every two pens are 1 repeat. During rearing, the room temperature is 33°C for 1–3 days and then drops by 3°C per week to maintain a room temperature of around 22°C and humidity control of 40–70%. The lighting program on days 1–7 and 36–42 was 24 h per day throughout the trial period. The lighting program on days 8–30 provided 20 h per day and 4 h of darkness. After day 31, the darkness hours were gradually reduced.
Test indicator determination
Growth performance
Broilers were weighed at 1, 21, and 42 d. Feed intake was recorded in replicates throughout the trial and average daily feed intake (ADG), average daily weight gain (ADFI) and meat to feed ratio (F/G) were calculated.
Carcass traits
At the end of the 42-day trial, one broiler from each replicate was randomly selected and weighed live after a 12-h fast. Selected broilers were euthanized and carotid artery blood was collected for serum preparation. Weighing of post-mortem broiler carcasses, breast and drumsticks. Calculate carcass yield, breast rate and drumsticks rate. The heart, liver, spleen, thymus and bursa were isolated, the blood aspirated on filter paper, the surface fat and connective tissue removed and weighed. Calculate the organ index.
Organ index = fresh weight of organ (g)/live weight before slaughter (kg).
Blood indicators
On day 21 of the test, 2 ml of blood was collected from the lower wing vein of the broiler and used to prepare the serum. Blood samples collected from 42-day-old broilers were left at room temperature for 30 min and centrifuged at 1,200 r/min for 15 min to extract the supernatant. The potency of serum antibodies to Newcastle disease and avian influenza H9 is determined by a hemagglutination inhibition test. Serum levels of albumin (ALB), total protein (TP), globulin (GLOB), alanine transaminase (ALT), alkaline phosphatase (ALP) and glucose (GLU) were measured using a fully automated biochemical analyzer.
Harmful gas emissions
On day 42 of the trial, fresh chicken manure was collected from each replicate and ammonia and hydrogen sulfide emissions from the manure were determined using the method of Dang et al. (2020). The manure was placed in a 2 l plastic box with small holes attached to the side and fermented at room temperature (25°C) for 6, 12, 24, and 48 h. The air sample is then collected with a gas collection pump from above the small holes on either side. NH3 and H2S concentrations are measured in the range of 0.00–100.00 mg/m3.
Fecal microbiota
A 1 g sample of broiler manure from each replicate was collected weekly and transported on ice to the laboratory following the method of Dang et al. (2020). Each replicate of 1 g fecal sample was diluted and mixed with 9 ml of 1% peptone broth. The viable counts of E. coli, Lactobacillus and Salmonella in fecal samples were determined on McConkey agar plates, MRS agar plates and BS agar plates (in a 10 g/l peptide solution) in a biosafety cabinet. The microbial count is ultimately expressed as log10 colony forming units per gram of feces.
Data analysis
The data was designed using a completely randomized grouping design. Replicate pen serves as the experimental unit. Multiple comparisons of significant differences in means were performed using the one-way ANOVA LSD method in SPSS 25.0 and visualization was completed using Graphpad Prism 8. Results are expressed as mean and standard deviation, with p < 0.05 indicating a significant difference. The results of the correlation analysis are completed and displayed by R (V4.0.2). Pearson correlation analysis was performed using the R (V4.0.2) corrplot package for the 42 days indicators, which were significantly correlated at p < 0.05 when R > 0.6 or R < –0.6.
Results
Growth performance
As shown in Figure 1, ADG and ADFI were significantly higher at 22–42 d and 1–42 days in T2 compared to T0 and T1 (p < 0.05). The addition of the probiotic complex to the diet had no significant effect on F/G among the groups (p > 0.05).
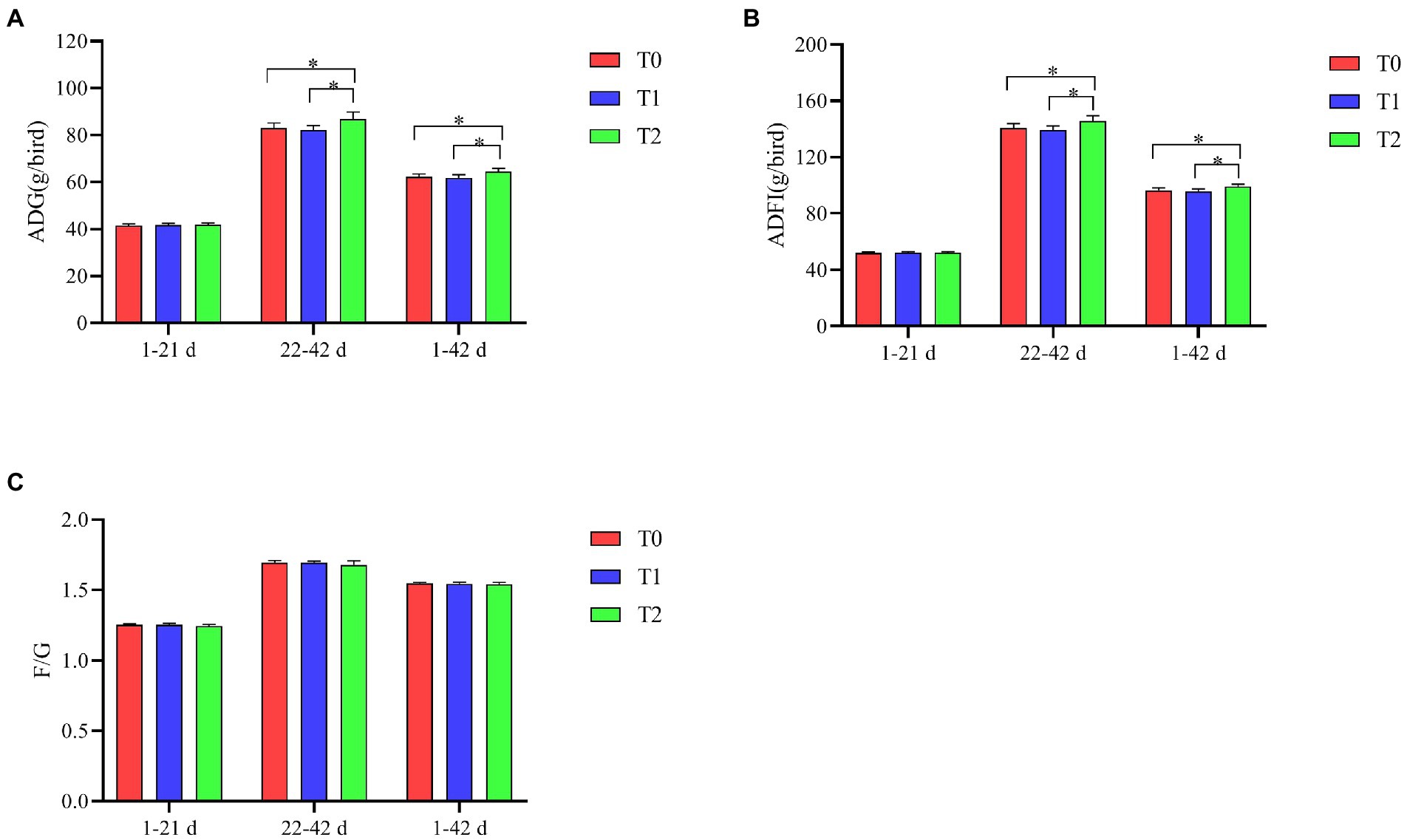
Figure 1. Effect of probiotic complex on growth performance of AA+ male broiler. (A) Average daily gain; (B) Average daily feed intake; and (C) Feed-to-weight ratio. T0: Feeding a basal diet; T1: Supplementation of the basal diet with 0.025% probiotic complex; T2: Supplementation of the basal diet with 0.05% probiotic complex. “*” means indicates a significant difference (p < 0.05). No “*” indicates that the difference is not significant (p > 0.05). The same as below.
Carcass traits
As shown in Figure 2, the addition of the probiotic complex to the diet improved the carcass yield and liver index of broilers in the test groups (T1 and T2), but did not reach a significant difference (p > 0.05). Breast was significantly higher in T1 than in T0 (p < 0.05). Thymic index was significantly higher in T2 than in T0 (p < 0.05).
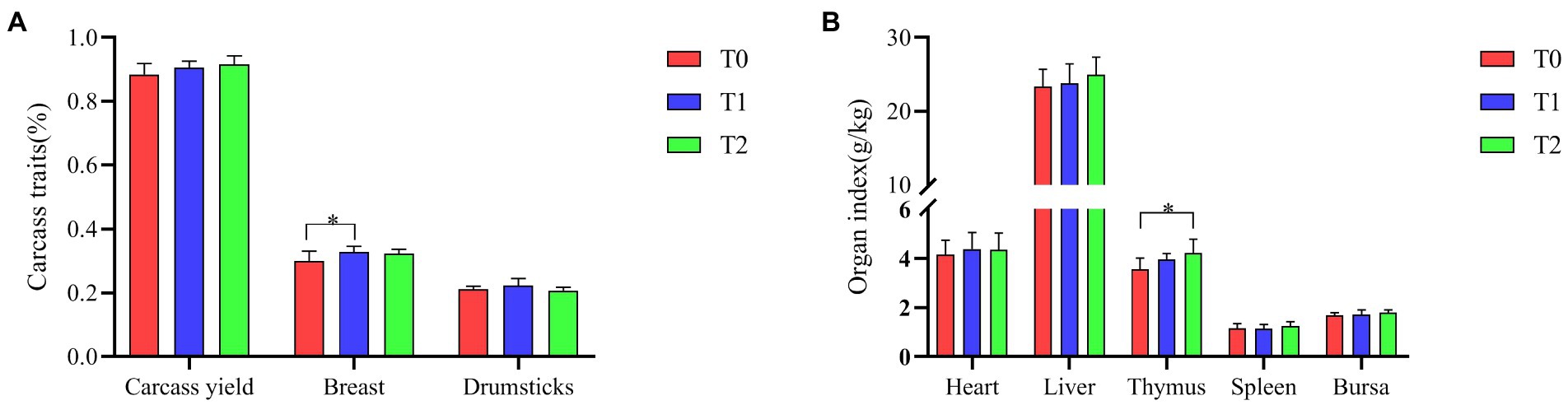
Figure 2. Effect of probiotic complex on carcass traits and organ indices of AA+ male broiler. (A) Carcass traits and (B) Organ index. T0: Feeding a basal diet; T1: Supplementation of the basal diet with 0.025% probiotic complex; T2: Supplementation of the basal diet with 0.05% probiotic complex.
Blood indicators
As shown in Figure 3, compared to the control group T0, supplementation of the diet with the probiotic complex increased the serum potency of Newcastle disease and avian influenza H9 antibodies in broiler in the test groups (T1 and T2), but did not reach a significant difference (p > 0.05).
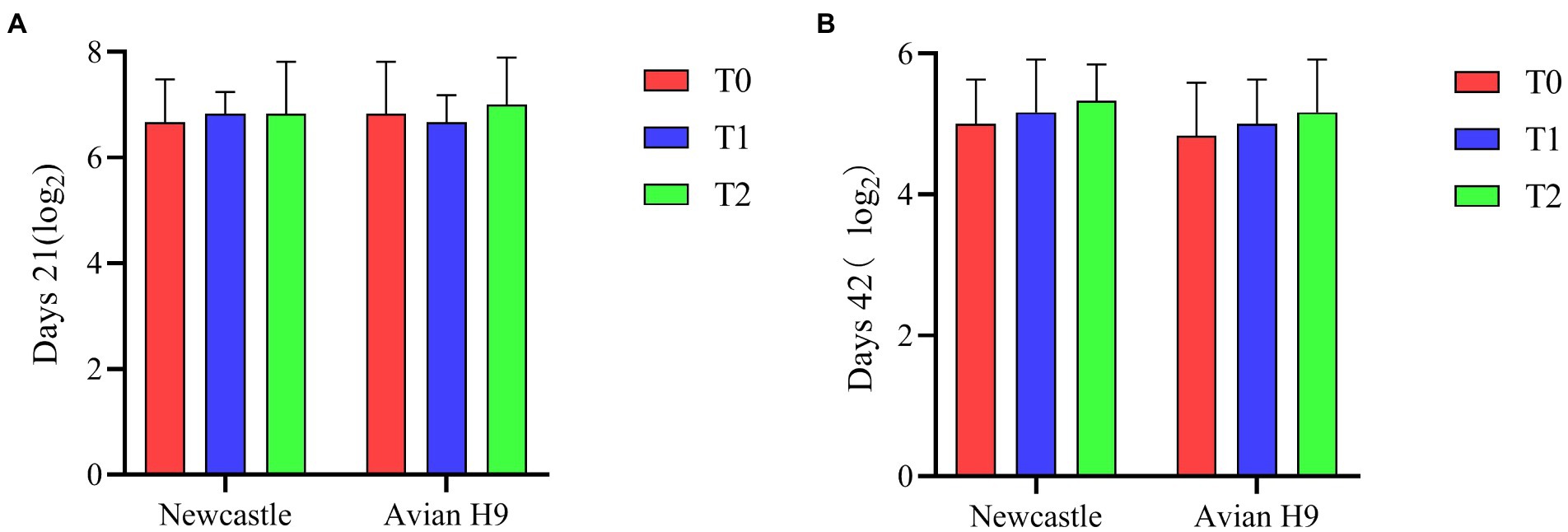
Figure 3. Effect of probiotic complex on serum antibody potency of AA+ male broiler. (A) Newcastle disease and Avian influenza H9 antibody potency in 21 days broiler sera. (B) Newcastle disease and Avian influenza H9 antibody potency in 42 days broiler sera. T0: Feeding a basal diet; T1: Supplementation of the basal diet with 0.025% probiotic complex; T2: Supplementation of the basal diet with 0.05% probiotic complex.
As shown in Figure 4, ALB and TP levels were significantly increased in T2 serum compared to T0 and T1 (p < 0.05). GLU levels in serum were significantly higher in the T1 group than in the T0 and T2 groups (p < 0.05). Supplementation of the diet with the probiotic complex had no significant effect on the serum levels of GLOB, ALT and ALP in broiler (p > 0.05).
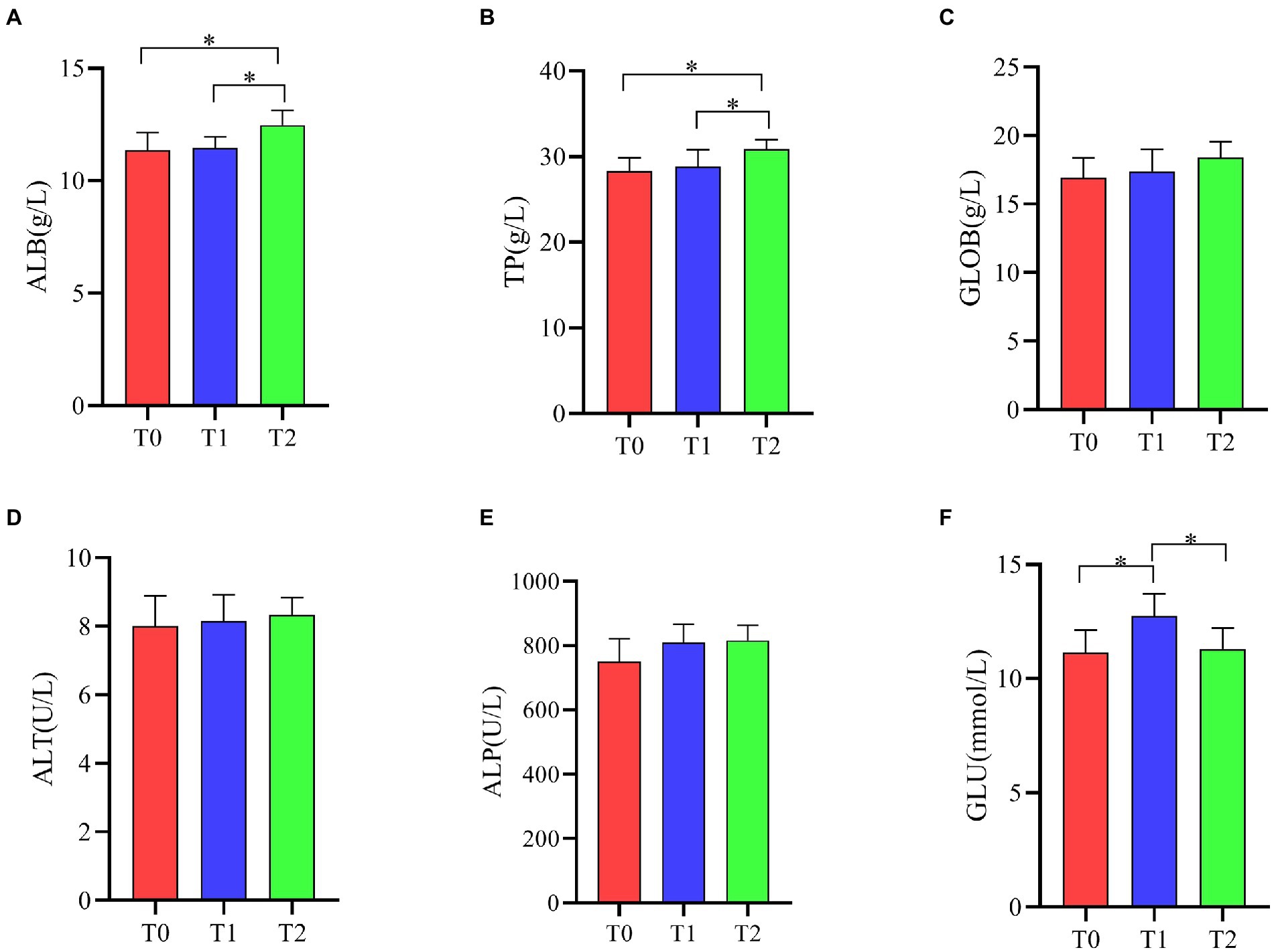
Figure 4. Effect of probiotic complex on serum biochemical parameters of AA+ male broiler. (A) Albumin; (B) Total protein; (C) Globulin; (D) Alanine transaminase; (E) Alkaline phosphatase; and (F) Glucose. T0: Feeding a basal diet; T1: Supplementation of the basal diet with 0.025% probiotic complex; T2: Supplementation of the basal diet with 0.05% probiotic complex.
Harmful gas emissions
As shown in Table 2 and Figure 5, supplementing the diet with a probiotic complex significantly reduced fecal H2S and NH3 emissions compared to the control group. And T2 was always kept the lowest level. As shown in Figure 5, it was clear that the emissions of H2S first increase and then decrease, while the emissions of NH3 showed a continuous increasing trend.
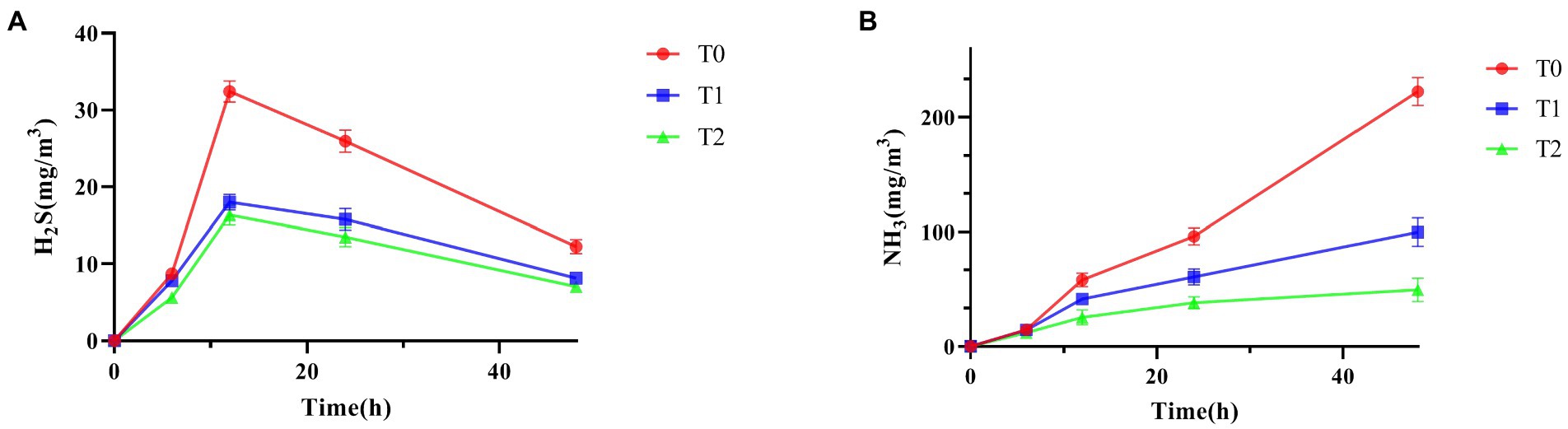
Figure 5. Effect of probiotic complex on harmful gas emissions of AA+ male broiler. (A) H2S; (B) NH3. T0: Feeding a basal diet; T1: Supplementation of the basal diet with 0.025% probiotic complex; T2: Supplementation of the basal diet with 0.05% probiotic complex.
Fecal microbiota
As shown in Table 3, the number of Salmonella in the feces of AA+ male broiler at 21 days was significantly lower in the T2 group than in the T0 group (p < 0.05). At 42 days, the number of E. coli in the feces of AA+ male broiler was significantly lower in groups T1 and T2 than in group T0 (p < 0.05), and there was no significant difference between groups T1 and T2 (p > 0.05). Supplementing the diet with a probiotic complex significantly reduced Salmonella levels in the feces of 42-day AA+ male broilers (p < 0.05) and had no significant effect on lactic acid bacteria levels in the feces (p > 0.05).
As shown in Figure 6, the levels of E. coli and Salmonella in the feces decreased throughout the breeding cycle after supplementation of the probiotic complex in the feed. In contrast, there was a slight increase in the levels of Lactobacillus.
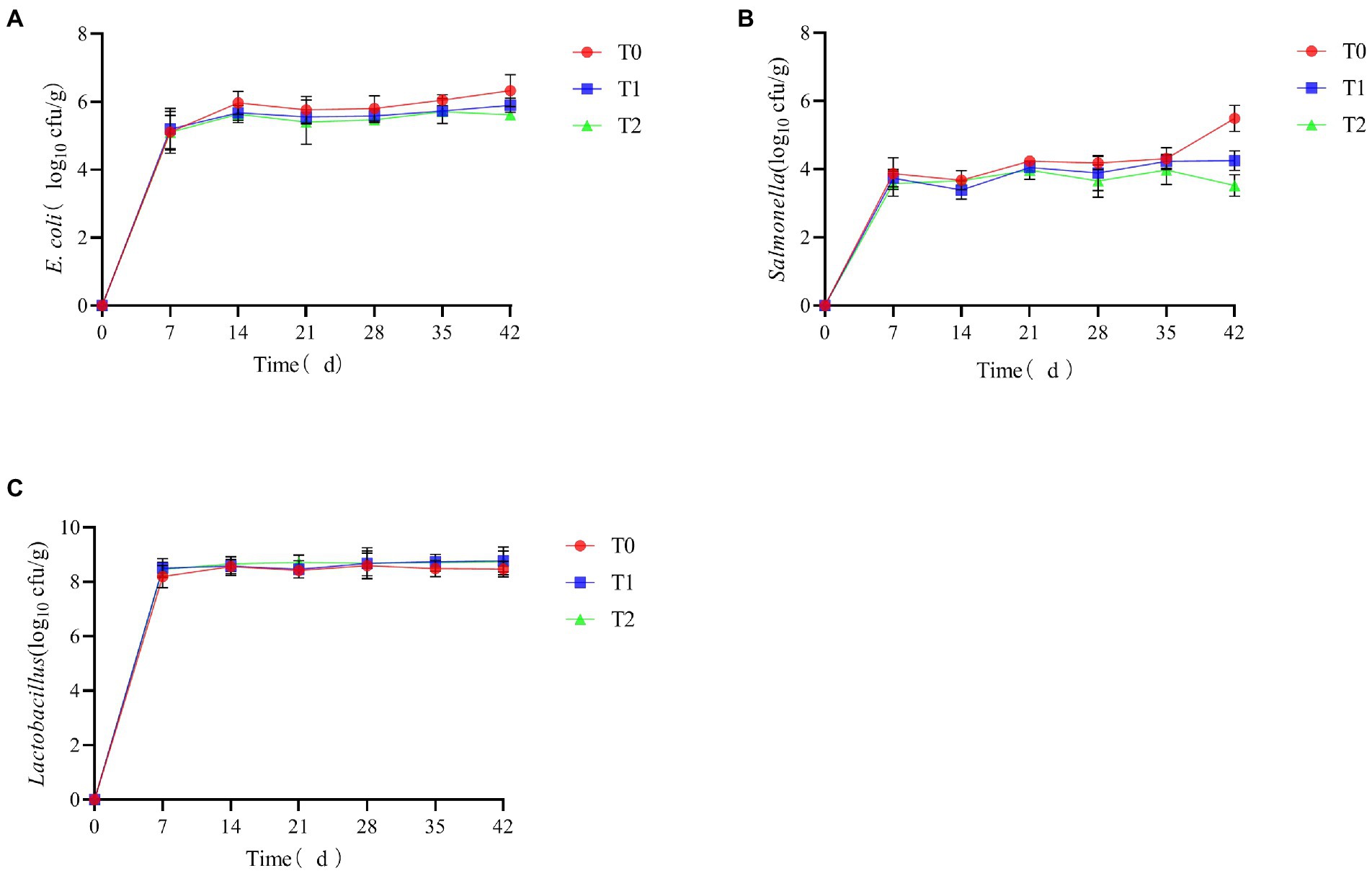
Figure 6. The effect of probiotic complex on the microbial content of AA+ male broiler feces. (A) Escherichia coli; (B) Salmonella; and (C) Lactobacillus. T0: Feeding a basal diet; T1: Supplementation of the basal diet with 0.025% probiotic complex; T2: Supplementation of the basal diet with 0.05% probiotic complex.
Correlation analysis of microbiological and surface indicators
As shown in Figure 7, carcass yield was significantly and negatively correlated with E. coli (R = −0.605). Thymus index was negatively and significantly correlated with NH3 emissions (R = −0.605). Newcastle disease antibody potency was significantly and positively correlated with Lactobacillus (R = 0.622). TP was significantly and positively correlated with ALB (R = 0.665) and GLOB (R = 0.912). E. coli was positively and significantly correlated with Salmonella (R = 0.643) and positively and significantly correlated with H2S (R = 0.745) and NH3 (R = 0.695). Salmonella was positively and significantly correlated with H2S (R = 0.840), NH3 (R = 0.934). H2S and NH3 (R = 0.943) were significantly and positively correlated.
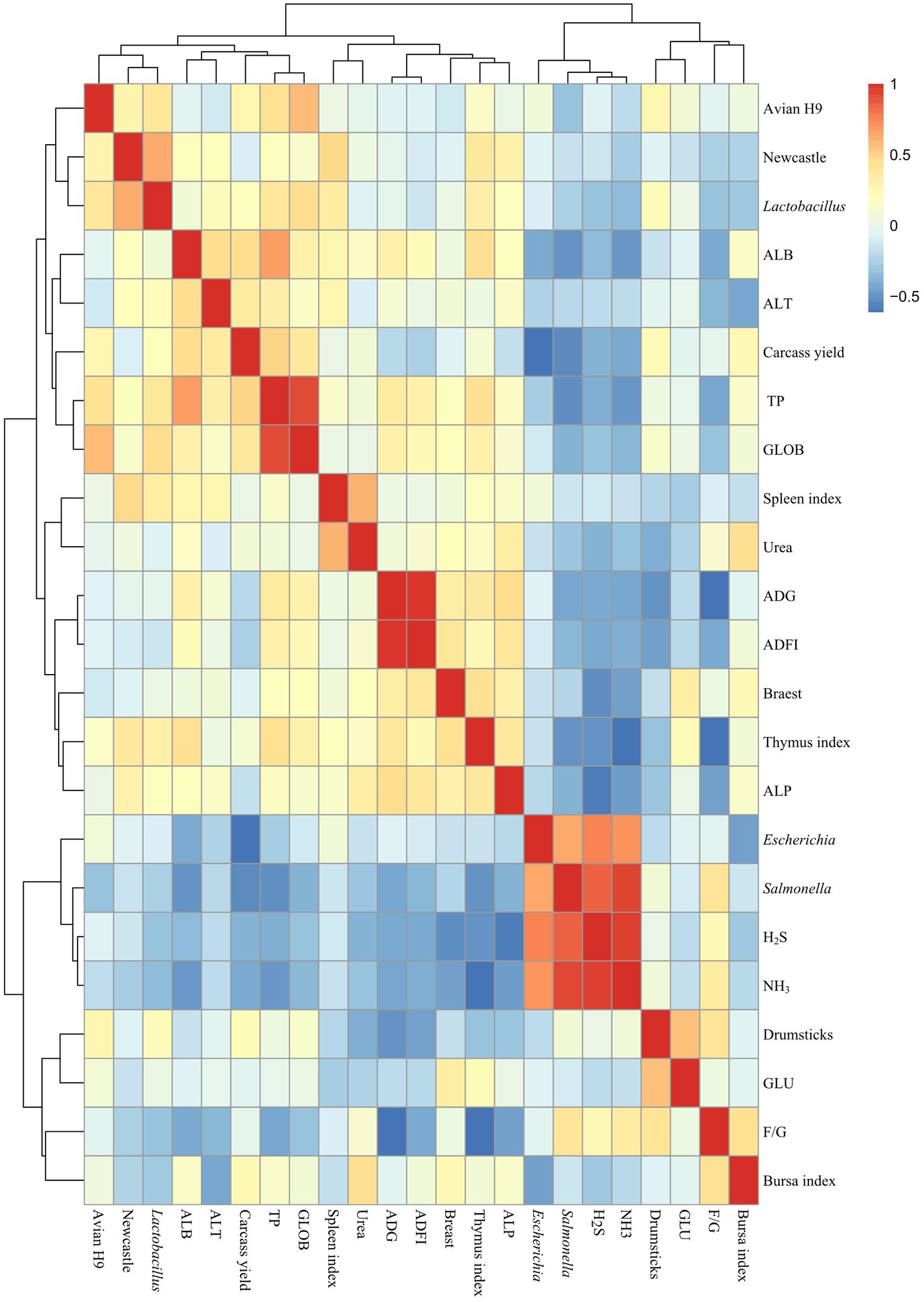
Figure 7. Correlation analysis of microbiological and surface indicators. T0: Feeding a basal diet; T1: Supplementation of the basal diet with 0.025% probiotic complex; T2: Supplementation of the basal diet with 0.05% probiotic complex.
Discussion
Average daily gain, ADFI and F/G, as important indicators for evaluating growth performance, had a direct impact on the economic performance of the farming industry. Supplementation of broiler diets with single or multiple probiotics had been reported to promote growth performance and health by regulating the intestinal microbiota, improving digest and enhancing immune regulation (Yang et al., 2012). The results of this study showed that the addition of 0.05% PC, including significantly increased ADG and ADFI in broilers. The addition of 0.025% of probiotic complex also improved growth performance compared to the control, but not to a significant level. Previous studies have reported that the synergistic effects of combined probiotics are superior to those of single probiotics (Javandel et al., 2019; Salehizadeh et al., 2019). Wang et al. (2022) reported that the addition of Bacillus sp. KC1 to the diet improved the growth performance and body weight of broilers. Zhang et al. (2021) also confirmed that the improvement in growth performance of broiler chickens by B. subtilis may be associated with improved microecology in the intestinal tract. Zeng et al. (2021) found that the addition of a mixture of C. butyricum, B. subtilis and B. licheniformis to broiler diets increased the body weight and average body weight of broilers. Shehata et al. (2020) added Enterococcus faecalis-1 to broiler diets and found that E. faecalis improved growth performance in broilers. The results of this trial were similar to those previously reported above. In this trial, it was found that the addition of B. subtilis, C. butyricum and E. faecalis as the main components of the probiotic complex to the diet mainly improved growth performance during the late growth period (22–42 days) and indirectly during the full growth period (1–42 days). This may be related to the accumulation of probiotics in the intestinal tract. Probiotics can only have a positive effect on the organism when they have accumulated in the intestinal tract in certain quantities.
Carcass yield, breast rates and drumsticks rates reflect, to some extent, the meat production performance of broilers and, indirectly, their growth performance. The results of this study showed that the addition of 0.025% of a probiotic complex to the diet significantly increased the breast rates compared to the control group. However, the addition of a probiotic complex had no effect on carcass yield and drumsticks rates, which was consistent with the findings of Yadav et al. (2018) and Tang et al. (2021). The organ index was an important indicator of organ development in broilers. The results of this study showed that the addition of probiotic complexes to the diet had no effect on the cardiac index, liver index, spleen index and bursal index of broilers. However, the broiler thymus index increased significantly when added at 0.05%. The thymus was the lymphatic organ of the broiler. The increased weight of the lymphoid organs may indicate that treated broilers had acquired a higher level of immunity (Chen et al., 2013; Pournazari et al., 2017). Chen et al. (2013) reported that an increase in relative organ weight may coincide with an increase in lymphocyte concentration. Al-Sagan et al. (2020) reported a significant difference in the effect of probiotic addition on 29.3% of lymphoid organs associated with immune response compared to the supplemented group. In this trial, the addition of B. subtilis, C. butyricum and E. faecalis increased the thymus weight of broilers, which also suggests that the addition of probiotic complex could potentially improve the immunity of the broiler organism and enhance its ability to produce humoral and cellular immunity in response to antigenic stimuli.
Blood indicators were used for assessment to determine the quality of the feed/additive tested, reflecting the physiological state of the animal (Ogbuewu et al., 2015). The spleen, a lymphoid organ in broilers, activated the proliferation and differentiation of T and B cells and was the main site of antibody production. The results of this study showed that the addition of a probiotic complex to the diet increased antibody levels in broiler serum at 21 and 42 days, but did not reach a significant difference. Salehizadeh et al. (2019) reported that complex probiotics were effective in increasing Newcastle disease antibody levels in broilers. He et al. (2019) showed that B. subtilis had a positive effect on increasing Newcastle disease antibody levels in the blood of broiler. The possible reason was that the probiotic complex promotes the development of the spleen, which leads to an increase in serum antibody levels. Abudabos et al. (2020) found that the addition of B. licheniformis and B. subtilis to diets significantly increased serum cholesterol, blood glucose, total protein and globulin levels in Salmonella-infected broilers. Similar results were obtained in this trial, where the addition of the probiotic complex resulted in a significant increase in both albumin and total protein in the serum of broiler. The increase in blood protein levels might be due to the fact that probiotics improves dietary protein utilization through its ability to inhibit pathogens growth, which reduces protein breakdown into nitrogen and increases the absorption of nutrients in the gut (Lee et al., 2013; Abd El-Moneim, 2017; Abd El-Moneim et al., 2020). In this study, blood glucose levels in the serum of broilers supplemented with 0.025% probiotic complex in the diet were found to be significantly higher than in the control and test broilers supplemented with 0.05% probiotic complex. This was inconsistent with the results reported by Al-Kassie et al. (2008) and Reuben et al. (2022), where the addition of probiotics reduced blood glucose levels in broilers. A dose-dependent relationship between blood glucose levels and the strains contained in probiotics had been previously reported (Samanya and Yamauchi, 2002). It is speculated that the reason for the inconsistency between this trial and previous results might be due to differences in the type of probiotics and the amount of additives, the exact reasons for which needed to be further investigated.
NH3, H2S, CH4 and CO2 were considered to be the most dangerous gases in the poultry organism (Gates et al., 2008). In this study, the addition of a probiotic complex was found to reduce NH3 and H2S emissions, reduced levels of E. coli and Salmonella and increase levels of Lactobacillus in broiler feces. Analysis of fecal microbiological and surface indicators showed positive and significant associations between E. coli and Salmonella levels and NH3 and H2S emissions. As previously reported by researchers, Misiukiewicz et al. (2021) concluded that fecal odor and NH3 emissions were associated with nutrient utilization and the gut microbial ecosystem. Along with Pseudomonas, Citrobacter, Aeromonas and Salmonella, E. coli was identified as the most promising H2S-producing bacterium (Maker and Washington, 1974). Jeong and Kim (2014) showed a decrease in E. coli and Salmonella cecum and an increase in Lactobacillus following the addition of B. subtilis preparations to broiler diets. The addition of a probiotic complex affected the fecal microbiota, increasing the number of beneficial bacteria and reducing the number of harmful bacteria in the broiler’s gut. When the number of E. coli and Salmonella decreases, the NH3 and H2S emissions in feces also decrease. And this test found a negative and significant relationship between E. coli and carcass yield. It also showed that with the reduction in E. coli numbers, the carcass yield of broilers is improved and more high-quality, healthy broiler meat was available. There was also a significant negative correlation between NH3 emissions and thymus index. As previously reported, growth performance of broilers was improved when the farm environment was improved (Jeni et al., 2021). Providing broilers with a better living environment will enable them to develop their thymus and thus achieve higher levels of immunity.
Conclusion
Probiotic complexes: B. subtilis, C. butyricum and E. faecalis were added to AA+ male broiler diets to enhance growth performance and improve carcass performance. The addition of probiotic complex led to a reduction in NH3 and H2S emissions, a decrease in the number of E. coli and Salmonella, and an increase in Lactobacillus in the feces of AA+ male broilers. And based on the results of this trial, the recommended dose for use in on-farm production was 0.05%.
Data availability statement
The original contributions presented in the study are included in the article/supplementary material, further inquiries can be directed to the corresponding authors.
Ethics statement
The animal study was reviewed and approved by The Animal Conservation and Utilization Committee of the JZMU.
Author contributions
All authors listed have made a substantial, direct, and intellectual contribution to the work and approved it for publication.
Funding
This work was financed by the grants from the central government-guided local science and technology development fund projects (Grant No. 2021001). 2021 basic scientific research project of colleges and universities of Liaoning Provincial Department of Education (Grant No. LJKZ0801).
Acknowledgments
We thank the College of Animal Husbandry and Veterinary Medicine of Jinzhou Medical University for its support of the experiment. We thank Liaoning Kaiwei Biological Co Ltd. for providing the breeding base for this trial. We thank Jinzhou Zhongke Genetic Testing Service Co Ltd. for providing the antigens for antibody detection, and for assisting us with microbiological testing.
Conflict of interest
The authors declare that the research was conducted in the absence of any commercial or financial relationships that could be construed as a potential conflict of interest.
Publisher’s note
All claims expressed in this article are solely those of the authors and do not necessarily represent those of their affiliated organizations, or those of the publisher, the editors and the reviewers. Any product that may be evaluated in this article, or claim that may be made by its manufacturer, is not guaranteed or endorsed by the publisher.
References
Aalaei, M., Khatibjoo, A., Zaghari, M., Taherpou, K., Akbari-Gharaei, M., and Soltani, M. (2019). Effect of single and multi-strain probiotics on broiler breeder performance, immunity and intestinal toll-like receptors expression. J. Appl. Anim. Res. 47, 236–242. doi: 10.1080/09712119.2019.1618311
Abd El-Moneim, E. A. (2017). Influence of in ovo injection with an effective bacterial preparation (Bifidobacterium spp.) on some productive and physiological traits in poultry. Doctoral dissertation. Egypt: Ain Shams University.
Abd El-Moneim, A. E., Selim, D. A., Basuony, H. A., Sabic, E. M., Saleh, A. A., and Ebeid, T. A. (2020). Effect of dietary supplementation of Bacillus subtilis spores on growth performance, oxidative status, and digestive enzyme activities in Japanese quail birds. Trop. Anim. Health Prod. 52, 671–680. doi: 10.1007/s11250-019-02055-1
Abudabos, A. M., Aljumaah, M. R., Alkhulaifi, M. M., Alabdullatif, A., Suliman, G. M., Al Sulaiman, R., et al. (2020). Comparative effects of Bacillus subtilis and Bacillus licheniformis on live performance, blood metabolites and intestinal features in broiler inoculated with salmonella infection during the finisher phase. Microb. Pathog. 139:103870. doi: 10.1016/j.micpath.2019.103870
Adil, S., and Magray, S. N. (2012). Impact and manipulation of gut microflora in poultry: a review. J. Anim. Vet. Adv. 11, 873–877. doi: 10.3923/javaa.2012.873.877
Al-Balawi, M., and Morsy, F. M. (2020). Enterococcus faecalis is a better competitor than other lactic acid bacteria in the initial colonization of colon of healthy newborn babies at first week of their life. Front. Microbiol. 11:2017. doi: 10.3389/fmicb.2020.02017
Al-Kassie, G., Al-Jumaa, Y., and Jameel, Y. (2008). Effect of probiotic (Aspergillus niger) and prebiotic (Taraxacum officinale) on blood picture and biochemical properties of broiler chicks. Int. J. Poult. Sci. 7, 1182–1184. doi: 10.3923/ijps.2008.1182.1184
Al-Sagan, A. A., Al-Yemni, A. H., Al-Abdullatif, A. A., Attia, Y. A., and Hussein, E. (2020). Effects of different dietary levels of blue lupine (Lupinus angustifolius) seed meal with or without probiotics on the performance, carcass criteria, immune organs, and gut morphology of broiler chickens. Front. Vet. Sci. 7:124. doi: 10.3389/fvets.2020.00124
Bai, K., Huang, Q., Zhang, J., He, J., Zhang, L., and Wang, T. (2017). Supplemental effects of probiotic Bacillus subtilis fmbJ on growth performance, antioxidant capacity, and meat quality of broiler chickens. Poult. Sci. 96, 74–82. doi: 10.3382/ps/pew246
Borda-Molina, D., Seifert, J., and Camarinha-Silva, A. (2018). Current perspectives of the chicken gastrointestinal tract and its microbiome. Comput. Struct. Biotechnol. J. 16, 131–139. doi: 10.1016/j.csbj.2018.03.002
Cao, G. T., Zeng, X. F., Chen, A. G., Zhou, L., Zhang, L., Xiao, Y. P., et al. (2013). Effects of a probiotic, enterococcus faecium, on growth performance, intestinal morphology, immune response, and cecal microflora in broiler chickens challenged with Escherichia coli K88. Poult. Sci. 92, 2949–2955. doi: 10.3382/ps.2013-03366
Chen, W., Wang, J. P., Yan, L., and Huang, Y. Q. (2013). Evaluation of probiotics in diets with different nutrient densities on growth performance, blood characteristics, relative organ weight and breast meat characteristics in broilers. Br. Poult. Sci. 54, 635–641. doi: 10.1080/00071668.2013.825369
Dang, D. X., Yong, M. K., and Kim, I. H. (2020). Effects of a root extract from Achyranthes Japonica Nakai on the growth performance, blood profile, fecal microbial community, fecal gas emission, and meat quality of finishing pigs. Livest. Sci. 239, 104160–104165. doi: 10.1016/j.livsci.2020.104160
Dev, K., Mir, N. A., Biswas, A., Kannoujia, J., Begum, J., Kant, R., et al. (2020). Dietary synbiotic supplementation improves the growth performance, body antioxidant pool, serum biochemistry, meat quality, and lipid oxidative stability in broiler chickens. Anim. Nutr. 6, 325–332. doi: 10.1016/j.aninu.2020.03.002
Gates, R. S., Casey, K. D., Wheeler, E. F., Xin, H., and Pescatore, A. J. (2008). U.S. broiler housing ammonia emissions inventory. Atmos. Environ. 42, 3342–3350. doi: 10.1016/j.atmosenv.2007.06.057
Ghareeb, K., Awad, W. A., Mohnl, M., Porta, R., Biarnés, M., Böhm, J., et al. (2012). Evaluating the efficacy of an avian-specific probiotic to reduce the colonization of Campylobacter jejuni in broiler chickens. Poult. Sci. 91, 1825–1832. doi: 10.3382/ps.2012-02168
Hanifeh, M., Spillmann, T., Huhtinen, M., Sclivagnotis, Y. S., Grönthal, T., and Hynönen, U. (2021). Ex-vivo adhesion of Enterococcus faecalis and Enterococcus faecium to the intestinal mucosa of healthy beagles. Animals 11:3283. doi: 10.3390/ani11113283
He, T., Long, S., Mahfuz, S., Wu, D., Wang, X., Wei, X., et al. (2019). Effects of probiotics as antibiotics substitutes on growth performance, serum biochemical parameters, intestinal morphology, and barrier function of broilers. Animals 9:985. doi: 10.3390/ani9110985
Huang, T., Peng, X. Y., Gao, B., Wei, Q. L., Xiang, R., Yuan, M. G., et al. (2019). The effect of Clostridium butyricum on gut microbiota, immune response and intestinal barrier function during the development of necrotic enteritis in chickens. Front. Microbiol. 10:2309. doi: 10.3389/fmicb.2019.02309
Javandel, F., Nosrati, M., van den Hoven, R., Seidavi, A., Laudadio, V., and Tufarelli, V. (2019). Effects of hogweed (Heracleum persicum) powder, flavophospholipol, and probiotics as feed supplements on the performance, carcass and blood characteristics, intestinal microflora, and immune response in broilers. J. Poult. Sci. 56, 262–269. doi: 10.2141/jpsa.0180081
Jeni, R. E., Dittoe, D. K., Olson, E. G., Lourenco, J., Corcionivoschi, N., Ricke, S. C., et al. (2021). Probiotics and potential applications for alternative poultry production systems. Poult. Sci. 100:101156. doi: 10.1016/j.psj.2021.101156
Jeong, J. S., and Kim, I. H. (2014). Effect of Bacillus subtilis C-3102 spores as a probiotic feed supplement on growth performance, noxious gas emission, and intestinal microflora in broilers. Poult. Sci. 93, 3097–3103. doi: 10.3382/ps.2014-04086
Khan, R. U., Naz, S., Dhama, K., Karthik, K., Tiwari, R., Abdelrahman, M. M., et al. (2016). Direct-fed microbial: beneficial applications, modes of action and prospects as a safe tool for enhancing ruminant production and safeguarding health. Int. J. Pharmacol. 12, 220–231. doi: 10.3923/ijp.2016.220.231
Kim, J., Hlaing, S. P., Lee, J., Saparbayeva, A., Kim, S., Hwang, D. S., et al. (2021). Exfoliated bentonite/alginate nanocomposite hydrogel enhances intestinal delivery of probiotics by resistance to gastric pH and on-demand disintegration. Carbohydr. Polym. 272:118462. doi: 10.1016/j.carbpol.2021.118462
Lee, K., Kyung, D., Lillehoj, H. S., Jang, S. I., and Lee, S. H. (2015). Immune modulation by Bacillus subtilis-based direct-fed microbials in commercial broiler chickens. Anim. Feed Sci. Technol. 200, 76–85. doi: 10.1016/j.anifeedsci.2014.12.006
Lee, K. W., Lillehoj, H. S., Jang, S. I., Lee, S. H., Bautista, D. A., and Siragusa, G. R. (2013). Effect of bacillus subtilis-based direct-fed microbials on immune status in broiler chickens raised on fresh or used litter. Asian Australas. J. Anim. Sci. 26, 1592–1597. doi: 10.5713/ajas.2013.13178
Maker, M. D., and Washington, J. A. (1974). Hydrogen sulfide-producing variants of Escherichia coli. Appl. Microbiol. 28, 303–305. doi: 10.1128/am.28.2.303-305.1974
Misiukiewicz, A., Gao, M., Filipiak, W., Cieslak, A., Patra, A. K., and Szumacher-Strabel, M. (2021). Review: methanogens and methane production in the digestive systems of nonruminant farm animals. Animal 15:100060. doi: 10.1016/j.animal.2020.100060
Molnár, A., Such, N., Farkas, V., Pál, L., Menyhárt, L., Wágner, L., et al. (2020). Effects of wheat bran and Clostridium butyricum supplementation on cecal microbiota, short-chain fatty acid concentration, pH and histomorphometry in broiler chickens. Animals 10:2230. doi: 10.3390/ani10122230
National Research Council. (1994). Nutrient Requirements of Poultry Ninth Revised Edition. National Academies Press. 145.
Ogbuewu, I. P., Emenalom, O. O., and Okoli, I. C. (2015). Alternative feedstuffs and their effects on blood chemistry and haematology of rabbits and chickens: a review. Comp. Clin. Pathol. 26, 277–286. doi: 10.1007/s00580-015-2210-0
Ognik, K., Krauze, M., Cholewińska, E., and Abramowicz, K. (2017). The effect of a probiotic containing enterococcus faecium DSM 7134 on redox and biochemical parameters in chicken blood. Ann. Anim. Sci. 17, 1075–1088. doi: 10.1515/aoas-2016-0097
Pournazari, M., AA-Qotbi, A., Seidavi, A., and Corazzin, M. (2017). Prebiotics, probiotics and thyme (Thymus vulgaris) for broilers: performance, carcass traits and blood variables. Revista Colombiana de Ciencias Pecuarias 30, 3–10. doi: 10.17533/udea.rccp.v30n1a01
Qiu, K., Li, C. L., Wang, J., Qi, G. H., Gao, J., Zhang, H. J., et al. (2021). Effects of dietary supplementation with Bacillus subtilis, as an alternative to antibiotics, on growth performance, serum immunity, and intestinal health in broiler chickens. Front. Nutr. 8:786878. doi: 10.3389/fnut.2021.786878
Reuben, R. C., Sarkar, S. L., Ibnat, H., Roy, P. C., and Jahid, I. K. (2022). Novel mono- and multi-strain probiotics supplementation modulates growth, intestinal microflora composition and haemato-biochemical parameters in broiler chickens. Vet. Med. Sci. 8, 668–680. doi: 10.1002/vms3.709
Rhayat, L., Jacquier, V., Brinch, K. S., Nielsen, P., Nelson, A., Geraert, P. A., et al. (2017). Bacillus subtilis strain specificity affects performance improvement in broilers. Poult. Sci. 96, 2274–2280. doi: 10.3382/ps/pex018
Salehizadeh, M., Modarressi, M. H., Mousavi, S. N., and Ebrahimi, M. T. (2019). Effects of probiotic lactic acid bacteria on growth performance, carcass characteristics, hematological indices, humoral immunity, and IGF-I gene expression in broiler chicken. Trop. Anim. Health Prod. 51, 2279–2286. doi: 10.1007/s11250-019-01935-w
Samanya, M., and Yamauchi, K. E. (2002). Histological alterations of intestinal villi in chickens fed dried Bacillus subtilis var. natto. Comp. Biochem. Physiol. A Mol. Integr. Physiol. 133, 95–104. doi: 10.1016/s1095-6433(02)00121-6
Shehata, A. A., Tarabees, R., Basiouni, S., ElSayed, M. S., Gaballah, A., and Krueger, M. (2020). Effect of a potential probiotic candidate enterococcus faecalis-1 on growth performance, intestinal microbiota, and immune response of commercial broiler chickens. Probiotics Antimicrob. Proteins 12, 451–460. doi: 10.1007/s12602-019-09557-2
Shi, Y., Cui, X., Gu, S., Yan, X., Li, R., Xia, S., et al. (2019). Antioxidative and probiotic activities of lactic acid bacteria isolated from traditional artisanal Milk cheese from Northeast China. Probiotics Antimicrob. Proteins 11, 1086–1099. doi: 10.1007/s12602-018-9452-5
Takahashi, M., McCartney, E., Knox, A., Francesch, M., Oka, K., Wada, K., et al. (2018). Effects of the butyric acid-producing strain Clostridium butyricum MIYAIRI 588 on broiler and piglet zootechnical performance and prevention of necrotic enteritis. Anim. Sci. J. 89, 895–905. doi: 10.1111/asj.13006
Tang, X., Liu, X., and Liu, H. (2021). Effects of dietary probiotic (Bacillus subtilis) supplementation on carcass traits, meat quality, amino acid, and fatty acid profile of broiler chickens. Front. Vet. Sci. 8:767802. doi: 10.3389/fvets.2021.767802
Wang, J., Ishfaq, M., Miao, Y., Liu, Z., Hao, M., Wang, C., et al. (2022). Dietary administration of Bacillus subtilis KC1 improves growth performance, immune response, heat stress tolerance, and disease resistance of broiler chickens. Poultry science. 101:101693. doi: 10.1016/j.psj.2021.101693
Wealleans, A. L., Walsh, M. C., Romero, L. F., and Ravindran, V. (2017). Comparative effects of two multi-enzyme combinations and a bacillus probiotic on growth performance, digestibility of energy and nutrients, disappearance of non-starch polysaccharides, and gut microflora in broiler chickens. Poult. Sci. 96, 4287–4297. doi: 10.3382/ps/pex226
Wu, Y., Wang, B., Zeng, Z., Liu, R., Tang, L., Gong, L., et al. (2019). Effects of probiotics Lactobacillus plantarum 16 and Paenibacillus polymyxa 10 on intestinal barrier function, antioxidative capacity, apoptosis, immune response, and biochemical parameters in broilers. Poult. Sci. 98, 5028–5039. doi: 10.3382/ps/pez226
Yadav, M., Dubey, M., Yadav, M., and Shankar, K. S. (2018). Effect of supplementation of probiotic (Bacillus subtilis) on growth performance and carcass traits of broiler chickens. Int. J. Curr. Microbiol. App. Sci. 7, 4840–4849. doi: 10.20546/ijcmas.2018.708.510
Yahfoufi, N., Mallet, J. F., Graham, E., and Matar, C. (2018). Role of probiotics and prebiotics in immunomodulation. Curr. Opin. Food Sci. 20, 82–91. doi: 10.1016/j.cofs.2018.04.006
Yang, C. M., Cao, G. T., Ferket, P. R., Liu, T. T., Zhou, L., Zhang, L., et al. (2012). Effects of probiotic, Clostridium butyricum, on growth performance, immune function, and cecal microflora in broiler chickens. Poult. Sci. 91, 2121–2129. doi: 10.3382/ps.2011-02131
Zeng, X., Li, Q., Yang, C., Yu, Y., Fu, Z., Wang, H., et al. (2021). Effects of Clostridium butyricum- and Bacillus spp.-Based Potential Probiotics on the Growth Performance, Intestinal Morphology, Immune Responses, and Caecal Microbiota in Broilers. Antibiotics (Basel, Switzerland). 10:624. doi: 10.3390/antibiotics10060624
Zhang, X., Feng, H., He, J., Muhammad, A., Zhang, F., and Lu, X. (2022). Features and colonization strategies of Enterococcus faecalis in the gut of Bombyx mori. Front. Microbiol. 13:921330. doi: 10.3389/fmicb.2022.921330
Zhang, L., Zhang, L., Zhan, X., Zeng, X., Zhou, L., Cao, G., et al. (2016). Effects of dietary supplementation of probiotic, Clostridium butyricum, on growth performance, immune response, intestinal barrier function, and digestive enzyme activity in broiler chickens challenged with Escherichia coli K88. J. Anim. Sci. Biotechnol. 7:3. doi: 10.1186/s40104-016-0061-4
Zhang, S., Zhong, G., Shao, D., Wang, Q., Hu, Y., Wu, T., et al. (2021). Dietary supplementation with Bacillus subtilis promotes growth performance of broilers by altering the dominant microbial community. Poult. Sci. 100:100935. doi: 10.1016/j.psj.2020.12.032
Keywords: probiotic complex, AA+ male broiler, growth performance, blood parameters, fecal harmful gas, fecal microbiota
Citation: Zou Q, Fan X, Xu Y, Wang T and Li D (2022) Effects of dietary supplementation probiotic complex on growth performance, blood parameters, fecal harmful gas, and fecal microbiota in AA+ male broilers. Front. Microbiol. 13:1088179. doi: 10.3389/fmicb.2022.1088179
Edited by:
Wenyi Zhang, Inner Mongolia Agricultural University, ChinaReviewed by:
Shad Mahfuz, Sylhet Agricultural University, BangladeshEric Auclair, Phileo Lesaffre Animal Care, France
Doaa Ibrahim, Zagazig University, Egypt
Copyright © 2022 Zou, Fan, Xu, Wang and Li. This is an open-access article distributed under the terms of the Creative Commons Attribution License (CC BY). The use, distribution or reproduction in other forums is permitted, provided the original author(s) and the copyright owner(s) are credited and that the original publication in this journal is cited, in accordance with accepted academic practice. No use, distribution or reproduction is permitted which does not comply with these terms.
*Correspondence: Tieliang Wang, bG5qend0bEBzaW5hLmNvbQ==; Desheng Li, bGlkZXNoZW5nMDcyNjUyMUAxMjYuY29t
†These authors have contributed equally to this work