- 1Departamento de Genética, Ecologia e Evolução, Programa de Pós-Graduação em Genética, Instituto de Ciências Biológicas, Universidade Federal de Minas Gerais, Belo Horizonte, Minas Gerais, Brazil
- 2Departamento de Bioquímica e Imunologia, Instituto de Ciências Biológicas, Universidade Federal de Minas Gerais, Belo Horizonte, Minas Gerais, Brazil
- 3Departamento de Biologia Celular e Molecular e Bioagentes Patogênicos, Faculdade de Medicina de Ribeirão Preto, Universidade de São Paulo, Ribeirão Preto, Brazil
- 4Departamento de Imunologia, Instituto de Ciências Biomédicas, Universidade de São Paulo, São Paulo, Brazil
Programmed cell death (PCD) is an important mechanism of innate immunity against bacterial pathogens. The innate immune PCD pathway involves the molecules caspase-7 and caspase-8, among others. Brucella abortus is a gram-negative bacterium that causes a zoonotic disease termed brucellosis. The innate immune response against this pathogen involves activation of inflammasome components and induction of pyroptosis. However, no studies so far have revealed the role of caspase-7 or caspase-8 during this bacterial infection. Herein, we demonstrate that caspase-7 is dispensable for caspase-1 processing, IL-1β secretion and cell death in macrophages. Additionally, caspase-7 deficient animals control B. abortus infection as well as the wild type mice. Furthermore, we addressed the role of caspase-8 in inflammasome activation and pyroptosis during this bacterial infection. Macrophages deficient in caspase-8 secreted reduced amounts of IL-1β that parallels with diminished caspase-1 activity when compared to wild type cells. Additionally, caspase-8 KO macrophages showed reduced LDH release when compared to wild type, suggesting that caspase-8 may play an important role in pyroptosis in response to B. abortus. Finally, caspase-8 KO animals were more susceptible to Brucella infection when compared to wild type mice. Overall, this study contributes to a better understanding of the involvement of caspase-7 and caspase-8 in innate immunity against B. abortus infection.
Introduction
Brucellosis is a zoonosis caused by bacteria of the genus Brucella of worldwide distribution. Great advance has been made in the control of brucellosis in recent years. However, in many regions of the world, Brucella infection in domestic animals still persists, leading to frequent transmission to the human population. In regions such as the Mediterranean countries of Europe, like Portugal, Italy and Greece, brucellosis is still considered an important human disease and it is often neglected (Corbel, 1997).
Innate immunity is an important arm of the immune system involved in the control of Brucella infection. In previous studies performed by our research group, we demonstrated that receptors and adaptor molecules such as TLR9, AIM2, MyD88, and STING are important components in the protective response against Brucella infection (Macedo et al., 2008; Gomes et al., 2016; Costa Franco et al., 2018, 2019). In addition, we and others have shown that pyroptosis triggered upon activation of inflammasomes, is also an important mechanism in restricting in vivo infection against Brucella abortus (Cerqueira et al., 2018; Lacey et al., 2018). In recent study, we showed that caspase-11/GSDMD-dependent pyroptosis process triggered by B. abortus contributed to the restriction of infection in vivo by assisting in the recruitment and activation of immune cells such as neutrophils, macrophages, and dendritic cells (Cerqueira et al., 2018).
Programmed cell death (PCD) is a intricate circuit that involves the cross-talk among different caspases, and their substrates. Caspase-3 and caspase-7 are considered executioner molecules triggering host cell apoptosis (Kim et al., 2005). Recent study demonstrated that Brucella inhibited the PCD in early stage of infection to allow bacterial replication in host cells and promoted apoptosis in the later stage during infection in macrophages (Zhang et al., 2022). In the literature, there are some controversies in the role of caspase-7 during bacterial infections. Caspase-7 has been implicated in resistance to L. pneumophila through the NLRC4 inflammasome (Akhter et al., 2009). In contrast, Gonçalves et al. (Goncalves et al., 2019) demonstrated that mice with a single deletion in caspase-7 are not fully susceptible to L. pneumophila. The Casp7−/− did not phenocopy the susceptibility to L. pneumophila infection as observed in Nlrc4−/− animals.
Caspase-8, early on classified as an apoptotic caspase, has lately been shown to have a role in several inflammatory processes. Caspase-8 is involved in the inflammasome pathway and can be activated by NLRP3, AIM2 and NLRC4 in macrophages (Man et al., 2013; Sagulenko et al., 2013) and NLRP3 inflammasome in dendritic cells (Antonopoulos et al., 2015). Caspase-8 can act by controlling NF-kB signaling, influencing the positive regulation of components of the inflammasome, such as the NLRP3 and pro-IL-1β (Weng et al., 2014). It can also activate the inflammasome pathway in response to C. albicans β-glucans (Ganesan et al., 2014). Interestingly, upon TLR or death receptor activation, active caspase-8 can cleave the IL-1β precursor into its bioactive fragment at the same site as caspase-1 (Shenderov et al., 2014), and can directly cleave GSDMD into its N-terminal fragment, triggering pyroptosis during Yersinia infection (Sarhan et al., 2018). In addition, once the inflammasome is activated, but pyroptosis is impaired, caspase-8 can act leading to a cell death program. This has been demonstrated in studies with intracellular bacteria such as L. pneumophila and S. Typhimurium in the absence of caspase-1 or GSDMD (Mascarenhas et al., 2017; Lee et al., 2018).
To the best of our knowledge, the role of caspase-7 and caspase-8 in Brucella infection has not been addressed so far. Therefore, in order to expand the understanding of the mechanisms involved in the innate immune response and inflammatory cell death, we investigated the participation of the caspase-7 and caspase-8 molecules during B. abortus infection.
Materials and methods
Mice
Wild-type C57BL/6 (WT) mice were purchased from the Federal University of Minas Gerais (UFMG) and, Casp7−/−, Casp7/1/11−/−, Casp7/Gsdmd−/−, Gsdmd−/−, Casp8+/+/RIPK3−/−, and Casp8/RIPK3−/− were kindly provided by Dr. Prof. Dario Simões Zamboni, Department of Cell and Molecular Biology and Pathogenic Bioagents, Ribeirão Preto Medical School, University of Sao Paulo, Brazil. Genetically deficient and control mice were maintained at our facilities and used at 6–8 weeks of age. Mice were housed in filter top cages and provided with sterile water and food ad libitum. Groups of 5–7 animals were used to perform all experiments. The procedures for animal experimentation were approved by the Ethics Committee for the Use of Animals of the Federal University of Minas Gerais-CEUA/UFMG under protocol number 69/2020.
Bacteria and culture conditions
Brucella abortus virulent strain 2,308 was used in this study. To prepare the inoculum, the bacteria were grown in BB (Brucella Broth) medium (BD Biosciences, United States) for 24 h at 37°C under 180 rpm shaking, washed in PBS for 10 min, 5,000 rpm at 4°C, and resuspended in sterile PBS. The OD of the culture was measured at 600 nm in a spectrophotometer to determine the bacterial number in the solution.
Mice infection with Brucella abortus
Five to seven mice from each group were infected intraperitoneally (i.p.) with 1 × 106 B. abortus in 100 μl of PBS and the animals sacrificed at 14 days post-infection. The spleens were harvested and macerated in 10 ml saline (NaCl 0.9%), serially diluted, and plated in duplicated on Brucella Broth agar. Plates were incubated for 3 days at 37°C and the CFU number was determined.
Bone marrow-derived macrophages
BMDMs were differentiated in vitro from bone marrow cells extracted from mouse femurs. Cultures were differentiated for 7 days in an incubator at 37°C, 5% CO2 in DMEM medium supplemented with 1% HEPES, 20% fetal bovine serum (FBS), 30% L929 cell-conditioned medium (LCCM) source of M-CSF (important for differentiation of progenitor cells into macrophages), 100 U/ml penicillin and 100 μg/ml streptomycin (Thermo Fischer Scientific). After differentiation, macrophages were collected by washing the monolayers with ice-cold PBS, distributed on culture plates, and cultured in DMEM medium containing 1% SFB and 1% HEPES or 10% SFB and 1% HEPES and they were ready for use.
Lactate dehydrogenase release assay
For the lactate dehydrogenase (LDH) release assay, BMDMs were plated at 5 × 105 cells/well in 24-well plates and infected with B. abortus (MOI 100) for 8 h. RPMI 1640 medium without phenol red, with 1% glutamine, 1% FBS was used. Supernatants were collected, and LDH was quantified using the Cytotox96 LDH kit (Promega, Madison, WI) according to the manufacturer’s instructions. During infection, bacteria were opsonized with a polyclonal mouse antibody (anti-B.abortus, dilution 1:1,000) to ensure more efficient bacterial phagocytosis. This polyclonal antibody was generated by injecting 1 × 106 heat-killed bacteria/mouse. The animals were injected three times during a 15-day interval, and after this period, serum from each mouse was tested for the presence of the specific antibody and stored at −80°C.
Cytokine measurement
For cytokine determination, BMDMs were plated at a concentration of 5 × 105 cells/well in 24-well plates and the cells were infected with B. abortus at an MOI of 100 for 17 h. Supernatants were collected and cytokines were measured with the mouse IL-1β, ELISA kit (R&D systems, Minneapolis, MN) according to the manufacturer’s instructions.
Western blot analysis
BMDMs were cultured at 5 × 105 cells/well in 24-well plates. The cells were infected with B. abortus for 17 h as described above. After 17 h of infection, culture supernatants were harvested and cells were lysed with M-PER Mammalian Protein Extraction Reagent (Thermo Fisher Scientific) supplemented with 1:100 protease inhibitor mixture (Sigma-Aldrich). Cell lysates and supernatants were subjected to SDS-PAGE analysis as already described in previous studies by our research group (Cerqueira et al., 2018). The primary Abs used included a mouse monoclonal against the p20 subunit of caspase-1 (Adipogen, San Diego, CA, United States) at a dilution of 1:1,000. Loading control was performed using anti-β-actin mAb (Cell Signaling Technology, Danvers, MA) at a dilution of 1:1,000.
Statistical analysis
Statistical analysis was performed using Prism 5.0 software (GraphPad Software, San Diego, CA). The unpaired Student t-test was used to compare two groups. One-way ANOVA followed by multiple comparisons according to Tukey procedure was used to compare three or more groups. Unless otherwise stated, data are expressed as the mean ± SD. Differences were considered statistically significant at a p-value <0.05.
Results
Il-1β secretion in response to Brucella abortus occurs in a caspase-7-independent manner
The classical executioner caspases (caspase-7 and -3) are activated to initiate the process that culminate in the classical cell death signals (Nagata, 2018). Previous studies demonstrate that caspase-7 activation requires caspase-1 processing under inflammatory conditions (Lamkanfi and Kanneganti, 2010). To investigate whether caspase-7 participates in caspase-1 cleavage and IL-1β secretion during Brucella abortus infection, we infected BMDMs of C57BL/6 (WT), Casp7−/−, Casp7/1/11−/−, Casp7/Gsdmd−/−, and Gsdmd−/− with Brucella. After 17 h of infection, we evaluated IL-1β secretion in the supernatant of the cells (Figure 1A) and the lysate was properly prepared for the assay of caspase-1 processing by Western blot analysis (Figure 1B). In all assays, C57BL/6 and Gsdmd−/− animals were used as controls, since the importance of gasdermin-D (GSDMD) for the control of B.abortus infection had been demonstrated previously by our research group (Cerqueira et al., 2018). We observed that BMDMs from Casp7−/− mice secreted similar amounts of IL-1β as WT animals. In macrophages from Casp7/1/11−/−, Casp7/Gsdmd−/−, and Gsdmd−/− animals the amount of IL-1β secreted was dramatically reduced compared to C57BL/6. Caspase-1 cleavage was observed only in the C57BL/6 and Casp7−/− strains, corroborating with the IL-1β cytokine secretion profile. Collectively, these data suggest that caspase-7 has no significant impact in IL-1β secretion and caspase-1 cleavage in response to B. abortus infection.
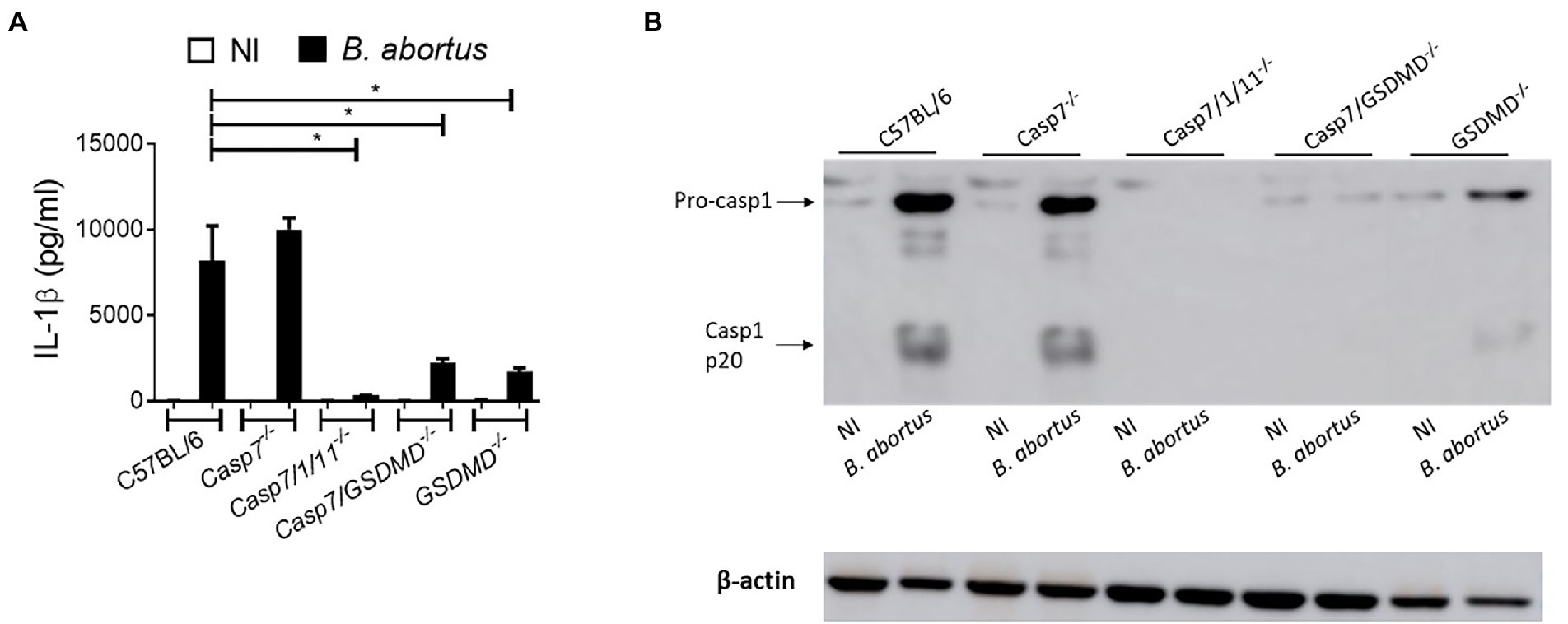
Figure 1. IL-1β production in response to Brucella abortus occurs in a caspase-7-independent manner. BMDMs were infected with B. abortus MOI: 100 for 17 h. (A) IL-1β measurement in the supernatant by ELISA. (B) Immunoblot analysis of caspase-1 processing. Data show the mean ± standard deviation of triplicates. The data are representative of three independent experiments. One-way ANOVA, *p < 0.05 compared to C57BL/6.
Caspase-7 does not participate in macrophage pyroptosis during Brucella abortus infection
Next, we addressed the role of caspase-7 in B. abortus induced cell death by quantifying LDH release in cell culture supernatants (Figure 2). BMDMs from C57BL/6, Casp7−/−, Casp7/1/11−/−, Casp7/Gsdmd−/−, and Gsdmd−/− mice were infected with B.abortus and after 8 h of infection, LDH was quantified in the supernatant. B. abortus infection triggered higher LDH release in BMDMs of C57BL6 and Casp7−/− strains when compared to Casp7/1/11−/−, Casp7/Gsdmd−/−, and Gsdmd−/− cells. This finding suggests that caspase-7 does not have a role in the induction of programmed cell death in response to B. abortus infection.
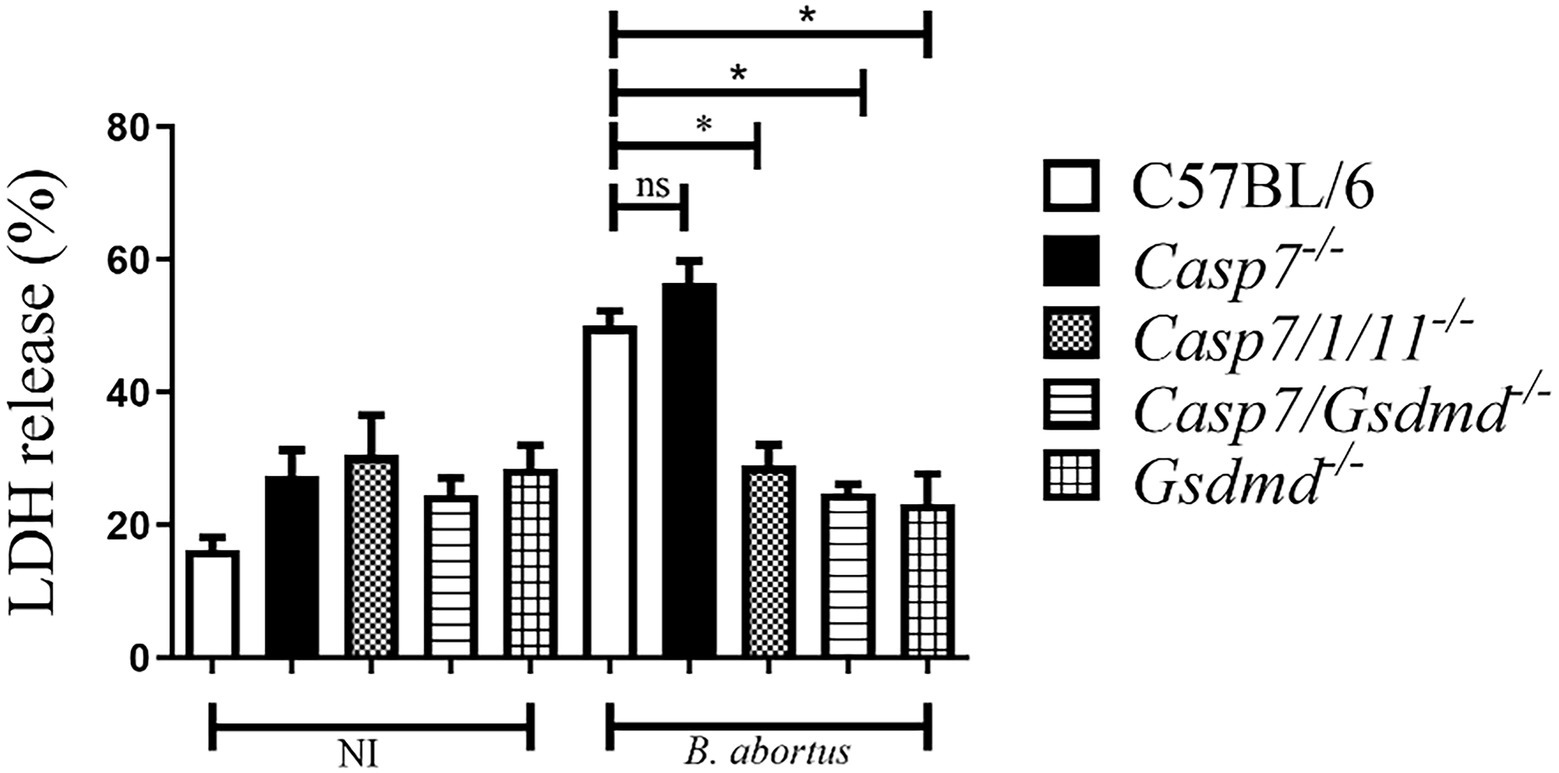
Figure 2. Caspase-7 does not participate in macrophage pyroptosis during B. abortus infection. BMDMs were infected with Brucella abortus MOI: 100 for 8 h and LDH quantification was performed in the cell supernatant. Values represent the percentage of LDH released compared to control cells lysed with Triton X-100. The data show the mean ± standard deviation representative of three independent experiments. One-way ANOVA, *p < 0.05 compared to C57BL/6.
Caspase-7 plays no role in Brucella abortus infection in vivo
To determine whether the absence of caspase-7 influences the control of Brucella infection in vivo, we infected C57BL/6, Casp7−/−, Casp7/1/11−/−, Casp7/Gsdmd−/−, and Gsdmd−/− mouse strains intraperitoneally and after 2 weeks the animals were sacrificed and the spleens were removed for quantification of the number of bacterial CFU. As shown in Figure 3, the bacterial burden measured in Casp7−/− animals showed no difference compared to the C57BL/6 controls. Higher bacterial numbers were observed in Casp7/1/11−/−, Casp7/Gsdmd−/−, and Gsdmd−/− mice. This result demonstrates that this susceptibility profile to infection occurred not because the lack of caspase-7, but rather, because of the deletion of Casp1/11 or Gsdmd.
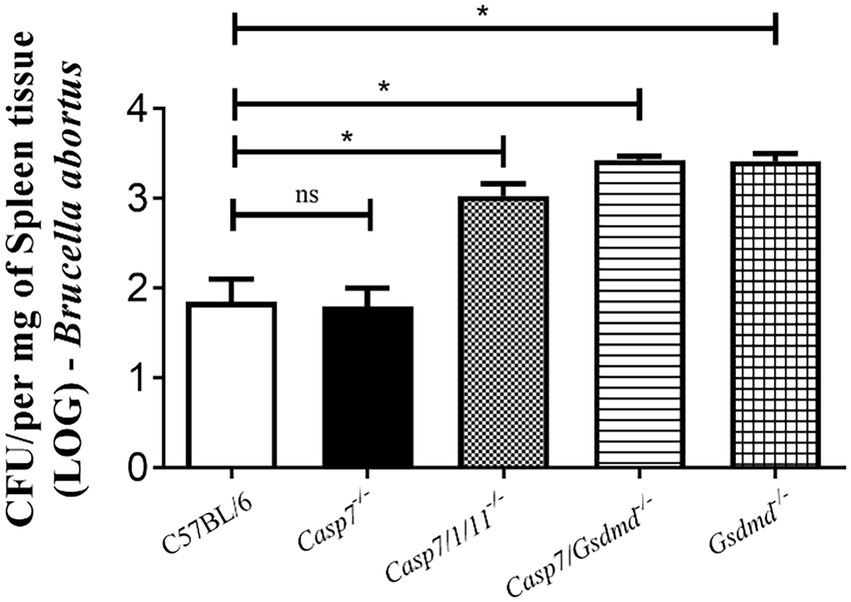
Figure 3. The role of caspase-7 in controlling Brucella abortus infection in vivo. Mice were infected intraperitoneally with 1 × 106 CFU of B. abortus and sacrificed after 2 weeks of infection, and spleen homogenates were seeded onto plates containing BB agar medium for CFU determination. Data shown are the mean ± standard deviation of five mice/group. The data are representative of three independent experiments. One-way ANOVA, *p < 0.05, compared to wild-type mice.
Caspase-8 participates in caspase-1 cleavage and IL-1β secretion in response to Brucella abortus
Further, we addressed the role of caspase-8 in regulating the inflammatory response during B.abortus infection. Deletion of caspase-8 results in RIPK3-dependent embryonic lethality. To rescue this viability, additional deletion of the RIPK3 kinase via the CRISPR/Cas9 technique was required (Wang et al., 2013). Therefore, the animals used in this study deficient for caspase-8 possess the additional deletion of RIPK3. We infected BMDMs of C57BL/6, Gsdmd−/−, Casp8/RIPK3−/−, and Casp8+/+/RIPK3−/− mouse strains with B. abortus, and after 17 h of infection, we evaluated the secretion of IL-1β in the supernatant of the cells. Additionally, cell lysates were properly prepared for caspase-1 processing by Western blot analysis. BMDMs of Casp8/RIPK3−/− secreted reduced amounts of IL-1β compared to the WT and Casp8+/+/RIPK3−/− controls, similar to the profile observed in Gsdmd−/− macrophages (Figure 4A). Further, the immunoblot data corroborate with the IL-1β secretion profile, where caspase-1 cleavage was detected only in the C57BL/6 and Casp8+/+/RIPK3−/− cells (Figure 4B). These data suggest an important role of caspase-8 in caspase-1 cleavage and consequent IL-1β secretion during B. abortus infection.
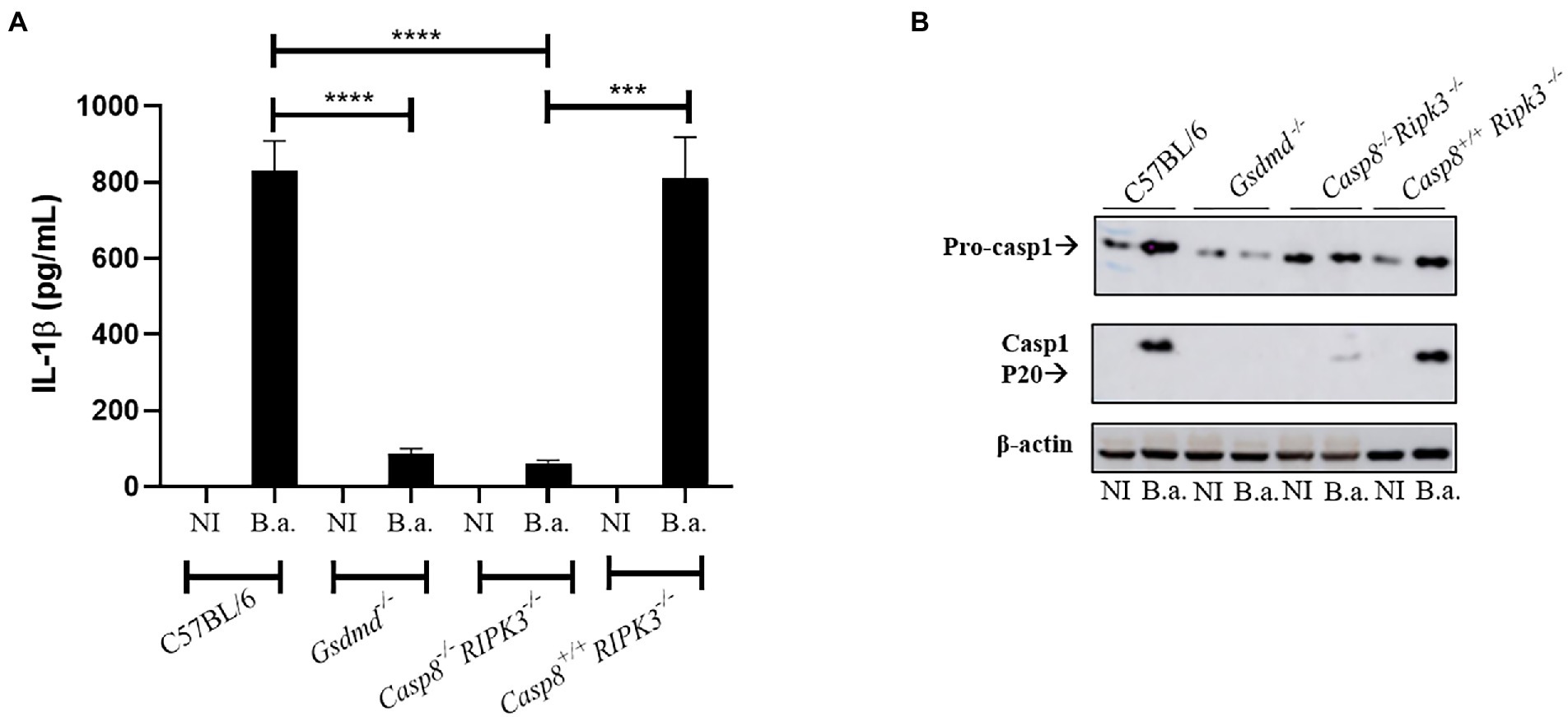
Figure 4. Caspase-8 participates in caspase-1 cleavage and IL-1β secretion in response to B. abortus. BMDMs obtained from C57BL/6, Gsdmd−/−, Casp8/RIPK3−/− and Casp8+/+/RIPK3−/− mice were uninfected (NI) or infected with B. abortus S2308 with MOI 100 for 17 h. The supernatant was collected and subjected to ELISA assay to estimate the concentration of IL-1β (A). The supernatant was labeled with anti-caspase-1 p20 monoclonal antibody (B). Data show the mean ± the standard deviation of triplicates. The data are representative of three independent experiments. Student t-test, ****p < 0.0001 compared to C57BL/6 or Casp8+/+/RIPK3−/−. ***p <0.001.
Lack of caspase-8 interferes with cell death induced by Brucella abortus infection
Our results demonstrate that caspase-8 influences inflammasome activation induced by Brucella, since caspase-1 activation and IL-1β secretion occurs in a caspase-8-dependent manner. Thus, we sought to investigate whether caspase-8 is involved in pyroptosis induced by B.abortus. Macrophages from C57BL/6, Gsdmd−/−, and Casp8/RIPK3−/− strains were infected with B.abortus for 8 h. After this period, we performed the quantification of LDH release in cell culture supernatants (Figure 5). LDH release was greatly reduced in cells from animals deficient for caspase-8 and GSDMD, suggesting a potential role of caspase-8 in the induction of cell death in response to B.abortus.
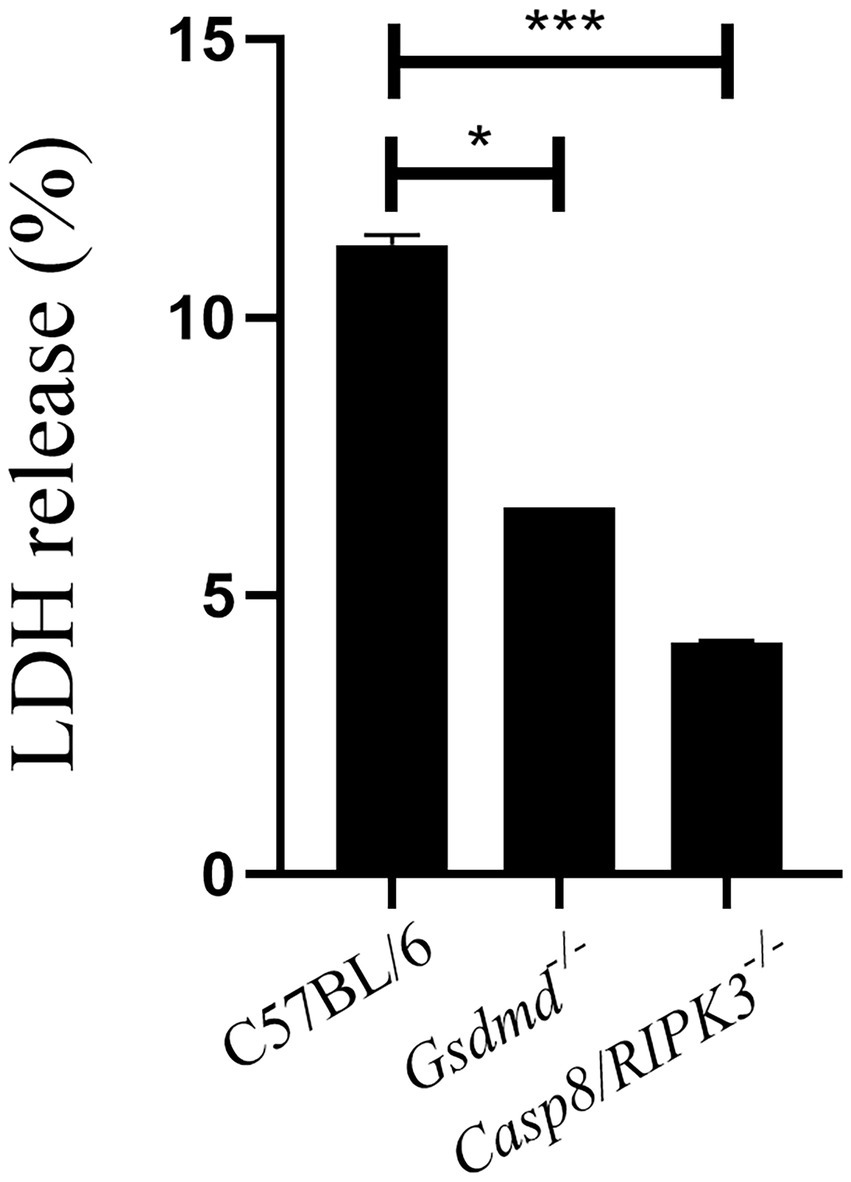
Figure 5. Caspase-8 is important to induce cell death during B.abortus infection. BMDMs of C57BL/6, Gsdmd−/− and Casp8/RIPK3−/− were infected with B. abortus with MOI 100 for 8hs and LDH quantification was performed in the cell supernatant. Values represent the percentage of LDH released compared to control cells lysed with Triton X-100. Data show the mean ± the representative standard deviation of triplicates. The data are representative of three independent experiments. Student t-test, *p < 0.05, ***p < 0.001 compared to C57BL/6.
Absence of caspase-8 enhances susceptibility to Brucella abortus infection in vivo
Since BMDMs deficient in caspase-8 showed reduced caspase-1 activation and secretion of IL-1β levels, we finally evaluated whether caspase-8 also played a role in restricting Brucella infection in mice. First, C57BL/6, Gsdmd−/−, and Casp8/RIPK3−/− mice were infected intraperitoneally with B. abortus. After 2 weeks of infection, bacterial colony forming units (CFU) were determined from spleen homogenates. The recovery of bacteria in the spleen of Casp8/RIPK3−/− animals was higher than the WT control group, in a manner very similar to that observed in Gsdmd−/− animals (Figure 6). Collectively, these data suggest that caspase-8 is involved in an inflammatory response and in the control of B. abortus in vivo.
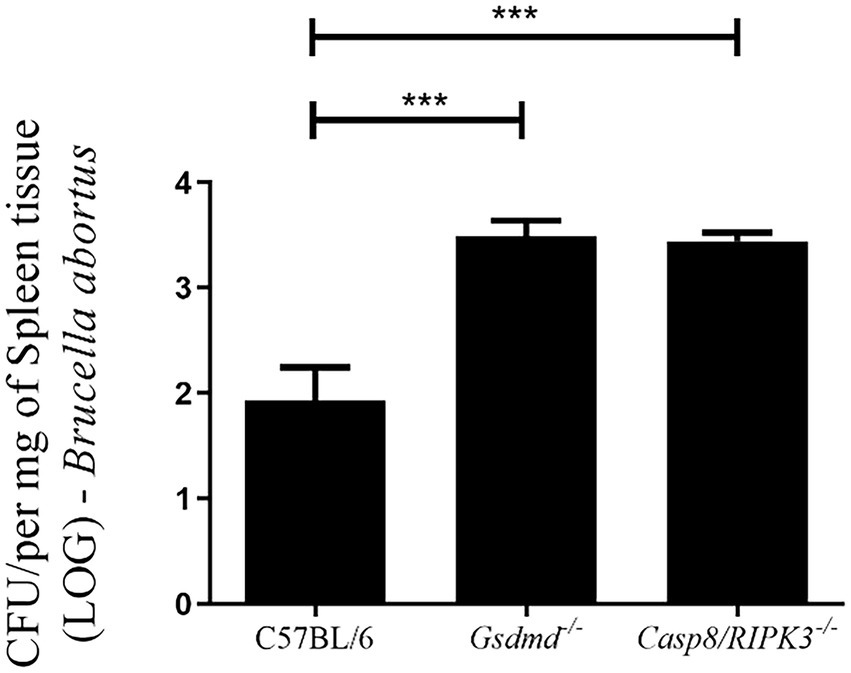
Figure 6. Caspase-8 influences the resistance to Brucella infection in vivo. C57BL/6, Gsdmd−/− and Casp8/RIPK3−/− m ice were infected intraperitoneally with 1×106 CFU of B. abortus. Animals were sacrificed 2 weeks after infection and diluted spleen homogenates were plated on agar plates containing BB medium for CFU determination. Data shown are the mean ± standard deviation of five mice/group. The graph is representative of three independent experiments. One-way ANOVA, ***p < 0.001 compared to C57BL/6.
Discussion
Programmed cell death (PCD) can be activated in response to different stimuli (Nagata, 2018). Apoptosis, necroptosis and pyroptosis are three types of cell death that have major involvement in immune response and disease control (Schwarzer et al., 2020). Apoptosis helps in the destruction and removal of infected cells during bacterial infections (Speir et al., 2016). In the case of Brucella, some studies have already established that a virulent strain inhibits cell death in macrophages to allow bacterial replication (Chen et al., 2011). In contrast, in dendritic cells, astrocytes and T lymphocytes, the smooth strain induced apoptotic cell death (Garcia Samartino et al., 2010; Velasquez et al., 2012). Caspase-7, like caspase-3, is an executing caspase, both of which are activated by caspases-8 and -9 during death receptor-induced apoptosis, under certain conditions (Lamkanfi et al., 2002). Recently, some studies show that caspase-7 has a distinct role from caspase-3 during activation of apoptosis and also has a role in the inflammatory response against bacterial pathogens (Slee et al., 2001). Studies using Salmonella typhymurium infection of macrophages or cells stimulated with LPS and ATP, showed that caspase-7 activation was caspase-1-dependent. Additionally, Akhter et al. have observed in the absence of caspase-7 impaired ability of macrophages to restrict intracellular replication of Legionella pneumophila (Akhter et al., 2009). In contrast, Gonçalves et al. (Goncalves et al., 2019) demonstrated that lack of caspase-7 is not required to control L. pneumophila replication in vitro and in vivo. In another study, using an in vivo septic shock model, caspase-7-deficient mice were shown to be resistant to lethality induced by intraperitoneal injections of LPS (Lamkanfi et al., 2009). However, in our study caspase-7 does not appear to participate in the control of B.abortus infection. Inflammasome activation with cleavage of caspase-1 and secretion of IL-1β is not affected in the absence of caspase-7, as well as induction of cell death and in vivo susceptibility to infection. We have previously shown the participation of caspase-1, caspase-11 and GSDMD in controlling B. abortus (Cerqueira et al., 2018). Therefore, susceptibility to Brucella infection observed in Casp7/1/11−/− and Casp7/Gsdmd−/− animals is not due to lack of caspase-7 but rather the absence of caspase-1/11 and GSDMD.
Necroptosis is a programmed cell death pathway, with inflammatory features, that involves the kinases RIPK1 and RIPK3 and the pore-forming pseudokinase MLKL. When RIPK3 is phosphorylated, oligomerization of MLKL is initiated and subsequently inserts into the plasma membrane of the cell, leading to pore formation and cell rupture (Tummers and Green, 2017). Caspase-8 is involved in apoptosis and pyroptosis mechanisms of cell death, and when caspase-8 is inhibited RIPK1 interacts with RIPK3 leading to necroptosis (Pandian and Kanneganti, 2022). Pyroptosis is another type of inflammatory programmed cell death triggered by inflammasome activation, and for a long time, it was considered to be caspase-1-mediated in response to bacterial challenge. However, when caspase-11 was shown to detect intracellular LPS and also serve as a trigger to pyroptosis, the role of pyroptosis expanded widely (Kayagaki et al., 2011). Herein, we observed that in WT infected cells LDH release occurs, corroborating with data from our previous study where we showed that B. abortus infection triggers pyroptosis, and this phenomenon is GSDMD-dependent (Cerqueira et al., 2018). Additionally, in this study, we demonstrated that cell death induced by Brucella was also shown to be caspase-8-dependent. Caspase-8 contributes to activation of canonical and noncanonical inflammasomes. During Salmonella infection, caspase-8 can be recruited to the NLRC4 inflammasome regulating IL-1β secretion, but not playing a role in cell death (Man et al., 2013). In contrast, in Yersinia infection model, like we observed in this study, caspase-8 activates GSDMD to induce cell death (Sarhan et al., 2018). Furthermore, we observed here that mice deficient for caspase-8 and GSDMD are more susceptible to Brucella infection in vivo compared to wild type animals, suggesting that pyroptosis triggered during B. abortus infection is an important mechanism to control infection.
Several studies have already identified cellular functions for the GSDMD-mediated pore, such as secretion of molecules such as IL-1β and IL-1α and eicosanoids, which are important for recruiting neutrophils to the site of infection and promoting phagocytosis of infected cells and contributing to infection restriction (Jorgensen et al., 2016). Herein, reduced IL-1β secretion and pyroptosis observed in Casp8/RIPK3−/− mice are possible mechanisms that may contribute to increased susceptibility to infection. Although we did not investigate cell recruitment in this study, we hypothesize that innate cells recruitment to the site of infection may be impaired by the absence of pyroptosis in caspase-8 deficient animals, which could in part explain the increased bacterial load observed in these animals. Recently, caspase-8 was involved in Aspergillus fumigatus keratitis being critical in the recruitment of inflammatory cells and the clearance of the fungus (Wang et al., 2022). In summary, we suggest that caspase-8 plays an important role in cell death induced during B.abortus infection, contributing to inflammation and infection control in mice.
Data availability statement
The raw data supporting the conclusions of this article will be made available by the authors, without undue reservation.
Ethics statement
The animal study was reviewed and approved by the procedures for animal experimentation were approved by the Ethics Committee for the Use of Animals of the Federal University of Minas Gerais-CEUA/UFMG under protocol number 69/2020.
Author contributions
RS performed all the experiments and wrote the manuscript. DC participated in the design of this study, provided assistance with data acquisition, data analysis, and statistical analysis. DZ participated in the design of this study and provided reagents to perform the experiments. SO participated in the design of this study, provided assistance with data acquisition and wrote and reviewed the manuscript. All authors contributed to the article and approved the submitted version.
Funding
This work was supported by grants from the Conselho Nacional de Desenvolvimento Científico e Tecnológico to SO (CNPq, www.cnpq.br; grant# 303044/2020-9), Fundação de Amparo a Pesquisa do Estado de Minas Gerais to SO (FAPEMIG, www.fapemig.br; grants# APQ #01945/17 and Rede Mineira de Imunobiológicos #00140-16), National Institutes of Health to SO (NIH, www.nih.gov; grant# R01 AI116453).
Conflict of interest
The authors declare that the research was conducted in the absence of any commercial or financial relationships that could be construed as a potential conflict of interest.
Publisher’s note
All claims expressed in this article are solely those of the authors and do not necessarily represent those of their affiliated organizations, or those of the publisher, the editors and the reviewers. Any product that may be evaluated in this article, or claim that may be made by its manufacturer, is not guaranteed or endorsed by the publisher.
References
Akhter, A., Gavrilin, M. A., Frantz, L., Washington, S., Ditty, C., Limoli, D., et al. (2009). Caspase-7 activation by the Nlrc 4/Ipaf inflammasome restricts legionella pneumophila infection. PLoS Pathog. 5:e1000361. doi: 10.1371/journal.ppat.1000361
Antonopoulos, C., Russo, H. M., El Sanadi, C., Martin, B. N., Li, X., Kaiser, W. J., et al. (2015). Caspase-8 as an effector and regulator of NLRP3 Inflammasome signaling. J. Biol. Chem. 290, 20167–20184. doi: 10.1074/jbc.M115.652321
Cerqueira, D. M., Gomes, M. T. R., Silva, A. L. N., Rungue, M., Assis, N. R. G., Guimaraes, E. S., et al. (2018). Guanylate-binding protein 5 licenses caspase-11 for Gasdermin-D mediated host resistance to Brucella abortus infection. PLoS Pathog. 14:e1007519. doi: 10.1371/journal.ppat.1007519
Chen, F., Ding, X., Ding, Y., Xiang, Z., Li, X., Ghosh, D., et al. (2011). Proinflammatory caspase-2-mediated macrophage cell death induced by a rough attenuated Brucella suis strain. Infect. Immun. 79, 2460–2469. doi: 10.1128/IAI.00050-11
Corbel, M. J. (1997). Brucellosis: an overview. Emerg. Infect. Dis. 3, 213–221. doi: 10.3201/eid0302.970219
Costa Franco, M. M. S., Marim, F. M., Alves-Silva, J., Cerqueira, D., Rungue, M., Tavares, I. P., et al. (2019). AIM2 senses Brucella abortus DNA in dendritic cells to induce IL-1beta secretion, pyroptosis and resistance to bacterial infection in mice. Microbes Infect. 21, 85–93. doi: 10.1016/j.micinf.2018.09.001
Costa Franco, M. M., Marim, F., Guimaraes, E. S., Assis, N. R. G., Cerqueira, D. M., Alves-Silva, J., et al. (2018). Brucella abortus triggers a cGAS-independent STING pathway to induce host protection that involves guanylate-binding proteins and Inflammasome activation. J. Immunol. 200, 607–622. doi: 10.4049/jimmunol.1700725
Ganesan, S., Rathinam, V. A. K., Bossaller, L., Army, K., Kaiser, W. J., Mocarski, E. S., et al. (2014). Caspase-8 modulates dectin-1 and complement receptor 3-driven IL-1beta production in response to beta-glucans and the fungal pathogen, Candida albicans. J Immunol 193, 2519–2530. doi: 10.4049/jimmunol.1400276
Garcia Samartino, C., Delpino, M. V., Pott Godoy, C., Di Genaro, M. S., Pasquevich, K. A., Zwerdling, A., et al. (2010). Brucella abortus induces the secretion of proinflammatory mediators from glial cells leading to astrocyte apoptosis. Am. J. Pathol. 176, 1323–1338. doi: 10.2353/ajpath.2010.090503
Gomes, M. T., Campos, P. C., Pereira Gde, S., Bartholomeu, D. C., Splitter, G., and Oliveira, S. C. (2016). TLR9 is required for MAPK/NF-kappa B activation but does not cooperate with TLR2 or TLR6 to induce host resistance to Brucella abortus. J. Leukoc. Biol. 99, 771–780. doi: 10.1189/jlb.4A0815-346R
Goncalves, A. V., Margolis, S. R., Quirino, G. F. S., Mascarenhas, D. P. A., Rauch, I., Nichols, R. D., et al. (2019). Gasdermin-D and Caspase-7 are the key Caspase-1/8 substrates downstream of the NAIP5/NLRC4 inflammasome required for restriction of legionella pneumophila. PLoS Pathog. 15:e1007886. doi: 10.1371/journal.ppat.1007886
Jorgensen, I., Zhang, Y., Krantz, B. A., and Miao, E. A. (2016). Pyroptosis triggers pore-induced intracellular traps (PITs) that capture bacteria and lead to their clearance by efferocytosis. J. Exp. Med. 213, 2113–2128. doi: 10.1084/jem.20151613
Kayagaki, N., Warming, S., Lamkanfi, M., Vande Walle, L., Louie, S., Dong, J., et al. (2011). Non-canonical inflammasome activation targets caspase-11. Nature 479, 117–121. doi: 10.1038/nature10558
Kim, H. E., Du, F., Fang, M., and Wang, X. (2005). Formation of apoptosome is initiated by cytochrome c-induced dATP hydrolysis and subsequent nucleotide exchange on Apaf-1. Proc. Natl. Acad. Sci. U. S. A. 102, 17545–17550. doi: 10.1073/pnas.0507900102
Lacey, C. A., Mitchell, W. J., Dadelahi, A. S., and Skyberg, J. A. (2018). Caspase-1 and Caspase-11 mediate Pyroptosis, inflammation, and control of Brucella joint infection. Infect. Immun. 86, e00361–18. doi: 10.1128/IAI.00361-18
Lamkanfi, M., Declercq, W., Kalai, M., Saelens, X., and Vandenabeele, P. (2002). Alice in caspase land. A phylogenetic analysis of caspases from worm to man. Cell Death Differ. 9, 358–361. doi: 10.1038/sj.cdd.4400989
Lamkanfi, M., and Kanneganti, T. D. (2010). Caspase-7: a protease involved in apoptosis and inflammation. Int. J. Biochem. Cell Biol. 42, 21–24. doi: 10.1016/j.biocel.2009.09.013
Lamkanfi, M., Moreira, L. O., Makena, P., Spierings, D. C., Boyd, K., Murray, P. J., et al. (2009). Caspase-7 deficiency protects from endotoxin-induced lymphocyte apoptosis and improves survival. Blood 113, 2742–2745. doi: 10.1182/blood-2008-09-178038
Lee, B. L., Mirrashidi, K. M., Stowe, I. B., Kummerfeld, S. K., Watanabe, C., Haley, B., et al. (2018). ASC- and caspase-8-dependent apoptotic pathway diverges from the NLRC4 inflammasome in macrophages. Sci. Rep. 8:3788. doi: 10.1038/s41598-018-21998-3
Macedo, G. C., Magnani, D. M., Carvalho, N. B., Bruna-Romero, O., Gazzinelli, R. T., and Oliveira, S. C. (2008). Central role of MyD88-dependent dendritic cell maturation and proinflammatory cytokine production to control Brucella abortus infection. J. Immunol. 180, 1080–1087. doi: 10.4049/jimmunol.180.2.1080
Man, S. M., Tourlomousis, P., Hopkins, L., Monie, T. P., Fitzgerald, K. A., and Bryant, C. E. (2013). Salmonella infection induces recruitment of Caspase-8 to the inflammasome to modulate IL-1beta production. J. Immunol. 191, 5239–5246. doi: 10.4049/jimmunol.1301581
Mascarenhas, D. P. A., Cerqueira, D. M., Pereira, M. S. F., Castanheira, F. V. S., Fernandes, T. D., Manin, G. Z., et al. (2017). Inhibition of caspase-1 or gasdermin-D enable caspase-8 activation in the Naip 5/NLRC4/ASC inflammasome. PLoS Pathog. 13:e1006502. doi: 10.1371/journal.ppat.1006502
Nagata, S. (2018). Apoptosis and clearance of apoptotic cells. Annu. Rev. Immunol. 36, 489–517. doi: 10.1146/annurev-immunol-042617-053010
Pandian, N., and Kanneganti, T. D. (2022). PANoptosis: a unique innate immune inflammatory cell death modality. J. Immunol. 209, 1625–1633. doi: 10.4049/jimmunol.2200508
Sagulenko, V., Thygesen, S. J., Sester, D. P., Idris, A., Cridland, J. A., Vajjhala, P. R., et al. (2013). AIM2 and NLRP3 inflammasomes activate both apoptotic and pyroptotic death pathways via ASC. Cell Death Differ. 20, 1149–1160. doi: 10.1038/cdd.2013.37
Sarhan, J., Liu, B. C., Muendlein, H. I., Li, P., Nilson, R., Tang, A. Y., et al. (2018). Caspase-8 induces cleavage of gasdermin D to elicit pyroptosis during Yersinia infection. Proc. Natl. Acad. Sci. U. S. A. 115, E10888–E10897. doi: 10.1073/pnas.1809548115
Schwarzer, R., Laurien, L., and Pasparakis, M. (2020). New insights into the regulation of apoptosis, necroptosis, and pyroptosis by receptor interacting protein kinase 1 and caspase-8. Curr. Opin. Cell Biol. 63, 186–193. doi: 10.1016/j.ceb.2020.02.004
Shenderov, K., Riteau, N., Yip, R., Mayer-Barber, K. D., Oland, S., Hieny, S., et al. (2014). Cutting edge: endoplasmic reticulum stress licenses macrophages to produce mature IL-1beta in response to TLR4 stimulation through a caspase-8- and TRIF-dependent pathway. J. Immunol. 192, 2029–2033. doi: 10.4049/jimmunol.1302549
Slee, E. A., Adrain, C., and Martin, S. J. (2001). Executioner caspase-3, -6, and -7 perform distinct, non-redundant roles during the demolition phase of apoptosis. J. Biol. Chem. 276, 7320–7326. doi: 10.1074/jbc.M008363200
Speir, M., Lawlor, K. E., Glaser, S. P., Abraham, G., Chow, S., Vogrin, A., et al. (2016). Eliminating legionella by inhibiting BCL-XL to induce macrophage apoptosis. Nat. Microbiol. 1:15034. doi: 10.1038/nmicrobiol.2015.34
Tummers, B., and Green, D. R. (2017). Caspase-8: regulating life and death. Immunol. Rev. 277, 76–89. doi: 10.1111/imr.12541
Velasquez, L. N., Delpino, M. V., Ibanez, A. E., Coria, L. M., Miraglia, M. C., Scian, R., et al. (2012). Brucella abortus induces apoptosis of human T lymphocytes. Microbes Infect. 14, 639–650. doi: 10.1016/j.micinf.2012.02.004
Wang, L., Yan, H., Chen, X., Lee, J., Sun, J., Liu, G., et al. (2022). Caspase-8 is involved in pyroptosis, necroptosis and the maturation and release of IL-1beta in aspergillus fumigatus keratitis. Int. Immunopharmacol. 113:109275. doi: 10.1016/j.intimp.2022.109275
Wang, H., Yang, H., Shivalila, C. S., Dawlaty, M. M., Cheng, A. W., Zhang, F., et al. (2013). One-step generation of mice carrying mutations in multiple genes by CRISPR/Cas-mediated genome engineering. Cells 153, 910–918. doi: 10.1016/j.cell.2013.04.025
Weng, D., Marty-Roix, R., Ganesan, S., Proulx, M. K., Vladimer, G. I., Kaiser, W. J., et al. (2014). Caspase-8 and RIP kinases regulate bacteria-induced innate immune responses and cell death. Proc. Natl. Acad. Sci. U. S. A. 111, 7391–7396. doi: 10.1073/pnas.1403477111
Keywords: caspase-8, caspase-7, Brucella abortus, inflammasome, pyroptosis, innate immunity
Citation: Santos RA, Cerqueira DM, Zamboni DS and Oliveira SC (2022) Caspase-8 but not caspase-7 influences inflammasome activation to act in control of Brucella abortus infection. Front. Microbiol. 13:1086925. doi: 10.3389/fmicb.2022.1086925
Edited by:
Axel Cloeckaert, Institut National de recherche pour l’agriculture, l’alimentation et l’environnement (INRAE), FranceReviewed by:
Clayton Caswell, Virginia Tech, United StatesJuan Esteban Ugalde, Universidad Nacional de San Martin, Argentina
Gary Splitter, University of Wisconsin-Madison, United States
Copyright © 2022 Santos, Cerqueira, Zamboni and Oliveira. This is an open-access article distributed under the terms of the Creative Commons Attribution License (CC BY). The use, distribution or reproduction in other forums is permitted, provided the original author(s) and the copyright owner(s) are credited and that the original publication in this journal is cited, in accordance with accepted academic practice. No use, distribution or reproduction is permitted which does not comply with these terms.
*Correspondence: Sergio C. Oliveira, scozeus@icb.ufmg.br