- 1College of Health Preservation and Rehabilitation, Chengdu University of Traditional Chinese Medicine, Chengdu, Sichuan, China
- 2College of Acupuncture and Tuina, Chengdu University of Traditional Chinese Medicine, Chengdu, Sichuan, China
Short-chain fatty acids (SCFAs) are metabolites of gut microbes that can modulate the host inflammatory response, and contribute to health and homeostasis. Since the introduction of the gut-skin axis concept, the link between SCFAs and inflammatory skin diseases has attracted considerable attention. In this review, we have summarized the literature on the role of SCFAs in skin inflammation, and the correlation between SCFAs and inflammatory skin diseases, especially atopic dermatitis, urticaria, and psoriasis. Studies show that SCFAs are signaling factors in the gut-skin axis and can alleviate skin inflammation. The information presented in this review provides new insights into the molecular mechanisms driving gut-skin axis regulation, along with possible pathways that can be targeted for the treatment and prevention of inflammatory skin diseases.
1. Introduction
Inflammatory skin disease refers to the inflammation response in the skin, which manifests as skin redness, swelling, itching or scaling. Common inflammatory skin diseases, including atopic dermatitis (AD), psoriasis, acne, and urticaria, are associated with other comorbidities and impose a significant burden on the patients (Narla and Silverberg, 2020). Although the above diseases vary in their clinical presentation, they may share common physio-pathological pathways (Diotallevi et al., 2022). Recent studies have shown that the gut microbiome is an important factor influencing host immunity, inflammation and metabolism (Yao et al., 2022). The gut-skin axis is a fairly recent concept that refers to the bidirectional relationship between the gut microbiome and skin, and an increasing body of evidence suggests that changes in the gut microbiome is related with skin inflammation (Kim et al., 2020; Chun et al., 2021). The gut and the skin are both highly innervated and vascularized organs, and harbor numerous resident microorganisms with similar functions (O’Neill et al., 2016). Furthermore, various skin conditions have been linked to an altered gut microbiome (Pessemier et al., 2021).
Short-chain fatty acids (SCFAs), mainly including acetate, propionate, butyrate, isobutyrate, valerate, and isovalerate, contain less than 6 carbon atoms and are the final product of the fermentation of resistant starch and dietary fiber by specific gut microbiota (Tan et al., 2014; Rauf et al., 2022). SCFAs are transported from the intestine to distant organs and tissues through the peripheral circulation (Canfora et al., 2015; Hee and Wells, 2021), and bind to G protein-coupled receptors (GPCRs) that are expressed on skin cells, leukocytes, neutrophils, and other types of cells, thereby exerting direct influence on tissue metabolism and function (Krejner et al., 2018; Schlatterer et al., 2021). Recent studies have shown that SCFAs mitigate inflammation by regulating the production of cytokines by immune cells such as neutrophils, macrophages, dendritic cells (DCs) and T-cells (Yao et al., 2022). However, the role of SCFAs in inflammatory skin diseases has not been completely elucidated. In this review, we summarized the literature on the relationship between SCFAs and skin inflammation, and discussed the therapeutic potential of SCFAs against inflammatory skin diseases.
2. The potential pathway of SCFAs in inflammatory skin diseases
The potential anti-inflammatory mechanisms of SCFAs in inflammatory skin diseases are related to specific membrane receptors, histone deacetylase (HDAC) inhibitors, and metabolic pathways (Figure 1).
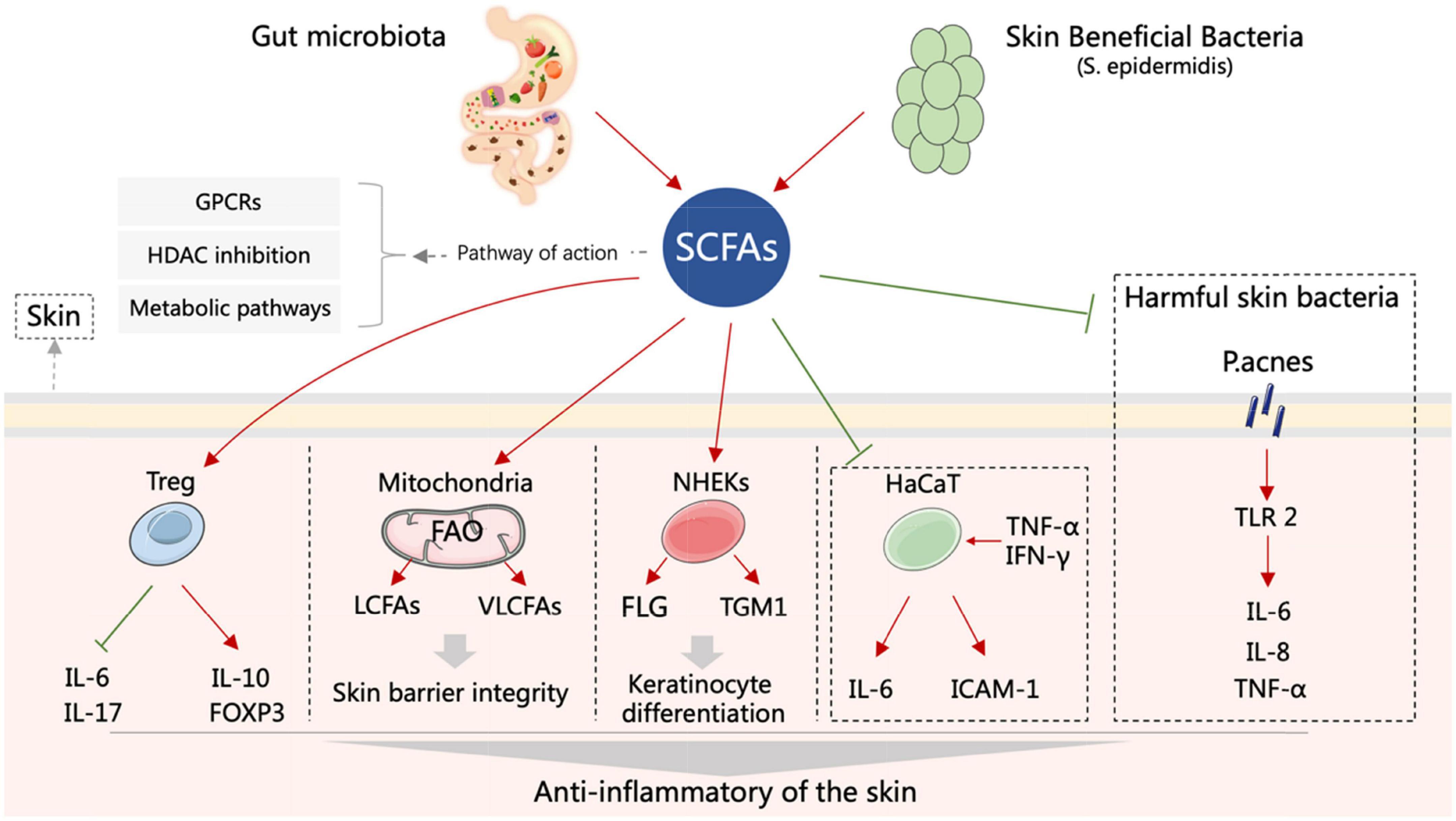
Figure 1. Schematic representation of the roles of SCFAs in inflammatory skin diseases. Both gut and skin microbiota can produce SCFAs. SCFAs can enhance the activity of Treg, improve mitochondrial metabolism, promote keratinocyte differentiation, reduce the expression of inflammatory factors in HaCaT cells, and inhibit the inflammatory response induced by P. acnes that results in skin inflammation relief and skin barrier improvement. FLG, filaggrin; FOXP3, forkhead box protein 3; GPCRs, G protein-coupled receptors; HaCaT, human immortalized keratinocytes; ICAM-1, intercellular adhesion molecule-1; IFN-γ, interferon-γ; IL-6, interleukin-6; IL-8, interleukin-8; IL-10, interleukin-10; IL-17, interleukin-17; LCFAs, long-chain fatty acids; NHEKs, normal human epidermal keratinocytes; P. acnes, Propionibacterium acnes; S. epidermidis, Staphylococcus epidermidis; SCFAs, short chain fatty acids; TGM1, transglutaminase-1; TLR-2, toll-like receptor-2; TNF-α, tumor necrosis factor-α; Treg, regulatory T-cells; VLCFAs, very long-chain fatty acids.
2.1. SCFA receptors pathway
Short-chain fatty acid receptors are activated upon binding to the ligand and facilitate SCFAs entry into cells (Thiruvengadam et al., 2021). The established SCFAs receptors include G protein-coupled receptor 41 (GPR41) [a.k.a free fatty acid receptor 3 (FFAR3)] (Deng et al., 2022), G protein-coupled receptor 43 (GPR43) [a.k.a. free fatty acid receptor 2 (FFAR2)] (Schlatterer et al., 2021), G protein-coupled receptor 109A (GPR109A) [a.k.a. hydroxycarboxylic acid receptor 2 (HCA2)] (Krejner et al., 2018), Olfr-78 (for murine), OR51E2 (for human) (Ohira et al., 2017), aryl-hydrocarbon receptor (AHR) (Rosser et al., 2020) and peroxisome proliferator-activated receptors γ (PPARγ) (Aguilar et al., 2018; Yao et al., 2022). SCFA-mediated receptors play an important role in regulating the inflammatory response of the host. FFAR2-deficient mice exhibit exacerbated inflammation in mouse models of arthritis, colitis, and asthma, and similar dysregulation in germ-free mice with low or no SCFA expression (Maslowski et al., 2009). These results show that SCFAs interact with GPR43 profoundly affect the inflammatory response (Maslowski et al., 2009). SCFAs act via FFAR2/3 to attenuate the secretion of various inflammatory cytokine and modulate the inflammatory response of human monocytes (Ang et al., 2016). Moreover, SCFAs activate GPR43 and GPR109a to regulate inflammatory response of the host by downregulation of NF-κB signaling pathway (Singh et al., 2014; Cleophas et al., 2016). Additionally, the PPARγ not only competitively inhibits the activation of NF-κB (Mao et al., 2012), but also cooperates with SCFAs to suppress the phosphorylation of NF-κB signaling pathway (Zhang et al., 2022).
Recent studies have shown that SCFA receptors are closely related to the pathogenesis of inflammatory skin diseases. AHR expression significantly increase in the serum and skin lesions of patients with AD (Kim et al., 2014; Beránek et al., 2018; Hu et al., 2020), whereas PPARγ, GPR43, and GPR109a downregulate in psoriasis (Lin et al., 2022). Moreover, a study has indicated that butyrate can increase the expression of GPR43 and GPR109a in psoriasis and exert anti-inflammatory effects (Krejner et al., 2018). The binding of these receptors to SCFAs activates intracellular signaling pathways that regulate cellular responses, immune function, and inflammation (Marinissen and Gutkind, 2001; Trompette et al., 2014; Haase et al., 2018).
Taken together, alterations in the expression of SCFAs-mediated receptors may involve in the pathogenesis of inflammatory skin diseases (Krejner et al., 2018). Existing evidence suggests that SCFAs may play an anti-inflammatory role in inflammatory skin diseases by restoring the expression of SCFAs-mediated receptors, but the specific mechanism is rarely reported. Future studies are needed to gain insight into the anti-inflammatory role of SCFAs in inflammatory skin diseases.
2.2. HDAC inhibition and associated pathway
The dynamic balance between histone acetylases (HATs) and HDACs controls chromatin structure and gene expression (Koh et al., 2016). HDAC inhibitors deacetylate histones in the promoter regions, resulting in the transcriptional activation of the downstream genes. The anti-inflammatory effects of SCFAs have been attributed to their ability to inhibit HDAC activity. For instance, SCFA blocks CXCL10 release through HDAC inhibition, reduce inflammation and maintain immune homeostasis and gut health (Korsten et al., 2022). Furthermore, butyrate promotes FOXP3 expression in naive CD4+ T-cells and induces their differentiation into peripheral-derived Tregs (pTregs) by inhibiting HDACs (Martin-Gallausiaux et al., 2018), while acetate, propionate and butyrate can modulate the immune response of DCs, macrophages and Treg cells by inhibiting HDACs (Thiruvengadam et al., 2021). Imiquimod (IMQ)-induced psoriasis-like skin inflammation model reduces the suppressive activity of Treg, and then upregulates IL-17 and IL-6, and downregulates IL-10 and FOXP3, whereas butyrate can reverse these progressions by inhibiting HDACs (Schwarz et al., 2021) (Figure 1). In LPS-activated neutrophils, application of both butyrate and propionate inhibits NF-κB activity and reduces TNF-α production mainly by inhibiting HDACs (Aoyama et al., 2010). In addition, SCFAs can alleviate systemic inflammation by significantly reducing the production of TNF-α and IL-6 through the downregulation of HDACs mRNAs (Eslick et al., 2022). Propionibacterium acnes in the skin activate toll-like receptor-2 (TLR-2) and promote the secretion of inflammatory factors such as IL-6, IL-8, and TNF-α, thereby inducing skin inflammation. This process may be attenuated by SCFAs via inhibiting HDACs (Wang et al., 2016). Furthermore, Butyrate enhances the expression of filaggrin (FLG) and transglutaminase-1 (TGM1) and promotes normal human epidermal keratinocytes (NHEKs) terminal differentiation to maintain skin homeostasis by inhibiting HDACs (Carrion et al., 2014) (Figure 1).
Overall, SCFAs can reduce the release of inflammatory cytokines and promote the differentiation of epidermal keratinocytes via inhibiting HDACs, thereby playing an anti-inflammatory role in inflammatory skin diseases.
2.3. Metabolic pathway
Short-chain fatty acids can affect cellular metabolism by promoting mitochondrial fatty acid β-oxidation (FAO) (Trompette et al., 2018; Bachem et al., 2019). Skin barrier dysfunction is a common pathological feature of inflammatory skin diseases (Diotallevi et al., 2022; Trompette et al., 2022). Recent studies have shown that SCFAs is able to improve the skin barrier and relieve skin inflammation by altering mitochondrial metabolism and function (Trompette et al., 2022). Butyrate is metabolized by epidermal keratinocytes, which in turn enhances the synthesis of keratinocyte-derived long-chain fatty acids (LCFAs) and very long-chain fatty acids (VLCFAs), a key event in the subsequent generation of ceramides that are critical to skin barrier function. Interestingly, this phenomenon is limited to the skin, since butyrate has little effect on systemic LCFA and VLCFA levels. Furthermore, butyrate can also enhance LCFA uptake (Bachem et al., 2019; Trompette et al., 2022) (Figure 1). Genetic variation in the mitochondrial genome is associated with chronic inflammatory skin disease. Intriguingly, in mice with antibody-induced dermatitis, propionate-treated disease progression pattern is similar to that of mitochondrial gene variant (B6-mtFVB) mice, both effectively reducing the severity of inflammatory skin disease (Schilf et al., 2021).
Thus, the anti-inflammatory effects of butyrate on the skin can be attributed to fueling FAO (directly and indirectly) and altering mitochondrial metabolism, promoting keratinocyte differentiation, and improving the skin barrier.
3. Relationship between SCFAs and inflammatory skin diseases
Many studies have reported the interaction between SCFAs and inflammatory skin diseases. We reviewed the effects of SCFAs on AD, eczema, acne, chronic spontaneous urticaria (CSU), and psoriasis.
3.1. SCFAs in atopic dermatitis
Atopic dermatitis is a chronic inflammatory skin disease that is caused by a number of genetic, environmental, and immunological factors (Williams and Flohr, 2006). Recent studies show that the symptoms of AD are the result of a systemic immune response induced by changes in the gut microecology (Kim et al., 2020). Furthermore, evidence demonstrates that the intestinal levels of SCFAs correlate with the onset of AD. For instance, Patients with AD have low levels of fecal SCFAs, which corresponds to a significant reduction in the abundance of SCFA-producing bacteria (Reddel et al., 2019). Similar trends have been observed in animal models as well (Kim et al., 2019). Moreover, the severity of AD correlates negatively with the abundance of butyrate-producing bacteria (Nylund et al., 2015). A follow-up study of children aged 6–24 months revealed lower levels of butyrate and valerate in infants with transient AD compared to the healthy infants and those with persistent AD (Park et al., 2020). Other studies have shown that infants younger than 3 years with AD have lower amounts of propionate and butyrate in their first year (Ta et al., 2020). Similar patterns have been observed in mixed age groups (Song et al., 2016) (see Table 1). Consistent with the above findings, mice exhibiting AD-like symptoms induced with transdermal injection of 2,4-dinitrochlorobenzene have lower SCFA levels compared to the healthy littermates. Furthermore, the symptoms of AD in these mice are relieved after treatment, and the SCFA levels are normalized (Chun et al., 2021). These findings underscore the role of the SCFAs as signaling molecules of the gut-skin axis.
The development of AD is closely related to immune dysregulation, microbial exposure, disrupted skin barrier and intestinal dysbiosis (Elias and Steinhoff, 2008). An imbalance between the pro-inflammatory CD4+IL17+ T-cells and the anti-inflammatory CD4+FOXP3+ regulatory T-cells (Tregs) in the gut may be a key determinant of early AD development (Kim et al., 2020). Both propionate and butyrate contribute to the accumulation of Tregs in the colon through activation of DCs and T-cells or G protein signaling (Arpaia et al., 2013; Smith et al., 2013). Therefore, restoring the balance between the intestinal T-cell subpopulations by SCFAs supplementation may mitigate the symptoms of AD (Kim et al., 2020). Low levels of SCFAs may also increase intestinal permeability and disrupt its barrier function, eventually triggering AD (Benedetto et al., 2011). SCFAs are partly absorbed by the intestine and the remainder enter the bloodstream, and are distributed to various organs and tissues (Boets et al., 2017; Vonk and Reckman, 2017). Therefore, SCFAs may act directly on skin cells to regulate the barrier function (Schauber et al., 2006, 2008; Sunkara et al., 2012; Carrion et al., 2014; Wei et al., 2017). Keratinocytes exposed to TNF-α and IFN-γ express aberrantly high levels of the pro-inflammatory cytokine IL-6, and the intercellular adhesion molecule-1 (ICAM-1), which in turn recruit monocytes to the epidermis. These inflammatory keratinocytes involve in the pathophysiology of AD. SFCAs markedly reduce IL-6 and ICAM-1 mRNA levels in human keratinocytes (HaCaT cells) stimulated with TNF-α and INF-γ in vitro (Chun et al., 2021) (Figure 1). In addition, butyrate also inhibits the inflammatory phenotype of cultured human keratinocytes by inducing acetylation of histone H3 lysine 9 (AcH3K9) (Meijer et al., 2010; Traisaeng et al., 2019).
The pathogenesis of AD is also related to an imbalance in the skin microecology. The skin of AD patients is more susceptible to the colonization and overgrowth of pathogenic bacteria due to the lower abundance of probiotic bacteria (Leung, 2013), which inhibit the growth of pathogens through metabolites (Iwase et al., 2010; Naik et al., 2012; Ren et al., 2013). For instance, the SCFAs produced during fermentation by beneficial bacteria have an inhibitory effect on community-associated methicillin-resistant Staphylococcus aureus (Kao et al., 2017). S. aureus is abundant in the AD skin, and promotes inflammation by binding to and activating TLR-2, resulting in IL-4-mediated inhibition of IL-10 (Leung, 2013; Kaesler et al., 2014). SCFAs not only improve the local skin microflora but also restrain the migration of immune cells and the production of TNF-α, IL-6, and IL-8 (Wang et al., 2016) (Figure 1).
In summary, SCFAs are closely related to the pathogenesis of AD. The level of SCFAs in AD patients is generally lower than that in healthy controls. Low levels of fecal SCFAs may increase intestinal permeability and induce AD. However, transcutaneously induced AD-like animal models can also reduce intestinal SCFAs levels, but little is known about SCFAs expression in lesion skin. In future studies, it is recommended to add the measurement of SCFAs expression in AD-damaged skin to better illustrate the role of SCFAs in inflammatory skin diseases.
3.2. SCFAs in eczema
Eczema is a common inflammatory skin disease with underlying genetic and environmental causes. Studies show that gut microecology is an important environmental factor in the occurrence of eczema, and SCFA levels can predict the risk of eczema (Kim H. K. et al., 2015). The decrease in SCFA level precedes eczema symptoms (Sandin et al., 2009; Kim H. K. et al., 2015). Lower levels of fecal butyrate and valerate have been observed in 6-month-old infants with eczema, and butyrate levels, in particular, have negative correlation with the development of eczema (Wopereis et al., 2018; Kang M. J. et al., 2021). This is consistent with the inverse correlation between low microbial complexity in early infancy and the likelihood of allergies in later life (Wang et al., 2007; Bisgaard et al., 2011; Ismail et al., 2012; Victora et al., 2016; Roduit et al., 2019; Cheng et al., 2022). Furthermore, most patients with eczema have low levels of SCFAs, and low fecal valerate levels in childhood is related to a higher incidence of eczema in youth (Gio-Batta et al., 2020; Batta et al., 2021). These findings indicate that low levels of SCFAs may increase the susceptibility to eczema. However, research concerning the role of SCFAs in the pathogenesis of eczema is mainly focused on infants and young children, and it is unclear whether these findings can be extrapolated to adult patients as well. More clinical evidence is needed to elucidate the correlation between SCFAs and eczema.
Interleukin-17 (IL-17) is expressed in different subtypes of eczema and can enhance the inflammatory symptoms (Simon et al., 2014). Valerate can inhibit IL-17 release by promoting IL-10 production via the CD4+ T-cells and regulatory B-cells, a process which involves reprogramming lymphocyte metabolism and inhibition of HDAC activity (Yuille et al., 2018; Luu et al., 2019). Butyrate can also diffuse through the intestinal epithelial cells, promote the differentiation of naive T-cells to Tregs, and prevent allergic diseases such as eczema (Kim H. K. et al., 2015). Furthermore, the development of eczema is often associated with impaired skin barrier function (Lee et al., 2021). Keratin (KRT1) and FLG play a crucial role in the maintenance of a healthy skin barrier (Freedberg et al., 2001; Pfisterer et al., 2021). KRT1-deficient mice have a compromised skin barrier, and their skin transcriptome resembles that of eczema skin (Roth et al., 2012). Valerate can improve skin tissue integrity and barrier function by upregulating KRT1 and tight junction proteins (Li et al., 2020; Nguyen et al., 2020). Recent studies reveal that the etiology of eczema is closely related to the genetic loss of FLG, which leads to dryness and impaired skin barrier function (Kalb et al., 2022). Butyrate and propionate can increase the expression of FLG protein by inhibiting the activity of HDAC, and restoring the function and permeability of the epidermal barrier (Kleuskens et al., 2022).
Taken together, low levels of SCFAs may increase susceptibility to eczema. SCFAs are capable of improving the skin barrier and alleviating the skin inflammation of eczema. However, the clinical application of SCFA in eczema needs further investigation.
3.3. SCFAs in acne
Acne is a common inflammatory skin condition caused by the overgrowth of P. acnes (Kong and Segre, 2012; Scanlan et al., 2012; Wu et al., 2021). The probiotic Staphylococcus epidermidis can inhibit bacterial colonization and P. acnes-induced inflammation at the lesions by producing SCFAs (Wang et al., 2016). Propionate and butyrate are ligands of GPR41 (Park et al., 2007), which are upregulated through the SCFAs produced by S. epidermidis. In addition, the latter may alleviate P. acnes-induced inflammation by inhibiting HDAC activity (Wang et al., 2016). The protective function of SCFAs depends on the routes of administration. Subcutaneous injection of SCFAs and P. acnes in mice abrogated the upregulation of inflammatory genes, as opposed to topical application of the SCFAs on the back (Sanford et al., 2016). These results indicate that SCFAs can inhibit the growth of P. acnes, and their anti-inflammatory effects depend on the mode of application. The exact mechanisms underlying the differential effects of SCFAs on the epidermal and subcutaneous tissues are unclear. The same SCFAs may exert different effects in different application environments. How to apply SCFAs safely and effectively against skin inflammation (the dose of SCFAs, the site, and the application environment, etc.) become research highlights.
3.4. SCFAs in chronic spontaneous urticaria
Chronic spontaneous urticaria is a type of chronic inflammatory dermatosis that is predominantly mast cell-driven (Zuberbier et al., 2021; Peng et al., 2022). Alterations in gut metabolites may exacerbate the inflammatory response and immune dysfunction during the pathogenesis of CSU (Wang et al., 2020). In addition, reduced SCFAs accumulation due to an imbalance in the intestinal flora may play an important role in the pathogenesis of CSU (Wang et al., 2020). Clinical studies have shown that the levels of fecal isobutyrate (Wang et al., 2021) and serum butyrate (Wang et al., 2020) are lower in CSU patients compared to that in healthy individuals. This is consistent with the observation that butyrate represses mast cell proliferation, degranulation and cytokine production in mice (Galli et al., 1982; Diakos et al., 2006), and reduces the levels of inflammatory cytokines (Wang et al., 2018). SCFAs bind to receptors (such as GPR43 and PPARγ) that are expressed on mast cells (Sugiyama et al., 2000; Karaki et al., 2006), and inhibit the inflammatory response, mast cell maturation, and even dermatitis (Tachibana et al., 2008; Maslowski et al., 2009; Sina et al., 2009). Thus, SCFA-specific receptors expressed on mast cells are potential therapeutic targets for CSU. A recent study demonstrates that SCFAs suppress the activity of human and murine primary mast cells by inhibiting HDAC independent of GPR41, GPR43, and nuclear PPAR receptors (Folkerts et al., 2020). This suggests that SCFAs inhibit mast cell activation and inflammatory responses through multiple pathways. In addition, SCFAs can inhibit mast cell activation in vitro and in vivo at levels comparable to that in the intestine and serum of human, without affecting cell viability (Cummings et al., 1987; Wong et al., 2006). Therefore, it may be a promising direction to study the pathogenesis and treatment of CSU based on SCFAs.
3.5. SCFAs in psoriasis
Psoriasis is an immune-mediated inflammatory skin disease with complex pathogenesis (Rodriguez et al., 2014; Rendon and Schäkel, 2019). It manifests as chronic inflammation and subsequent epidermal hyperplasia, resulting in silvery scales and thickening of the skin (Rodriguez et al., 2014). Altered intestinal microecology is a pathological factor in psoriatic development (Lu et al., 2021). One study discovers that serum acetate and propionate levels are lower in patients with psoriasis compared to healthy individuals (Khyshiktuev et al., 2008). Furthermore, serum acetate and propionate levels correlate negatively with that of IL-23/IL-27 in an IMQ-induced mouse model of psoriasis, and administration of these SCFAs alleviates the inflammatory symptoms (Lu et al., 2021). Psoriatic progression depends on the activation of the TNF-α/IL-23/IL-17 signaling axis, and the hyperproliferation and aberrant differentiation of epidermal keratinocytes (Takeshita et al., 2017; Furue et al., 2018). IL-23 induces the transformation of Tregs to the T helper type 17 (Th17) cells, whereas IL-17A reduces transforming growth factor (TGF)-β1 production and Foxp3 expression, and inhibits Treg activity (Rodriguez et al., 2014; Stockenhuber et al., 2018; Kanda et al., 2021). Decreased Treg activity in patients with psoriasis is closely related to the level of SCFAs (Kanda et al., 2021), and the beneficial effects of SCFAs are also dependent on the role of Tregs (Schwarz et al., 2021). In addition, the genes involved in SCFA metabolism are significantly downregulated in patients with psoriasis, which may be associated with Treg dysfunction (Ahn et al., 2016). Administration of SCFAs can improve the symptoms of psoriasis by inhibiting HDACs in the Tregs and restoring their activity (Smith et al., 2013; Schwarz et al., 2021). Furthermore, SCFAs can promote the expansion of peripherally derived Tregs (Kaisar et al., 2017; Martin-Gallausiaux et al., 2018; Isobe et al., 2020), although there is a lack of psoriasis-targeted studies.
G protein-coupled receptor 109A and GPR43 are expressed at significantly lower levels in the keratinocytes of psoriasis patients compared to that of healthy individuals, and topical application of butyrate can restore their expression levels (Krejner et al., 2018). In addition, in vitro experiments have shown that butyrate can upregulate filaggrin and TGM1 transcripts in cultured human keratinocytes and promote the formation of cornified envelope (Carrion et al., 2014), which may help improve psoriatic lesions. In fact, topical application of SCFAs reduce both IMQ-induced psoriasis and systemic inflammatory responses in mice (Schwarz et al., 2021). Hence, these studies suggest that SCFAs may be involved in the regulation of psoriasis by restoring Treg activity and promoting the formation of cornified envelope.
4. SCFA-based therapy for inflammatory skin diseases
In recent years, SCFAs have emerged in the field of inflammatory skin diseases as an anti-inflammatory modulator, but it is still in its infancy. Therefore, understanding the application of SCFAs in the treatment of inflammatory skin diseases can pave a path for future clinical practice.
4.1. Topical administration of SCFAs in inflammatory skin diseases
The skin is the first line of defense against biological, physical, and chemical stresses (Coppola et al., 2022). Numerous viruses, bacteria, archaea, fungi, and mites reside on the skin surface which constitute the skin microbiome (Eisenstein, 2020). Skin-resident microbes maintain cutaneous homeostasis and regulate the local inflammatory response (Chen et al., 2018), and any disruption in the skin microbiome can induce an inflammatory reaction (Pessemier et al., 2021). Local application of SCFAs can promote the growth of beneficial microbes, and topical administration of probiotics promotes the release of SCFAs to mitigate skin inflammation (Kim C. H. et al., 2015). In a trial to validate acetate for AD, topical application of apple cider vinegar (0.5% acetate) for 2 weeks did not affect the skin microbiome of healthy subjects and AD patients compared to the water placebo group, and also did not prevent the colonization of S. aureus on the inflamed skin (Luu et al., 2021). However, no significant difference was observed in the diversity of the skin microbiome between the AD patients and healthy subjects at baseline (Luu et al., 2021). Therefore, the inhibitory effect of apple cider vinegar on S. aureus growth may depend on the bacterial strain and the levels of acetate (Fraise et al., 2013). On the other hand, in mice with IMQ-induced skin inflammation, topical injection of acetate led to further exacerbation of skin inflammation (Nadeem et al., 2017). Similarly, topically (2% w/w) and systemically administered (200 mmol/L) acetate was used to enhance psoriasis-like signs in an animal model of IMQ-induced psoriasis (Karamani et al., 2021). Therefore, the net effects of the actions of SCFAs are context-dependent and can be pro- or anti-inflammatory (Schilf et al., 2021). The exact effects of SCFAs may depend on the type of SCFAs, the delivery method, the levels and the health condition of hosts (Xiong et al., 2022). Moreover, fermentation initiators such as sucrose can selectively promote SCFA production by the probiotic S. epidermidis, and improve acne dysbiosis (Wang et al., 2016). Topical application of butyrate not only inhibits allergic contact inflammation in a mouse model (Schwarz et al., 2017), but also alleviates skin inflammation and the expression of inflammatory factors related to psoriasis (Schwarz et al., 2021).
However, most SCFAs have an offensive odor and are therapeutically effective only at certain concentrations (Kao et al., 2017; Traisaeng et al., 2019), which significantly limit their clinical use. SCFA derivatives can obviate these limitations. The butyrate derivative BA-NH-NH-BA consists of two butyrate molecules with a -NH-O-NH- linker. It inhibits HDAC activity at a lower level compared to butyrate, and mitigates S. aureus growth and the inflammatory response in a mouse model (Traisaeng et al., 2019). Taken together, the topical application of SCFAs have broad effects on the skin microbiome, skin barrier, and related inflammatory factors.
4.2. Oral supplementation of SCFAs in inflammatory skin diseases
Gut microbes have the ability to modulate systemic inflammation (Bowe et al., 2014), and imbalances in the gut microbes can manifest as inflammatory skin diseases (O’Neill et al., 2016). The beneficial effects of the gut microbiota on host health are closely associated with SCFAs (Tan et al., 2014). Given the similarities in the gut and skin microbiomes, orally administered SCFAs can potentially exert an anti-inflammatory effect on the skin as well (Schwarz et al., 2017).
Probiotics are living microorganisms that have beneficial effects on host health, and can maintain intestinal homeostasis by restoring SCFA levels (Zanten et al., 2012). Studies increasingly show that skin inflammation is closely related to SCFA production. Oral administration of Bifidobacterium adolescentis in mice with DNFB-induced AD significantly improves AD-like symptoms, such as skin damage and swelling, which correlates with increased levels of propionate and butyrate and decreased levels of isovalerate (Fang et al., 2020). Regular cheese consumption also introduces probiotics into the gastrointestinal tract and increases the production of SCFAs (Ríos-Covián et al., 2016; Kim et al., 2019). In one study, the neuroprotective effects of Lactococcus chungangensis CAU 28-fermented cream cheese and L. chungangensis CAU 28 dry cells are compared in a mouse model of AD. The CAU 28 cream cheese results in better outcomes, which can be attributed to a more abundant supply of SCFAs compared to the bacterial cells. Furthermore, oral administration of CAU 28 cream cheese induces a coordinated immune response involving SCFAs and gut microbiota, and effectively improves symptoms of AD (Kim et al., 2019). Oral supplementation with multistrain probiotics (IRT5) can be the alternative therapeutics for the prevention and treatment of skin allergies. Its mechanism of action is mainly attributed to IRT5-induced propionate which is the key immunomodulatory metabolite for the Treg cells expansion and relieving skin inflammation (Kang H. J. et al., 2021). Moreover, A study has showed that sialyllactose and galactooligosaccharides are capable to promote re-epithelialization and repair epidermal wound, which is related to differential changes in SCFA profiles (Perdijk et al., 2019).
Glycomacropeptides (GMPs) are bioactive peptides extracted from dietary proteins that are beneficial to human health (Artym and Zimecki, 2013). Prophylactic feeding of glycopeptides induces SCFA production, and prevents and reverses AD-like skin lesions in rats. Studies show that the protective effect of GMPs on the skin barrier may be mediated by the direct effects of acetate and butyrate on local skin cells (Jiménez et al., 2020). Taken together, oral administration of SCFAs can alleviate the symptoms of inflammatory skin diseases. More clinical studies are needed to confirm the effectiveness of oral supplementation of SCFAs.
5. Conclusion
The skin anti-inflammatory effects of SCFAs have been studied in rodents experimental models, but the implication of these findings to the human population is still debatable. Restoring the production of SCFAs may relieve the symptoms of skin inflammation by directly inhibiting the inflammatory factors, improving immune homeostasis, and preventing colonization by pathogenic bacteria. Nevertheless, the mechanism of SCFAs toxicities remains largely unknown.
The current understanding of SCFAs-associated anti-inflammatory effects in inflammatory skin diseases is still limited and is a nascent area of research that requires further investigation. Targeted metabolomics of lesioned skin specimens in patients with inflammatory dermatoses may provide new insights into the molecular basis of their action. Several studies focus on the predominant SCFAs including acetate, propionate, and butyrate. Nevertheless, little is known regarding the effects of other SCFAs, which may play a key anti-inflammatory role. Future studies on valerate and isobutyrate may bring new perspectives on the effects of SCFAs on inflammatory skin diseases. Given the unpleasant taste of SCFAs, it is necessary to develop derivatives that are functionally similar and can be incorporated into clinical application. However, the long-term safety and efficacy of SCFA derivatives warrant further investigation. Encouraging evidence supports the effect of SCFAs in the treatment of AD, psoriasis, acne, and urticaria and SCFAs have therapeutic potential against chronic wounds, alopecia, and seborrheic dermatitis as well. Given the close correlation between SCFA expression and skin inflammation occurrence, SCFAs could be used to detect the therapeutic efficacy and to predict the prognosis of inflammatory skin diseases. A deeper understanding of SCFAs in inflammatory skin diseases provides a systematic theoretical basis for studying SCFAs as potential drugs for promoting human skin health.
Author contributions
XX, XH, and JY: conceptualization and supervision. WC, ZZ, LW, HQ, DZ, and PX: resources. XX, XH, JY, ZZ, YXL, and LW: literature review and writing – original draft preparation. XX, XH, RJ, YL, YS, and JL: writing – review and editing. All authors have read and agreed to the published version of the manuscript.
Funding
This study was supported by grants from the National Natural Science Foundation of China (Nos. 82205283 and 82105026) and the China Postdoctoral Science Foundation (Nos. 2022MD723719 and 2021MD693787).
Conflict of interest
The authors declare that the research was conducted in the absence of any commercial or financial relationships that could be construed as a potential conflict of interest.
Publisher’s note
All claims expressed in this article are solely those of the authors and do not necessarily represent those of their affiliated organizations, or those of the publisher, the editors and the reviewers. Any product that may be evaluated in this article, or claim that may be made by its manufacturer, is not guaranteed or endorsed by the publisher.
References
Aguilar, E. C., Silva, J. F. D., Navia-Pelaez, J. M., Leonel, A. J., Lopes, L. G., Menezes-Garcia, Z., et al. (2018). Sodium butyrate modulates adipocyte expansion, adipogenesis, and insulin receptor signaling by upregulation of PPAR-γ in obese apo E knockout mice. Nutrition 47, 75–82. doi: 10.1016/j.nut.2017.10.007
Ahn, R., Gupta, R., Lai, K., Chopra, N., Arron, S. T., and Liao, W. (2016). Network analysis of psoriasis reveals biological pathways and roles for coding and long non-coding RNAs. BMC Genomics 17:841. doi: 10.1186/s12864-016-3188-y
Ang, Z., Er, J. Z., Tan, N. S., Lu, J., Liou, Y.-C., Grosse, J., et al. (2016). Human and mouse monocytes display distinct signalling and cytokine profiles upon stimulation with FFAR2/FFAR3 short-chain fatty acid receptor agonists. Sci. Rep. 6:34145. doi: 10.1038/srep34145
Aoyama, M., Kotani, J., and Usami, M. (2010). Butyrate and propionate induced activated or non-activated neutrophil apoptosis via HDAC inhibitor activity but without activating GPR-41/GPR-43 pathways. Nutrition 26, 653–661. doi: 10.1016/j.nut.2009.07.006
Arpaia, N., Campbell, C., Fan, X., Dikiy, S., Veeken, J. V. D., deRoos, P., et al. (2013). Metabolites produced by commensal bacteria promote peripheral regulatory T-cell generation. Nature 504, 451–455. doi: 10.1038/nature12726
Artym, J., and Zimecki, M. (2013). Milk-derived proteins and peptides in clinical trials. Postepy Hig. Med. Dosw. 67, 800–816. doi: 10.5604/17322693.1061635
Bachem, A., Makhlouf, C., Binger, K. J., Souza, D. P. D., Tull, D., Hochheiser, K., et al. (2019). Microbiota-derived short-chain fatty acids promote the memory potential of antigen-activated CD8(+) T cells. Immunity 51, 285–297. doi: 10.1016/j.immuni.2019.06.002
Batta, M. G., Spetz, K., Barman, M., Bråbäck, L., Norin, E., Björkstén, B., et al. (2021). Low concentration of fecal valeric acid at 1 year of age is linked with eczema and food allergy at 13 years of age: Findings from a Swedish birth cohort. Int. Arch. Allergy Immunol. 138, 398–408. doi: 10.1159/000520149
Benedetto, A. D., Rafaels, N. M., McGirt, L. Y., Ivanov, A. I., Georas, S. N., Cheadle, C., et al. (2011). Tight junction defects in patients with atopic dermatitis. J. Allergy Clin. Immunol. 127, 773–786. doi: 10.1016/j.jaci.2010.10.018
Beránek, M., Fiala, Z., Kremláček, J., Andrýs, C., Krejsek, J., Hamáková, K., et al. (2018). Serum levels of aryl hydrocarbon receptor, cytochromes P450 1A1 and 1B1 in patients with exacerbated psoriasis vulgaris. Folia Biol. 64, 97–102.
Bisgaard, H., Li, N., Bonnelykke, K., Chawes, B. L. K., Skov, T., Paludan-Müller, G., et al. (2011). Reduced diversity of the intestinal microbiota during infancy is associated with increased risk of allergic disease at school age. J. Allergy Clin. Immunol. 128, 646–652.e1-5. doi: 10.1016/j.jaci.2011.04.060
Boets, E., Gomand, S. V., Deroover, L., Preston, T., Vermeulen, K., Preter, V. D., et al. (2017). Systemic availability and metabolism of colonic-derived short-chain fatty acids in healthy subjects: A stable isotope study. J. Physiol. 595, 541–555. doi: 10.1113/JP272613
Bowe, W., Patel, N. B., and Logan, A. C. (2014). Acne vulgaris, probiotics and the gut-brain-skin axis: From anecdote to translational medicine. Benef. Microbes 5, 185–199. doi: 10.3920/BM2012.0060
Canfora, E. E., Jocken, J. W., and Blaak, E. E. (2015). Short-chain fatty acids in control of body weight and insulin sensitivity. Nat. Rev. Endocrinol. 11, 577–591. doi: 10.1038/nrendo.2015.128
Carrion, S. L., Sutter, C. H., and Sutter, T. R. (2014). Combined treatment with sodium butyrate and PD153035 enhances keratinocyte differentiation. Exp. Dermatol. 23, 211–214. doi: 10.1111/exd.12333
Chen, Y. E., Fischbach, M. A., and Belkaid, Y. (2018). Skin microbiota-host interactions. Nature 553, 427–436. doi: 10.1038/nature25177
Cheng, H. Y., Chan, J. C. Y., Yap, G. C., Huang, C.-H., Kioh, D. Y. Q., Tham, E. H., et al. (2022). Evaluation of stool short chain fatty acids profiles in the first year of life with childhood atopy-related outcomes. Front. Allergy 3:873168. doi: 10.3389/falgy.2022.873168
Chun, J., Lee, S. M., Ahn, Y. M., Baek, M.-G., Yi, H., Shin, S., et al. (2021). Modulation of the gut microbiota by sihocheonggan-tang shapes the immune responses of atopic dermatitis. Front. Pharmacol. 12:722730. doi: 10.3389/fphar.2021.722730
Cleophas, M. C. P., Crişan, T. O., Lemmers, H., Toenhake-Dijkstra, H., Fossati, G., Jansen, T. L., et al. (2016). Suppression of monosodium urate crystal-induced cytokine production by butyrate is mediated by the inhibition of class I histone deacetylases. Ann. Rheum. Dis. 75, 593–600. doi: 10.1136/annrheumdis-2014-206258
Coppola, S., Avagliano, C., Sacchi, A., Laneri, S., Calignano, A., Voto, L., et al. (2022). Potential clinical applications of the postbiotic butyrate in human skin diseases. Molecules 27:1849. doi: 10.3390/molecules27061849
Cummings, J. H., Pomare, E. W., Branch, W. J., Naylor, C. P., and Macfarlane, G. T. (1987). Short chain fatty acids in human large intestine, portal, hepatic and venous blood. Gut 28, 1221–1227. doi: 10.1136/gut.28.10.1221
Deng, F., Zhang, L.-Q., Wu, H., Chen, Y., Yu, W.-Q., Han, R.-H., et al. (2022). Propionate alleviates myocardial ischemia-reperfusion injury aggravated by angiotensin II dependent on caveolin-1/ACE2 axis through GPR41. Int. J. Biol. Sci. 18, 858–872. doi: 10.7150/ijbs.67724
Diakos, C., Prieschl, E. E., Säemann, M. D., Böhmig, G. A., Csonga, R., Sobanov, Y., et al. (2006). N-Butyrate inhibits Jun NH(2)-terminal kinase activation and cytokine transcription in mast cells. Biochem. Biophys. Res. Commun. 349, 863–868. doi: 10.1016/j.bbrc.2006.08.117
Diotallevi, F., Campanati, A., Martina, E., Radi, G., Paolinelli, M., Marani, A., et al. (2022). The role of nutrition in immune-mediated, inflammatory skin disease: A narrative review. Nutrients 14:591. doi: 10.3390/nu14030591
Elias, P. M., and Steinhoff, M. (2008). “Outside-to-inside” (and now back to “outside”) pathogenic mechanisms in atopic dermatitis. J. Invest. Dermatol. 128, 1067–1070. doi: 10.1038/jid.2008.88
Eslick, S., Williams, E. J., Berthon, B. S., Wright, T., Karihaloo, C., Gately, M., et al. (2022). Weight loss and short-chain fatty acids reduce systemic inflammation in monocytes and adipose tissue macrophages from obese subjects. Nutrients 14:765. doi: 10.3390/nu14040765
Fang, Z., Li, L., Zhao, J., Zhang, H., Lee, Y.-K., Lu, W., et al. (2020). Bifidobacteria adolescentis regulated immune responses and gut microbial composition to alleviate DNFB-induced atopic dermatitis in mice. Eur. J. Nutr. 59, 3069–3081. doi: 10.1007/s00394-019-02145-8
Folkerts, J., Redegeld, F., Folkerts, G., Blokhuis, B., Berg, M. P. M. V. D., Bruijn, M. J. W. D., et al. (2020). Butyrate inhibits human mast cell activation via epigenetic regulation of FcεRI-mediated signaling. Allergy 75, 1966–1978. doi: 10.1111/all.14254
Fraise, A. P., Wilkinson, M. A. C., Bradley, C. R., Oppenheim, B., and Moiemen, N. (2013). The antibacterial activity and stability of acetic acid. J. Hosp. Infect. 84, 329–331. doi: 10.1016/j.jhin.2013.05.001
Freedberg, I. M., Tomic-Canic, M., Komine, M., and Blumenberg, M. (2001). Keratins and the keratinocyte activation cycle. J. Invest. Dermatol. 116, 633–640. doi: 10.1046/j.1523-1747.2001.01327.x
Furue, K., Ito, T., and Furue, M. (2018). Differential efficacy of biologic treatments targeting the TNF-α/IL-23/IL-17 axis in psoriasis and psoriatic arthritis. Cytokine 111, 182–188. doi: 10.1016/j.cyto.2018.08.025
Galli, S. J., Dvorak, A. M., Marcum, J. A., Ishizaka, T., Nabel, G., Simonian, H. D., et al. (1982). Mast cell clones: A model for the analysis of cellular maturation. J. Cell Biol. 95(2 Pt 1), 435–444. doi: 10.1083/jcb.95.2.435
Gio-Batta, M., Sjöberg, F., Jonsson, K., Barman, M., Lundell, A.-C., Adlerberth, I., et al. (2020). Fecal short chain fatty acids in children living on farms and a link between valeric acid and protection from eczema. Sci. Rep. 10:22449. doi: 10.1038/s41598-020-79737-6
Haase, S., Haghikia, A., Wilck, N., Müller, D. N., and Linker, R. A. (2018). Impacts of microbiome metabolites on immune regulation and autoimmunity. Immunology 154, 230–238. doi: 10.1111/imm.12933
Hee, B. V. D., and Wells, J. M. (2021). Microbial regulation of host physiology by short-chain fatty acids. Trends Microbiol. 29, 700–712. doi: 10.1016/j.tim.2021.02.001
Hu, Y.-Q., Liu, P., Mu, Z.-L., and Zhang, J.-Z. (2020). Aryl hydrocarbon receptor expression in serum, peripheral blood mononuclear cells, and skin lesions of patients with atopic dermatitis and its correlation with disease severity. Chin. Med. J. 133, 148–153. doi: 10.1097/CM9.0000000000000591
Ismail, I. H., Oppedisano, F., Joseph, S. J., Boyle, R. J., Licciardi, P. V., Robins-Browne, R. M., et al. (2012). Reduced gut microbial diversity in early life is associated with later development of eczema but not atopy in high-risk infants. Pediatr. Allergy Immunol. 23, 674–681. doi: 10.1111/j.1399-3038.2012.01328.x
Isobe, J., Maeda, S., Obata, Y., Iizuka, K., Nakamura, Y., Fujimura, Y., et al. (2020). Commensal-bacteria-derived butyrate promotes the T-cell-independent IgA response in the colon. Int. Immunol. 32, 243–258. doi: 10.1093/intimm/dxz078
Iwase, T., Uehara, Y., Shinji, H., Tajima, A., Seo, H., Takada, K., et al. (2010). Staphylococcus epidermidis Esp inhibits Staphylococcus aureus biofilm formation and nasal colonization. Nature 465, 346–349. doi: 10.1038/nature09074
Jiménez, M., Muñoz, F. C., Cervantes-García, D., Cervantes, M. M., Hernández-Mercado, A., Barrón-García, B., et al. (2020). Protective effect of glycomacropeptide on the atopic dermatitis-like dysfunctional skin barrier in rats. J. Med. Food 23, 1216–1224. doi: 10.1089/jmf.2019.0247
Kaesler, S., Volz, T., Skabytska, Y., Köberle, M., Hein, U., Chen, K.-M., et al. (2014). Toll-like receptor 2 ligands promote chronic atopic dermatitis through IL-4–mediated suppression of IL-10. J. Allergy Clin. Immunol. 134, 92–99. doi: 10.1016/j.jaci.2014.02.017
Kaisar, M. M. M., Kaisar, M. M. M., Pelgrom, L. R., Ham, A. J. V. D., Yazdanbakhsh, M., and Everts, B. (2017). Butyrate conditions human dendritic cells to prime type 1 regulatory t cells via both histone deacetylase inhibition and g protein-coupled receptor 109a signaling. Front. Immunol. 8:1429. doi: 10.3389/fimmu.2017.01429
Kalb, B., Marenholz, I., Jeanrenaud, A. C. S. N., Meixner, L., Arnau-Soler, A., Rosillo-Salazar, O. D., et al. (2022). Filaggrin loss-of-function mutations are associated with persistence of egg and milk allergy. J. Allergy Clin. Immunol. 150, 1125–1134. doi: 10.1016/j.jaci.2022.05.018
Kanda, N., Hoashi, T., and Saeki, H. (2021). The defect in regulatory T cells in psoriasis and therapeutic approaches. J. Clin. Med. 10:3880. doi: 10.3390/jcm10173880
Kang, H. J., Kim, G.-C., Lee, C.-G., Park, S., Sharma, G., Verma, R., et al. (2021). Probiotics-derived metabolite ameliorates skin allergy by promoting differentiation of FOXP3(+) regulatory T cells. J. Allergy Clin. Immunol. 147, 1517–1521. doi: 10.1016/j.jaci.2020.11.040
Kang, M. J., Lee, S. Y., Park, Y. M., Kim, B. S., Lee, M. J., Kim, J. H., et al. (2021). Interactions between IL-17 variants and Streptococcus in the gut contribute to the development of atopic dermatitis in infancy. Allergy Asthma Immunol. Res. 13, 404–419. doi: 10.4168/aair.2021.13.3.404
Kao, M. S., Huang, S., Chang, W. L., Hsieh, M. F., Huang, C. J., Gallo, R. L., et al. (2017). Microbiome precision editing: Using PEG as a selective fermentation initiator against methicillin-resistant Staphylococcus aureus. Biotechnol. J. 12, 1–20. doi: 10.1002/biot.201600399
Karaki, S.-I., Mitsui, R., Hayashi, H., Kato, I., Sugiya, H., Iwanaga, T., et al. (2006). Short-chain fatty acid receptor, GPR43, is expressed by enteroendocrine cells and mucosal mast cells in rat intestine. Cell Tissue Res. 324, 353–360. doi: 10.1007/s00441-005-0140-x
Karamani, C., Antoniadou, I. T., Dimou, A., Andreou, E., Kostakis, G., Sideri, A., et al. (2021). Optimization of psoriasis mouse models. J. Pharmacol. Toxicol. Methods 108:107054. doi: 10.1016/j.vascn.2021.107054
Khyshiktuev, B. S., Karavaeva, T. M., and Fal’ko, E. V. (2008). Variability of quantitative changes in short-chain fatty acids in serum and epidermis in psoriasis. Klin. Lab. Diagn. 8:22.
Kim, C. H., Kim, J. Y., and Lee, A. Y. (2015). Therapeutic and immunomodulatory effects of glucosamine in combination with low-dose cyclosporine A in a murine model of imiquimod-induced psoriasis. Eur. J. Pharmacol. 756, 43–51. doi: 10.1016/j.ejphar.2015.03.010
Kim, H. J., Lee, S. H., and Hong, S. J. (2020). Antibiotics-induced dysbiosis of intestinal microbiota aggravates atopic dermatitis in mice by altered short-chain fatty acids. Allergy Asthma Immunol. Res. 12, 137–148. doi: 10.4168/aair.2020.12.1.137
Kim, H. K., Rutten, N. B. M. M., Vaart, I. B.-V. D., Niers, L. E. M., Choi, Y. H., Rijkers, G. T., et al. (2015). Probiotic supplementation influences faecal short chain fatty acids in infants at high risk for eczema. Benef. Microbes 6, 783–790. doi: 10.3920/BM2015.0056
Kim, H. O., Kim, J. H., Chung, B. Y., Choi, M. G., and Park, C. W. (2014). Increased expression of the aryl hydrocarbon receptor in patients with chronic inflammatory skin diseases. Exp. Dermatol. 23, 278–281. doi: 10.1111/exd.12350
Kim, J.-H., Kim, K., and Kim, W. (2019). Cream cheese-derived Lactococcus chungangensis CAU 28 modulates the gut microbiota and alleviates atopic dermatitis in BALB/c Mice. Sci. Rep. 9:446. doi: 10.1038/s41598-018-36864-5
Kleuskens, M. T. A., Haasnoot, M. L., Herpers, B. M., Ampting, M. T. J. V., Bredenoord, A. J., Garssen, J., et al. (2022). Butyrate and propionate restore interleukin 13-compromised esophageal epithelial barrier function. Allergy 77, 1510–1521. doi: 10.1111/all.15069
Koh, A., Vadder, F. D., Kovatcheva-Datchary, P., and Bäckhed, F. (2016). From dietary fiber to host physiology: Short-chain fatty acids as key bacterial metabolites. Cell 165, 1332–1345. doi: 10.1016/j.cell.2016.05.041
Kong, H. H., and Segre, J. A. (2012). Skin microbiome: Looking back to move forward. J. Invest. Dermatol. 132(3 Pt 2), 933–939. doi: 10.1038/jid.2011.417
Korsten, S. G. P. J., Peracic, L., Groeningen, L. M. B. V., Diks, M. A. P., Vromans, H., Garssen, J., et al. (2022). Butyrate prevents induction of CXCL10 and non-canonical IRF9 expression by activated human intestinal epithelial cells via HDAC inhibition. Int. J. Mol. Sci. 23:3980. doi: 10.3390/ijms23073980
Krejner, A., Bruhs, A., Mrowietz, U., Wehkamp, U., Schwarz, T., and Schwarz, A. (2018). Decreased expression of G-protein-coupled receptors GPR43 and GPR109a in psoriatic skin can be restored by topical application of sodium butyrate. Arch. Dermatol. Res. 310, 751–758. doi: 10.1007/s00403-018-1865-1
Lee, Y. I., Lee, S. G., Kim, J., Choi, S., Jung, I., and Lee, J. H. (2021). Proteoglycan combined with hyaluronic acid and hydrolyzed collagen restores the skin barrier in mild atopic dermatitis and dry, eczema-prone skin: A pilot study. Int. J. Mol. Sci. 22:10189. doi: 10.3390/ijms221910189
Leung, D. Y. M. (2013). New insights into atopic dermatitis: Role of skin barrier and immune dysregulation. Allergol. Int. 62, 151–161. doi: 10.2332/allergolint.13-RAI-0564
Li, Y., Dong, J., Xiao, H., Zhang, S., Wang, B., Cui, M., et al. (2020). Gut commensal derived-valeric acid protects against radiation injuries. Gut Microbes 11, 789–806. doi: 10.1080/19490976.2019.1709387
Lin, X., Meng, X., Song, Z., and Lin, J. (2022). Peroxisome proliferator-activator receptor γ and psoriasis, molecular and cellular biochemistry. Mol. Cell Biochem. 477, 1905–1920. doi: 10.1007/s11010-022-04417-0
Lu, W., Deng, Y., Fang, Z., Zhai, Q., Cui, S., Zhao, J., et al. (2021). Potential role of probiotics in ameliorating psoriasis by modulating gut microbiota in imiquimod-induced psoriasis-like mice. Nutrients 13:2010. doi: 10.3390/nu13062010
Luu, L. A., Flowers, R. H., Gao, Y., Wu, M., Gasperino, S., Kellams, A. L., et al. (2021). Apple cider vinegar soaks do not alter the skin bacterial microbiome in atopic dermatitis. PLoS One 16:e0252272. doi: 10.1371/journal.pone.0252272
Luu, M., Pautz, S., Kohl, V., Singh, R., Romero, R., Lucas, S., et al. (2019). The short-chain fatty acid pentanoate suppresses autoimmunity by modulating the metabolic-epigenetic crosstalk in lymphocytes. Nat. Commun. 10:760. doi: 10.1038/s41467-019-08711-2
Mao, J. W., Tang, H. Y., and Wang, Y. D. (2012). Influence of rosiglitazone on the expression of PPARγ, NF-κB, and TNF-α in rat model of ulcerative colitis. Gastroenterol. Res. Pract. 2012:845672. doi: 10.1155/2012/845672
Marinissen, M. J., and Gutkind, J. S. (2001). G-protein-coupled receptors and signaling networks: Emerging paradigms. Trends Pharmacol. Sci. 22, 368–376. doi: 10.1016/s0165-6147(00)01678-3
Martin-Gallausiaux, C., Béguet-Crespel, F., Marinelli, L., Jamet, A., Ledue, F., Blottière, H. M., et al. (2018). Butyrate produced by gut commensal bacteria activates TGF-beta1 expression through the transcription factor SP1 in human intestinal epithelial cells. Sci. Rep. 8:9742. doi: 10.1038/s41598-018-28048-y
Maslowski, K. M., Vieira, A. T., Ng, A., Kranich, J., Sierro, F., Yu, D., et al. (2009). Regulation of inflammatory responses by gut microbiota and chemoattractant receptor GPR43. Nature 461, 1282–1286. doi: 10.1038/nature08530
Meijer, K., Vos, P. D., and Priebe, M. G. (2010). Butyrate and other short-chain fatty acids as modulators of immunity: What relevance for health? Curr. Opin. Clin. Nutr. Metab. Care 13, 715–721. doi: 10.1097/MCO.0b013e32833eebe5
Nadeem, A., Ahmad, S. F., Al-Harbi, N. O., El-Sherbeeny, A. M., Al-Harbi, M. M., and Almukhlafi, T. S. (2017). GPR43 activation enhances psoriasis-like inflammation through epidermal upregulation of IL-6 and dual oxidase 2 signaling in a murine model. Cell. Signal. 33, 59–68. doi: 10.1016/j.cellsig.2017.02.014
Naik, S., Bouladoux, N., Wilhelm, C., Molloy, M. J., Salcedo, R., Kastenmuller, W., et al. (2012). Compartmentalized control of skin immunity by resident commensals. Science 337, 1115–1119. doi: 10.1126/science.1225152
Narla, S., and Silverberg, J. I. (2020). Multimorbidity and mortality risk in hospitalized adults with chronic inflammatory skin disease in the United States. Arch. Dermatol. Res. 312, 507–512. doi: 10.1007/s00403-020-02043-8
Nguyen, T. D., Hållenius, F. F., Lin, X., Nyman, M., and Prykhodko, O. (2020). Monobutyrin and monovalerin affect brain short-chain fatty acid profiles and tight-junction protein expression in ApoE-knockout rats fed high-fat diets. Nutrients 12:1202. doi: 10.3390/nu12041202
Nylund, L., Nermes, M., Isolauri, E., Salminen, S., Vos, W. M. D., and Satokari, R. (2015). Severity of atopic disease inversely correlates with intestinal microbiota diversity and butyrate-producing bacteria. Allergy 70, 241–244. doi: 10.1111/all.12549
Ohira, H., Tsutsui, W., and Fujioka, Y. (2017). Are short chain fatty acids in gut microbiota defensive players for inflammation and atherosclerosis? J. Atheroscler. Thromb. 24, 660–672. doi: 10.5551/jat.RV17006
O’Neill, C. A., Monteleone, G., McLaughlin, J. T., and Paus, R. (2016). The gut-skin axis in health and disease: A paradigm with therapeutic implications. Bioessays 38, 1167–1176. doi: 10.1002/bies.201600008
Park, J. S., Lee, E. J., Lee, J. C., Kim, W. K., and Kim, H. S. (2007). Anti-inflammatory effects of short chain fatty acids in IFN-gamma-stimulated RAW 264.7 murine macrophage cells: Involvement of NF-kappaB and ERK signaling pathways. Int. Immunopharmacol. 7, 70–77. doi: 10.1016/j.intimp.2006.08.015
Park, Y. M., Lee, S. Y., Kang, M. J., Kim, B. S., Lee, M. J., Jung, S. S., et al. (2020). Imbalance of Gut Streptococcus, Clostridium, and Akkermansia determines the natural course of atopic dermatitis in infant. Allergy Asthma Immunol. Res. 12, 322–337. doi: 10.4168/aair.2020.12.2.322
Peng, S., Zhang, T., Zhang, S., Tang, Q., Yan, Y., and Feng, H. (2022). Integrated bioinformatics and validation reveal IL1B and Its related molecules as potential biomarkers in chronic spontaneous urticaria. Front. Immunol. 13:850993. doi: 10.3389/fimmu.2022.850993
Perdijk, O., Baarlen, P. V., Fernandez-Gutierrez, M. M., Brink, E. V. D., Schuren, F. H. J., Brugman, S., et al. (2019). Sialyllactose and galactooligosaccharides promote epithelial barrier functioning and distinctly modulate microbiota composition and short chain fatty acid production in vitro. Front. Immunol. 10:94. doi: 10.3389/fimmu.2019.00094
Pessemier, B. D., Grine, L., Debaere, M., Maes, A., Paetzold, B., and Callewaert, C. (2021). Gut-skin axis: Current knowledge of the interrelationship between microbial dysbiosis and skin conditions. Microorganisms 9:353. doi: 10.3390/microorganisms9020353
Pfisterer, K., Shaw, L. E., Symmank, D., and Weninger, W. (2021). The extracellular matrix in skin inflammation and infection. Front. Cell Dev. Biol. 9:682414. doi: 10.3389/fcell.2021.682414
Rauf, A., Khalil, A. A., Rahman, U.-U., Khalid, A., Naz, S., Shariati, M. A., et al. (2022). Recent advances in the therapeutic application of short-chain fatty acids (SCFAs): An updated review. Crit. Rev. Food Sci. Nutr. 62, 6034–6054. doi: 10.1080/10408398.2021.1895064
Reddel, S., Chierico, F. D., Quagliariello, A., Giancristoforo, S., Vernocchi, P., Russo, A., et al. (2019). Gut microbiota profile in children affected by atopic dermatitis and evaluation of intestinal persistence of a probiotic mixture. Sci. Rep. 9:4996. doi: 10.1038/s41598-019-41149-6
Ren, T., Glatt, D. U., Nguyen, T. N., Allen, E. K., Early, S. V., Sale, M., et al. (2013). 16S rRNA survey revealed complex bacterial communities and evidence of bacterial interference on human adenoids. Environ. Microbiol. 15, 535–547. doi: 10.1111/1462-2920.12000
Rendon, A., and Schäkel, K. (2019). Psoriasis pathogenesis and treatment. Int. J. Mol. Sci. 20:1475. doi: 10.3390/ijms20061475
Ríos-Covián, D., Ruas-Madiedo, P., Margolles, A., Gueimonde, M., Reyes-Gavilán, C. G. D. L., and Salazar, N. (2016). Intestinal short chain fatty acids and their link with diet and human health. Front. Microbiol. 7:185. doi: 10.3389/fmicb.2016.00185
Rodriguez, R. S., Pauli, M. L., Neuhaus, I. M., Yu, S. S., Arron, S. T., Harris, H. W., et al. (2014). Memory regulatory T cells reside in human skin. J. Clin. Invest. 124, 1027–1036. doi: 10.1172/JCI72932
Roduit, C., Frei, R., Ferstl, R., Loeliger, S., Westermann, P., Rhyner, C., et al. (2019). High levels of butyrate and propionate in early life are associated with protection against atopy. Allergy 74, 799–809. doi: 10.1111/all.13660
Rosser, E. C., Piper, C. J. M., Matei, D. E., Blair, P. A., Rendeiro, A. F., Orford, M., et al. (2020). Microbiota-derived metabolites suppress arthritis by amplifying aryl-hydrocarbon receptor activation in regulatory B cells. Cell Metab. 31, 837–851. doi: 10.1016/j.cmet.2020.03.003
Roth, W., Kumar, V., Beer, H.-D., Richter, M., Wohlenberg, C., Reuter, U., et al. (2012). Keratin 1 maintains skin integrity and participates in an inflammatory network in skin through interleukin-18. J. Cell Sci. 125(Pt 22), 5269–5279. doi: 10.1242/jcs.116574
Sandin, A., Bråbäck, L., Norin, E., and Björkstén, B. (2009). Faecal short chain fatty acid pattern and allergy in early childhood. Acta Paediatr. 98, 823–827. doi: 10.1111/j.1651-2227.2008.01215.x
Sanford, J. A., Zhang, L.-J., Williams, M. R., Gangoiti, J. A., Huang, C.-M., and Gallo, R. L. (2016). Inhibition of HDAC8 and HDAC9 by microbial short-chain fatty acids breaks immune tolerance of the epidermis to TLR ligands. Sci. Immunol. 1:eaah4609. doi: 10.1126/sciimmunol.aah4609
Scanlan, P. D., Buckling, A., Kong, W., Wild, Y., Lynch, S. V., and Harrison, F. (2012). Gut dysbiosis in cystic fibrosis. J. Cyst. Fibros. 11, 454–455. doi: 10.1016/j.jcf.2012.03.007
Schauber, J., Dorschner, R. A., Yamasaki, K., Brouha, B., and Gallo, R. L. (2006). Control of the innate epithelial antimicrobial response is cell-type specific and dependent on relevant microenvironmental stimuli. Immunology 118, 509–519. doi: 10.1111/j.1365-2567.2006.02399.x
Schauber, J., Oda, Y., Büchau, A. S., Yun, Q.-C., Steinmeyer, A., Zügel, U., et al. (2008). Histone acetylation in keratinocytes enables control of the expression of cathelicidin and CD14 by 1,25-dihydroxyvitamin D3. J. Invest. Dermatol. 128, 816–824. doi: 10.1038/sj.jid.5701102
Schilf, P., Künstner, A., Olbrich, M., Waschina, S., Fuchs, B., Galuska, C. E., et al. (2021). A mitochondrial polymorphism alters immune cell metabolism and protects mice from skin inflammation. Int. J. Mol. Sci. 22:1006. doi: 10.3390/ijms22031006
Schlatterer, K., Peschel, A., and Kretschmer, D. (2021). Short-chain fatty acid and FFAR2 activation - A new option for treating infections? Front. Cell. Infect. Microbiol. 11:785833. doi: 10.3389/fcimb.2021.785833
Schwarz, A., Bruhs, A., and Schwarz, T. (2017). The short-chain fatty acid sodium butyrate functions as a regulator of the skin immune system. J. Invest. Dermatol. 137, 855–864. doi: 10.1016/j.jid.2016.11.014
Schwarz, A., Philippsen, R., and Schwarz, T. (2021). Induction of regulatory T cells and correction of cytokine disbalance by short-chain fatty acids: Implications for psoriasis therapy. J. Invest. Dermatol. 141, 95–104. doi: 10.1016/j.jid.2020.04.031
Simon, D., Aeberhard, C., Erdemoglu, Y., and Simon, H.-U. (2014). Th17 cells and tissue remodeling in atopic and contact dermatitis. Allergy 69, 125–131. doi: 10.1111/all.12351
Sina, C., Gavrilova, O., Förster, M., Till, A., Derer, S., Hildebrand, F., et al. (2009). G protein-coupled receptor 43 is essential for neutrophil recruitment during intestinal inflammation. J. Immunol. 183, 7514–7522. doi: 10.4049/jimmunol.0900063
Singh, N., Gurav, A., Sivaprakasam, S., Brady, E., Padia, R., Shi, H., et al. (2014). Activation of Gpr109a, receptor for niacin and the commensal metabolite butyrate, suppresses colonic inflammation and carcinogenesis. Immunity 40, 128–139. doi: 10.1016/j.immuni.2013.12.007
Smith, P. M., Howitt, M. R., Panikov, N., Michaud, M., Gallini, C. A., Bohlooly-Y, M., et al. (2013). The microbial metabolites. short-chain fatty acids, regulate colonic treg cell homeostasis. Science 341, 569–573. doi: 10.1126/science.1241165
Song, H., Yoo, Y., Hwang, J., Na, Y.-C., and Kim, H. S. (2016). Faecalibacterium prausnitzii subspecies–level dysbiosis in the human gut microbiome underlying atopic dermatitis. J. Allergy Clin. Immunol. 137, 852–860. doi: 10.1016/j.jaci.2015.08.021
Stockenhuber, K., Hegazy, A. N., West, N. R., Ilott, N. E., Stockenhuber, A., Bullers, S. J., et al. (2018). Foxp3(+) T reg cells control psoriasiform inflammation by restraining an IFN-I-driven CD8(+) T cell response. J. Exp. Med. 215, 1987–1998. doi: 10.1084/jem.20172094
Sugiyama, H., Nonaka, T., Kishimoto, T., Komoriya, K., Tsuji, K., and Nakahata, T. (2000). Peroxisome proliferator-activated receptors are expressed in mouse bone marrow-derived mast cells. Jpn. J. Pharmacol. 82, 259–262. doi: 10.1016/s0014-5793(00)01169-8
Sunkara, L. T., Jiang, W., and Zhang, G. (2012). Modulation of antimicrobial host defense peptide gene expression by free fatty acids. PLoS One 7:e49558. doi: 10.1371/journal.pone.0049558
Ta, L. D. H., Chan, J. C. Y., Yap, G. C., Purbojati, R. W., Drautz-Moses, D. I., Koh, Y. M., et al. (2020). A compromised developmental trajectory of the infant gut microbiome and metabolome in atopic eczema. Gut Microbes 12, 1–22. doi: 10.1080/19490976.2020.1801964
Tachibana, M., Wada, K., Katayama, K., Kamisaki, Y., Maeyama, K., Kadowaki, T., et al. (2008). Activation of peroxisome proliferator-activated receptor gamma suppresses mast cell maturation involved in allergic diseases. Allergy 63, 1136–1147. doi: 10.1111/j.1398-9995.2008.01677.x
Takeshita, J., Grewal, S., Langan, S. M., Mehta, N. N., Ogdie, A., Voorhees, A. S. V., et al. (2017). Psoriasis and comorbid diseases epidemiology. J. Am. Acad. Dermatol. 76, 377–390. doi: 10.1016/j.jaad.2016.07.064
Tan, J., McKenzie, C., Potamitis, M., Thorburn, A. N., Mackay, C. R., and Macia, L. (2014). The role of short-chain fatty acids in health and disease. Adv. Immunol. 121, 91–119. doi: 10.1016/B978-0-12-800100-4.00003-9
Thiruvengadam, M., Subramanian, U., Venkidasamy, B., Thirupathi, P., Samynathan, R., Shariati, M. A., et al. (2021). Emerging role of nutritional short-chain fatty acids (SCFAs) against cancer via modulation of hematopoiesis. Crit. Rev. Food Sci. Nutr. 1–18. doi: 10.1080/10408398.2021.1954874
Traisaeng, S., Herr, D. R., Kao, H.-J., Chuang, T.-H., and Huang, C.-M. (2019). A derivative of butyric acid, the fermentation metabolite of Staphylococcus epidermidis, inhibits the growth of a Staphylococcus aureus strain isolated from atopic dermatitis patients. Toxins 11:311. doi: 10.3390/toxins11060311
Trompette, A., Gollwitzer, E. S., Pattaroni, C., Lopez-Mejia, I. C., Riva, E., Pernot, J., et al. (2018). Dietary fiber confers protection against Flu by shaping Ly6c(-) patrolling monocyte hematopoiesis and CD8(+) T cell metabolism. Immunity 48, 992.e8–1005.e8. doi: 10.1016/j.immuni.2018.04.022
Trompette, A., Gollwitzer, E. S., Yadava, K., Sichelstiel, A. K., Sprenger, N., Ngom-Bru, C., et al. (2014). Gut microbiota metabolism of dietary fiber influences allergic airway disease and hematopoiesis. Nat. Med. 20, 159–166. doi: 10.1038/nm.3444
Trompette, A., Pernot, J., Perdijk, O., Alqahtani, R. A. A., Domingo, J. S., Camacho-Muñoz, D., et al. (2022). Gut-derived short-chain fatty acids modulate skin barrier integrity by promoting keratinocyte metabolism and differentiation. Mucosal Immunol. 15, 908–926. doi: 10.1038/s41385-022-00524-9
Victora, C. G., Bahl, R., Barros, A. J. D., França, G. V. A., Horton, S., Krasevec, J., et al. (2016). Breastfeeding in the 21st century: Epidemiology, mechanisms, and lifelong effect. Lancet 387, 475–490. doi: 10.1016/s0140-6736(15)01024-7
Vonk, R. J., and Reckman, G. (2017). Progress in the biology and analysis of short chain fatty acids. J. Physiol. 595, 419–420. doi: 10.1113/JP273260
Wang, C. C., Wu, H., Lin, F. H., Gong, R., Xie, F., Peng, Y., et al. (2018). Sodium butyrate enhances intestinal integrity, inhibits mast cell activation, inflammatory mediator production and JNK signaling pathway in weaned pigs. Innate Immun. 24, 40–46. doi: 10.1177/1753425917741970
Wang, D., Guo, S., He, H., Gong, L., and Cui, H. (2020). Gut microbiome and serum metabolome analyses identify unsaturated fatty acids and butanoate metabolism induced by gut microbiota in patients with chronic spontaneous urticaria. Front. Cell. Infect. Microbiol. 10:24. doi: 10.3389/fcimb.2020.00024
Wang, M., Karlsson, C., Olsson, C., Adlerberth, I., Wold, A. E., Strachan, D. P., et al. (2007). Reduced diversity in the early fecal microbiota of infants with atopic eczema. J. Allergy Clin. Immunol. 121, 129–134. doi: 10.1016/j.jaci.2007.09.011
Wang, X., Yi, W., He, L., Luo, S., Wang, J., Jiang, L., et al. (2021). Abnormalities in gut microbiota and metabolism in patients with chronic spontaneous urticaria. Front. Immunol. 12:691304. doi: 10.3389/fimmu.2021.691304
Wang, Y., Kao, M.-S., Yu, J., Huang, S., Marito, S., Gallo, R. L., et al. (2016). A precision microbiome approach using sucrose for selective augmentation of Staphylococcus epidermidis fermentation against Propionibacterium acnes. Int. J. Mol. Sci. 17:1870. doi: 10.3390/ijms17111870
Wei, Z., Xiao, C., Guo, C., Zhang, X., Wang, Y., Wang, J., et al. (2017). Sodium acetate inhibits Staphylococcus aureus internalization into bovine mammary epithelial cells by inhibiting NF-κB activation. Microb. Pathog. 107, 116–121. doi: 10.1016/j.micpath.2017.03.030
Williams, H., and Flohr, C. (2006). How epidemiology has challenged 3 prevailing concepts about atopic dermatitis. J. Allergy Clin. Immunol. 118, 209–213. doi: 10.1016/j.jaci.2006.04.043
Wong, J. M. W., Souza, R. D., Kendall, C. W. C., Emam, A., and Jenkins, D. J. A. (2006). Colonic health: Fermentation and short chain fatty acids. J. Clin. Gastroenterol. 40, 235–243. doi: 10.1097/00004836-200603000-00015
Wopereis, H., Sim, K., Shaw, A., Warner, J. O., Knol, J., and Kroll, J. S. (2018). Intestinal microbiota in infants at high risk for allergy: Effects of prebiotics and role in eczema development. J. Allergy Clin. Immunol. 141, 1334–1342. doi: 10.1016/j.jaci.2017.05.054
Wu, J., Guo, R., Chai, J., Xiong, W., Tian, M., Lu, W., et al. (2021). The protective effects of Cath-MH with anti-Propionibacterium acnes and anti-inflammation functions on acne vulgaris. Front. Pharmacol. 12:788358. doi: 10.3389/fphar.2021.788358
Xiong, R.-G., Zhou, D.-D., Wu, S.-X., Huang, S.-Y., Saimaiti, A., Yang, Z.-J., et al. (2022). Health benefits and side effects of short-chain fatty acids. Foods 11:2863. doi: 10.3390/foods11182863
Yao, Y., Cai, X., Fei, W., Ye, Y., Zhao, M., and Zheng, C. (2022). The role of short-chain fatty acids in immunity, inflammation and metabolism. Crit. Rev. Food Sci. Nutr. 62, 1–12. doi: 10.1080/10408398.2020.1854675
Yuille, S., Reichardt, N., Panda, S., Dunbar, H., and Mulder, I. E. (2018). Human gut bacteria as potent class I histone deacetylase inhibitors in vitro through production of butyric acid and valeric acid. PLoS One 13:1073. doi: 10.1371/journal.pone.0201073
Zanten, G. C. V., Knudsen, A., Röytiö, H., Forssten, S., Lawther, M., Blennow, A., et al. (2012). The effect of selected synbiotics on microbial composition and short-chain fatty acid production in a model system of the human colon. PLoS One 7:e47212. doi: 10.1371/journal.pone.0047212
Zhang, Z., Yang, P., and Zhao, J. (2022). Ferulic acid mediates prebiotic responses of cereal-derived arabinoxylans on host health. Anim. Nutr. 9, 31–38. doi: 10.1016/j.aninu.2021.08.004
Keywords: short-chain fatty acid (SCFA), inflammatory skin disease, histone deacetylase (HDAC), atopic dermatitis, urticaria, psoriasis, eczema, acne
Citation: Xiao X, Hu X, Yao J, Cao W, Zou Z, Wang L, Qin H, Zhong D, Li YX, Xue P, Jin R, Li Y, Shi Y and Li J (2023) The role of short-chain fatty acids in inflammatory skin diseases. Front. Microbiol. 13:1083432. doi: 10.3389/fmicb.2022.1083432
Received: 29 October 2022; Accepted: 28 December 2022;
Published: 02 February 2023.
Edited by:
Sabbir Khan, The University of Texas MD Anderson Cancer Center, United StatesReviewed by:
Pratibha Sharma, The University of Texas MD Anderson Cancer Center, United StatesPriti Gupta, The University of Texas MD Anderson Cancer Center, United States
Copyright © 2023 Xiao, Hu, Yao, Cao, Zou, Wang, Qin, Zhong, Li, Xue, Jin, Li, Shi and Li. This is an open-access article distributed under the terms of the Creative Commons Attribution License (CC BY). The use, distribution or reproduction in other forums is permitted, provided the original author(s) and the copyright owner(s) are credited and that the original publication in this journal is cited, in accordance with accepted academic practice. No use, distribution or reproduction is permitted which does not comply with these terms.
*Correspondence: Yunzhou Shi, shiyunzhou@cdutcm.edu.cn; Juan Li,
785939016@qq.com
†These authors have contributed equally to this work and share first authorship