- 1Faculty of Veterinary Medicine, Institute of Hygiene and Infectious Diseases of Animals, Justus Liebig University Giessen, Giessen, Germany
- 2NG1 Microbial Genomics, Robert Koch Institute, Berlin, Germany
Introduction: The global emergence of plasmid-mediated colistin resistance is threatening the efficacy of colistin as one of the last treatment options against multi-drug resistant Gram-negative bacteria. To date, ten mcr-genes (mcr-1 to mcr-10) were reported. While mcr-1 has disseminated globally, the occurrence of mcr-2 was reported scarcely.
Methods and results: We determined the occurrence of mcr-1 and mcr-2 genes among Escherichia coli isolates from swine and performed detailed genomic characterization of mcr-2-positive strains. In the years 2010-2017, 7,614 porcine E. coli isolates were obtained from fecal swine samples in Europe and isolates carrying at least one of the virulence associated genes predicting Shiga toxin producing E. coli (STEC), enterotoxigenic E. coli (ETEC) or enteropathogenic E. coli (EPEC) were stored. 793 (10.4%) of these isolates carried the mcr-1 gene. Of 1,477 additional E. coli isolates obtained from sheep blood agar containing 4 mg/L colistin between 2018 and 2020, 36 (2.4%) isolates were mcr-1-positive. In contrast to mcr-1, the mcr-2 gene occurred at a very low frequency (0.13%) among the overall 9,091 isolates. Most mcr-2-positive isolates originated from Belgium (n = 9), one from Spain and two from Germany. They were obtained from six different farms and revealed multilocus sequence types ST10, ST29, ST93, ST100, ST3057 and ST5786. While the originally described mcr-2.1 was predominant, we also detected a new mcr-2 variant in two isolates from Belgium, which was termed mcr-2.8. MCR-2 isolates were mostly classified as ETEC or ETEC-like, while one isolate from Spain represented an atypical enteropathogenic E. coli (aEPEC; eae+). The ST29-aEPEC isolate carried mcr-2 on the chromosome. Another eight isolates carried their mcr-2 gene on IncX4 plasmids that resembled the pKP37-BE MCR-2 plasmid originally described in Belgium in 2015. Three ST100 E. coli isolates from a single farm in Belgium carried the mcr-2.1 gene on a 47-kb self-transmissible IncP type plasmid of a new IncP-1 clade.
Discussion: This is the first report of mcr-2 genes in E. coli isolates from Germany. The detection of a new mcr-2 allele and a novel plasmid backbone suggests the presence of so far undetected mcr-2 variants and mobilizable vehicles.
Introduction
Antimicrobial resistance against colistin has emerged worldwide and poses a serious challenge to the treatment of diseases caused by multidrug resistant Gram-negative bacteria. The value of colistin as a last resort antimicrobial is compromised by the occurrence of mobile colistin resistance (mcr) genes (Schwarz and Johnson, 2016). After the first description of plasmid-encoded mcr-1 from Escherichia coli and Klebsiella pneumoniae isolated from patients, food, and animals in China in 2015 (Liu et al., 2016), nine additional mcr genes (mcr-2 to mcr-10) and their products were described in different Gram-negative bacterial species (Xavier et al., 2016; AbuOun et al., 2017; Borowiak et al., 2017; Carattoli et al., 2017; Yin et al., 2017; Wang et al., 2018; Yang et al., 2018; Carroll et al., 2019; Wang et al., 2020a).
Since the first report of plasmid-borne mcr-1 gene, MCR-1-producing Enterobacterales, mostly Escherichia coli, have been described to occur with different frequencies in livestock, companion animals, wildlife, food and humans across the globe (Ewers et al., 2016; Falgenhauer et al., 2016; Irrgang et al., 2016; Schwarz and Johnson, 2016; Zhang et al., 2016; Unger et al., 2017; Nang et al., 2019). Much less is known about the occurrence of the other mcr genes, particularly about mcr-2 in Europe. MCR-2 is a member of the MCR-family of bacterial phosphoethanolamine transferases and shares 80.6% amino acid identity with MCR-1. The mcr-2 gene was discovered in some E. coli isolates from diarrheic pigs and calves in Belgium (Xavier et al., 2016). It was located on an IncX4 incompatibility-type plasmid (pKP37-BE) of 35,104 bp in size, and it was harbored by mobile insertion element ISEc69 which belongs to the IS1595 insertion sequence family (Xavier et al., 2016). This plasmid was identified in two porcine ST10 E. coli isolates and in one bovine ST167 isolate in the original publication.
A similar plasmid has, to the best of our knowledge, only been reported in two further studies. Among 105 colistin-resistant Salmonella isolates collected from 2012 to 2015 in the national surveillance program in Belgium, Garcia-Graells and co-authors identified a mcr-2-carrying plasmid in a Salmonella Derby strain isolated from a pork carcass in 2012 that was almost identical to pKP37-BE (Garcia-Graells et al., 2018). Timmermans et al. (2021) detected mcr-2 on a pKP37-like IncX4 plasmid in a colistin-resistant mcr-2-positive E. coli strain from a fattening pig, also from Belgium, in 2016 (Timmermans et al., 2021). Apart from the findings in Belgium, the mcr-2 gene was only scarcely detected in other European countries, such as Spain, Great Britain and Italy (Dobrzanska et al., 2020; Miguela-Villoldo et al., 2020). In contrast, various studies from non-European countries, predominantly from Asia, confirmed the occurrence of mcr-2 in Gram-negative bacteria not only from animal (Dutta et al., 2018; Zhang et al., 2018b; Ahmed et al., 2019; Rhouma et al., 2019; Zhang et al., 2019; Islam et al., 2020; Javed et al., 2020; Cilia et al., 2021; Ketkhao et al., 2021) but also from human sources (Zhang et al., 2018a; Ahmed et al., 2019; Mitra et al., 2020; Ara et al., 2021; Ejaz et al., 2021; Imtiaz et al., 2021; Stosic et al., 2021).
Soon after the discovery of mcr-2, Poirel et al. (2017) identified a novel allele of mcr-2 on the chromosome of a Moraxella pluranimalium strain isolated from a pig in Spain. Based on molecular data, they proposed that this gene, termed mcr-2.2, was likely the progenitor of the mcr-2 gene identified in Belgium, thereafter designated as mcr-2.1. As of 2nd July 2021, seven mcr-2 alleles (mcr-2.1 to mcr-2.7) are available in the Bacterial Antimicrobial Resistance Reference Gene Database.1
In veterinary medicine, colistin has been widely used for the control of neonatal and post-weaning diarrhea in pigs, mainly caused by enterotoxigenic Escherichia coli (ETEC) (Luppi et al., 2016; Rhouma et al., 2017). In our microbiological diagnostic laboratory, we receive a large number of samples from pigs for E. coli pathotyping and have created an extensive collection of putative pathogenic E. coli from pigs in Germany and other European countries in the last decade. In this study, we aimed to examine these E. coli isolates for the presence of mcr-1 and mcr-2 genes and to perform a detailed characterization of mcr-2-positive strains with respect to clonal lineage, mcr-2 gene variant and location, plasmid types and pathotype of the bacterial host.
Materials and methods
Bacterial isolates
Escherichia coli isolates (n = 9,091) had been obtained from feces or mucosal swabs (rectum or small intestine) collected from pigs during routine microbiological diagnostics at our institute from 2010 through 2020. Samples were collected mainly in Germany (n = 7,155) and in 17 other European countries including the Netherlands (n = 888), Poland (n = 349), Denmark (n = 145), Switzerland (n = 141), Belgium (n = 131), Austria (n = 87), Hungary (n = 56), Italy (n = 42), Spain (n = 28), Portugal (n = 28), and seven other countries (n = 41) (Table 1). The majority of samples had been collected from piglets suffering from post weaning diarrhea or edema disease.
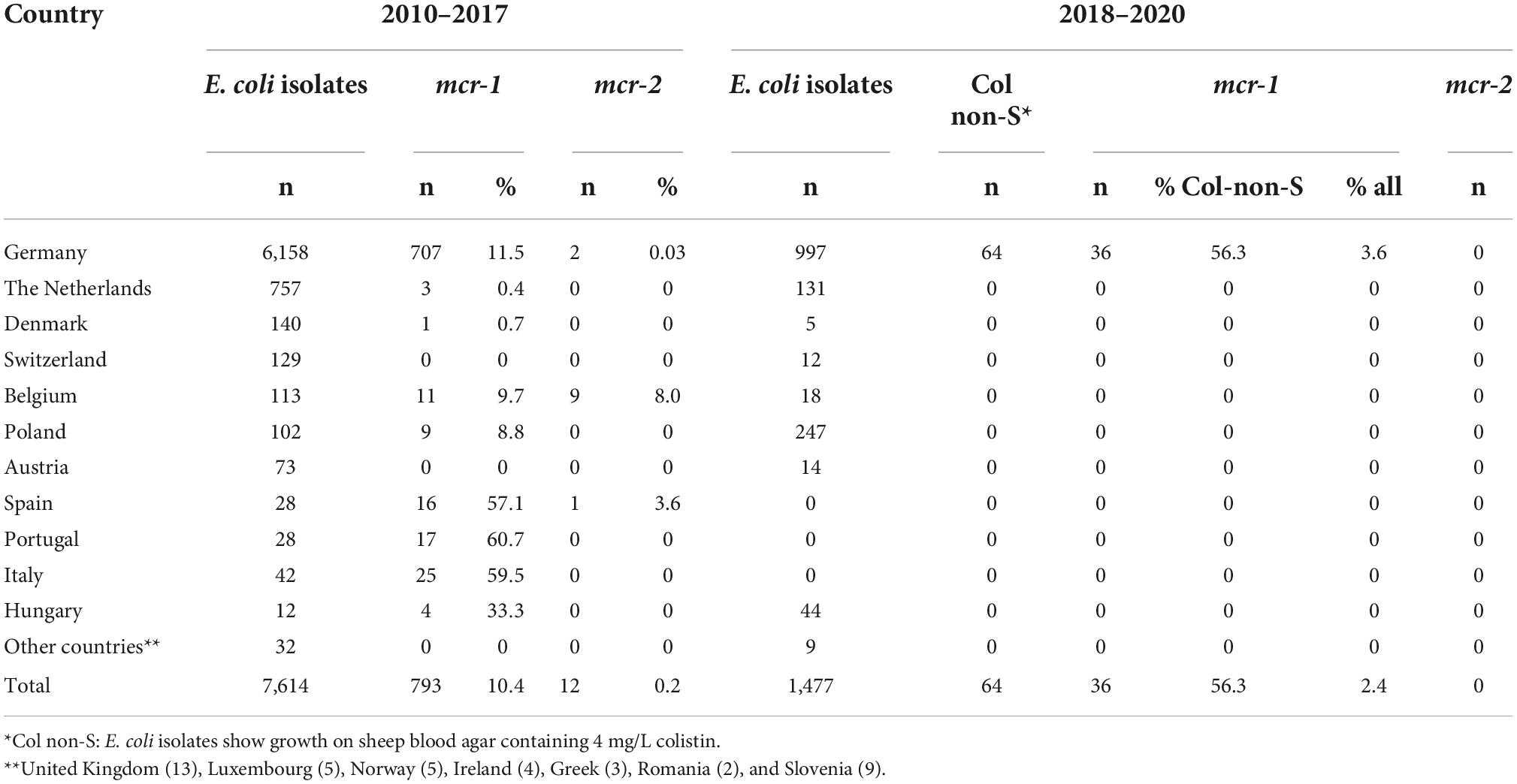
Table 1. Frequency of mcr-1 and mcr-2 in E. coli isolates collected from pigs in Germany and other European countries (2010 – 2020).
Porcine E. coli isolates were screened for mcr-1 and mcr-2 following two different approaches. In a first approach, we included 7,614 E. coli isolates that were archived in our institute’s strain collection from 2010 to 2017. Only those isolates had been stored that proved positive by a modified multiplex polymerase chain reaction (PCR) for the gene of at least one of the following virulence factors: adhesive fimbriae F4, F5, F6, F18 and F41; intimin; heat-labile/stable E. coli enterotoxins LT-I, ST-I, ST-II; Shiga toxins of type Stx2 (Casey and Bosworth, 2009). These factors are related with enterotoxigenic E. coli (ETEC), enteropathogenic E. coli (EPEC), and shiga toxin producing E. coli (STEC). Cultivation of samples was performed on non-selective media, namely sheep blood agar without antibiotics. Basically, one isolate per pig was stored. The maximum number of samples per farm was limited to 6 pigs per submission. In case isolates from the same pig revealed different virulence gene profiles, one representative isolate of each profile was stored.
In a second approach, we screened 1,477 E. coli isolates. The isolates were obtained from fecal and mucosal samples from swine that were sent for routine PCR-based pathotyping to our diagnostic laboratory from May 2018 to December 2020. They were predominantly from Germany (n = 997) and from eight other European countries (Table 1). The isolates were streaked on sheep blood agar containing 4 mg/L colistin and were incubated at 37°C overnight. All isolates that showed growth, irrespective of the presence or absence of virulence-associated genes (VAGs), were stored in a glycerin stock at –80°C until further use.
Polymerase chain reaction screening for mcr-1 and mcr-2 genes and clonal grouping of mcr-2-positive isolates by pulsed-field gel electrophoresis
Escherichia coli isolates collected from 2010 to 2017 were separately cultured overnight in lysogeny broth. Broth cultures of 10 E. coli isolates at a time were pooled and screened for mcr-1 and mcr-2 using recently published primers and protocols (Liu et al., 2016; Xavier et al., 2016). In case pooled material gave a positive PCR result, single isolates were separately tested by the same PCRs. If pooled material was tested negative, all respective isolates were regarded as negative. E. coli isolated from colistin selective media were individually tested for mcr-1 and mcr-2 as described before. Prior to whole genome sequencing mcr-2-positive E. coli isolates were submitted to macrorestriction analysis (XbaI) to determine their clonal identity according to a previously published protocol (Ewers et al., 2014). XbaI-generated PFGE profiles were compared using BioNumerics software (Version 6.6, Applied Maths, Belgium) and cluster analysis of Dice similarity indices based on UPGMA.
Whole genome sequencing
DNA for whole genome sequencing was extracted from E. coli isolates using the DNA Blood & Tissue Kit according to the manufacturer‘s instruction (Qiagen, Hilden, Germany), followed by library preparation, using Nextera XT library (Illumina, San Diego, USA). DNA was sequenced using Illumina HiSeq 1500 with multiplexing of 70 samples per flow cell using 250 bp paired end reads with a coverage >90 x. Raw reads were adapter-trimmed by Flexbar v.3.0.3 (Resource Identification Portal RRID:SCR_013001), corrected using BayesHammer and assembled de novo using SPAdes v3.12.1 (RRID:SCR_000131). Assembled draft genomes were annotated using Prodigal (Prodigal, RRID: SCR_011936).
Antimicrobial susceptibility testing and resistance gene screening
Minimum inhibitory concentrations (MICs) of colistin were determined by the broth microdilution method according to EUCAST guidelines.2 The isolates were further evaluated against 17 other antimicrobial agents by using the VITEK2 compact system (AST-GN38, AST-N248; bioMérieux, Nürtingen, Germany). Results were interpreted according to EUCAST break point tables (EUCAST, 2020) for tigecycline and polymyxin B and according to CLSI guidelines (CLSI, 2018) for the remaining antimicrobial agents.
The web-based tool ResFinder 4.1,3 hosted at the Center for Genomic Epidemiology (CGE), was used to identify resistance genes and chromosomal mutations related to β-lactam (ampC promoter mutation), fluoroquinolone (mutations in gyrA, gyrB, parA, and parC), and colistin resistance (pmrAB) based on WGS data (Zankari et al., 2012). Resistance gene screening was carried out by BLASTn (90% identity and 90% query coverage) analysis against homologous genes present in the Comprehensive Antibiotic Resistance Database.4
Genomic location of mcr-2 and transconjugation assays
The genomic location of the mcr-2 gene was verified by S1 nuclease digestion of genomic DNA followed by electrophoretic separation and Southern hybridization. A digoxigenin-labeled DNA probe (“DIG luminescent detection Kit”, Boehringer Mannheim GmbH, Mannheim) targeting a 567-bp PCR fragment specific for the mcr-2 gene using the aforementioned primers (Xavier et al., 2016) was used. In addition, we performed an in silico search of whole genome sequences with mlplasmids v. 1.0.0 (Arredondo-Alonso et al., 2018). To test whether the colistin resistance determinant was transferable, conjugation was performed by the broth filter mating method at 37°C using plasmid-free sodium azide resistant E. coli K12-J53 (J53 AziR) as recipient. Prior to the conjugation assays, all mcr-2-positive isolates were tested for their susceptibility to sodium azide. Transconjugants were selected on Endo agar plates containing 100 mg/L sodium azide and 2 mg/L colistin sulfate or containing 100 mg/L sodium azide and 4 mg/L colistin sulfate (Sigma-Aldrich, Germany, Karlsruhe, Germany). To confirm successful plasmid transfer, antimicrobial susceptibility testing of transconjugants, a PCR targeting the mcr-2 gene and plasmid profiling was performed as described above.
Plasmid analysis
To characterize the identified mcr-2 gene harboring contigs, all contigs were aligned using Geneious (v. 8.1.9, Biomatters Ltd., Auckland, New Zealand) (Geneious, RRID:SCR_010519) to the respective gene. Contigs containing mcr-2 were aligned to publicly available plasmid sequences from GenBank (GenBank, RRID:SCR_002760) using BLASTn analysis (RRID:SCR_004870). All contigs of a respective isolate were then aligned to the reference plasmid that revealed highest similarity. In addition, contigs were mapped to the selected reference plasmids using the Geneious Map to Reference. In silico constructed plasmids were further examined for mobile genetic elements, using ISfinder (RRID:SCR_003020). To display circular comparisons between plasmids we used the blast ring image generator software BRIG Version 0.95 (RRID:SCR_007802). Plasmid maps were generated using GenomeVx (Conant and Wolfe, 2008).
PlasmidFinder 2.15 was applied to determine plasmid replicons. In addition, the assignment of MCR-2 plasmids to an incompatibility group was carried out by phylogenetic analysis of the sequences of the replication initiator protein TrfA and of plasmid “backbone” gene proteins TrfB, KlcA, KleA, KorC, TraD, TraE, TraF, TraG, TraJ, TraK, TraL, TrbA, TrbB, TrbC, TrbD, TrbF, TrgB, TrbI, TrbJ, and TrbK (Smith et al., 1993; Luppi et al., 2016).
Phylogenetic comparison of mcr-2 genes
To compare our mcr-2 genes with currently known mcr-2 genes, we screened the literature (PubMed, RRID:SCR_004846) and DNA sequence data repositories (GenBank, RRID:SCR_002760; EMBL, RRID:SCR_004473). Nucleotide and deduced amino acid sequences of mcr-2 genes were downloaded from GenBank and aligned with Mafft v7.407 (RRID:SCR_011811). The resulting alignment was used to calculate a maximum likelihood-based phylogeny with RAxML v.8.2.10 (RRID:SCR_006086) with 100 bootstraps under the assumption of the gtr-gamma DNA substitution model (gamma BLOSUM62 protein substitution model).
Detection of serotype and virulence-associated genes
The sero(geno)types of E. coli strains were determined using the web-based serotyping tool SerotypeFinder 2.06 (Joensen et al., 2015). Screening for VAGs was carried out by NCBI BLASTn (RRID:SCR_004870) analysis against homologous genes present in an in-house database of 800 VAGs, gene variants or genomic islands from a subset of the VirulenceFinder database and in-house created and manually curated VAG reference sequences. We searched for genes that were previously linked with porcine intestinal pathogenic E. coli pathovars but also for VAGs of extraintestinal pathovars, such as uropathogenic E. coli (UPEC). Genes belonged to different categories (adhesin, toxin, iron uptake system, capsule synthesis, colicins, effector proteins, and secretion systems). Coverage length and sequence identity thresholds were 80% and 90%, respectively. E. coli isolates were further analyzed for the presence of the fimH gene and the allele type by aligning to a FimH database using FimTyper 1.0.7
Phylogenetic grouping, multilocus sequence typing and core genome analysis
Phylogenetic groups were determined by using the ClermonTyping method and its associated web-interface ClermonTyper, that allows a given strain sequence to be assigned to E. albertii, E. fergusonii, Escherichia clades I-V, E. coli sensu stricto as well as to the seven main E. coli phylogroups A, B1, C, E, D, F, and B2 (Clermont et al., 2013; Beghain et al., 2018). MLST 2.08 (Larsen et al., 2012) was applied to identify the multilocus sequence type (RRID:SCR_010245) of E. coli isolates following the Achtman scheme,9 which represents a 7-gene-scheme including genes adk, fumC, gyrB, icd, mdh, purA, and recA. Phylogenetic and population genetic relationships were determined by applying a gene-by-gene approach on the dataset to generate a core genome alignment and subsequently a phylogenetic tree. The core genome alignment was assembled by a gene-wise alignment (Mafft v7.407: RRID:SCR_011811) of 1,366 core genes that were present in at least 99% of the strains (sequence similarity min. 70%, sequence coverage min. 90%) and were concatenated afterward. The resulting alignment was used to infer a phylogeny with 100 bootstrap replicates using RAxML v.8.2.10 (RAxML, RRID:SCR_006086) with a General Time Reversible model and gamma correction for among site rate variation.
Results
Number and origin of Escherichia coli carrying mcr-1 and mcr-2 genes
Among the first collection of 7,614 E. coli isolates obtained from 2010 to 2017, 793 (10.4%) isolates were positive for mcr-1 (Table 1). The rates of mcr-1-positive strains with respect to countries differed significantly, ranging from low (0–0.7%) (e.g., Switzerland, Austria, Netherlands, and Denmark) to moderate (8.8%–11.5%; Poland, Belgium and Germany) and high (33.3%–60.7%; Hungary, Spain, Italy and Portugal).
However, due to the biased sample material and the low number of E. coli isolates available from several countries, the data cannot be regarded as true prevalence data for most of the countries.
In Germany, where the highest number of samples was available, the rate of mcr-1 among porcine E. coli isolates increased from 2010 (8.9%) to 2015 (15.2%), dropped slightly in the year 2016 (11.4%) and revealed the lowest percentage in 2017 (6.3%) (Figure 1, Supplementary Table 1).
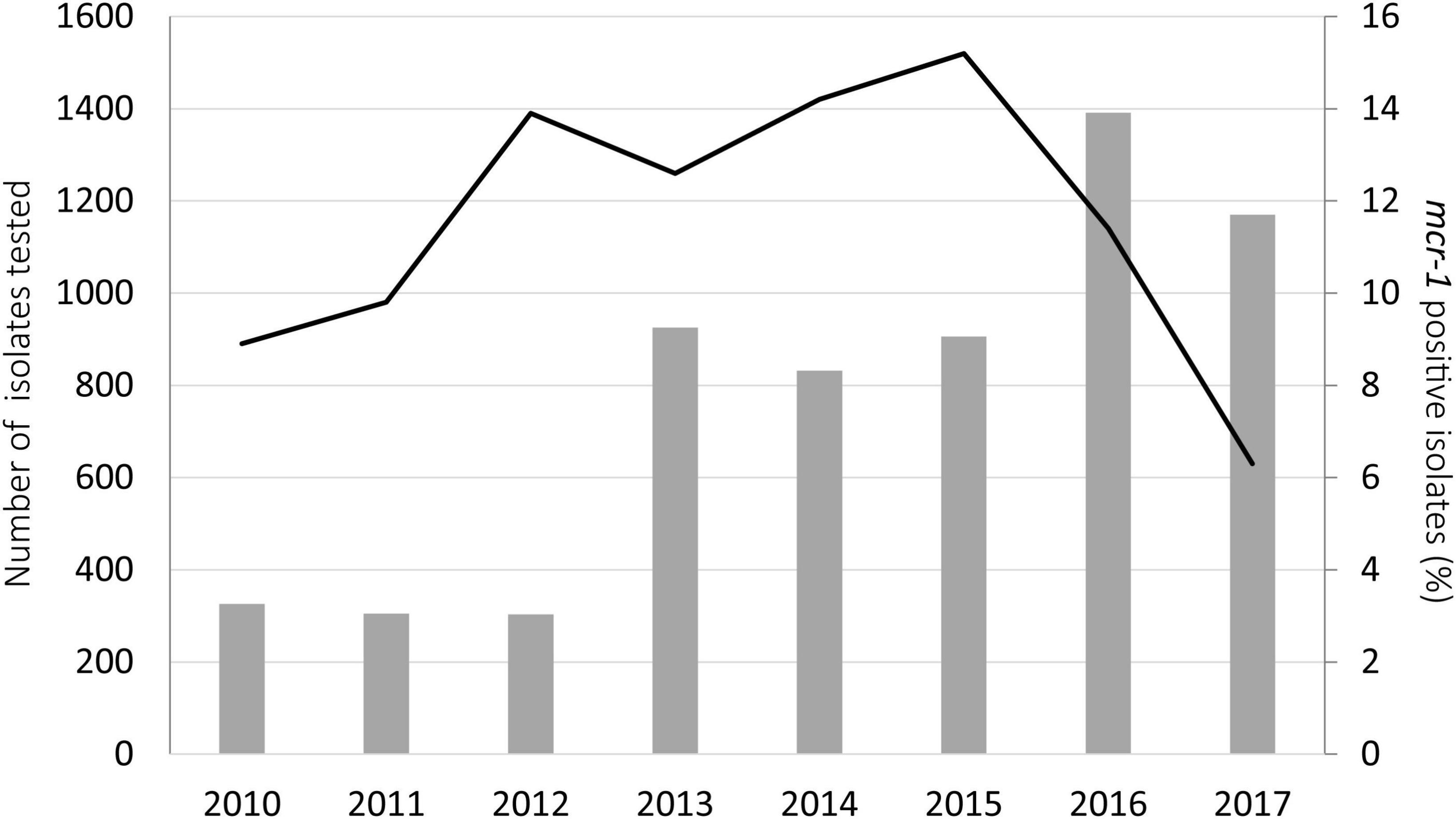
Figure 1. Annual rates of mcr-1 (line) among 6,158 E. coli isolates (annual numbers as columns) collected from pigs in Germany from 2010 to 2017.
In contrast to mcr-1, the mcr-2 gene was only rarely detected in the first collection of 7,614 isolates. Twelve isolates were positive for mcr-2, representing 0.2% of the total number of isolates tested (Table 1). The majority of mcr-2-positive isolates was obtained from Belgium (9 isolates/within country rate of 8.0%), followed by Germany (2/0.03%) and Spain (1/3.6%). The Belgian isolates were all isolated in 2015 and originated from three different swine farms (farms I-III) (Table 2). The Spanish isolate dates back to October 2013 (farm IV) and the two strains from Germany were obtained in August and December 2014 on two different farms (farms V and VI).
Among the 1,477 E. coli isolates that we pre-screened on sheep blood agar containing 4 mg/L colistin, 64 isolates (4.3%) showed growth and were regarded as colistin non-susceptible (Col-non-S) isolates. Based on PCR analysis, none of the 64 Col-non-S isolates possessed mcr-2, while 36 isolates, i.e., 56.3% of Col-non-S strains and 2.4% from all 1,477 isolates tested, harbored the mcr-1 gene (Table 1). All mcr-1-positive isolates were from Germany, accounting to 3.6% among 997 isolates tested in the years 2018-2020.
Clonal relatedness of mcr-2-positive Escherichia coli
PFGE analyses separated the 12 mcr-2-positive E. coli isolates into eight different groups (A–H). Macrorestriction patterns revealed the clonality of three isolates from two pigs on farm I (pulsotype A) and of two isolates from two pigs from farm II (pulsotype B), respectively (data not shown). On farm II, mcr-2-positive isolates displayed different PFGE patterns (pulsotypes B and C), suggesting that they were not clonally related to those on farm I and III. Five different pulsotypes (A–E) were identified among the nine isolates from three farms in Belgium (n = 9). Isolates from Spain and Germany differed from Belgian isolates in their PFGE profiles (pulsotypes F–H). Among the total of eight PFGE profiles, seven different multilocus sequence types were determined (Table 2). We found ST100 in four isolates from Belgium collected on two different farms (farm I and III). On farm III, two ST10 isolates (IHIT323204 and IHIT32397) were additionally present. Sequence type ST5786, which is a single locus variant of ST10 (IHIT32302 and IHIT32399), and ST3057 (IHIT32303) were identified in isolates obtained from farm II. The isolate from Spain belonged to ST29 and the two German isolates were assigned to ST93 and a single locus variant of ST93 (ST11875) that differed in the gyrB allele (ST93: gyrB-6; ST11875: gyrB-405). With the exception of the ST29 isolate (phylogroup B1) and the ST3057 isolate (clade I), all mcr-2-positive isolates belonged to phylogenetic group A (Table 2).
Antimicrobial susceptibility and antimicrobial resistance genes
Minimum inhibitory concentration (MIC) data for the 12 mcr-2-positive E. coli isolates are provided in Supplementary Table 2. All but one repeatedly tested isolate (IHIT32302, MIC 0.5 mg/L) were resistant to colistin (MICs 4 mg/L–16 mg/L). Additional resistances were determined for ampicillin (100%), piperacillin (100%), tetracycline (91.7%), trimethoprim/sulfamethoxazole (91.7%), and chloramphenicol (33.3%) (Table 3). Only one isolate (ST100-IHIT32305, Belgium) was resistant to the aminoglycosides gentamicin and tobramycin, which correlated with the presence of aminoglycoside acetyltransferase gene aac(3)-IV in this strain (Table 3). None of the isolates showed resistance to fluoroquinolone, third-generation cephalosporins or to carbapenems. The phenotypic antimicrobial resistance profile almost always corresponded with the presence of resistance genes in the respective isolates (Table 3).
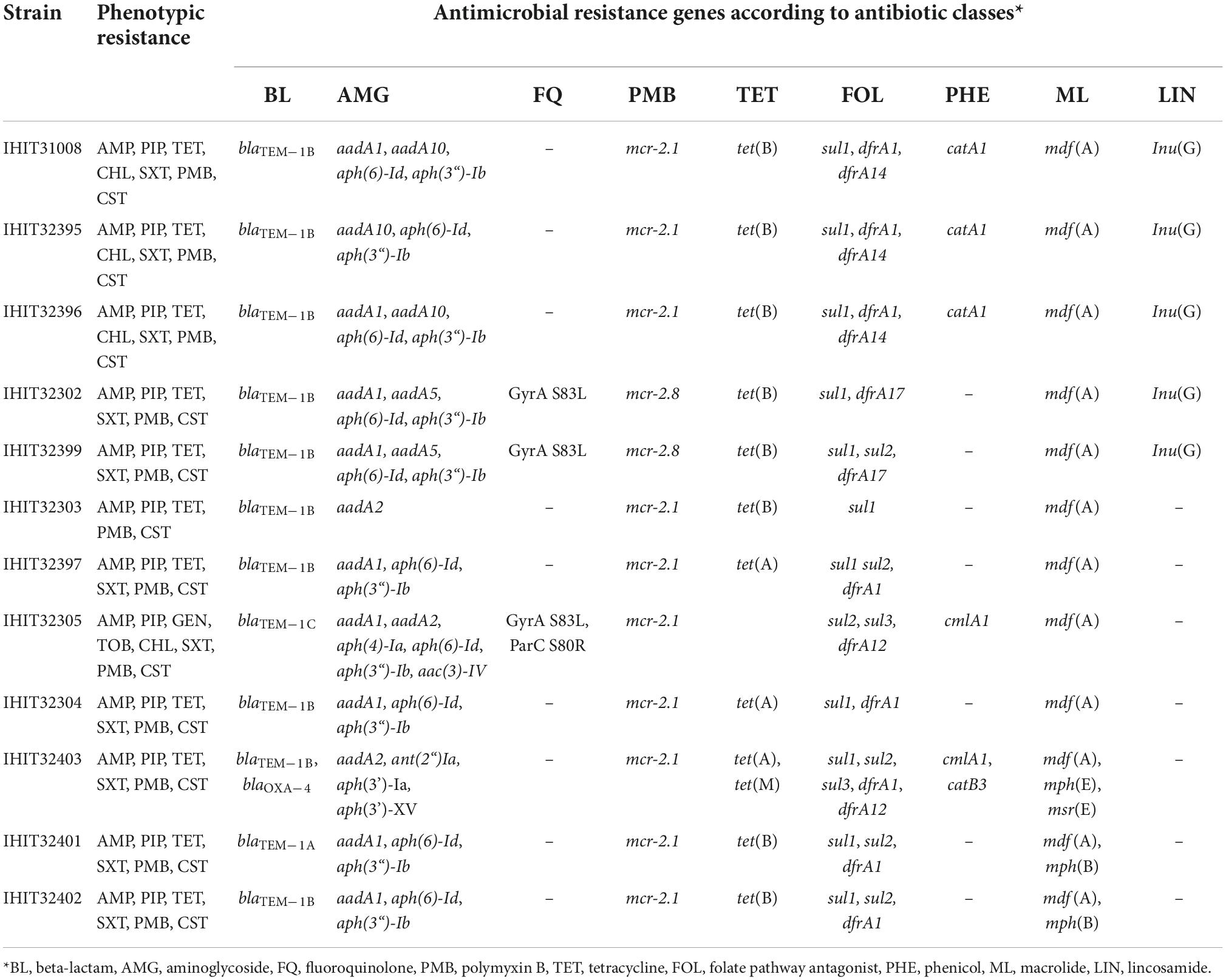
Table 3. Antimicrobial susceptibility and resistance genes of mcr-2-positive E. coli isolates from pigs.
Virulence genes and serotypes of mcr-2-positive Escherichia coli isolates
Based on the presence of VAGs obtained from WGS data we conducted a pathotype prediction of the mcr-2-positive E. coli isolates. Enterotoxigenic E. coli (ETEC) are characterized by the presence of genes for heat labile (eltA, eltB) and/or stable (esta, estb) E. coli enterotoxins and genes for adhesive fimbriae (F4, fae; F5, fan; F6, fas; F17, f17; F18, fed). With a percentage of 83.3%, most of our isolates were classified as ETEC or ETEC-like (Table 2), as they harbored estb and/or eltA, often in combination with genes for F4 or F18 fimbriae, respectively (Supplementary Table 3). The ST29 E. coli isolate IHIT32403 represented an atypical enteropathogenic E. coli (aEPEC) as it harbored intimin gene eae and lacked bundle-forming pili adhesin genes bfpA-L, which together with eae are indicative for typical EPEC. Only one isolate, namely ST100-IHIT31008, could not be assigned to a recognized E. coli intestinal or extraintestinal pathotype. Apart from enterotoxin and ETEC fimbriae genes, all isolates possessed a number of additional VAGs related to adhesion, tissue damage, iron acquisition, secretion and serum resistance. In particular aEPEC strain IHIT32403 from Spain revealed a number of additional genes that are known to play a significant role in the pathogenesis of EPEC-induced disease, including adhesin genes cfa, efa1, lpf, and paa as well as type III secretion system and effector protein genes (esp, sep, lifA, nle, tccP, and tir) (Supplementary Table 3). Sero(geno)typing revealed the presence of serotypes O138:H10, O182:H4, O35:H6, O149:H10, and O132:H25/Hnt among ETEC and ETEC-like isolates and of serotype O45:H11 in the aEPEC isolate (Table 2).
Identification of a novel mcr-2 variant
At the time of writing, seven valid mcr-2 genes (mcr-2.1 to mcr-2.7) have been recognized. Gene mcr-2.1 was discovered in E. coli isolates from pigs and cattle in Belgium (Xavier et al., 2016) and mcr-2.2 was detected in Moraxella pluranimalium strain 248-01T (CCUG 54913) isolated from a pig in Spain in 2001 (Poirel et al., 2017). Another five genes, available in GenBank, were confirmed as reference sequences for mcr-2 genes after curation of records by the NCBI staff (see Figure 2 for NCBI reference numbers). Nucleotide sequences obtained for the mcr-2 genes (1,617 bp) of our E. coli isolates revealed that all but two isolates harbored mcr-2.1 (Table 2). Two isolates from Belgium carried a novel variant of mcr-2, which had highest similarity (99.94%) to mcr-2.1 from E. coli KP37-BE that harbored the originally described mcr-2 gene (NG_051171). The novel mcr-2 allele differed from mcr-2.1 by encoding a single amino acid variation at position 390 (Met→Thr). Overall, mcr-2.1 to mcr-2.7 and our novel mcr-2 allele had a similarity ranging from 94.19% (mcr-2.4 vs. novel mcr-2 from this study) to 99.94%. A comparison of the deduced amino acid sequences (538 aa) of mcr-2 genes revealed similarity levels of 96.47% (MCR-2.4 vs. MCR-2.5) to 99.81% (MCR-2.1 vs. novel MCR-2 from this study). A maximum likelihood-based phylogeny of mcr-2 gene alleles and MCR-2 proteins is provided in Figure 2.
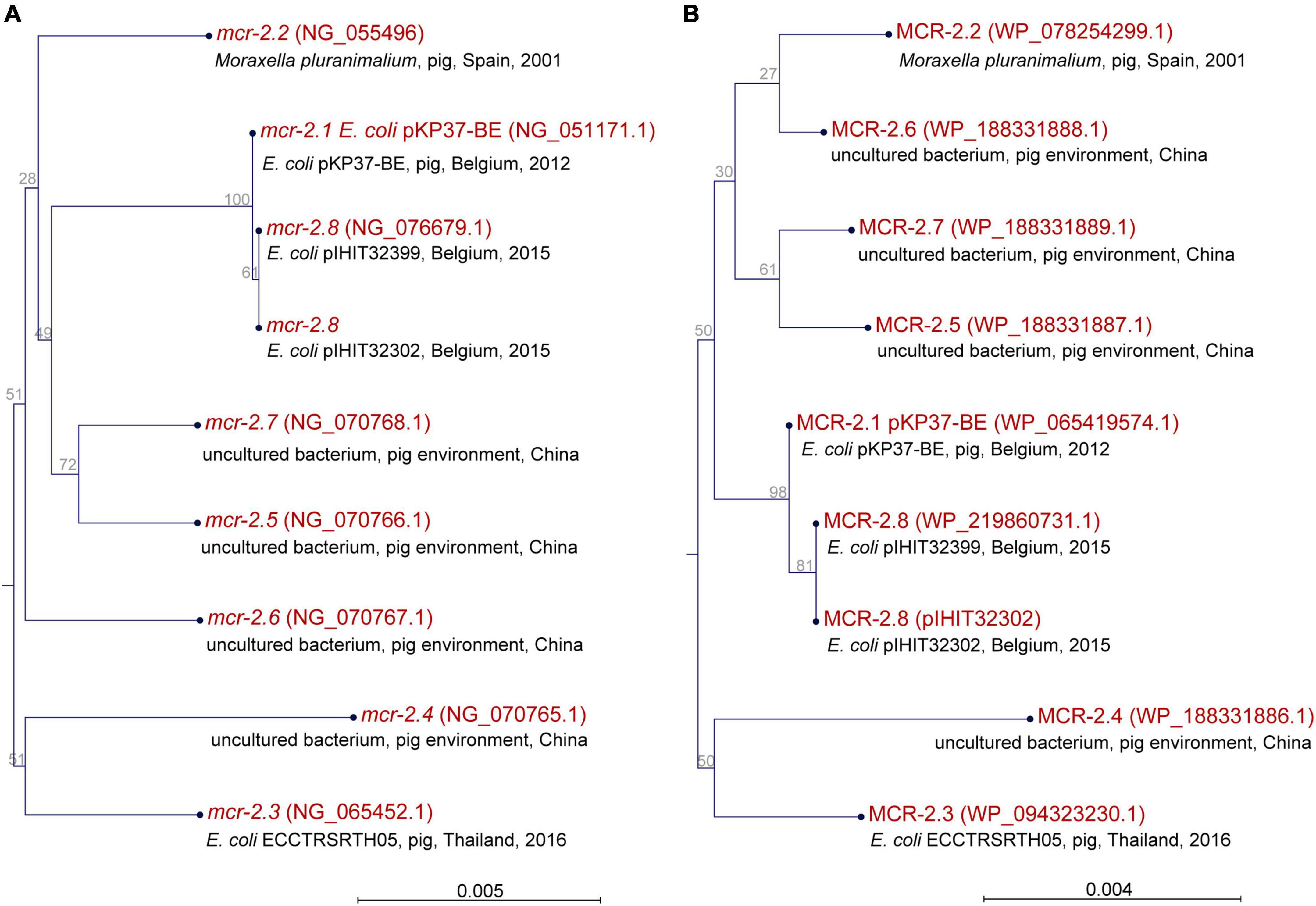
Figure 2. Phylogeny of seven confirmed and a novel mcr-2 genes (A) and deduced MCR-2 proteins (B) based on a maximum-likelihood method.
Genomic location and transferability of mcr-2 genes
We identified mcr-2 on two different plasmids (n = 11 isolates) and on the chromosome of our E. coli strains (n = 1 isolate). This was initially confirmed by S1 nuclease PFGE and Southern blotting (Supplementary Figure 1). Based on BLASTn analysis of constructed plasmid sequences, the MCR-2 plasmids of six E. coli isolates from Belgium and of the two German isolates were found to be highly similar (>99.8%) to the mcr-2-bearing IncX4 plasmid pKP37-BE (GenBank LT598652.1). Like pKP37-BE, they were 35 kb in size, belonged to incompatibility group IncX4, and possessed mcr-2 as the sole resistance gene. An overview of plasmid incompatibility groups and estimated plasmid sizes of all mcr-2-positive E. coli found in this study is given in Supplementary Table 4.
Three other isolates from Belgium revealed 47 kb MCR-2 plasmids (pIHIT31008-MCR-2, pIHIT32395-MCR-2, and pIHIT32396-MCR-2) that were identical in size and structure and also harbored mcr-2 as the sole resistance gene (Table 2). The genetic organization of pIHIT31008-MCR-2 as a representative of these plasmids is shown in Supplementary Figure 2. BLASTn analysis of the pIHIT31008-MCR-2 nucleotide sequence against the GenBank database revealed that this plasmid shared conserved backbones with previously published plasmids of 41.4 to 46.5 kb in size that were identified in E. coli, E. fergusonii and Salmonella Enteritidis. Seven plasmids with highest query coverage (84.0%–88.0%) and query cover plasmid identity (96.95%–100%) to our plasmid are provided in Supplementary Table 5. They were identified in bacteria from food in China (S. enterica, pCFSA664-2), pooled sheep fecal samples in the UK (E. coli, pRHB15-C18_3; E. fergusonii, pRHB23-C01_5), pig feces in Canada (E. coli, plasmid unnamed_novel_0), and also from human patient samples collected in Australia (S. Enteritidis, pAUSMDU00010527), Japan (E. coli, pTHO-015-2), and the U.S.A. (E. coli, pYDC107_41) from 2008 to 2018. In contrast to our plasmid, the previous plasmids commonly lacked the mcr-2-containing cassette (ISEc69-mcr-2-ORF-ISEc69) and an insertion sequence element IS91 that disrupted the type IV secretion system gene virB4 genetic region on our 47 kb plasmids (Figure 3).
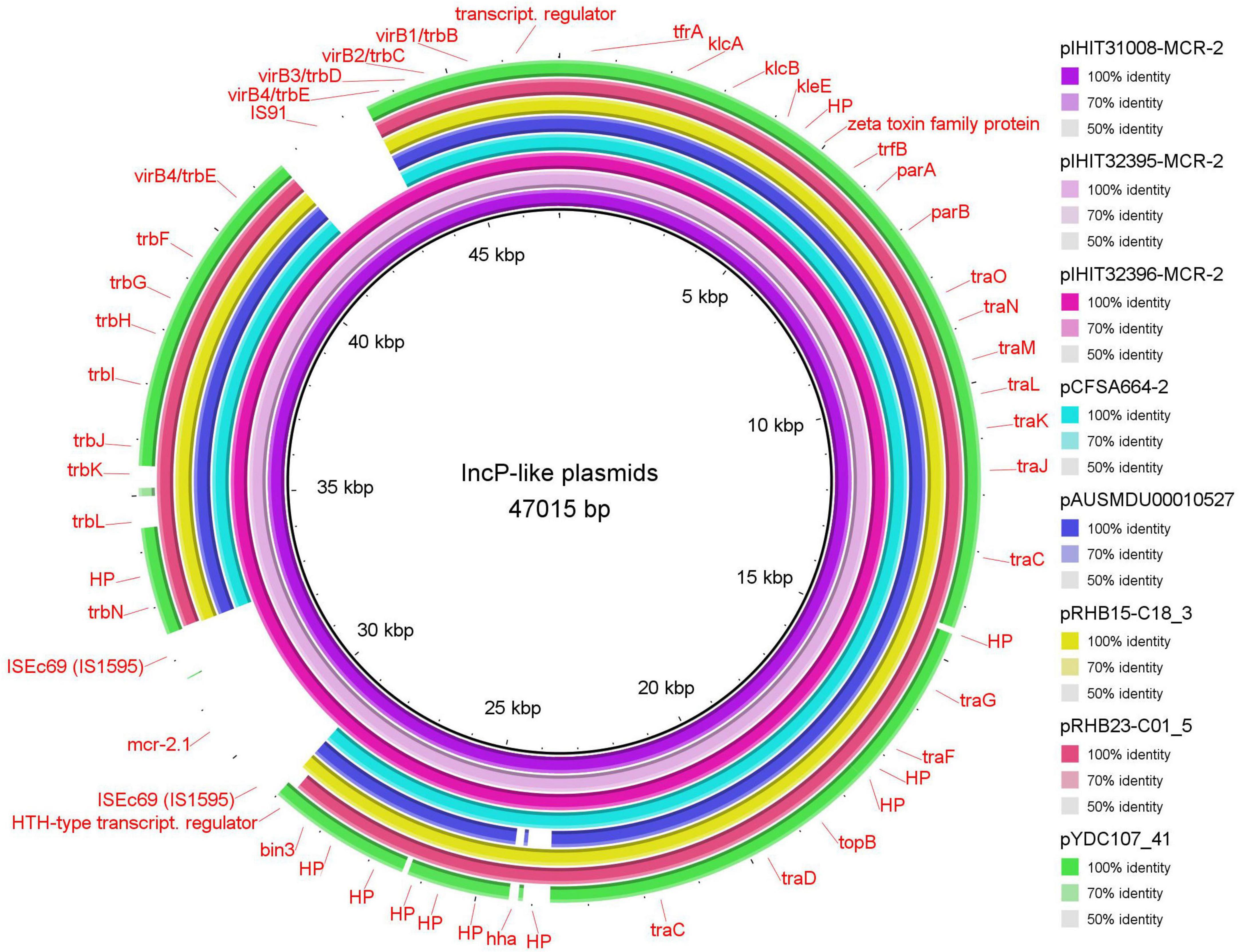
Figure 3. Circular representation of novel MCR-2 plasmids compared with five of the most similar reference plasmids available in GenBank (see Supplementary Table 4).
Figure 4A shows the integration site of the 3,489 bp mcr-2 gene cassette into the common plasmid backbone region of the published plasmids. The mcr-2 gene cassette of pIHIT31008-MCR-2 and of the two other 47 kb plasmids is almost identical (99.9%) to that described for pKP37-BE. Like in that IncX4 plasmid, the 1,617 bp mcr-2 gene is flanked by directly oriented copies of insertion sequence element ISEc69 of the IS1595 family (Partridge, 2017). We also identified a 297 bp open reading frame downstream of mcr-2 on this element, which encodes a PAP2 membrane-associated lipid phosphatase with 41% identity to Moraxella osloensis phosphatidic acid phosphatase (71% query coverage).
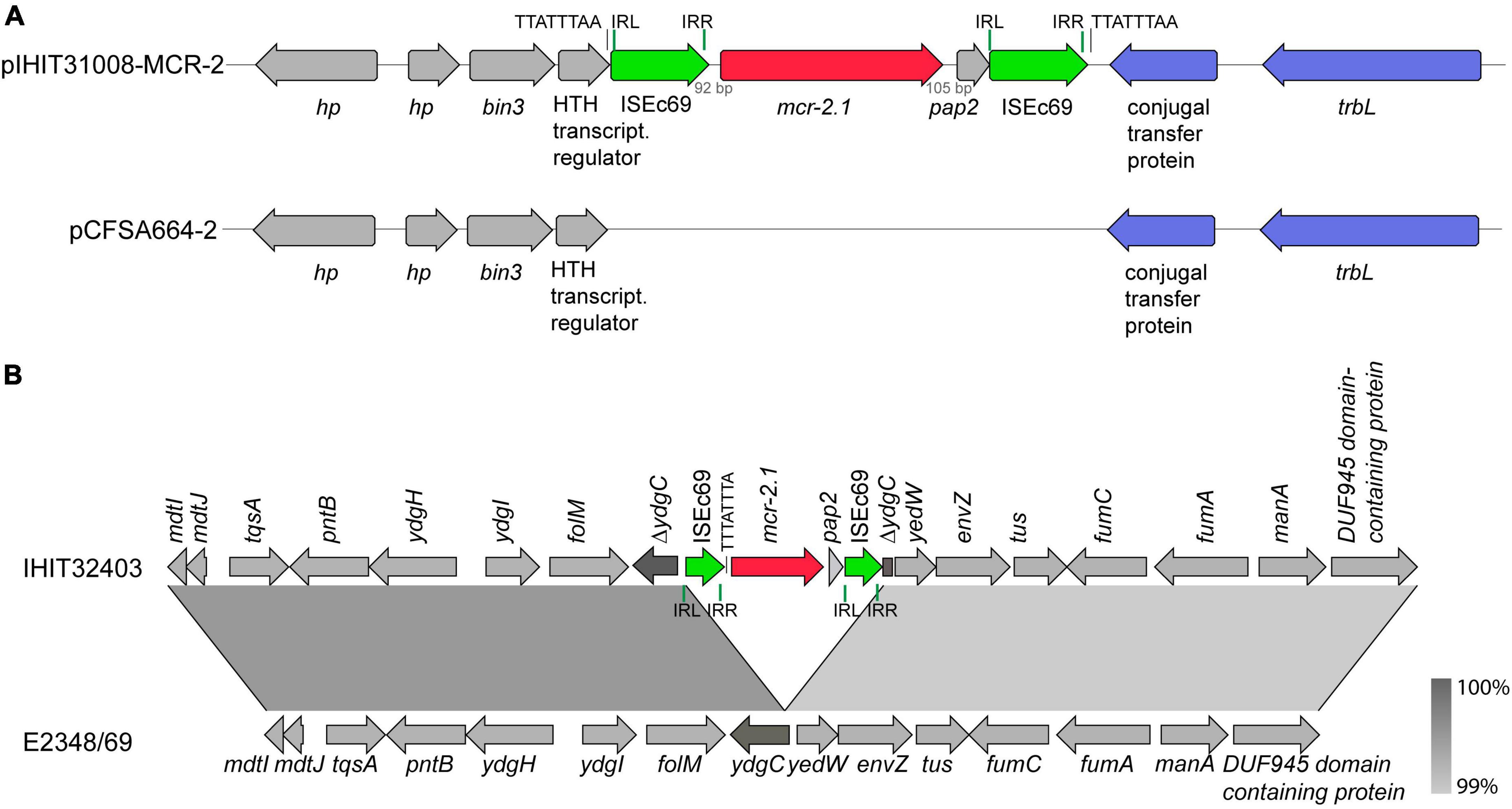
Figure 4. Genetic characterization of mcr-2 genes. (A) Scheme of the integration site of the mcr-2-containing cassette into a plasmid backbone present in pCFSA664-2 (CP033354.2; Salmonella enterica ssp. enterica from food, China, 2015) and other plasmids, shown in Figure 3. (B) Linear comparison of a partial region (18,296 bp) of the genome of mcr-2-positive atypical EPEC strain IHIT32403 (diarrheic pig, Spain, 2013, ST29, O45:H11, phylogroup B1) and prototype EPEC strain E2348/69 (infantile diarrhea, UK, 1969, ST15, O45:H11, phylogroup B2; NZ_BDOY01.1). Boxed arrows represent the position and transcriptional direction of open reading frames. Insertion sequences are highlighted with green arrows, transfer genes with light blue arrows. Green vertical bars represent inverted regions (IR) at the left (IRL) and right site (IRR) of each IS element; black vertical bars represent 8-bp direct repeat sequences. Region of >99% identity are shaded in gray.
The novel 47 kb MCR-2 plasmid was not predicted to have an Inc-type using PlasmidFinder. However, a BLASTn search of the IHIT31008 trfA gene, which encodes the initiation of plasmid replication, revealed four hits with a 100% nucleotide sequence identity to the IncP-1-like trfA replication genes of plasmids pAUSMDU00010527, pCFSA664-2, pRHB15-C18_3, and pRHB23-C01_5). In addition, the trfA genes of plasmids pYCD107, pTHO, and plasmid unnamednovel_0 showed 99.7% to 99.9% similarity to our genes (Supplementary Table 5).
A Neighbor-joining tree based on the alignment of TrfA amino acid sequences of representative members of the different IncP1 plasmid subfamilies α, β1, β2, δ, γ, ε, and ζ, our 47 kb MCR-2 plasmid and the aforementioned plasmids with identical backbones was created (Supplementary Figure 3). Interestingly, this showed that our MCR-2 plasmid and those with the shared backbone fall within the IncP1 family, but are phylogenetically different from the currently known IncP1 sub-families, indicating that they might represent a new subfamily. This clustering was also supported when performing a phylogeny inferred from concatenated sequences of the 22 plasmid backbone gene encoded proteins TrfA, TrfB, KfrA, KfrB, KlcA, KlcB, KorC, TraC, TraD, TraF, TraG, TraJ, TraK, TraL, TrbB, TrbC, TrbD, TrbF, TrbG, TrbI, TrbJ, and TrbK (data not shown) (Krol et al., 2012).
Seven out of eleven mcr-2-bearing plasmids from this study were successfully transferred to the recipient strain E. coli K12-J53. In case of strains IHIT32396 (47 kb IncP-1-like plasmid), IHIT32302, IHIT32399, and IHIT32397 (all IncX4 plasmids) conjugation experiments failed to transfer the pMCR-2 plasmid into the E. coli laboratory recipient. The seven pMCR-2 transformants showed a MIC of 4–8 mg/L for colistin, 16 to 32-fold higher than the empty J53 recipient (MIC = 0.25 mg/L).
The atypical EPEC isolate IHIT32403 carried its mcr-2.1 gene on the chromosome. Attempts to transfer mcr-2 by transconjugation failed, further confirming a chromosomal location of the gene. A linear comparison of a partial region (18,296 bp) of the genome of this ST29-B1 isolate of serotype O45:H11, that was obtained from a diarrheic pig in Spain in 2013, and of prototype O45:H11-ST15-B2 EPEC strain E2348/69, obtained from infantile diarrhea in Great Britain in 1969 (NZ_BDOY01.1) is shown in Figure 4B. The two regions were 100% identical, except for the insertion of gene cassette ISEc69-mcr-2-pap2-ISEc69 into the inner membrane protein gene ydgC in isolate IHIT32403.
Discussion
The colistin resistance gene mcr-1 gene is disseminated in many countries on all continents except Antarctica, and has been reported from various Gram-negative bacterial species from animals, human and the environment, such as E. coli, Klebsiella spp., Salmonella enterica serovars, Enterobacter spp. and Moraxella spp. (Nang et al., 2019; Anyanwu et al., 2021; Valiakos and Kapna, 2021). Our study revealed a prevalence of 11.5% mcr-1-positive isolates among pathogenic E. coli isolates collected from German pig farms in 2010-2017. A retrospective study on preselected bacterial cultures from asymptomatic fattening pigs, isolated in 2011 and 2012 in Germany, showed a similar prevalence of 9.9% (Roschanski et al., 2017). In a more recent study, Effelsberg et al. (2021) investigated the prevalence of mobile colistin resistance genes mcr-1 to mcr-5 in Enterobacterales from 81 pig farms in North-West Germany. In that study, the authors included fecal samples from pigs and stool samples from directly exposed humans working on the respective farms in 2018-2020. Two (1.4%) out of 138 stool samples from farmers, farm workers and their family members were tested positive for mcr-1, though a direct transmission could not be verified. The mcr-1 gene was detected in 5.7% of the porcine samples (Effelsberg et al., 2021).
Considering the overall rate of mcr-1 determined in the present study, including isolates from other European countries, prevalences were 10.4% in 2010-2017 and 2.4% in 2018-2020. From 2018 to 2020 we used selective culturing of bacteria on sheep blood agar containing colistin. Therefore, we cannot not exclude that some isolates carrying the mcr-1 gene were missed. Thus, no conclusions on prevalence dynamics can be drawn from comparison of these two data, but noteworthy, annual rates of mcr-1-positive E. coli isolates started to decrease already in 2016. Similar results were reported from Spain. Miguela-Villoldo et al. described a steady increase of mcr-1 in bacteria from cecal samples from pigs between 2004 and 2015 and a downward trend between 2017 and 2021 (Miguela-Villoldo et al., 2022). Another group from Spain screened 200 E. coli isolates collected in 1999 to 2018 from swine and reported a peak of colistin resistance (17.5% of the strains) in 2011–2014 (Aguirre et al., 2020). Our observation that mcr-1 occurrence is much less frequent in samples collected after 2015 is in line with the studies reported.
It needs to be verified whether observed decreases are already a consequence of the restrictions recommended by the WHO for the use of important antimicrobials, including colistin (WHO, 2017). Indeed, an observational study from Great Britain revealed that the stoppage of colistin usage in pig farms could lead to a lower persistence of mobile colistin resistance genes on a long-term (Duggett et al., 2018). Based on data from longitudinal studies performed in China, authors could determine an association between significantly reduced colistin sales and a decreased frequency of mcr-1-producing E. coli in pig feces and in the intestinal tract of healthy humans as well as decreased rates of human infections with colistin resistant E. coli after 2017. It was suggested that this might be a direct consequence of banning colistin as an animal growth promoter by the Chinese government in April 2017 (Wang et al., 2020b; Zhao et al., 2022). On the other hand, no significant association could be found in a cross-sectional study on 48 pig farms in 2011-2012 between presence of mcr-1 resistance genes in isolated E. coli and antimicrobial treatment of the sampled fattening pigs (Hille et al., 2018). In another study, healthy weaned piglets were inoculated with a colistin-resistant E. coli strain harboring mcr-1. Following this, one group of piglets was force-fed with colistin sulfate for five consecutive days. The double dose of colistin sulfate was given to a second group of piglets and a placebo group received water over the same period of time. A selection of mcr-positive strains was not observed, as the prevalence of the inoculated mcr-1-positive E. coli remained almost at the same levels in all groups over 25 days (Viel et al., 2018).
The mcr-2 gene, which was detected soon after mcr-1, was globally found on rare occasions and predominantly in Asian countries, including Bangladesh, China, India, Pakistan, Thailand, and Turkey (Valiakos and Kapna, 2021). Nine out of 40 studies performed in Europe reported infrequent to moderate findings of mcr-2 (0.15% – 11.4%) in samples, isolates or in the microbiota of livestock animals in Great Britain, Spain, Italy, and especially in Belgium in the years 2001 to 2019 (Xavier et al., 2016; AbuOun et al., 2017; Carattoli et al., 2017; Poirel et al., 2017; Garcia-Graells et al., 2018; Dobrzanska et al., 2020; Miguela-Villoldo et al., 2020; Bertelloni et al., 2022) (Supplementary Table 6). Although we tested 9,091 E. coli isolates from Germany and other European countries, only 12 isolates were mcr-2-positive. Out of these, nine isolates were obtained from three different pig farms in Belgium, which underlines the local spread of mcr-2 in this country. Interestingly, the two mcr-2-positive E. coli isolates from two different pig farms in Germany, collected in 2014, are, to the best of our knowledge, the first reported mcr-2 findings in this country. In two studies from 2011/2012 and 2011-2018 healthy animals from Germany, in particular pigs, were sampled and Gram-negative bacteria were tested for the presence of mcr-1 to mcr-2 and mcr-1 to mcr-9, respectively. While 9.9% of the 436 tested mixed bacterial cultures and 45% of 407 tested Salmonella enterica isolates were mcr-1-positive, none of the cultures and isolates harbored mcr-2 (Roschanski et al., 2017; Borowiak et al., 2020). In 2016 and 2017, raw municipal wastewater was sampled all over Germany and analyzed in a metagenomics approach. Genes mcr-3, mcr-4, mcr-5, and mcr-7 were ubiquitous in all 14 samples, but only one proved positive for mcr-1 and none for mcr-2 (Kneis et al., 2019). Likewise, mcr-2 was not detected in a very recent study performed on 456 samples obtained from pigs and humans in Germany, where all isolated Enterobacterales were tested for the genes mcr-1 to mcr-5 (Effelsberg et al., 2021). The systematic review by Valiakos et al. reported mcr-2 in five studies from swine, three from bovine and three from poultry (Valiakos and Kapna, 2021). Total numbers of mcr-2 were low, in particular in Europe and Africa. The vast majority of mcr-2-positive bacteria in that survey were E. coli. An exception was one Moraxella pluranimalium-like isolate harboring a mcr-2.2 variant isolated from pooled cecal samples of healthy pigs (AbuOun et al., 2017). A more recent study from Egypt tested Enterobacterales isolates, collected between 2018 and 2020 from bovine milk samples associated with mastitis for the genes mcr-1 to mcr-9. Among 117 tested isolates, 12.0% possessed mcr-2 and another 28.2% carried mcr-1, mcr-3, mcr-4 or mcr-7 genes (Tartor et al., 2021).
In non-European countries mcr-2 mediated colistin resistance was systematically tested in 64 studies (Supplementary Table 6). Twenty-five studies (38.5%) found bacteria harboring mcr-2 resistance genes. Peak prevalences were as high as 56.3% in healthy pigs in 2016 and 14.9% in healthy chickens in 2015-2016 in China (Zhang et al., 2018b; Zhang et al., 2019).
Also wild animals, reptiles and pets have been identified as a source of mcr-2-positive bacteria. In 2018 and 2019, 26/168 (15.5%) E. coli isolates from hunted wild boar harbored mcr-2 (Cilia et al., 2021). A study from Egypt identified mcr-2 in 2.5% of 122 Gram-negative bacterial isolates from wild birds (Ahmed et al., 2019). In pet animals, the prevalence of mcr-1 ranged from 0% to 12.5%, that of mcr-2 from 0% to 0.9% (Borowiak et al., 2020; Ilbeigi et al., 2021; Wang et al., 2021; Bertelloni et al., 2022; Hamame et al., 2022).
In 2018, Partridge et al. (2018) proposed a nomenclature scheme for mcr genes and their variants, therefore systematizing future registration of new discoveries of genes and allele numbers (Partridge et al., 2018). Their scheme suggested pre-publication submission of new mcr sequences to the International Nucleotide Sequence Database Collaboration.10 New mcr gene variants are defined by deduced amino acid sequence and assigned by the National Center for Biotechnology Information (NCBI). To date, a regularly updated overview of known mcr genes and variants is available in the Bacterial Antimicrobial Resistance Reference Gene Database, maintained by the NCBI.11 So far, seven genetically different mcr-2 variants are described in the literature (mcr-2.1 – mcr-2.7). We here report an additional genetic variant, termed mcr-2.8, which is closely related to mcr-2.1 and was found in E. coli isolates from pigs in Belgium. All other mcr-2 genes in our samples were mcr-2.1 (Table 2), which is also the most frequent variant worldwide.
Out of 12 mcr-2-positive bacterial isolates, ten could be typed as enterotoxigenic E. coli (ETEC) or ETEC-like and one as an atypical enteropathogenic E. coli (aEPEC). Another isolate from Belgium could not be grouped to any known E. coli pathotype as it lacked all taxonomical relevant combinations of virulence genes. ETEC are known for their frequent association with profuse neonatal and post-weaning diarrhea in pigs and calves (Foster and Smith, 2009; Liu et al., 2014). In an epidemiological study from 2018, ETEC was prevalent in 67% of post-weaning piglets suffering from diarrhea, followed by aEPEC (21.7%) (Garcia-Menino et al., 2018). In the same study, aEPEC was the most commonly detected pathovar (60.3%) among samples from diarrheic suckling piglets. The Global Burden of Disease study, performed between 1990 and 2016 in the USA, analyzed the impact of human diarrhea caused by ETEC on social and economic factors of health. At all age groups, ETEC was responsible for about 3.2% of deaths due to diarrhea in 2016 (Khalil et al., 2018). In humans, aEPEC are also regarded as important bacterial pathogens that are particularly involved in persistent diarrhea in children under five years of age (Afset et al., 2004; Mora et al., 2016; Snehaa et al., 2021). Unfortunately, the occurrence of mcr genes was not tested in the studies reporting on human ETEC and aEPEC isolates. Therefore, it remains to be determined whether mcr-mediated colistin resistance is relevant in E. coli strains causing diarrhea in humans.
Six mcr-2 genes and the two novel mcr-2 variants were found on IncX4 plasmids, which are known to play a vital role in the distribution of mcr-1 genes among Enterobacterales (Doumith et al., 2016; Jamin et al., 2021; Abou Fayad et al., 2022; Xie et al., 2022). The IncX4 plasmids detected in our study showed high sequence similarity (>99.8%) to the originally described plasmid pKP37-BE from E. coli in 2011/2012 (Xavier et al., 2016). This is in line with previously reported occurrences of pKP37-BE-like plasmids, indicating a steady appearance of this plasmid in Belgium in association with mcr-2 (Garcia-Graells et al., 2018; Timmermans et al., 2021). Our findings support the idea that IncX4 plasmids also play a role in the spread of mcr-2 genes.
So far, the only plasmid groups identified in association with mcr-2 were IncX4 and IncHI1B/IncFIB (Stosic et al., 2021). We found one new mcr-2-harboring plasmid (IncP-like plasmid), which was detected in three pigs on the same farm in Belgium. Plasmid transferability was observed in two of three E. coli isolates. This newly described plasmid represents to the best of our knowledge the third plasmid group beside IncX4 and IncHI1B/IncFIB harboring the mcr-2 gene. The low frequency of mcr-2-IncP-like plasmids in our strain collection suggests that this plasmid type has rarely spread yet. However, only 9 of 39 studies mentioned in Supplementary Table 6 explored the genomic location of mcr-2 and characterized the plasmids in more detail (Xavier et al., 2016; AbuOun et al., 2017; Poirel et al., 2017; Garcia-Graells et al., 2018; Stosic et al., 2021; Tartor et al., 2021; Timmermans et al., 2021; Trongjit and Chuanchuen, 2021; Phuadraksa et al., 2022). Thus, occurrence of IncP-like plasmids carrying the mcr-2 gene may be underestimated.
This study has some limitations. We have few information about pig housing conditions, hygiene standards, previous antimicrobial treatment and animal trafficking. Due to differing numbers of sample submissions from the different European countries throughout the years, the collected data are not representative of the target population. At the time of writing, ten different mcr genes (mcr-1 to mcr-10) were reported. In this study, we concentrated on mobilizable colistin resistant genes mcr-1 and mcr-2. Thus, future investigations on the distribution of the remaining mcr genes among the strain collection would be preferable. The chosen laboratory method since 2018, i.e., selective culturing of isolates on sheep blood agar containing 4 mg/L colistin, may have led to a fewer detection rate of mcr-positive isolates with low or diminished growth rates under these conditions (Smelikova et al., 2022).
On the other hand, our study has several strengths. We performed an extensive screening of plasmid-mediated colistin resistance genes (mcr-1 and mcr-2) on a large pool of putative pathogenic E. coli collected from pigs over a longer period of time. Moreover, mcr-2-positive isolates and their plasmids underwent detailed genotyping, while also the transferability of mcr-2 genes was explored.
Conclusion
We could confirm a continuous and substantial decrease in the percentage of porcine E. coli isolates harboring mcr-1 from 2015 to 2017. As expected, mcr-2-mediated colistin resistance was much less frequent than that conferred by mcr-1. We here described the new mcr-2.8 variant and a new mcr-2.1-bearing IncP1-like plasmid, which is capable of transferring colistin resistance to susceptible E. coli by conjugation. These novel variants and recent reports of mcr-2-positive bacteria in previously less frequently tested samples like bovine milk, pet animals and reptiles suggest that colistin resistance genes are highly variable and represent a constant threat to animal and human welfare. There is an urgent need for a longitudinal monitoring of mcr genes in Gram-negative bacteria from different sectors, including humans, animals, food products and the environment. This would ensure that the distribution of colistin resistant strains and putative novel resistance mechanisms are detected at an early stage. Gained knowledge about the distribution and epidemiology of mcr genes might help to prevent epidemic occurrence of multidrug resistant bacteria.
Data availability statement
Read genome sequences used in this study were submitted to the Sequence Read Archive (SRA) platform available from NCBI (https://trace.ncbi.nlm.nih.gov/Traces/sra) under biosample accession numbers: SAMN17614371 – SAMN17614382 and Sequence Read Run (SRR) numbers: SRR13570475 – SRR13570486.
Author contributions
CE and RB supervised the entire project. CE, LG, and RB drafted the manuscript. CE, KK, and RB designed the study. EP-B, CE, TS, and LG have provided raw data and/or analyzed the data and conducted part of the laboratory experiments. CE, TS, and LG performed analyses of sequencing data. All authors critically reviewed the manuscript.
Funding
TS was funded by the Federal Ministry of Education and Research (BMBF) under project number 01KI1703B as part of the ERA-NET COFUND antimicrobial resistances (JPI-EC-AMR) “HECTOR”. CE was funded by the Federal Ministry of Education and Research (BMBF) under project number 01KI1908B as part of the JPI-AMR project “OASIS”. The APC was partially funded by the Open Access Publication Funds of the Justus Liebig University Giessen, granted by the Germany Research Foundation.
Acknowledgments
We thank all colleagues of the in-house microbiology diagnostic laboratory for collecting E. coli isolates. We thank Anja Schwanitz for conducting E. coli pathotyping PCR and Regina Weil for screening of E. coli isolates on colistin agar. We also thank Maria Buelte and Ursula Leidner for molecular and phenotypic typing of E. coli isolates and plasmids.
Conflict of interest
The authors declare that the research was conducted in the absence of any commercial or financial relationships that could be construed as a potential conflict of interest.
Publisher’s note
All claims expressed in this article are solely those of the authors and do not necessarily represent those of their affiliated organizations, or those of the publisher, the editors and the reviewers. Any product that may be evaluated in this article, or claim that may be made by its manufacturer, is not guaranteed or endorsed by the publisher.
Supplementary material
The Supplementary Material for this article can be found online at: https://www.frontiersin.org/articles/10.3389/fmicb.2022.1076315/full#supplementary-material
Abbreviations
AMR, antimicrobial resistance; CARD, comprehensive antibiotic resistance database; CGE, center for genomic epidemiology; EPEC, enteropathogenic E. coli; aEPEC, atypical EPEC; ETEC, enterotoxigenic E. coli; MALDI-TOF MS, matrix-assisted laser desorption/ionization- time of flight mass spectrometry; MLST, multilocus sequence typing; NCBI, national center for biotechnology information; ST, sequence type; STEC, shiga toxin producing E. coli; VAG, virulence-associated gene; WGS, whole genome sequencing.
Footnotes
- ^ https://www.ncbi.nlm.nih.gov/pathogens/isolates#/refgene/
- ^ http://www.eucast.org
- ^ https://cge.food.dtu.dk/services/ResFinder/
- ^ https://card.mcmaster.ca
- ^ https://cge.food.dtu.dk/services/PlasmidFinder/
- ^ https://cge.food.dtu.dk/services/SerotypeFinder/
- ^ https://cge.food.dtu.dk/services/FimTyper/
- ^ https://cge.food.dtu.dk/services/MLST/
- ^ https://pubmlst.org/mlst/
- ^ https://www.insdc.org/
- ^ https://www.ncbi.nlm.nih.gov/pathogens/refgene/#mcr
References
Abou Fayad, A., El Azzi, M., Sleiman, A., Kassem, I., Bawazeer, R. A., Okdah, L., et al. (2022). Acquired resistome and plasmid sequencing of mcr-1 carrying MDR Enterobacteriaceae from poultry and their relationship to STs associated with humans. JAC Antimicrob. Resist. 4:dlab198. doi: 10.1093/jacamr/dlab198
AbuOun, M., Stubberfield, E. J., Duggett, N. A., Kirchner, M., Dormer, L., Nunez-Garcia, J., et al. (2017). mcr-1 and mcr-2 variant genes identified in Moraxella species isolated from pigs in Great Britain from 2014 to 2015. J. Antimicrob. Chemother. 72, 2745–2749. doi: 10.1093/jac/dkx286
Afset, J. E., Bevanger, L., Romundstad, P., and Bergh, K. (2004). Association of atypical enteropathogenic Escherichia coli (EPEC) with prolonged diarrhoea. J. Med. Microbiol. 53, 1137–1144. doi: 10.1099/jmm.0.45719-0
Aguirre, L., Vidal, A., Seminati, C., Tello, M., Redondo, N., Darwich, L., et al. (2020). Antimicrobial resistance profile and prevalence of extended-spectrum beta-lactamases (ESBL), AmpC beta-lactamases and colistin resistance (mcr) genes in Escherichia coli from swine between 1999 and 2018. Porcine Health Manag. 6:8. doi: 10.1186/s40813-020-00146-2
Ahmed, Z. S., Elshafiee, E. A., Khalefa, H. S., Kadry, M., and Hamza, D. A. (2019). Evidence of colistin resistance genes (mcr-1 and mcr-2) in wild birds and its public health implication in Egypt. Antimicrob. Resist. Infect. Control 8:197. doi: 10.1186/s13756-019-0657-5
Anyanwu, M. U., Jaja, I. F., Okpala, C. O. R., Jaja, C. I., Oguttu, J. W., Chah, K. F., et al. (2021). Potential sources and characteristic occurrence of mobile colistin resistance (mcr) gene-harbouring bacteria recovered from the poultry sector: A literature synthesis specific to high-income countries. PeerJ. 9, e11606. doi: 10.7717/peerj.11606
Ara, B., Urmi, U. L., Haque, T. A., Nahar, S., Rumnaz, A., Ali, T., et al. (2021). Detection of mobile colistin-resistance gene variants (mcr-1 and mcr-2) in urinary tract pathogens in Bangladesh: The last resort of infectious disease management colistin efficacy is under threat. Expert Rev. Clin. Pharmacol. 14, 513–522. doi: 10.1080/17512433.2021.1901577
Arredondo-Alonso, S., Rogers, M. R. C., Braat, J. C., Verschuuren, T. D., Top, J., Corander, J., et al. (2018). mlplasmids: A user-friendly tool to predict plasmid- and chromosome-derived sequences for single species. Microb Genom 4:e000224. doi: 10.1099/mgen.0.000224
Beghain, J., Bridier-Nahmias, A., Le Nagard, H., Denamur, E., and Clermont, O. (2018). ClermonTyping: An easy-to-use and accurate in silico method for Escherichia genus strain phylotyping. Microb. Genom. 4:e000192. doi: 10.1099/mgen.0.000192
Bertelloni, F., Cagnoli, G., Turchi, B., and Ebani, V. V. (2022). Low level of colistin resistance and mcr genes presence in Salmonella spp.: Evaluation of isolates collected between 2000 and 2020 from animals and environment. Antibiotics (Basel) 11:272. doi: 10.3390/antibiotics11020272
Borowiak, M., Baumann, B., Fischer, J., Thomas, K., Deneke, C., Hammerl, J. A., et al. (2020). Development of a novel mcr-6 to mcr-9 multiplex PCR and assessment of mcr-1 to mcr-9 occurrence in colistin-resistant Salmonella enterica isolates from environment, feed, animals and food (2011–2018) in Germany. Front. Microbiol. 11:80. doi: 10.3389/fmicb.2020.00080
Borowiak, M., Fischer, J., Hammerl, J. A., Hendriksen, R. S., Szabo, I., and Malorny, B. (2017). Identification of a novel transposon-associated phosphoethanolamine transferase gene, mcr-5, conferring colistin resistance in d-tartrate fermenting Salmonella enterica subsp. enterica serovar Paratyphi B. J. Antimicrob. Chemother. 72, 3317–3324. doi: 10.1093/jac/dkx327
Carattoli, A., Villa, L., Feudi, C., Curcio, L., Orsini, S., Luppi, A., et al. (2017). Novel plasmid-mediated colistin resistance mcr-4 gene in Salmonella and Escherichia coli. Italy 2013, Spain and Belgium, 2015 to 2016. Euro Surveill. 22:30589. doi: 10.2807/1560-7917.ES.2017.22.31.30589
Carroll, L. M., Gaballa, A., Guldimann, C., Sullivan, G., Henderson, L. O., and Wiedmann, M. (2019). Identification of novel mobilized colistin resistance gene mcr-9 in a multidrug-resistant, colistin-susceptible Salmonella enterica Serotype Typhimurium isolate. mBio 10, e853–e819. doi: 10.1128/mBio.00853-19
Casey, T. A., and Bosworth, B. T. (2009). Design and evaluation of a multiplex polymerase chain reaction assay for the simultaneous identification of genes for nine different virulence factors associated with Escherichia coli that cause diarrhea and edema disease in swine. J. Vet. Diagn. Invest. 21, 25–30. doi: 10.1177/104063870902100104
Cilia, G., Turchi, B., Fratini, F., Ebani, V. V., Turini, L., Cerri, D., et al. (2021). Phenotypic and genotypic resistance to colistin in E. coli isolated from wild boar (Sus scrofa) hunted in Italy. Eur. J. Wild. Res. 67:57. doi: 10.1007/s10344-021-01501-6
Clermont, O., Christenson, J. K., Denamur, E., and Gordon, D. M. (2013). The clermont Escherichia coli phylotyping method revisited: Improvement of specificity and detection of new phylogroups. Environ. Microbiol. Rep. 5, 58–65. doi: 10.1111/1758-2229.12019
CLSI. (2018). Performance Standards for Antimicrobial Susceptibility Testing, 28th Edn. Wayne, PA: Clinical and Laboratory Standards Institute.
Conant, G. C., and Wolfe, K. H. (2008). GenomeVx: Simple web-based creation of editable circular chromosome maps. Bioinformatics 24, 861–862. doi: 10.1093/bioinformatics/btm598
Dobrzanska, D. A., Lamaudiere, M. T. F., Rollason, J., Acton, L., Duncan, M., Compton, S., et al. (2020). Preventive antibiotic treatment of calves: Emergence of dysbiosis causing propagation of obese state-associated and mobile multidrug resistance-carrying bacteria. Microb. Biotechnol. 13, 669–682. doi: 10.1111/1751-7915.13496
Doumith, M., Godbole, G., Ashton, P., Larkin, L., Dallman, T., Day, M., et al. (2016). Detection of the plasmid-mediated mcr-1 gene conferring colistin resistance in human and food isolates of Salmonella enterica and Escherichia coli in England and Wales. J. Antimicrob. Chemother. 71, 2300–2305. doi: 10.1093/jac/dkw093
Duggett, N. A., Randall, L. P., Horton, R. A., Lemma, F., Kirchner, M., Nunez-Garcia, J., et al. (2018). Molecular epidemiology of isolates with multiple mcr plasmids from a pig farm in Great Britain: The effects of colistin withdrawal in the short and long term. J. Antimicrob. Chemother. 73, 3025–3033. doi: 10.1093/jac/dky292
Dutta, A., Barua, H., Jalal, M. S., Dhar, P. K., Biswas, S. K., and Biswas, P. K. (2018). An investigation of plasmid-mediated colistin resistance mechanism, MCR in Escherichia coli of human, veterinary and environmental origin in Bangladesh. Int. J. Inf. Dis 73:54. doi: 10.1016/j.ijid.2018.04.3547
Effelsberg, N., Kobusch, I., Linnemann, S., Hofmann, F., Schollenbruch, H., Mellmann, A., et al. (2021). Prevalence and zoonotic transmission of colistin-resistant and carbapenemase-producing Enterobacterales on German pig farms. One Health 13:100354. doi: 10.1016/j.onehlt.2021.100354
Ejaz, H., Younas, S., Qamar, M. U., Junaid, K., Abdalla, A. E., Abosalif, K. O. A., et al. (2021). Molecular epidemiology of extensively drug-resistant mcr encoded colistin-resistant bacterial strains co-expressing multifarious beta-lactamases. Antibiotics (Basel) 10:467. doi: 10.3390/antibiotics10040467
EUCAST (2020). The European committee on antimicrobial susceptibility testing. Breakpoint tables for interpretation of MICs and zone diameters. Version 10.0, 2020. http://www.eucast.org (accessed July 21, 2022)
Ewers, C., Bethe, A., Stamm, I., Grobbel, M., Kopp, P. A., Guerra, B., et al. (2014). CTX-M-15-D-ST648 Escherichia coli from companion animals and horses: Another pandemic clone combining multiresistance and extraintestinal virulence? J. Antimicrob. Chemother. 69, 1224–1230. doi: 10.1093/jac/dkt516
Ewers, C., Goettig, S., Buelte, M., Fiedler, S., Tietgen, M., Leidner, U., et al. (2016). Genome sequence of avian Escherichia coli strain IHIT25637, an extraintestinal pathogenic E. coli strain of ST131 encoding colistin resistance determinant MCR-1. Genome Announc 4, e863–e816. doi: 10.1128/genomeA.00863-16
Falgenhauer, L., Waezsada, S. E., Yao, Y., Imirzalioglu, C., Kaesbohrer, A., Roesler, U., et al. (2016). Colistin resistance gene mcr-1 in extended-spectrum beta-lactamase-producing and carbapenemase-producing Gram-negative bacteria in Germany. Lancet Infect. Dis. 16, 282–283. doi: 10.1016/S1473-3099(16)00009-8
Foster, D. M., and Smith, G. W. (2009). Pathophysiology of diarrhea in calves. Vet. Clin. North. Am. Food Anim. Pract 25, 13–36. doi: 10.1016/j.cvfa.2008.10.013
Garcia-Graells, C., De Keersmaecker, S. C. J., Vanneste, K., Pochet, B., Vermeersch, K., Roosens, N., et al. (2018). Detection of plasmid-mediated colistin resistance, mcr-1 and mcr-2 genes, in Salmonella spp. isolated from food at retail in Belgium from 2012 to 2015. Foodborne Pathog. Dis. 15, 114–117. doi: 10.1089/fpd.2017.2329
Garcia-Menino, I., Garcia, V., Mora, A., Diaz-Jimenez, D., Flament-Simon, S. C., Alonso, M. P., et al. (2018). Swine enteric colibacillosis in Spain: Pathogenic potential of mcr-1 ST10 and ST131 E. coli isolates. Front. Microbiol. 9:2659. doi: 10.3389/fmicb.2018.02659
Hamame, A., Davoust, B., Rolain, J. M., and Diene, S. M. (2022). Screening of colistin-resistant bacteria in domestic pets from France. Animals (Basel) 12:633. doi: 10.3390/ani12050633
Hille, K., Roschanski, N., Ruddat, I., Woydt, J., Hartmann, M., Roesler, U., et al. (2018). Investigation of potential risk factors for the occurrence of Escherichia coli isolates from German fattening pig farms harbouring the mcr-1 colistin-resistance gene. Int. J. Antimicrob. Agents 51, 177–180. doi: 10.1016/j.ijantimicag.2017.08.007
Ilbeigi, K., Askari Badouei, M., Vaezi, H., Zaheri, H., Aghasharif, S., and Kafshdouzan, K. (2021). Molecular survey of mcr1 and mcr2 plasmid mediated colistin resistance genes in Escherichia coli isolates of animal origin in Iran. BMC Res. Notes 14:107. doi: 10.1186/s13104-021-05519-6
Imtiaz, W., Syed, Z., Rafaque, Z., Andrews, S. C., and Dasti, J. I. (2021). Analysis of antibiotic resistance and virulence traits (genetic and phenotypic) in Klebsiella pneumoniae clinical isolates from Pakistan: Identification of significant levels of carbapenem and colistin resistance. Infect. Drug Resist. 14, 227–236. doi: 10.2147/IDR.S293290
Irrgang, A., Roschanski, N., Tenhagen, B. A., Grobbel, M., Skladnikiewicz-Ziemer, T., Thomas, K., et al. (2016). Prevalence of mcr-1 in E. coli from Livestock and Food in Germany, 2010-2015. PLoS One 11:e0159863. doi: 10.1371/journal.pone.0159863
Islam, S., Urmi, U. L., Rana, M., Sultana, F., Jahan, N., Hossain, B., et al. (2020). High abundance of the colistin resistance gene mcr-1 in chicken gut-bacteria in Bangladesh. Sci. Rep. 10:17292. doi: 10.1038/s41598-020-74402-4
Jamin, C., Sanders, B. K., Zhou, M., Costessi, A., Duijsings, D., Kluytmans, J., et al. (2021). Genetic analysis of plasmid-encoded mcr-1 resistance in Enterobacteriaceae derived from poultry meat in the Netherlands. JAC Antimicrob. Resist 3:dlab156. doi: 10.1093/jacamr/dlab156
Javed, H., Saleem, S., Zafar, A., Ghafoor, A., Shahzad, A. B., Ejaz, H., et al. (2020). Emergence of plasmid-mediated mcr genes from Gram-negative bacteria at the human-animal interface. Gut Pathog. 12:54. doi: 10.1186/s13099-020-00392-3
Joensen, K. G., Tetzschner, A. M., Iguchi, A., Aarestrup, F. M., and Scheutz, F. (2015). Rapid and easy in silico serotyping of Escherichia coli isolates by use of whole-genome sequencing data. J. Clin. Microbiol. 53, 2410–2426. doi: 10.1128/JCM.00008-15
Ketkhao, P., Thongratsakul, S., Poolperm, P., Poolkhet, C., and Amavisit, P. (2021). Antimicrobial resistance profiles of Escherichia coli from swine farms using different antimicrobials and management systems. Vet. World 14, 689–695. doi: 10.14202/vetworld.2021.689-695
Khalil, I. A., Troeger, C., Blacker, B. F., Rao, P. C., Brown, A., Atherly, D. E., et al. (2018). Morbidity and mortality due to shigella and enterotoxigenic Escherichia coli diarrhoea: The global burden of disease study 1990-2016. Lancet Infect. Dis. 18, 1229–1240. doi: 10.1016/S1473-3099(18)30475-4
Kneis, D., Berendonk, T. U., and Hess, S. (2019). High prevalence of colistin resistance genes in German municipal wastewater. Sci. Total Environ. 694:133454. doi: 10.1016/j.scitotenv.2019.07.260
Krol, J. E., Penrod, J. T., McCaslin, H., Rogers, L. M., Yano, H., Stancik, A. D., et al. (2012). Role of IncP-1beta plasmids pWDL7::rfp and pNB8c in chloroaniline catabolism as determined by genomic and functional analyses. Appl. Environ. Microbiol. 78, 828–838. doi: 10.1128/AEM.07480-11
Larsen, M. V., Cosentino, S., Rasmussen, S., Friis, C., Hasman, H., Marvig, R. L., et al. (2012). Multilocus sequence typing of total-genome-sequenced bacteria. J. Clin. Microbiol. 50, 1355–1361. doi: 10.1128/JCM.06094-11
Liu, W., Yuan, C., Meng, X., Du, Y., Gao, R., Tang, J., et al. (2014). Frequency of virulence factors in Escherichia coli isolated from suckling pigs with diarrhoea in China. Vet. J. 199, 286–289. doi: 10.1016/j.tvjl.2013.11.019
Liu, Y. Y., Wang, Y., Walsh, T. R., Yi, L. X., Zhang, R., Spencer, J., et al. (2016). Emergence of plasmid-mediated colistin resistance mechanism MCR-1 in animals and human beings in China: A microbiological and molecular biological study. Lancet Infect. Dis. 16, 161–168. doi: 10.1016/S1473-3099(15)00424-7
Luppi, A., Gibellini, M., Gin, T., Vangroenweghe, F., Vandenbroucke, V., Bauerfeind, R., et al. (2016). Prevalence of virulence factors in enterotoxigenic Escherichia coli isolated from pigs with post-weaning diarrhoea in Europe. Porcine Health Manag. 2:20. doi: 10.1186/s40813-016-0039-9
Miguela-Villoldo, P., Moreno, M. A., Hernandez, M., Rodriguez-Lazaro, D., Gallardo, A., Borge, C., et al. (2020). Complementarity of selective culture and qPCR for colistin resistance screening in fresh and frozen pig cecum samples. Front. Microbiol. 11:572712. doi: 10.3389/fmicb.2020.572712
Miguela-Villoldo, P., Moreno, M. A., Rodriguez-Lazaro, D., Gallardo, A., Hernandez, M., Serrano, T., et al. (2022). Longitudinal study of the mcr-1 gene prevalence in Spanish food-producing pigs from 1998 to 2021 and its relationship with the use of polymyxins. Porcine Health Manag. 8, 12. doi: 10.1186/s40813-022-00255-0
Mitra, S., Basu, S., Rath, S., and Sahu, S. K. (2020). Colistin resistance in Gram-negative ocular infections: Prevalence, clinical outcome and antibiotic susceptibility patterns. Int. Ophthalmol. 40, 1307–1317. doi: 10.1007/s10792-020-01298-4
Mora, F. X., Aviles-Reyes, R. X., Guerrero-Latorre, L., and Fernandez-Moreira, E. (2016). Atypical enteropathogenic Escherichia coli (aEPEC) in children under five years old with diarrhea in Quito (Ecuador). Int. Microbiol. 19, 157–160. doi: 10.2436/20.1501.01.273
Nang, S. C., Li, J., and Velkov, T. (2019). The rise and spread of mcr plasmid-mediated polymyxin resistance. Crit. Rev. Microbiol. 45, 131–161. doi: 10.1080/1040841X.2018.1492902
Partridge, S. R. (2017). mcr-2 in the IncX4 plasmid pKP37-BE is flanked by directly oriented copies of ISEc69. J. Antimicrob. Chemother. 72, 1533–1535. doi: 10.1093/jac/dkw575
Partridge, S. R., Di Pilato, V., Doi, Y., Feldgarden, M., Haft, D. H., Klimke, W., et al. (2018). Proposal for assignment of allele numbers for mobile colistin resistance (mcr) genes. J. Antimicrob. Chemother. 73, 2625–2630. doi: 10.1093/jac/dky262
Phuadraksa, T., Wichit, S., Arikit, S., Songtawee, N., and Yainoy, S. (2022). Co-occurrence of mcr-2 and mcr-3 genes on chromosome of multidrug-resistant Escherichia coli isolated from healthy individuals in Thailand. Int. J. Antimicrob. Agents 60:106662. doi: 10.1016/j.ijantimicag.2022.106662
Poirel, L., Kieffer, N., Fernandez-Garayzabal, J. F., Vela, A. I., Larpin, Y., and Nordmann, P. (2017). MCR-2-mediated plasmid-borne polymyxin resistance most likely originates from Moraxella pluranimalium. J. Antimicrob. Chemother. 72, 2947–2949. doi: 10.1093/jac/dkx225
Rhouma, M., Fairbrother, J. M., Beaudry, F., and Letellier, A. (2017). Post weaning diarrhea in pigs: Risk factors and non-colistin-based control strategies. Acta Vet. Scand. 59:31. doi: 10.1186/s13028-017-0299-7
Rhouma, M., Theriault, W., Rabhi, N., Duchaine, C., Quessy, S., and Fravalo, P. (2019). First identification of mcr-1/mcr-2 genes in the fecal microbiota of Canadian commercial pigs during the growing and finishing period. Vet. Med. (Auckl.) 10, 65–67. doi: 10.2147/VMRR.S202331
Roschanski, N., Falgenhauer, L., Grobbel, M., Guenther, S., Kreienbrock, L., Imirzalioglu, C., et al. (2017). Retrospective survey of mcr-1 and mcr-2 in German pig-fattening farms, 2011-2012. Int. J. Antimicrob. Agents 50, 266–271. doi: 10.1016/j.ijantimicag.2017.03.007
Schwarz, S., and Johnson, A. P. (2016). Transferable resistance to colistin: A new but old threat. J. Antimicrob. Chemother. 71, 2066–2070. doi: 10.1093/jac/dkw274
Smelikova, E., Tkadlec, J., and Krutova, M. (2022). How to: Screening for mcr-mediated resistance to colistin. Clin. Microbiol. Infect. 28, 43–50. doi: 10.1016/j.cmi.2021.09.009
Smith, C. A., Pinkney, M., Guiney, D. G., and Thomas, C. M. (1993). The ancestral IncP replication system consisted of contiguous oriV and trfA segments as deduced from a comparison of the nucleotide sequences of diverse IncP plasmids. J. Gen. Microbiol. 139, 1761–1766. doi: 10.1099/00221287-139-8-1761
Snehaa, K., Singh, T., Dar, S. A., Haque, S., Ramachandran, V. G., Saha, R., et al. (2021). Typical and atypical enteropathogenic Escherichia coli in children with acute diarrhoea: Changing trend in East Delhi. Biomed. J. 44, 471–478. doi: 10.1016/j.bj.2020.03.011
Stosic, M. S., Leangapichart, T., Lunha, K., Jiwakanon, J., Angkititrakul, S., Jarhult, J. D., et al. (2021). Novel mcr-3.40 variant co-located with mcr-2.3 and blaCTX–M–63 on an IncHI1B/IncFIB plasmid found in Klebsiella pneumoniae from a healthy carrier in Thailand. J. Antimicrob. Chemother. 73, 2218–2220. doi: 10.1093/jac/dkab147
Tartor, Y. H., Gharieb, R. M. A., Abd El-Aziz, N. K., El Damaty, H. M., Enany, S., Khalifa, E., et al. (2021). Virulence determinants and plasmid-mediated colistin resistance mcr genes in Gram-negative bacteria isolated from bovine milk. Front. Cell Infect. Microbiol. 11:761417. doi: 10.3389/fcimb.2021.761417
Timmermans, M., Wattiau, P., Denis, O., and Boland, C. (2021). Colistin resistance genes mcr-1 to mcr-5, including a case of triple occurrence (mcr-1, -3 and -5), in Escherichia coli isolates from faeces of healthy pigs, cattle and poultry in Belgium, 2012-2016. Int. J. Antimicrob. Agents 57:106350. doi: 10.1016/j.ijantimicag.2021.106350
Trongjit, S., and Chuanchuen, R. (2021). Whole genome sequencing and characteristics of Escherichia coli with co-existence of ESBL and mcr genes from pigs. PLoS One 16:e0260011. doi: 10.1371/journal.pone.0260011
Unger, F., Eisenberg, T., Prenger-Berninghoff, E., Leidner, U., Ludwig, M. L., Rothe, M., et al. (2017). Imported reptiles as a risk factor for the global distribution of Escherichia coli harbouring the colistin resistance gene mcr-1. Int. J. Antimicrob. Agents 49, 122–123. doi: 10.1016/j.ijantimicag.2016.10.007
Valiakos, G., and Kapna, I. (2021). Colistin resistant mcr genes prevalence in livestock animals (swine, bovine, poultry) from a multinational perspective. A systematic review. Vet. Sci. 8:265. doi: 10.3390/vetsci8110265
Viel, A., Henri, J., Perrin-Guyomard, A., Laroche, J., Couet, W., Gregoire, N., et al. (2018). Lack of experimental evidence to support mcr-1-positive Escherichia coli strain selection during oral administration of colistin at recommended and higher dose given by gavage in weaned piglets. Int. J. Antimicrob. Agents 51, 128–131. doi: 10.1016/j.ijantimicag.2017.04.013
Wang, C., Feng, Y., Liu, L., Wei, L., Kang, M., and Zong, Z. (2020a). Identification of novel mobile colistin resistance gene mcr-10. Emerg. Microbes Infect. 9, 508–516. doi: 10.1080/22221751.2020.1732231
Wang, G., Liu, H., Feng, Y., Zhang, Z., Hu, H., Liu, J., et al. (2021). Colistin-resistance mcr genes in Klebsiella pneumoniae from companion animals. J. Glob. Antimicrob. Resist. 25, 35–36. doi: 10.1016/j.jgar.2021.02.023
Wang, X., Wang, Y., Zhou, Y., Li, J., Yin, W., Wang, S., et al. (2018). Emergence of a novel mobile colistin resistance gene, mcr-8, in NDM-producing Klebsiella pneumoniae. Emerg. Microbes Infect. 7, 122. doi: 10.1038/s41426-018-0124-z
Wang, Y., Xu, C., Zhang, R., Chen, Y., Shen, Y., Hu, F., et al. (2020b). Changes in colistin resistance and mcr-1 abundance in Escherichia coli of animal and human origins following the ban of colistin-positive additives in China: An epidemiological comparative study. Lancet Infect. Dis. 20, 1161–1171. doi: 10.1016/S1473-3099(20)30149-3
WHO. (2017). WHO guidelines on use of medically important antimicrobials in food-producing animals. Geneva: World Health Organization, Licence.
Xavier, B. B., Lammens, C., Ruhal, R., Kumar-Singh, S., Butaye, P., Goossens, H., et al. (2016). Identification of a novel plasmid-mediated colistin-resistance gene, mcr-2, in Escherichia coli, Belgium, June 2016. Euro Surveill. 21:30280. doi: 10.2807/1560-7917.ES.2016.21.27.30280
Xie, J., Liang, B., Xu, X., Yang, L., Li, H., Li, P., et al. (2022). Identification of mcr-1-positive multidrug-resistant Escherichia coli isolates from clinical samples in Shanghai. China. J. Glob. Antimicrob. Resist. 29, 88–96. doi: 10.1016/j.jgar.2022.02.008
Yang, Y. Q., Li, Y. X., Lei, C. W., Zhang, A. Y., and Wang, H. N. (2018). Novel plasmid-mediated colistin resistance gene mcr-7.1 in Klebsiella pneumoniae. J. Antimicrob. Chemother. 73, 1791–1795. doi: 10.1093/jac/dky111
Yin, W., Li, H., Shen, Y., Liu, Z., Wang, S., Shen, Z., et al. (2017). Novel plasmid-mediated colistin resistance gene mcr-3 in Escherichia coli. mBio 8:e00543-17.
Zankari, E., Hasman, H., Cosentino, S., Vestergaard, M., Rasmussen, S., Lund, O., et al. (2012). Identification of acquired antimicrobial resistance genes. J. Antimicrob. Chemother. 67, 2640–2644. doi: 10.1093/jac/dks261
Zhang, J., Chen, L., Wang, J., Butaye, P., Huang, K., Qiu, H., et al. (2018a). Molecular detection of colistin resistance genes (mcr-1 to mcr-5) in human vaginal swabs. BMC Res. Notes 11:143. doi: 10.1186/s13104-018-3255-3
Zhang, J., Chen, L., Wang, J., Yassin, A. K., Butaye, P., Kelly, P., et al. (2018b). Molecular detection of colistin resistance genes (mcr-1, mcr-2 and mcr-3) in nasal/oropharyngeal and anal/cloacal swabs from pigs and poultry. Sci. Rep. 8:3705. doi: 10.1038/s41598-018-22084-4
Zhang, X. F., Doi, Y., Huang, X., Li, H. Y., Zhong, L. L., Zeng, K. J., et al. (2016). Possible Transmission of mcr-1-Harboring Escherichia coli between Companion Animals and Human. Emerg. Infect. Dis. 22, 1679–1681.
Zhang, X., Zhang, B., Guo, Y., Wang, J., Zhao, P., Liu, J., et al. (2019). Colistin resistance prevalence in Escherichia coli from domestic animals in intensive breeding farms of Jiangsu Province. Int. J. Food Microbiol. 291, 87–90. doi: 10.1016/j.ijfoodmicro.2018.11.013
Zhao, Q., Li, Y., Tian, Y., Shen, Y., Wang, S., and Zhang, Y. (2022). Clinical impact of colistin banning in food animal on mcr-1-positive Enterobacteriaceae in patients from Beijing. China, 2009-2019: A long-term longitudinal observational study. Front. Microbiol. 13:826624. doi: 10.3389/fmicb.2022.826624
Keywords: mobile colistin resistance, mcr-2, Escherichia coli, swine, pathotype, plasmid, IncP, IncX4
Citation: Ewers C, Göpel L, Prenger-Berninghoff E, Semmler T, Kerner K and Bauerfeind R (2022) Occurrence of mcr-1 and mcr-2 colistin resistance genes in porcine Escherichia coli isolates (2010–2020) and genomic characterization of mcr-2-positive E. coli. Front. Microbiol. 13:1076315. doi: 10.3389/fmicb.2022.1076315
Received: 21 October 2022; Accepted: 22 November 2022;
Published: 09 December 2022.
Edited by:
Chang-Wei Lei, Sichuan University, ChinaReviewed by:
Madubuike Umunna Anyanwu, University of Nigeria, NigeriaJan Tkadlec, Charles University, Czechia
Baiyuan Li, Hunan University of Science and Engineering, China
Copyright © 2022 Ewers, Göpel, Prenger-Berninghoff, Semmler, Kerner and Bauerfeind. This is an open-access article distributed under the terms of the Creative Commons Attribution License (CC BY). The use, distribution or reproduction in other forums is permitted, provided the original author(s) and the copyright owner(s) are credited and that the original publication in this journal is cited, in accordance with accepted academic practice. No use, distribution or reproduction is permitted which does not comply with these terms.
*Correspondence: Christa Ewers, Christa.Ewers@vetmed.uni-giessen.de