- 1Institute of Animal Husbandry and Veterinary Medicine, Tibet Academy of Agriculture and Animal Husbandry Sciences, Lhasa, China
- 2The State Key Laboratory of Animal Nutrition, Institute of Animal Sciences, Chinese Academy of Agricultural Sciences, Beijing, China
- 3College of Animal Science, Xinjiang Agricultural University, Urumqi, China
This study aimed to investigate the effects of different feeding patterns on meat quality, gut microbiota and its metabolites of Tibetan pigs. Tibetan pigs with similar body weight were fed the high energy diets (HEP, 20 pigs) and the regular diets (RFP, 20 pigs), and free-ranging Tibetan pigs (FRP, 20 pigs) were selected as the reference. After 6 weeks of experiment, meat quality indexes of semitendinosus muscle (SM) and cecal microbiota were measured. The results of meat quality demonstrated that the shear force of pig SM in FRP group was higher than that in HEP and RFP groups (p < 0.001); the pH-value of SM in HEP pigs was higher at 45 min (p < 0.05) and lower at 24 h (p < 0.01) after slaughter than that in FRP and RFP groups; the SM lightness (L* value) of FRP pigs increased compared with RFP and HEP groups (p < 0.001), while the SM redness (a* value) of FRP pigs was higher than that of RFP group (p < 0.05). The free fatty acid (FA) profile exhibited that the total FAs and unsaturated FAs of pig SM in HEP and RFP groups were higher than those in FRP group (p < 0.05); the RFP pigs had more reasonable FA composition with higher n-3 polyunsaturated FAs (PUFAs) and lower n-6/n-3 PUFA ratio than HEP pigs (p < 0.05). Based on that, we observed that Tibetan pigs fed high energy diets (HEP) had lower microbial α-diversity in cecum (p < 0.05), and distinct feeding patterns exhibited a different microbial cluster. Simultaneously, the short-chain FA levels in cecum of FRP and RFP pigs were higher compared with HEP pigs (p < 0.05). A total of 11 genera related to muscle lipid metabolism or meat quality, including Alistipes, Anaerovibrio, Acetitomaculun, etc., were identified under different feeding patterns (p < 0.05). Spearman correlation analysis demonstrated that alterations of free FAs in SM were affected by the genera Prevotellaceae_NK3B31_group, Prevotellaceae UCG-003 and Christensenellaceae_R-7_group (p < 0.05). Taken together, distinct feeding patterns affected meat quality of Tibetan pigs related to gut microbiota alterations.
Introduction
Tibetan pig is a rare plateau pig species, mostly living in the alpine and cold areas of semi-farming and semi-grazing (Yang et al., 2021). In addition to its developed cardiopulmonary function, strong lipid-settling ability, and ability to endure plateau anoxic environments, it has a high level of cold or stress resistance, delicious meat, and has a lot of fat between the muscles (Fan et al., 2016; Luo et al., 2022; Niu et al., 2022). In recent years, with the increasing demand for ecological, green and high-quality pork products, Tibetan pig breeding industry has attracted widespread attention.
The traditional way of feeding Tibetan pigs is grazing, which mainly relies on grass, fruits, roots and other food sources of grassland and understory, and rarely supplements with refined feed, so the breeding cost is low (Wang et al., 2013). Understory resources are abundant in summer and autumn, and a lot of nutrients can be obtained by Tibetan pigs; however, in winter and spring with cold climate, the food resources are gradually reduced, and the nutrients that can be absorbed are limited, far from meeting the growth needs of pigs (Zhang et al., 2019; Yang et al., 2021). This traditional feeding pattern seriously slows down the growth and development of pigs and reduces the production performance of Tibetan pigs. Changing the traditional breeding way, therefore, adopting the appropriate house feeding pattern is the only way for the development of industrialization of Tibetan pigs. However, whether changing the way of Tibetan pig breeding will change the quality of meat products needs further verification, especially the effects of diet transformation on Tibetan pork quality from extensive farming to fine farming needing to be clarified.
In recent years, with the depth exploration of microbial functions, gut-brain axis, gut-liver axis and other signaling pathways mediated by microorganisms and their metabolites (especially short-chain fatty acids) have been confirmed to be involved in the host’s energy metabolism (Morais et al., 2021; Wang et al., 2021). The microbiota-gut-skeletal muscle axis has also been gradually confirmed, and dietary components (including dietary fiber) can change the gut microbiota to regulate the glucose and lipid metabolism of the host skeletal muscle, resulting in the improvement of their meat quality (Sonnenburg and Backhed, 2016; Chen et al., 2022). Therefore, it is a new idea to regulate skeletal muscle metabolism by altering intestinal microbiota or its metabolites through diet and other factors, ultimately improving muscle production and quality.
Therefore, this study aimed to explore the effects of different feeding patterns on meat quality of Tibetan pigs, and analyze the role of cecal microorganisms and their metabolites short-chain fatty acids (SCFAs), which is expected to provide a theoretical basis for large-scale breeding of Tibetan pigs, and provide a reference for selecting specific feeding patterns according to different market orientation and demand structure to achieve accurate pig breeding.
Materials and methods
Ethics approval
The experimental protocol (Ethics Approval Code: IAS2021-241) was reviewed and approved by the Institutional Animal Care and Use Committee of the Institute of Animal Sciences, Chinese Academy of Agricultural Sciences (Beijing, China).
Animals, experimental design, and sample collection
This experiment was conducted in the research farm (Shannan District, Tibet, China) of Institute of Animal Husbandry and Veterinary Medicine, Tibet Academy of Agriculture and Animal Husbandry Sciences. A total of 40 house-feeding Tibetan pigs [initial body weight (IBW) = 22.4 ± 1.7 kg] with the same genetic background were allotted to 2 pens randomly and each pen was regarded as one dietary treatment. During the 42-day experimental period, Tibetan pigs were fed the regular Tibetan pig diets (RFP group) and the high energy content diets (HEP group; Table 1). All pigs were allowed to free access to feed and water. Diets were formulated to meet or exceed the vitamins and minerals of pigs according to the nutrient requirements of swine (GB/T 39235–2020). At the same time, another 20 free-ranging Tibetan pigs (IBW = 21.3 ± 1.0 kg) were selected and set as the reference (FRP group).
At the end of this experiment (day 42), 7 randomly selected pigs of each treatment were sacrificed by electric stunning and carcass weight (CW) of each pig was recorded. An incubation period of 3 h at room temperature was followed by centrifugation at 3,000 rpm for 10 min after drawing blood from the jugular vein with a sterilized syringe. We aliquoted and stored the serum samples at −80°C for subsequent analysis. The semitendinosus muscle (SM) samples (approximately 1 g) were collected into 2-mL sterile tubes for free fatty acids analysis. Aseptically collected chyme from the cecum was sequenced for microbial 16S rRNA genes and analyzed for SCFA levels. All samples of SM and cecum digesta were immediately frozen in liquid nitrogen and stored at −80°C.
Analysis of serum metabolites
Concentrations of low-density lipoprotein cholesterol (LDL-C, Cat # A113-2-1), alanine aminotransferase (ALT, Cat # C009-3-1), alkaline phosphatase (ALP, Cat # A112-1-1) and high-density lipoprotein cholesterol (HDL-C, Cat # A086-1-1) in serum were measured by commercial assay kits from Nanjing Jiancheng Bioengineering Institute (Nanjing, China).
Measurement of meat quality index
Meat color and pH value of SM samples were directly measured by OPTO-STAR and pH-STAR (Matthäus, Germany) according to the manufacturer’s instructions at 45 min and 24 h postmortem, respectively. The shear force value of SM samples was obtained by the Warner-Bratzler meat shear machine (Salter 235, Manhattan, Kansas, United States) following the procedure described by Lang et al. (2020).
Detection of free fatty acids in meat
Freeze-dried and ground SM samples were used to analyze medium- and long-chain fatty acid contents. Firstly, lipids were extracted from SM samples by the chloroacetyl-methanol (1:10, v/v) procedure. Hexanes were added for methylation at 80°C water incubation for 4 h. Then, the gas chromatography was utilized to detect the profile of free fatty acids in samples by targeted metabolomics according to the description by Tang et al. (2020).
Quantification of short-chain fatty acids
Approximately 1 g of cecal chyme was collected and immersed in 10 mL of ddH2O in 15-mL screw-capped vials, after which it was shaken for 30 min and set aside at 4°C overnight. For the analysis of SCFA concentration, the mixture was centrifuged at 10000 rpm for 10 min. The concentration was determined using gas chromatography according to Tang et al. (2021b).
Microbial 16S rRNA gene sequencing analysis
Each sample contained approximately 0.5–1 g of cecal chyme, from which the manufacturer’s instructions of the E.Z.N.A.® soil DNA Kit (D5625-02, Omega Bio-Tek Inc., Norcross, GA, United States) were followed to extract microbial community genomic DNA. A 1% agarose gel electrophoresis and NanoDrop2000 spectrophotometer (Thermo Fisher Scientific, Waltham, MA, United States) were used separately to determine the purity and DNA concentration. These primers [338F (5’-ACTCCTACGGGAGGCAGCAG-3′) and 806R (5’-GGACTACHVGGGTWTCTAAT-3′)] were used to amplify the V3-V4 regions of the 16S rRNA gene of bacteria. As described by Tang et al. (2021a), the reaction system, measuring amplified fragments, and purification were conducted according to their methods.
We obtained microbial sequence data from Majorbio Bio-Pharm Technology Co. Ltd. (Shanghai, China). Sequences were analyzed and classified into operational taxonomic units (OTUs; 97% identity). Additionally, QIIME (Version 174 1.7.0) generated alpha-diversity coverage based on the Ace, Chao and Sobs index within each sample, and an unweighted UniFrac distance based on Bray-Curtis distance was computed and PCoA was used to visualize beta-diversity. The significant difference between treatments at the phylum and genus level was tested by the Kruskal-Wallis H test (nonparametric test) with corrected p-value (FDR) < 0.05. Unless otherwise noted, the microbial data were analyzed on the Majorbio I-Sanger Cloud Platform.1
Statistical analysis
One-way ANOVA analysis of the data on CW, serum metabolites, meat quality index, free fatty acids in meat, SCFAs in cecal chyme and microbial alpha-diversity (including Ace, Chao and Sobs index) was used to test for differences among 3 groups using the SPSS software (version 23.0, IBM, Armonk, NY, United States). The least significant difference (LSD) as a post-hoc multiple comparison method was used to compare results between every 2 of 3 distinct groups. The correlation matrix between cecal microbes and free fatty acids or SCFAs was generated using Spearman’s correlation coefficient on the Majorbio I-Sanger Cloud Platform. All the above data were drawn using GraphPad 7.0. Lastly, the results were presented as means ± SE with p < 0.05 being regarded as statistically significant.
Results
Carcass weight and serum metabolites
The IBW and CW, as well as HDL-C, LDL-C, ALT and ALP in serum were shown in Figure 1. The CW and LDL-C of HEP pigs were significantly higher than those of the FRP or RFP pigs (p < 0.01), whereas no significant changes in CW and LDL-C were found between FRP and RFP pigs (p > 0.05; Figures 1B,D). The HDL-C of HEP pigs was significantly improved compared with the FRP pigs (p < 0.05), and the HDL-C of RFP pigs had an increased trend compared with the FRP pigs (p = 0.058; Figure 1C). The ALP of HEP pigs was significantly higher compared with the FRP or RFP pigs (p < 0.05), whereas no significant change in ALP was found between FRP and RFP pigs (p > 0.05; Figure 1F). In addition, no significant difference was observed in ALT among three group pigs (p > 0.05; Figure 1E).
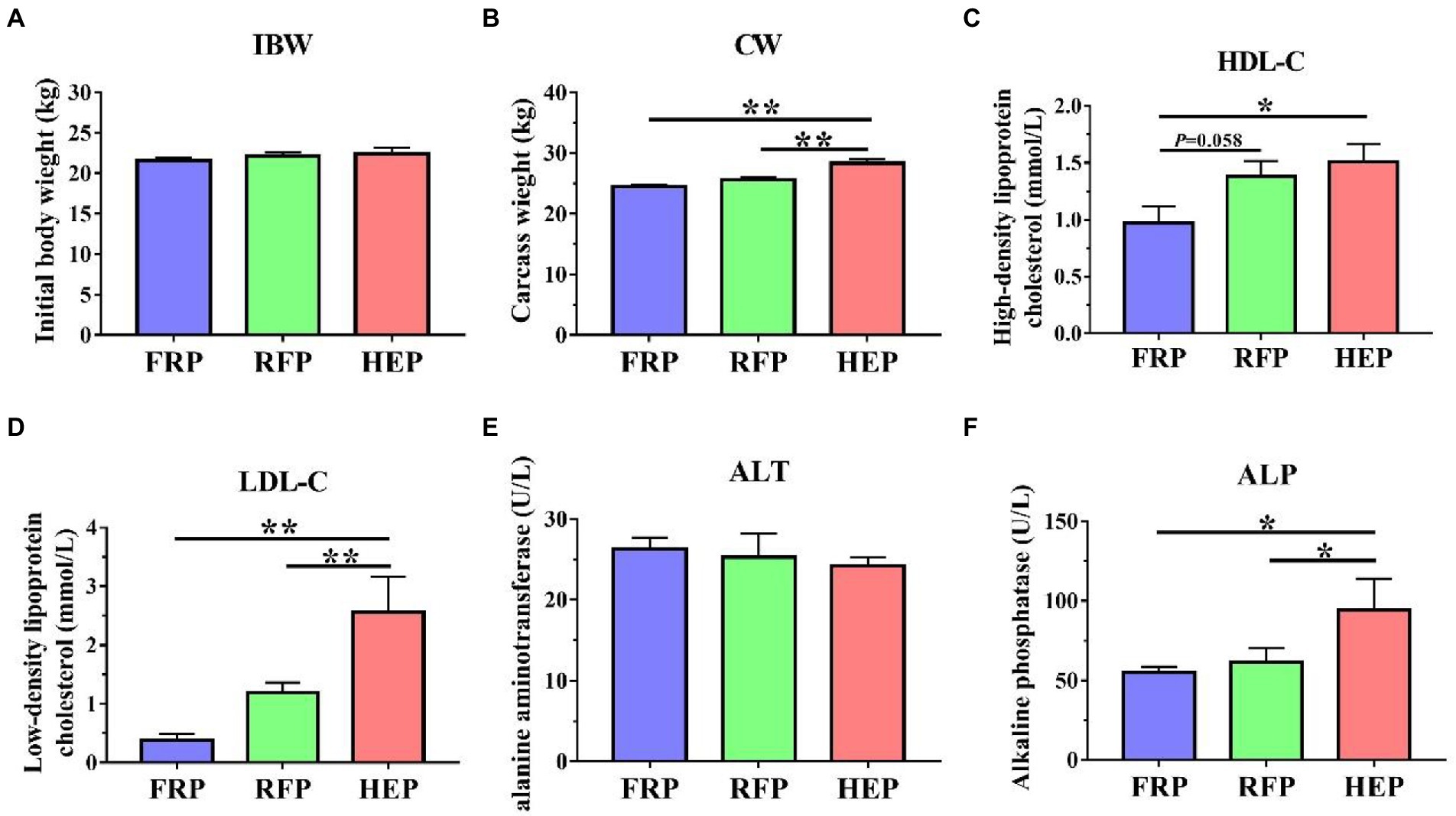
Figure 1. Effects of different feeding patterns on the CW, and HDL-C, LDL-C, ALT ALP in serum of Tibetan pigs. (A) The initial body weight (IBW) of Tibetan pigs. (B) The carcass weight (CW) of Tibetan pigs. (C) The content of high-density lipoprotein cholesterol (HDL-C) in serum of Tibetan pigs. (D) The content of low-density lipoprotein cholesterol (LDL-C) in serum of Tibetan pigs. (E) The activity of alanine aminotransferase (ALT) in serum of Tibetan pigs. (F) The activity of alkaline phosphatase (ALP) in serum of Tibetan pigs. Data are expressed as mean ± SE (n = 6–7). * and ** indicates p < 0.05 and p < 0.01, respectively. FRP: free-ranging Tibetan pigs; RFP: feeding the regular Tibetan pig diets; HEP: feeding the high energy content diets.
Alterations in meat quality
The meat color, pH value, and shear force of SM were shown in Table 2. The L* (45 min), L* (24 h) and shear force of SM in FRP pigs were significantly higher than that in RFP and HEP pigs (p < 0.001), whereas no significant changes in L* (45 min), L* (24 h) and shear force were found between HEP and RFP pigs (p > 0.05). The a* (45 min) and a* (24 h) of RFP pigs were significantly lower compared with FRP pigs (p < 0.05). The b* (45 min) and pH45min of HEP pigs were the highest, being significantly more elevated than those of RFP and FRP pigs (p < 0.05). However, the b* (24 h) and pH24h of HEP pigs were the lowest and were significantly lower than that of the FRP pigs (p < 0.01).
Concentrations of free fatty acids in meat
The concentrations of free fatty acids in SM were shown in Figure 2. The concentrations of saturated fatty acid (STA, p < 0.05; Figure 2A), monounsaturated fatty acid (MUFA, p = 0.081; Figure 2B), total fatty acid (TFA, p = 0.065; Figure 2D) and n-3 polyunsaturated fatty acid (PUFA, p < 0.01; Figure 2F) in SM of RFP pigs were higher compared with FRP pigs, and the n-3 PUFA in RFP pigs was still higher than that in HEP pigs (p < 0.01; Figure 2F). The concentrations of PUFA (p = 0.062, Figure 2C) and n-6 PUFA (p = 0.056, Figure 2E) in HEP pigs had an increased trend compared with the FRP pigs. In addition, the ratio of n-6/n-3 PUFA in HEP pigs was significantly higher compared with the FRP and RFP pigs (p < 0.01), whereas no significant change in the ratio was found between FRP and RFP pigs (p > 0.05; Figure 2G).
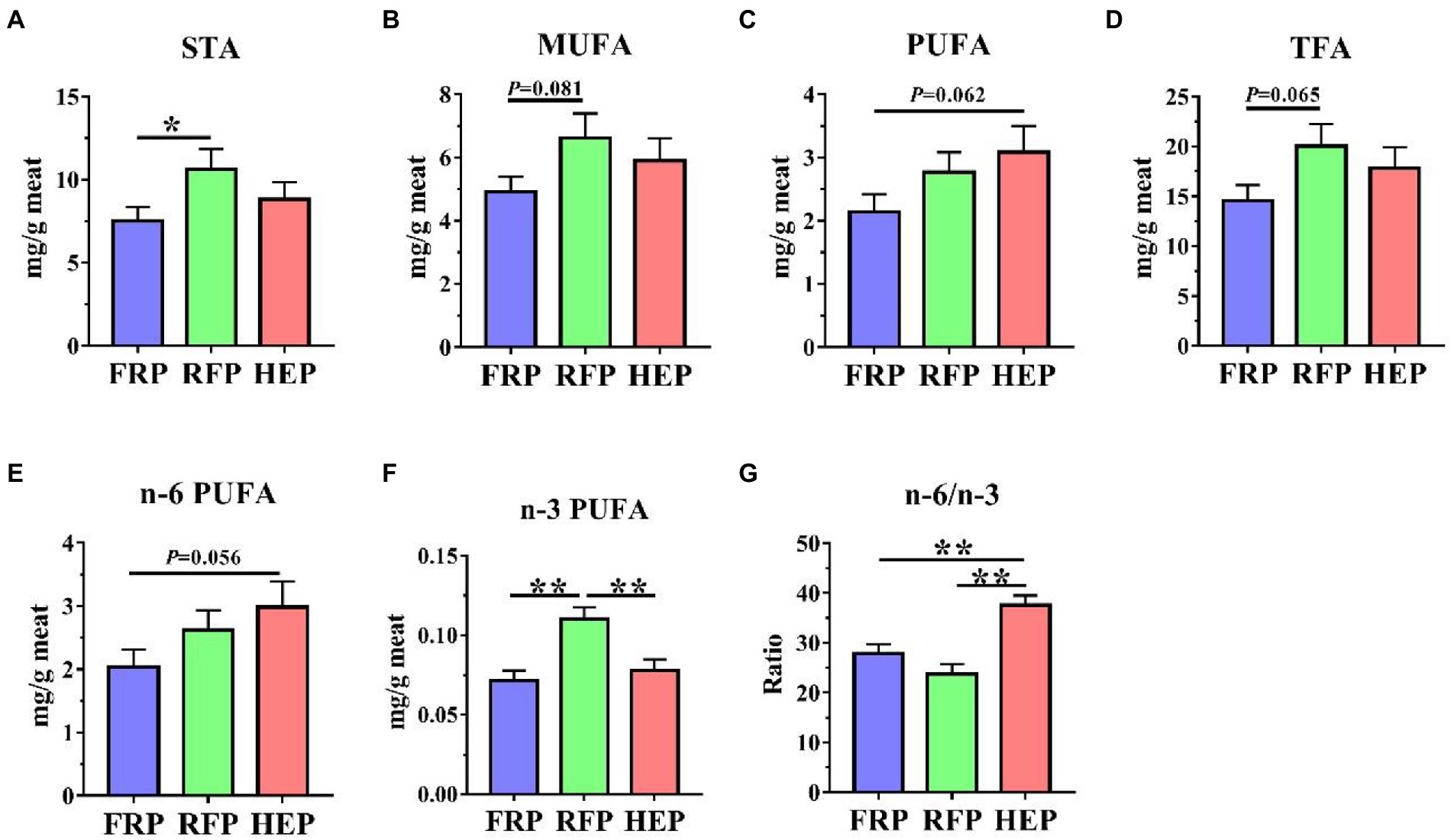
Figure 2. Effects of different feeding patterns on concentrations of fatty acids in semitendinosus muscle (SM) of Tibetan pigs. The concentrations of saturated fatty acid (STA, A), monounsaturated fatty acid (MUFA, B), polyunsaturated fatty acid (PUFA, C), total fatty acid (TFA, D), n-6 PUFA (E), n-3 PUFA (F) and n-6/n-3 PUFA ratio (G) in SM of Tibetan pigs, respectively. Data are expressed as mean ± SE (n = 6–7). * and ** indicates p<0.05 and p<0.01, respectively. FRP: free-ranging Tibetan pigs; RFP: feeding the regular Tibetan pig diets; HEP: feeding the high energy content diets.
As data shown in Table 3, the concentrations of C6:0, C20:2, C20:5 n3 and C22:0 of SM in FRP pigs were higher than that in HEP pigs (p < 0.05). The concentrations of C12:0, C14:0, C16:0, C17:0, C17:1, C18:0, C18:3 n3, C20:0, C20:1 n9, and C20:3 n3 of SM in RFP pigs were significantly higher compared with the FRP pigs (p < 0.05), whereas no significant change in concentrations of C12:0, C14:0, C16:0, C17:0, C17:1, C18:3 n3, and C20:1 n9 were found between HEP and RFP pigs (p > 0.05). The concentrations of C14:1 and C18:2,cis(n6) of SM in FRP pigs were lower compared with the RFP and HEP pigs, and significantly lower than that in the HEP pigs (p < 0.05). In addition, the concentration of C20:4 n6 of RFP pigs was the highest among three group pigs, and significantly higher than that in the HEP pigs (p < 0.05).
Concentrations of short-chain fatty acids in cecal chyme
The concentrations of SCFAs in cecal chyme were shown in Figure 3. The levels of acetic acid and butyric acid in RFP pigs were higher than that in FRP and HEP pigs (p < 0.05), whereas no significant changes of that were found between HEP and FRP pigs (p > 0.05). The levels of propionic acid and total SCFAs in cecal chyme of FRP or RFP pigs were higher compared with HEP pigs (p < 0.01). The concentrations of isobutyrate, isovalerate and valerate in RFP pigs were lower compared with FRP or HEP pigs (p < 0.05).
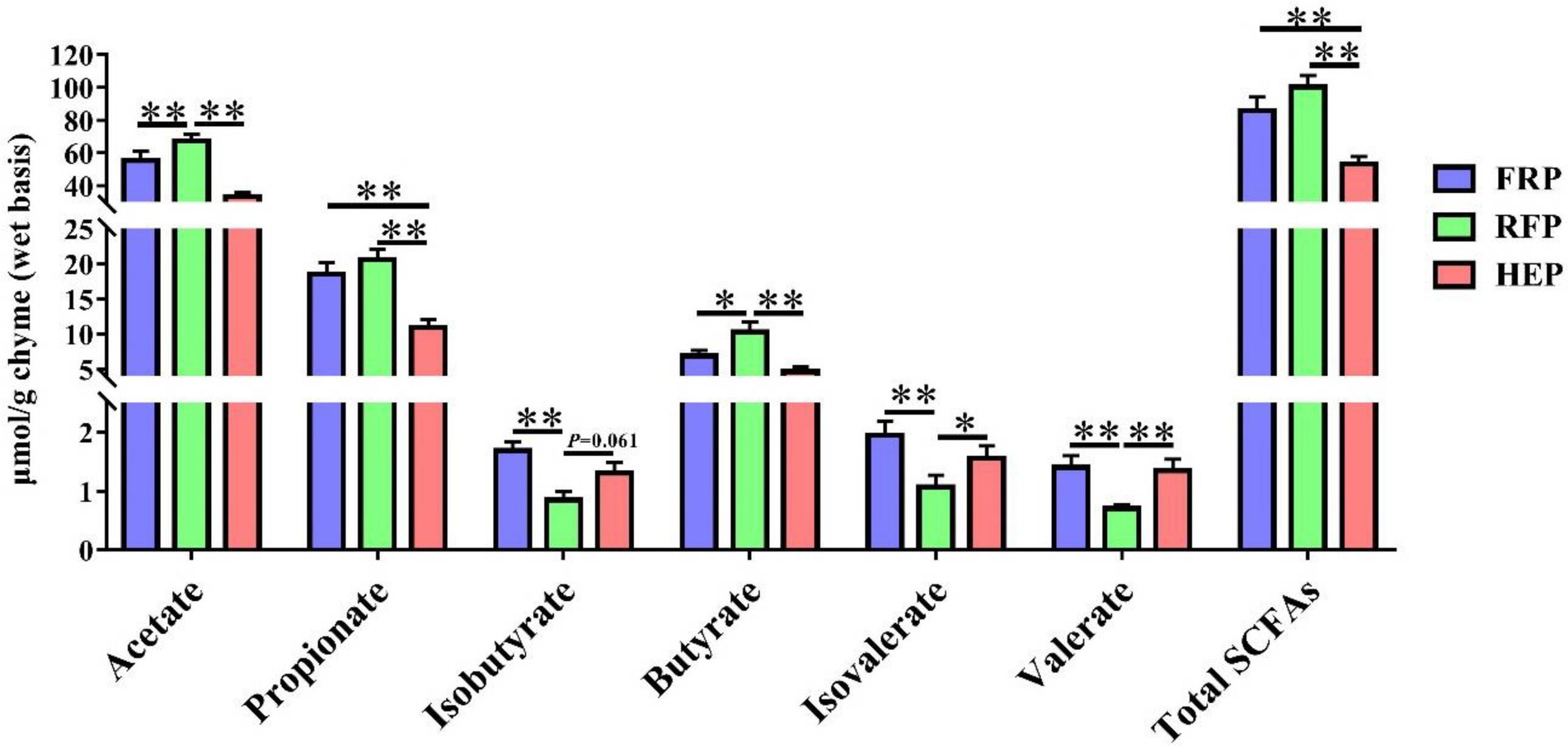
Figure 3. Effects of different feeding patterns on concentrations of short-chain fatty acid (SCFA), including acetate acid, propionic acid, isobutyric acid, butyric acid, isovaleric acid, valeric acid and total SCFAs in cecal chyme of Tibetan pigs. Data are expressed as mean ± SE (n = 6–7). * and ** indicates p < 0.05 and p < 0.01, respectively. FRP: free-ranging Tibetan pigs; RFP: feeding the regular Tibetan pig diets; HEP: feeding the high energy content diets.
Variations in cecal microbes
The fresh cecal chyme was obtained from FRP, RFP and HEP pigs, and 16 s rRNA gene sequencing analysis was performed. The Ace (Figure 4A), Chao (Figure 4B) and Sobs index (Figure 4C) of cecal chyme microbes at OTU level in HEP pigs were significantly lower than that in FRP pigs (p < 0.05), and extremely significantly lower than that in RFP pigs (p < 0.01). The PCoA analysis based on Bray-Curtis distance revealed that beta-diversity shifted due to distinct feeding patterns and notable differences were observed in the cecal chyme at the OTU level (Figure 4D). There were 1103 (208), 1138 (232) and 1009 (214) OTUs (genera) obtained from FRP, RFP and HEP pigs, respectively, of which 778 (181) were common OTUs (genera) among the three different feeding patterns (Figure 4E).
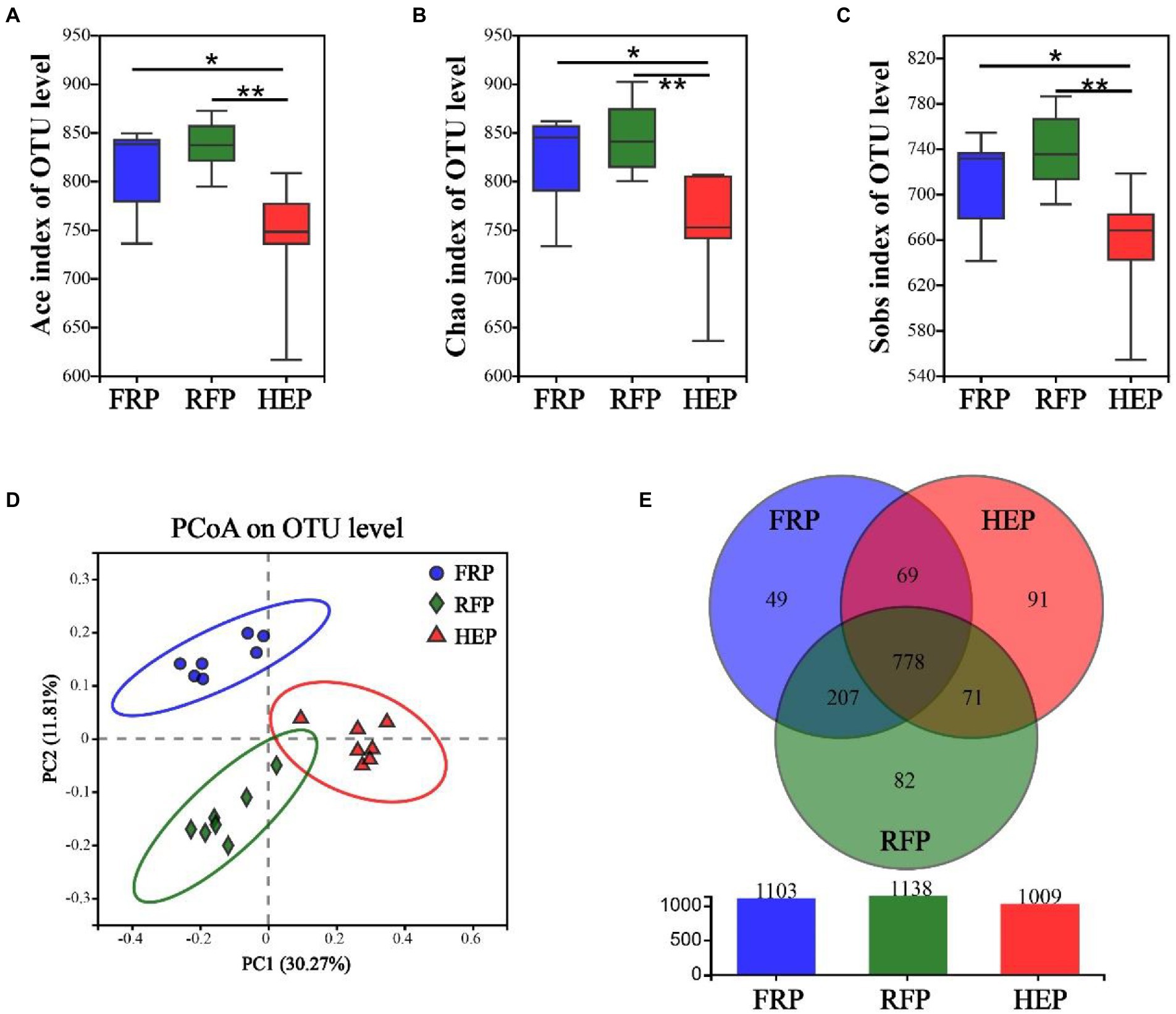
Figure 4. Effects of different feeding patterns on gut microbiota diversity of Tibetan pigs. The Ace (A), Chao (B) and Sobs index (C) of microbiota in cecal chyme. Data are expressed as minimum to maximum (n = 7). * and ** indicates p<0.05 and p<0.01, respectively. (D) PCoA analysis of microbiota in cecal chyme at the OTU level based on the Bray-Curtis distance. (E) The Venn figure of microbiota in cecal chyme. FRP: free-ranging Tibetan pigs; RFP: feeding the regular Tibetan pig diets; HEP: feeding the high energy content diets.
Microbial community composition at the phylum and genus level of the three feeding patterns was presented in Figure 5. The cecal chyme samples comprised five major phyla including Firmicutes, Bacteroidota, Spirochaetota, Actinobacteria and Proteobacteria, and the Firmicutes and Bacteroidetes were the most predominant phyla in the cecal chyme of FRP, RFP and HEP pigs (Figure 5A). In addition, there were also significant differences in the abundance of Firmicutes and Bacteroidota, as well as the ratio of Firmicutes to Bacteroidota among three groups (p < 0.01; Figure 5B). At the genus level, the top three most abundant genera in different feeding patterns, in turn, were norank_f_p-251-o5, Prevotellaceae_UCG-003 and Rikenellaceae_RC9_gut_group (Figures 5C,D). The Prevotellaceae_UCG-003 was extremely significantly enriched in the FRP pigs (p < 0.01); the Parabacteroides, Clostridium_sensu_stricto_1, Clostridium_sensu_stricto_6, and Anaerovibrio were extremely significantly enriched in the RFP pigs (p < 0.01); and Lactobacillus was extremely significantly enriched in the HEP pigs (p < 0.01; Figure 5E).
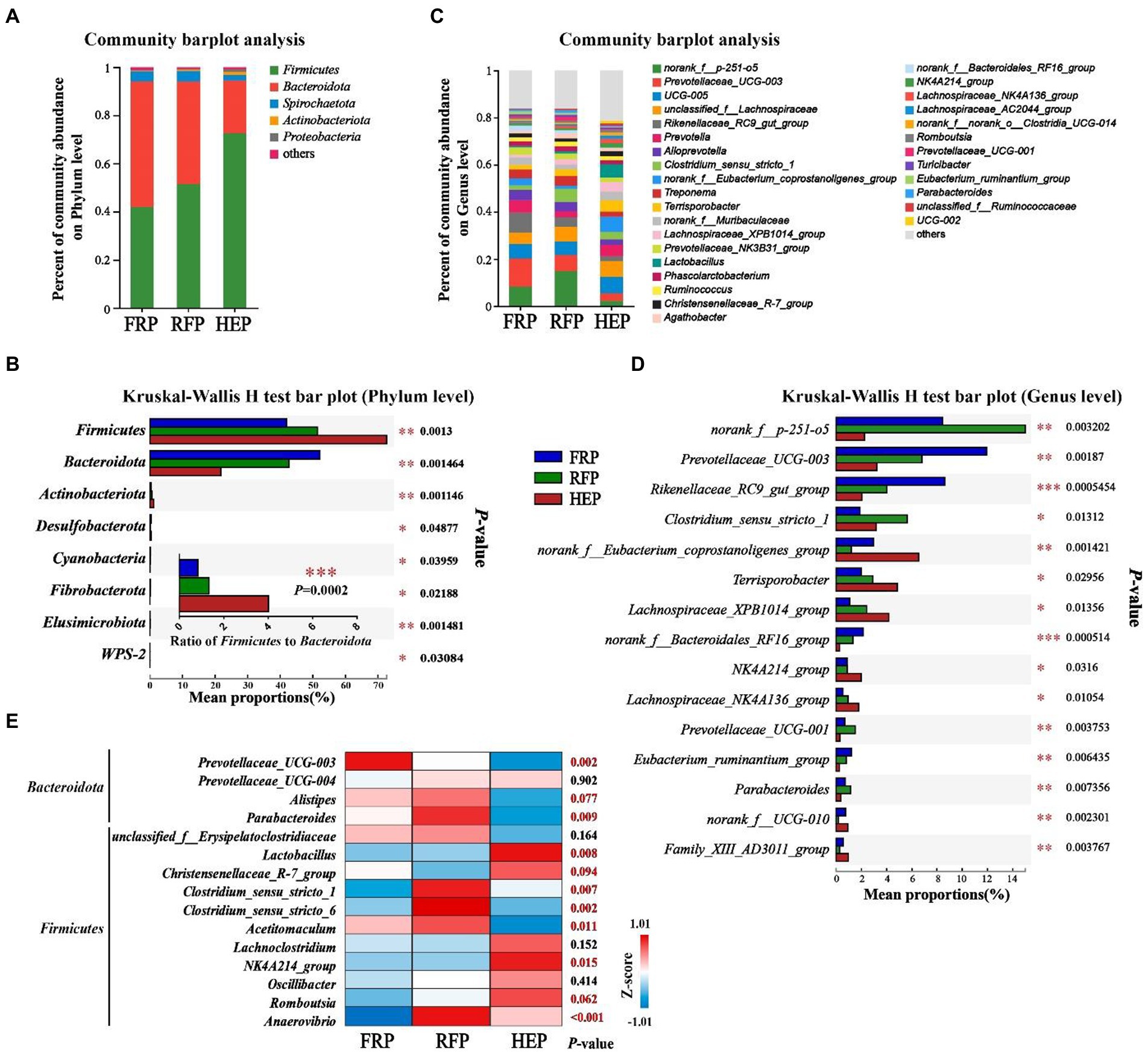
Figure 5. Effects of different feeding patterns on gut microbiota community composition in the cecal chyme of Tibetan pigs. The relative abundances of microbiota in cecal chyme at the phylum (A) or genus level (C). Analysis of variance in the gut microbiota with significant differences at the phylum (B) and genus level (the top 15 genera, D). (E) heat map of the different enrichment of bacteria related to meat quality among three groups. Data are expressed as mean value (n = 7). *, ** and *** indicates p<0.05, p<0.01 and p<0.001, respectively. FRP: free-ranging Tibetan pigs; RFP: feeding the regular Tibetan pig diets; HEP: feeding the high energy content diets.
Correlation between microbiota and free fatty acids in meat or SCFAs in cecal chyme
Spearman’s correlation analysis between microbiota and free fatty acids or SCFAs were shown in Figure 6. The relative abundance of Christensenellaceae_R-7_group was positively associated with the levels of STA, MUFA, PUFA, TFA, n-6 PUFA and n-6/n-3 ratio in SM, except for n-3 PUFA (p < 0.05, Figure 6A). The relative abundance of Terrisporobacter and norank_f_Eubacterium_coprostanoligenes_group were positively associated with the ratio of n-6/n-3 in SM (p < 0.05), whereas the ratio of n-6/n-3 in SM was negatively correlated with the relative abundance of norank_f_bacteroidales_RF16_group and norank_f_p-251-o5 (p < 0.05; Figure 6A). In addition, we found that the concentrations of PUFA and n-6 PUFA in SM were negatively correlated with the relative abundance of Prevotallaceae_UCG-003 (p < 0.05, Figure 6A). The concentrations of valerate and total SCFAs were positively associated with the relative abundance of Rikenellaceae_RC9_gut_group and norank_f_Eubacterium_coprostanoligenes_group, respectively (p < 0.05, Figure 6B). However, the concentration of butyrate was negatively correlated with the relative abundance of norank_f_Eubacterium_coprostanoligenes_group (p < 0.01, Figure 6B).
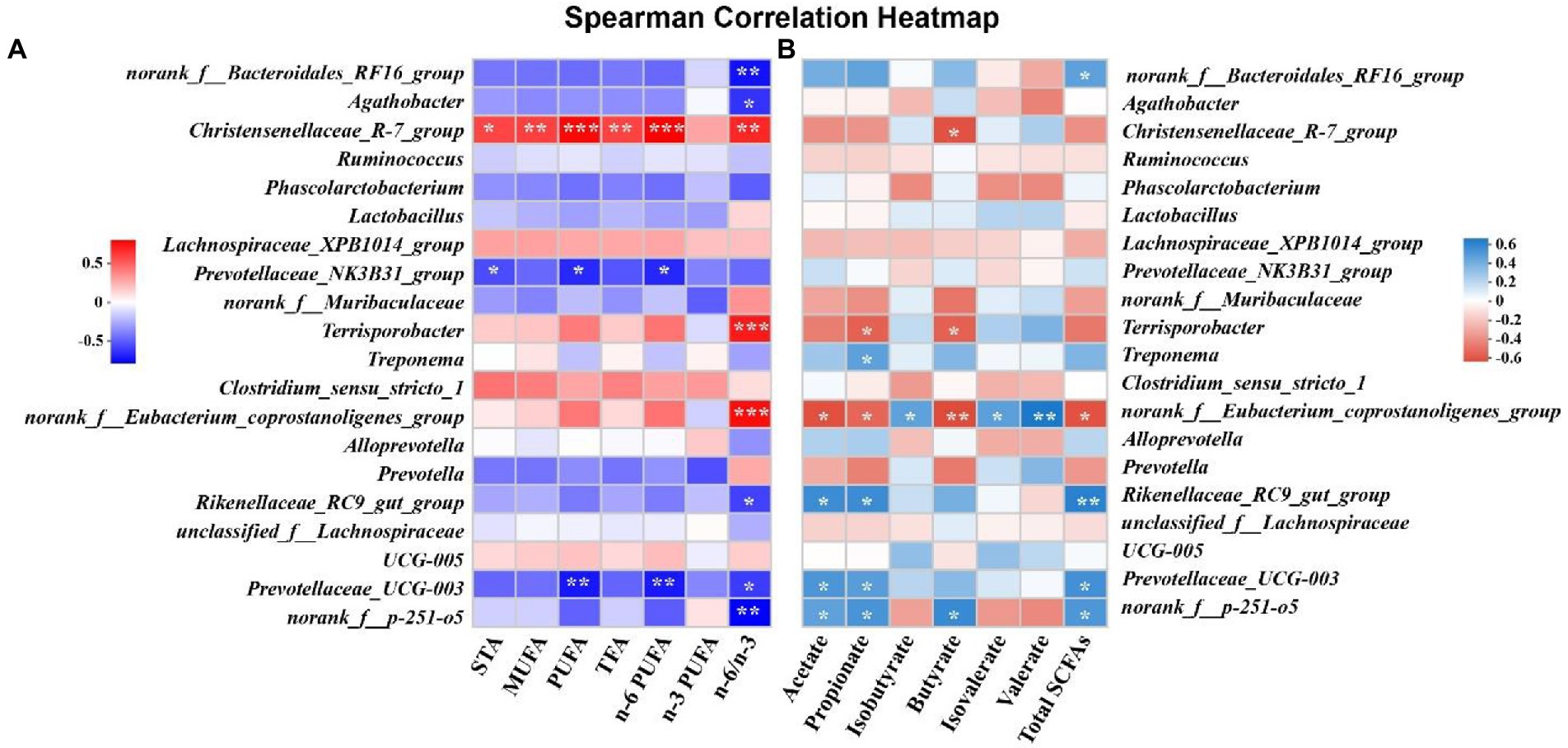
Figure 6. The correlation matrix of cecal genera, free fatty acids and short-chain fatty acids (SCFAs). (A) The correlation between cecal genera and free fatty acids in semitendinosus muscle. (B) The correlation between cecal genera and SCFAs in cecal chyme. *, ** and *** indicates p<0.05, p<0.01 and p<0.001, respectively. STA, saturated fatty acid; MUFA, monounsaturated fatty acid; PUFA, polyunsaturated fatty acid; TFA, total fatty acid.
Discussion
Tibetan pig breeding, not only the focus of the development of agriculture and animal husbandry with local characteristics, but also a crucial industry for people in Tibetan areas to get rid of poverty and become rich, is a vital part of traditional animal husbandry in Tibet. As is known to all, the traditional Tibetan pig breeding method is mainly grazing with a long breeding cycle, which cannot be scientifically managed to maximize the utilization of Tibetan pig resources (Wang et al., 2013; Yang et al., 2021). House feeding can provide full mixed diet for Tibetan pigs without foraging and less exercise, which is conducive to muscle and fat deposition (Zhang et al., 2019). In this study, the carcass weight of Tibetan pigs in HEP group was significantly higher than those in RFP and FRP groups, indicating that the house feeding pattern with whole mixed diet was beneficial to promote the growth of Tibetan pigs and produce more meat, which was consistent with the results of Zhang et al. (2019).
The serum lipid-related metabolites may reflect the overall metabolic state to a certain extent (Tang et al., 2021b). The HDL-C, mainly synthesized in the liver, is an anti-atherogenic lipoprotein that transports cholesterol from extrahepatic tissues to the liver for metabolism and is excreted from the body by bile (Rigotti et al., 2003). By contrast, the LDL-C is a lipoprotein particle that carries cholesterol into peripheral tissue cells (van der Wulp et al., 2013). In this study, it was found that the levels of HDL-C and LDL-C in Tibetan pig varies due to the different energy intake of feeding patterns. The contents of LDL-C and HDL-C in serum of HEP group pigs were the highest, indicating that cholesterol turnover and metabolism in liver were increased. Similar results were also found in broilers with increased dietary energy levels causing elevated serum HDL and LDL levels (Hu et al., 2021). In addition, it was found in this study that the serum ALP level of Tibetan pigs under different feeding patterns was within the normal range (40–160 U/L), but the ALP level in Tibetan pigs fed high energy content diet was significantly higher than that in Tibetan pigs fed regular diet or free-ranging, meaning that the liver metabolic process was significantly enhanced (Siller and Whyte, 2018).
Tibetan pork, as an ecological, green and high-quality pork product, has attracted public attention. Meat quality is a crucial economic trait, which directly affects consumers’ preference for pork, and different meat quality characteristics are applied to different meat processing methods (Modzelewska-Kapitula et al., 2012; Wang et al., 2022a). For example, tender meat is suitable for grilling, while chewy meat is better for braising. This study showed that differences in energy intake levels caused by different feeding patterns did result in alterations in meat quality (including tenderness, pH value, meat color, medium and long-chain fatty acid content). We observed that the shear force of Tibetan pork in the grazing group was significantly higher than those of the other two groups, and the meat color was brighter and redder (L* and a* values). The type and composition of muscle fibers were closely related to meat quality, and the type I myofiber was more enriched in the muscle of grazing Tibetan pigs due to frequent exercise and running (Jackson et al., 2015). The meat color depends on the content of myoglobin in the muscle (Bekhit and Faustman, 2005), and the free-range Tibetan pork was red and bright because of increased type I myofiber, oxidative myofiber, with high content of myoglobin (Ryu and Kim, 2005). The reason why Tibetan pork became dark red with the increase of exposure time under any feeding pattern was that oxymyoglobin (bright red) was oxidized to methemoglobin (brown) (Bekhit and Faustman, 2005). In addition, the researcher believed that the glycolytic myofiber (type IIb) has a faster maturation rate than the oxidative myofiber (type I), so the higher proportion of type I myofiber exhibited a higher shear force in muscle (Seideman, 1986), which provided a reasonable explanation for the alterations of pork tenderness under different feeding pattern in this experiment. Generally, meat with a pH45min ≥ 6 measured after 45 min of slaughter is considered as high-quality meat, and meat with a pH24h > 6 measured after 24 h is considered as dark firm dry meat (Yin, 2011). Hence, from the point of view of pH value, we found high quality of Tibetan pork in any feeding pattern, and the highest pH24h was found in the free-ranging group. Due to the high proportion of type I myofiber with low glycogen content and weak glycolytic ability (Yin, 2011) in free-ranging Tibetan pigs, the lactic acid production was low, finally leading to higher pH24h in muscle. Intriguingly, the pH value of Tibetan pigs in free-ranging group and regular diet feeding group at 45 min was lower than that of Tibetan pigs in high energy group, which might be closely related to dietary fiber content.
Additionally, fatty acids are essential for human health with various physiological functions (Calder, 2015). We observed that the total fatty acids and unsaturated fatty acids in meat of Tibetan pigs fed high energy diets and regular diets were significantly higher than those in free-ranging Tibetan pigs, indicating the crucial roles of energy intake level for the fatty acid content in meat (Wang et al., 2020). It is widely recognized that n-6 PUFAs have potentially negative effects, while n-3 PUFAs [especially eicosapentaenoic acid (EPA) and docosahexaenoic acid (DHA)] have significant positive effects on human health (Jeromson et al., 2015; Sears and Perry, 2015). Meanwhile, researchers have demonstrated that n-3 PUFA exhibited curative effects on bronchial asthma, neuropsychiatric disorders and cognitive brain function in children and can also prevent future cardiovascular events in adults (Ciccone et al., 2013). The fatty acid composition, especially n-3 PUFA, is modulated by altering dietary lipid intake and absorption levels (Poorghasemi et al., 2013). In this study, Tibetan pigs fed regular diets had more reasonable fatty acid composition with higher n-3 PUFAs and lower n-6/n-3 PUFA ratio than high energy intake Tibetan pigs. In addition to n-3 PUFAs, other fatty acids including C18:3n-3 (alpha-linolenic acid, ALA), C20:5n-3 (EPA) and C22:6n-3 (DHA) also have health benefits in preventing brain, retina and cardiovascular diseases (Howe et al., 2006). In this research, the higher content of EPA in free-ranging and regular diets group pork might be closely related to the alterations of gut microbiota caused by fiber intake (Chen et al., 2022). For other unsaturated fatty acids [linoleic acid (LA), ALA and arachidonic acid (ARA)] that are beneficial to human beings, different feeding patterns have their own advantages.
In recent years, the interaction between gut microbiota and muscle has been confirmed by more and more studies, and the importance of its microbiota-gut-muscle axis has been fully recognized (Ticinesi et al., 2019). Gut microbiota can affect skeletal muscle metabolism and muscle fiber phenotype (Lahiri et al., 2019; Nay et al., 2019), and several species of bacteria also have similar effects on regulating skeletal muscle metabolism (Ni et al., 2019; Scheiman et al., 2019). Hence, we analyzed the microbial composition in the cecum of Tibetan pigs, and the results exhibited that the microbial community was obviously divided into three different clusters under different feeding patterns, and the microbial diversity in the cecum of Tibetan pigs fed high energy diets was significantly reduced due to the lack of fiber in the diets (Pu et al., 2022). Besides, microbiota-derived SCFA was affected by dietary fiber intake (Pu et al., 2022), which was consistent with the results that the content of SCFAs (especially acetic acid, propionic acid, and butyric acid) was higher in the cecum of Tibetan pigs in free-ranging and regular diet groups with more fiber intake in this study. The results of Spearman correlation analysis suggested that the genera Prevotellaceae UCG-003, norank_f_Eubacterium_coprostanoligenes_group, Rikenellaceae_RC9_gut_group and norank_f_p-251-o5 were significantly correlated with the production of SCFA in intestinal tract of Tibetan pigs. It has been reported that SCFA infusion from ileum inhibited the expression of fatty acid synthase and acetyl-CoA carboxylase in longissimus dorsi muscle and altered meat quality after antibiotic clearance of endogenous SCFA-produced microbiota in hindgut of pigs (Jiao et al., 2021). Feeding alfalfa and SCFA in goats significantly increased the content of C16:0 and C18:0 in meat (Wang et al., 2022b), which was highly similar to the results of this experiment. Simultaneously, increasing evidence confirmed that gut microbes (for example, Parabacteroides, Unclassified Erysipelotrichaceae, Prevotellaceae UCG-001, Butyrivibrio, Alistipes, Phocaeicola, Acetitomaculun, Corynebacterium, Anaerovibrio, Lachnoclostridium_1) affect lipid deposition and fatty acid content in skeletal muscle by altering lipid metabolism (Chen et al., 2022). A total of 11 genera related to lipid metabolism, including Unclassified_f_Erysipelotrichaceae, Alistipes, Anaerovibrio, Acetitomaculun, etc., were identified under different feeding patterns in this study. Among that, the high content of C18:1 cis-9 and C18:2n-6 trans in Tibetan pork with higher energy intake (REP and HEP group pigs) might be caused by the increased abundance of Anaerovibrio bacteria in intestinal tract (Wang et al., 2019). Besides, the high content of saturated fatty acids in Tibetan pork with higher energy intake was also closely related to the probiotic and antioxidant effects of Lactobacillus (Lactobacillus johnsonii) in intestinal tract (Wang et al., 2017). In this study, Spearman correlation analysis demonstrated that alterations in the content of medium or long-chain fatty acids in meat were affected by the genera Prevotellaceae_NK3B31_group, Prevotellaceae UCG-003 and Christensenellaceae_R-7_group. In addition to the bacteria related to lipid metabolism, this study also found that the bacteria Prevotella and Clostridium related to meat quality (Fang et al., 2017) changed significantly in the cecum of Tibetan pigs under different feeding patterns.
Conclusion
Taken together, our results demonstrated that high energy feeding pattern for house feeding improved carcass weight and enhanced feeding efficiency of Tibetan pigs, yet distinct feeding patterns affected meat quality of Tibetan pigs closely associated with altering gut microbiota, which could provide a reference for choosing specific feeding pattern based on various market orientation and demand to achieve precision breeding.
Data availability statement
The datasets presented in this study can be found in online repositories. The names of the repository/repositories and accession number(s) can be found at: https://www.ncbi.nlm.nih.gov, PRJNA899682.
Ethics statement
The animal study was reviewed and approved by the Institutional Animal Care and Use Committee of the Institute of Animal Sciences, Chinese Academy of Agricultural Sciences (Ethics Approval Code: IAS2021-241).
Author contributions
YZ, CL, ST, and RZ designed the research. YZ, Cy, CL, GS, BS, and JD conducted the experiments. YZ and ST analyzed the data. YZ, RZ, Bw, and ST wrote the paper and revised the manuscript. YZ, TM, RZ, LC, and HZ provided the funding and supervision. All authors contributed to the article and approved the submitted version.
Funding
This study was funded by the Tibet Science and Technology Projects (XZ-2019-NK-NS-003), the National Key Research and Development Program of China (2022YFD1600201), and Agricultural Science and Technology Innovation Program (CAAS-ZDRW202006-02, ASTIPIAS07).
Conflict of interest
The authors declare that the research was conducted in the absence of any commercial or financial relationships that could be construed as a potential conflict of interest.
Publisher’s note
All claims expressed in this article are solely those of the authors and do not necessarily represent those of their affiliated organizations, or those of the publisher, the editors and the reviewers. Any product that may be evaluated in this article, or claim that may be made by its manufacturer, is not guaranteed or endorsed by the publisher.
Footnotes
References
Bekhit, A. E., and Faustman, C. (2005). Metmyoglobin reducing activity. Meat Sci. 71, 407–439. doi: 10.1016/j.meatsci.2005.04.032
Calder, P. C. (2015). Functional roles of fatty acids and their effects on human health. JPEN J. Parenter. Enteral Nutr. 39, 18S–32S. doi: 10.1177/0148607115595980
Chen, B., Li, D., Leng, D., Kui, H., Bai, X., and Wang, T. (2022). Gut microbiota and meat quality. Front. Microbiol. 13:951726. doi: 10.3389/fmicb.2022.951726
Ciccone, M. M., Scicchitano, P., Gesualdo, M., Zito, A., Carbonara, S., Ricci, G., et al. (2013). The role of omega-3 polyunsaturated fatty acids supplementation in childhood: a review. Recent Pat. Cardiovasc. Drug Discov. 8, 42–55. doi: 10.2174/1574890111308010006
Fan, B., Zhang, H., Bai, J., Liu, X., Li, Y., Wang, X., et al. (2016). Pathogenesis of highly pathogenic porcine reproductive and respiratory syndrome virus in Chinese Tibetan swine. Res. Vet. Sci. 108, 33–37. doi: 10.1016/j.rvsc.2016.07.012
Fang, S., Xiong, X., Su, Y., Huang, L., and Chen, C. (2017). 16S rRNA gene-based association study identified microbial taxa associated with pork intramuscular fat content in feces and cecum lumen. BMC Microbiol. 17:162. doi: 10.1186/s12866-017-1055-x
Howe, P., Meyer, B., Record, S., and Baghurst, K. (2006). Dietary intake of long-chain omega-3 polyunsaturated fatty acids: contribution of meat sources. Nutrition 22, 47–53. doi: 10.1016/j.nut.2005.05.009
Hu, X., Li, X., Xiao, C., Kong, L., Zhu, Q., and Song, Z. (2021). Effects of dietary energy level on performance, plasma parameters, and central AMPK levels in stressed broilers. Front Vet Sci. 8:681858. doi: 10.3389/fvets.2021.681858
Jackson, J. R., Kirby, T. J., Fry, C. S., Cooper, R. L., McCarthy, J. J., Peterson, C. A., et al. (2015). Reduced voluntary running performance is associated with impaired coordination as a result of muscle satellite cell depletion in adult mice. Skelet. Muscle 5:41. doi: 10.1186/s13395-015-0065-3
Jeromson, S., Gallagher, I. J., Galloway, S. D., and Hamilton, D. L. (2015). Omega-3 fatty acids and skeletal muscle health. Mar. Drugs 13, 6977–7004. doi: 10.3390/md13116977
Jiao, A., Diao, H., Yu, B., He, J., Yu, J., Zheng, P., et al. (2021). Infusion of short chain fatty acids in the ileum improves the carcass traits, meat quality and lipid metabolism of growing pigs. Anim Nutr. 7, 94–100. doi: 10.1016/j.aninu.2020.05.009
Lahiri, S., Kim, H., Garcia-Perez, I., Reza, M. M., Martin, K. A., Kundu, P., et al. (2019). The gut microbiota influences skeletal muscle mass and function in mice. Sci. Transl. Med. 11:662. doi: 10.1126/scitranslmed.aan5662
Lang, Y., Zhang, S., Xie, P., Yang, X., Sun, B., and Yang, H. (2020). Muscle fiber characteristics and postmortem quality of longissimus thoracis, psoas major and semitendinosus from Chinese Simmental bulls. Food Sci. Nutr. 8, 6083–6094. doi: 10.1002/fsn3.1898
Luo, C., Sun, G., Duan, J., Han, H., Zhong, R., Chen, L., et al. (2022). Effects of high-altitude hypoxic environment on colonic inflammation, intestinal barrier and gut microbiota in three-way crossbred commercial pigs. Front. Microbiol. 13:521. doi: 10.3389/fmicb.2022.968521
Modzelewska-Kapitula, M., Dabrowska, E., Jankowska, B., Kwiatkowska, A., and Cierach, M. (2012). The effect of muscle, cooking method and final internal temperature on quality parameters of beef roast. Meat Sci. 91, 195–202. doi: 10.1016/j.meatsci.2012.01.021
Morais, L. H., Schreiber, H. L. T., and Mazmanian, S. K. (2021). The gut microbiota-brain axis in behaviour and brain disorders. Nat. Rev. Microbiol. 19, 241–255. doi: 10.1038/s41579-020-00460-0
Nay, K., Jollet, M., Goustard, B., Baati, N., Vernus, B., Pontones, M., et al. (2019). Gut bacteria are critical for optimal muscle function: a potential link with glucose homeostasis. Am. J. Physiol. Endocrinol. Metab. 317, E158–E171. doi: 10.1152/ajpendo.00521.2018
Ni, Y., Yang, X., Zheng, L., Wang, Z., Wu, L., Jiang, J., et al. (2019). Lactobacillus and Bifidobacterium improves physiological function and cognitive ability in aged mice by the regulation of gut microbiota. Mol. Nutr. Food Res. 63:e1900603. doi: 10.1002/mnfr.201900603
Niu, H., Feng, X. Z., Shi, C. W., Zhang, D., Chen, H. L., Huang, H. B., et al. (2022). Gut bacterial composition and functional potential of Tibetan pigs under semi-grazing. Front. Microbiol. 13:850687. doi: 10.3389/fmicb.2022.850687
Poorghasemi, M., Seidavi, A., Qotbi, A. A., Laudadio, V., and Tufarelli, V. (2013). Influence of dietary fat source on growth performance responses and carcass traits of broiler chicks. Asian-Australas J Anim Sci. 26, 705–710. doi: 10.5713/ajas.2012.12633
Pu, G., Hou, L., Du, T., Wang, B., Liu, H., Li, K., et al. (2022). Effects of short-term feeding with high fiber diets on growth, utilization of dietary fiber, and microbiota in pigs. Front. Microbiol. 13:963917. doi: 10.3389/fmicb.2022.963917
Rigotti, A., Miettinen, H. E., and Krieger, M. (2003). The role of the high-density lipoprotein receptor SR-BI in the lipid metabolism of endocrine and other tissues. Endocr. Rev. 24, 357–387. doi: 10.1210/er.2001-0037
Ryu, Y. C., and Kim, B. C. (2005). The relationship between muscle fiber characteristics, postmortem metabolic rate, and meat quality of pig longissimus dorsi muscle. Meat Sci. 71, 351–357. doi: 10.1016/j.meatsci.2005.04.015
Scheiman, J., Luber, J. M., Chavkin, T. A., MacDonald, T., Tung, A., Pham, L. D., et al. (2019). Meta-omics analysis of elite athletes identifies a performance-enhancing microbe that functions via lactate metabolism. Nat. Med. 25, 1104–1109. doi: 10.1038/s41591-019-0485-4
Sears, B., and Perry, M. (2015). The role of fatty acids in insulin resistance. Lipids Health Dis. 14:121. doi: 10.1186/s12944-015-0123-1
Seideman, S. C. (1986). Methods of expressing collagen characteristics and their relationship to meat tenderness and muscle fiber types. J. Food Sci. 51, 273–276. doi: 10.1111/j.1365-2621.1986.tb11107.x
Siller, A. F., and Whyte, M. P. (2018). Alkaline phosphatase: discovery and naming of our favorite enzyme. J. Bone Miner. Res. 33, 362–364. doi: 10.1002/jbmr.3225
Sonnenburg, J. L., and Backhed, F. (2016). Diet-microbiota interactions as moderators of human metabolism. Nature 535, 56–64. doi: 10.1038/nature18846
Tang, S., Xie, J., Wu, W., Yi, B., Liu, L., and Zhang, H. (2020). High ammonia exposure regulates lipid metabolism in the pig skeletal muscle via mTOR pathway. Sci. Total Environ. 740:139917. doi: 10.1016/j.scitotenv.2020.139917
Tang, S., Zhang, S., Zhong, R., Su, D., Xia, B., Liu, L., et al. (2021a). Time-course alterations of gut microbiota and short-chain fatty acids after short-term lincomycin exposure in young swine. Appl. Microbiol. Biotechnol. 105, 8441–8456. doi: 10.1007/s00253-021-11627-x
Tang, S., Zhong, R., Yin, C., Su, D., Xie, J., Chen, L., et al. (2021b). Exposure to high aerial ammonia causes hindgut dysbiotic microbiota and alterations of microbiota-derived metabolites in growing pigs. Front. Nutr. 8:689818. doi: 10.3389/fnut.2021.689818
Ticinesi, A., Lauretani, F., Tana, C., Nouvenne, A., Ridolo, E., and Meschi, T. (2019). Exercise and immune system as modulators of intestinal microbiome: implications for the gut-muscle axis hypothesis. Exerc. Immunol. Rev. 25, 84–95.
van der Wulp, M. Y., Verkade, H. J., and Groen, A. K. (2013). Regulation of cholesterol homeostasis. Mol. Cell. Endocrinol. 368, 1–16. doi: 10.1016/j.mce.2012.06.007
Wang, H., He, Y., Li, H., Wu, F., Qiu, Q., Niu, W., et al. (2019). Rumen fermentation, intramuscular fat fatty acid profiles and related rumen bacterial populations of Holstein bulls fed diets with different energy levels. Appl. Microbiol. Biotechnol. 103, 4931–4942. doi: 10.1007/s00253-019-09839-3
Wang, Y., Li, T., Chen, X., Liu, C., Jin, X., Tan, H., et al. (2022b). Preliminary investigation of mixed orchard hays on the meat quality, fatty acid profile, and gastrointestinal microbiota in goat kids. Animals (Basel). 12:780. doi: 10.3390/ani12060780
Wang, S., Li, J., Wang, H., Han, S., Guo, C., and Wang, Y. (2013). A review on Tibetan swine(a)-carcass, meat quality, basic nutrition component, amino acids, fatty acids, inosine monophosphate and muscle fiber. Agric Sci & Technol. 14: 1369–1374, 1391. doi: 10.16175/j.cnki.1009-4229.2013.10.030
Wang, H., Ni, X., Liu, L., Zeng, D., Lai, J., Qing, X., et al. (2017). Controlling of growth performance, lipid deposits and fatty acid composition of chicken meat through a probiotic, lactobacillus johnsonii during subclinical Clostridium perfringens infection. Lipids Health Dis. 16:38. doi: 10.1186/s12944-017-0408-7
Wang, B., Shen, C., Cai, Y., Liu, D., and Gai, S. (2022a). The purchase willingness of consumers for red meat in China. Meat Sci. 192:108908. doi: 10.1016/j.meatsci.2022.108908
Wang, R., Tang, R., Li, B., Ma, X., Schnabl, B., and Tilg, H. (2021). Gut microbiome, liver immunology, and liver diseases. Cell. Mol. Immunol. 18, 4–17. doi: 10.1038/s41423-020-00592-6
Wang, Y., Wang, Q., Dai, C., Li, J., Huang, P., Li, Y., et al. (2020). Effects of dietary energy on growth performance, carcass characteristics, serum biochemical index, and meat quality of female Hu lambs. Anim Nutr. 6, 499–506. doi: 10.1016/j.aninu.2020.05.008
Yang, L., Wang, G., Zhou, J., Yang, Y., Pan, H., Zeng, X., et al. (2021). Exploration of the potential for efficient fiber degradation by intestinal microorganisms in Diqing Tibetan pigs. Fermentation. 7:275. doi: 10.3390/fermentation7040275
Keywords: Tibetan pigs, meat quality, intestinal microbiota, feeding patterns, long-chain fatty acid
Citation: Zhu Y, Cidan-yangji, Sun G, Luo C, Duan J, Shi B, Ma T, Tang S, Zhong R, Chen L, Basang-wangdui and Zhang H (2022) Different feeding patterns affect meat quality of Tibetan pigs associated with intestinal microbiota alterations. Front. Microbiol. 13:1076123. doi: 10.3389/fmicb.2022.1076123
Edited by:
Jinxuan Cao, Beijing Technology and Business University, ChinaReviewed by:
Lv Zengpeng, China Agricultural University, ChinaKai Zhang, Qingdao Agricultural University, China
Copyright © 2022 Zhu, Cidan-yangji, Sun, Luo, Duan, Shi, Ma, Tang, Zhong, Chen, Basang-wangdui and Zhang. This is an open-access article distributed under the terms of the Creative Commons Attribution License (CC BY). The use, distribution or reproduction in other forums is permitted, provided the original author(s) and the copyright owner(s) are credited and that the original publication in this journal is cited, in accordance with accepted academic practice. No use, distribution or reproduction is permitted which does not comply with these terms.
*Correspondence: Shanlong Tang, dGFuZ3NoYW5sb25nMDFAMTI2LmNvbQ==; Basang-wangdui, YncwODkxQDE2My5jb20=