- 1Research Chair in Meat Safety, Department of Pathology and Microbiology, Faculté de Médecine Vétérinaire, Université de Montréal, Saint-Hyacinthe, QC, Canada
- 2Swine and Poultry Infectious Diseases Research Center (CRIPA), Department of Pathology and Microbiology, Faculté de Médecine Vétérinaire, Université de Montréal, Saint-Hyacinthe, QC, Canada
- 3Groupe de Recherche sur les Maladies Infectieuses en Production Animale (GREMIP), Department of Pathology and Microbiology, Faculté de Médecine Vétérinaire, Université de Montréal, Saint-Hyacinthe, QC, Canada
- 4Chaire Agroalimentaire du Conservatoire National des Arts et Métiers, Paris, France
Campylobacter jejuni is the most prevalent bacterial foodborne pathogen in humans. Given the wide genetic diversity of C. jejuni strains found in poultry production, a better understanding of the relationships between these strains within chickens could lead to better control of this pathogen on farms. In this study, 14-day old broiler chickens were inoculated with two C. jejuni strains (103 or 107 CFU of D2008b and 103 CFU of G2008b, alone or together) that were previously characterized in vitro and that showed an opposite potential to compete for gut colonization in broilers. Liver samples and ileal and cecal contents were collected and used to count total C. jejuni and to quantify the presence of each strain using a strain specific qPCR or PCR approach. Ileal tissue samples were also collected to analyze the relative expression level of tight junction proteins. While a 103 CFU inoculum of D2008b alone was not sufficient to induce intestinal colonization, this strain benefited from the G2008b colonization for its establishment in the gut and its extraintestinal spread. When the inoculum of D2008b was increased to 107 CFU – leading to its intestinal and hepatic colonization – a dominance of G2008b was measured in the gut and D2008b was found earlier in the liver for birds inoculated by both strains. In addition, a transcript level decrease of JAM2, CLDN5 and CLDN10 at 7 dpi and a transcript level increase of ZO1, JAM2, OCLN, CLDN10 were observed at 21 dpi for groups of birds having livers contaminated by C. jejuni. These discoveries suggest that C. jejuni would alter the intestinal barrier function probably to facilitate the hepatic dissemination. By in vitro co-culture assay, a growth arrest of D2008b was observed in the presence of G2008b after 48 h of culture. Based on these results, commensalism and competition seem to occur between both C. jejuni strains, and the dynamics of C. jejuni intestinal colonization and liver spread in broilers appear to be strain dependent. Further in vivo experimentations should be conducted to elucidate the mechanisms of commensalism and competition between strains in order to develop adequate on-farm control strategies.
1. Introduction
Campylobacter jejuni is responsible for approximatively 90% of campylobacteriosis cases in humans (Kaakoush et al., 2015). Campylobacteriosis is a severe but self-limiting gastro-enteritis with an infectious dose as low as 500 bacteria (Robinson, 1981; Black et al., 1988) that lasts an average of 6 days. In some cases, it can lead to severe conditions such as the Guillain-Barré syndrome, Miller Fisher syndrome, reactive arthritis, irritable bowel syndrome, and celiac disease (Facciolà et al., 2017). In Canada, there was an annual incidence rate of 29.14 per 100,000 population of campylobacteriosis in 2018 (Public Health Agency of Canada, 2021), while in Europe, there were 220,682 human cases attributed to this pathogen in 2019, that was reported as the first cause of notified zoonosis (European Food Safety Authority and European Centre for Disease Prevention and Control, 2021). The principal sources of human contamination by C. jejuni are poultry, dairy products like unpasteurized milk, and water (Domingues et al., 2012). Poultry products are recognized as the primary source of exposure for humans, mainly due to the high C. jejuni loads that can be found in the intestines of poultry carriers (up to 109 CFU/g of intestinal content; Greig and Ravel, 2009). Humans can be infected by the consumption of contaminated or cross-contaminated undercooked chicken products (Nadeau et al., 2002). The majority of campylobacteriosis cases in humans are sporadic, except for virulent Campylobacter species that would appear to be associated with diffuse outbreaks (Llarena et al., 2016). Some outbreaks are reported in the literature after the consumption of undercooked chicken meat (Yu et al., 2010; Llarena and Kivistö, 2020), chicken liver pâté (Little et al., 2010; Moffatt et al., 2016; Lanier et al., 2018), and unpasteurized milk (Centers for Disease Control and Prevention (CDC), 2002; Burakoff et al., 2018).
According to the season and country, up to 95% of broiler chicken flocks can be contaminated with C. jejuni by 2 weeks of age (Stern et al., 2001; Newell and Fearnley, 2003; van Gerwe et al., 2009). Absence of intestinal colonization by C. jejuni before 2 weeks of age could be partially due to the presence of maternal antibodies in younger birds (Sahin et al., 2003) and/or to intestinal microbiota properties (Laisney et al., 2004; Han et al., 2016). In birds, the infection occurs via the fecal-oral route, leading to a rapid spread of C. jejuni in the flock (Sahin et al., 2015). Once ingested, C. jejuni migrates to the chicken intestine and especially to the caeca; this migration is most probably promoted by colonization factors related to chemotaxis and motility (Hermans et al., 2011). After colonizing the gut, the bacterium can disseminate to internal organs such as the liver through the bloodstream (Cox et al., 2005, 2007; Richardson et al., 2011). According to the literature, an increase of the paracellular permeability, associated with alterations of the expression of tight junctions proteins could explain this phenomenon (Lamb-Rosteski et al., 2008; von Buchholz et al., 2022). At the slaughterhouse, sources of contamination are numerous: contaminated feathers, gut leakage caused by the defeathering process, evisceration, and finally cross-contamination via direct contact with various contaminated surfaces within the production environment (Newell et al., 2001). Moreover, C. jejuni extraintestinal spread may present an additional challenge as C. jejuni was detected inside of retail chicken livers, with a prevalence ranging from 10 to 100% and with bacterial loads varying from 10 to 105 CFU/g of liver (Barot et al., 1983; Whyte et al., 2006; Noormohamed and Fakhr, 2012; Harrison et al., 2013; Berrang et al., 2018).
Usually, C. jejuni colonization is asymptomatic in chickens, which explains why this microorganism is often considered by some authors to be a commensal or commensal-like bacterium of the chicken gut. A few studies have observed in experimental settings that particular strains of C. jejuni have caused mild clinical signs in broiler chickens, mainly transient diarrhea, arthritis, and reduction in body weight gain (Ruiz-Palacios et al., 1981; Dhillon et al., 2006; Gharib Naseri et al., 2012; Awad et al., 2015; Alpigiani et al., 2017). Interestingly, one study isolated C. jejuni from the liver of broiler chickens affected with avian vibrionic hepatitis, but a causative link remained unclear (Jennings et al., 2011).
The wide genetic diversity of C. jejuni strains in poultry production has been revealed using molecular biology techniques (Wassenaar and Newell, 2000). Most of the studies have shown that multiple C. jejuni genotypes could be simultaneously isolated from the cecal content or feces of broiler chickens from the same flock (Thomas et al., 1997; Hiett et al., 2002; Messens et al., 2009), suggesting that birds can be colonized with different C. jejuni strains on the same farm. Among these multiple genotypes, the dominance of one or sometimes two C. jejuni strains in the cecal content or feces of broiler chickens within the same flock is noted most of the time, from the time of contamination until slaughter (Newell et al., 2001; Nadeau et al., 2002; Ring et al., 2005; Ellerbroek et al., 2010), and this has been reported to be due to the different abilities of these strains to colonize broiler chickens (Ringoir and Korolik, 2003; Chaloner et al., 2014; Pielsticker et al., 2016). Thus, when a multi-strain colonization occurs, the involved C. jejuni strains compete and some may become predominant, displacing the others, as it has been confirmed experimentally (Korolik et al., 1998; Konkel et al., 2007; Coward et al., 2008; Calderón-Gómez et al., 2009). In a previous study aimed at better understanding these different abilities to colonize the chicken gut, C. jejuni strains isolated from the caeca of broiler chickens were characterized in vitro and classified according to their autoagglutination, chemotaxis, adhesion, and invasion properties known to contribute to an effective gut colonization. It was also found that these strains showing different phenotypes possess different abilities to compete for gut colonization in birds (Thibodeau et al., 2015a).
As for extraintestinal spread, one or more genotypes of C. jejuni have been found inside retail chicken livers but only one genotype seemed to be dominant (Berrang et al., 2018, 2019). Experimentally, the strains’ ability to colonize the chicken intestine and to spread to organs, including the liver, was determined using a model where birds were inoculated with a dominant C. jejuni isolate (Jennings et al., 2011; Firlieyanti et al., 2016; Pielsticker et al., 2016). Despite successful intestinal colonization, hepatic spread was highly variable. Using animal experimentations, other authors also demonstrated that C. jejuni strains from human and avian origin would have different abilities to spread to the liver in broiler chickens (Chaloner et al., 2014; Pielsticker et al., 2016). Finally, in a recent study conducted at the slaughterhouse, the authors isolated a dominant strain of C. jejuni from the cecal content of broilers that was different from the one isolated from the inside of the liver of the same bird (Berrang et al., 2019). Thus, the extra-intestinal C. jejuni spread to the liver appears to also be strain specific and would involve other unknown factors and mechanisms that appear to be different from those governing intestinal colonization.
These new insights into the relationship between some C. jejuni strains inside the chicken intestinal environment and liver is therefore raising concerns that the presence of the pathogen in birds might impact animal and public health. However, how intestinal colonization and extraintestinal spread are linked or related, especially in the context of a multi-C. jejuni strain colonization, remains unclear.
The aim of this study was therefore to further analyze the intestinal co-colonization of two thoroughly characterized C. jejuni strains with an opposite potential to compete for gut colonization in broiler chickens. Using different ratios of these strains, we investigated the impact of this multi-strain inoculation on each strain, on cecal and ileal colonization of birds, on the extra-intestinal spread of each strain to the liver and on the expression of tight junction proteins from ileal tissue. We also explored the underlying mechanisms of the intestinal competition between the same two C. jejuni strains by in vitro co-culture assays.
2. Materials and methods
2.1. Campylobacter jejuni strain selection and culture conditions
Two C. jejuni strains, isolated from the caeca of commercial broiler chickens, were used in the current study. These strains, identified as G2008b and D2008b, have different comparative genetic fingerprinting profiles. These strains were previously characterized in vitro in our laboratory and identified as strong competitor (G2008b) and weak competitor (D2008b; Thibodeau et al., 2015a). For the inoculation of the birds in the in vivo trial, C. jejuni strains were grown on tryptic soy blood agar plates (Fisher Scientific, Ottawa, ON, Canada) for 24 h at 42°C in jars (2.5 l) under microaerobic conditions (80% N2, 10% CO2, 5% H2, and 5% O2) using the gas pack CampyGen system (Oxoid, Ottawa, ON, Canada) before being suspended in sterile tryptone salt solution (0.1% tryptone (w/v) and 0.85% NaCl (w/v), Fisher Scientific). An absorbance of 1.0 at 600 nm corresponding to about 109 CFU/ml, was measured and bacterial suspensions were diluted to obtain the desired concentration. After oral gavage, inoculate were plated on tryptic soy blood agar plates to verify the correct dose.
The co-culture assays were realized after the animal phase in an attempt to confirm some observation made in vivo. For co-culture assays, both C. jejuni strains were grown on blood agar plates as described above. Bacteria were suspended separately in a sufficient volume of sterile tryptone salt solution to obtain an absorbance of 1.0 measured at 600 nm using a Novaspec II Spectrophotometer (Pharmacia Biotech, NJ, United States). Five milliliters of D2008b or 5 ml of G2008b were inoculated separately in 45 ml of Mueller-Hinton (MH) broth (BD, BBL, Franklin Lakes, NJ, United States), and 5 ml of D2008b and 5 ml of G2008b were inoculated together in 40 ml of MH broth in a 250 ml Erlenmeyer flask. Bacterial suspensions were incubated in a MaxQ 4000 orbital shaker (Fisher Scientific) at 150 rpm for 24 h at 42°C under microaerobic conditions (Oxoid), as previously described, corresponding to the pre-culture step. For each condition, 5 ml was then suspended in 45 ml of fresh MH broth in a 250 ml Erlenmeyer flask and were incubated in the MaxQ 4000 orbital shaker at 150 rpm for 72 h at 42°C in jars under microaerobic conditions as previously described (Oxoid). At T = 0, T = 24 h, T = 48 h, and T = 72 h, 1 ml of C. jejuni suspensions were used to measure absorbance at 600 nm, and 2 ml was collected and centrifuged at 15,000 × g for 5 min at 4°C (VWR, Mississauga, ON, Canada). Supernatants were removed and bacterial pellets were stored at −20°C until further analysis. MH broth without bacteria was used as negative control to verify the absence of contamination. Co-culture assays were performed in three independent replicates.
2.2. Animal experiments
The current in vivo study was conducted with the approval by the Comité d’Éthique sur l’Utilisation des Animaux (CÉUA) of the Faculté de Médecine Vétérinaire of the Université de Montréal (certificate number: 19-Rech-2039). A total of 197 one-day-old Ross 308 male broiler chickens were purchased from a local hatchery where they were vaccinated against Marek’s disease and infectious bronchitis. Chicks were carried to the Centre de Recherche Avicole of the Faculté de Médecine Vétérinaire, an animal facility suitable for experiments requiring level 2 biosecurity measures. Birds were divided randomly into two groups (Room 1 and Room 2), with an ad-libitum access to feed and water. Feed consisted of a standard mash commercial formulation (Supplementary Table S1). Birds were raised on wood-shavings that were not changed during the trial. In-house heating and lighting programs were applied. The birds were orally inoculated at 14 days old. Room 1 was divided into four groups: (1) not inoculated (control #1); (2) inoculated with 103 CFU of D2008b (103 D2008b); (3) inoculated with 103 CFU of G2008b (103 G2008b); and (4) inoculated with 103 CFU of both strains at the same time (mix #1). In room 2, we decided to increase the inoculum of D2008b only – a weakly competitive strain – to explore its impact on competition with G2008b – a strongly competitive strain. Therefore, room 2 was also divided into four groups: (1) not inoculated (control #2); (2) inoculated with 107 CFU of D2008b (107 D2008b); (3) inoculated with 103 CFU of G2008b (103 G2008b); and (4) inoculated with 107 CFU of D2008b and 103 CFU of G2008b at the same time (mix #2). For both rooms, each group was housed in individual pens that were separated by pieces of Plexiglas to prevent contamination between groups. When there was a need to enter the pens, a clean new pair of boots was required to be put on right before stepping into the pens. Birds were visited daily. At 1 dpi (day post inoculation), 7 dpi, and 21 dpi, eight or nine birds per group were weighed and sedated with 0.8 ml/kg of a stock solution containing 50 ml of ketamine (100 mg/ml) and 12.5 ml of xylazine (100 mg/ml). Chickens were then euthanized by cervical dislocation. The same lobe of liver, distal ileum, and caeca were collected for each bird, kept on ice, and carried to the laboratory. The intestinal content and livers were used fresh for total C. jejuni counts. In cryotubes, about 1 g of intestinal contents were also frozen in liquid nitrogen and stored at −80°C until DNA extraction for strain specific qPCR. Prior to being processed for C. jejuni enumeration, livers were dipped in 70% ethanol for 5 s and the ethanol excess was burned-off to remove possible external contaminations. Moreover, ileal tissue samples of birds were freshly collected during necropsies, washed with RNase-free PBS (Invitrogen) and stored at −80°C in RNAlater Stabilization Solution (Invitrogen) until further analyzes.
2.3. Total Campylobacter jejuni counts
For each inoculated bird, 1 g of cecal and ileal content was serially diluted 10-fold in sterile tryptone salt solution. For uninoculated birds, 1 g of cecal and ileal contents were diluted 10-fold in sterile tryptone salt. Surface-sterilized liver lobes from inoculated and uninoculated birds were smashed to expose the internal tissue, resuspended 5-fold in sterile tryptone salt, and stomached for 1 min. One hundred microliters of each dilution were plated on Butzler agar plates (Oxoid) that were incubated in a microaerobic atmosphere (Oxoid) at 42°C for 48 h to enumerate total C. jejuni. A maximum of 10 isolated colonies from each contaminated liver were cultivated on blood agar plates and incubated under microaerobic atmosphere (Oxoid) at 42°C for 48 h. Each isolate was stored at −80°C in a freezing medium containing Brucella Broth (BD BBL) supplemented with 5% of sucrose (w/v), 20% of glycerol (v/v), 0.4% of ascorbic acid (w/v), and 0.12% of agar (w/v) until strain identification.
2.4. DNA extraction
2.4.1. DNA extraction from cecal and ileal contents
In tubes containing 500 mg of 0.1 mm silica spheres (MP Biomedical, Solon, OH, United States), 200 mg of cecal content or 300 mg of ileal contents were weighed. Seven hundred microliters of lysis buffer (500 mM Tris–HCl pH 8, 100 mM EDTA pH 8, 100 mM NaCl, and 1% SDS) were added in tubes. A mechanical lysis was accomplished with a FastPrep-24 5G Instrument (MP Biomedical), using three runs of 60 s at 6 m/s with incubation for 5 min on ice between each run. Samples were heated for 20 min at 95°C then kept again on ice for 5 min. A centrifugation was performed at 18,000 × g for 15 min at 4°C (VWR) and supernatant was used for DNA purification by phenol/chloroform (Sigma-Aldrich, St. Louis, MO, United States) as previously described (Thibodeau et al., 2015b). DNA purity was confirmed by Nanodrop 1000 (Fisher, Ottawa, ON, Canada).
2.4.2. DNA extraction from Campylobacter jejuni colonies of contaminated livers
Colonies isolated from contaminated livers were grown on blood agar plates (Fisher scientific) under microaerobic conditions (Oxoid) at 42°C for 48 h. Bacteria were collected in 1.5 ml tubes and 100 μl of 6% Chelex® 100 Resin solution (Bio-Rad, Mississauga, ON, Canada) were added. Tubes were vortexed 10 s and heated at 55°C for 30 min. After this step, tubes were heated at 98°C for 15 min and centrifuged at 14,000 × g for 5 min at 4°C (VWR). Supernatants containing DNA were collected for quantification.
2.4.3. DNA extraction from Campylobacter jejuni pellets from co-culture assays
DNA extractions from C. jejuni pellets were performed with a PowerLyser PowerSoil DNA Isolation Kit (QIAGEN, Toronto, ON, Canada) according to the manufacturer’s instructions. A FastPrep-24 5G Instrument (MP Biomedical) was used for the mechanic lysis step, consisting of two runs of 60 s at 6 m/s.
All DNA samples were quantified by DeNovix QFX Fluorometer using a Qubit dsDNA BR assay kit (Fisher Scientific) and stored at −80°C or − 20°C until further analysis.
2.5. Selection of strain specific genes
Both C. jejuni strains were grown overnight at 42°C on OMHA + blood plates under microaerobic conditions and genomic DNA was extracted using Epicentre Metagenomic DNA Isolation kits for Water (Illumina) according to the manufacturer’s instructions, as previously described (Clark et al., 2016). Briefly, quantification of DNA was performed by DeNovix QFX Fluorometer using Qubit dsDNA BR assay kit (Fisher Scientific). Sample libraries were prepared using a MiSeq Nextera® XT DNA library preparation kit (Illumina). Whole genome sequencing was performed by 250 bp paired end read sequencing on the Illumina MiSeq sequencer using a MiSeq® Reagent Kit V2 and 500 cycles on the Illumina MiSeq platform. Sequence reads were assembled into contigs using INNUca 2.6. Pangenomic annotations were performed by Roary (Supplementary Data S1). Raw reads can be accessed on NCBI under the reference number PRJNA903792. From pangenomic annotations, strain specificity, using the NCBI BLAST tool and conventional PCRs, was verified for many genes. Among these genes, two genes unique to G2008b (LpsA and DmsB) and two genes unique to D2008b (McrBC and RimP) were selected as candidates for qPCR/PCR targets for the quantification/identification of each strain in the samples (Supplementary Figure S1).
2.6. Specific quantitative/conventional PCR for Campylobacter jejuni strains
Both strains were quantified from ileal and caecal contents as well as from co-culture assays using strain specific quantitative PCRs. C. jejuni strains were identified from colonies isolated from contaminated livers by strain specific conventional PCRs. The same primers (Table 1), designed using the NCBI Primer-BLAST tool, were used to perform the strain specific conventional and quantitative PCRs described below.
For strain specific conventional PCRs, for each gene, 10 ng of DNA from colonies isolated from livers were amplified in a final reaction volume of 20 μl containing 500 nM of each primer (Invitrogen, Burlington, ON, Canada), 1X PCR Buffer (Biobasic, Markham, ON, Canada), 2 mM of MgSO4 (Biobasic), 0.2 μM of dNTPs (Biobasic), and 1 unit of Taq DNA Polymerase High Purity (Biobasic). The PCR program consisted of an initial denaturation step of 10 min at 95°C followed by 35 cycles of 10 s at 95°C, 10 s at 60°C, 10 s at 72°C, and a final elongation step of 1 min at 72°C. Conventional PCRs were performed using a Mastercycler® nexus thermocycler (Eppendorf Canada, Mississauga, ON, Canada). The PCR products were analyzed by electrophoresis in a 2% agarose gel containing 0.01% SYBR Safe DNA gel stain (Invitrogen).
For strain specific quantitative PCRs, 10 ng of DNA were amplified in a final reaction volume of 20 μl containing 500 nM of each primer (Invitrogen) and 1X Master Mix EvaGreen (Montréal Biotech Inc., Montréal, QC, Canada) for each gene. This PCR program described above was used with a final step consisting of a high-resolution melting analysis to verify the specificity of PCR products. Quantitative PCRs were performed using a Roche LightCycler® 96 Real Time PCR thermocycler (Roche Canada, Laval, QC, Canada). The abundance of each specific gene was determined using a standard curve that was prepared by the following method. DNA from both C. jejuni strains were amplified with a strain specific conventional PCR as described. The specificity of each PCR was confirmed in 2% agarose gels. The PCR product concentration was measured by a DeNovix QFX Fluorometer using a Qubit dsDNA BR assay kit (Fisher Scientific) to determine the number of gene copies for each gene. PCR products were serially diluted 10-fold to obtain a standard curve from 108 to 102 gene copies per microliter. For all samples and standard curves, quantitative PCRs were performed in duplicate for each gene. The proportion of each C. jejuni strain in cecal content, ileal content, and C. jejuni pellets from co-culture assays was determined by averaging the abundance of LpsA and DmsB copy numbers for G2008b and the abundance of McrBC and RimP for D2008b for each sample. According to the weight of cecal content, ileal content, or the volume of C. jejuni suspension from co-culture assays used for DNA extractions, the amount of C. jejuni strains were expressed in number of gene copies/g of intestinal content or in number of gene copies/mL of MH broth.
2.7. RNA extraction of ileal tissue samples and reverse transcription
Approximately 20 mg of ileum samples stored in RNAlater Stabilization Solution were rinsed twice with RNase-free PBS (Invitrogen). Tissues samples were put in tubes containing 700 mg of 1.4 mm ceramic spheres (MP Biomedical) and 1 ml of TRIzol Reagent (Invitrogen). A FastPrep-24 5G Instrument (MP Biomedical) was used for the mechanic lysis step, consisting of two runs of 30 s at 4 m/s with incubation for 5 min on ice between each run. Tubes were incubated at room temperature for 5 min. A centrifugation was performed at 12,000 × g for 10 min at 4°C and the supernatant was collected to perform RNA isolation according to the manufacturer’s protocol from TRIzol Reagent. RNA purity was confirmed by Nanodrop 1000 (Fisher). RNA was quantified by DeNovix QFX Fluorometer using a Qubit RNA BR assay kit (ThermoFisher Scientific). One microgram of RNA was used for the reverse transcription to cDNA using SuperScript IV VILO Master Mix (Invitrogen) according to the manufacturer’s instructions.
2.8. Real-time quantitative PCR (qRT-PCR) of cDNA
For each gene and bird, 1 μl of cDNA was amplified in duplicate in a final reaction volume of 10 μl containing 500 nM of each primer (Invitrogen), 1X PowerTrack SYBR Green Master Mix (ThermoFisher Scientific) and 1X Yellow Sample Buffer (ThermoFisher Scientific). The PCR program consisted of an initial denaturation step of 10 min at 95°C followed by 30 cycles of 10 s at 95°C, 10 s at an annealing temperature indicated in Table 2 and a final step constituting a high-resolution melting to verify the specificity of PCR products. Quantitative PCRs were performed using a Roche LightCycler® 96 Real Time PCR thermocycler (Roche Canada). Primers efficiency was considered adequate when it was between 90 and 110%. Due to their stable transcript level in ileal tissue in presence of C. jejuni, β-actin and RPL32 were used as reference genes (Bagés et al., 2015). Relative transcript levels of target genes (ZO1, JAM2, OCLN, CLDN5, CLDN10) for inoculated birds were normalized to those of two reference genes and to the mean value of the control group for each room and time point using the 2−ΔΔCt formula (Livak and Schmittgen, 2001).
2.9. Statistical analyzes
Figures and statistical analyzes were performed with GraphPad Prism 9.2.0 (GraphPad Software Inc., La Jolla, CA). A Shapiro–Wilk test was used to verify data normality. To analyze statistical differences between groups according to the time point and room for the in vivo trial and co-culture assays, the specific statistical tests used are indicated in the figure legends. The limit of detection was set to 102 CFU/g of cecal or ileal content for total C. jejuni counts and to 104 gene copies/g of cecal or ileal content for qPCR results. Thus, in absence of detection, 9 × 101 CFU/g of cecal or ileal contents were used to compare total C. jejuni counts and 9 × 103 CFU/g to compare qPCR results between colonized chicken groups. Differences were statistically different when *p < 0.05, **p < 0.01, ***p < 0.001.
3. Results
3.1. General bird health
No hepatic gross lesions were observed at the time of necropsy and no significant difference in body weight was noted at 1, 7, and 21 days post infection (dpi; data not shown). Nevertheless, a transient diarrhea was noted at 1 dpi for 24 h only for birds infected simultaneously by both C. jejuni strains independently of the ratio of the two strains.
3.2. Cecal colonization of Campylobacter jejuni strains
Total C. jejuni counts were analyzed from the cecal content of all chickens by bacterial culture at 1, 7, and 21 dpi (Figure 1). In rooms 1 (same proportion of both strains) and 2 (higher ratio of D2008b), the absence of C. jejuni counts in the caeca from control birds was confirmed for each time point. At 1 dpi, only one bird and six birds were colonized by C. jejuni in ceca in room 1 and 2, respectively. Unlike birds inoculated with 103 CFU of G2008b and both strains in room 1, the absence of C. jejuni from the caeca of birds inoculated with 103 CFU of D2008b was noted at 7 and 21 dpi. At 7 and 21 dpi, no significant difference was observed for the total C. jejuni counts between birds inoculated with 103 CFU of G2008b and with both strains. At 7 dpi, the number of C. jejuni in the cecal content of birds inoculated with G2008b was significantly higher (8.21 log10 CFU/g) than those from birds inoculated with D2008b (6.97 log10 CFU/g; p < 0.05) in room 2. At 21 dpi, no significant difference was observed between these two groups.
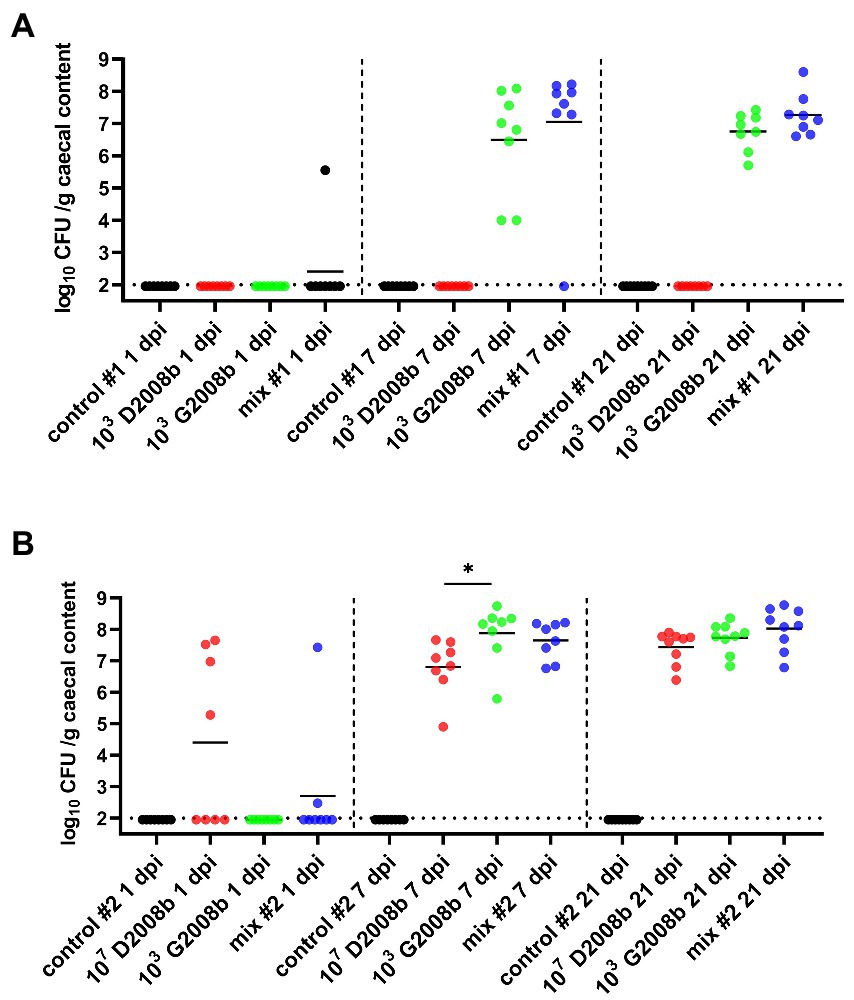
Figure 1. Total Campylobacter jejuni counts in cecal content (log10 CFU/g of cecal content) from birds at 1, 7, and 21 dpi for room 1 (A) and room 2 (B). Dots correspond to individual birds. Horizontal bars represent median values, and horizontal dotted lines denote the limit of detection (2 log10 CFU/g of cecal content). Due to the low number of birds colonized at 1 dpi, statistical analyses were not performed. At 7 dpi and 21 dpi, statistical analyzes were conducted using a Mann–Whitney test (A) and a Kruskal-Wallis test followed by Dunn’s post-hoc tests with Bonferroni adjustments (B) to compare median values between colonized groups according to the time point and room. * indicates p<0.05.
To assess the load of each C. jejuni strain in the cecal content, particularly in co-inoculation cases, strain specific qPCRs were performed (Figure 2). Strain specific genes McrBC and RimP were targeted by qPCR to evaluate the D2008b load, while LpsA and DmsB were targeted for the G2008b load. Due to a low number of birds colonized at 1 dpi, qPCRs were only performed on samples collected at 7 and 21 dpi. For both time points, no strain specific genes were detected from the cecal content of birds infected with D2008b alone in room 1. For samples collected from this same room, only the G2008b specific genes were detected at 7 and 21 dpi in the cecal content of birds inoculated with G2008b. For birds inoculated with both C. jejuni strains, D2008b specific genes were detected from one bird at 7 dpi, while the other birds revealed the presence of G2008b solely. At 21 dpi, an increase in D2008b levels close to the G2008b levels was noticed for birds that had been co-inoculated, with the two strains reaching similar levels in the caeca. In room 2, at 7 and 21 dpi, only G2008b or D2008b specific genes were detected from the cecal content of birds inoculated with these single strains alone, respectively. For birds inoculated with both strains, similar levels of strain specific genes were observed in the cecal content at 7 dpi. At 21 dpi, a decrease in the abundance of D2008b was observed in co-inoculated birds. The number of gene copies of D2008b in the cecal content of co-inoculated birds (5.91 log10 gene copies/g) was significantly lower than the number found in birds inoculated with D2008b alone (8.65 log10 gene copies/g; p < 0.05). In addition, from the cecal content of broiler chickens inoculated with both strains, a 2.85 log10 difference was noted between the number of gene copies of D2008b and the number of gene copies of G2008b (p < 0.05).
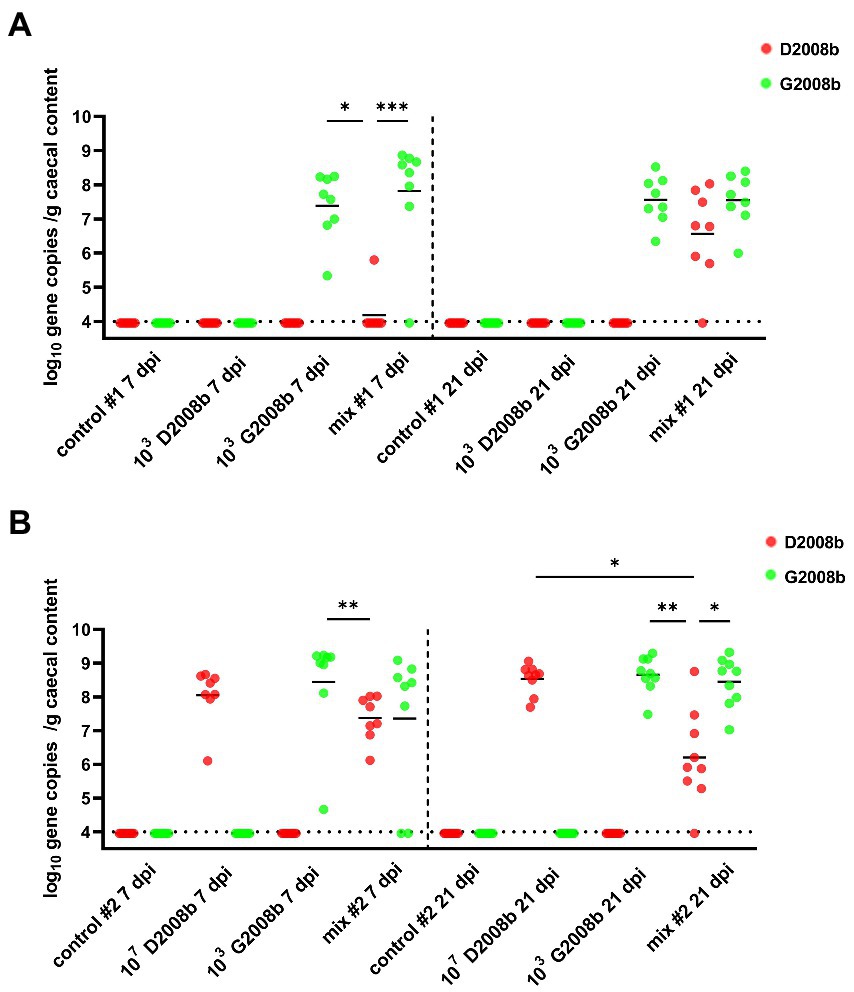
Figure 2. Amounts of Campylobacter jejuni strains in cecal content (log10 gene copies/g of cecal content) from birds at 7 and 21 dpi for room 1 (A) and room 2 (B). Red and green dots correspond to the amount of D2008b and G2008b, respectively, for individual birds. Horizontal bars represent median values, and horizontal dotted lines denote the detection limit (4 log10 CFU/g of cecal content). Statistical analyzes were conducted using a Kruskal-Wallis test followed by Dunn’s post-hoc tests with Bonferroni adjustments to compare median values between colonized groups according to the time point and room. *, ** and *** indicate p<0.05, p<0.01 and p<0.001 respectively.
3.3. Ileal colonization of Campylobacter jejuni strains
Total C. jejuni counts were also analyzed from the ileal content of birds at 1, 7, and 21 dpi (Figure 3). For both rooms, absence of C. jejuni counts in the ileum from control birds was confirmed for each time point. At 1 dpi, only one bird was colonized by C. jejuni in ileum of birds in room 2. In room 1, the ileal content of birds inoculated with D2008b did not reveal the presence of C. jejuni at 7 and 21 dpi. At 7 dpi, total C. jejuni counts in the ileum of birds inoculated with G2008b and birds receiving both strains were very similar (2.45 log10 CFU/g and 2.15 log10 CFU/g respectively). At 21 dpi, the number of C. jejuni in the ileal content of co-inoculated chickens (5.69 log10 CFU/g) was significantly higher than those found in the ileum of birds inoculated with G2008b (3.84 log10 CFU/g; p < 0.05). In room 2 at 7 dpi, total C. jejuni counts in the ileal content of birds inoculated with D2008b and with both strains were higher by 1.91 log10 (p < 0.05) and 2.82 log10 (p < 0.001), respectively, in comparison to the counts observed for birds inoculated with G2008b. At 21 dpi, a 1.60 log10 increase in the number of C. jejuni in the ileal content of birds inoculated with G2008b was observed. Nevertheless, the number of C. jejuni found in the ileal content of chickens inoculated with G2008b was still lower by 1.00 log10 (p < 0.01) in comparison to the numbers found in the ileums of birds inoculated with D2008b.
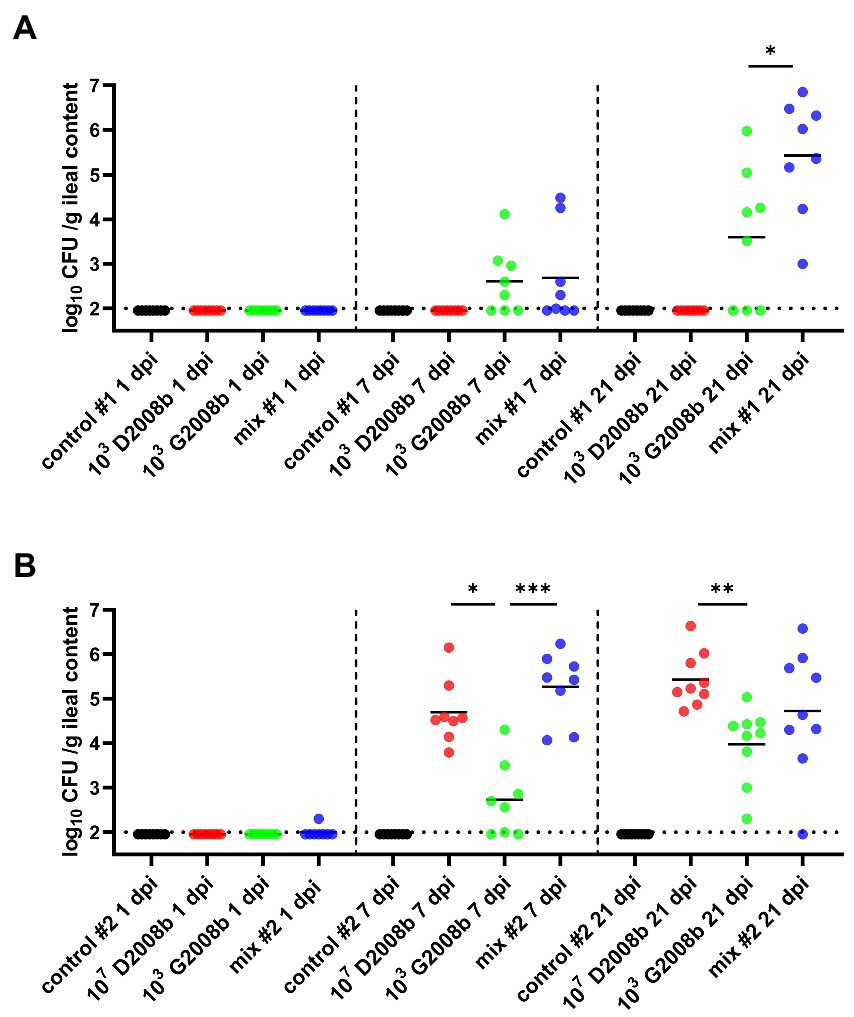
Figure 3. Total Campylobacter jejuni counts in ileal contents (log10 CFU/g of ileal content) from birds at 1, 7, and 21 dpi for room 1 (A) and room 2 (B). Dots correspond to individual birds. Horizontal bars represent median values, and horizontal dotted lines denote the threshold (2 log10 CFU/g of ileal content). Due to the low number of birds colonized at 1 dpi, statistical analyzes were not performed. At 7 and 21 dpi, statistical analyzes were conducted using a Mann–Whitney test (A) and a Kruskal-Wallis test followed by Dunn’s post-hoc tests with Bonferroni adjustments (B) to compare median values between colonized groups according to the time point and room. *, ** and *** indicate p<0.05, p<0.01 and p<0.001 respectively.
To assess the amount of each C. jejuni strain in the ileal content, strain specific qPCRs were performed (Figure 4). Strain specific genes McrBC and RimP were targeted by qPCR to evaluate the D2008b load, while LpsA and DmsB were targeted for the G2008b load. Due to a low number of birds colonized at 1 dpi and to the low counts of C. jejuni enumerated by bacterial culture at 7 dpi, qPCRs were only performed on samples collected at 21 dpi. No strain specific gene was identified in the ileal content of chickens inoculated with D2008b in room 1. Only G2008b specific genes were detected in the ileal content of chickens inoculated with G2008b but close amounts of both strains were quantified in the ileal content of co-inoculated birds. At 21 dpi, only G2008b or D2008b specific genes were detected from the ileal content of birds inoculated with a single strain G2008b or D2008b, respectively, in room 2. From the ileal content of birds inoculated with D2008b, significantly higher numbers of D2008b gene copies (5.97 log10 gene copies/g) were detected compared to the number of G2008b gene copies found in birds inoculated with G2008b (4.24 log10 gene copies/g; p < 0.05) and to the number of D2008b gene copies found in birds receiving both strains (3.95 log10 gene copies/g; p < 0.01). No significant difference was noticed in the number of gene copies for D2008b and for G2008b in the ileal content of birds inoculated with both strains.
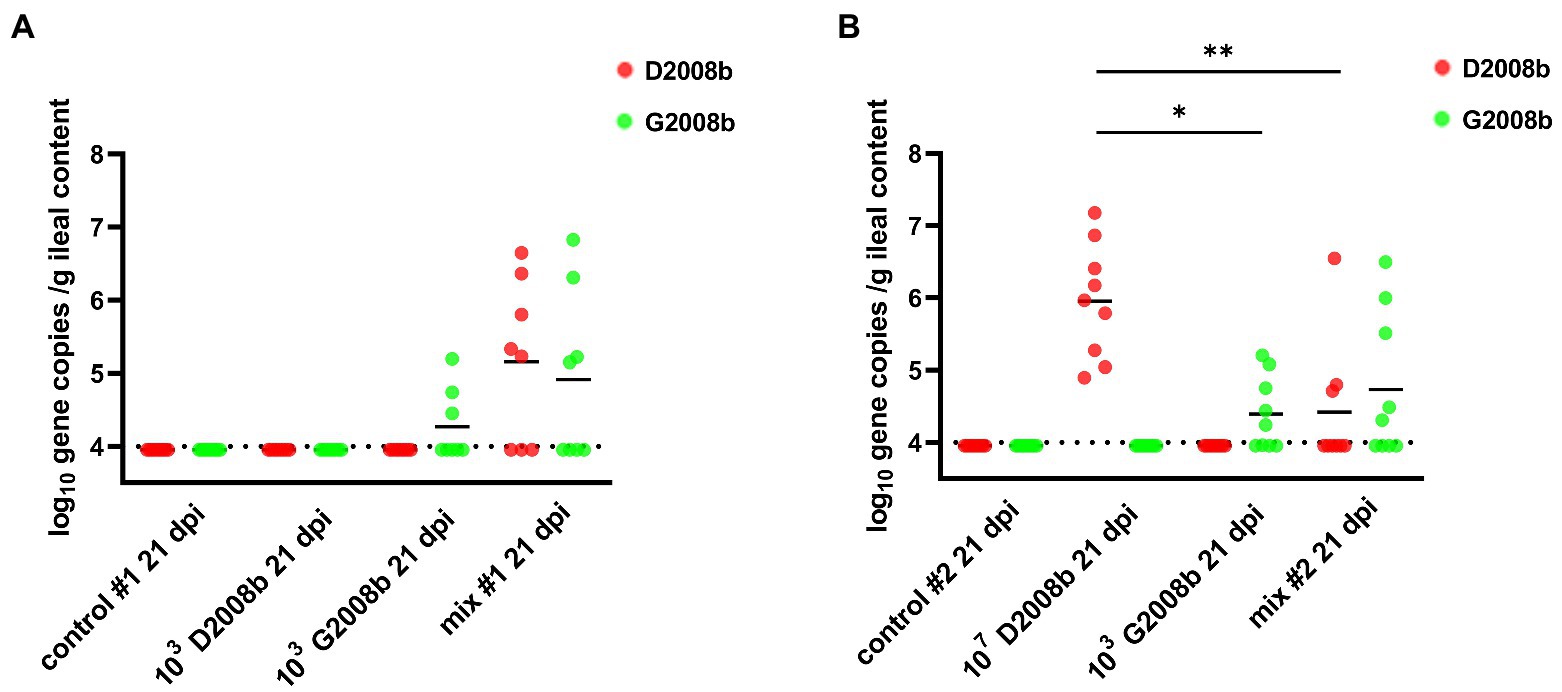
Figure 4. Amounts of Campylobacter jejuni strains in ileal content (log10 gene copies/g of ileal content) from birds at 7 and 21 dpi for room 1 (A) and room 2 (B). Red and green dots correspond to the amount of D2008b and G2008b, respectively, for individual birds. Horizontal bars represent median values, and horizontal dotted lines denote the threshold (4 log10 CFU/g of ileal content). Statistical analyzes were conducted using a Kruskal-Wallis test followed by Dunn’s post-hoc tests with Bonferroni adjustments to compare median values between colonized groups according to the time point and room. * and ** indicate p<0.05 and p<0.01 respectively.
3.4. Extra-intestinal spread of Campylobacter jejuni strains to the liver
To evaluate the extra-intestinal spread to the liver of C. jejuni strains, total C. jejuni counts were determined at 1, 7, and 21 dpi by bacterial culture (Table 3). In both rooms, C. jejuni was not detected inside the liver of control birds and of birds inoculated with G2008b alone. In room 2, a significant extra-intestinal spread (>5 × 103 CFU/g) of C. jejuni to the liver of birds inoculated with both strains was noticed at 7 dpi for seven of eight birds and to a lesser extent at 21 dpi for four of nine birds. However, in the liver of chickens inoculated with D2008b alone, the presence of C. jejuni was observed at 21 dpi only. Given that bacterial loads found in the liver were below the qPCRs threshold, bacterial DNA was extracted from C. jejuni colonies and specific conventional PCRs were performed to assess the identity of the isolates. Only D2008b specific genes (RimP and McrBC) were detected for isolates recovered from the colonized livers (Table 4).
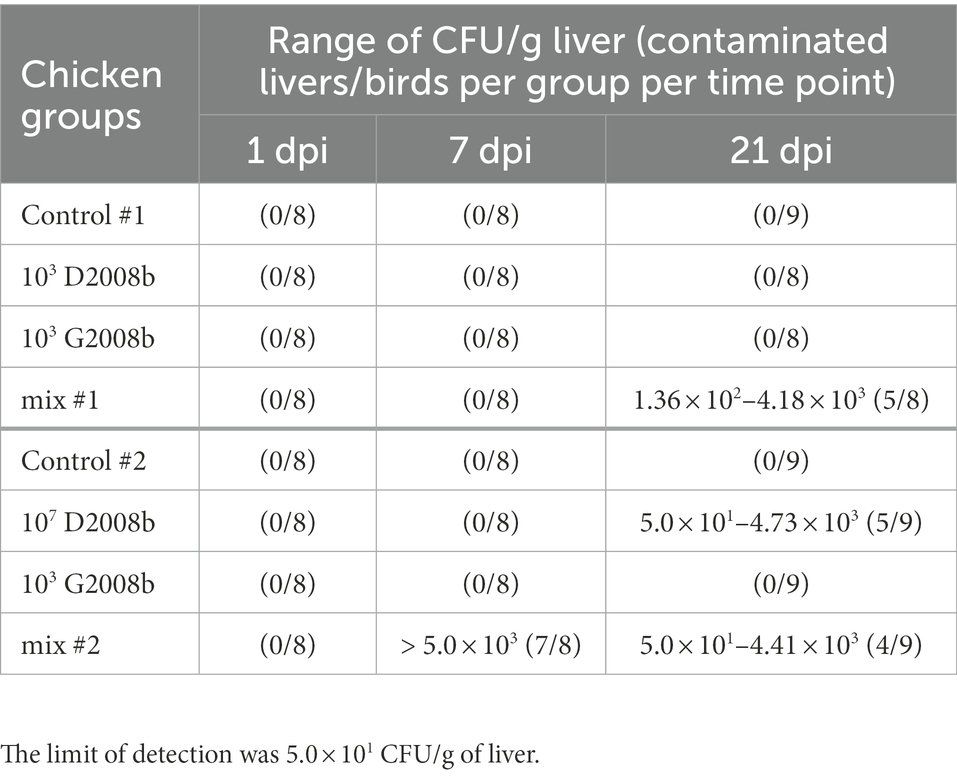
Table 3. Total Campylobacter jejuni counts in liver (CFU/g of liver) from birds at 1 dpi, 7 dpi and 21 dpi for rooms 1 and 2.
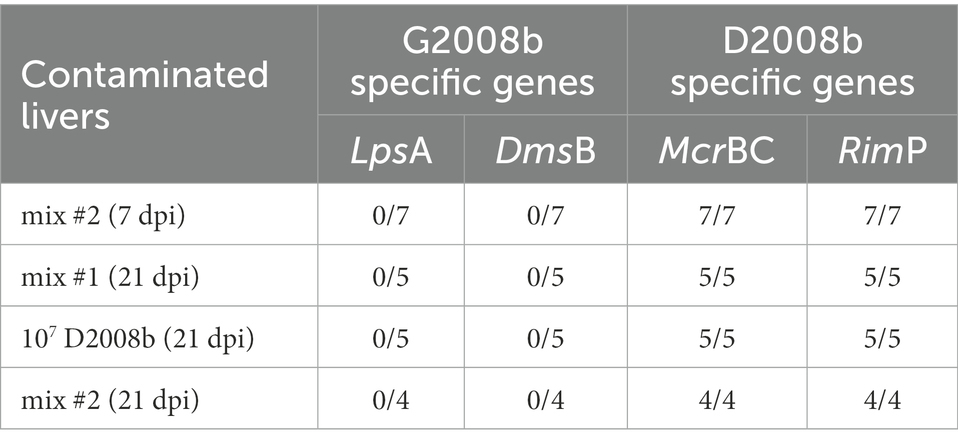
Table 4. Identification of Campylobacter jejuni strains from contaminated livers by strain specific PCR.
3.5. Relative transcript level of tight junction proteins in ileum
To evaluate the C. jejuni inoculation effect on relative transcript level of different tight junction proteins, RT-qPCR of ZO1, JAM2, OCLN, CLDN5 and CLDN10 were performed in ileal tissue samples from birds at 7 dpi and 21 dpi (Figures 5, 6). At 7 dpi, a significant decrease of the transcript level of JAM2 and CLDN5 was noted in ileal tissue from birds inoculated with both strains in room 1, and CLDN10 from birds inoculated with both strains in both rooms. In contrast, at 21 dpi, a significant upregulation of the transcript level of ZO1 and OCLN was observed in ileal tissue from birds inoculated with both strains in room 1; of JAM2, OCLN and CLDN10 from birds inoculated with 107 CFU of D2008b; of JAM2 and OCLN from birds inoculated with both strains in room 2.
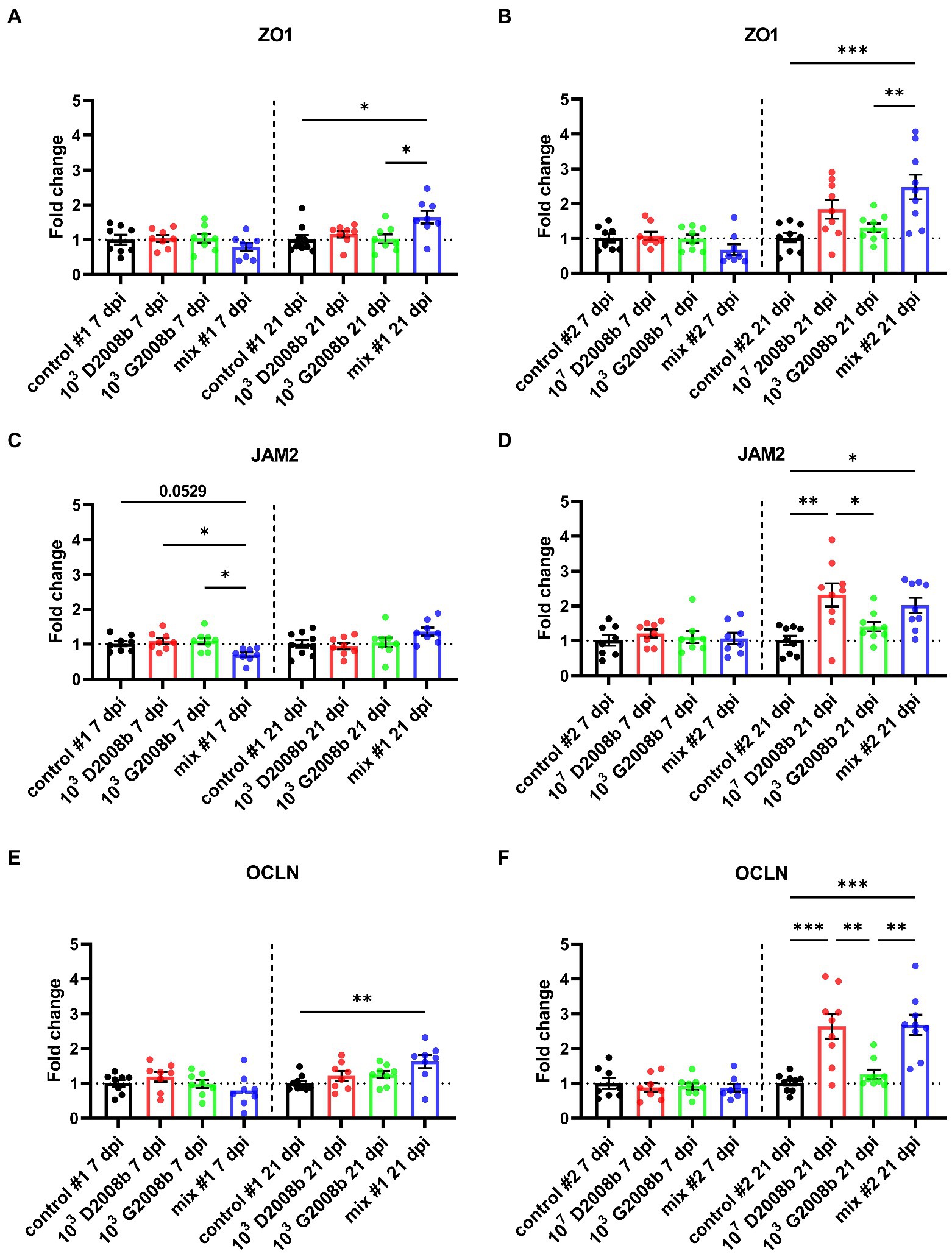
Figure 5. Relative gene expression of ZO1 (A,B), JAM2 (C,D) and OCLN (E,F) in ileal tissue for rooms 1 and 2, respectively, at 7 dpi and 21 dpi. Dots correspond to the protein expression level normalized to two reference genes, RPL32 and β-actin, and to the mean value of the control group. Vertical bars represent mean values ± SEM. ANOVA test followed by Tukey’s multiple comparisons tests were used to analyze statistical differences between chicken groups within the same time point. *, ** and *** indicate p<0.05, p<0.01 and p<0.001 respectively.
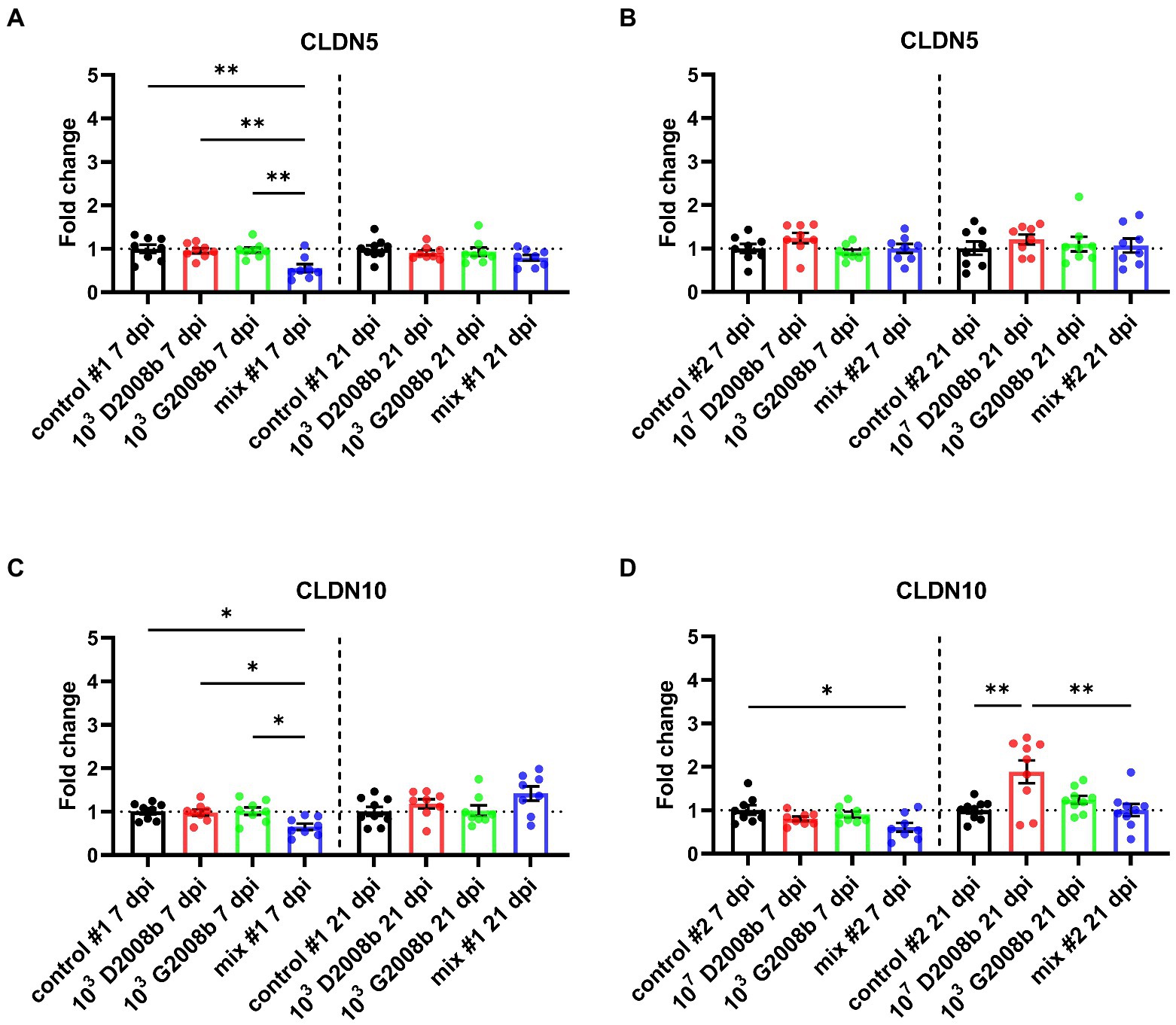
Figure 6. Relative gene expression of CLDN5 (A,B) and CLDN10 (C,D) in ileal tissue for rooms 1 and 2, respectively, at 7 dpi and 21 dpi. Dots correspond to the protein expression level normalized to two reference genes, RPL32 and β-actin, and to the mean value of the control group. Vertical bars represent mean values ± SEM. ANOVA test followed by Tukey’s multiple comparison tests were used to analyze statistical differences between chicken groups within the same time point. * and ** indicate p<0.05 and p<0.01 respectively.
3.6. In vitro co-culture of Campylobacter jejuni strains
Both C. jejuni strains were cultivated alone and together in MH broth in three independent culture assays, and absorbances were measured at different time points (Figure 7A). After 24 h, the absorbance of D2008b culture was significantly lower than that of G2008b (p < 0.05). Additionally, we observed that the absorbance of the co-culture broth was significantly higher than that of the single strain culture broths [G2008b (p < 0.05) and D2008b (p < 0.01)]. No significant difference was observed between conditions when co-cultures were extended to 48 h and 72 h. Quantitative PCRs were performed to evaluate the relative amount of each C. jejuni strain specific gene in bacterial co-culture and single strain cultures (Figure 7B). At T = 0, no significant difference was observed between conditions. At T = 24 h and T = 48 h, no significant difference between single strain cultures was observed. However, at T = 48 h, the number of D2008b gene copies was lower in the presence of G2008b (p < 0.01).
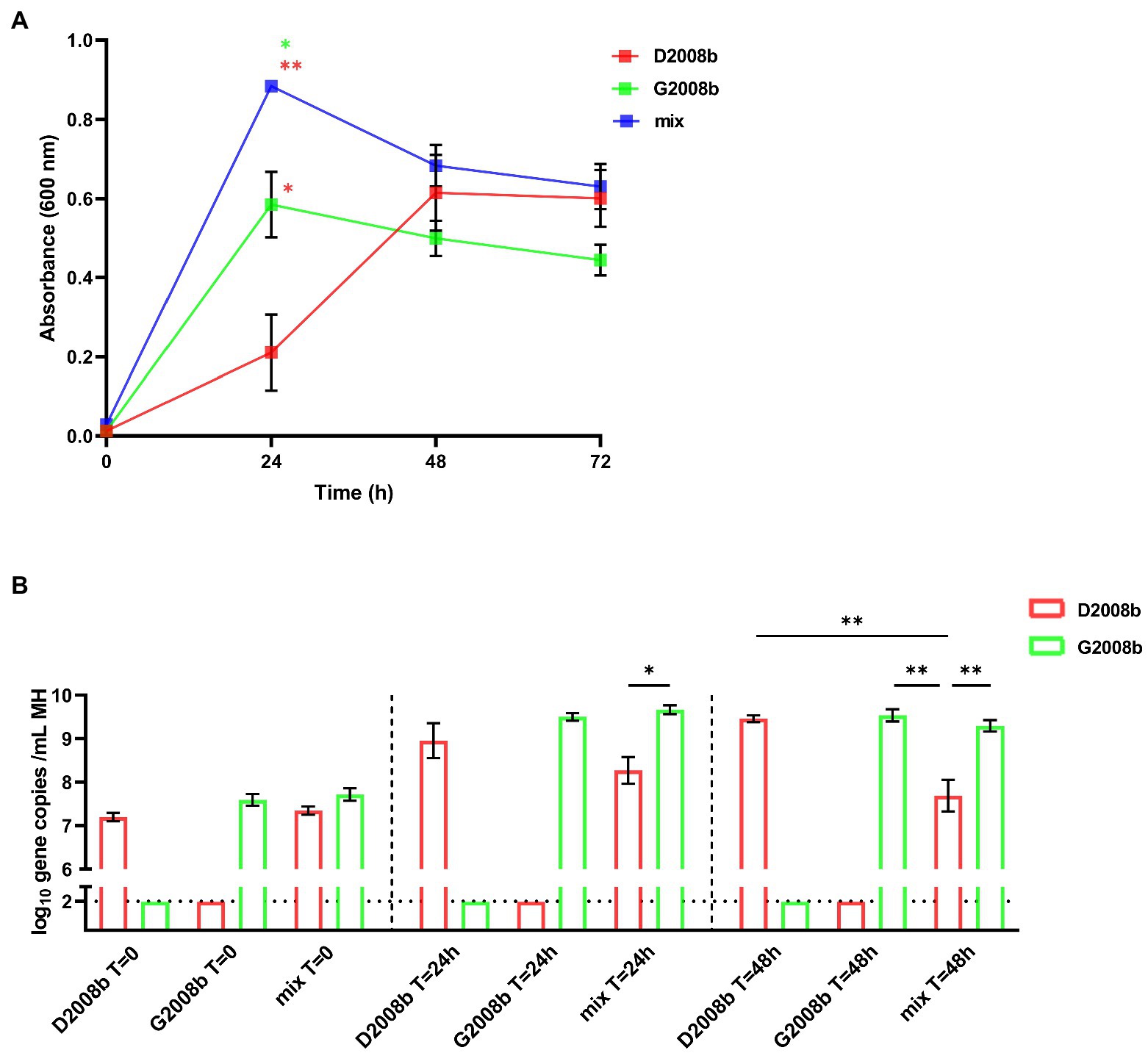
Figure 7. In vitro co-culture of both Campylobacter jejuni strains in MH broth. Both C. jejuni strains were grown separately and together in MH broth and absorbances at 600 nm were measured at different time points (A). To evaluate the amounts of each strain, strain specific qPCRs were performed from C. jejuni pellets (B). Vertical bars represent mean values ± SEM, and horizontal dotted lines denote the threshold (2 log10 gene copies/mL of MH broth). Student t tests were used to analyze statistical differences of absorbance and ANOVA test followed by Tukey’s multiple comparison tests to compare qPCR results by time point between conditions. Co-culture assays were conducted in three independent replicates. * and ** indicate p<0.05 and p<0.01 respectively.
4. Discussion
Most in vivo experiments in broilers available in the literature have been conducted using a single C. jejuni strain. However, considering the wide variety of C. jejuni strains observed at the broiler farm level, the current study evaluates the impact of the presence of two distinct C. jejuni strains – alone and together – on the intestinal colonization and hepatic spread of the bacterium in broiler chickens to approximate the reality on farms more closely.
Although uninoculated birds were raised in the same room as inoculated birds, they were not colonized by C. jejuni throughout the animal experiments. Moreover, birds inoculated with a single C. jejuni strain were shown to have been colonized only by that strain. All measures taken in the animal facility to prevent contamination between groups were very effective, thereby to compare the intestinal colonization level, the hepatic spread of C. jejuni and the transcript level of tight junctions for birds inoculated by single or both strain(s) without room effect.
In both rooms, few birds were colonized in caeca at 1 day post infection (dpi), and even fewer birds were found to be colonized in ileum after analysis, due especially to the fact that the dose and inoculated strain(s) would have had an influence on early intestinal colonization. It has already been described that, depending on the strain, the cecal colonization of broilers could start at 1 dpi or later, and that all birds were colonized between 3 dpi and 14 dpi (Young et al., 1999; Knudsen et al., 2006). In this study, at 7 and 21 dpi, broiler chickens inoculated with 103 CFU of D2008b alone were never shown to be colonized throughout the experiment, unlike birds inoculated with 103 CFU of G2008b alone, with 107 CFU of D2008b, or with both strains. Although both strains were originally isolated from the caeca of broiler chickens, it seems that a 103 CFU inoculum of D2008b was not sufficient to induce colonization and the minimum dose of D2008b required for an effective intestinal colonization appears to be higher for this strain compared to G2008b. It has already been shown that a minimum dose of 102 to 104 CFU per bird, according to the C. jejuni strain used, is required for effective colonization in 14-day-old chickens (Ringoir and Korolik, 2003; Dhillon et al., 2006; Knudsen et al., 2006). These variations between strains are likely due to differences in phenotypic properties such as autoagglutination, chemotaxis, adhesion, and invasion, as previously reported (Thibodeau et al., 2015a). Although similar cecal colonization levels were observed between birds inoculated with 107 CFU of the D2008b and those inoculated with 103 CFU of G2008b at 21 dpi, D2008b appears to be better able to colonize the ileum. We suspect that the inoculum difference could impact colonization levels in the ileum or that the colonization dynamics of these two C. jejuni strains at the caeca and ileum levels could be different. Interestingly, our observations support similar observations reported by in broiler chickens inoculated with a same inoculum of two C. jejuni reference strains separately: M1 or 13126 (Chaloner et al., 2014). These authors showed that M1 preferentially colonized the caeca, whereas 13126 colonized the distal ileum at a higher level. The authors suggested that these strains would have different metabolic capacity and that variations throughout the gastrointestinal tract, including pH, oxygen tension, levels of bile, mucins, or even differences in the intestinal microbiota could explain these findings.
For birds housed in room 1 inoculated with both strains, D2008b seemed to benefit from the presence of G2008b for its establishment in the caeca and in the ileum as both strains were found at similar average levels at 21 dpi; D2008b alone did not colonize the birds. As the presence of D2008b did not affect the colonization levels of G2008b in the caeca and in the ileum, we suggest that a commensalism could occur between both C. jejuni strains at the intestinal level. Commonly, the term commensalism is used to define an interaction between two organisms that is beneficial for one without negatively or positively impacting the other. On the contrary, no commensalism was observed for the in vitro co-culture assays. Consequently, we think that direct interactions between strains would not underlie this proposed relationship observed in vivo. It was also demonstrated that other bacterial species can enhance Campylobacter colonization in mice (Wang et al., 2018) and in chickens (Yan et al., 2021), such as Clostridium. Therefore, investigation on the microbiota of the study animals is mandatory to see if different bacterial populations are associated to the different inoculated strains. When the inoculum of D2008b was increased by 4 log10 for birds housed in room 2, similar cecal colonization levels of D2008b were observed at 7 dpi, whether or not birds were inoculated with G2008b. However, we noted an average decrease of about 3 log10 in the colonization levels of D2008b in the presence of G2008b in caeca at 21 dpi. We therefore suggest that competition occurs between C. jejuni strains for cecal colonization between 7 dpi and 21 dpi, as reported in other experiments (Konkel et al., 2007; Coward et al., 2008; Calderón-Gómez et al., 2009). Our findings show that the in vitro classification method developed by our group successfully predicted G2008b as a dominant strain during this cecal co-colonization (Thibodeau et al., 2015a), despite a higher inoculum of D2008b. In addition, in room 2, we found variations in strain ratios between birds inoculated with both strains at 21 dpi, as already observed by other authors (Konkel et al., 2007; Coward et al., 2008). For these authors, stochastic effects including bird-to-bird transmission, population crashes, and phenotypic variations would contribute to these variations. Despite lower levels found in the ileum, we saw that D2008b colonization was decreased in the presence of G2008b at 21 dpi. We therefore demonstrated that C. jejuni strains compete for establishment in the ileum. Authors have also reported this competition in their dual infection model with two C. jejuni reference strains in chickens (Chaloner et al., 2014). For birds from room 1, there might be a dominance effect observable at the last time point in coherence with the differences in the amount of both strains for some birds.
To explore the underlying mechanisms, both strains were co-cultivated in vitro in MH broth. After 48 h of culture, the lower gene copies number observed for D2008b in the presence of G2008b suggests that an interaction occurs between strains. We hypothesize that G2008b could secrete antimicrobial factors in the presence of D2008b to stop its growth, which would explain the absorbance increase at T = 24 h when strains were grown together. This increase in the optic density could also be due to an increased overall bacterial death of D2008b. Another hypothesis would be based on a different susceptibility of both strains to oxidative stress resulting from bacterial growth (Nennig et al., 2022). Moreover, both strains might not be able to use the same nutrients as the culture media is becoming depleted, therefore giving an advantage to one strain over the other. Similar in vitro experiments have been performed with two C. jejuni strains competing for cecal colonization in chickens (Konkel et al., 2007). However, the authors observed no significant difference in the growth rate of strains when cultured alone or together. Future work should focus on investigating the nature of interactions that occur between C. jejuni strains. Although differences observed between both strains in our in vitro co-culture assays are lower than that of our in vivo trial, this suggests that other mechanisms could be involved in strain dominance. However, all of these findings remain to be validated with other competitive strains by performing in vitro assays.
Additionally, we observed that a 4 log10 increase in the inoculum of D2008b led to its greater dissemination to the liver, likewise reported in the literature (Awad et al., 2020). Moreover, we also noted that G2008b appears to facilitate and accelerate the hepatic dissemination of mainly D2008b, and this was clearly observed when a low dose of D2008b – insufficient on its own to cause intestinal colonization – was used. Recently, similar observations were reported in female turkeys co-inoculated with C. jejuni and C. coli (Rzeznitzeck et al., 2022). For the first time, we therefore demonstrate that an inoculation with two C. jejuni strains would increase liver contamination. Our findings could explain why the majority of studies conducted with a single C. jejuni strain show a much lower rate of liver contamination than is found inside retail chicken livers (Knudsen et al., 2006; Pielsticker et al., 2016). Based on these new data, the hepatic spread variability of isolates inoculated separately (Knudsen et al., 2006; Jennings et al., 2011; Pielsticker et al., 2016) would illustrate the need for additional C. jejuni strains to improve the hepatic dissemination of a specific strain. In the current study, the dominance of D2008b inside the liver confirms that C. jejuni strains have different abilities to invade the internal organs of broiler chickens (Young et al., 1999; Chaloner et al., 2014; Pielsticker et al., 2016). Moreover, in some birds inoculated with both strains, a dominance of D2008b in the liver was associated with a dominance of G2008b in intestinal content. Similar observations have already been reported in a recent study conducted by at the slaughterhouse, showing the isolation of distinct C. jejuni strains from the caeca and liver of the same chickens (Berrang et al., 2019). It therefore seems that intestinal colonization and extraintestinal spread involve different mechanisms. In addition, the transcript level decrease of JAM2, CLDN5 and CLDN10 at 7 dpi observed for some chicken groups inoculated with both strains in both rooms and having livers contaminated by C. jejuni suggest that the presence of C. jejuni could alter the intestinal epithelial barrier function and enhance the intestinal paracellular permeability, likewise reported in the literature (Lamb-Rosteski et al., 2008; Awad et al., 2020; von Buchholz et al., 2022), leading to extraintestinal spread to internal organs through the bloodstream because of a leaky gut, likely for specific strains only. In view of our results, we suggest that this hypothetical mechanism, leading to the hepatic spread of D2008b, would be enhanced in the presence of G2008b. However, the reason why G2008b did not seem to benefit from this leaky gut by entering the liver remains to be determined. Moreover at 21 dpi, the host response to the hepatic dissemination, like a tissue repair, could explain the increased transcript level of ZO1, JAM2, OCLN and CLDN10 for the groups of birds having livers contaminated by C. jejuni. This hypothesis could thus explain the transient extraintestinal dissemination of C. jejuni to the liver. Future research should thoroughly study genotypic and phenotypic characteristics of C. jejuni strains able to invade the liver and study the underlying mechanisms of this hepatic dissemination during a multi-strain colonization in broiler chickens.
In conclusion, we showed that the in vitro classification developed by our group successfully predicted the competition between D2008b and G2008b, with a dominance of G2008b in the ileum and caeca of birds. For the first time, we observed that commensalism seems to exist between both C. jejuni strains, and that this relationship could contribute to intestinal colonization and enhance the hepatic spread of one strain in particular. These results highlight the importance of limiting the introduction of other C. jejuni strains by implementing effective biosecurity measures that would help avoid the potential amplification of gut colonization and liver dissemination of certain strains already present on the farm.
However, given the variance of Campylobacter colonization in chickens, more animal experimentations should be performed with C. jejuni strains in order to confirm the hypotheses of the current study since the lack of replicates in the present study is a clear limitation. For instance, it would be interesting to vary the strains used for inoculation and to sequence them to be able to increase the number of strains for comparative genomics to narrow down the list of genes that might be involved in the differential spread of the strains to the liver. Additionally, using different dose for inoculation and following the animals every day instead of every week would help to better understand what is going on when multiple strains of C. jejuni colonize the chicken at the same time. One last limitation of our study is that the number of copy numbers of genes found in each strain was not validated, results that could slightly modify the qPCR results. Future studies should focus on the underlying mechanisms of the commensalism and competition between C. jejuni strains during multi-strain colonization in chickens. Such as analysis of bird immune response and intestinal microbiota as an improved understanding of the colonization dynamics between C. jejuni strains, alone and together, should be considered in order to develop adequate on-farm control strategies.
Data availability statement
The original contributions presented in the study are included in the article/Supplementary material, further inquiries can be directed to the corresponding authors.
Ethics statement
The animal study was reviewed and approved by Comité d’Éthique sur l’Utilisation des Animaux (CÉUA) of the Faculté de Médecine Vétérinaire of the Université de Montréal (certificate number: 19-Rech-2039).
Author contributions
SC, PF, and AT conceived and designed the experiments. WT provided essential scientific and technical advice. SC, M-LG, WT, and AT actively participated in conducting the in vivo experimentations, including bird inoculations, necropsies, and sample processing. SC performed all the experiments in the laboratory. SC and AT analyzed data and wrote the manuscript. M-LG, WT, and PF revised the manuscript. All authors contributed to the article and approved the submitted version.
Funding
The current study was financially supported by the Natural Sciences and Engineering Research Council of Canada (NSERC grant no. RDCPJ 520873-17), the Consortium de recherche et innovations en bioprocédés industriels au Québec, the Swine and Poultry Infectious Diseases Research Center (CRIPA), The Groupe de Recherche sur les maladies infectieuses en production animale (GREMIP) and the Fonds du Centenaire of the Faculté de Médecine Vétérinaire of the Université de Montréal.
Acknowledgments
We would like to thank all members of the Research Chair in Meat Safety for their help during necropsies and the staff of the Centre de Recherche Avicole of the Faculté de Médecine Vétérinaire for their rigorous work and assistance. We are also grateful to JEFO Nutrition Inc. (Saint-Hyacinthe, QC, Canada) for providing the chicken feed, to Eduardo N. Taboada for performing the whole genome sequencing, and to Neda Barjesteh for providing scientific advice.
Conflict of interest
The authors declare that the research was conducted in the absence of any commercial or financial relationships that could be construed as a potential conflict of interest.
Publisher’s note
All claims expressed in this article are solely those of the authors and do not necessarily represent those of their affiliated organizations, or those of the publisher, the editors and the reviewers. Any product that may be evaluated in this article, or claim that may be made by its manufacturer, is not guaranteed or endorsed by the publisher.
Supplementary material
The Supplementary material for this article can be found online at: https://www.frontiersin.org/articles/10.3389/fmicb.2022.1071175/full#supplementary-material
References
Alpigiani, I., Abrahantes, J. C., Michel, V., Huneau-Salaün, A., Chemaly, M., Keeling, L. J., et al. (2017). Associations between animal welfare indicators and campylobacter spp. in broiler chickens under commercial settings: a case study. Prev. Vet. Med. 147, 186–193. doi: 10.1016/j.prevetmed.2017.09.005
Awad, W. A., Molnár, A., Aschenbach, J. R., Ghareeb, K., Khayal, B., Hess, C., et al. (2015). Campylobacter infection in chickens modulates the intestinal epithelial barrier function. Innate Immun. 21, 151–160. doi: 10.1177/1753425914521648
Awad, W. A., Ruhnau, D., Hess, C., and Hess, M. (2020). Campylobacter jejuni increases the paracellular permeability of broiler chickens in a dose-dependent manner. Poult. Sci. 99, 5407–5414. doi: 10.1016/j.psj.2020.08.014
Bagés, S., Estany, J., Tor, M., and Pena, R. N. (2015). Investigating reference genes for quantitative real-time PCR analysis across four chicken tissues. Gene 561, 82–87. doi: 10.1016/j.gene.2015.02.016
Barekatain, R., Chrystal, P. V., Howarth, G. S., McLaughlan, C. J., Gilani, S., and Nattrass, G. S. (2019). Performance, intestinal permeability, and gene expression of selected tight junction proteins in broiler chickens fed reduced protein diets supplemented with arginine, glutamine, and glycine subjected to a leaky gut model. Poult. Sci. 98, 6761–6771. doi: 10.3382/ps/pez393
Barot, M. S., Mosenthal, A. C., and Bokkenheuser, V. D. (1983). Location of Campylobacter jejuni in infected chicken livers. J. Clin. Microbiol. 17, 921–922. doi: 10.1128/jcm.17.5.921-922.1983
Berrang, M. E., Meinersmann, R. J., and Cox, N. A. (2019). Campylobacter subtypes detected in broiler ceca and livers collected at slaughter1. Poult. Sci. 98, 5908–5912. doi: 10.3382/ps/pez340
Berrang, M. E., Meinersmann, R. J., Cox, N. A., and Thompson, T. M. (2018). Multilocus sequence subtypes of Campylobacter detected on the surface and from internal tissues of retail chicken livers. J. Food Prot. 81, 1535–1539. doi: 10.4315/0362-028X.JFP-18-131
Black, R. E., Levine, M. M., Clements, M. L., Hughes, T. P., and Blaser, M. J. (1988). Experimental Campylobacter jejuni infection in humans. J. Infect. Dis. 157, 472–479. doi: 10.1093/infdis/157.3.472
Burakoff, A., Brown, K., Knutsen, J., Hopewell, C., Rowe, S., Bennett, C., et al. (2018). Outbreak of fluoroquinolone-resistant Campylobacter jejuni infections associated with raw Milk consumption from a Herdshare dairy-Colorado, 2016. MMWR Morb. Mortal. Wkly Rep. 67, 146–148. doi: 10.15585/mmwr.mm6705a2
Calderón-Gómez, L. I., Hartley, L. E., McCormack, A., Ringoir, D. D., and Korolik, V. (2009). Potential use of characterised hyper-colonising strain(s) of Campylobacter jejuni to reduce circulation of environmental strains in commercial poultry. Vet. Microbiol. 134, 353–361. doi: 10.1016/j.vetmic.2008.09.055
Centers for Disease Control and Prevention (CDC) (2002). Outbreak of Campylobacter jejuni infections associated with drinking unpasteurized milk procured through a cow-leasing program--Wisconsin, 2001. MMWR Morb. Mortal. Wkly Rep. 51, 548–549.
Chaloner, G., Wigley, P., Humphrey, S., Kemmett, K., Lacharme-Lora, L., Humphrey, T., et al. (2014). Dynamics of dual infection with Campylobacter jejuni strains in chickens reveals distinct strain-to-strain variation in infection ecology. Appl. Environ. Microbiol. 80, 6366–6372. doi: 10.1128/AEM.01901-14
Clark, C. G., Berry, C., Walker, M., Petkau, A., Barker, D. O. R., Guan, C., et al. (2016). Genomic insights from whole genome sequencing of four clonal outbreak Campylobacter jejuni assessed within the global C. jejuni population. BMC Genomics 17:990. doi: 10.1186/s12864-016-3340-8
Coward, C., van Diemen, P. M., Conlan, A. J. K., Gog, J. R., Stevens, M. P., Jones, M. A., et al. (2008). Competing isogenic Campylobacter strains exhibit variable population structures in vivo. Appl. Environ. Microbiol. 74, 3857–3867. doi: 10.1128/AEM.02835-07
Cox, N. A., Hofacre, C. L., Bailey, J. S., Buhr, R. J., Wilson, J. L., Hiett, K. L., et al. (2005). Presence of Campylobacter jejuni in various organs one hour, one day, and one week following oral or intracloacal inoculations of broiler chicks. Avian Dis. 49, 155–158. doi: 10.1637/7234-070704r
Cox, N. A., Richardson, L. J., Buhr, R. J., Northcutt, J. K., Bailey, J. S., Cray, P. F., et al. (2007). Recovery of Campylobacter and Salmonella serovars from the spleen, liver and gallbladder, and ceca of six-and eight-week-old commercial broilers. J. Appl. Poult. Res. 16, 477–480. doi: 10.3382/japr.2006-00123
Dhillon, A. S., Shivaprasad, H. L., Schaberg, D., Wier, F., Weber, S., and Bandli, D. (2006). Campylobacter jejuni infection in broiler chickens. Avian Dis. 50, 55–58. doi: 10.1637/7411-071405R.1
Domingues, A. R., Pires, S. M., Halasa, T., and Hald, T. (2012). Source attribution of human campylobacteriosis using a meta-analysis of case-control studies of sporadic infections. Epidemiol. Infect. 140, 970–981. doi: 10.1017/S0950268811002676
Ellerbroek, L. I., Lienau, J.-A., and Klein, G. (2010). Campylobacter spp. in broiler flocks at farm level and the potential for cross-contamination during slaughter. Zoonoses Public Health 57, e81–e88. doi: 10.1111/j.1863-2378.2009.01267.x
European Food Safety Authority and European Centre for Disease Prevention and Control (2021). The European Union one health 2019 Zoonoses report. EFS2 19:e06406. doi: 10.2903/j.efsa.2021.6406
Facciolà, A., Riso, R., Avventuroso, E., Visalli, G., Delia, S. A., and Laganà, P. (2017). Campylobacter: from microbiology to prevention. J. Prev. Med. Hyg. 58, E79–E92.
Firlieyanti, A. S., Connerton, P. L., and Connerton, I. F. (2016). Campylobacters and their bacteriophages from chicken liver: the prospect for phage biocontrol. Int. J. Food Microbiol. 237, 121–127. doi: 10.1016/j.ijfoodmicro.2016.08.026
Gharib Naseri, K., Rahimi, S., and Khaki, P. (2012). Comparison of the effects of probiotic, organic acid and medicinal plant on Campylobacter jejuni challenged broiler chickens. J. Agric. Sci. Technol. 14, 1485–1496.
Greig, J. D., and Ravel, A. (2009). Analysis of foodborne outbreak data reported internationally for source attribution. Int. J. Food Microbiol. 130, 77–87. doi: 10.1016/j.ijfoodmicro.2008.12.031
Han, Z., Pielsticker, C., Gerzova, L., Rychlik, I., and Rautenschlein, S. (2016). The influence of age on Campylobacter jejuni infection in chicken. Dev. Comp. Immunol. 62, 58–71. doi: 10.1016/j.dci.2016.04.020
Harrison, D., Corry, J., Tchórzewska, M. A., Morris, V. K., and Hutchison, M. L. (2013). Freezing as an intervention to reduce the numbers of Campylobacters isolated from chicken livers. Lett. Appl. Microbiol. 57, 206–213. doi: 10.1111/lam.12098
Hermans, D., van Deun, K., Martel, A., van Immerseel, F., Messens, W., Heyndrickx, M., et al. (2011). Colonization factors of Campylobacter jejuni in the chicken gut. Vet. Res. 42:82. doi: 10.1186/1297-9716-42-82
Hiett, K. L., Stern, N. J., Fedorka-Cray, P., Cox, N. A., Musgrove, M. T., and Ladely, S. (2002). Molecular subtype analyses of Campylobacter spp. from Arkansas and California poultry operations. Appl. Environ. Microbiol. 68, 6220–6236. doi: 10.1128/AEM.68.12.6220-6236.2002
Jennings, J. L., Sait, L. C., Perrett, C. A., Foster, C., Williams, L. K., Humphrey, T. J., et al. (2011). Campylobacter jejuni is associated with, but not sufficient to cause vibrionic hepatitis in chickens. Vet. Microbiol. 149, 193–199. doi: 10.1016/j.vetmic.2010.11.005
Kaakoush, N. O., Castaño-Rodríguez, N., Mitchell, H. M., and Man, S. M. (2015). Global epidemiology of Campylobacter infection. Clin. Microbiol. Rev. 28, 687–720. doi: 10.1128/CMR.00006-15
Knudsen, K. N., Bang, D. D., Andresen, L. O., and Madsen, M. (2006). Campylobacter jejuni strains of human and chicken origin are invasive in chickens after Oral challenge. Avian Dis. 50, 10–14. doi: 10.1637/7376-051005R.1
Konkel, M. E., Christensen, J. E., Dhillon, A. S., Lane, A. B., Hare-Sanford, R., Schaberg, D. M., et al. (2007). Campylobacter jejuni strains compete for colonization in broiler chicks. Appl. Environ. Microbiol. 73, 2297–2305. doi: 10.1128/AEM.02193-06
Korolik, V., Alderton, M. R., Smith, S. C., Chang, J., and Coloe, P. J. (1998). Isolation and molecular analysis of colonising and non-colonising strains of Campylobacter jejuni and Campylobacter coli following experimental infection of young chickens. Vet. Microbiol. 60, 239–249. doi: 10.1016/s0378-1135(98)00145-x
Laisney, M. J., Gillard, M. O., and Salvat, G. (2004). Influence of bird strain on competitive exclusion of Campylobacter jejuni in young chicks. Br. Poult. Sci. 45, 49–54. doi: 10.1080/00071660410001668851
Lamb-Rosteski, J. M., Kalischuk, L. D., Inglis, G. D., and Buret, A. G. (2008). Epidermal growth factor inhibits Campylobacter jejuni-induced Claudin-4 disruption, loss of epithelial barrier function, and Escherichia coli translocation. Infect. Immun. 76, 3390–3398. doi: 10.1128/IAI.01698-07
Lanier, W. A., Hale, K. R., Geissler, A. L., and Dewey-Mattia, D. (2018). Chicken liver–associated outbreaks of Campylobacteriosis and salmonellosis, United States, 2000–2016: identifying opportunities for prevention. Foodborne Pathog. Dis. 15, 726–733. doi: 10.1089/fpd.2018.2489
Little, C. L., Gormley, F. J., Rawal, N., and Richardson, J. F. (2010). A recipe for disaster: outbreaks of campylobacteriosis associated with poultry liver pâté in England and Wales. Epidemiol. Infect. 138, 1691–1694. doi: 10.1017/S0950268810001974
Livak, K. J., and Schmittgen, T. D. (2001). Analysis of relative gene expression data using real-time quantitative PCR and the 2(-Delta Delta C(T)) method. Methods San Diego Calif 25, 402–408. doi: 10.1006/meth.2001.1262
Llarena, A.-K., and Kivistö, R. (2020). Human Campylobacteriosis cases traceable to chicken meat—evidence for disseminated outbreaks in Finland. Pathogens 9:868. doi: 10.3390/pathogens9110868
Llarena, A.-K., Zhang, J., Vehkala, M., Välimäki, N., Hakkinen, M., Hänninen, M.-L., et al. (2016). Monomorphic genotypes within a generalist lineage of Campylobacter jejuni show signs of global dispersion. Microb Genom 2:e000088. doi: 10.1099/mgen.0.000088
Messens, W., Herman, L., De Zutter, L., and Heyndrickx, M. (2009). Multiple typing for the epidemiological study of contamination of broilers with thermotolerant Campylobacter. Vet. Microbiol. 138, 120–131. doi: 10.1016/j.vetmic.2009.02.012
Moffatt, C. R. M., Greig, A., Valcanis, M., Gao, W., Seemann, T., Howden, B. P., et al. (2016). A large outbreak of Campylobacter jejuni infection in a university college caused by chicken liver pâté, Australia, 2013. Epidemiol. Infect. 144, 2971–2978. doi: 10.1017/S0950268816001187
Nadeau, É., Messier, S., and Quessy, S. (2002). Prevalence and comparison of genetic profiles of Campylobacter strains isolated from poultry and sporadic cases of Campylobacteriosis in humans. J. Food Prot. 65, 73–78. doi: 10.4315/0362-028X-65.1.73
Nennig, M., Clément, A., Longueval, E., Bernardi, T., Ragimbeau, C., and Tresse, O. (2022). Metaphenotypes associated with recurrent genomic lineages of Campylobacter jejuni responsible for human infections in Luxembourg. Front. Microbiol. 13:901192. doi: 10.3389/fmicb.2022.901192
Newell, D. G., and Fearnley, C. (2003). Sources of Campylobacter colonization in broiler chickens. Appl. Environ. Microbiol. 69, 4343–4351. doi: 10.1128/AEM.69.8.4343-4351.2003
Newell, D. G., Shreeve, J. E., Toszeghy, M., Domingue, G., Bull, S., Humphrey, T., et al. (2001). Changes in the carriage of Campylobacter strains by poultry carcasses during processing in abattoirs. Appl. Environ. Microbiol. 67, 2636–2640. doi: 10.1128/AEM.67.6.2636-2640.2001
Noormohamed, A., and Fakhr, M. K. (2012). Incidence and antimicrobial resistance profiling of Campylobacter in retail chicken livers and gizzards. Foodborne Pathog. Dis. 9, 617–624. doi: 10.1089/fpd.2011.1074
Pielsticker, C., Glünder, G., Aung, Y. H., and Rautenschlein, S. (2016). Colonization pattern of C. jejuni isolates of human and avian origin and differences in the induction of immune responses in chicken. Vet. Immunol. Immunopathol. 169, 1–9. doi: 10.1016/j.vetimm.2015.11.005
Public Health Agency of Canada (2021). Food net Canada annual report 2018. Available at: https://www.canada.ca/en/public-health/services/surveillance/foodnet-canada/publications/foodnet-canada-annual-report-2018.html (Accessed December 14, 2022).
Richardson, L. J., Cox, N. A., Buhr, R. J., and Harrison, M. A. (2011). Isolation of Campylobacter from circulating blood of commercial broilers. Avian Dis. 55, 375–378. doi: 10.1637/9613-121310-Reg.1
Ring, M., Zychowska, M. A., and Stephan, R. (2005). Dynamics of Campylobacter spp. spread investigated in 14 broiler flocks in Switzerland. Avian Dis. 49, 390–396. doi: 10.1637/7319-010305R1.1
Ringoir, D. D., and Korolik, V. (2003). Colonisation phenotype and colonisation potential differences in Campylobacter jejuni strains in chickens before and after passage in vivo. Vet. Microbiol. 92, 225–235. doi: 10.1016/S0378-1135(02)00378-4
Robinson, D. A. (1981). Infective dose of Campylobacter jejuni in milk. Br. Med. J. Clin. Res. Ed. 282:1584. doi: 10.1136/bmj.282.6276.1584
Ruiz-Palacios, G. M., Escamilla, E., and Torres, N. (1981). Experimental Campylobacter diarrhea in chickens. Infect. Immun. 34, 250–255. doi: 10.1128/iai.34.1.250-255.1981
Rzeznitzeck, J., Breves, G., Rychlik, I., Hoerr, F. J., von Altrock, A., Rath, A., et al. (2022). The effect of Campylobacter jejuni and Campylobacter coli colonization on the gut morphology, functional integrity, and microbiota composition of female turkeys. Gut Pathog. 14:33. doi: 10.1186/s13099-022-00508-x
Sahin, O., Kassem, I. I., Shen, Z., Lin, J., Rajashekara, G., and Zhang, Q. (2015). Campylobacter in poultry: ecology and potential interventions. Avian Dis. 59, 185–200. doi: 10.1637/11072-032315-Review
Sahin, O., Luo, N., Huang, S., and Zhang, Q. (2003). Effect of Campylobacter-specific maternal antibodies on Campylobacter jejuni colonization in young chickens. Appl. Environ. Microbiol. 69, 5372–5379. doi: 10.1128/AEM.69.9.5372-5379.2003
St. Paul, M., Mallick, A. I., Haq, K., Orouji, S., Abdul-Careem, M. F., and Sharif, S. (2011). In vivo administration of ligands for chicken toll-like receptors 4 and 21 induces the expression of immune system genes in the spleen. Vet. Immunol. Immunopathol. 144, 228–237. doi: 10.1016/j.vetimm.2011.09.004
Stern, N. J., Cox, N. A., Musgrove, M. T., and Park, C. M. (2001). Incidence and levels of Campylobacter in broilers after exposure to an inoculated seeder bird. J. Appl. Poult. Res. 10, 315–318. doi: 10.1093/japr/10.4.315
Thibodeau, A., Fravalo, P., Taboada, E. N., Laurent-Lewandowski, S., Guévremont, E., Quessy, S., et al. (2015a). Extensive characterization of Campylobacter jejuni chicken isolates to uncover genes involved in the ability to compete for gut colonization. BMC Microbiol. 15:97. doi: 10.1186/s12866-015-0433-5
Thibodeau, A., Fravalo, P., Yergeau, É., Arsenault, J., Lahaye, L., and Letellier, A. (2015b). Chicken Caecal microbiome modifications induced by Campylobacter jejuni colonization and by a non-antibiotic feed additive. PLoS One 10:e0131978. doi: 10.1371/journal.pone.0131978
Thomas, L. M., Long, K. A., Good, R. T., Panaccio, M., and Widders, P. R. (1997). Genotypic diversity among Campylobacter jejuni isolates in a commercial broiler flock. Appl. Environ. Microbiol. 63, 1874–1877. doi: 10.1128/aem.63.5.1874-1877.1997
van Gerwe, T., Miflin, J. K., Templeton, J. M., Bouma, A., Wagenaar, J. A., Jacobs-Reitsma, W. F., et al. (2009). Quantifying transmission of Campylobacter jejuni in commercial broiler flocks. Appl. Environ. Microbiol. 75, 625–628. doi: 10.1128/AEM.01912-08
von Buchholz, J. S., Bilic, I., Aschenbach, J. R., Hess, M., Mitra, T., and Awad, W. A. (2021). Establishment of a novel probe-based RT-qPCR approach for detection and quantification of tight junctions reveals age-related changes in the gut barriers of broiler chickens. PLoS One 16:e0248165. doi: 10.1371/journal.pone.0248165
von Buchholz, J. S., Ruhnau, D., Hess, C., Aschenbach, J. R., Hess, M., and Awad, W. A. (2022). Paracellular intestinal permeability of chickens induced by DON and/or C. jejuni is associated with alterations in tight junction mRNA expression. Microb. Pathog. 168:105509. doi: 10.1016/j.micpath.2022.105509
Wang, G., He, Y., Jin, X., Zhou, Y., Chen, X., Zhao, J., et al. (2018). The effect of co-infection of food-borne pathogenic bacteria on the progression of Campylobacter jejuni infection in mice. Front. Microbiol. 9:1977. doi: 10.3389/fmicb.2018.01977
Wassenaar, T. M., and Newell, D. G. (2000). Genotyping of Campylobacter spp. Appl. Environ. Microbiol. 66, 1–9. doi: 10.1128/AEM.66.1.1-9.2000
Whyte, R., Hudson, J., and Graham, C. (2006). Campylobacter in chicken livers and their destruction by pan frying. Lett. Appl. Microbiol. 43, 591–595. doi: 10.1111/j.1472-765X.2006.02020.x
Yan, W., Zhou, Q., Yuan, Z., Fu, L., Wen, C., Yang, N., et al. (2021). Impact of the gut microecology on Campylobacter presence revealed by comparisons of the gut microbiota from chickens raised on litter or in individual cages. BMC Microbiol. 21:290. doi: 10.1186/s12866-021-02353-5
Young, C. R., Ziprin, R. L., Hume, M. E., and Stanker, L. H. (1999). Dose response and organ invasion of day-of-hatch Leghorn chicks by different isolates of Campylobacter jejuni. Avian Dis. 43, 763–767. doi: 10.2307/1592745
Keywords: Campylobacter jejuni, broiler chickens, gut colonization, extraintestinal dissemination, commensalism, competition, tight junction proteins
Citation: Chagneau S, Gaucher M-L, Thériault WP, Fravalo P and Thibodeau A (2023) Observations supporting hypothetical commensalism and competition between two Campylobacter jejuni strains colonizing the broiler chicken gut. Front. Microbiol. 13:1071175. doi: 10.3389/fmicb.2022.1071175
Edited by:
François J. M. A. Meurens, INRA Ecole Nationale Vétérinaire, Agroalimentaire et de l'alimentation de Nantes-Atlantique (Oniris), FranceReviewed by:
Geertrui Rasschaert, Institute for Agricultural, Fisheries, and Food Research (ILVO), BelgiumJean-Michel Cappelier, French National Institute forAgricultural Research (INRA), France
Copyright © 2023 Chagneau, Gaucher, Thériault, Fravalo and Thibodeau. This is an open-access article distributed under the terms of the Creative Commons Attribution License (CC BY). The use, distribution or reproduction in other forums is permitted, provided the original author(s) and the copyright owner(s) are credited and that the original publication in this journal is cited, in accordance with accepted academic practice. No use, distribution or reproduction is permitted which does not comply with these terms.
*Correspondence: Sophie Chagneau, ✉ c29waGllLmNoYWduZWF1QHVtb250cmVhbC5jYQ==; Alexandre Thibodeau, ✉ YWxleGFuZHJlLnRoaWJvZGVhdUB1bW9udHJlYWwuY2E=