- 1School of Clinical Medicine, Tsinghua University, Beijing, China
- 2Department of Obstetrics and Gynecology, Beijing Tsinghua Changgung Hospital, School of Clinical Medicine, Tsinghua University, Beijing, China
Objective: This study aimed to compare the dynamics of lower and upper genital tract microbiota in normal term pregnancy, histological chorioamnionitis (HCA), and clinical chorioamnionitis (CCA) patients to provide a reference for the diagnosis and treatment of chorioamnionitis (CAM) patients.
Methods: We prospectively collected vaginal and cervical secretions, as well as placenta tissues, fetal membranes, and amniotic fluid from normal-term pregnant women, HCA and CCA patients. Then, we performed genomic DNA extraction and PCR amplification for all samples. The eligible samples were analyzed by 16S ribosomal RNA (16S rRNA) sequencing. Additionally, all placenta tissues were histopathologically examined, and neonatal pharyngeal swabs and placenta tissues from the HCA and CCA groups were subjected to microbial culture.
Results: A total of 85 term pregnant women were enrolled in this study, including 34 in the normal group (N), 37 in the HCA group, and 14 in the CCA group. A total of 171 qualified samples were analyzed by 16S rRNA sequencing. The results suggested that the cervical microbiota was highly similar to the vaginal microbiota in normal term parturients, with Lactobacillus as the dominant bacterium. Moreover, there was no difference in the alpha and beta diversity of vaginal microbiota between the N, HCA, and CCA groups at the genus level. Besides, no significant differences were detected in cervical microbiome among the three groups. Regarding intrauterine microorganisms, the N and HCA groups had similar microbial composition but were different from the CCA group. No microbe was detected in the placental tissue of normal term parturients, while some microorganisms were found in the intrauterine amniotic fluid and fetal membrane samples. Regardless of cultivation or 16S rRNA sequencing, an extremely low microbial positive rate was detected in HCA and CCA intrauterine samples. Compared to the normal group, Lactobacillus was significantly reduced in the CCA group intrauterine, and Ureaplasma and Enterococcus increased with no statistically significant.
Conclusion: The N, HCA and CCA groups had similar composition of vaginal and cervical microflora. Some normal-term pregnant women can harbor non-pathogenic microbiota in the uterine cavity. Sterile inflammation is more frequent than microbial-associated inflammation in term HCA and CCA parturients.
Introduction
Chorioamnionitis (CAM) or intraamniotic infection is a common pregnancy complication. It comprehends an inflammation or infection of the fetal membrane, amniotic fluid, placenta, umbilical cord, or any part of the fetus (Committee on Obstetric Practice, 2017). According to the existence of clinical manifestations, CAM is divided into clinical chorioamnionitis (CCA) and histological chorioamnionitis (HCA; Beck et al., 2021). Additionally, CCA is characterized by the following clinical symptoms: maternal fever, uterine tenderness, maternal and/or fetal tachycardia, maternal leukocytosis, and foul-smelling or purulent amniotic fluid (Tita and Andrews, 2010). Moreover, HCA (also known as subclinical chorioamnionitis) is more common than CCA and is defined by diffuse infiltration of neutrophils into the chorioamniotic membranes (Levy et al., 2021).
Traditionally, it was assumed that the healthy uterus and intrauterine environment (placenta, chorion/amnion, amniotic fluid, fetus, and meconium) were sterile during pregnancy, and CAM was often associated with bacterial invasion. Currently, the concept of the “sterile womb” means that microbes can be acquired both vertically (from the mother) and horizontally (from other humans or the environment) during and after birth (Funkhouser and Bordenstein, 2013). However, due to the development of high-throughput sequencing technology, this view has been unprecedentedly challenged. First, Aagaard et al. (2014) have demonstrated that the placenta of healthy pregnant women harbors a unique low-abundance placental microbiome by 16S ribosomal DNA and whole-genome shotgun (WGS) metagenomic sequencing. Further, many studies have challenged the sterility of the intrauterine environment during pregnancy (Seferovic et al., 2019; Stinson et al., 2019; Ennamorati et al., 2020; Hockney et al., 2020; Rackaityte et al., 2020; Mishra et al., 2021). For example, Tuominen et al. (2019) have shown that the neonatal oral cavity microbiota seems to share common characteristics with the microbes in the placenta but not the birth canal, indicating that the neonatal oral microbiota might have a prenatal origin. Moreover, Rackaityte et al. (2020) studied meconium samples in the mid-gestation and found that 18 taxa were enriched in the fetal meconium, especially Micrococcaceae and Lactobacillus. This view is supported by Mishra et al. (2021) who identified several live bacterial strains including Staphylococcus and Lactobacillus in fetal tissues, which induced in vitro activation of memory T cells in fetal mesenteric lymph node, supporting the role of microbial exposure in fetal immune initiation. Whereas, it is debated whether the microbiome detected during reproduction, especially low-biomass microbial communities, are in fact merely a result of contamination and an artifact of the study design (Lauder et al., 2016; Kim et al., 2017). Besides, increasing studies have shown a high incidence of sterile inflammation, that is, when microorganisms cannot be detected, in preterm or term CAM patients. Thus, the expert panel of the National Institute of Child Health and Human Development (NICHD) has proposed a descriptive term: “intrauterine inflammation or infection or both,” abbreviated as “Triple I,” to replace chorioamnionitis (Higgins et al., 2016). Romero et al., (2014b) analyzed amniotic fluid samples from 135 premature women with intact membranes and demonstrated that sterile intra-amniotic inflammation is more frequent than microbial-associated inflammation. Additionally, a retrospective cohort study has suggested that 24% of preterm CCA patients had no evidence of intraamniotic infection or inflammation, and 66% had negative amniotic fluid cultures (Oh et al., 2017).
A better understanding of the composition and alterations of the upper and lower genital tract microbiome might help develop better treatment strategies for CAM either in maternal or infants. Therefore, this study aimed to: (a) Quantify the bacterial load and diversity in the vagina, cervix, and uterine cavity of normal term parturients and (b) compare the microbiota composition of the upper and lower genital tracts of normal, HCA, and CCA in term pregnancy.
Materials and methods
Study design and enrolment
We prospectively collected vaginal and cervical secretions, as well as placenta tissue, fetal membranes, and amniotic fluid from term pregnant women who delivered at Beijing Tsinghua Changgung Hospital between March 1 and September 30, 2019. Before beginning the study, ethical clearance was granted by the Ethics Committee of Beijing Tsinghua Changgung Hospital (16121-0110). The inclusion and exclusion criteria are presented in Table 1. According to the clinical manifestations and histopathological examination results, patients were divided into the normal pregnancy (N), CCA, and HCA groups. Simultaneously, we collected clinical information from electronic medical records, mainly including maternal age, body mass index (BMI), weight gain during labor, fetal heart rate (FHR), maternal peripheral blood white blood cells (WBC), and C-reactive protein (CRP), birth weight, and neonatal infection.
Sample collection
Before planning induced labor or cesarean sections, samples were taken with the patient’s consent. Vaginal secretions were obtained from the lateral wall of the upper 1/3 of the vagina, and a cervical swab was taken from 2 to 3 cm inside the cervical canal. All intrauterine tissue samples were collected following strict and uniform protocols established before the study begins using standard operating procedures by a specially trained physician. During the operation, samples were taken immediately after delivery from participants, and strict aseptic operation was performed. Then, samples were placed in sterile ice-cold phosphate-buffered saline solution (1 × PBS) and stored at −80°C within 1 h. About 2–5 ml of amniotic fluid was absorbed from the neonatal oropharynx in a sterile tube for subsequent experiments.
Placental histopathologic examination
At the same time, another placental tissue was collected, fixed with 10% neutral buffered formalin, embedded in paraffin wax, and stained with hematoxylin and eosin (H&E) for histopathological examination. The diagnosis of pathological sections was assessed by two gynecological/placental histopathologists without knowing the clinical data. As previously mentioned, HCA staging was based on the area of neutrophil infiltration in the tissue anatomy, and the grading was based on the degree of neutrophil infiltration in a particular area (Zheng et al., 2013). Simply speaking, staging is based on the area of neutrophil infiltration in tissue anatomy, and grading is based on the degree of neutrophil infiltration in a particular area.
Bacterial cultures
Neonatal oropharyngeal swabs and placental grind fluid were inoculated on Columbia blood agar plates using the four-zone method. Then, blood plates were cultured in aerobic and anaerobic environments for 18–24 h, respectively. Colonies were identified by matrix-assisted laser desorption ionization time-of-flight mass spectrometry (MALDI-TOF MS).
Genomic DNA extraction and PCR amplification
Microbial community genomic DNA was extracted from above mentioned samples (vaginal and cervical secretions, placenta, fetal membrane and amniotic fluid) using the TIANamp Bacteria DNA Kit (TIANGEN BIOTECH, Beijing, China) according to manufacturer’s instructions. The DNA extract was checked on 1% agarose gel, and DNA concentration and purity were determined with NanoDrop 2000 UV–vis spectrophotometer (Thermo Scientific, Wilmington, USA). Then, the hypervariable region V3-V4 of the bacterial 16S rRNA gene were amplified with primer pairs 338F(5’-ACTCCTACGGGAGGCAGCAG-3′) and 806R(5’-GGACTACHVGGGTWTCTAAT-3′) by an ABI GeneAmp® 9,700 PCR thermocycler (ABI, CA, USA). The PCR product was extracted from 2% agarose gel and purified using the AxyPrep DNA Gel Extraction Kit (Axygen Biosciences, Union City, CA, USA) according to manufacturer’s instructions and quantified using Quantus™ Fluorometer (Promega, USA). All the amplification products were stored at −20°C for later sequencing.
Illumina read data processing and analysis
Purified amplicons were pooled in equimolar and paired-end sequenced on an Illumina MiSeq PE300 platform/NovaSeq PE250 platform (Illumina, San Diego, USA) according to the standard protocols by Majorbio Bio-Pharm Technology Co. Ltd. (Shanghai, China). The raw 16S rRNA gene sequencing reads were demultiplexed, quality-filtered by fastp version 0.20.0 (Chen et al., 2018) and merged by FLASH version 1.2.7 (Magoč and Salzberg, 2011). Operational taxonomic units (OTUs) with 97% similarity cutoff (Stackebrandt and Goebel, 1994; Edgar, 2013) were clustered using UPARSE version 7.1 (Edgar, 2013), and chimeric sequences were identified and removed. The taxonomy of each OTU representative sequence was analyzed by RDP Classifier version 2.2 (Wang et al., 2007) against the 16S rRNA database (e.g. Silva v138) using confidence threshold of 0.7.
Bioinformatics and statistical analysis
SPSS 23.0 (IBM Corporation, USA) software and R (v. 3.5.1) was used to process data, measurement data were expressed as mean ± standard deviation (x ± s), paired sample means were compared using paired T test, multiple sample means were compared by one-way ANOVA, and measurement data were compared using χ2 test. p < 0.05 was considered statistically significant. Biology statistical methods using multivariate statistical analysis, including: analysis of variance, rank and inspection and ternary phase diagram.
Results
Patient characteristics
A total of 85 term pregnant women were enrolled in this study, including 34 in the N group, 37 in the HCA group, and 14 in the CCA group. The average age of pregnant women was 30.08 ± 2.77 years old, and the average gestational age was 39.7 ± 1.0 weeks. At the end of the research, 85 neonates (42 males and 43 females) were delivered without neonatal asphyxia or perinatal death. Clinical characteristics are shown in Table 2. No differences were identified between groups regarding age, maternal weight gain, number of deliveries, and birth weight. It is apparent from Table 2 that the gestational age of the N group was smaller than the CCA and HCA groups, and the BMI of the CCA group was higher compared to other groups. Closer inspection of the table shows the WBC, CRP of pregnant women, fetal distress, neonatal infection, and transfer rate to the neonatal department were also higher in the CCA group compared to the N and HCA groups, and there was no significant difference between N and HCA group.
Pathological examination
All the placentas of 85 pregnant women were sent for histopathological examination. Partial pathological results were shown in Figure 1, and there were no stage III patients were detected in the HCA and CCA groups. In HCA group, 21 patients diagnosed stage I and 16 patients diagnosed stage II. Interestingly, there were 4 patients had normal placental pathology in CCA group. Of the remaining 10 patients, 9 were stage II and 1 was stage I.
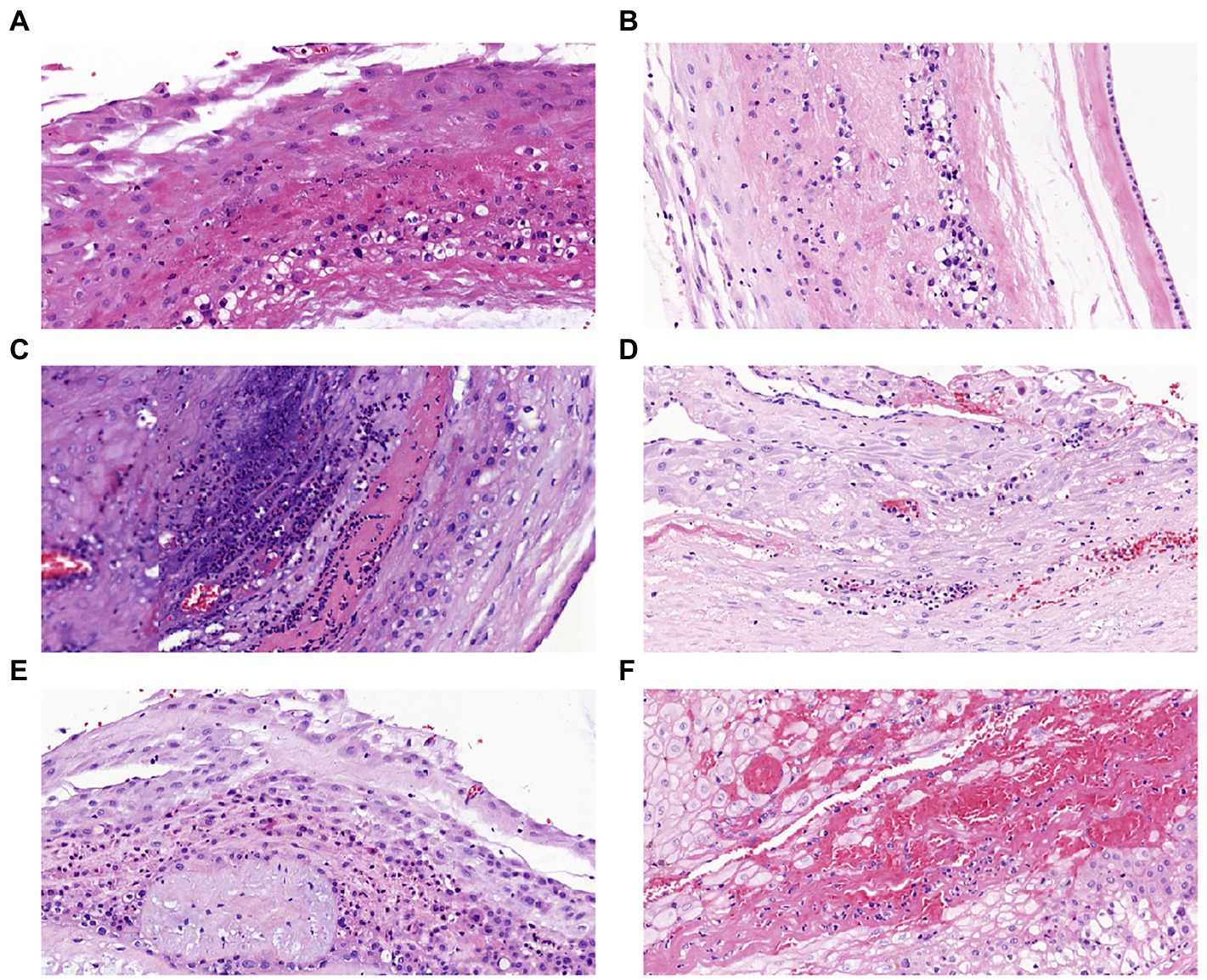
Figure 1. Placental histopathology chorioamnionitis (H&E × 200): (A) Stage I grade I, (B) Stage I grade II, (C) Stage I grade III, (D) Stage II grade I, (E) Stage II grade II, (F) Stage II grade III.
Microbiologic cultures
In the HCA group, the negative rate of neonatal pharyngeal swabs was 83.3% (5/6), and no bacteria could be cultured from placenta tissues (0/11), and Escherichia coli and Enterococcus faecalis were detected in positive pharyngeal specimens. Next, in the CCA group, the negative rate for neonatal specimens was 58.3% (7/12) and for placental tissues was 64.3% (9/14). Similar to the HCA group, except the above two bacteria, the CCA group also cultured Streptococcus agalactiae, Enterococcus faecium and Corynebacterium.
Analysis of 16S rRNA sequencing
A total of 85 × 5 (425) samples were collected during the research, of which 171 were qualified (few of them detected with bacterial DNA) for 16S rRNA sequencing (Table 3). The optimized sequence number was 8,678,452, the total number of bases was 3,709,462,018, and the average length was 427 bp, which were included in subsequent analysis. Herein, we analyzed the samples of placentae, fetal membranes, and amniotic fluid together, which are collectively referred to as intrauterine samples (marked as Up). Additionally, vaginal and cervical specimens were abbreviated as ‘V’ and ‘C’, respectively.
As can be seen from Figure 2A, the alpha diversity test (Shannon index) showed that the intrauterine bacterial diversity significantly increased compared to the vagina and cervix (p < 0.001). And there was no difference in the alpha diversity of vaginal microbiota between the N, HCA, and CCA groups at the genus level. Similarly, no significant differences were found between the N and HCA groups when the richness of the cervical microbiota was evaluated. The cervical microorganisms in the CCA group presented a clear downward trend compared to the N group (Figure 2A). The Principal Coordinates analysis (PCoA) showed that vaginal and cervical samples were clustered together with concentrated distribution and high similarity, while the intrauterine flora distribution was different from the other two groups with large dispersion and considerable differences, indicating that there were significant differences between intrauterine bacteria and vaginal and cervical bacteria (Figure 2B). The above groups of vaginal microbiota were clustered together and were highly similar (p = 0.39). Besides, no significant differences were detected in the cervical microbiome among the three groups (p = 0.88), indicating that there was no meaningful difference in the composition of cervical microbiome. Regarding the changes in intrauterine microorganisms, the normal and HCA groups were clustered together, while the CCA group was dispersed from the other two groups, implying that the composition of intrauterine bacteria in CCA was different from the other two groups and had its uniqueness (Figure 2B).
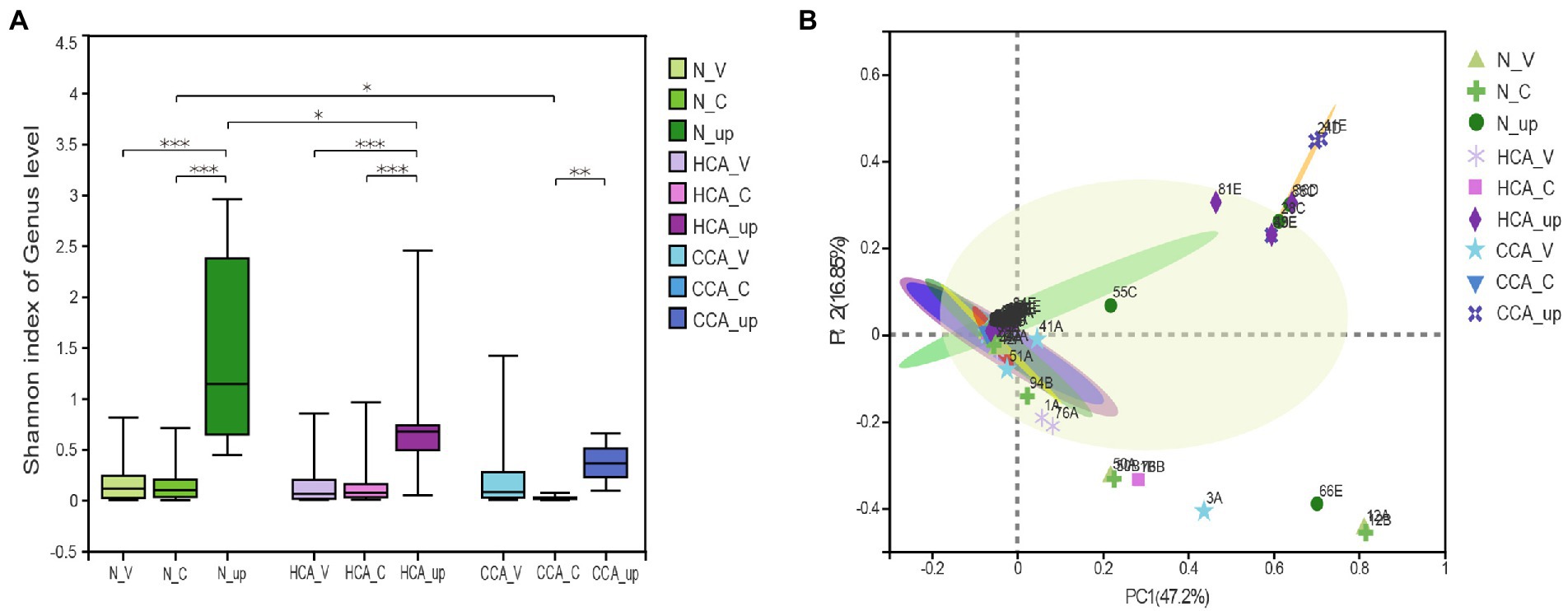
Figure 2. The diversity of the upper and lower genital tract microbiome were sequenced using 16S rRNA. (A) Alpha diversity was calculated using the Shannon diversity index in N, HCA, and CCA groups. (B) Beta-diversity was visualized using Principal Coordinate analysis (PCoA). The first two principal coordinate axes, which together explain 64.05% of variation, are shown. (*0.01 ≤ p < 0.05; **0.001 ≤ p < 0.01; ***0.0001 ≤ p < 0.001).
Next, the number of common and unique operational taxonomic units (OTUs) among these three groups is represented by a Venn diagram (Figure 3A). The number of OTUs in the N group was the highest and decreased with the aggravation of inflammation. The vaginal and cervical microbiota in the N group were similar at the genus level and mainly composed of Lactobacillus, followed by Gardnerella, Atopobium, and Streptococcus. Additionally, a significant decrease in Lactobacillus was detected in the utero accompanied by an increase in Gardnerella, Staphylococcus, Methylobacterium and other bacteria (Figure 3B). Unlike the N and HCA groups, the intrauterine flora of the CCA group was dominated by Ureaplasma spp. and Enterococcus spp.
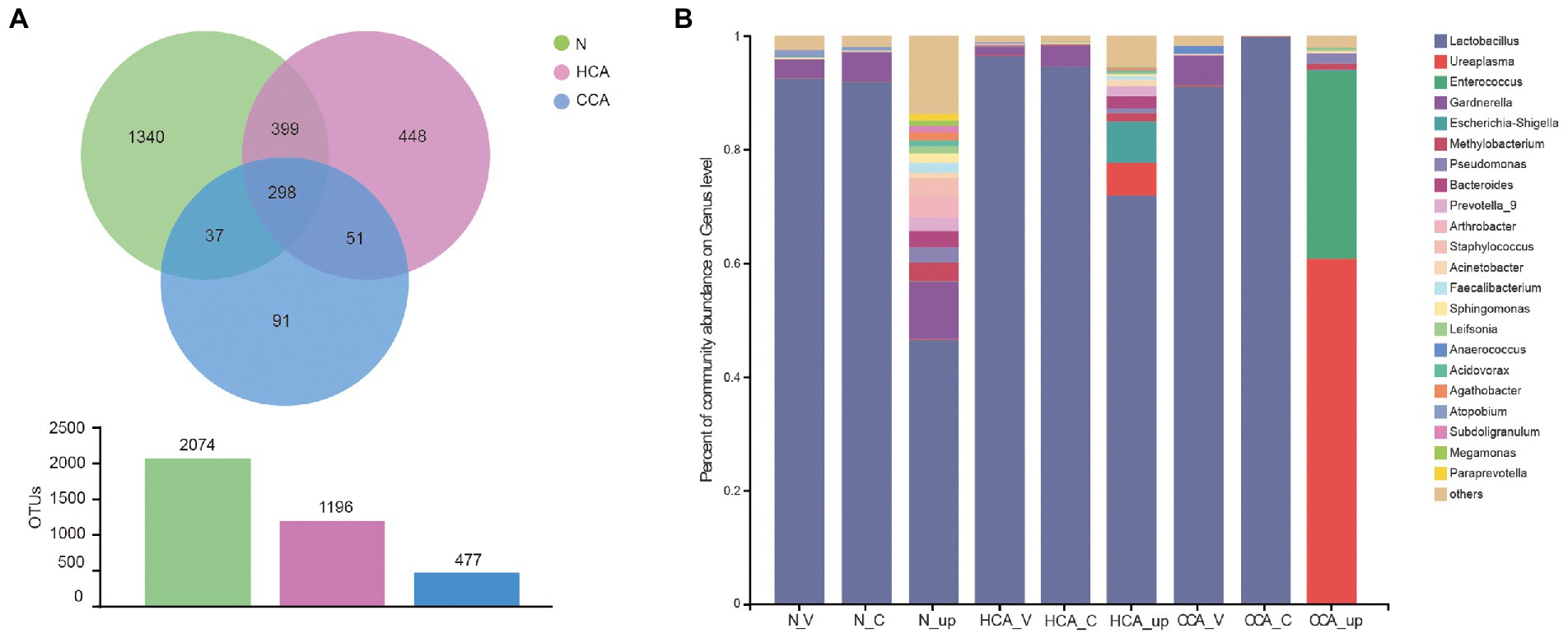
Figure 3. (A) Venn diagrams indicating the number of OTUs in the N, HCA, and CCA groups by 16S rRNA sequences; (B) Stacked bar graph showing the relative abundance of bacterial 16S rRNA sequences at genus level in N, HCA, and CCA groups.
Further studies were conducted at the species level on lower and upper genital tract of pregnant women in these three groups by clustering heatmap analysis. The results, as shown in Figure 4A, denote that the vagina and cervix of pregnant women had the highest abundance of L. crispatus and L. iners in all groups, while Lactobacillus sharply decreased in the utero of HCA and CCA groups. These differences in species can also be seen in Figure 4B. In the LEfSe analysis, significant differences in intrauterine microbial abundances were observed among these three groups. L. iners (LDA score = 5.3, p = 0.027). and L. jensenii (LDA score = 4.2, p = 0.02) were over-represented in HCA group on the specises level; Cnuella spp. (LDA score = 4.0, p = 0.03) and Asinibacterium spp. (LDA score = 4.0, p = 0.03) were over-represented in CCA group on the genus level. The most interesting aspect of Figure 4B is that Gardnerella spp. levels were significantly higher in the uterine cavity of group N (LDA score = 4.9, p = 0.017). Furthermore, the Wilcoxon rank-sum test was used to analyze the intrauterine differential microbiota of each group. No significant disparity was found between the N and HCA groups (Figure 4C). Additionally, Lactobacillus spp. (p = 0.04) and Staphylococcus spp. (p = 0.04) were notably increased in the N group compared to the CCA group, while Ureaplasma spp. and Enterococcus spp. were higher in the CCA group but without no statistical significance (p > 0.05; Figure 4D).
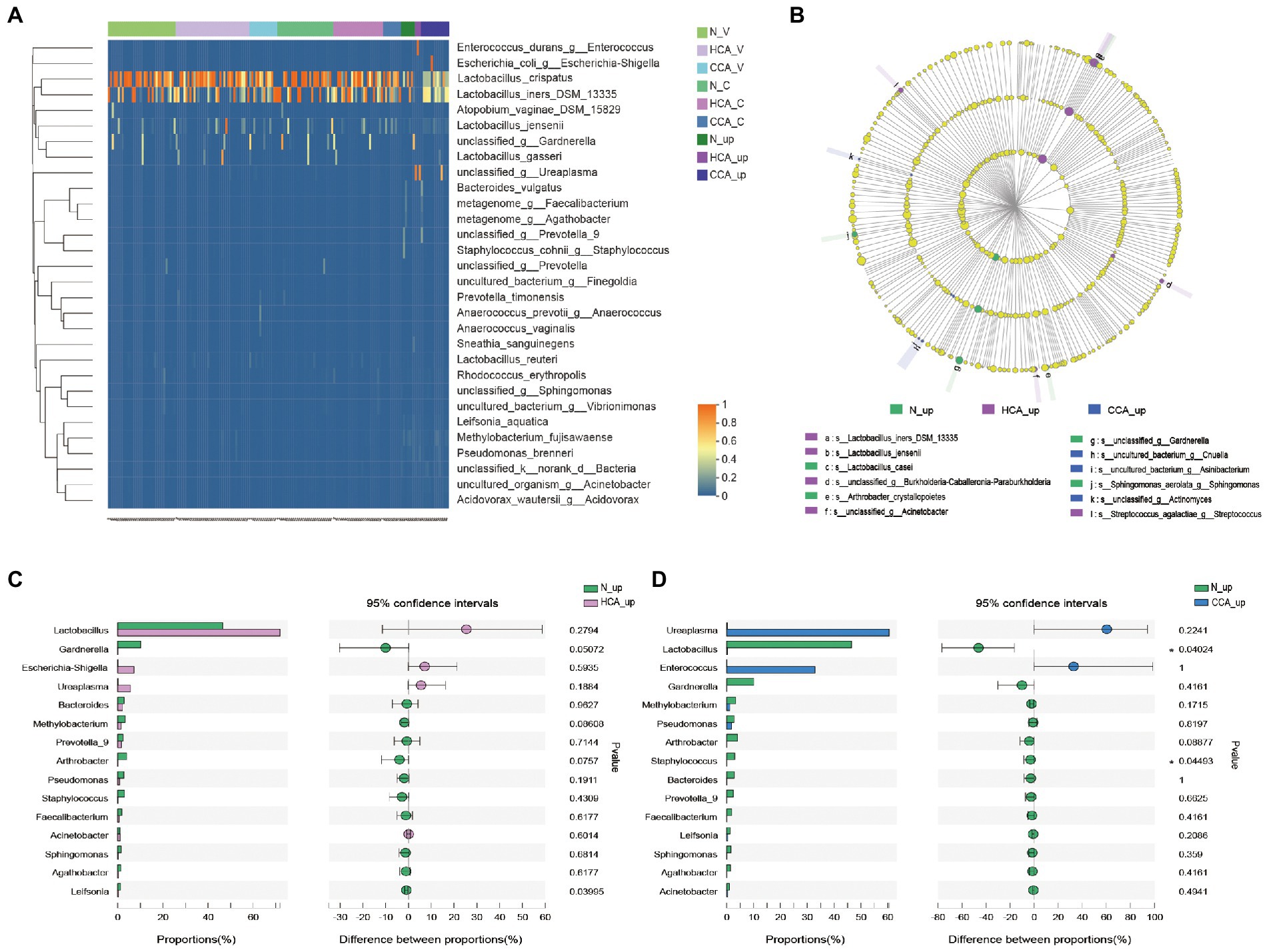
Figure 4. (A) Heatmap of upper and lower genital tract microbiota in the N, HCA, and CCA groups showing relative abundances of bacterial taxa per sample (columns). Reads were aggregated at the species level, which are shown as the different rows. (B) LEfSe analysis identified the microbes whose abundances significantly differed among N, HCA, and CCA groups. The findings with regards to family, genus and species are shown in the plot. Only species-level differences are shown in the legend. (Light yellow nodes indicate microbial taxa that are not significantly different in any of the different groups or have no significant effect on the differences between groups; different color nodes indicate microbial taxa that are significantly enriched in the corresponding groups and have a significant effect on the differences between groups). Analysis of intrauterine differential microbiota between N and HCA group (C) or between N and CCA group (D). (The X-axis represents different subgroups, and the Y-axis represents the average relative abundance of a genus in different subgroups).
Discussion
The vaginal microbiota can be categorized into one of five major community state types (CSTs), of which four community state types are dominated by Lactobacillus, namely: CST-I (L. crispatus) CST-II (L. gasseri) CST-III (L. iners) and CST-V (L. jensenii; Ravel et al., 2011). Due to the substantial increasing circulating estrogen levels in pregnancy, the physicochemical environment and microecological flora of the vagina also change accordingly (Kohlhepp et al., 2018). What’s more, high estrogen status during pregnancy is associated with proliferation of vaginal epithelial cells and glycogen deposition, which is more conducive to the dominance of Lactobacillus (Taddei et al., 2018). Similar to non-pregnant women, the vaginal microbiota of normal pregnant women is also dominated by Lactobacillus spp., and this homeostasis is stable throughout whole pregnancy (Romero et al., 2014a; McMillan et al., 2015). In our research, the vaginal flora of normal term pregnant women was consistent with previous studies. This Lactobacillus-led physiological state can resist the invasion of pathogenic microorganisms, reduce the chance of upper genital tract infection during term delivery, and maximize protecting the health of maternal and infants (Fettweis et al., 2019; Serrano et al., 2019).
Very little was found in the literature on the research of the cervical microbiome during pregnancy. Previously published studies disclose that the cervical microbiology group is analogous to the vaginal microbiome, mainly composed of Lactobacillus and Gardnerella (Smith et al., 2012). Aagaard et al. (2012) also detected that the cervical microbiota of women in the third trimester of pregnancy may be similar to non-pregnant women. In the present study, we demonstrated that the cervical microbiota was highly similar to the vaginal microbiota in normal term parturients, with Lactobacillus as the dominant bacterium (Figures 3B, 4A). Meanwhile, in the alpha and beta diversity analyzes, no statistical differences were detected in the diversity and composition of vaginal and cervical microbiota. The high consistency of these two parts provided a theoretical basis for the collection of genital tract specimens of pregnant women in clinical work. That is, the relatively simple operation and low risk of upper 1/3 vaginal secretion sampling can replace cervical secretion in clinical work.
Recently, researchers have shown an increased interest in the microecology of different parts of the human body due to the development of next-generation sequencing. The two most widely used culture-independent methods are 16S rRNA amplicon and shotgun metagenomic sequencing (Malla et al., 2018). Shotgun metagenomics allows indiscriminate sequencing of all DNA material in a sample, which can provide species-level assignment, and can provide direct evidence of functional variation in genes of existing strains. In present study, 16S sequencing was selected mainly because of its cost-effective characters and higher sensitivity to samples with human cells. In addition, it is focused on the dynamics of upper and lower genital microbiota in CAM patients rather than the relationship between microbiota and function. Besides the studies on the intrauterine microbiome mentioned in the introduction, many studies have been conducted on multiple mammals and reconfirmed the existence of a microbiome in the placenta (Younge et al., 2019; Yu et al., 2019; Zakošek Pipan et al., 2020; Bi et al., 2021). The debate about whether the intrauterine environment is sterile has gained prominence with many arguing that bacterial DNA contamination from sampling sites, clinical or laboratory environments, as well as reagents and consumables, can greatly influence the results of microbiota studies, especially for low-biomass specimen types (Salter et al., 2014). Consequently, several investigators have examined the effects of strict controls for contamination on subsequent sequencing demonstrating a sterile intrauterine environment (Leiby et al., 2018; de Goffau et al., 2019; Kuperman et al., 2020; Olomu et al., 2020; Winters et al., 2022). Furthermore, de Goffau et al. (2021) re-analyzed the data from Rackaityte et al. (2020) and provided evidence that the identification of Micrococcus in fetal gut samples was contaminated and several findings were caused by an unrecognized batch effect. In our study, no microbe was discovered in the placental tissue of 34 term normal pregnant women by 16S rRNA sequencing, which is in line with the above perspective. However, some microorganisms were detected in the intrauterine amniotic fluid and fetal membrane samples in normal group, among which Lactobacillus spp. was still the dominant bacterium, accounting for 45.77% (Figure 3B). Apart from Lactobacillus spp., there are also Gardnerella spp., Staphylococcus spp., Methylobacterium spp. and other bacteria. It is worth noting that the gonadal hormones strongly influence the overall structure and function of vaginal microbiota. On the other hand, microbiota also affect the release and functions of hormones that modulate various physiological functions, such as the circadian rhythms (Kennaway, 2005; Sciarra et al., 2020). More importantly, numerous evidence on the correlation between the vagina microbiota and the development of infertility and Polycystic ovarian syndrome (PCOS), and the variation of its constitution might be useful marker of pregnancy outcomes (Heil et al., 2019; Venneri et al., 2022). Data from several studies suggest that gut dysbiosis and circadian rhythm disorder are associated with obesity, metabolic syndrome and inflammatory bowel disease. However, the formation mechanism of gut microbial rhythm, the dynamic dialog between microbes and the host remain unclear and require further investigation (Samuelson et al., 2019; Wang et al., 2022).
Herein, the inflammatory indexes CRP and WBC were significantly increased in the CCA group, as well as the incidence of abnormal FHR monitoring, while no difference was detected between the HCA and normal groups. Moreover, the proportion of neonatal transfer rate and incidence of neonatal infection was notably higher in the CCA group than in the other two groups. Therefore, we propose that the postoperative histopathology for the diagnosis of CAM is not reliable in term pregnancy, and recommend that the diagnosis and delivery management should be based on clinical indicators. As stated by the NICHD expert panel, maternal fever alone should not automatically lead to the diagnosis of infection (or CAM) and antimicrobial treatment (Higgins et al., 2016). Meanwhile, researchers have searched for either antenatal or postnatal potential biomarkers to guide neonatal management. A recent meta-analysis suggested that there is insufficient evidence to support the use of CRP, procalcitonin, or IL6 in maternal blood for HCA diagnosis in PPROM (Etyang et al., 2020). As for other diagnostic indicators, Myntti et al. (2016) have demonstrated that increased lactate dehydrogenase and decreased blood glucose in amniotic fluid are connected with HCA by amniocentesis in 70 women with suspected intraamniotic infection. Another study has speculated that metalloproteinase (MMP-8) in amniotic fluid is a sensitive index for the diagnosis of microbial invasion of the amniotic cavity (Chaemsaithong et al., 2018). However, amniocentesis is an invasive procedure, which would also increase the risk of infection. Furthermore, given the correlation between genital tract microbiota and hormones, hormonal evaluation may also be a predictor of CAM. Except the positive correlation between E2 serum concentration and Lactobacillus abundance mentioned above, there are many other hormone and flora correlations reported in the literature. For example, Prevotella show a strong positive correlation with testosterone and negative associations with estradiol concentrations (Kumar, 2013); Gemella was detected as positively related with both FSH and LH (Sabo et al., 2020); a negative correlation was found between Aerococcus, Atopobium and LH hormone (Ling et al., 2010). Hence, we propose that parturients should be comprehensively evaluated for clinical symptoms, laboratory examination (WBC, CRP, or sex hormones), and fetal heart monitoring in the clinic.
It has long been thought that the diagnosis and progression of CAM were inextricably linked to infection, especially ascending infections. Previous studies have found that the placental microbiota is influenced by inflammation, with some oral and urogenital commensal bacteria playing a key role in CAM etiology (Prince et al., 2016). In a follow-up study, Hockney et al. (2020) confirmed that HCA is associated with infection and increased the bacterial load in a dose–response relationship. Common microorganisms in female genital tract infections include Group B Streptococcus, E. coli, Bacillus, E. faecalis, U. urealyticum, M. hominis, and bacterial vaginosis (Tibaldi et al., 2016). Moreover, several reports have shown that Ureaplasma spp. is the most prevalent pathogen in early-preterm birth or late-preterm (Sweeney et al., 2016; Matasariu et al., 2022). A prospective cohort study also detected Mycoplasma and/or Ureaplasma in 81% (29/36) of the vaginal secretions of preterm pregnant women (Paramel Jayaprakash et al., 2016). As can be seen from the samples, whether culture or 16S rRNA sequencing detected only a low percentage of positive rates. The most interesting finding was that the frequency of HCA is higher than that of CCA with positive bacterial cultures (37.8% in HCA group; 21.4% in CCA group). Therefore, we assume that histological and microbiological evidence of inflammation or infection may not always accompany one another. Similar to the above study, in the samples for which bacterial DNA could be detected, Ureaplasma spp. was higher in both the HCA and CCA groups than the normal group, especially in the CCA group. Furthermore, Romero et al. (2019) have conducted a cross-sectional study of women with intra-amniotic infection with intact membranes, and the results implied that the bacteria in the infected amniotic fluid were mainly Sneathia, Ureaplasma, Prevotella, Lactobacillus, Escherichia, Gardnerella, Peptostreptococcus, Peptoniphilus and Streptococcus, many of which were not cultured from the amniotic fluid samples. These results were highly similar to our current results for the intrauterine microbiota. The opportunistic pathogen Ureaplasma has been found in the urogenital tract and amniotic fluid of asymptomatic healthy and symptomatic women (Rumyantseva et al., 2019). Due to its high requirements for culture in vitro and slow growth, traditional culture detection is very complicated (van der Schalk et al., 2020). Thus, we hypothesized that this opportunistic pathogen not only causes premature labor but also plays a key role in term CAM. Hence, it is necessary to consider Ureaplasma infections when cephalosporin antibiotics are ineffective in CAM parturients in clinical practice, and a nucleic acid test should be used to improve the detection rate.
Moreover, Combs et al. (2014) have found that intra-amniotic inflammation is connected with adverse pregnancy outcomes regardless of the detection of microorganisms. In other words, despite the etiology, inflammation is a major driver of CAM. One retrospective study has shown that the term CCA is a syndrome that might be caused by intra-amniotic infection (63%) and sterile intra-amniotic inflammation (5%). Additionally, a considerable number of patients have no evidence of intraamniotic infection or inflammation (Romero et al., 2021). Besides, Roberts et al. (2012) have suggested that CAM might be a non-infectious inflammatory process. Thus, the activation of the maternal immune state at term might lead to similar changes in the placenta. Infection is inflammation caused by the invasion of bacteria, viruses, fungi or other pathogens, while inflammation is a basic pathological process in which the tissue with vascular system reacts to various injury factors. Increasing evidence has shown that inflammation without microorganisms might be elicited by activation of endogenous danger signals derived from cellular stress or necrosis, known as damage-associated molecular patterns (DAMPs) or alarmins (Romero et al., 2011). Furthermore, a recent study compared sterile intra-amniotic inflammation and those with intra-amniotic infection via RNA sequencing, confirm that the immune response in sterile inflammation is notably different and milder than microbial-induced inflammation (Motomura et al., 2021). Nevertheless, to date, there is no approved treatment for sterile intra-amniotic inflammation. Meanwhile, Galaz et al. (2021) used a mouse model of HMGB1-induced sterile inflammation and demonstrated that betamethasone treatment prevents preterm birth but does not reduce neonatal mortality. In addition, a recent study had proved that intravenous clarithromycin could reduce the intensity of sterile inflammation in patients with PPROM by monitoring IL-6 concentrations (Kacerovsky et al., 2020). Future studies should consider the mechanisms of intrauterine inflammation and focus on the treatment of sterile inflammation.
In current study, we not only analyzed the intrauterine microbiota in normal and CAM patients but also comprehensively considered its trend in the upper and lower genital tracts from a macroscopic perspective. However, our research also has some limitations. First, negative control samples (operating room environment, surgical instruments, and DNA extraction kits) were not subjected to 16S sequencing because no DNA of acceptable quality was extracted. Second, our sample size was small and experiments with larger samples are required to further prove our current results.
Conclusion
In summary, some normal-term pregnant women can harbor non-pathogenic microbiota in the uterine cavity. Additionally, sterile inflammation is more frequent than microbial-associated inflammation in full-term HCA and CCA patients. Besides, we recommend a combined evaluation of clinical symptoms, laboratory examination, and FHR monitoring to diagnose CAM at term. Finally, it is necessary to determine a sensitive biomarker in the diagnosis of CAM in future studies.
Data availability statement
The data presented in the study are deposited in the NCBI repository, accession number PRJNA904128.
Ethics statement
The studies involving human participants were reviewed and approved by Ethics Committee of Beijing Tsinghua Changgung Hospital (16121-0110). The patients/participants provided their written informed consent to participate in this study. Written informed consent was obtained from the individual(s) for the publication of any potentially identifiable images or data included in this article.
Author contributions
ML and ZH: conceptualization and writing – original draft. ZT, YM, and JW: data curation and formal analysis. QZ, MS, and YL: funding acquisition, investigation, and methodology. YiW, YuW, XW, YC, and RC: project administration, resources, and software. QL and LZ: supervision, validation, visualization, and writing – reviewing and editing. All authors contributed to the article and approved the submitted version.
Funding
Tsinghua University Chunfeng Fund domestic research Project (405-10001000221) 2021Z99CFY032; Key Laboratory of Precision Medicine, Tsinghua University (20219990012); National Natural Science Foundation of China, Key instrument Research and development Project (61927819); Beijing Natural Fund Committee, general project (7202239).
Acknowledgments
We thank the principal investigators and researchers, as well as the women who participated in the trial, who contributed time and effort to make this study a success.
Conflict of interest
The authors declare that the research was conducted in the absence of any commercial or financial relationships that could be construed as a potential conflict of interest.
Publisher’s note
All claims expressed in this article are solely those of the authors and do not necessarily represent those of their affiliated organizations, or those of the publisher, the editors and the reviewers. Any product that may be evaluated in this article, or claim that may be made by its manufacturer, is not guaranteed or endorsed by the publisher.
References
Aagaard, K., Ma, J., Antony, K. M., Ganu, R., Petrosino, J., and Versalovic, J. (2014). The placenta harbors a unique microbiome. Sci. Transl. Med. 6:237ra65. doi: 10.1126/scitranslmed.3008599
Aagaard, K., Riehle, K., Ma, J., Segata, N., Mistretta, T.-A., Coarfa, C., et al. (2012). A metagenomic approach to characterization of the vaginal microbiome signature in pregnancy. PLoS One 7:e36466. doi: 10.1371/journal.pone.0036466
Beck, C., Gallagher, K., Taylor, L. A., Goldstein, J. A., Mithal, L. B., and Gernand, A. D. (2021). Chorioamnionitis and risk for maternal and neonatal sepsis: a systematic review and meta-analysis. Obstet. Gynecol. 137, 1007–1022. doi: 10.1097/AOG.0000000000004377
Bi, Y., Tu, Y., Zhang, N., Wang, S., Zhang, F., Suen, G., et al. (2021). Multiomics analysis reveals the presence of a microbiome in the gut of fetal lambs. Gut 70, 853–864. doi: 10.1136/gutjnl-2020-320951
Chaemsaithong, P., Romero, R., Docheva, N., Chaiyasit, N., Bhatti, G., Pacora, P., et al. (2018). Comparison of rapid MMP-8 and interleukin-6 point-of-care tests to identify intra-amniotic inflammation/infection and impending preterm delivery in patients with preterm labor and intact membranes. J. Matern. Fetal Neonatal Med. 31, 228–244. doi: 10.1080/14767058.2017.1281904
Chen, S., Zhou, Y., Chen, Y., and Gu, J. (2018). Fastp: an ultra-fast all-in-one FASTQ preprocessor. Bioinformatics 34, i884–i890. doi: 10.1093/bioinformatics/bty560
Combs, C. A., Gravett, M., Garite, T. J., Hickok, D. E., Lapidus, J., Porreco, R., et al. (2014). Amniotic fluid infection, inflammation, and colonization in preterm labor with intact membranes. Am. J. Obstet. Gynecol. 210, 125.e1–125.e15. doi: 10.1016/j.ajog.2013.11.032
Committee on Obstetric Practice (2017). Committee Opinion No. 712: intrapartum Management of Intraamniotic Infection. Obstet. Gynecol. 130, e95–e101. doi: 10.1097/AOG.0000000000002236
De Goffau, M. C., Charnock-Jones, D. S., Smith, G. C. S., and Parkhill, J. (2021). Batch effects account for the main findings of an in utero human intestinal bacterial colonization study. Microbiome 9:6. doi: 10.1186/s40168-020-00949-z
De Goffau, M. C., Lager, S., Sovio, U., Gaccioli, F., Cook, E., Peacock, S. J., et al. (2019). Human placenta has no microbiome but can harbour potential pathogens. Nature 572, 329–334. doi: 10.1038/s41586-019-1451-5
Edgar, R. C. (2013). UPARSE: highly accurate OTU sequences from microbial amplicon reads. Nat. Methods 10, 996–998. doi: 10.1038/nmeth.2604
Ennamorati, M., Vasudevan, C., Clerkin, K., Halvorsen, S., Verma, S., Ibrahim, S., et al. (2020). Intestinal microbes influence development of thymic lymphocytes in early life. Proc. Natl. Acad. Sci. U. S. A. 117, 2570–2578. doi: 10.1073/pnas.1915047117
Etyang, A. K., Omuse, G., Mukaindo, A. M., and Temmerman, M. (2020). Maternal inflammatory markers for chorioamnionitis in preterm prelabour rupture of membranes: a systematic review and meta-analysis of diagnostic test accuracy studies. Syst. Rev. 9:141. doi: 10.1186/s13643-020-01389-4
Fettweis, J. M., Serrano, M. G., Brooks, J. P., Edwards, D. J., Girerd, P. H., Parikh, H. I., et al. (2019). The vaginal microbiome and preterm birth. Nat. Med. 25, 1012–1021. doi: 10.1038/s41591-019-0450-2
Funkhouser, L. J., and Bordenstein, S. R. (2013). Mom knows best: the universality of maternal microbial transmission. PLoS Biol. 11:e1001631. doi: 10.1371/journal.pbio.1001631
Galaz, J., Romero, R., Arenas-Hernandez, M., Panaitescu, B., Para, R., and Gomez-Lopez, N. (2021). Betamethasone as a potential treatment for preterm birth associated with sterile intra-amniotic inflammation: a murine study. J. Perinat. Med. 49, 897–906. doi: 10.1515/jpm-2021-0049
Heil, B. A., Paccamonti, D. L., and Sones, J. L. (2019). Role for the mammalian female reproductive tract microbiome in pregnancy outcomes. Physiol. Genomics 51, 390–399. doi: 10.1152/physiolgenomics.00045.2019
Higgins, R. D., Saade, G., Polin, R. A., Grobman, W. A., Buhimschi, I. A., Watterberg, K., et al. (2016). Evaluation and Management of Women and Newborns with a maternal diagnosis of Chorioamnionitis: summary of a workshop. Obstet. Gynecol. 127, 426–436. doi: 10.1097/AOG.0000000000001246
Hockney, R., Waring, G. J., Taylor, G., Cummings, S. P., Robson, S. C., Orr, C. H., et al. (2020). Fetal membrane bacterial load is increased in histologically confirmed inflammatory chorioamnionitis: a retrospective cohort study. Placenta 91, 43–51. doi: 10.1016/j.placenta.2020.01.006
Kacerovsky, M., Romero, R., Stepan, M., Stranik, J., Maly, J., Pliskova, L., et al. (2020). Antibiotic administration reduces the rate of intraamniotic inflammation in preterm prelabor rupture of the membranes. Am. J. Obstet. Gynecol. 223, 114.e1–114.e20. doi: 10.1016/j.ajog.2020.01.043
Kennaway, D. J. (2005). The role of circadian rhythmicity in reproduction. Hum. Reprod. Update 11, 91–101. doi: 10.1093/humupd/dmh054
Kim, D., Hofstaedter, C. E., Zhao, C., Mattei, L., Tanes, C., Clarke, E., et al. (2017). Optimizing methods and dodging pitfalls in microbiome research. Microbiome 5:52. doi: 10.1186/s40168-017-0267-5
Kohlhepp, L. M., Hollerich, G., Vo, L., Hofmann-Kiefer, K., Rehm, M., Louwen, F., et al. (2018). Physiological changes during pregnancy. Anaesthesist 67, 383–396. doi: 10.1007/s00101-018-0437-2
Kumar, P. S. (2013). Sex and the subgingival microbiome: do female sex steroids affect periodontal bacteria? Periodontol. 61, 103–124. doi: 10.1111/j.1600-0757.2011.00398.x
Kuperman, A. A., Zimmerman, A., Hamadia, S., Ziv, O., Gurevich, V., Fichtman, B., et al. (2020). Deep microbial analysis of multiple placentas shows no evidence for a placental microbiome. BJOG 127, 159–169. doi: 10.1111/1471-0528.15896
Lauder, A. P., Roche, A. M., Sherrill-Mix, S., Bailey, A., Laughlin, A. L., Bittinger, K., et al. (2016). Comparison of placenta samples with contamination controls does not provide evidence for a distinct placenta microbiota. Microbiome 4:29. doi: 10.1186/s40168-016-0172-3
Leiby, J. S., McCormick, K., Sherrill-Mix, S., Clarke, E. L., Kessler, L. R., Taylor, L. J., et al. (2018). Lack of detection of a human placenta microbiome in samples from preterm and term deliveries. Microbiome 6:196. doi: 10.1186/s40168-018-0575-4
Levy, M., Kovo, M., Feldstein, O., Dekalo, A., Schreiber, L., Levanon, O., et al. (2021). The effect of concomitant histologic chorioamnionitis in pregnancies complicated by fetal growth restriction. Placenta 104, 51–56. doi: 10.1016/j.placenta.2020.11.009
Ling, Z., Kong, J., Liu, F., Zhu, H., Chen, X., Wang, Y., et al. (2010). Molecular analysis of the diversity of vaginal microbiota associated with bacterial vaginosis. BMC Genomics 11:488. doi: 10.1186/1471-2164-11-488
Magoč, T., and Salzberg, S. L. (2011). FLASH: fast length adjustment of short reads to improve genome assemblies. Bioinformatics 27, 2957–2963. doi: 10.1093/bioinformatics/btr507
Malla, M. A., Dubey, A., Kumar, A., Yadav, S., Hashem, A., and Abd Allah, E. F. (2018). Exploring the human microbiome: the potential future role of next-generation sequencing in disease diagnosis and treatment. Front. Immunol. 9:2868. doi: 10.3389/fimmu.2018.02868
Matasariu, D. R., Ursache, A., Agache, A., Mandici, C. E., Boiculese, V. L., Bujor, I. E., et al. (2022). Genital infection with Ureaplasma urealyticum and its effect on pregnancy. Exp. Ther. Med. 23:89. doi: 10.3892/etm.2021.11012
McMillan, A., Rulisa, S., Sumarah, M., Macklaim, J. M., Renaud, J., Bisanz, J. E., et al. (2015). A multi-platform metabolomics approach identifies highly specific biomarkers of bacterial diversity in the vagina of pregnant and non-pregnant women. Sci. Rep. 5:14174. doi: 10.1038/srep14174
Mishra, A., Lai, G. C., Yao, L. J., Aung, T. T., Shental, N., Rotter-Maskowitz, A., et al. (2021). Microbial exposure during early human development primes fetal immune cells. Cells 184, 3394–3409.e20. doi: 10.1016/j.cell.2021.04.039
Motomura, K., Romero, R., Galaz, J., Tarca, A. L., Done, B., Xu, Y., et al. (2021). RNA sequencing reveals distinct immune responses in the chorioamniotic membranes of women with preterm labor and microbial or sterile intra-amniotic inflammation. Infect. Immun. 89, e00819–e00820. doi: 10.1128/IAI.00819-20
Myntti, T., Rahkonen, L., Tikkanen, M., Pätäri-Sampo, A., Paavonen, J., and Stefanovic, V. (2016). Amniotic fluid rapid biomarkers are associated with intra-amniotic infection in preterm pregnancies regardless of the membrane status. J. Perinatol. 36, 606–611. doi: 10.1038/jp.2016.59
Oh, K. J., Kim, S. M., Hong, J.-S., Maymon, E., Erez, O., Panaitescu, B., et al. (2017). Twenty-four percent of patients with clinical chorioamnionitis in preterm gestations have no evidence of either culture-proven intraamniotic infection or intraamniotic inflammation. Am. J. Obstet. Gynecol. 216, 604.e1–604.e11. doi: 10.1016/j.ajog.2017.02.035
Olomu, I. N., Pena-Cortes, L. C., Long, R. A., Vyas, A., Krichevskiy, O., Luellwitz, R., et al. (2020). Elimination of “kitome” and “splashome” contamination results in lack of detection of a unique placental microbiome. BMC Microbiol. 20:157. doi: 10.1186/s12866-020-01839-y
Paramel Jayaprakash, T., Wagner, E. C., van Schalkwyk, J., Albert, A. Y. K., Hill, J. E., Money, D. M., et al. (2016). High diversity and variability in the vaginal microbiome in women following preterm premature rupture of membranes (PPROM): a prospective cohort study. PLoS One 11:e0166794. doi: 10.1371/journal.pone.0166794
Prince, A. L., Ma, J., Kannan, P. S., Alvarez, M., Gisslen, T., Harris, R. A., et al. (2016). The placental microbiome is altered among subjects with spontaneous preterm birth with and without chorioamnionitis. Am. J. Obstet. Gynecol. 214, 627.e1–627.e16. doi: 10.1016/j.ajog.2016.01.193
Rackaityte, E., Halkias, J., Fukui, E., Mendoza, V., Hayzelden, C., Crawford, E., et al. (2020). Viable bacterial colonization is highly limited in the human intestine in utero. Nat. Med. 26, 599–607. doi: 10.1038/s41591-020-0761-3
Ravel, J., Gajer, P., Abdo, Z., Schneider, G. M., Koenig, S. S. K., McCulle, S. L., et al. (2011). Vaginal microbiome of reproductive-age women. Proc. Natl. Acad. Sci. U. S. A. 108, 4680–4687. doi: 10.1073/pnas.1002611107
Roberts, D. J., Celi, A. C., Riley, L. E., Onderdonk, A. B., Boyd, T. K., Johnson, L. C., et al. (2012). Acute histologic chorioamnionitis at term: nearly always noninfectious. PLoS One 7:e31819. doi: 10.1371/journal.pone.0031819
Romero, R., Chaiworapongsa, T., Alpay Savasan, Z., Xu, Y., Hussein, Y., Dong, Z., et al. (2011). Damage-associated molecular patterns (DAMPs) in preterm labor with intact membranes and preterm PROM: a study of the alarmin HMGB1. J. Matern. Fetal Neonatal Med. 24, 1444–1455. doi: 10.3109/14767058.2011.591460
Romero, R., Gomez-Lopez, N., Winters, A. D., Jung, E., Shaman, M., Bieda, J., et al. (2019). Evidence that intra-amniotic infections are often the result of an ascending invasion – a molecular microbiological study. J. Perinat. Med. 47, 915–931. doi: 10.1515/jpm-2019-0297
Romero, R., Hassan, S. S., Gajer, P., Tarca, A. L., Fadrosh, D. W., Nikita, L., et al. (2014a). The composition and stability of the vaginal microbiota of normal pregnant women is different from that of non-pregnant women. Microbiome 2:4. doi: 10.1186/2049-2618-2-4
Romero, R., Miranda, J., Chaiworapongsa, T., Korzeniewski, S. J., Chaemsaithong, P., Gotsch, F., et al. (2014b). Prevalence and clinical significance of sterile intra-amniotic inflammation in patients with preterm labor and intact membranes. Am. J. Reprod. Immunol. 72, 458–474. doi: 10.1111/aji.12296
Romero, R., Pacora, P., Kusanovic, J. P., Jung, E., Panaitescu, B., Maymon, E., et al. (2021). Clinical chorioamnionitis at term X: microbiology, clinical signs, placental pathology, and neonatal bacteremia - implications for clinical care. J. Perinat. Med. 49, 275–298. doi: 10.1515/jpm-2020-0297
Rumyantseva, T., Khayrullina, G., Guschin, A., and Donders, G. (2019). Prevalence of Ureaplasma spp. and mycoplasma hominis in healthy women and patients with flora alterations. Diagn. Microbiol. Infect. Dis. 93, 227–231. doi: 10.1016/j.diagmicrobio.2018.10.001
Sabo, M. C., Lehman, D. A., Wang, B., Richardson, B. A., Srinivasan, S., Osborn, L., et al. (2020). Associations between vaginal bacteria implicated in HIV acquisition risk and proinflammatory cytokines and chemokines. Sex. Transm. Infect. 96, 3–9. doi: 10.1136/sextrans-2018-053949
Salter, S. J., Cox, M. J., Turek, E. M., Calus, S. T., Cookson, W. O., Moffatt, M. F., et al. (2014). Reagent and laboratory contamination can critically impact sequence-based microbiome analyses. BMC Biol. 12:87. doi: 10.1186/s12915-014-0087-z
Samuelson, D. R., Gu, M., Shellito, J. E., Molina, P. E., Taylor, C. M., Luo, M., et al. (2019). Intestinal microbial products from alcohol-fed mice contribute to intestinal permeability and peripheral immune activation. Alcohol. Clin. Exp. Res. 43, 2122–2133. doi: 10.1111/acer.14176
Sciarra, F., Franceschini, E., Campolo, F., Gianfrilli, D., Pallotti, F., Paoli, D., et al. (2020). Disruption of circadian rhythms: a crucial factor in the etiology of infertility. Int. J. Mol. Sci. 21:39437. doi: 10.3390/ijms21113943
Seferovic, M. D., Pace, R. M., Carroll, M., Belfort, B., Major, A. M., Chu, D. M., et al. (2019). Visualization of microbes by 16S in situ hybridization in term and preterm placentas without intraamniotic infection. Am. J. Obstet. Gynecol. 221, 146.e1–146.e23. doi: 10.1016/j.ajog.2019.04.036
Serrano, M. G., Parikh, H. I., Brooks, J. P., Edwards, D. J., Arodz, T. J., Edupuganti, L., et al. (2019). Racioethnic diversity in the dynamics of the vaginal microbiome during pregnancy. Nat. Med. 25, 1001–1011. doi: 10.1038/s41591-019-0465-8
Smith, B. C., McAndrew, T., Chen, Z., Harari, A., Barris, D. M., Viswanathan, S., et al. (2012). The cervical microbiome over 7 years and a comparison of methodologies for its characterization. PLoS One 7:e40425. doi: 10.1371/journal.pone.0040425
Stackebrandt, E., and Goebel, B. M. (1994). Taxonomic note: a place for DNA-DNA Reassociation and 16S rRNA sequence analysis in the present species definition in bacteriology. Int. J. Syst. Evol. Microbiol. 44, 846–849. doi: 10.1099/00207713-44-4-846
Stinson, L. F., Boyce, M. C., Payne, M. S., and Keelan, J. A. (2019). The not-so-sterile womb: evidence that the human fetus is exposed to bacteria prior to birth. Front. Microbiol. 10:1124. doi: 10.3389/fmicb.2019.01124
Sweeney, E. L., Kallapur, S. G., Gisslen, T., Lambers, D. S., Chougnet, C. A., Stephenson, S.-A., et al. (2016). Placental infection with Ureaplasma species is associated with histologic Chorioamnionitis and adverse outcomes in moderately preterm and late-preterm infants. J. Infect. Dis. 213, 1340–1347. doi: 10.1093/infdis/jiv587
Taddei, C. R., Cortez, R. V., Mattar, R., Torloni, M. R., and Daher, S. (2018). Microbiome in normal and pathological pregnancies: a literature overview. Am. J. Reprod. Immunol. 80:e12993. doi: 10.1111/aji.12993
Tibaldi, C., Cappello, N., Latino, M. A., Polarolo, G., Masuelli, G., Cavallo, F., et al. (2016). Maternal risk factors for abnormal vaginal flora during pregnancy. Int. J. Gynaecol. Obstet. 133, 89–93. doi: 10.1016/j.ijgo.2015.07.035
Tita, A. T. N., and Andrews, W. W. (2010). Diagnosis and management of clinical chorioamnionitis. Clin. Perinatol. 37, 339–354. doi: 10.1016/j.clp.2010.02.003
Tuominen, H., Collado, M. C., Rautava, J., Syrjänen, S., and Rautava, S. (2019). Composition and maternal origin of the neonatal oral cavity microbiota. J. Oral Microbiol. 11:1663084. doi: 10.1080/20002297.2019.1663084
van der Schalk, T. E., Braam, J. F., and Kusters, J. G. (2020). Molecular basis of antimicrobial resistance in Mycoplasma genitalium. Int. J. Antimicrob. Agents 55:105911. doi: 10.1016/j.ijantimicag.2020.105911
Venneri, M. A., Franceschini, E., Sciarra, F., Rosato, E., D’Ettorre, G., and Lenzi, A. (2022). Human genital tracts microbiota: dysbiosis crucial for infertility. J. Endocrinol. Investig. 45, 1151–1160. doi: 10.1007/s40618-022-01752-3
Wang, Q., Garrity, G. M., Tiedje, J. M., and Cole, J. R. (2007). Naive Bayesian classifier for rapid assignment of rRNA sequences into the new bacterial taxonomy. Appl. Environ. Microbiol. 73, 5261–5267. doi: 10.1128/AEM.00062-07
Wang, H., Zhang, H., and Su, Y. (2022). New insights into the diurnal rhythmicity of gut microbiota and its crosstalk with host circadian rhythm. Animals 12:1677. doi: 10.3390/ani12131677
Winters, A. D., Romero, R., Greenberg, J. M., Galaz, J., Shaffer, Z. D., Garcia-Flores, V., et al. (2022). Does the amniotic fluid of mice contain a viable microbiota? Front. Immunol. 13:820366. doi: 10.3389/fimmu.2022.820366
Younge, N., McCann, J. R., Ballard, J., Plunkett, C., Akhtar, S., Araújo-Pérez, F., et al. (2019). Fetal exposure to the maternal microbiota in humans and mice. JCI Insight 4:e127806. doi: 10.1172/jci.insight.127806
Yu, K., Rodriguez, M. D., Paul, Z., Gordon, E., Rice, K., Triplett, E. W., et al. (2019). Proof of principle: physiological transfer of small numbers of bacteria from mother to fetus in late-gestation pregnant sheep. PLoS One 14:e0217211. doi: 10.1371/journal.pone.0217211
Zakošek Pipan, M., Kajdič, L., Kalin, A., Plavec, T., and Zdovc, I. (2020). Do newborn puppies have their own microbiota at birth? Influence of type of birth on newborn puppy microbiota. Theriogenology 152, 18–28. doi: 10.1016/j.theriogenology.2020.04.014
Keywords: pregnancy, chorioamnionitis, microbiome, infection, inflammation
Citation: Li M, Huang Z, Tao Z, Meng Y, Wen J, Zhang Q, Liu Y, Shang M, Wang Y, Wang Y, Chen R, Wang X, Cao Y, Zhang L and Liao Q (2022) The role of upper and lower genital tract microbiota alterations in term chorionamnionitis: A prospective study. Front. Microbiol. 13:1069254. doi: 10.3389/fmicb.2022.1069254
Edited by:
George Grant, University of Aberdeen, United KingdomReviewed by:
Mary Anna Venneri, Sapienza University of Rome, ItalyNicoletta Di Simone, Humanitas University, Italy
Valerio Rossini, Spanish National Research Council (CSIC), Spain
Copyright © 2022 Li, Huang, Tao, Meng, Wen, Zhang, Liu, Shang, Wang, Wang, Chen, Wang, Cao, Zhang and Liao. This is an open-access article distributed under the terms of the Creative Commons Attribution License (CC BY). The use, distribution or reproduction in other forums is permitted, provided the original author(s) and the copyright owner(s) are credited and that the original publication in this journal is cited, in accordance with accepted academic practice. No use, distribution or reproduction is permitted which does not comply with these terms.
*Correspondence: Lei Zhang, MTg2MDExMzA0NTlAMTYzLmNvbQ==; Qinping Liao, MTM3MDExMjQ1MjdAMTYzLmNvbQ==
†These authors have contributed equally to this work and share first authorship