- 1Department of Forest Protection, College of Forestry and Biotechnology, Zhejiang A&F University, Hangzhou, China
- 2Department of Silviculture, College of Forestry and Biotechnology, Zhejiang A&F University, Hangzhou, China
Ganoderma lucidum (GL) is a well-known medicinal mushroom that has been extensively cultivated. Our previous study has shown that abundant Trichoderma colonies grow on the casing soil surface, posing cultivation obstacles for GL. However, an understanding of species-level characteristics of Trichoderma strains and their adverse effects on GL growth is limited. This study aimed to investigate the diversity and potential effects of Trichoderma from GL-cultivated soils. Over 700 Trichoderma isolates were collected from two trails in Longquan Country, southeast China. Eight Trichoderma species, including T. atrioviride, T. guizhouense, T. hamatum, T. harzianum, T. koningiopsis, T. pleuroticola, T. sp. irale, and T. virens, were identified based on the combination alignment of tef-1α and rpb2 sequences. The number of Trichoderma colonies increased dramatically during GL cultivation, with an increase of 9.2-fold in the Lanju trail. T. virens accounted for the most colonies (33.33 and 32.50% in Lanju and Chengbei, respectively) at the end of GL cultivation. The Trichoderma species growth varied but was satisfactory under different temperature or pH conditions. Moreover, Trichoderma species showed different adverse effects on GL growth. The non-volatile metabolites from T. virens and volatile metabolites from T. atroviride displayed the strongest antagonistic activity. Furthermore, the volatile 6-pentyl-2H-pyran-2-one (6-PP) showed a significant inhibitory effect on GL growth with an 8.79 μl mL−1 headspace of 50% effective concentration. The different Trichoderma spp. produced different amounts of 6-PP. The most efficient 6-PP producer was T. atroviride. To the best of our knowledge, this study is the first to demonstrate the abundance of competitive Trichoderma species associated with GL cultivation. Our results would contribute to.
Introduction
Ganoderma lucidum (GL, commonly known as ‘Lingzhi’ in Chinese or ‘Reishi’ in Japanese) is a traditional medicinal mushroom, which has been consumed for a long time for its high nutritive and medicinal properties (Cao et al., 2012). GL and its byproducts have multiple biological functions, including melanin synthesis inhibition and gut microbiota regulation, and possess anti-inflammatory and anti-tumor properties (Bishop et al., 2015; Chang et al., 2015). These diverse nutraceutical and pharmacological effects are mainly attributed to the high triterpenoid and polysaccharide contents in GL spores and fruiting bodies (Boh et al., 2007). Owing to its limited production in nature, GL has been artificially cultivated extensively, especially in China. Among the GL cultivation regions, Longquan County in Zhejiang Province is a famous geo-herbalism region owing to its long history of cultivation (Li et al., 2016).
GL is a wood-degrading basidiomycete that utilizes lignin as its preferred carbon source in nature (Liu et al., 2012). Short wood logs have been cultivated in Longquan County and other regions of China for a high yield of spores containing abundant active triterpenoids and polysaccharides (Zhang et al., 2004). The GL-inoculated wood logs are transferred to a mushroom house and embedded in the soil. Two harvests of GL spores within 2 years can be achieved with one cultivation season (Zhou, 2017). However, GL cultivation is hindered due to the occurrence of large amounts of competitive fungi, such as Trichoderma, during cultivation, incurring loss to local farmers (Tong et al., 2020). Some Trichoderma species, such as T. harzianum and T. atroviride, cause green mold disease in cultivated GL (Lu et al., 2016; Yan et al., 2019).
The genus Trichoderma Pers. (Ascomycetes, Hypocreales) is cosmopolitan and found in soil or decaying woods (Schuster and Schmoll, 2010). Many species in this genus are economically and ecologically important. The species T. harzianum and T. virens are biostimulants or biocontrol agents applied in the field (Harman, 2006). T. reesi is a well-known industrial cellulose producer (Bischof et al., 2016). Some species, such as T. longibrachiatum and T. virens, are used to remediate soil and water pollution (Babu et al., 2014; Andreolli et al., 2016). However, a few species are causal agents of green mold disease in mushroom cultivation (Komon-Zelazowska et al., 2007; Kim et al., 2012; An et al., 2022). Hatvani et al. (2007) reported that T. aggressivum is an epidemic species causing green mold disease on champignon (Agaricus bisporus) and oyster mushrooms (Pleurotus ostreatus) in Europe. Six Trichoderma species, including T. harzianum, T. atroviride, T. viride, T. pleuroticola, T. longibrachiatum, and T. oblongisporum, have been associated with green mold disease in Shiitake (Lentinula edodes) in China (Wang et al., 2016). The species T. pleuroticola and T. pleuroti have been associated with green mold diseases in champignon mushrooms in America (Royse et al., 1999; Innocenti et al., 2019).
Our previous investigation revealed that the accumulation of antagonistic fungi, mainly Trichoderma, contributed to GL cultivation problems in Longquan County, China (Tong et al., 2020). This finding provided a basis for determining Trichoderma biodiversity in Longquan County and its competitive effects on GL growth. However, the species-level population dynamic of Trichoderma strains during GL cultivation and their potential effects on GL growth are still unknown. Therefore, this study aimed to investigate the diversity and effect of Trichoderma strains on GL-cultivated soils. We collected Trichoderma isolates and identified their species based on sequence analyzes of the combined partial sequences of translation elongation factor 1-alpha (tef1-α) and the second largest RNA polymerase subunit encoding genes (rpb2). The population changes of Trichoderma colonies on the surface of GL-cultivated soils during cultivation were also investigated. The results provide information on Trichoderma diversity and its antagonistic characteristics on GL development.
Materials and methods
Ganoderma lucidum cultivation
GL Hu-nongke 1, widely cultivated in Longquan County, Zhejiang Province, China, was used in this study for GL production by the short wood-log cultivation method as described previously (Tong et al., 2020). The wood logs from a local sawtooth oak (Quercus acutissima) were used for GL cultivation. The GL-inoculated wood logs were embedded in the soil of mushroom houses (10 m width × 50 m length), as shown in Figure 1.
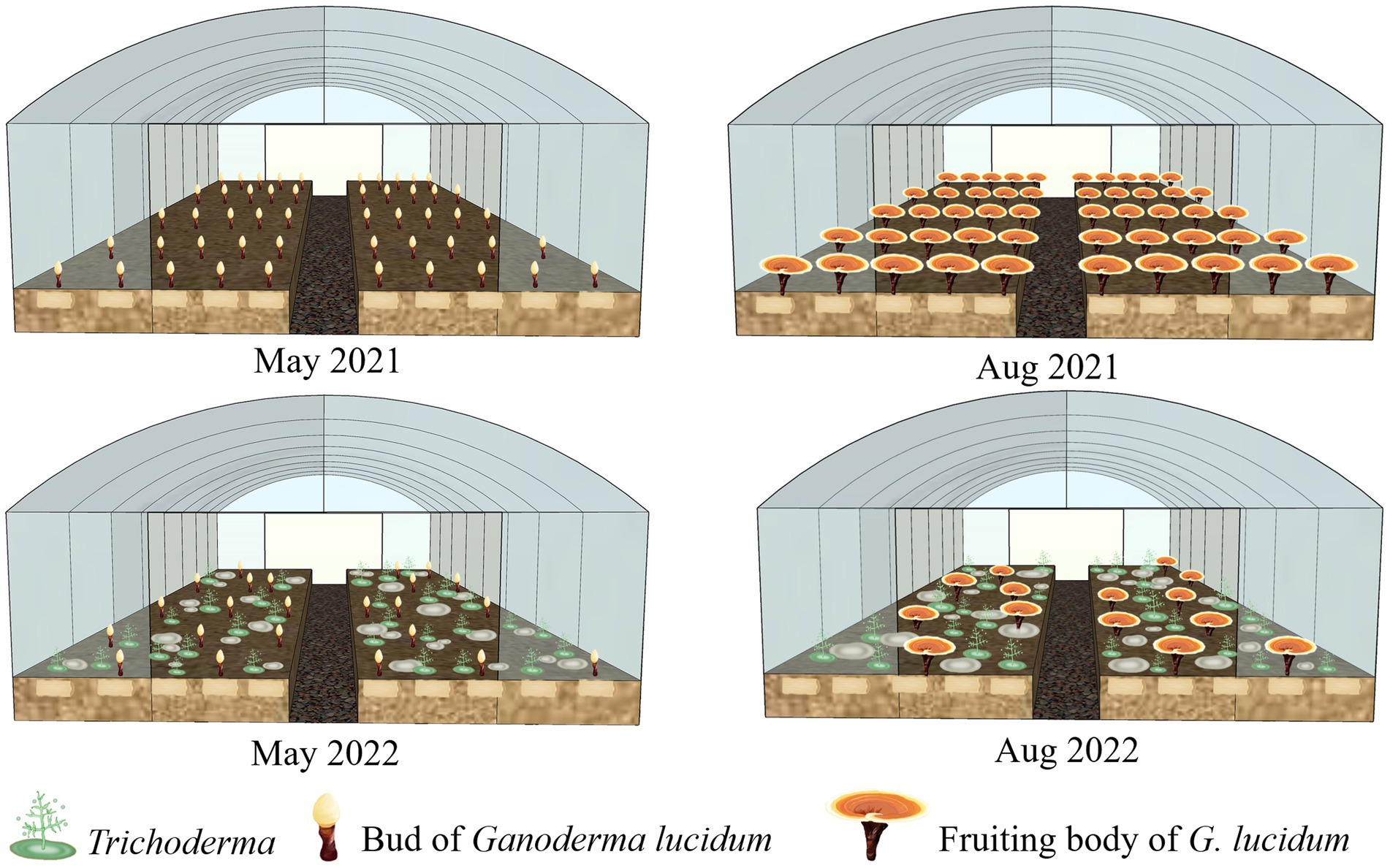
Figure 1. An illustrative scheme of Ganoderma lucidum cultivation in Longquan County of China and the sampling period.
Collection and isolation of Trichoderma colonies
The fungal samples were scraped and labeled from Trichoderma colonies on the surface of GL-cultivated soils in two trails, Lanju (N27°57′55″, E119°02′31″) and Chengbei (N28°13′22″, E119 °04′34″), in Longquan County, southeast China. Field sampling was conducted in May and August of 2021 and 2022 (Figure 1). Trichoderma strains were isolated and maintained as described previously (Hatvani et al., 2007). All isolates were deposited in the culture collection of the Department of Forest Protection, Zhejiang A&F University, China. The Trichoderma isolates were inoculated on 9 cm-diameter Petri dishes containing potato dextrose agar (PDA; 200 g potato, 20 g glucose, 18 g agar, 1 l H2O) or cornmeal dextrose agar (CMD; Oxoid, Hampshire, United Kingdom) at 26°C. Morphological characteristics of the colonies were recorded. Microscopic characteristics were observed under a Motic M200 microscope (Motic, China).
DNA extraction, PCR amplification, and DNA sequencing
Fungal DNA was extracted using the Ezup Column Fungi Genomic DNA Purification Kit (Sangon Biotech, Shanghai, China), following the manufacturer’s instructions. The primers used to amplify the tef-1α and rpb2 genes from Trichoderma were: tef1-F (5′-CATCGAGAAGTTCGAGAAGG-3′), tef1-R (5′-AACTTGCAGGCAATGTGG-3′), rpb2-F (5′-TGGGGWGAYCARAARAAGG-3′), and rpb2-R (5′-CATRATGACSGAATCTTCCTGGT-3′) (Cai and Druzhinina, 2021). The PCR amplification products were separated using electrophoresis in 1.0% (w/v) agarose gels. The purified PCR products were sent to Tsingke Biotechnology Co., Ltd. for Sanger sequencing. The obtained sequences were deposited in the GenBank database at the National Center for Biotechnology Information (NCBI), and their accession numbers are listed in Supplementary Table S1.
Phylogenetic analysis and identification
Nucleotide quality and contig assembly were performed using Staden Package v.2.0.0b11 (Staden et al., 2000). The DNA sequence dataset was constructed using MEGA v.7.0 (Kumar et al., 2016). The aligned tef1-1α and rpb2 gene sequences of all Trichoderma isolates were used for BLAST interface analysis and alignment in the NCBI database.1 After identification, the aligned sequences were submitted to the NCBI GenBank to obtain accession numbers. Molecular phylogenetic analysis between these selected fungi with our strains was performed using the maximum-likelihood method. Nodal robustness was tested using the bootstrap method, and phylogenetic robustness was determined using MEGA v.7.0 with 1,000 replications (Kumar et al., 2016). Protocrea pallida CBS299.78 was used as an outgroup to root the tree.
Trichoderma population assay
Trichoderma population assays were conducted in the two trails: Lanju and Chengbei. Trichoderma colonies grown on the surface of GL-cultivated soil from three randomly chosen mushroom houses in each trail were collected and counted from 2021 to 2022. The numbers of Trichoderma colonies were individually recorded at the time points in April and August each year. The species affiliation of each Trichoderma colony was determined by molecular identification, as described above.
Effects of temperature on mycelial growth of Trichoderma strains
One representative strain for each of the eight Trichoderma species was selected to test the effect of different temperatures on fungal mycelial growth: T. atroviride LZ043, T. hamatum LZ007, T. harzianum LZ013, T. koningiopsis LZ033, T. pleuroticola LZ004, T. guizhouense LZ056, T. spirale LZ023, and T. virens LZ045. A 5 mm-diameter mycelial plug was inoculated in a PDA plate and cultured separately at 13, 23, 31, and 35°C. Colony diameter was measured every day using a Vernier caliper. Each treatment was replicated three times. The assays were conducted using two biological replicates.
Effects of pH on mycelial growth of Trichoderma strains
The eight representative strains were used to test the effect of different pH levels on mycelial growth. Before inoculation, the pH values of PDA were individually adjusted to 4.5, 5.5, 6.5, and 7.5 by adding HCl or NaOH solution filtered through a biofilter after sterilization. A 5 mm-diameter mycelial plug was inoculated into the PDA plate and cultured at 26°C. Colony diameter was measured using a Vernier caliper. Each treatment was replicated three times. The assays were conducted using two biological replicates.
Effects of Trichoderma strains on GL mycelia in petri plates
The eight representative strains were chosen to test the antagonistic potential of the Trichoderma species using the dual confrontation method. Mycelial agar plugs (5 mm in diameter) of GL and each Trichoderma strain were cut individually from the actively growing front of 7-day-old colonies. Each Trichoderma plug was paired against GL at equal distances opposite to each in 90 mm diameter Petri plates containing 20 ml PDA. The plates were incubated at 26°C in darkness for 7 days. The GL growth were recorded. The experiments were carried out with three replicates. The assays were conducted using two biological replicates.
Effects of non-volatile metabolites from different Trichoderma strains on GL hyphal growth
The fermentation broths from eight representative Trichoderma strains were used to test the effects of non-volatile metabolites from different Trichoderma spp. on GL growth. The Trichoderma fermentation broth was prepared as previously described (Wang et al., 2016). Briefly, a 5 mm-diameter plug from each Trichoderma culture on PDA was inoculated in 500 ml PD broth, followed by 7 days culturing in darkness at 26°C at 180 rpm. Each Trichoderma culture was filtered sequentially through cheesecloth, Whatman filter paper, and a 0.2 μm nitrocellulose filter. The filtrates were added to the melted PDA to a volume of 10%; the same volume of sterile water was used as a control. A GL mycelium plug (5 mm in diameter) was inoculated into the medium and cultured in darkness at 26°C. After 7 d, the diameters of the colonies were measured to test the inhibition ratios. The percent inhibition ratio (IR) was computed by the formula: IR = [(R1-R2) /R1] × 100, Where R1 is radial growth of GL in control; R2 is radial growth of GL in treatment. Each treatment was replicated three times. The assays were conducted using two biological replicates.
Effects of volatiles emitted by different Trichoderma strains on GL hyphal growth
The sandwich Petri plate setup described previously was employed to test the antagonistic activity of the volatiles emitted by the representative Trichoderma strains against GL (Li et al., 2018). After inoculating GL and Trichoderma on PDA plates, the GL plate was placed on top of a Trichoderma plate, sealed with three layers of Parafilm, and then incubated at 26°C. A non-inoculated PDA plate was used as the control. GL colony diameters were measured after 5 days. The percent inhibition ratio (IR) was computed by the formula: IR = [(R1-R2) /R1] × 100, Where R1 is radial growth of GL in control; R2 is radial growth of GL in treatment. Each treatment included two biological replicates and was repeated three times.
Analysis of the volatile contents produced by different Trichoderma strains
Solid-phase microextraction coupled with gas chromatography–tandem mass spectrometry (SPME–GC–MS) was used to determine the composition of the volatiles produced by the representative Trichoderma strains (Stoppacher et al., 2010). The detailed procedure and conditions have been described previously (Rao et al., 2022). Trichoderma was cultured on PDA and incubated at 26°C for 5 days. SPME inserted into the injection port of a GC 2010 gas chromatograph was used to collect the volatiles. SPME fiber was exposed to the vapor phase above strain LZ42 for 45 min in a culture tube. A Hewlett Packard 7890GC/5975MSD gas chromatograph (Agilent Technologies, Santa Clara, CA, United States) equipped with an HP-5MS capillary column was used. The derived data were analyzed and identified based on a comparison with the mass spectrum in the NIST08.L data bank (National Institute of Standards and Technology). Each experiment was conducted in triplicates. Pure volatile 6-pentyl-2H-pyran-2-one (6-PP, Sigma-Aldrich, St. Louis, MO, United States) was used as the standard.
Antifungal activity assay of the volatile 6-PP
The antifungal activity of volatile 6-PP on GL was tested by fumigation in an isolated container, as described previously (Wu et al., 2020). The EC50 value of 6-PP on GL was calculated as the 50% effective concentration that inhibits GL mycelial growth using the IBM SPSS Statistics v.19 program (SPSS, Inc., Chicago, IL, United States). A 5 mm-diameter mycelial plug was placed in the center of the PDA. Meanwhile, the 6-PP aliquots of 1, 2, 4, 8, 16, and 32 μl were individually loaded on the filter paper (60 mm in diameter) before incubation, resulting in 0.01, 0.03, 0.05, 0.11, 0.21, and 0.43 μl mL−1 headspace, respectively. Subsequently, the loaded plates were immediately sealed with Parafilm and incubated at 26°C for 5 days. The assays were conducted in triplicate, and plates with filter paper alone were used as controls.
Statistical analysis
The obtained data were analyzed using the IBM SPSS Statistics 20.0 (SPSS Inc.). Statistical significance was identified at 95% confidence interval (p < 0.05) in all tests.
Results
Sample collection and identification of Trichoderma spp. in GL mushrooms
During the investigation period, Trichoderma colonies began appearing on the soil surface of cultivation ridges in the mushroom houses in April 2021 when the sprouts of GL fruiting bodies developed (Figure 1). From the GL mushroom houses, 701 and 685 Trichoderma isolates were collected after 2-year GL cultivation in the Lanju and Chengbei trails, respectively. These isolates presented diverse morphological characteristics (data not shown). A phylogenetic tree of the 73 representative Trichoderma isolates with different morphological characters were constructed using the maximum-likelihood method based on the combination of tef-1α and rpb2 sequences (Figure 2). The combined sequence matrix contained 2,271 bp (1,118 for tef1-α and 1,031 for rpb2). Eight Trichoderma species were identified among these isolates with those that were previously documented as threshold of species: T. atrioviride, T. guizhouense, T. hamatum, T. harzianum, T. koningiopsis, T. pleuroticola, T. spirale, and T. virens.
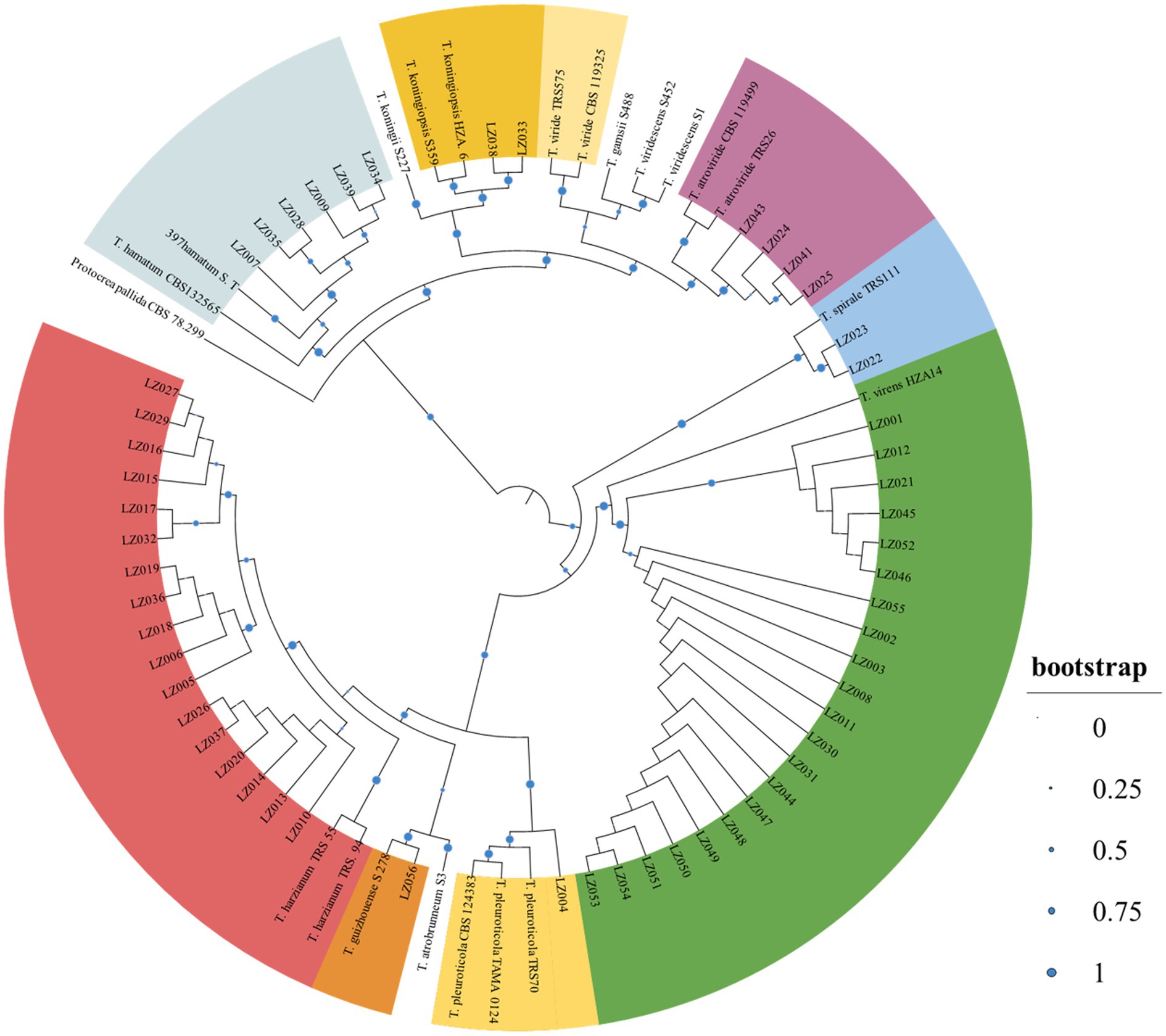
Figure 2. Phylogenetic tree of the representative Trichoderma isolates using the maximum-likelihood method based on the condensed tef-1α and rpb2. The tree was constructed using Mega 7.0 with 1,000 bootstrap replications condensed with a 70% cut-off value and viewed on iTOL. Protocrea pallida CBS 78.299 was used as an outgroup.
Population changes of Trichoderma spp. during GL cultivation
Trichoderma propagation on the surfaces of GL-cultivated soils was analyzed individually in the Lanju and Chengbei trails since April 2021, based on colony sampling and identification of Trichoderma isolates. The colonies of Trichoderma spp. in both trails started appearing in April 2021 and increased significantly thereafter (Figure 3). In the Lanju trail, the average number of Trichoderma colonies in each house was 21 in April 2021 but increased by 2.8-fold in August 2021 and 5.8-fold in April 2022. The average number of Trichoderma colonies reached 214 in August 2022, an increase of 9.2-fold. Similarly, dynamic changes in Trichoderma colonies were observed in the Chengbei trail.
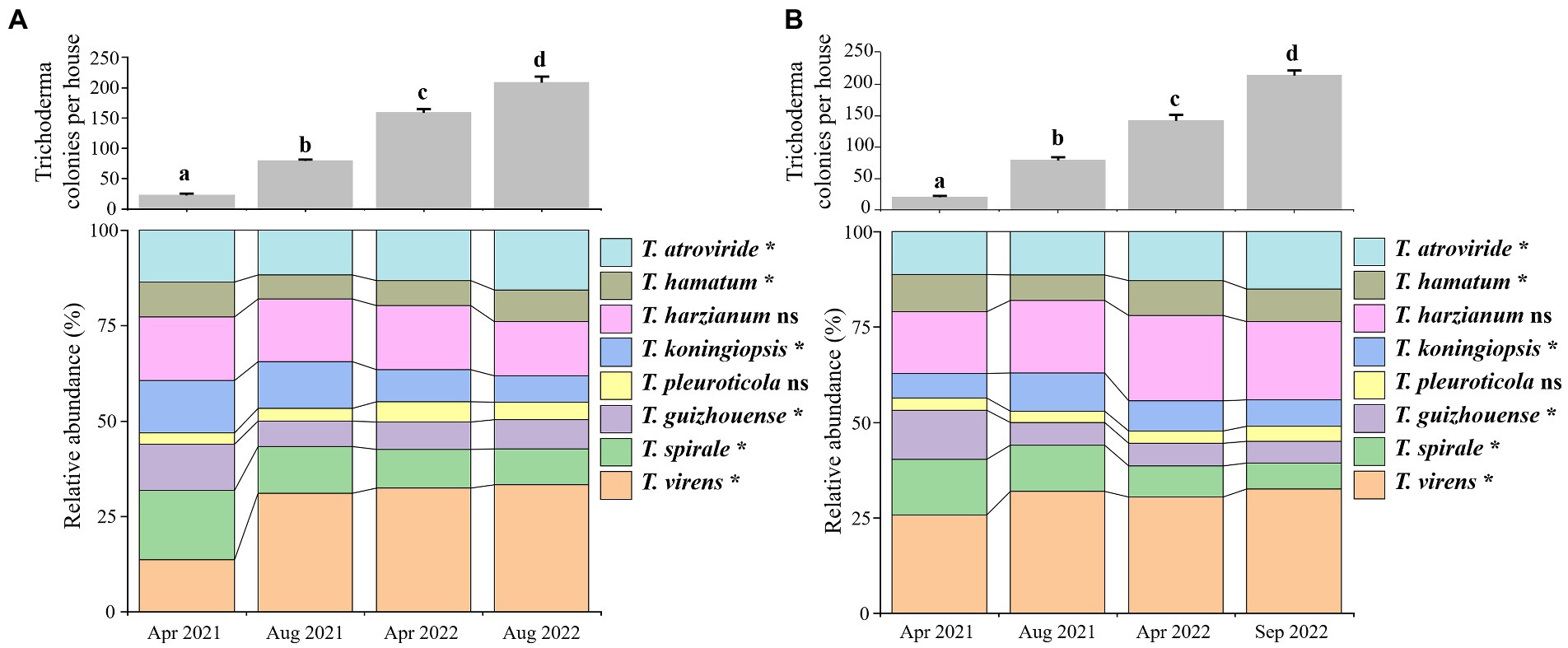
Figure 3. Population changes of Trichoderma spp. on the soil surface of Ganoderma lucidum cultivation houses in Lanju (A) and Chengbei (B) trails. The bar graphs show the increase in the colony number of all Trichoderma species on the soil surface per house. Data were the average number of Trichoderma colonies in six mushroom houses. Means in the plot topped by the different letters indicate the significant difference based on Duncan’s multiple range test of one-way ANOVA at p < 0.05. The lower panels show the taxonomic composition of Trichoderma communities in the different investigation periods at the species level. ns, not significant at p < 0.05; asterisk indicates significance at p < 0.05 based on Games–Howell post-hoc test and Benjamini–Hochberg false discovery rate (FDR) as the multiple test correction methods.
Moreover, population and proportion changes of the eight Trichoderma species in both trails were obtained (Figures 3A,B). Significant differences were observed in Trichoderma community during GL cultivation. T. virens was the most dominant species at the end of GL cultivation, accounting for 32.50 and 33.33% of the community in the Lanju and Chengbei trails, respectively. T. harzianum accounted for 20.37 and 14.10% of the community in the Lanju and Chengbei trails, respectively. T. pleuroticola was found in the lowest amount, accounting for 3.89 and 4.65% of the community in the Lanju and Chengbei trails, respectively.
Effects of different temperatures and pH levels on the mycelial growth of Trichoderma spp.
The strains from the eight Trichoderma spp. showed different growth rates at different temperatures (Figure 4). T. hamatum had the highest radium length at 15°C within 7 days post-inoculation, whereas T. pleuroticola had the lowest radium length, followed by T. virens. All Trichoderma species grew rapidly at 23°C and 31°C. The growth rate of T. pleuroticola at 31°C was higher than that at 23°C. T. harzianum grew rapidly compared to other species at 38°C. The eight Trichoderma species grew normally at pH 4.5–7.5 (Figure 5). Among these species, T. koningiopsis exhibited the lowest growth rate at pH 4.5. Overall, the eight Trichoderma species isolated from GL-cultivated soils could survive over a broad range of environmental temperatures and pH values.
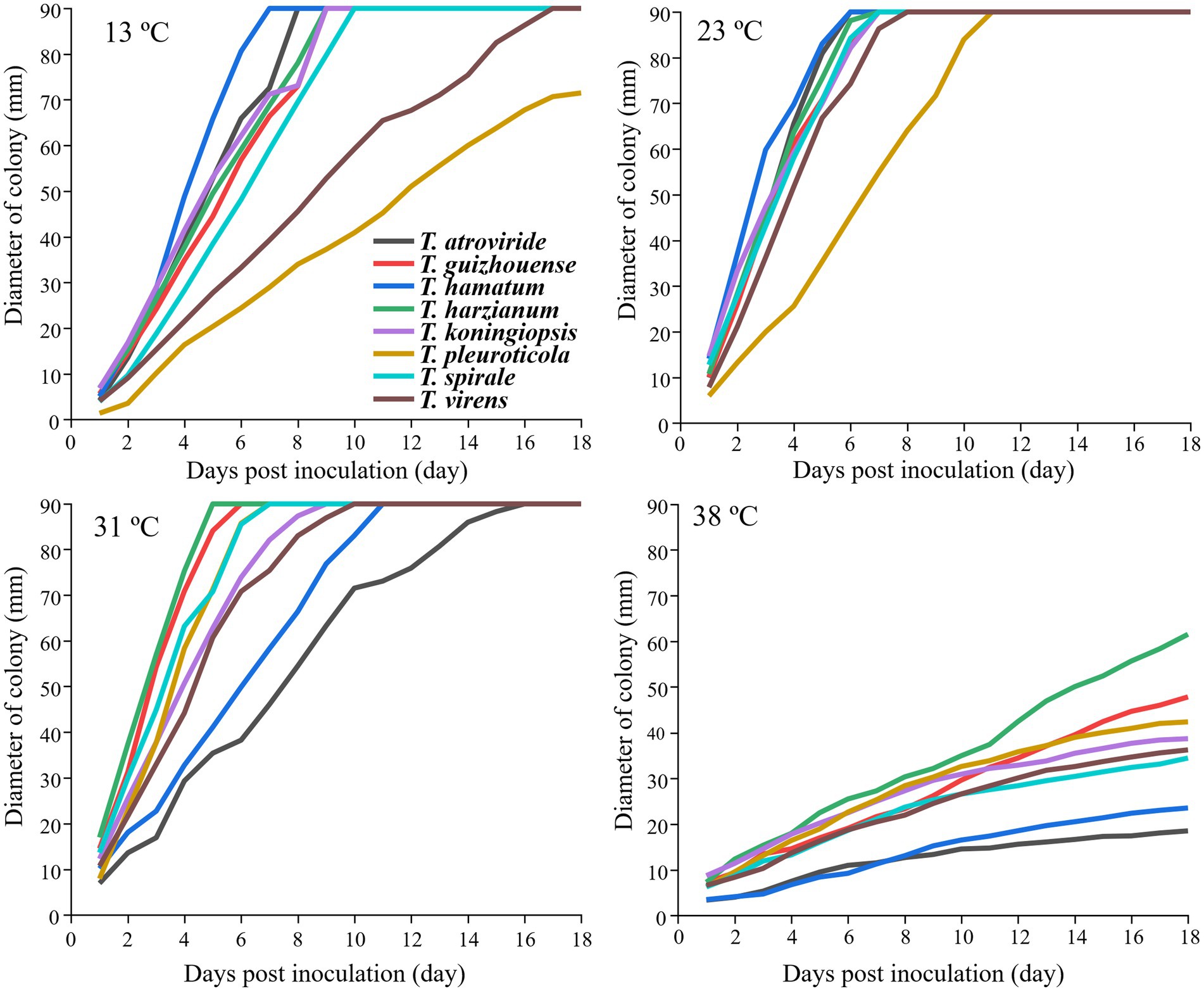
Figure 4. Effects of different temperatures on the mycelial growth of different Trichoderma strains on the PDA plates. Data represent the mean of two independent replicates with three plates each.
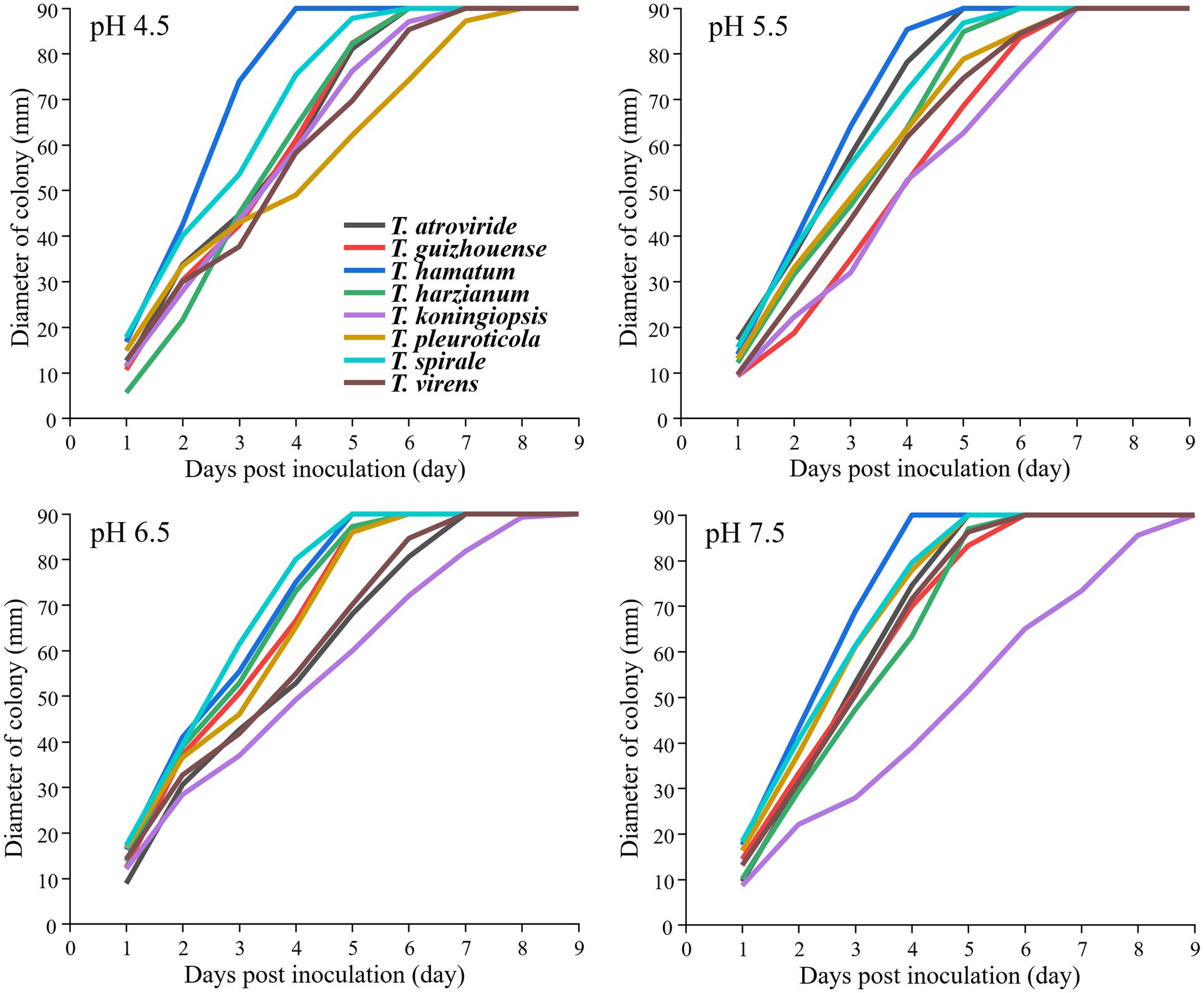
Figure 5. Effects of different pH levels on the mycelial growth of different Trichoderma strains on the PDA plates at 26°C. Data represent the mean of two independent replicates with three plates each.
Antagonistic activities of different Trichoderma species against GL
In dual confrontation assays, antagonistic zones were observed in the interactions between the eight representative isolates of different Trichoderma species and GL on PDA plates, which indicated that all eight species exhibited antifungal activities against GL (Figure 6). T. atroviride, T. harzianum and T. virens inhibited heavily GL mycelium growth. The growth of GL was also inhibited by T. guizhouense, T. hamatum, or T. koningiopsis. T. pleuroticola and T. spirale showed less inhibitive activity, but overgrew and spread on GL mycelia. Moreover, the inhibitory effects of non-volatile and volatile metabolites on GL varied among different species (Figure 7). The non-volatile metabolites from T. virens showed an inhibition rate of 85.42% for GL, exhibiting the strongest antagonistic activity against GL. T. guizhouense, T. harzianum, and T. koningiopsis showed low activities, accounting for inhibition rates of 67.62, 66.07, and 64.29%, respectively. Meanwhile, the volatiles from T. atroviride inhibited the mycelial growth of GL at the highest level (70.29%), followed by T. harzianum and T. koningiopsis. The inhibition rate of T. hamatum was the lowest (17.4%), followed by T. spirale.
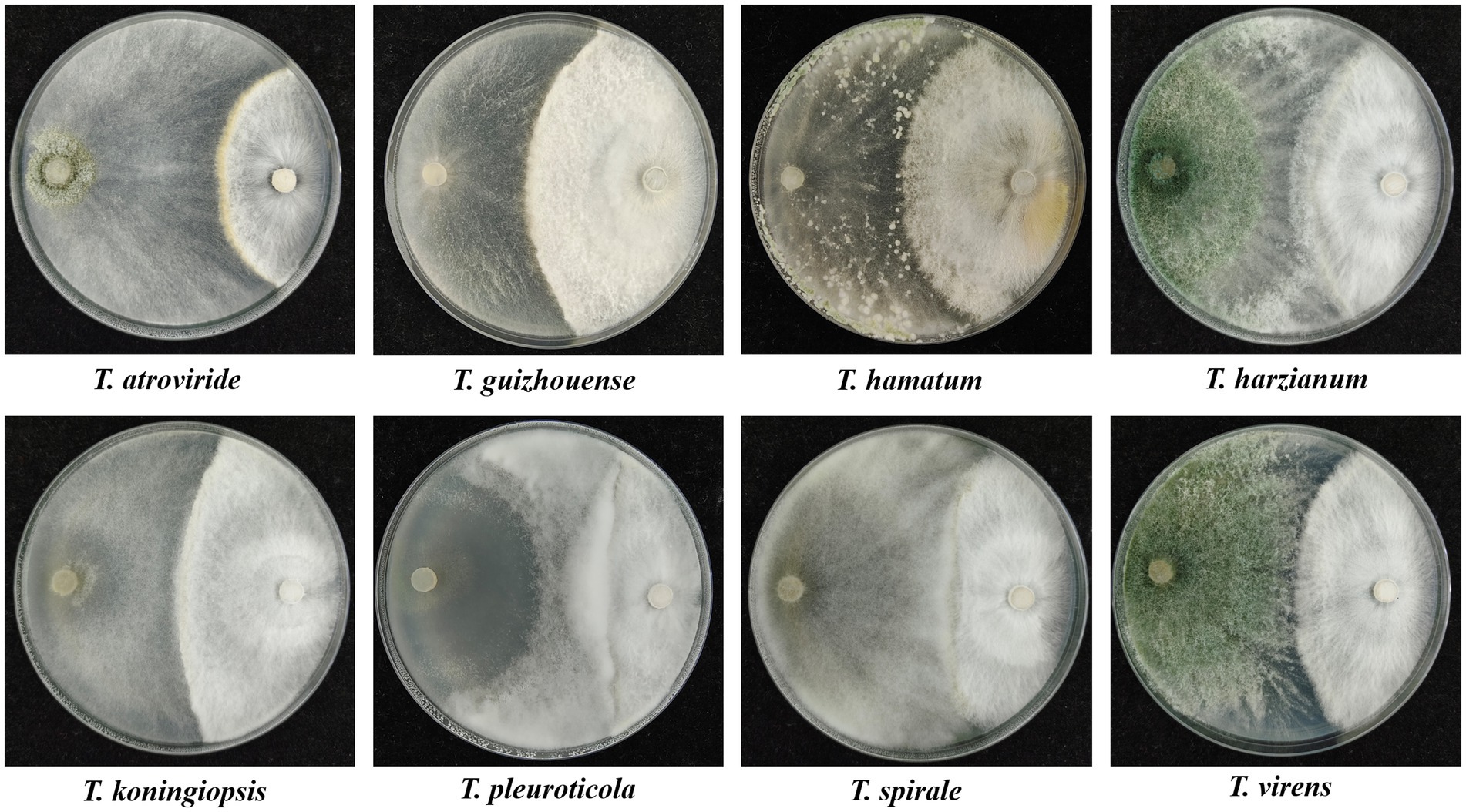
Figure 6. Dual cultures of the representative isolates of different Trichoderma species (left) against Ganoderma lucidum (right) on PDA plates for 6 days.
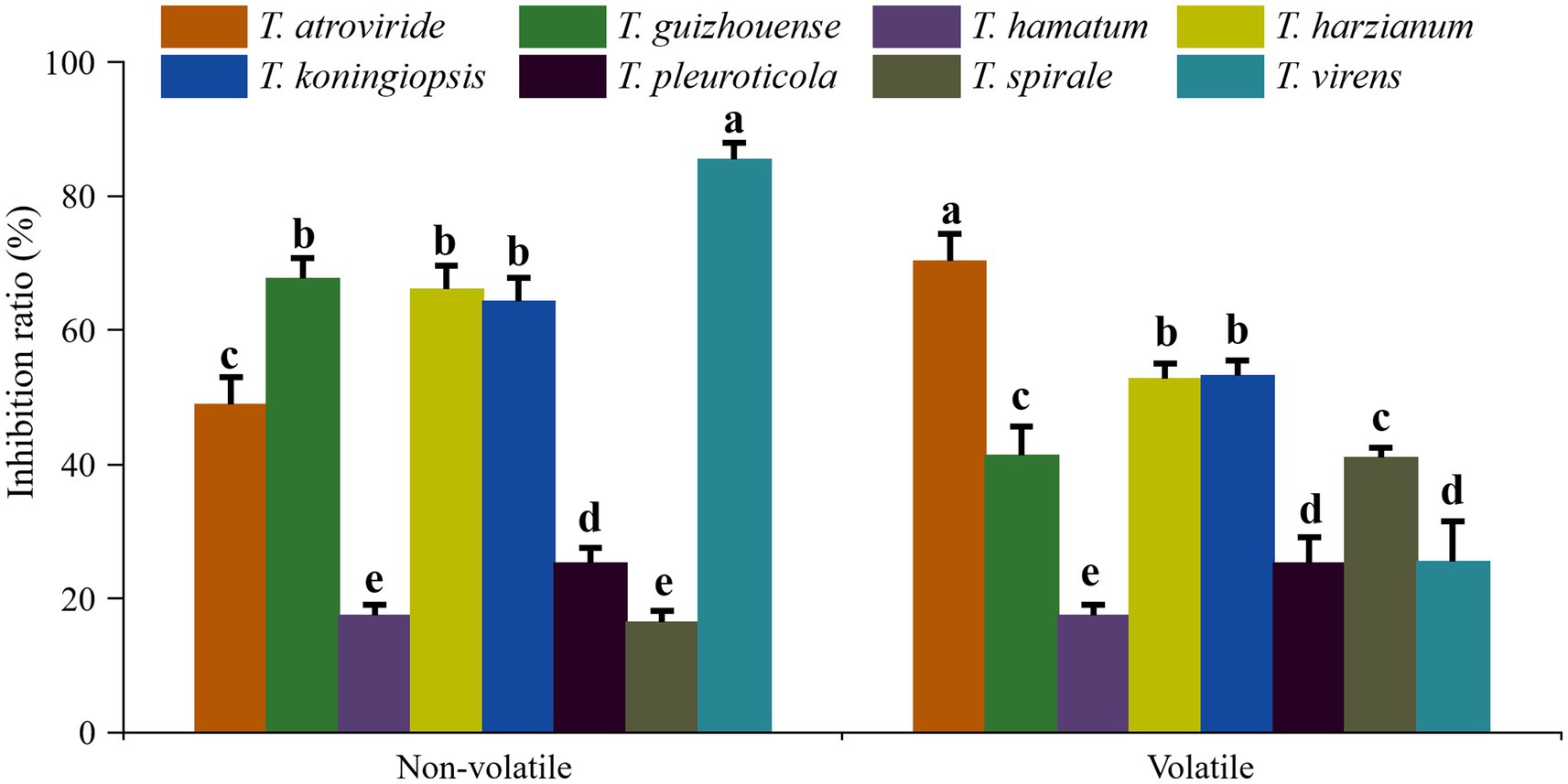
Figure 7. Inhibition rates of different Trichoderma species on the mycelial growth of Ganoderma lucidum. Data represent the mean of two independent replicates with five plates each. Significant differences (p < 0.05) between means are indicated using different letters.
Production of antifungal 6-PP in different Trichoderma species
In this study, the pure chemical 6-PP exhibited an inhibitory effect on GL mycelial growth (Table 1). The EC50 of 6-PP against GL was 8.79 μl mL−1. Moreover, the SPME–GC–MS analysis revealed that all the representative strains for eight Trichoderma species produced 6-PP, but with different levels of production (Figure 8). The differences in the peak areas showed that T. atroviride produced the largest amount of 6-PP, followed by T. guizhouense and T. harzianum. The production of 6-PP by T. pleuroticola was the lowest.

Table 1. Inhibitory effects of 6-pentyl-2H-pyran-2-one on mycelial growth of Ganoderma lucidum at 96 h post-inoculation.
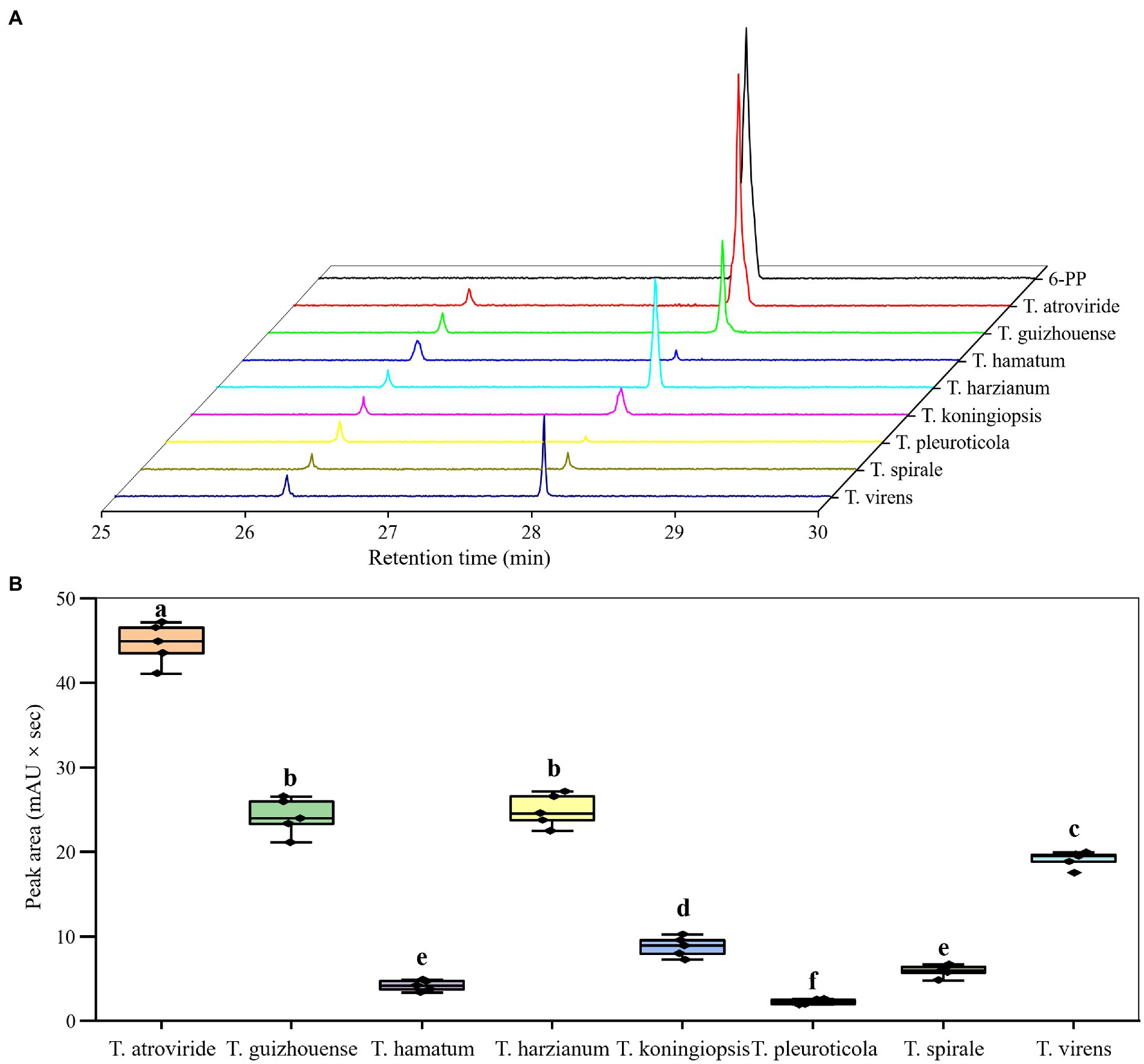
Figure 8. Production of 6–pentyl–2H–pyran–2–one (6-PP) by different Trichoderma species. (A) SPME–GC–MS profiles of the fungal volatiles produced by different Trichoderma species. (B) Difference peak areas of 6–PP from different Trichoderma species. Data represent the mean of three independent replicates. Significant differences (p < 0.05) between means are indicated by different letters.
Discussion
Edible and medical mushrooms are cultivated on a large scale in many countries; it is a key production in the circle of “turn waste into treasure” of agriculture chains (Grimm and Wosten, 2018). The traditional medical mushroom GL, which has high value for human medical supply and utilization of agricultural and forest resources, has been cultivated extensively (Li and Xu, 2022). Compared to many plant crops, GL cultivation requires higher costs and more labor owing to precise management and expensive equipment (Zhou, 2017). Mushroom diseases and growth inhibitors present risks to GL production. However, competition caused by many environmental microorganisms and mushroom diseases caused by some pathogens-present serious problems in GL cultivation (Ke et al., 2019; An et al., 2022). In this study, diverse Trichoderma species isolated from GL-cultivated soils were investigated. Eight Trichoderma species, including T. atrioviride, T. guizhouense, T. hamatum, T. harzianum, T. koningiopsis, T. pleuroticola, T. spirale, and T. virens, were identified. Competitive effects of these Trichoderma spp. on GL growth were demonstrated. These results provided detailed information on the cultivation challenges displayed by Trichoderma, affecting GL production in China (Tong et al., 2020).
Trichoderma spp. are well-known as soil inhabitants, existing under a wide range of environmental conditions (Harman, 2006). The diversity of Trichoderma species in soils has been extensively studied by different researchers worldwide (Jaklitsch and Voglmayr, 2015; Jiang et al., 2016; Nawaz et al., 2018; Dou et al., 2019). Moreover, ecosystem and climatic factors significantly influence Trichoderma diversity (Sariah et al., 2005; Widden and Scattolin, 2018; Hu et al., 2020). During mushroom cultivation, excess exogenous carbon and nitrogen resources are released into the casing soil from the degraded culture substrate, making it a unique ecosystem (Cai et al., 2009; McGee, 2018; Carrasco et al., 2019). Plenty of Trichoderma strains have been isolated from soils with mushroom cultivation (Hatvani et al., 2007; Wang et al., 2016; Oh et al., 2018; Allaga et al., 2021). Seven known Trichoderma species and a novel species, T. ganodermatigerum, were identified from the soils or fruiting bodies of G. sichuanense in northeast China (An et al., 2022). The high diversity of Trichoderma and increased population changes during GL cultivation in southeast China were demonstrated in this study.
Trichoderma spp. exhibit antagonistic activity against a broad spectrum of microorganisms (López-Bucio et al., 2015). This highly antagonistic characteristic would contribute to their rapid colonization and propagation in ecological niches (Schuster and Schmoll, 2010). In this study, all the eight representative strains for Trichoderma species exhibited antagonistic activities or competitive growth toward GL. Our results further evidenced that Trichoderma species showed various effects against GL. Similar phenomenon had been observed on A. bisporus (Innocenti et al., 2019) and L. edodes (Wang et al., 2016). The competitive activity based on antifungal metabolites and/or enzymes production, mycoparasitism, or ecological competition, might varied for different Trichoderma species (Vinale et al., 2008; Anees et al., 2010; Mukherjee et al., 2012). The direct effects of these Trichoderma spp. were expressed as the inhibition of GL growth by non-volatile and volatile metabolites. It was well documented that Trichoderma produced plenty of secondary metabolites with antifungal activity, including terpenes, pyrones, gliotoxin, peptaibols, etc., (Zeilinger et al., 2016; Khan et al., 2020). This highly antagonistic function may contribute to the accumulation of their population and threaten the growth and development of GL. In contrast, the broad perspective of microbial competition and antagonism of Trichoderma makes it one of the front-line microorganisms commonly employed in the preventive control of different plant pathogens (Zin and Badaluddin, 2020). Our data provide a potential strategy for investigating novel Trichoderma bioresources for application in sustainable agriculture.
The volatile metabolites produced by different Trichoderma spp. were highlighted in this study. Microbial volatile metabolites, mainly volatile organic compounds (VOCs), exhibit multiple biological functions (Contreras-Cornejo et al., 2014; Kanchiswamy et al., 2015; Kottb et al., 2015; Wonglom et al., 2020). High VOC production might require screening of the isolates or modification of the culture conditions (Flores et al., 2019; Wu et al., 2020). Among the various VOCs produced by Trichoderma spp., 6-PP is the most common and well-known compound that exhibits a broad-spectrum antagonism (El-Hasan et al., 2016; Elsherbiny et al., 2020; Bello et al., 2022). In this study, GL growth was substantially inhibited by 6-PP. Moreover, T. atroviride was the most efficient producer of 6-PP on PDA among the eight species and showed the highest level of inhibition against GL. These results were consistent with the findings of Jeleń et al. (2014).
Both T. harzianum and T. atroviride are aggressive pathogens causing green mold diseases in Ganoderma mushrooms (Lu et al., 2016; Yan et al., 2019). Several other Trichoderma spp., such as T. guizhouense, T. koningiopsis, and T. pleuroticola, are important pathogens in other mushrooms (Innocenti et al., 2019; Chen et al., 2021). These Trichoderma spp. can grow under a broad range of environmental temperatures and pH levels, which is consistent with our results. Moreover, Trichoderma spp. utilize diverse substrates and resist noxious chemicals (Ramírez-Valdespino and Orrantia-Borunda, 2021). Trichoderma produces large amounts of conidia for propagation and survival (Schuster and Schmoll, 2010). These factors impede the elimination of Trichoderma from mushroom cultivation. Currently, the management of Trichoderma-associated problems depends on fungicide use, such as prochloraz manganese and thiabendazole (Pecchia and Beyer, 2013; Subedi et al., 2021). Some beneficial Bacillus species have been used in the disease management of Agaricus bisporus (Stanojevic et al., 2019). In some cases, sanitation with waterlogging reduces Trichoderma inocula in mushroom cultivation (Bruno et al., 2015; Tong et al., 2020). The high diversity of Trichoderma and their rapid population growth in GL mushrooms suggest a further evaluation to control individual species.
In conclusion, the findings of this study provide detailed information on Trichoderma diversity in GL-cultivated soils in China. The accumulation of these antagonistic Trichoderma species might be a challenge in GL cultivation. Diverse effects of different Trichoderma species on GL were demonstrated in this study. To the best of our knowledge, our study is the first to provide data on the abundance of Trichoderma species associated with continuous cultivation problems in GL production. The information obtained in this study may provide a basis for the integrated management of cultivation problems in GL. Moreover, studies on the abundant Trichoderma species may provide novel resources for plant-beneficial microorganisms.
Data availability statement
The datasets presented in this study can be found in online repositories. The names of the repository/repositories and accession number(s) can be found in the article/Supplementary material.
Author contributions
YW: conceptualization, methodology, investigation, and writing-original draft preparation. LZ: investigation, data curation, and visualization. JW: software, validation. HJ: visualization, investigation. LM: supervision and writing-reviewing and editing. All authors contributed to the article and approved the submitted version.
Funding
This study was financially supported by a grant from the National Key Research and Development Program of China (2017YFD0201100-7) and grants from Zhejiang Key Research and Development Program of China (2019C0203002 and 2018C02034).
Acknowledgments
The authors give very special thanks to Senfu Zhou (Mushroom manager, Longquan County, China) for his assistance in the mushroom greenhouse.
Conflict of interest
The authors declare that the research was conducted in the absence of any commercial or financial relationships that could be construed as a potential conflict of interest.
Publisher’s note
All claims expressed in this article are solely those of the authors and do not necessarily represent those of their affiliated organizations, or those of the publisher, the editors and the reviewers. Any product that may be evaluated in this article, or claim that may be made by its manufacturer, is not guaranteed or endorsed by the publisher.
Supplementary material
The Supplementary material for this article can be found online at: https://www.frontiersin.org/articles/10.3389/fmicb.2022.1067822/full#supplementary-material
Footnotes
References
Allaga, H., Zhumakayev, A., Buchner, R., Kocsube, S., Szucs, A., Vagvolgyi, C., et al. (2021). Members of the Trichoderma harzianum species complex with mushroom pathogenic potential. Agronomy 11:2434. doi: 10.3390/agronomy11122434
An, X.-Y., Cheng, G.-H., Gao, H.-X., Li, X.-F., Yang, Y., Li, D., et al. (2022). Phylogenetic analysis of Trichoderma species associated with green mold disease on mushrooms and two new pathogens on Ganoderma sichuanense. J. Fungi 8:704. doi: 10.3390/jof8070704
Andreolli, M., Lampis, S., Brignoli, P., and Vallini, G. (2016). Trichoderma longibrachiatum Evx1 is a fungal biocatalyst suitable for the remediation of soils contaminated with diesel fuel and polycyclic aromatic hydrocarbons. Environ. Sci. Pollut. Res. Int. 23, 9134–9143. doi: 10.1007/s11356-016-6167-6
Anees, M., Tronsmo, A., Edel-Hermann, V., Hjeljord, L. G., Heraud, C., and Steinberg, C. (2010). Characterization of field isolates of Trichoderma antagonistic against Rhizoctonia solani. Fungal Biol. 114, 691–701. doi: 10.1016/j.funbio.2010.05.007
Babu, A. G., Shim, J., Bang, K. S., Shea, P. J., and Oh, B. T. (2014). Trichoderma virens PDR-28: a heavy metal-tolerant and plant growth-promoting fungus for remediation and bioenergy crop production on mine tailing soil. J. Environ. Manag. 132, 129–134. doi: 10.1016/j.jenvman.2013.10.009
Bello, F., Montironi, I. D., Medina, M. B., Munitz, M. S., Ferreira, F. V., Williman, C., et al. (2022). Mycofumigation of postharvest blueberries with volatile compounds from Trichoderma atroviride IC-11 is a promising tool to control rots caused by Botrytis cinerea. Food Microbiol. 106:104040. doi: 10.1016/j.fm.2022.104040
Bischof, R. H., Ramoni, J., and Seiboth, B. (2016). Cellulases and beyond: the first 70 years of the enzyme producer Trichoderma reesei. Microb. Cell Factories 15:106. doi: 10.1186/s12934-016-0507-6
Bishop, K. S., Kao, C. H., Xu, Y., Glucina, M. P., Paterson, R. R., and Ferguson, L. R. (2015). From 2000 years of Ganoderma lucidum to recent developments in nutraceuticals. Phytochemistry 114, 56–65. doi: 10.1016/j.phytochem.2015.02.015
Boh, B., Berovic, M., Zhang, J., and Zhi-Bin, L. (2007). Ganoderma lucidum and its pharmaceutically active compounds. Biotechnol. Annu. Rev. 13, 265–301. doi: 10.1016/S1387-2656(07)13010-6
Bruno, G. L., De Corato, U., Rana, G. L., De Luca, P., Pipoli, V., Lops, R., et al. (2015). Suppressiveness of white vinegar and steam-exploded liquid waste against the causal agents of Pleurotus eryngii yellowing. Crop Prot. 70, 61–69. doi: 10.1016/j.cropro.2015.01.006
Cai, F., and Druzhinina, I. S. (2021). In honor of John Bissett: authoritative guidelines on molecular identification of Trichoderma. Fungal Divers. 107, 1–69. doi: 10.1007/s13225-020-00464-4
Cai, W.-M., Yao, H.-Y., Feng, W.-L., Jin, Q.-L., Liu, Y.-Y., Li, N.-Y., et al. (2009). Microbial community structure of casing soil during mushroom growth. Pedosphere 19, 446–452. doi: 10.1016/S1002-0160(09)60137-5
Cao, Y., Wu, S. H., and Dai, Y. C. (2012). Species clarification of the prize medicinal Ganoderma mushroom “Lingzhi”. Fungal Divers. 56, 49–62. doi: 10.1007/s13225-012-0178-5
Carrasco, J., Tello, M. L., De Toro, M., Tkacz, A., Poole, P., Perez-Clavijo, M., et al. (2019). Casing microbiome dynamics during button mushroom cultivation: implications for dry and wet bubble diseases. Microbiology 165, 611–624. doi: 10.1099/mic.0.000792
Chang, C. J., Lin, C. S., Lu, C. C., Martel, J., Ko, Y. F., Ojcius, D. M., et al. (2015). Ganoderma lucidum reduces obesity in mice by modulating the composition of the gut microbiota. Nat. Commun. 6:7489. doi: 10.1038/ncomms8489
Chen, X., Zhou, X. H., Zhao, J., Tang, X., Pasquali, M., Migheli, Q., et al. (2021). Occurrence of green mold disease on Dictyophora rubrovolvata caused by Trichoderma koningiopsis. J. Plant Pathol. 103, 981–984. doi: 10.1007/s42161-021-00861-x
Contreras-Cornejo, H. A., Macías-Rodríguez, L., Herrera-Estrella, A., and López-Bucio, J. (2014). The 4-phosphopantetheinyl transferase of Trichoderma virens plays a role in plant protection against Botrytis cinerea through volatile organic compound emission. Plant Soil 379, 261–274. doi: 10.1007/s11104-014-2069-x
Dou, K., Gao, J., Zhang, C., Yang, H., Jiang, X., Li, J., et al. (2019). Trichoderma biodiversity in major ecological systems of China. J. Microbiol. 57, 668–675. doi: 10.1007/s12275-019-8357-7
El-Hasan, A., Walker, F., Schöne, J., and Buchenauer, H. (2016). Antagonistic effect of 6-pentyl-alpha-pyrone produced by Trichoderma harzianum toward Fusarium moniliforme. J. Plant Dis. Prot. 114, 62–68. doi: 10.1007/s12275-019-8357-7
Elsherbiny, E. A., Amin, B. H., Aleem, B., Kingsley, K. L., and Bennett, J. W. (2020). Trichoderma volatile organic compounds as a biofumigation tool against late blight pathogen Phytophthora infestans in postharvest potato tubers. J. Agric. Food Chem. 68, 8163–8171. doi: 10.1021/acs.jafc.0c03150
Flores, C., Nieto, M., Millán-Gómez, D. V., Caro, M., Galindo, E., and Serrano-Carreón, L. (2019). Elicitation and biotransformation of 6-pentyl-α-pyrone in Trichoderma atroviride cultures. Process Biochem. 82, 68–74. doi: 10.1016/j.procbio.2019.04.019
Grimm, D., and Wosten, H. A. B. (2018). Mushroom cultivation in the circular economy. Appl. Microbiol. Biotechnol. 102, 7795–7803. doi: 10.1007/s00253-018-9226-8
Harman, G. E. (2006). Overview of mechanisms and uses of Trichoderma spp. Phytopathology 96, 190–194. doi: 10.1094/PHYTO-96-0190
Hatvani, L., Antal, Z., Manczinger, L., Szekeres, A., Druzhinina, I. S., Kubicek, C. P., et al. (2007). Green mold diseases of Agaricus and Pleurotus spp. are caused by related but phylogenetically different Trichoderma species. Phytopathology 97, 532–537. doi: 10.1094/PHYTO-97-4-0532
Hu, J., Zhou, Y., Chen, K., Li, J., Wei, Y., Wang, Y., et al. (2020). Large-scale Trichoderma diversity was associated with ecosystem, climate and geographic location. Environ. Microbiol. 22, 1011–1024. doi: 10.1111/1462-2920.14798
Innocenti, G., Montanari, M., Righini, H., and Roberti, R. (2019). Trichoderma species associated with green mould disease of Pleurotus ostreatus and their sensitivity to prochloraz. Plant Pathol. 68, 392–398. doi: 10.1111/ppa.12953
Jaklitsch, W. M., and Voglmayr, H. (2015). Biodiversity of Trichoderma (Hypocreaceae) in Southern Europe and Macaronesia. Stud. Mycol. 80, 1–87. doi: 10.1016/j.simyco.2014.11.001
Jeleń, H., Blaszczyk, L., Chelkowski, J., Rogowicz, K., and Strakowska, J. (2014). Formation of 6-n-pentyl-2H-pyran-2-one (6-PAP) and other volatiles by different Trichoderma species. Mycol. Prog. 13, 589–600. doi: 10.1007/s11557-013-0942-2
Jiang, Y., Wang, J. L., Chen, J., Mao, L. J., Feng, X. X., Zhang, C. L., et al. (2016). Trichoderma biodiversity of agricultural fields in East China reveals a gradient distribution of species. PLoS One 11:e0160613. doi: 10.1371/journal.pone.0160613
Kanchiswamy, C. N., Malnoy, M., and Maffei, M. E. (2015). Bioprospecting bacterial and fungal volatiles for sustainable agriculture. Trends Plant Sci. 20, 206–211. doi: 10.1016/j.tplants.2015.01.004
Ke, L. Q., Li, P. D., Xu, J. P., Wang, Q. S., Wang, L. L., and Wen, H. P. (2019). Microbial communities and soil chemical features associated with commercial production of the medicinal mushroom Ganoderma lingzhi in soil. Sci. Rep. 9:15839. doi: 10.1038/s41598-019-52368-2
Khan, R. A. A., Najeeb, S., Hussain, S., Xie, B., and Li, Y. (2020). Bioactive secondary metabolites from Trichoderma spp. against phytopathogenic fungi. Microorganisms 8:817. doi: 10.3390/microorganisms8060817
Kim, C. S., Park, M. S., Kim, S. C., Maekawa, N., and Yu, S. H. (2012). Identification of Trichoderma, a competitor of shiitake mushroom (Lentinula edodes), and competition between Lentinula edodes and Trichoderma species in Korea. Plant Pathol. J. 28, 137–148. doi: 10.5423/PPJ.2012.28.2.137
Komon-Zelazowska, M., Bissett, J., Zafari, D., Hatvani, L., Manczinger, L., Woo, S., et al. (2007). Genetically closely related but phenotypically divergent Trichoderma species cause green mold disease in oyster mushroom farms worldwide. Appl. Environ. Microbiol. 73, 7415–7426. doi: 10.1128/AEM.01059-07
Kottb, M., Gigolashvili, T., Grosskinsky, D. K., and Piechulla, B. (2015). Trichoderma volatiles effecting Arabidopsis: from inhibition to protection against phytopathogenic fungi. Front. Microbiol. 6:995. doi: 10.3389/fmicb.2015.00995
Kumar, S., Stecher, G., and Tamura, K. (2016). MEGA7: molecular evolutionary genetics analysis version 7.0 for bigger datasets. Mol. Biol. Evol. 33, 1870–1874. doi: 10.1093/molbev/msw054
Li, N., Alfiky, A., Wang, W., Islam, M., Nourollahi, K., Liu, X., et al. (2018). Volatile compound-mediated recognition and inhibition between Trichoderma biocontrol agents and Fusarium oxysporum. Front. Microbiol. 9:2614. doi: 10.3389/fmicb.2018.02614
Li, C., and Xu, S. (2022). Edible mushroom industry in China: current state and perspectives. Appl. Microbiol. Biotechnol. 106, 3949–3955. doi: 10.1007/s00253-022-11985-0
Li, Q.-Y., Zhong, Y.-Y., Chen, Y.-X., Zhou, W.-X., Zeng, Z.-J., and Song, B. (2016). Research advances on the production technology of Ganoderma in China (in Chinese). Edible Fungi Chin. 35, 8–12. doi: 10.13629/j.cnki.53-1054.2016.01.002
Liu, D., Gong, J., Dai, W., Kang, X., Huang, Z., Zhang, H. M., et al. (2012). The genome of Ganoderma lucidum provides insights into triterpenes biosynthesis and wood degradation. PLoS One 7:e36146. doi: 10.1371/journal.pone.0036146
López-Bucio, J., Pelagio-Flores, R., and Herrera-Estrella, A. (2015). Trichoderma as biostimulant: exploiting the multilevel properties of a plant beneficial fungus. Sci. Hortic. 196, 109–123. doi: 10.1016/j.scienta.2015.08.043
Lu, B. H., Zuo, B., Liu, X. L., Feng, J., Wang, Z. M., and Gao, J. (2016). Trichoderma harzianum causing green mold disease on cultivated Ganoderma lucidum in Jilin Province, China. Plant Dis. 100, 2524–2525. doi: 10.1094/PDIS-04-16-0422-PDN
McGee, C. F. (2018). Microbial ecology of the Agaricus bisporus mushroom cropping process. Appl. Microbiol. Biotechnol. 102, 1075–1083. doi: 10.1007/s00253-017-8683-9
Mukherjee, P. K., Horwitz, B. A., and Kenerley, C. M. (2012). Secondary metabolism in Trichoderma--a genomic perspective. Microbiology 158, 35–45. doi: 10.1099/mic.0.053629-0
Nawaz, A. K., Shahida, A. A., Bengyella, L., Subhani, M. N., Ali, M., Anwar, W., et al. (2018). Diversity of Trichoderma species in chili rhizosphere that promote vigor and antagonism against virulent Phytophthora capsici. Sci. Hortic. 239, 242–252. doi: 10.1016/j.scienta.2018.05.048
Oh, S. Y., Park, M. S., Cho, H. J., and Lim, Y. W. (2018). Diversity and effect of Trichoderma isolated from the roots of Pinus densiflora within the fairy ring of pine mushroom (Tricholoma matsutake). PLoS One 13:e0205900. doi: 10.1371/journal.pone.0205900
Pecchia, J., and Beyer, D. (2013). Pest management on US commercial mushroom farms. Outlooks Pest Manage. 24, 28–29. doi: 10.1564/v24_feb_08
Ramírez-Valdespino, C. A., and Orrantia-Borunda, E. (2021). Trichoderma and nanotechnology in sustainable agriculture: a review. Front. Fungal Biol. 2:764675. doi: 10.3389/ffunb.2021.764675
Rao, Y., Zeng, L., Jiang, H., Mei, L., and Wang, Y. (2022). Trichoderma atroviride LZ42 releases volatile organic compounds promoting plant growth and suppressing Fusarium wilt disease in tomato seedlings. BMC Microbiol. 22:88. doi: 10.1186/s12866-022-02511-3
Royse, D. J., Boomer, K., Du, Y., Handcock, M., Coles, P. S., and Romaine, C. P. (1999). Spatial distribution of green mold foci in 30 commercial mushroom crops. Plant Dis. 83, 71–76. doi: 10.1094/PDIS.1999.83.1.71
Sariah, M., Choo, C. W., Zakaria, H., and Norihan, M. S. (2005). Quantification and characterisation of Trichoderma spp. from different ecosystems. Mycopathologia 159, 113–117. doi: 10.1007/s11046-004-4432-6
Schuster, A., and Schmoll, M. (2010). Biology and biotechnology of Trichoderma. Appl. Microbiol. Biotechnol. 87, 787–799. doi: 10.1007/s00253-010-2632-1
Staden, R., Beal, K. F., and Bonfield, J. K. (2000). “The Staden Package, 1998”, in Bioinformatics Methods and Protocols. eds. S. Misener and S. A. Krawetz (Totowa: Humana Press).
Stanojevic, O., Beric, T., Potocnik, I., Rekanovic, E., Stankovic, S., and Milijaevic-Marcic, S. (2019). Biological control of green mould and dry bubble diseases of cultivated mushroom (Agaricus bisporus L.) by bacillus spp. Crop Prot. 126:104944. doi: 10.1016/j.cropro.2019.104944
Stoppacher, N., Kluger, B., Zeilinger, S., Krska, R., and Schuhmacher, R. (2010). Identification and profiling of volatile metabolites of the biocontrol fungus Trichoderma atroviride by HS-SPME-GC-MS. J. Microbiol. Methods 81, 187–193. doi: 10.1016/j.mimet.2010.03.011
Subedi, S., Joshi, Y. R., Pandey, K. R., and Ghimire, A. (2021). Major fungal contaminants of mushrooms and their management. Int. J. Appl. Sci. Biotechnol. 9, 80–93. doi: 10.3126/ijasbt.v9i2.37513
Tong, X., Jiang, H., Liang, Y., Rao, Y., Mei, L., and Wang, Y. (2020). Waterlogging reduces soil colonization by antagonistic fungi and restores production in Ganoderma lucidum continuous cultivation. Crop Prot. 137:105314. doi: 10.1016/j.cropro.2020.105314
Vinale, F., Sivasithamparam, K., Ghisalberti, E. L., Marra, R., Woo, S. L., and Lorito, M. (2008). Trichoderma–plant–pathogen interactions. Soil Biol. Biochem. 40, 1–10. doi: 10.1016/j.soilbio.2007.07.002
Wang, G., Cao, X., Ma, X., Guo, M., Liu, C., Yan, L., et al. (2016). Diversity and effect of Trichoderma spp. associated with green mold disease on Lentinula edodes in China. Microbiology 5, 709–718. doi: 10.1002/mbo3.364
Widden, P., and Scattolin, V. (2018). Competitive interactions and ecological strategies of Trichoderma species colonizing spruce litter. Mycologia 80, 795–803. doi: 10.1080/00275514.1988.12025726
Wonglom, P., Ito, S., and Sunpapao, A. (2020). Volatile organic compounds emitted from endophytic fungus Trichoderma asperellum T1 mediate antifungal activity, defense response and promote plant growth in lettuce (Lactuca sativa). Fungal Ecol. 43:100867. doi: 10.1016/j.funeco.2019.100867
Wu, F., Tong, X., Zhang, L., Mei, L., Guo, Y., and Wang, Y. (2020). Suppression of Rhizopus fruit rot by volatile organic compounds produced by Paenibacillus polymyxa CF05. Biocontrol Sci. Tech. 30, 1351–1364. doi: 10.1080/09583157.2020.1826902
Yan, Y. H., Zhang, C. L., Moodley, O., Zhang, L., and Xu, J. Z. (2019). Green mold caused by Trichoderma atroviride on the lingzhi medicinal mushroom, Ganoderma lingzhi (Agaricomycetes). Int. J. Med. Mushrooms 21, 515–521. doi: 10.1615/IntJMedMushrooms.2019030352
Zeilinger, S., Gruber, S., Bansal, R., and Mukherjee, P. K. (2016). Secondary metabolism in Trichoderma–chemistry meets genomics. Fungal Biol. Rev. 30, 74–90. doi: 10.1016/j.fbr.2016.05.001
Zhang, H., Cao, R., and Ye, S. (2004). Short wood-log cultivation of Ganoderma lucidum with high performance (in Chinese). J. Zhejiang Agric. Sci. 3, 136–138. doi: 10.16178/j.issn.0528-9017.2004.03.013
Zhou, X.-W. (2017). “Cultivation of Ganoderma lucidum” in Edible and Medicinal Mushrooms: Technology and Applications. ed. A. Pardo-Giménez (Chichester: Wiley), 385–413.
Keywords: species diversity, adverse effect, cultivation obstacle, volatile compounds, Ganoderma lucidum, Trichoderma spp.
Citation: Wang Y, Zeng L, Wu J, Jiang H and Mei L (2022) Diversity and effects of competitive Trichoderma species in Ganoderma lucidum–cultivated soils. Front. Microbiol. 13:1067822. doi: 10.3389/fmicb.2022.1067822
Edited by:
Entao Wang, Instituto Politécnico Nacional (IPN), MexicoReviewed by:
Lourdes Macias-Rodriguez, Universidad Michoacana de San Nicolás de Hidalgo, MexicoAida Veronica Rodriguez-Tovar, Instituto Politécnico Nacional (IPN), Mexico
Copyright © 2022 Wang, Zeng, Wu, Jiang and Mei. This is an open-access article distributed under the terms of the Creative Commons Attribution License (CC BY). The use, distribution or reproduction in other forums is permitted, provided the original author(s) and the copyright owner(s) are credited and that the original publication in this journal is cited, in accordance with accepted academic practice. No use, distribution or reproduction is permitted which does not comply with these terms.
*Correspondence: Yongjun Wang, yongjun_wang@hotmail.com; Li Mei, meili@zafu.edu.cn
†These authors have contributed equally to this work and share first authorship