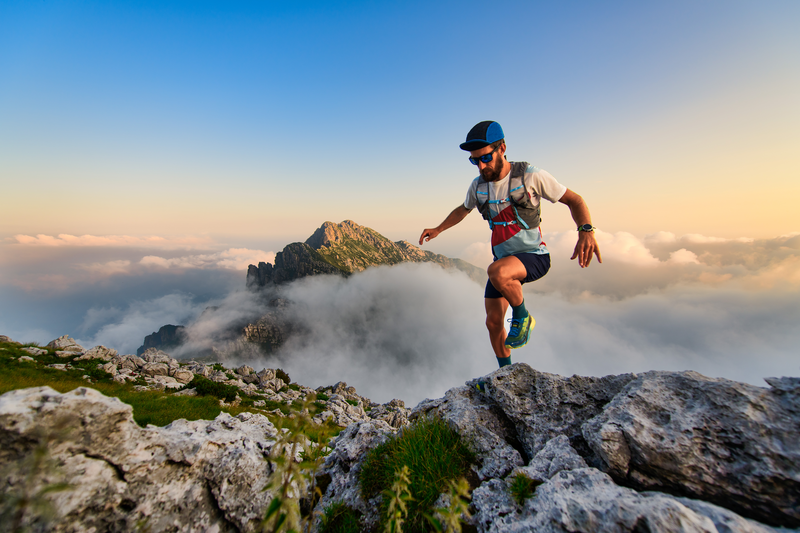
94% of researchers rate our articles as excellent or good
Learn more about the work of our research integrity team to safeguard the quality of each article we publish.
Find out more
METHODS article
Front. Microbiol. , 05 January 2023
Sec. Antimicrobials, Resistance and Chemotherapy
Volume 13 - 2022 | https://doi.org/10.3389/fmicb.2022.1062577
This article is part of the Research Topic Emerging Multidrug-Resistant Bacterial Pathogens "superbugs": A Rising Public Health Threat View all 7 articles
The worrying emergence of multiple resistance genes to last-resort antibiotics in food animals and human populations throughout the food chain and relevant environments has been increasingly reported worldwide. Enterobacteriaceae pathogens are considered the most common reservoirs of such antibiotic resistance genes (ARGs). Thus, a rapid, efficient and accurate detection method to simultaneously screen and monitor such ARGs in Enterobacteriaceae pathogens has become an urgent need. Our study developed a recombinase polymerase amplification (RPA) assay combined with a lateral flow dipstick (LFD) for simultaneously detecting predominant resistance genes to last-resort antibiotics of Enterobacteriaceae pathogens, including mcr-1, blaNDM-1 and tet(X4). It is allowed to complete the entire process, including crude DNA extraction, amplification as well as reading, within 40 min at 37°C, and the detection limit is 101 copies/μl for mcr-1, blaNDM-1 and tet(X4). Sensitivity analysis showed obvious association of color signals with the template concentrations of mcr-1, blaNDM-1 and tet(X4) genes in Enterobacteriaceae pathogens using a test strip reader (R2 = 0.9881, R2 = 0.9745, and R2 = 0.9807, respectively), allowing for quantitative detection using multiplex RPA-LFD assays. Therefore, the RPA-LFD assay can suitably help to detect multiple resistance genes to last-resort antibiotics in foodborne pathogens and has potential applications in the field.
Imprudently using antibiotics in medicine and farming has resulted in increasingly severe bacterial resistance problems around the world (Fu et al., 2022). According to the World Health Organization (WHO), antibiotic resistance remarkably threatens food security, and global health and development (World Health Organization, 2022). Multidrug resistance (MDR) occurs when bacteria obtain resistance to three or more categories of antibiotics, causing them to pose more urgent threats (Cheng et al., 2019). Among the currently available antibiotics, carbapenems, colistin as well as tigecycline are last-resort antibiotics for managing MDR bacterial infections, especially those caused by Enterobacteriaceae (Tang et al., 2021). Unfortunately, the worrying emergence of the predominantly plasmid-borne colistin resistance gene mcr-1, carbapenem resistance gene blaNDM-1 as well as tigecycline resistance gene tet(X4) in Enterobacteriaceae pathogens from different sources, such as animals, food and humans, has increasingly been reported in different continents (Singh et al., 2018; Zhong et al., 2019; Anyanwu et al., 2022; Lu et al., 2022; Zhang et al., 2022). Additionally, tet(X) genes have regularly been reported to coexist with blaNDM-1 and/or mcr-1 genes (Chen et al., 2019; Sun C. et al., 2019), and such plasmid-borne antibiotic resistance genes (ARGs) are capable of transferring between epidemic strains of Enterobacteriaceae (Leshaba et al., 2022). Thus, a fast, efficient and accurate detection method for simultaneously screening and monitoring the above-mentioned ARGs in Enterobacteriaceae pathogens has become urgently needed for managing the dissemination of resistance in food animal and human populations throughout the food chain and relevant environments.
The most widely used molecular-based method for MDR gene detection is polymerase chain reaction (PCR), including conventional PCR (Adekunle et al., 2021; Cooper et al., 2021), real-time quantitative PCR (Park et al., 2021; Tolosi et al., 2021) and digital PCR (Xu et al., 2021; Merino et al., 2022). However, most farms in China are equipped with laboratories capable of isolating bacteria from environmental samples, but are generally not equipped with complex large-scale instruments such as qPCR (Ma et al., 2020). A method which is simpler to operate and less dependent on instruments is urgently needed. To date, researchers have developed novel simple, rapid and efficient and cost-effective molecular biology techniques, such as isothermal nucleic acid amplification, as alternative protocols for the rapid on-site evaluation of MDR genes (Wong et al., 2018; Li et al., 2019; Tran et al., 2022). Recombinase polymerase amplification (RPA) acts as a common nucleic acid isothermal amplification technologies first developed by Piepenburg et al. (2006). It adopts a phage recombinase for forming complexes with oligonucleotide primers, thereby facilitating oligonucleotide primers to bind to homologous sequences of the double-stranded DNA molecules (Lobato and O'Sullivan, 2018). It is allowed to accomplish amplification within 30 min at a constant low temperature around 37°C by combining with a single-stranded DNA binding protein and a strand-displacing polymerase (Lobato and O'Sullivan, 2018). In addition, only a few copies of target DNA are initially needed, and highly specific DNA amplification at detectable levels can be achieved within a short time (Kersting et al., 2014). Furthermore, multiplexing RPA amplifications in the same solution is feasible, despite its strong dependence on the amplicon size, target sequences, as well as primer design (Kersting et al., 2014). Detection of RPA products is mainly completed via probe-based florescence (Kim and Lee, 2016), gel electrophoresis (Cao et al., 2018), or visualization by nucleic acid lateral flow dipstick (LFD) immunoassays (Xu et al., 2018). Among these, LFD immunoassays are simple, fast (~5 min), cost-effective and accurate for detection of amplified products and are more suitable for point-of-care testing (Lin et al., 2022). Additionally, multiplex LFD immunoassays that analyze multiple targets simultaneously have emerged in several research fields. The most common solution is to prepare several test lines on one test strip (Di Nardo et al., 2021). For instance, Cavalera et al. reported a multi-target LFD immunoassay with two test lines that bound to several classes of immunoglobulins, which realized detection of total antibodies against SARS-CoV-2 (Cavalera et al., 2021). Such designs further reduce cost and increase throughput of detection, which is vital to the study of MDR genes. Recently, to meet increased accuracy requirements for quantitative detection, test strip readers (Li et al., 2019) and image software (Wang et al., 2021) have been employed to scan and evaluate the color signals of LFD.
The study focused on developing an RPA-LFD method for simultaneously identifying the highly prevalent genes mcr-1, blaNDM-1 and tet(X4) in certain Enterobacteriaceae pathogens isolated from different food and animal fecal samples. In addition, a TSR-200 test strip reader (Allsheng Instruments Co., Ltd., Hangzhou, China) assisted in evaluating color signals to use LFD for accurate quantitative analysis. This allows for immediate monitoring of MDR genes in pathogenic bacteria in the field and will inform risk assessment and remediation strategies in a timely manner.
We used 19 MDR Enterobacteriaceae strains, including 14 Escherichia coli strains, 1 Escherichia fergusonii strain, 2 Klebsiella pneumoniae strains and 2 Salmonella spp. strains, for confirming the multiplex RPA-LFD assay specificity and its application in the field in our study. Detailed information on isolates is shown in Supplementary Table S1. The three recombinant E. coli strains containing standard plasmids carrying mcr-1, blaNDM-1 or tet(X4) genes were previously constructed in our laboratory to be used as reference strains for optimizing the reaction system and analyzing the sensitivity (Supplementary Table S1).
The standard DNA plasmids that carried mcr-1, blaNDM-1 and tet(X4) genes were extracted from recombinant E. coli Top10-pUC-mcr-1, E. coli Top10-pUC-blaNDM-1 and E. coli Top10-pUC-tet(X4) using a QIAGEN Plasmid Mini Kit (QIAGEN, Hilden, Germany) according to the manufacturer’s instructions.
The DNA of all Enterobacteriaceae strains was prepared using the previously described boil-up protocol with some modifications (Bordin et al., 2019). Briefly, a 1 μl solution of each Enterobacteriaceae strain was added into 30 μl 10 mM Tris buffer, boiled for 5 min in a metal bath, cooled on ice for 2 min together with 2 min of centrifugation at 12, 000 × g. RPA-LFD assays took the supernatant as the DNA template.
The ARG sequences of mcr-1 (KP347127.1), blaNDM-1 (NG_049326.1) and tet(X4) (MK134376.1) came from GenBank.1 Multiple gene sequence alignments of mcr-1, blaNDM-1 and tet(X4) available from GenBank were analyzed using ClustalW. Based on the conserved sequences, Primer Premier 5 served for designing RPA primers and LF probes following the instruction manual provided by TwistDX (Cambridge, United Kingdom). The specificity exhibited by all RPA primers and LF probes was evaluated in silico using the BLASTn tool of the NCBI database. In this study, the 5′ ends of the reverse primers for mcr-1, blaNDM-1 and tet(X4) were all labeled with digoxin. The 5′ ends of the LF probes for mcr-1, blaNDM-1 and tet(X4) were labeled with biotin, cyanine 5 (Cy5) and carboxytetramethylrhodamine (TAMRA), respectively. The 3′ end of the LF probe was modified with 3′ spacer C3. The inside of the LF probe was modified with an internal abasic nucleotide analog dSpacer (tetrahydrofuran, THF) (Table 1). GENEray Biotechnology (Shanghai, China) helped to synthesize all PRA primers and LF probes.
LFD strips were prepared via methods described in previous studies with some modifications (Ma et al., 2020; Jin et al., 2022). Briefly, the LFD strips were composed of continuous superposition: sample pad, conjugate pad with gold nanoparticles (AuNPs), nitrocellulose filter (NC) membrane, adsorption pad as well as backing card. The AuNPs were synthesized using sodium citrate tannin reduction, labeled by anti-digoxin monoclonal antibody (mAb) and sprayed onto conjugate pads. We prepared the three test lines (T-lines) using anti-biotin mAb (0.65 mg/ml, for detection of mcr-1 in T1), anti-Cy5 mAb (0.3 mg/ml, for detection of blaNDM-1 in T2) and anti-TAMRA mAb (0.3 mg/ml, for detection of tet(X4) in T3). Immobilization of an anti-mouse polyclonal secondary antibody (pAb, 2.0 mg/ml) on the control line (C-line) was completed. The immobilized NC membrane received 12 h of drying treatment at 37°C and a cutter was used to cut it into 2.5 mm wide strips. We stored the assembled LFD test strips in a vacuum bag at room temperature before use. We diluted the RPA amplification products 50 times (mixing 2 μl product with 98 μl running buffer containing phosphate buffered saline and 3% Tween20) and pipetted them onto the LFD strips. The labeled RPA products were then migrated by capillary action. A test strip reader assisted in scanning as well as calculating the T1–T3 lines and C-line intensities within 5 min.
Single RPA assays were performed to test the validity of the primer/probe sets. The reaction components and conditions were set following the operating manual regarding the TwistAmp nfo kits (TwistDX) with some modifications. Each reaction had 29.5 μl rehydration buffer to dissolve the freeze-dried enzyme pellet, 2.1 μl of each reverse and forward primer (10 μM), 0.6 μl LF probes (10 μM), 2.5 μl magnesium-acetate (280 mM), 2 μl DNA template and 11.2 μl nuclease-free water. The tube was mixed by inversion and brief centrifugation, and the single RPA amplification reaction proceeded for 20 min at 37°C. The RPA amplification products were diluted 50 times and pipetted onto LFD strips. The T1–T3 lines and C-line intensities were analyzed within 5 min.
Based on the single RPA assay, concentrations of the primer/probe sets, reaction temperature, incubation time as well as magnesium-acetate concentration were optimized for multiplex RPA assays to simultaneously amplify mcr-1, blaNDM-1 and tet(X4) genes. First, the primer/probe concentrations were evaluated in the following ratios: (1) high primer/probe concentrations (mcr-1 0.42 μM/0.12 μM, blaNDM-1 0.42 μM/0.12 μM and tet(X4) 0.42 μM/0.12 μM); (2) medium primer/probe concentrations (mcr-1 0.30 μM/0.09 μM, blaNDM-1 0.30 μM/0.09 μM and tet(X4) 0.30 μM/0.09 μM; mcr-1 0.42 μM/0.12 μM, blaNDM-1 0.30 μM/0.09 μM and tet(X4) 0.15 μM/0.06 μM; mcr-1 0.30 μM/0.09 μM, blaNDM-1 0.42 μM/0.12 μM and tet(X4) 0.15 μM/0.06 μM; mcr-1 0.15 μM/0.06 μM, blaNDM-1 0.30 μM/0.09 μM and tet(X4) 0.42 μM/0.12 μM; mcr-1 0.30 μM/0.09 μM, blaNDM-1 0.42 μM/0.12 μM and tet(X4) 0.30 μM/0.09 μM); and (3) low primer/probe concentrations (mcr-1 0.15 μM/0.06 μM, blaNDM-1 0.15 μM/0.06 μM and tet(X4) 0.15 μM/0.06 μM).
Second, multiplex RPA reactions were performed at a 30–50°C temperature range (30, 35, 37, 39, 45 and 50°C). Finally, we assessed the optimal incubation time from 2.5 to 30 min.
For specificity analysis regarding the multiplex RPA-LFD assay, DNA from the recombinant E. coli strains containing mcr-1, blaNDM-1 or tet(X4) and E. coli ATCC25922 (quality control reference strain in all antimicrobial susceptibility tests) was extracted and added to the optimized reaction system.
For sensitivity analysis, the three standard recombinant plasmids for the mcr-1, blaNDM-1 and tet(X4) genes were chosen as the standard DNA templates. Concentrations of the standard DNA were determined using a BioDrop μLITE (BioDrop, Cambridge, United Kingdom). Below is the calculation formula of the copy number:
where X is the concentration of the standard DNA (g/μl) measured at 260 nm wavelength and a is the number of base pairs of the standard DNA molecule (bp).
The diluted standard DNA was prepared by serial 10-fold dilution in the range of 100 copies/μl to 107 copies/μl. Serially diluted plasmids containing the mcr-1, blaNDM-1 and tet(X4) genes served as the DNA templates, respectively, for sensitivity analysis of the multiplex RPA-LFD assay.
Quantitative analysis of the mcr-1, blaNDM-1 and tet(X4) genes in Enterobacteriaceae was also performed. The TSR-200 test strip reader (Allsheng Instruments Co. Ltd.) assisted in reading the RPA-LFD assay test results. Standard curves were established for the mcr-1, blaNDM-1 and tet(X4) genes in Enterobacteriaceae strains considering the T/C value of the LFD strips and the logarithm of the copy number of the corresponding target resistance gene.
Enterobacteriaceae strains for the application of multiplex RPA-LFD assays are listed in Supplementary Table S1. All isolates were confirmed to carry mcr-1, blaNDM-1 and/or tet(X4) genes by sequencing. The triplex RPA-LFD assays were compared with conventional PCR methods as described previously (Xu et al., 2018; Ji et al., 2020; Ayfan et al., 2021).
We scanned the intensities exhibited by the T-line and C-line, and calculated the T/C value using a TSR-200 test strip reader (Allsheng Instruments Co. Ltd.). We carried out all experiments in triplicate, and the results are in the form of mean ± standard deviation. SPSS 20.0 (IBM Corp., Armonk, NY, United States) together with Origin 2021 (OriginLab, Northampton, MA, United States) served for the statistical analysis.
Here, a fast boil-up method for DNA extraction from Enterobacteriaceae strains was applied accrding to previous studies with some modification (Bordin et al., 2019; Liu et al., 2022). Compared with the commercial DNA extraction kits (taking around 30 min or more, Dimitrakopoulou et al., 2020), our method significantly reduces extraction time (taking around 10 min for the entire extraction process; Figure 1A).
Figure 1. RPA-LFD assay strategy. (A) DNA extraction of Enterobacteriaceae strains; (B) RPA amplification; (C) LFD lateral flow; (D) quantitative analysis.
A TwistAmp nfo kit served for conducting the triplex RPA amplification to generate three target amplicons. Various chemical groups were labeled for designing primers and LF probes compatible with the TwistAmp nfo technology. The resulting double-stranded DNA amplicons for the mcr-1 target were digoxin- and biotin-labeled, the amplicons for the blaNDM-1 target were digoxin- and Cy5-labeled and the amplicons for the tet(X4) target were digoxin- and TAMRA-labeled. These labeled double-stranded DNA amplicons could be detected by the LFD assay (Figure 1B).
On the LFD test strip, when amplicons moved on the conjugated pad, the digoxin-labeled amplicons combined with AuNP-labeled anti-digoxin mAb. With further migration, the biotin-, Cy5- and TAMRA-labeled amplicons combined with the AuNP-labeled anti-digoxin mAb were captured by a corresponding mAb coated on the T-lines. The uncaptured AuNP-labeled anti-digoxin mAb continued moving and was captured by the pAb coated on the C-line (Figure 1C). Using the test strip reader, quantitative analysis was then achieved. First, the test strip reader served for scanning the intensities exhibited by T-line and C-line, and T and C values were calculated. The T/C value was positively correlated with the concentration of target DNA. Standard curves were plotted considering the T/C value and the copy number logarithm of target DNA (Figure 1D).
Triplex RPA amplification conditions for simultaneous detection of mcr-1, blaNDM-1 and tet(X4) using the TwistAmp nfo kit were optimized. For initial optimization, the concentration of the primer/probe set was optimized for the RPA reaction. As shown in Figure 2A, the results of RPA-LFD assays indicated that a medium concentration of primer/probe (mcr-1 0.30 μM/0.06 μM, blaNDM-1 0.42 μM/0.12 μM and tet(X4) 0.30 μM/0.09 μM) in a 50 μl reaction volume gave results similar to a high concentration of primer/probe (mcr-1 0.42 μM/0.12 μM, blaNDM-1 0.42 μM/0.12 μM and tet(X4) 0.42 μM/0.12 μM).
Figure 2. Optimization of triplex RPA amplification conditions. (A) Concentration of primer/LF probe; (B) reaction temperature (1–6: 30, 35, 37, 39, 45, 50°C); (C) concentration of magnesium-acetate (1–7: 0, 2.8, 5.6, 8.4, 11.2, 14, 16.8 mM); (D) reaction time (1–10: 0, 2.5, 5, 7.5, 10, 12.5, 15, 20, 25, 30 min).
The RPA assay was run at incubation temperatures ranging from 30 to 50°C, and amplicons were run on LFDs to identify the optimal amplification temperature. As shown in Figure 2B, the results of RPA-LFD assays indicated that 37°C was confirmed as the optimal temperature.
Using the optimal concentrations of primer/probe sets and the optimal reaction temperature, the concentration of magnesium-acetate was tested from 0 to 16.8 mM. The results showed that the optimal concentration of magnesium-acetate was 14 mM (Figure 2C).
Finally, the RPA reaction time was validated. As shown in Figure 2D, all T1–T3 lines were visible at or after 5 min. The T/C values of T1–T3 were not significantly different at 20 min compared with 25 or 30 min. Thus, to shorten the detection time, a reaction time of 20 min was deemed sufficient.
Standard recombinant E. coli strains carrying mcr-1, blaNDM-1 or tet(X4) and E. coli ATCC25922, which does not harbor mcr-1, blaNDM-1 or tet(X4), served for evaluating the specificity exhibited by the triplex RPA-LFD assay. Based on specificity analyses results, the triplex RPA-LFD assay performed equally well for specific samples containing the target resistance genes (Figure 3).
Figure 3. Specificity analysis of the triplex RPA-LFD assay. 1: Top10-pUC-mcr-1 + Top10-pUC-blaNDM-1 + Top10-pUC-tet(X4); 2: Top10-pUC-mcr-1 + Top10-pUC-blaNDM-1; 3: Top10-pUC-mcr-1 + Top10-pUC-tet(X4); 4: Top10-pUC-blaNDM-1 + Top10-pUC-tet(X4); 5: Top10-pUC-mcr-1; 6: Top10-pUC-blaNDM-1; 7: Top10-pUC-tet(X4); 8: ATCC25922; 9: negative control.
The study focused on preparing the 10-fold serial dilutions of standard plasmid solutions for assessing the sensitivity exhibited by the triplex RPA-LFD assays. The concentration of the plasmids was measured, and the copy numbers of the plasmids were calculated. As shown in Figure 4A, when the concentration of the target gene was ≥101 copies/μl, the T-line was visible and T values of the T1, T2, and T3 lines were measurable with the test strip reader. Hence, the detection limit of the RPA-LFD assay for all three target resistance genes was 101 copies/μl.
Figure 4. Sensitivity analysis of the triplex RPA-LFD assay. (A) Results of RPA-LFD assays. Blank means only the running buffer was used. (B) Standard curves. The standard linear equation and correlation coefficient (R2) between the T/C value and the logarithm of the copy number of target DNA (copies/μl).
The T/C value was positively correlated with the concentration of target DNA. Standard curves for the mcr-1, blaNDM-1 and tet(X4) genes in Enterobacteriaceae strains were established according to the T/C values of the LFD strips and the logarithm of the copy number of the corresponding target resistance gene. As shown in Figure 4B, the results indicated that there were significant linear correlations between the T/C values and the copy number logarithm of the target DNA. The standard linear equation and correlation coefficients (R2) of the mcr-1, blaNDM-1 and tet(X4) genes were y = 0.1215x − 0.0752 (R2 = 0.9881), y = 0.1139x − 0.081 (R2 = 0.9745) and y = 0.1187x + 0.051 (R2 = 0.9807), respectively.
The triplex RPA-LFD assay together with conventional PCR methods assisted in analyzing 19 different Enterobacteriaceae strains. As shown in Table 2, the two methods also served for detecting six mcr-1 positive strains, three blaNDM-1 positive strains, six tet(X4) positive strains and four mcr-1 and blaNDM-1 positive strains. The results for the target resistance genes were consistent for the two methods. The triplex RPA-LFD assay, including sample pretreatment, RPA amplification and LFD lateral flow, could be achieved within 40 min. In contrast, the PCR method, including sample pretreatment, PCR amplification and agarose gel electrophoresis, required at least 3 h. Thus, the triplex RPA-LFD assay consumes less time.
Colistin, carbapenems and tigecycline are considered last-resort antibiotics for defense against MDR bacterial infections (Tang et al., 2021). However, these antibiotics become less effective because their respective predominant resistance genes, mcr, blaNDM and tet(X), emerge and spread in animals, food and humans (Singh et al., 2018; Zhong et al., 2019; Anyanwu et al., 2022; Lu et al., 2022; Zhang et al., 2022). Among these last-resort antibiotics, colistin was heavily used in food-producing animal industries in China for a long period of time until it was banned as a growth promoter in 2017 (Walsh and Wu, 2016). However, colistin-resistant Gram-negative pathogens, especially Enterobacteriaceae pathogens, still have high presence in poultry/livestock farm environments (Liu et al., 2016; Tang et al., 2022), and the plasmid-borne resistance gene mcr-1 is the main factor contributing to widespread colistin resistance (Arcilla et al., 2016). In addition, although the use of carbapenems, broad-spectrum β-lactam class antibiotics, is authorized only in clinics (Ahmad et al., 2019; Farhat and Khan, 2020; Nasser et al., 2020), their main resistance gene, blaNDM-1, is still frequently identified in Enterobacteriaceae pathogens in poultry/livestock farm environments. This is because of the extensive application of all β-lactam antibiotics (except carbapenems) in the prophylaxis and growth promotion in food-producing animal industries over the last decade in China and have provided long-term selective pressure for the blaNDM-1 gene (Shen et al., 2016; Shi et al., 2021). Similarly, although the animal husbandry field has never adopted tigecycline, a third-generation tetracycline, excessively using of first- and second-generation tetracyclines may have provided a selective pressure for the tet(X4) gene in poultry/livestock farm environments (Tang et al., 2021). Of note, a growing number of reports indicate that tet(X4) is often detected together with blaNDM-1 genes or mcr-1 genes (Bai et al., 2019; Sun C. et al., 2019). Additionally, tet(X4)-positive strains also harboring both mcr-1 and blaNDM genes have been found in food animal samples (Sun J. et al., 2019).
MDR Enterobacteriaceae pathogens in poultry/livestock farm environments are considered the most common reservoirs of the mcr-1, blaNDM-1 and tet(X4) genes (Xiong et al., 2018; Xia et al., 2019; Shi et al., 2021). Besides, such ARGs may be spread along the food chain in poultry/livestock farm environments and meat production facilities, and finally be applied in community and even hospital environments (Wang et al., 2017; Shi et al., 2021). As resistance genes to last-resort antibiotics potentially threaten the food safety as well as public health, predominant ARGs such as mcr-1, blaNDM-1 and tet(X4) in their common Enterobacteriaceae pathogen hosts should be more closely monitored along the food chain worldwide over the long-term (Shi et al., 2021). Therefore, it is in urgent need to develop a rapid, and accurate detection method to simultaneously screen and monitor multiple resistance genes to last-resort antibiotics in the field more effectively.
Multiple nucleic acid detection methods, including multiplex PCR, multiplex loop-mediated isothermal amplification (LAMP) and multiplex RPA assays, can achieve rapid, accurate and simultaneous amplification of multiple resistance genes to last-resort antibiotics in a single reaction. However, multiplex PCR assays require thermal cycling steps and specialized instruments, giving them limited application in the field (Ma et al., 2020). Similarly, multiplex LAMP assays require more target-specific primers (normally four to six) for amplification under isothermal conditions between 60 and 65°C in 40 to 60 min (Fang et al., 2018). Compared with these two methods, multiplex RPA assays require fewer target-specific primers (two primers or two primers with one probe) for amplification, lower incubation temperatures (25–43°C) and shorter incubation times (less than 30 min) and have a high tolerance to sample impurities (Lillis et al., 2014; Ma et al., 2020). Thus, in our study, a fast, innovative boil-up method for crude DNA extraction (taking around 10 min for the entire extraction process) from Enterobacteriaceae pathogens was used to increase the time savings of the multiplex RPA procedure. Studies have confirmed the application of multiplex RPA assays for simultaneously detecting multiple foodborne pathogens (Ma et al., 2020; Jin et al., 2022). However, studies on its detection for ARG remain less. Our study focused on explaining certain and quantitative multiplex RPA-LFD method to simultaneously identify multiple resistance genes to last-resort antibiotics in certain Enterobacteriaceae pathogens when the temperature remained unchanged and no specialized instruments were used, which has great potential for applications in the field.
For multiplex RPA assays for ARG detection, primer/probe concentration, incubation temperature and time and magnesium-acetate concentration are the most vital extrinsic factors contributing to the assay’s maximum efficiency (Hu et al., 2019; Ma et al., 2020). Our study first adjusted the primer/probe concentration to 0.30 μM/0.06 μM for the mcr-1 gene, 0.42 μM/0.12 μM for the blaNDM-1 gene and 0.30 μM/0.09 μM for the tet(X4) gene with 14 mM magnesium-acetate for obtaining an equivalent amplification for three ARGs. Second, we set the optimal time at 20 min and the temperature conditions at 37°C. This reaction time is significantly faster than that of multiplex PCR (around 4 h) and multiplex LAMP (40–60 min) assays. Furthermore, it is allowed to complete the RPA reaction in a heating block or water bath, even at ambient temperature or body temperature (Lillis et al., 2014; Wang et al., 2017).
Experiments confirm LFD as a faster and simpler tool for detecting RPA product, featuring an antibody labeled with antigen-specific gold nanoparticles, thereby ensuring that it is not needed to clean up nucleotide. The resulting visualization can be observed within 5 min (Wu et al., 2017). To achieve triplex detection in our study, we labeled each of the LF probes with biotin, Cy5 and TAMRA at the 5′ end, with a C3 spacer at the 3′ end and modified inside the LF probe with THF. We labeled all the reverse primers with digoxin at the 5′ end. We finally obtained 3 detectable double-labeled amplicons. Besides, the preparation of LFDs with three test lines served for RPA amplicon visualization (Ma et al., 2020). In general, LFD is a qualitative or semi-quantitative detection method, but the current test strip reader, which combines facile colorimetric readouts with test strips, can realize quantitative detection (Wang et al., 2022). In our study, sensitivity analyses showed a close association of color signals with template concentrations of mcr-1, blaNDM-1 and tet(X4) genes in Enterobacteriaceae pathogens (R2 = 0.9881, R2 = 0.9745, and R2 = 0.9807, respectively). Use of the test strip reader also eliminates errors associated with assessment by eye.
Sensitivity and specificity analyses verified that RPA-LFD assays were practical for multiple resistance genes to last-resort antibiotics detection in Enterobacteriaceae pathogens. In our study, this assay simultaneously detected as few as 101 copies/μl of mcr-1, blaNDM-1 and tet(X4) genes in Enterobacteriaceae pathogens, with a sensitivity 10-fold higher than that of PCR (102 copies/μl). Furthermore, such ARGs were specifically detected in different Enterobacteriaceae pathogens. These results showed that RPA-LFD is a suitable method for detecting multiple resistance genes to last-resort antibiotics in foodborne pathogens and has potential applications in the field.
In conclusion, the multiplex RPA-LFD method is fast, sensitive, specific and user-friendly for direct detection of the mcr-1, blaNDM-1 as well as tet(X4) genes of Enterobacteriaceae pathogens and does not require expensive equipment. It can provide immediate monitoring results of multiple resistance genes to last-resort antibiotics in foodborne pathogens in the field in a timely manner. On this basis, we will further study the pretreatment technology of the complex sample, and establish the RPA-LFD method for direct analysis of ARGs in water, soil and feces and other environmental samples.
The original contributions presented in the study are included in the article/Supplementary material, further inquiries can be directed to the corresponding authors.
CL took charge of original draft writing, data curation, and review and editing. JW was responsible for original draft writing, data curation, and software. XG, WL, DC, CZ, QY, CX, and BT together took charge of data curation and software. LP, YT, and PL acquired the funding. GH also participated in review and editing. JF and HJ participated in funding acquisition, methodology, supervision, and review and editing. All authors contributed to the article and approved the submitted version.
This research was completed with the support of the Public Welfare Technology Application Research Project of Zhejiang Province (No. LGN22C200013), the Public Welfare Technology Application Research Project of Ningbo (No. 2022S014), the Major Science and Technology Project of Xiaoshan District (No. 2021226), the Open Fund of the Key Laboratory of Biosafety detection for Zhejiang Market Regulation (No. 2022BS002), Science and Technology Innovation Activity Plan for College Students in Zhejiang Province (No. 2022R409A057 and 2022R409A050), the National Science Foundation of China (Nos. 31901792 and 31801655), and the National Program on Key Research Project of China (No. 2019YFE0103900).
LP was employed by Zhejiang Hongzheng Testing Co., Ltd, Ningbo, Zhejiang, China. XG was employed by Zhejiang Gongzheng Testing Center Co., Ltd, Hangzhou, Zhejiang, China. YT was employed by Hangzhou Tiannie Technology Co., Ltd, Hangzhou, Zhejiang, China.
The remaining authors declare that the research was conducted in the absence of any commercial or financial relationships that could be construed as a potential conflict of interest.
All claims expressed in this article are solely those of the authors and do not necessarily represent those of their affiliated organizations, or those of the publisher, the editors and the reviewers. Any product that may be evaluated in this article, or claim that may be made by its manufacturer, is not guaranteed or endorsed by the publisher.
The Supplementary material for this article can be found online at: https://www.frontiersin.org/articles/10.3389/fmicb.2022.1062577/full#supplementary-material
Adekunle, C., Mustapha, A., Odewale, G., and Richard, O. (2021). Detection of antibiotic resistance genes among multiple drug resistant Pseudomonas aeruginosa isolated from clinical sources in selected health institutions in Kwara state. Infect. Disord. Drug Target. 21, 53–59. doi: 10.2174/1871526520666201116103625
Ahmad, N., Ali, S. M., and Khan, A. U. (2019). Molecular characterization of novel sequence type of carbapenem-resistant New Delhi metallo-β-lactamase-1-producing Klebsiella pneumoniae in the neonatal intensive care unit of an Indian hospital. Int. J. Antimicrob. Agents 53, 525–529. doi: 10.1016/j.ijantimicag.2018.12.005
Anyanwu, M. U., Anyogu, D. C., Chah, K. F., and Shoyinka, V. S. (2022). Mobile colistin resistance (mcr-1) gene-positive Escherichia coli from chickens in Nigeria is potentially pathogenic and transfers colistin resistance to other organisms. Comp. Clin. Pathol. 31, 323–332. doi: 10.1007/s00580-022-03336-2
Arcilla, M. S., van Hattem, J. M., Matamoros, S., Melles, D. C., Penders, J., de Jong, M. D., et al. (2016). Dissemination of the mcr-1 colistin resistance gene. Lancet Infect. Dis. 16, 147–149. doi: 10.1016/S1473-3099(15)00541-1
Ayfan, A. K., Macdonald, J., Harris, P. N., Heney, C., Paterson, D. L., Trembizki, E., et al. (2021). Rapid detection of NDM and VIM carbapenemase encoding genes by recombinase polymerase amplification and lateral flow-based detection. Eur. J. Clin. Microbiol. Infect. Dis. 40, 2447–2453. doi: 10.1007/s10096-021-04267-6
Bai, L., Du, P., Du, Y., Sun, H., Zhang, P., Wan, Y., et al. (2019). Detection of plasmid-mediated tigecycline-resistant gene tet(X4) in Escherichia coli from pork, Sichuan and Shandong Provinces, China, February 2019. Eur. Secur. 24:1900340. doi: 10.2807/1560-7917.ES.2019.24.25.1900340
Bordin, A., Trembizki, E., Windsor, M., Wee, R., Tan, L. Y., Buckley, C., et al. (2019). Evaluation of the SpeeDx Carba (beta) multiplex real-time PCR assay for detection of NDM, KPC, OXA-48-like, IMP-4-like and VIM carbapenemase genes. BMC Infect. Dis. 19, 1–7. doi: 10.1186/s12879-019-4176-z
Cao, Y., Zheng, K., Jiang, J., Wu, J., Shi, F., Song, X., et al. (2018). A novel method to detect meat adulteration by recombinase polymerase amplification and SYBR green I. Food Chem. 266, 73–78. doi: 10.1016/j.foodchem.2018.05.115
Cavalera, S., Colitti, B., Rosati, S., Ferrara, G., Bertolotti, L., Nogarol, C., et al. (2021). A multi-target lateral flow immunoassay enabling the specific and sensitive detection of total antibodies to SARS-CoV-2. Talanta 223:121737. doi: 10.1016/j.talanta.2020.121737
Chen, C., Cui, C. Y., Zhang, Y., He, Q., Wu, X. T., Li, G., et al. (2019). Emergence of mobile tigecycline resistance mechanism in Escherichia coli strains from migratory birds in China. Emerg. Microbes Infect. 8, 1219–1222. doi: 10.1080/22221751.2019.1653795
Cheng, H., Jiang, H., Fang, J., and Zhu, C. (2019). Antibiotic resistance and characteristics of integrons in Escherichia coli isolated from Penaeus vannamei at a freshwater shrimp farm in Zhejiang Province, China. J. Food Prot. 82, 470–478. doi: 10.4315/0362-028X.JFP-18-444
Cooper, A. L., Carter, C., Mcleod, H., Wright, M., and Blais, B. W. (2021). Detection of carbapenem-resistance genes in bacteria isolated from wastewater in Ontario. FACETS 6, 569–591. doi: 10.1139/facets-2020-0101
Di Nardo, F., Chiarello, M., Cavalera, S., Baggiani, C., and Ten Anfossi, L. (2021). Years of lateral flow immunoassay technique applications: trends, challenges and future perspectives. Sensors 21:5185. doi: 10.3390/s21155185
Dimitrakopoulou, M. E., Stavrou, V., Kotsalou, C., and Vantarakis, A. (2020). Boiling extraction method VS commercial kits for bacterial DNA isolation from food samples. J. Food Sci. Nutr. Res. 3, 311–319. doi: 10.26502/jfsnr.2642-11000057
Fang, J., Wu, Y., Qu, D., Ma, B., Yu, X., Zhang, M., et al. (2018). Propidium monoazide real-time loop-mediated isothermal amplification for specific visualization of viable Salmonella in food. Lett. Appl. Microbiol. 67, 79–88. doi: 10.1111/lam.12992
Farhat, N., and Khan, A. U. (2020). Evolving trends of New Delhi Metallo-betalactamse (NDM) variants: a threat to antimicrobial resistance. Infect. Genet. Evol. 86:104588. doi: 10.1016/j.meegid.2020.104588
Fu, X., Sun, J., Ye, Y., Zhang, Y., and Sun, X. (2022). A rapid and ultrasensitive dual detection platform based on Cas12a for simultaneous detection of virulence and resistance genes of drug-resistant Salmonella. Biosens. Bioelectron. 195:113682. doi: 10.1016/j.bios.2021.113682
Hu, J., Huang, R., Sun, Y., Wei, X., Wang, Y., Jiang, C., et al. (2019). Sensitive and rapid visual detection of Salmonella typhimurium in milk based on recombinase polymerase amplification with lateral flow dipsticks. J. Microbiol. Methods 158, 25–32. doi: 10.1016/j.mimet.2019.01.018
Ji, K., Xu, Y., Sun, J., Huang, M., Jia, X., Jiang, C., et al. (2020). Harnessing efficient multiplex PCR methods to detect the expanding Tet (X) family of tigecycline resistance genes. Virulence 11, 49–56. doi: 10.1080/21505594.2019.1706913
Jin, B., Ma, B., Li, J., Hong, Y., and Zhang, M. (2022). Simultaneous detection of five foodborne pathogens using a mini automatic nucleic acid extractor combined with recombinase polymerase amplification and lateral flow immunoassay. Microorganisms 10:1352. doi: 10.3390/microorganisms10071352
Kersting, S., Rausch, V., Bier, F. F., and Nickisch-Rosenegk, M. (2014). Multiplex isothermal solid-phase recombinase polymerase amplification for the specific and fast DNA-based detection of three bacterial pathogens. Microchim. Acta 181, 1715–1723. doi: 10.1007/s00604-014-1198-5
Kim, J. Y., and Lee, J. L. (2016). Rapid detection of Salmonella Enterica serovar enteritidis from eggs and chicken meat by real-time recombinase polymerase amplification in comparison with the two-step real-time PCR. J. Food Saf. 36, 402–411. doi: 10.1111/jfs.12261
Leshaba, T. M. S., Mbelle, N. M., and Osei Sekyere, J. (2022). Current and emerging polymyxin resistance diagnostics: a systematic review of established and novel detection methods. J. Appl. Microbiol. 132, 8–30. doi: 10.1111/jam.15184
Li, J. L., Ma, B., Fang, J. H., Zhi, A. T., Chen, E. J., Xu, Y., et al. (2019). Recombinase polymerase amplification (RPA) combined with lateral flow immunoassay for rapid detection of salmonella in food. Foods 9:27. doi: 10.3390/foods9010027
Lillis, L., Lehman, D., Singhal, M. C., Cantera, J., Singleton, J., Labarre, P., et al. (2014). Non-instrumented incubation of a recombinase polymerase amplification assay for the rapid and sensitive detection of proviral HIV-1 DNA. PLoS One 9:e108189. doi: 10.1371/journal.pone.0108189
Lin, H., Zhao, S., Liu, Y., Shao, L., Ye, Y., Jiang, N., et al. (2022). Rapid visual detection of plasmodium using recombinase-aided amplification with lateral flow dipstick assay. Front. Cell. Infect. Microbiol. 12:922146. doi: 10.3389/fcimb.2022.922146
Liu, Y., Chen, J., Cheng, Y., Li, Y., Li, X., Zhang, Z., et al. (2022). A simple and rapid technique of template preparation for PCR. Front. Microbiol. 13:1024827. doi: 10.3389/fmicb.2022.1024827
Liu, Y. Y., Wang, Y., Walsh, T. R., Yi, L. X., Zhang, R., Spencer, J., et al. (2016). Emergence of plasmid-mediated colistin resistance mechanism MCR-1 in animals and human beings in China: a microbiological and molecular biological study. Lancet Infect. Dis. 16, 161–168. doi: 10.1016/S1473-3099(15)00424-7
Lobato, I. M., and O'Sullivan, C. K. (2018). Recombinase polymerase amplification: basics, applications and recent advances. TrAC Trends Anal. Chem. 98, 19–35. doi: 10.1016/j.trac.2017.10.015
Lu, X., Du, Y., Peng, K., Zhang, W., Li, J., Wang, Z., et al. (2022). Coexistence of tet(X4), mcr-1, and blaNDM-5 in ST6775 Escherichia coli isolates of animal origin in China. Microbiol. Spectr. 10:e0019622. doi: 10.1128/spectrum.00196-22
Ma, B., Li, J., Chen, K., Yu, X., Sun, C., and Zhang, M. (2020). Multiplex recombinase polymerase amplification assay for the simultaneous detection of three foodborne pathogens in seafood. Foods 9:278. doi: 10.3390/foods9030278
Merino, I., de la Fuente, A., Domínguez-Gil, M., Eiros, J. M., Tedim, A. P., and Bermejo-Martín, J. F. (2022). Digital PCR applications for the diagnosis and management of infection in critical care medicine. Crit. Care 26, 1–10. doi: 10.1186/s13054-022-03948-8
Nasser, M., Palwe, S., Bhargava, R. N., Feuilloley, M. G. J., and Kharat, A. S. (2020). Retrospective analysis on antimicrobial resistance trends and prevalence of β-lactamases in Escherichia coli and ESKAPE pathogens isolated from arabian patients during 2000-2020. Microorganisms 8:1626. doi: 10.3390/microorganisms8101626
Park, S., Rana, A., Sung, W., and Munir, M. (2021). Competitiveness of quantitative polymerase chain reaction (qPCR) and droplet digital polymerase chain reaction (ddPCR) technologies, with a particular focus on detection of antibiotic resistance genes (ARGs). Appl. Microbiol. 1, 426–444. doi: 10.3390/applmicrobiol1030028
Piepenburg, O., Williams, C. H., Stemple, D. L., and Armes, N. A. (2006). DNA detection using recombination proteins. PLoS Biol. 4:e204. doi: 10.1371/journal.pbio.0040204
Shen, Z., Wang, Y., Shen, Y., Shen, J., and Wu, C. (2016). Early emergence of mcr-1 in Escherichia coli from food-producing animals. Lancet Infect. Dis. 16:293. doi: 10.1016/S1473-3099(16)00061-X
Shi, X., Li, Y., Yang, Y., Shen, Z., Cai, C., Wang, Y., et al. (2021). High prevalence and persistence of carbapenem and colistin resistance in livestock farm environments in China. J. Hazard. Mater. 406:124298. doi: 10.1016/j.jhazmat.2020.124298
Singh, S., Pathak, A., Kumar, A., Rahman, M., Singh, A., Gonzalez-Zorn, B., et al. (2018). Emergence of chromosome-borne colistin resistance gene mcr-1 in clinical isolates of Klebsiella pneumoniae from India. Antimicrob. Agents Chemother. 62:e01885-17. doi: 10.1128/AAC.01885-17
Sun, J., Chen, C., Cui, C. Y., Zhang, Y., Liu, X., Cui, Z. H., et al. (2019). Plasmid-encoded tet(X) genes that confer high-level tigecycline resistance in Escherichia coli. Nat. Microbiol. 4, 1457–1464. doi: 10.1038/s41564-019-0496-4
Sun, C., Cui, M., Zhang, S., Wang, H., Song, L., Zhang, C., et al. (2019). Plasmid-mediated tigecycline-resistant gene tet(X4) in Escherichia coli from food-producing animals, China, 2008-2018. Emerg. Microbes Infect. 8, 1524–1527. doi: 10.1080/22221751.2019.1678367
Tang, B., Chang, J., Chen, Y., Lin, J., Xiao, X., Xia, X., et al. (2022). Escherichia fergusonii, an underrated repository for antimicrobial resistance in food animals. Microbiol. Spectr. 10, e01617–e01621. doi: 10.1128/spectrum.01617-21
Tang, B., Yang, H., Jia, X., and Feng, Y. (2021). Coexistence and characterization of Tet (X5) and NDM-3 in the MDR-Acinetobacter indicus of duck origin. Microb. Pathog. 150:104697. doi: 10.1016/j.micpath.2020.104697
Tolosi, R., Carraro, L., Laconi, A., and Piccirillo, A. (2021). Optimization of five qPCR protocols toward the detection and the quantification of antimicrobial resistance genes in environmental samples. MethodsX 8:101488. doi: 10.1016/j.mex.2021.101488
Tran, D. H., Tran, H. T., Pham, T. N. M., and Phung, H. T. T. (2022). Direct multiplex recombinase polymerase amplification for rapid detection of Staphylococcus aureus and Pseudomonas aeruginosa in food. Mol. Biol. Res. Commun. 11, 1–10. doi: 10.22099/mbrc.2021.41503.1664
Walsh, T. R., and Wu, Y. (2016). China bans colistin as a feed additive for animals. Lancet Infect. Dis. 16, 1102–1103. doi: 10.1016/S1473-3099(16)30329-2
Wang, P., Ma, C., Liao, L., Yu, J., Yi, L., Qiao, Y., et al. (2021). Simultaneous visual diagnosis of acute hepatopancreatic necrosis disease and Enterocytozoon hepatopenaei infection in shrimp with duplex recombinase polymerase amplification. J. Fish Dis. 44, 1753–1763. doi: 10.1111/jfd.13492
Wang, Y., Wang, T., Wang, M. L., Wang, J., Xu, Z. X., and Zhang, H. Y. (2022). Photothermal card reader assay using the commercial colloidal gold test strip for the rapid quantitative detection of food hazards. Mikrochim. Acta 189, 1–11. doi: 10.1007/s00604-022-05193-w
Wang, Y., Zhang, R., Li, J., Wu, Z., Yin, W., Schwarz, S., et al. (2017). Comprehensive resistome analysis reveals the prevalence of NDM and MCR-1 in Chinese poultry production. Nat. Microbiol. 2, 1–7. doi: 10.1038/nmicrobiol.2016.260
Wong, Y. P., Othman, S., Lau, Y. L., Radu, S., and Chee, H. Y. (2018). Loop-mediated isothermal amplification (LAMP): a versatile technique for detection of micro-organisms. J. Appl. Microbiol. 124, 626–643. doi: 10.1111/jam.13647
World Health Organization. (2022). Antibiotic resistance. Available at: https://www.who.int/news-room/fact-sheets/detail/antibiotic-resistance (Accessed October 1, 2022).
Wu, Y. D., Xu, M. J., Wang, Q. Q., Zhou, C. X., Wang, M., Zhu, X. Q., et al. (2017). Recombinase polymerase amplification (RPA) combined with lateral flow (LF) strip for detection of toxoplasma gondii in the environment. Vet. Parasitol. 243, 199–203. doi: 10.1016/j.vetpar.2017.06.026
Xia, X., Wang, Z., Fu, Y., Du, X. D., Gao, B., Zhou, Y., et al. (2019). Association of colistin residues and manure treatment with the abundance of mcr-1 gene in swine feedlots. Environ. Int. 127, 361–370. doi: 10.1016/j.envint.2019.03.061
Xiong, W., Wang, Y., Sun, Y., Ma, L., Zeng, Q., Jiang, X., et al. (2018). Antibiotic-mediated changes in the fecal microbiome of broiler chickens define the incidence of antibiotic resistance genes. Microbiome 6, 1–11. doi: 10.1186/s40168-018-0419-2
Xu, J., Wang, X., Yang, L., Kan, B., and Lu, X. (2018). Rapid detection of mcr-1 by recombinase polymerase amplification. J. Med. Microbiol. 67, 1682–1688. doi: 10.1099/jmm.0.000865
Xu, J., Zhang, N., Luo, M., Wang, M., Wang, L., Li, J., et al. (2021). Rapid identification of plasmid replicon type and coexisting plasmid-borne antimicrobial resistance genes by S1-pulsed-field gel electrophoresis-droplet digital polymerase chain reaction. Foodborne Pathog. Dis. 18, 298–305. doi: 10.1089/fpd.2020.2865
Zhang, Z., Zhan, Z., and Shi, C. (2022). International spread of Tet(X4)-producing Escherichia coli isolates. Foods 11:2010. doi: 10.3390/foods11142010
Keywords: recombinase polymerase amplification, lateral flow dipstick, Enterobacteriaceae, rapid detection, resistance genes to last-resort antibiotics
Citation: Lu C, Wang J, Pan L, Gu X, Lu W, Chen D, Zhang C, Ye Q, Xiao C, Liu P, Tang Y, Tang B, Huang G, Fang J and Jiang H (2023) Rapid detection of multiple resistance genes to last-resort antibiotics in Enterobacteriaceae pathogens by recombinase polymerase amplification combined with lateral flow dipstick. Front. Microbiol. 13:1062577. doi: 10.3389/fmicb.2022.1062577
Received: 06 October 2022; Accepted: 12 December 2022;
Published: 05 January 2023.
Edited by:
Helal F. Hetta, Assiut University, EgyptCopyright © 2023 Lu, Wang, Pan, Gu, Lu, Chen, Zhang, Ye, Xiao, Liu, Tang, Tang, Huang, Fang and Jiang. This is an open-access article distributed under the terms of the Creative Commons Attribution License (CC BY). The use, distribution or reproduction in other forums is permitted, provided the original author(s) and the copyright owner(s) are credited and that the original publication in this journal is cited, in accordance with accepted academic practice. No use, distribution or reproduction is permitted which does not comply with these terms.
*Correspondence: Jiehong Fang, ✉ ZmFuZ2poQGNqbHUuZWR1LmNu; Han Jiang, ✉ amlhbmdoYW44MjVAMTI2LmNvbQ==
Disclaimer: All claims expressed in this article are solely those of the authors and do not necessarily represent those of their affiliated organizations, or those of the publisher, the editors and the reviewers. Any product that may be evaluated in this article or claim that may be made by its manufacturer is not guaranteed or endorsed by the publisher.
Research integrity at Frontiers
Learn more about the work of our research integrity team to safeguard the quality of each article we publish.