- Chongqing Key Laboratory of Nutrition and Food Safety, Research Center for Nutrition and Food Safety, Chongqing Medical Nutrition Research Center, Institute of Military Preventive Medicine, Army Medical University (Third Military Medical University), Chongqing, China
Exercise is reported to play a crucial role in skeletal muscle performance. However, the underlying mechanism is still unknown. Thus, we investigated the effect of high-intensity aerobic exercise on skeletal muscle performance. In this study, the male C57BL/6J mice were accepted by high-intensity aerobic exercise for 8 weeks to establish an exercise model. It was observed that high-intensity aerobic exercise markedly affected the expression of genes in skeletal muscle. Moreover, high-intensity aerobic exercise significantly improved skeletal muscle grip strength and serum testosterone levels. HE staining showed that the cross-sectional area (CSA) of the skeletal muscle was successfully increased after 8 weeks of high-intensity aerobic exercise. Additionally, we found that high-intensity aerobic exercise changed gut microbiota structure by altering the abundance of Akkermansia, Allobaculum, and Lactobacillus, which might be related to testosterone production. However, the beneficial effects disappeared after the elimination of the gut microbiota and recovered after fecal microbiota transplantation (FMT) experiments for 1 week. These results indicated that the beneficial effects of high-intensity aerobic exercise on skeletal muscle were partly dependent on the gut microbiota. Our results suggested that long-term high loading intensity of aerobic exercise could improve skeletal muscle performance, which was probably due to the gut microbiota-testosterone axis.
Introduction
Skeletal muscle performance is crucial for athletes. Specifically, evidence suggests that increasing the muscle’s CSA could greatly impact the muscle’s ability (Suchomel et al., 2018). And exercise training could significantly induce skeletal muscle hypertrophy (Bahreinipour et al., 2018; Torma et al., 2021), which are well-known treatments for athletes to improve skeletal muscle performance. Recently we have shown that exercise has been linked to serum testosterone levels. Moderate-intense exercise and resistance training (Vingren et al., 2010) could improve serum testosterone levels (Sharma et al., 2013; Khajehnasiri et al., 2018). However, some studies indicated that aerobic exercise could also induce skeletal muscle hypertrophy (Harber et al., 2009, 2012; Konopka and Harber, 2014), but the underlying mechanism is still unknown.
Testosterone is an anabolic hormone regulated by the hypothalamic–pituitary-testicular axis (HPA; Bélanger et al., 2003; Anderson et al., 2018). Testosterone is produced mainly by the testis, metabolized by the liver, and reabsorbed by the intestinal tract (Sandberg and Slaunwhite, 1956; Baulieu, 1991; Li et al., 2022). Testosterone plays a crucial role in satellite cell activation, conversion of fiber, and synthesis of protein (Leproult and Van Cauter, 2011; Carson and Manolagas, 2015; Rossetti et al., 2017). Furthermore, low endogenous testosterone production cause muscle wasting, and injection of testosterone has been shown to improve muscle mass and strength (Falqueto et al., 2021; Harper et al., 2021; Alexander et al., 2022). Thus, serum testosterone levels of athletes are crucial for their skeletal muscle performance.
Gut microbiota is one of the most attractive research focuses in recent years (Heintz-Buschart and Wilmes, 2018; Whon et al., 2021). Exercise could alter the gut microbiota’s structure and affect the metabolic function of skeletal muscle (Frampton et al., 2020). Recent studies suggest that gut microbiota is related to testosterone metabolism (Liu et al., 2017; Colldén et al., 2019). The gut microbiota could convert T to dihydrotestosterone (DHT) and cause deglucuronidation of glucuronidated testosterone (T-G) (Soory, 1995; Colldén et al., 2019). Moreover, the serum testosterone levels in germ-free mice are lower (Markle et al., 2013), but the mice fed with Lactobacillus significantly increase serum testosterone levels compared with mice fed with a normal diet (Poutahidis et al., 2014). Lack of testosterone causes depression. The 3β-hydroxysteroid dehydrogenase expressed by gut microbes degrades testosterone which has been implicated in male depression (Li et al., 2022). What’s more, some studies have demonstrated that Akkermansia was positively correlated with serum testosterone levels (Zhang et al., 2021). Unfortunately, the effects of gut microbiota on testosterone metabolism are unclear.
In this study, we aimed to observe the effect of high-intensity aerobic exercise on skeletal muscle performance. Unexpectedly, we found that exercise could improve skeletal muscle performance by altering the gut microbiota’s structure (Lactobacillus, Allobaculum, and Akkermansia) and testosterone metabolism. Thus, our findings demonstrated that high-intensity aerobic exercise could improve skeletal muscle performance via the gut microbiota-testosterone axis. Together, we provided new insights into the role of gut microbiota in testosterone metabolism and offered new opportunities into improving skeletal muscle performance for athletes.
Materials and methods
Experimental design
The male C57BL/6J mice (7 weeks, 19–21 g) were purchased from the Laboratory Animal Centre of the Army Medical University (Chongqing, China). Mice were kept 4 per cage in a controlled environment (22°C–25°C, 50%–55% humidity, 12 h light/dark cycle) for 7 days before the experiment. All mice obtained the water and standard laboratory chow diet freely (Chen et al., 2019). Animal experiments included two parts (n = 12/group). Part 1: CON, Abx, EX, and EX + Abx. Mice in EX groups were accepted by exercise training for 8 weeks, and Abx groups were subjected to the Abx treatment for 1 week before the training. Part 2: CON-donor and EX-donor. Mice in donor groups were performed by FMT experiment for 1 week. We recorded the body weight and food intake every week.
Tissue isolation
We sacrificed the mice 24 h after the last training. The blood taken from the eyeball was centrifugation (3,000 rpm, 15 min, 4°C) to collect the serum. The small intestine, gastrocnemius muscles (GA), cecum content, and testis were collected and stored at −80°C. All animal studies were approved by the Animal Care and Use Committee of the Army Medical University.
Exercise training protocol
The EX groups were subjected to exercise on the motorized treadmill (Jiangsu, China). Mice were adapted to the treadmill environment for 1 week (0°, 15 m/min, 10 min; Fernando et al., 1993; Zhang et al., 2022). Then, according to Bedford’s method (Bedford et al., 1979; E et al., 2013; Kim et al., 2015), mice were randomly assigned to high-intensity aerobic exercise (10°, 20 m/min, 60 min, 5 day/week) for 8 weeks.
Antibiotic treatment and FMT experiments
Antibiotic treatment: mice were subjected to the antibiotic cocktail (Abx) (0.5 g/L vancomycin, 1 g/L ampicillin, 1 g/L metronidazole, and 1 g/L neomycin sulfate) for 1 week as literature reported (Miyauchi et al., 2020; Hui et al., 2020a; Secombe et al., 2021; Li et al., 2022). FMT experiments: we collected the fresh fecal from mice in CON and EX groups after the last intervention. The fecal (50 mg) was dissolved in 2.5 ml PBS, shaken (2 min), and centrifuged (10,000 g, 10 min, 4°C) to collect the supernatant. The mice in donor groups were treated with the Abx treatment for 1 week, then administered with the above 200 μl supernatant for 1 week (Sun et al., 2018). And the effects of gut microbiota elimination and FMT were confirmed by stool DNA concentration analysis. We collected fecal samples at 1 day before antibiotic treatment, 1 week after antibiotic treatment, and 1 week after FMT experiment.
Grip strength test
The muscle grip strength of the mice was measured by a grasping instrument (Ugo Basile, Italy) 24 h after the last exercise training (Huang et al., 2021). With the frequency by 3 s/time, we recorded the peak value until the mouse was pulled from the bar through the tail horizontally away.
Biochemical analysis
The serum testosterone levels were detected by ELISA kits (Ruixing Biological, Quanzhou, China). We used the biochemical analyzer to detect glucose, cholesterol, high-density lipoprotein cholesterol, low-density lipoprotein cholesterol, and triglyceride in serum.
Histological analysis
Eosin (H&E) staining and transmission electron microscopy were performed as previously described (Hou et al., 2020). Eosin (H&E) staining: tissues were incubated with fixed liquid (Servicebio, Wuhan, China) for at least 24 h. Then, tissues were embedded in paraffin, sectioned at 5 μm, and stained with hematoxylin–eosin. The CSA of myofibers was determined by ImageJ (NIH) software. Transmission electron microscopy: tissues were fixed in 2.5% glutaraldehyde solution (18 h) and fixed in osmium solution (2 h). Acetone gradient dehydration, acetone-resin (1:1) soaked (4 h), and embedding agent soaked (18 h). Then, tissues were placed in an embedding frame, embedded with resin, soaked, and polymerized at high temperatures for 48 h. Tissues were sectioned at 60 nm and stained with 2% uranyl acetate and lead citrate. Morphology of tissues was observed and photographed by the JEM-1400 microscope (JEOL, Tokyo, Japan; Liu et al., 2020).
Quantitative polymerase chain reaction
According to previous studies (Hui et al., 2019; Zeng et al., 2019; Hui et al., 2020b), we used RNAiso Plus (Takara, Japan) to isolate total RNA from GA and testis. Then we reverse-transcribed RNA into cDNA using PrimeScript RT reagent Kit (Takara, Japan). We performed the qPCR using qTower 2.2 real-time PCR system (Analytik Jena, Germany). The primers were synthesized by Sangon Biotech (Shanghai, China) and listed in Supplementary Table 1.
Sequencing of the gut microbiota
We collected the fecal samples using metabolic cages 24 h after the final training and saved them at −80°C until required. The 16S rRNA gene of the DNA sequence was analyzed by QILME2 software and the Illumina MiSeq platform (Illumina, San Diego, CA, United States; Bolyen et al., 2019). The α-diversity was analyzed via Ace, Chao, Sobs, Pd, Shannon, Shannoneven, Simpson, and Simpsoneven’s indices, and the Student’s t-test was used for statistical comparison. The principle coordinate analysis (PCoA) of β-diversity based on Hellinger was analyzed by analysis of similarities (ANOSIM). Then, we used the Linear Discriminant Analysis Effect Size (LEfSe) method to discover the bacterial biomarkers. We performed the LEfSe at a false discover rate (FDR) < 0.05 and linear discriminant analysis (LDA) score of >2.0.
RNA sequencing analysis
We collected GA from C57BL/6J mice and extracted the total RNA using TRIzol® Reagent. Then RNA quality was determined by 2100 Bioanalyser (Agilent Technologies, United States) and ND-2000 (NanoDrop Technologies, United States). The clean reads were obtained by FASTP software (Chen et al., 2018), and TPM indicated gene expression (Reads PerKilobases per Million reads). We selected the genes with at least 1.5-fold changes as differentially expressed.
Statistical analysis
All experimental data were analyzed by GraphPad Prism 7 and SPSS 19.0 software. The data were presented as the mean ± SEM. The Student’s t-test was used to analyze data between 2 groups. One-way analysis of variance (ANOVA) was conducted for the comparison of more than 2 groups. Two-way ANOVA was used for multiple factors analysis (∗p < 0.05; ∗∗p < 0.01; ∗∗∗p < 0.001).
Results
High-intensity aerobic exercise improved skeletal muscle performance
To explore the effect of exercise on C57BL/6J mice, mice were subjected to treadmill running for 8 weeks (Figure 1A). The EX group had significantly less body weight (Figure 1B) and food intake (Figure 1C) than the CON group. However, exercise significantly improved the grip strength (Figure 1D) and GA percentage (Figure 1E) of mice in the EX group, which was positively correlated with testosterone levels in serum (Figure 1F) and cecum content (Supplementary Figure 1A). The testis percentage (Supplementary Figure 1B) and serum lactate levels (Supplementary Figure 1C) were the same between CON and EX groups. Besides, exercise could significantly increase serum GLU levels (Figure 1G) and low serum lipids levels (Figure 1H), indicating that glycogen reserve was elevated in the EX group. Moreover, there were no significant differences in serum alanine aminotransferase (ALT) and aspartate aminotransferase (AST) levels between the 2 groups (CON and EX; Supplementary Figures 1D,E). These results indicated that high-intensity aerobic exercise had beneficial effects on C57BL/6J mice.
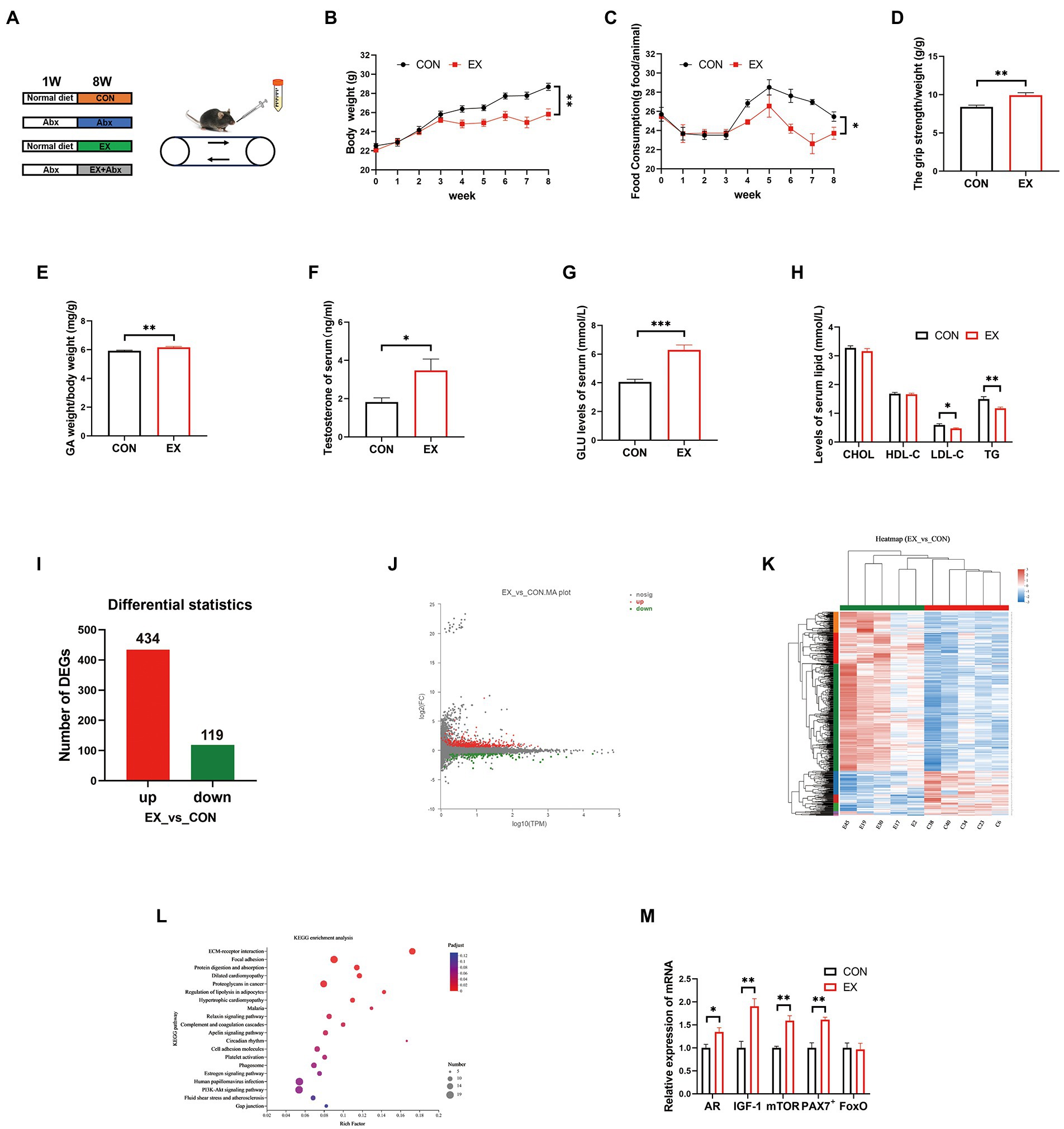
Figure 1. High loading intensity of aerobic exercise improved skeletal muscle performance (CON vs. EX). (A) Mice were subjected to treadmill training and antibiotic treatment for 8 weeks (CON, Abx, EX, and EX + Abx). (B) Body weight. (C) Food intake. (D) Grip strength. (E) GA percentage. (F) Serum testosterone levels. (G) Serum GLU levels. (H) Serum lipids levels. (I) Differentially expressed genes (DEGs). (J) MA plot. (K) Heat map of DEGs. (L) KEGG_pathway of DEGs. (M) The expression of related genes (AR, IGF-1, mTOR, PAX7+, and FoxO) by qPCR (∗p < 0.05; ∗∗p < 0.01; ∗∗∗p < 0.001).
To further confirm the effect of exercise on gene expression in GA, we performed RNA sequencing. The RNA quality was shown in Supplementary Figure 2A and Supplementary Table 2. There are significant differences in gene expression between CON and EX groups (Supplementary Table 3; Figure 1I; and Supplementary Figure 2B). The MA plot was performed by R package ggplot2 (Figure 1J) and the heatmap was generated by DESeq2 (Figure 1K). The KEGG analysis was further performed on the differential genes, revealing an improvement in the lipolysis signaling pathway (Figure 1L; Supplementary Table 4) under exercise. Moreover, exercise improved the expression of skeletal muscle hypertrophy-related genes (Figure 1M). Altogether, these data confirmed that high-intensity aerobic exercise could alter the expression of related genes in GA.
The CSA of the skeletal muscle had been increased in the EX group (Figures 2A,B). Unexpectedly, we found mitochondria oxidative stress damage of skeletal muscle in the EX group through transmission electron microscopy (Figures 2C,E). Besides, there was no obvious difference in the testis between the two groups by HE staining (Figure 2F). Exercise could not alter the expression of StAR, CYP11A1, and 17β-HSD (Figure 2G), indicating that the production of testosterone was not changed. Unexpectedly, the expression of barrier-related genes was substantially decreased in the EX group (Supplementary Figure 2C). Moreover, through transmission electron microscopy, exercise could substantially cause damage to the intestinal in the EX group (Figure 2H) and substantially decreased the expression of barrier-related genes (Figure 2I). These results indicated that high-intensity aerobic exercise could induce skeletal muscle hypertrophy, but also caused some damage to the body during the exercise injury period.
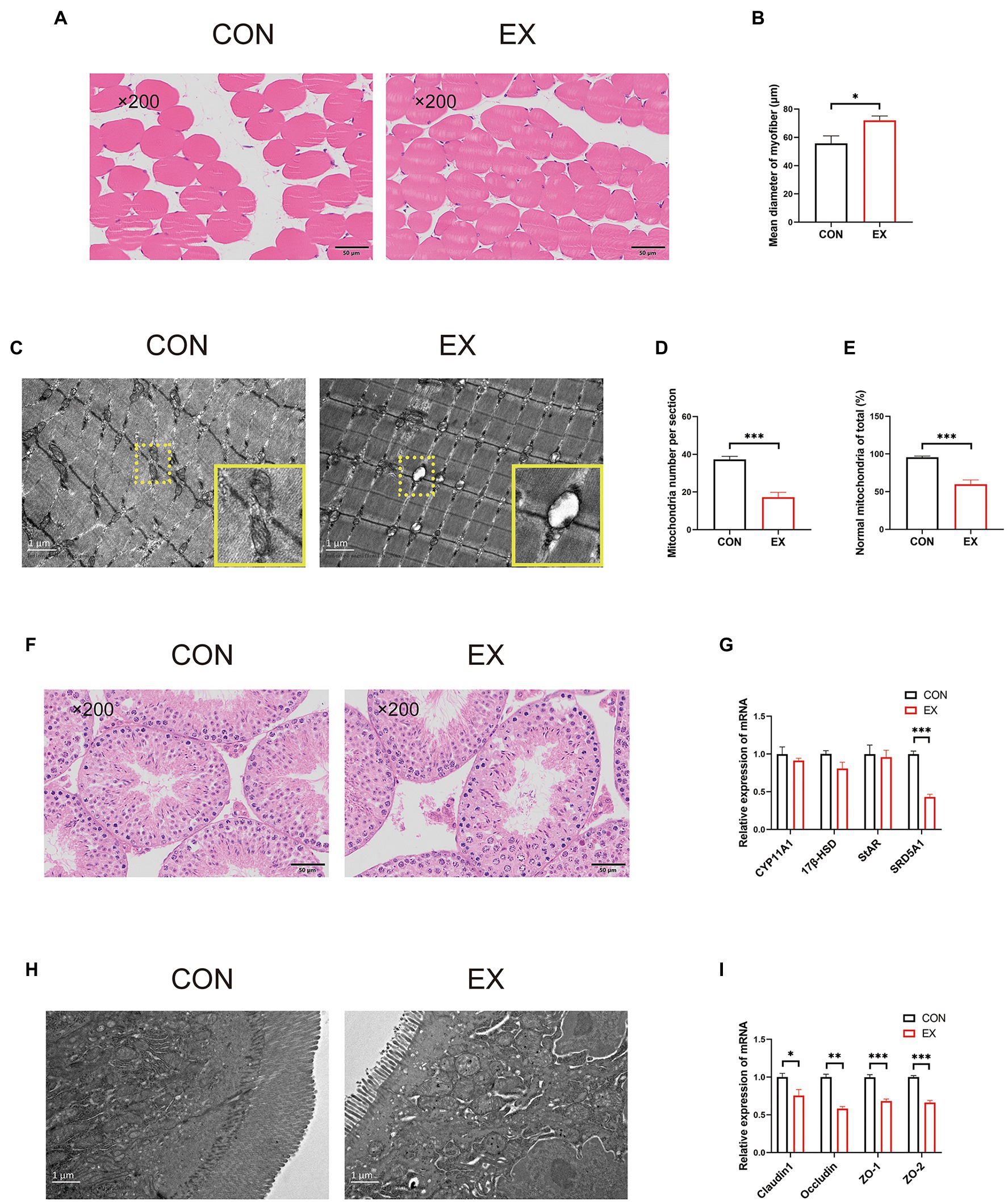
Figure 2. High-intensity aerobic exercise improved the CSA of the skeletal muscle (CON vs. EX). (A) HE staining of the skeletal muscle. (B) Mean diameter of the myofiber. (C–E) Mitochondria oxidative stress damage to skeletal muscle through transmission electron microscopy. (F) HE staining of the testis. (G) The expression of StAR, CYP11A,17β-HSD, and SRD5A1 by qPCR. (H) Transmission electron microscopy of the small intestine. (I) The expression of Claudin1, Occludin, ZO-1, and ZO-2 by qPCR (∗p < 0.05; ∗∗p < 0.01; ∗∗∗p < 0.001).
High-intensity aerobic exercise remodeled the gut microbiota’s structure
To explore the effects of exercise on the gut microbiota’s structure, we collected the fecal samples from CON and EX groups after the final training and performed 16S rRNA profiling. We used the Illumina platform (Majorbio, China) to generate 1,346,823 sequences ranging from 68,897 to 114,648 per sample (n = 16 in total) representing 10,114 ASVs (Supplementary Table 5). There were no significant differences in gene enrichment, species abundance, and community evenness on the genus level by Pan analysis (Supplementary Figure 3A) and Rank abundance curve (Supplementary Figure 3B). A comparison of alpha diversity based on the genus level were no statistical difference (Supplementary Figures 3C–J; Supplementary Table 6). Moreover, we found a clear separation based on the hellinger by principal coordinate analysis (PCoA; Figure 3A). The hierarchical cluster analysis was performed based on unweighted_unifrac (Figure 3B), suggesting that exercise altered the gut microbiota’s structure.
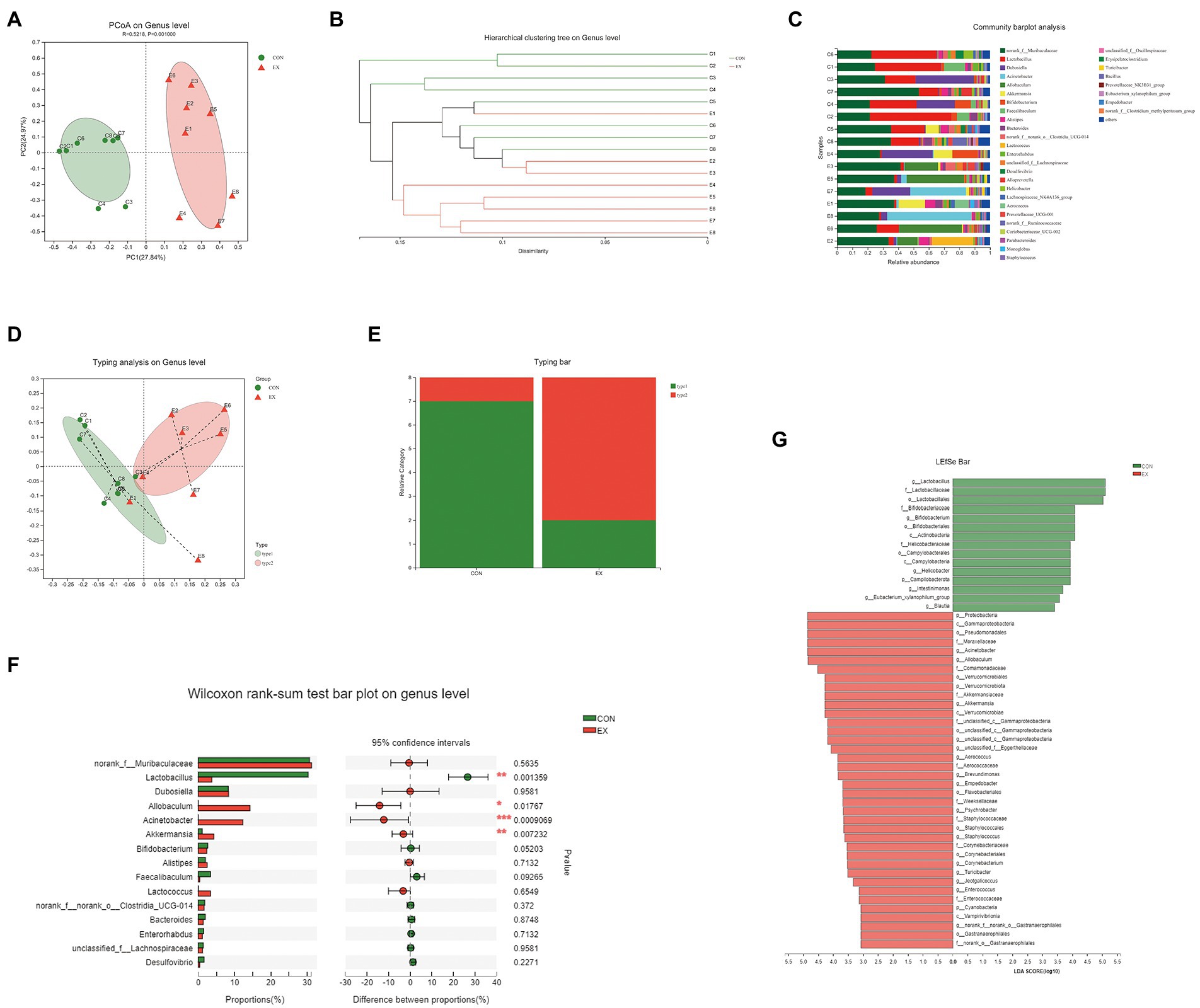
Figure 3. High-intensity aerobic exercise remodeled the gut microbiota structure (CON vs. EX). (A) A clear separation was observed by principal coordinate analysis (PCoA) based on the hellinger. (B) Hierarchical cluster analysis based on unweighted_unifrac. (C) Species composition of 16 samples on the genus level. (D,E) 16 samples were clustered into 2 distinct enterotypes using abund_jaccard. (F) The Wilcoxon rank-sum test bar plot on the genus level. (G) Results of the LEfSe analysis.
On the genus level, the species composition of samples was shown in Figure 3C. Then, all samples were clustered into 2 distinct enterotypes at the genus level using abund_jaccard (Figures 3D,E). The major contributors in type 1 (green) were Muribaculaceae (30.31%) and Lactobacillus (26.18%; Supplementary Table 7). And the key contributors in type 2 (red) were Muribaculaceae (30.11%) and Allobaculum (15.61%; Supplementary Table 8). The percentage of type 1 was 87.5% in the CON groups, and type 2 (75%) was more prevalent in the EX group. Moreover, exercise increased the abundance of Allobaculum, Acinetobacter, and Akkermansia, whereas reduced Lactobacillus (Figure 3F). The LEfSe analysis results indicated that exercise caused an increase in the relative abundance of members of the family Akkermansiaceae, genus Akkermansia, and genus Allobaculum (Figure 3G). Taken together, these results indicated that high-intensity aerobic exercise could modify the gut microbiota’s structure.
The beneficial effect of high-intensity aerobic exercise disappeared after the elimination of the gut microbiota
To observe the key role of the gut microbiota, we performed the Abx treatment (Figure 1A). The stool DNA concentration from stool samples was significantly decrease after Abx treatment (Supplementary Figure 4A). Compared with the normal groups, the Abx groups had significantly less body weight (Figure 4A; Supplementary Figure 4B) and food intake (Figure 4B; Supplementary Figure 4C). Besides, the grip strength (Figure 4C) and GA percentage (Figure 4D; Supplementary Figure 4D) of mice were significantly lower in the Abx groups. There was no obvious difference in testis percentage between EX and EX+Abx groups (Figure 4E). However, the serum testosterone levels was significantly lower in EX+Abx group compared with the EX group (Supplementary Figure 4E). Moreover, the Abx treatment could significantly decrease serum GLU levels (Figure 4F; Supplementary Figure 4F) and improve serum lipids levels (Figure 4G; Supplementary Figure 4G), showing that gut microbiota played a crucial role in glucose and lipid metabolism. Compared with the EX group, the Abx treatment decreased the CSA of the skeletal muscle by HE staining (Figures 4H,I). These results indicated that the beneficial effect of exercise disappeared after the elimination of the gut microbiota.
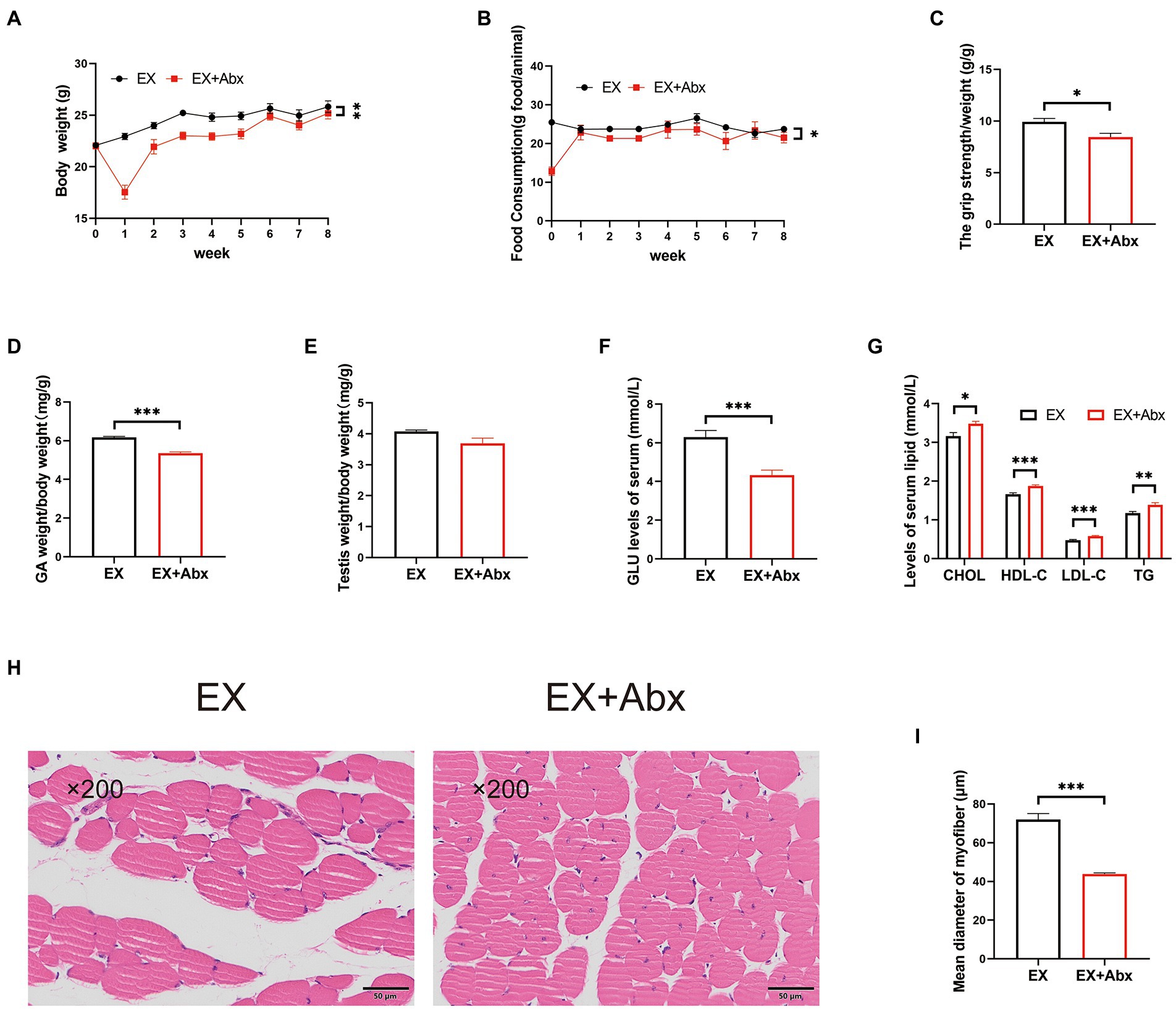
Figure 4. The high-intensity aerobic exercise disappeared after the elimination of the gut microbiota (EX vs. EX+Abx). (A) Body weight. (B) Food intake. (C) Grip strength. (D) GA percentage. (E) Testis percentage. (F) Serum GLU levels. (G) Serum lipids levels. (H) Histological staining of the skeletal muscle. (I) Mean diameter of the myofiber (∗p < 0.05; ∗∗p < 0.01; ∗∗∗p < 0.001).
Gut microbiota played a key role in skeletal muscle performance
To further prove the critical role of gut microbiota, we performed the FMT experiment (Figure 5A). After 1 week FMT experiment, the stool DNA concentration from stool samples was restored (Supplementary Figure 4A). The body weight was the same between the 2 groups (Figure 5B). but the food intake was increased in the EX-donor group (Figure 5C). Moreover, compared with the CON-donor group, the grip strength (Figure 5D) and GA percentage (Figure 5E) were improved in the EX-donor group. The serum testosterone and GLU levels were higher (Figures 5F,G), but the serum lipids levels were lower in the EX-donor group (Figure 5H), indicating that exercise could lower blood lipids. Through HE staining microscopy, the CSA of the skeletal muscle was increased in the EX-donor group (Figures 5I,J). These results suggested that gut microbiota played a key role in skeletal muscle performance.
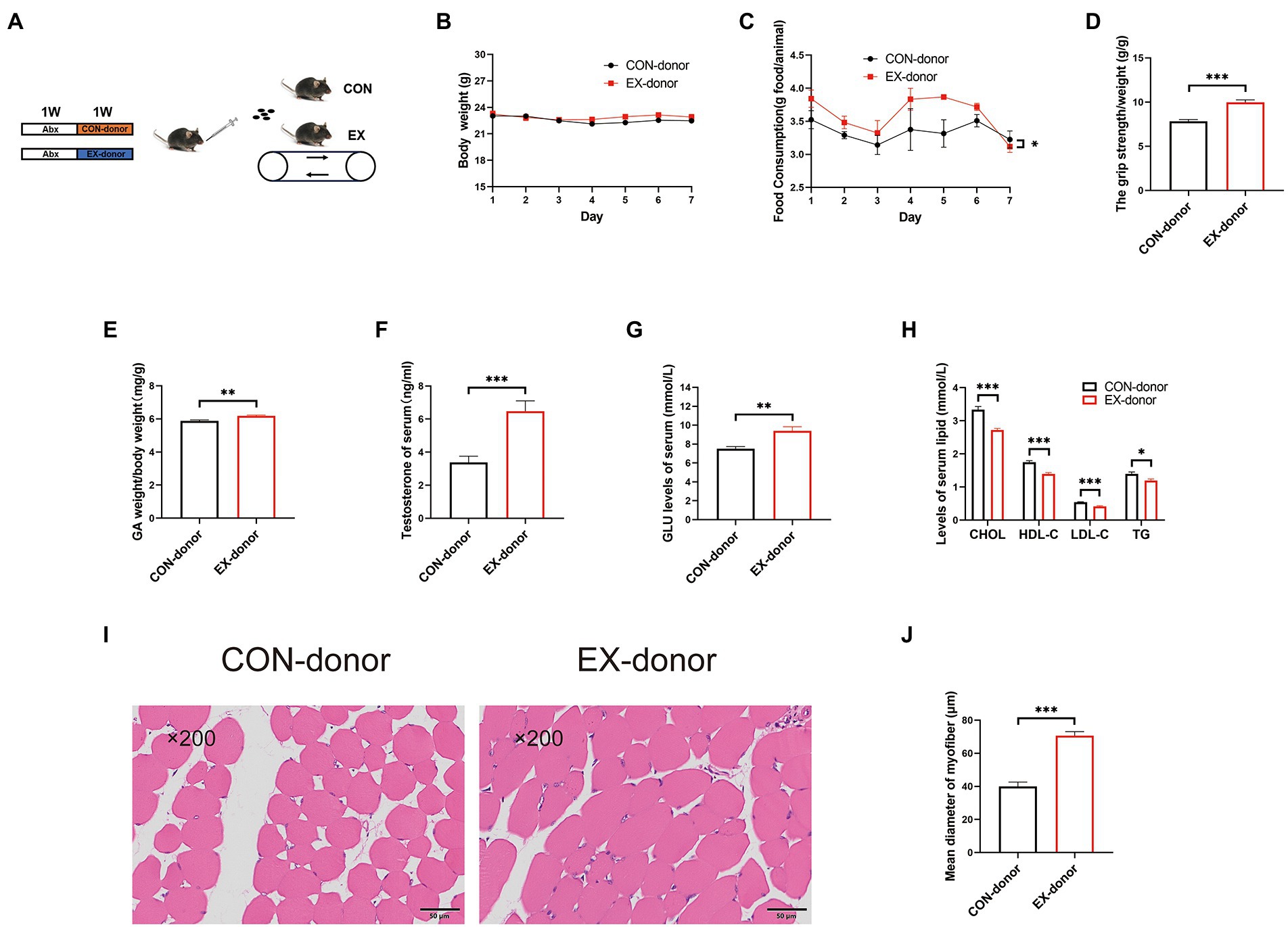
Figure 5. The gut microbiota played a key role in skeletal muscle performance (CON-donor, EX-donor). (A) Mice were subjected to FMT for 1 week. (B) Body weight. (C) Food intake. (D) Grip strength. (E) GA percentage. (F) Testis percentage. (G) Serum GLU levels. (H) Serum lipids levels. (I) HE staining of the skeletal muscle. (J) Mean diameter of the myofiber (∗p < 0.05; ∗∗p < 0.01; ∗∗∗p < 0.001).
Discussion
The quest to increase skeletal muscle performance is widely pursued by athletes. Exercise is widely used to increase skeletal muscle performance, especially for athletes (Camera et al., 2016; Schoenfeld et al., 2016; McKendry et al., 2021). Resistance exercise could significantly induce muscle hypertrophy. It has been reported that the CSA of muscle fiber has increased by more than 50% under resistance exercise (Hubal et al., 2005; Bamman et al., 2007). However, the beneficial effect of high-intensity aerobic exercise on skeletal muscle remains to be elucidated. In our study, we found that the 8-week high-intensity aerobic exercise could improve muscle performance by increasing the CSA of GA.
Testosterone is a key regulator of protein metabolism in muscle (Tipton and Wolfe, 2001). Accumulating evidence indicates that testosterone participates in satellite cell number, muscle mass, and grip strength (Serra et al., 2013). Long-term testosterone replacement therapy (TRT) improves muscle mass in humans with low serum testosterone levels (Kruse et al., 2020). Our results showed that high-intensity aerobic exercise could improve the serum testosterone levels, but not alter the testosterone production in the testis. These results indicated a new endogenous pathway to produce testosterone except the hypothalamic–pituitary-testicular axis (HPA).
It has been repeatedly shown that gut microbiota is related to skeletal muscle performance (Lahiri et al., 2019; Mailing et al., 2019; Scheiman et al., 2019). The germ-free mice show atrophy of skeletal muscle, which could be reversed by FMT (Hsu et al., 2015; Huang et al., 2019). Gut microbiota is the primary regulator of testosterone metabolism, which could convert T-G to T (Colldén et al., 2019). Akkermansia, considered to be a promising candidate for probiotics, might be related to testosterone metabolism (Zhang et al., 2021). Importantly, testosterone produced by the gut microbiota could improve muscle performance by enhancing skeletal muscle protein synthesis (Rossetti et al., 2017). At the genus level, we further demonstrated that exposure to the long-term high loading intensity of aerobic exercise increased the abundance of Allobaculum and Akkermansia, accompanied by testosterone production in C57BL/6 mice.
Our results revealed that the high-intensity aerobic exercise remodeled the gut microbiota’s structure and improved skeletal muscle performance via the gut microbiota-testosterone axis (Figure 6). We provided new insights into improving serum testosterone levels through endogenous pathways. However, exercise might cause mitochondrial oxidative stress injury in the skeletal muscle during the exercise injury period, further investigation was needed to prevent injury induced by the high-intensity exercise. Exercise combined with sports nutrition supplements might be a better choice for athletes. Further study should be required to observe the beneficial effect of high-intensity aerobic exercise, sports nutrition supplements, and gut microbiota on athletes.
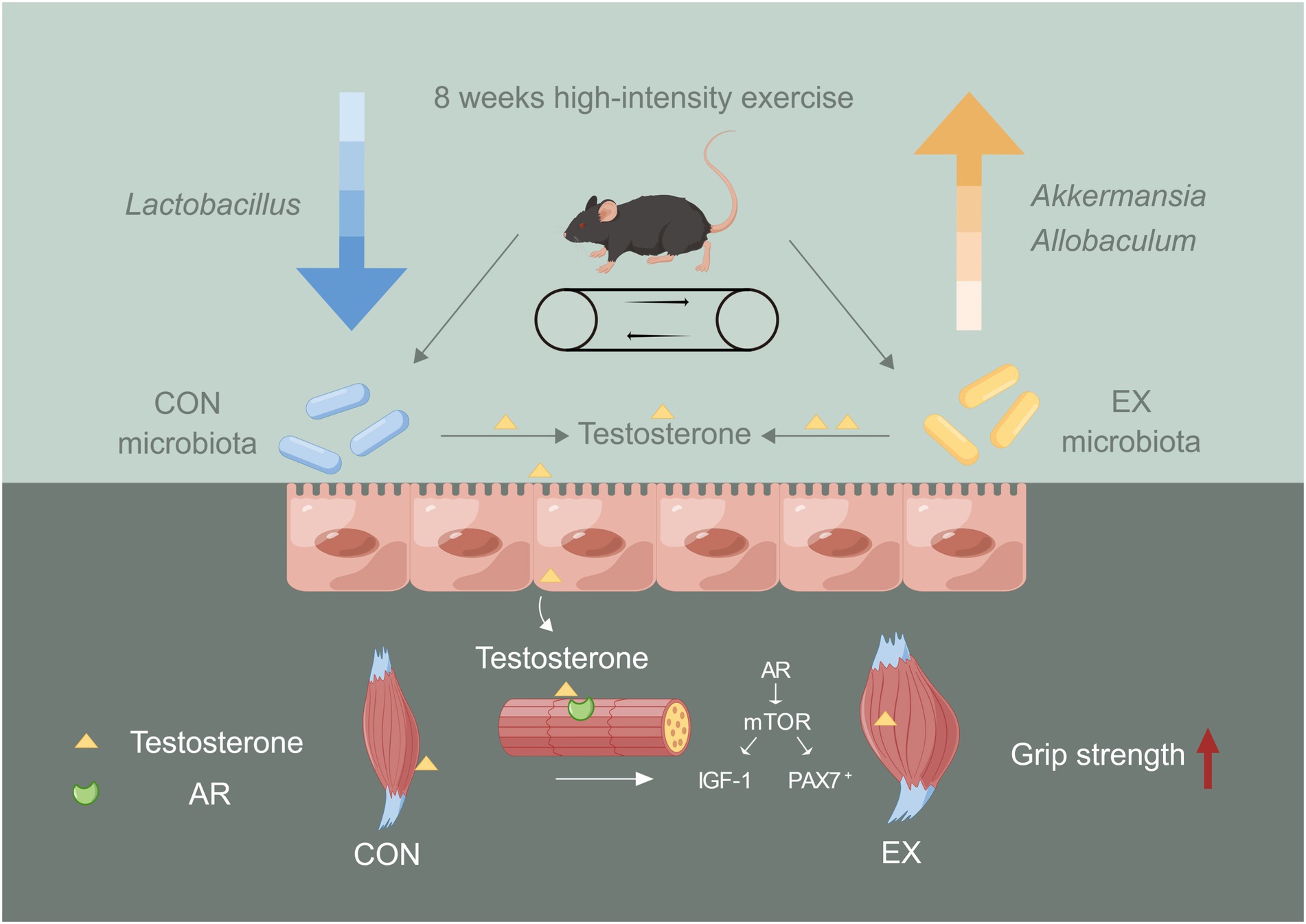
Figure 6. The role of gut microbiota on skeletal muscle performance by high-intensity aerobic exercise (by Figdraw). The long-term (8 weeks) high loading intensity of aerobic exercise improves serum testosterone levels and skeletal muscle performance by remodeling the gut microbiota’s structure (Lactobacillus, Allobaculum, and Akkermansia).
Conclusion
Overall, our study revealed that long-term high loading intensity of aerobic exercise appeared to remodel the gut microbiota’s structure (Lactobacillus, Allobaculum, and Akkermansia), affected testosterone metabolism, and improved skeletal muscle performance. And we provided new insights into the role of the gut microbiome in testosterone metabolism.
Data availability statement
The data presented in the study are deposited in the NCBI repository, accession number PRJNA 871043 and PRJNA872497.
Ethics statement
The animal study was reviewed and approved by the Animal Care and Use Committee of the Army Medical University.
Author contributions
LZ designed the study, instructed all experiments, and drafted the manuscript. HL carried out the data analysis. LR, GT, and HS assisted in running training and performing the experiments. MM, LY, JZ, and QZ provided many suggestions on the articles and obtained funding. All authors contributed to the article and approved the final manuscript.
Funding
This work was supported by the grants from the Key Projects for Scientific Research (AWS17J014 and HZ2022-158).
Conflict of interest
The authors declare that the research was conducted in the absence of any commercial or financial relationships that could be construed as a potential conflict of interest.
Publisher’s note
All claims expressed in this article are solely those of the authors and do not necessarily represent those of their affiliated organizations, or those of the publisher, the editors and the reviewers. Any product that may be evaluated in this article, or claim that may be made by its manufacturer, is not guaranteed or endorsed by the publisher.
Supplementary material
The Supplementary material for this article can be found online at: https://www.frontiersin.org/articles/10.3389/fmicb.2022.1049469/full#supplementary-material
Abbreviations
Abx, antibiotic cocktail; HPA, hypothalamic-pituitary-testicular axis; DHT, dihydrotestosterone; T-G, glucuronidated testosterone; GA, gastrocnemius muscles; FMT, fecal microbiota transplantation; CSA, cross-sectional area; ANOVA, one-way analysis of variance; ALT, alanine aminotransferase; AST, aspartate aminotransferase; PCoA, principal coordinate analysis; DEGs, differentially expressed genes; TRT, testosterone replacement therapy.
References
Alexander, S. E., Pollock, A. C., and Lamon, S. (2022). The effect of sex hormones on skeletal muscle adaptation in females. Eur. J. Sport Sci. 22, 1035–1045. doi: 10.1080/17461391.2021.1921854
Anderson, R. C., Newton, C. L., Anderson, R. A., and Millar, R. P. (2018). Gonadotropins and their analogs: current and potential clinical applications. Endocr. Rev. 39, 911–937. doi: 10.1210/er.2018-00052
Bahreinipour, M.-A., Joukar, S., Hovanloo, F., Najafipour, H., Naderi, V., Rajiamirhasani, A., et al. (2018). Mild aerobic training with blood flow restriction increases the hypertrophy index and MuSK in both slow and fast muscles of old rats: role of PGC-1α. Life Sci. 202, 103–109. doi: 10.1016/j.lfs.2018.03.051
Bamman, M. M., Petrella, J. K., Kim, J.-S., Mayhew, D. L., and Cross, J. M. (2007). Cluster analysis tests the importance of myogenic gene expression during myofiber hypertrophy in humans. J. Appl. Physiol. 102, 2232–2239. doi: 10.1152/japplphysiol.00024.2007
Baulieu, E. E. (1991). Neurosteroids: a new function in the brain. Biol. Cell. 71, 3–10. doi: 10.1016/0248-4900(91)90045-O
Bedford, T. G., Tipton, C. M., Wilson, N. C., Oppliger, R. A., and Gisolfi, C. V. (1979). Maximum oxygen consumption of rats and its changes with various experimental procedures. J. Appl. Physiol. Respir. Environ. Exerc. Physiol. 47, 1278–1283. doi: 10.1152/jappl.1979.47.6.1278
Bélanger, A., Pelletier, G., Labrie, F., Barbier, O., and Chouinard, S. (2003). Inactivation of androgens by UDP-glucuronosyltransferase enzymes in humans. Trends Endocrinol Metab 14, 473–479. doi: 10.1016/j.tem.2003.10.005
Bolyen, E., Rideout, J. R., Dillon, M. R., Bokulich, N. A., Abnet, C. C., Al-Ghalith, G. A., et al. (2019). Reproducible, interactive, scalable and extensible microbiome data science using QIIME 2. Nat. Biotechnol. 37, 852–857. doi: 10.1038/s41587-019-0209-9
Camera, D. M., Smiles, W. J., and Hawley, J. A. (2016). Exercise-induced skeletal muscle signaling pathways and human athletic performance. Free Radic. Biol. Med. 98, 131–143. doi: 10.1016/j.freeradbiomed.2016.02.007
Carson, J. A., and Manolagas, S. C. (2015). Effects of sex steroids on bones and muscles: similarities, parallels, and putative interactions in health and disease. Bone 80, 67–78. doi: 10.1016/j.bone.2015.04.015
Chen, M., Hui, S., Lang, H., Zhou, M., Zhang, Y., Kang, C., et al. (2019). SIRT3 deficiency promotes high-fat diet-induced nonalcoholic fatty liver disease in correlation with impaired intestinal permeability through gut microbial Dysbiosis. Mol. Nutr. Food Res. 63:e1800612. doi: 10.1002/mnfr.201800612
Chen, S., Zhou, Y., Chen, Y., and Gu, J. (2018). Fastp: an ultra-fast all-in-one FASTQ preprocessor. Bioinformatics 34, i884–i890. doi: 10.1093/bioinformatics/bty560
Colldén, H., Landin, A., Wallenius, V., Elebring, E., Fändriks, L., Nilsson, M. E., et al. (2019). The gut microbiota is a major regulator of androgen metabolism in intestinal contents. Am. J. Physiol. Endocrinol. Metab. 317, E1182–E1192. doi: 10.1152/ajpendo.00338.2019
E, L., Lu, J., Selfridge, J. E., Burns, J. M., and Swerdlow, R. H. (2013). Lactate administration reproduces specific brain and liver exercise-related changes. J. Neurochem. 127, 91–100. doi: 10.1111/jnc.12394
Falqueto, H., Júnior, J. L. R., Silvério, M. N. O., Farias, J. C. H., Schoenfeld, B. J., and Manfredi, L. H. (2021). Can conditions of skeletal muscle loss be improved by combining exercise with anabolic-androgenic steroids? A systematic review and meta-analysis of testosterone-based interventions. Rev. Endocr. Metab. Disord. 22, 161–178. doi: 10.1007/s11154-021-09634-4
Fernando, P., Bonen, A., and Hoffman-Goetz, L. (1993). Predicting submaximal oxygen consumption during treadmill running in mice. Can. J. Physiol. Pharmacol. 71, 854–857. doi: 10.1139/y93-128
Frampton, J., Murphy, K. G., Frost, G., and Chambers, E. S. (2020). Short-chain fatty acids as potential regulators of skeletal muscle metabolism and function. Nat. Metab. 2, 840–848. doi: 10.1038/s42255-020-0188-7
Harber, M. P., Konopka, A. R., Douglass, M. D., Minchev, K., Kaminsky, L. A., Trappe, T. A., et al. (2009). Aerobic exercise training improves whole muscle and single myofiber size and function in older women. Am. J. Physiol. Regul. Integr. Comp. Physiol. 297, R1452–R1459. doi: 10.1152/ajpregu.00354.2009
Harber, M. P., Konopka, A. R., Undem, M. K., Hinkley, J. M., Minchev, K., Kaminsky, L. A., et al. (2012). Aerobic exercise training induces skeletal muscle hypertrophy and age-dependent adaptations in myofiber function in young and older men. J. Appl. Physiol. 113, 1495–1504. doi: 10.1152/japplphysiol.00786.2012
Harper, J., O'Donnell, E., Sorouri Khorashad, B., McDermott, H., and Witcomb, G. L. (2021). How does hormone transition in transgender women change body composition, muscle strength and haemoglobin? Systematic review with a focus on the implications for sport participation. Br. J. Sports Med. 55, 865–872. doi: 10.1136/bjsports-2020-103106
Heintz-Buschart, A., and Wilmes, P. (2018). Human gut microbiome: function matters. Trends Microbiol. 26, 563–574. doi: 10.1016/j.tim.2017.11.002
Hou, P., Zhou, X., Yu, L., Yao, Y., Zhang, Y., Huang, Y., et al. (2020). Exhaustive exercise induces gastrointestinal syndrome through reduced ILC3 and IL-22 in mouse model. Med. Sci. Sports Exerc. 52, 1710–1718. doi: 10.1249/MSS.0000000000002298
Hsu, Y. J., Chiu, C. C., Li, Y. P., Huang, W. C., Huang, Y. T., Huang, C. C., et al. (2015). Effect of intestinal microbiota on exercise performance in mice. J. Strength Cond. Res. 29, 552–558. doi: 10.1519/JSC.0000000000000644
Huang, W.-C., Chen, Y.-H., Chuang, H.-L., Chiu, C.-C., and Huang, C.-C. (2019). Investigation of the effects of microbiota on exercise physiological adaption, performance, and energy utilization using a Gnotobiotic animal model. Front. Microbiol. 10:1906. doi: 10.3389/fmicb.2019.01906
Huang, L., Li, T., Zhou, M., Deng, M., Zhang, L., Yi, L., et al. (2021). Hypoxia improves endurance performance by enhancing short chain fatty acids production gut microbiota remodeling. Front. Microbiol. 12:820691. doi: 10.3389/fmicb.2021.820691
Hubal, M. J., Gordish-Dressman, H., Thompson, P. D., Price, T. B., Hoffman, E. P., Angelopoulos, T. J., et al. (2005). Variability in muscle size and strength gain after unilateral resistance training. Med. Sci. Sports Exerc. 37, 964–972.
Hui, S., Huang, L., Wang, X., Zhu, X., Zhou, M., Chen, M., et al. (2020a). Capsaicin improves glucose homeostasis by enhancing glucagon-like peptide-1 secretion through the regulation of bile acid metabolism via the remodeling of the gut microbiota in male mice. FASEB J. 34, 8558–8573. doi: 10.1096/fj.201902618RR
Hui, S., Liu, Y., Chen, M., Wang, X., Lang, H., Zhou, M., et al. (2019). Capsaicin improves glucose tolerance and insulin sensitivity through modulation of the gut microbiota-bile acid-FXR Axis in type 2 diabetic db/db mice. Mol. Nutr. Food Res. 63:e1900608. doi: 10.1002/mnfr.201900608
Hui, S., Liu, Y., Huang, L., Zheng, L., Zhou, M., Lang, H., et al. (2020b). Resveratrol enhances brown adipose tissue activity and white adipose tissue browning in part by regulating bile acid metabolism via gut microbiota remodeling. Int. J. Obes. 44, 1678–1690. doi: 10.1038/s41366-020-0566-y
Khajehnasiri, N., Khazali, H., and Sheikhzadeh, F. (2018). Various responses of male pituitary-gonadal axis to different intensities of long-term exercise: role of expression of KNDYrelated genes. J. Biosci. 43, 569–574. doi: 10.1007/s12038-018-9782-1
Kim, J. H., Pan, J. H., Lee, E. S., and Kim, Y. J. (2015). L-Carnitine enhances exercise endurance capacity by promoting muscle oxidative metabolism in mice. Biochem. Biophys. Res. Commun. 464, 568–573. doi: 10.1016/j.bbrc.2015.07.009
Konopka, A. R., and Harber, M. P. (2014). Skeletal muscle hypertrophy after aerobic exercise training. Exerc. Sport Sci. Rev. 42, 53–61. doi: 10.1249/JES.0000000000000007
Kruse, R., Petersson, S. J., Christensen, L. L., Kristensen, J. M., Sabaratnam, R., Ørtenblad, N., et al. (2020). Effect of long-term testosterone therapy on molecular regulators of skeletal muscle mass and fibre-type distribution in aging men with subnormal testosterone. Metabolism 112:154347. doi: 10.1016/j.metabol.2020.154347
Lahiri, S., Kim, H., Garcia-Perez, I., Reza, M. M., Martin, K. A., Kundu, P., et al. (2019). The gut microbiota influences skeletal muscle mass and function in mice. Sci. Transl. Med. 11:eaan5662. doi: 10.1126/scitranslmed.eaan5662
Leproult, R., and Van Cauter, E. (2011). Effect of 1 week of sleep restriction on testosterone levels in young healthy men. JAMA 305, 2173–2174. doi: 10.1001/jama.2011.710
Li, D., Liu, R., Wang, M., Peng, R., Fu, S., Fu, A., et al. (2022). 3β-Hydroxysteroid dehydrogenase expressed by gut microbes degrades testosterone and is linked to depression in males. Cell Host Microbe 30, 329–339.e5. doi: 10.1016/j.chom.2022.01.001
Liu, Y., Lang, H., Zhou, M., Huang, L., Hui, S., Wang, X., et al. (2020). The preventive effects of Pterostilbene on the exercise intolerance and circadian misalignment of mice subjected to sleep restriction. Mol. Nutr. Food Res. 64:e1900991. doi: 10.1002/mnfr.201900991
Liu, R., Zhang, C., Shi, Y., Zhang, F., Li, L., Wang, X., et al. (2017). Dysbiosis of gut microbiota associated with clinical parameters in polycystic ovary syndrome. Front. Microbiol. 8:324. doi: 10.3389/fmicb.2017.00324
Mailing, L. J., Allen, J. M., Buford, T. W., Fields, C. J., and Woods, J. A. (2019). Exercise and the gut microbiome: a review of the evidence, potential mechanisms, and implications for human health. Exerc. Sport Sci. Rev. 47, 75–85. doi: 10.1249/JES.0000000000000183
Markle, J. G. M., Frank, D. N., Mortin-Toth, S., Robertson, C. E., Feazel, L. M., Rolle-Kampczyk, U., et al. (2013). Sex differences in the gut microbiome drive hormone-dependent regulation of autoimmunity. Science 339, 1084–1088. doi: 10.1126/science.1233521
McKendry, J., Stokes, T., McLeod, J. C., and Phillips, S. M. (2021). Resistance exercise, aging, disuse, and muscle protein metabolism. Compr. Physiol. 11, 2249–2278. doi: 10.1002/cphy.c200029
Miyauchi, E., Kim, S.-W., Suda, W., Kawasumi, M., Onawa, S., Taguchi-Atarashi, N., et al. (2020). Gut microorganisms act together to exacerbate inflammation in spinal cords. Nature 585, 102–106. doi: 10.1038/s41586-020-2634-9
Poutahidis, T., Springer, A., Levkovich, T., Qi, P., Varian, B. J., Lakritz, J. R., et al. (2014). Probiotic microbes sustain youthful serum testosterone levels and testicular size in aging mice. PLoS One 9:e84877. doi: 10.1371/journal.pone.0084877
Rossetti, M. L., Steiner, J. L., and Gordon, B. S. (2017). Androgen-mediated regulation of skeletal muscle protein balance. Mol. Cell. Endocrinol. 447, 35–44. doi: 10.1016/j.mce.2017.02.031
Sandberg, A. A., and Slaunwhite, W. R. (1956). Metabolism of 4-C14-testosterone in human subjects. I. Distribution in bile, blood, feces and urine. J. Clin. Invest. 35, 1331–1339. doi: 10.1172/JCI103389
Scheiman, J., Luber, J. M., Chavkin, T. A., MacDonald, T., Tung, A., Pham, L.-D., et al. (2019). Meta-omics analysis of elite athletes identifies a performance-enhancing microbe that functions via lactate metabolism. Nat. Med. 25, 1104–1109. doi: 10.1038/s41591-019-0485-4
Schoenfeld, B. J., Ogborn, D., and Krieger, J. W. (2016). Effects of resistance training frequency on measures of muscle hypertrophy: a systematic review and meta-analysis. Sports Med. 46, 1689–1697. doi: 10.1007/s40279-016-0543-8
Secombe, K. R., Al-Qadami, G. H., Subramaniam, C. B., Bowen, J. M., Scott, J., Van Sebille, Y. Z. A., et al. (2021). Guidelines for reporting on animal fecal transplantation (GRAFT) studies: recommendations from a systematic review of murine transplantation protocols. Gut Microbes 13:1979878. doi: 10.1080/19490976.2021.1979878
Serra, C., Tangherlini, F., Rudy, S., Lee, D., Toraldo, G., Sandor, N. L., et al. (2013). Testosterone improves the regeneration of old and young mouse skeletal muscle. The journals of gerontology. Ser. A Biol. Sci. Med. Sci. 68, 17–26. doi: 10.1093/gerona/gls083
Sharma, R., Biedenharn, K. R., Fedor, J. M., and Agarwal, A. (2013). Lifestyle factors and reproductive health: taking control of your fertility. Reprod. Biol. Endocrinol. 11:66. doi: 10.1186/1477-7827-11-66
Soory, M. (1995). Bacterial steroidogenesis by periodontal pathogens and the effect of bacterial enzymes on steroid conversions by human gingival fibroblasts in culture. J. Periodontal Res. 30, 124–131. doi: 10.1111/j.1600-0765.1995.tb01261.x
Suchomel, T. J., Nimphius, S., Bellon, C. R., and Stone, M. H. (2018). The importance of muscular strength: training considerations. Sports Med. 48, 765–785. doi: 10.1007/s40279-018-0862-z
Sun, L., Xie, C., Wang, G., Wu, Y., Wu, Q., Wang, X., et al. (2018). Gut microbiota and intestinal FXR mediate the clinical benefits of metformin. Nat. Med. 24, 1919–1929. doi: 10.1038/s41591-018-0222-4
Tipton, K. D., and Wolfe, R. R. (2001). Exercise, protein metabolism, and muscle growth. Int. J. Sport Nutr. Exerc. Metab. 11, 109–132. doi: 10.1123/ijsnem.11.1.109
Torma, F., Gombos, Z., Fridvalszki, M., Langmar, G., Tarcza, Z., Merkely, B., et al. (2021). Blood flow restriction in human skeletal muscle during rest periods after high-load resistance training down-regulates miR-206 and induces Pax7. J. Sport Health Sci. 10, 470–477. doi: 10.1016/j.jshs.2019.08.004
Vingren, J. L., Kraemer, W. J., Ratamess, N. A., Anderson, J. M., Volek, J. S., and Maresh, C. M. (2010). Testosterone physiology in resistance exercise and training: the up-stream regulatory elements. Sports Med. 40, 1037–1053. doi: 10.2165/11536910-000000000-00000
Whon, T. W., Shin, N.-R., Kim, J. Y., and Roh, S. W. (2021). Omics in gut microbiome analysis. J. Microbiol. 59, 292–297. doi: 10.1007/s12275-021-1004-0
Zeng, X., Yang, J., Hu, O., Huang, J., Ran, L., Chen, M., et al. (2019). Dihydromyricetin ameliorates nonalcoholic fatty liver disease by improving mitochondrial respiratory capacity and redox homeostasis through modulation of SIRT3 signaling. Antioxid. Redox Signal. 30, 163–183. doi: 10.1089/ars.2017.7172
Zhang, L., Tian, G., Huang, L., Zhou, M., Zhu, J., Yi, L., et al. (2022). Pterostilbene attenuates intestinal epithelial barrier loss induced by high loading intensity of exercise. Front. Nutr. 9:965180. doi: 10.3389/fnut.2022.965180
Keywords: exercise, gut microbiota, testosterone, skeletal muscle, grip strength
Citation: Zhang L, Lang H, Ran L, Tian G, Shen H, Zhu J, Zhang Q, Yi L and Mi M (2022) Long-term high loading intensity of aerobic exercise improves skeletal muscle performance via the gut microbiota-testosterone axis. Front. Microbiol. 13:1049469. doi: 10.3389/fmicb.2022.1049469
Edited by:
Hu T. Huang, McKesson, United StatesReviewed by:
Yuji Naito, Kyoto Prefectural University of Medicine, JapanWei Yang, Huazhong University of Science and Technology, China
Copyright © 2022 Zhang, Lang, Ran, Tian, Shen, Zhu, Zhang, Yi and Mi. This is an open-access article distributed under the terms of the Creative Commons Attribution License (CC BY). The use, distribution or reproduction in other forums is permitted, provided the original author(s) and the copyright owner(s) are credited and that the original publication in this journal is cited, in accordance with accepted academic practice. No use, distribution or reproduction is permitted which does not comply with these terms.
*Correspondence: Long Yi, longgyin8341@hotmail.com; Mantian Mi, mi_mantian@sina.com