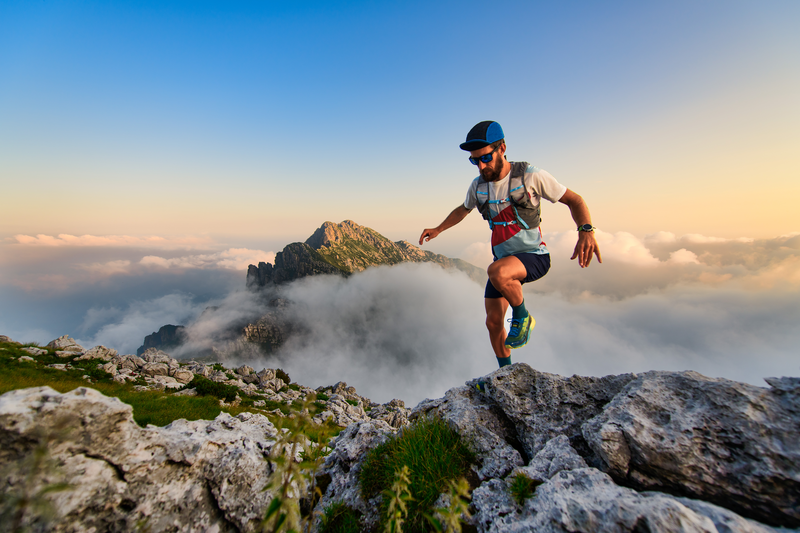
95% of researchers rate our articles as excellent or good
Learn more about the work of our research integrity team to safeguard the quality of each article we publish.
Find out more
REVIEW article
Front. Microbiol. , 13 December 2022
Sec. Food Microbiology
Volume 13 - 2022 | https://doi.org/10.3389/fmicb.2022.1049391
This article is part of the Research Topic Food Pathogens and Antimicrobial Resistance View all 11 articles
Although most Escherichia coli (E. coli) strains are commensal and abundant, certain pathogenic strains cause severe diseases from gastroenteritis to extraintestinal infections. Extraintestinal pathogenic E. coli (ExPEC) contains newborn meningitis E. coli (NMEC), uropathogenic E. coli (UPEC), avian pathogenic E. coli (APEC), and septicemic E. coli (SEPEC) based on their original host and clinical symptom. APEC is a heterogeneous group derived from human ExPEC. APEC causes severe respiratory and systemic diseases in a variety of avians, threatening the poultry industries, food security, and avian welfare worldwide. APEC has many serotypes, and it is a widespread pathogenic bacterium in poultry. In addition, ExPEC strains share significant genetic similarities and similar pathogenic mechanisms, indicating that APEC potentially serves as a reservoir of virulence and resistance genes for human ExPEC, and the virulence and resistance genes can be transferred to humans through food animals. Due to economic losses, drug resistance, and zoonotic potential, APEC has attracted heightened awareness. Various virulence factors and resistance genes involved in APEC pathogenesis and drug resistance have been identified. Here, we review the characteristics, epidemiology, pathogenic mechanism zoonotic potential, and drug resistance of APEC, and summarize the current status of diagnosis, alternative control measures, and vaccine development, which may help to have a better understanding of the pathogenesis and resistance of APEC, thereby reducing economic losses and preventing the spread of multidrug-resistant APEC to humans.
Escherichia coli (E. coli) colonizes the gastrointestinal tract and other mucosal surfaces of a variety of animals (Hill and Drasar, 1975). Although most E. coli strains are commensal and abundant, certain pathogenic strains can cause severe diseases from gastroenteritis to extraintestinal infections that affect health worldwide (Russo and Johnson, 2003). According to the anamnestic clinical reports and virulence features, pathogenic E. coli have been classified as either intestinal pathogenic E. coli (IPEC) or extraintestinal pathogenic E. coli (ExPEC) (Russo and Johnson, 2003). Six diarrhoeagenic pathovars of IPEC have been extensively studied. ExPEC contains newborn meningitis E. coli (NMEC), uropathogenic E. coli (UPEC), avian pathogenic E. coli (APEC), or septicemic E. coli (SEPEC) based on their original host and clinical symptoms. Among these typical infections caused by ExPEC in humans are urinary tract infections (UTIs) and neonatal meningitis (Russo and Johnson, 2000). Similarly, APEC is mostly associated with respiratory tract or systemic infections and results in a variety of diseases in chickens, ducks, and other avian species worldwide, which are economically devastating to poultry industries. There is an increasing risk of ExPEC due to its abundance and multidrug resistance (Antão et al., 2008).
More and more shreds of evidence indicated that ExPEC strains involved in animal and human infections have a highly similar range of phylogenetic and pathogenic mechanisms (Moulin-Schouleur et al., 2007; Reid et al., 2019). Moreover, the whole-genome sequencing of E. coli strains indicated that the genomic level of human ExPEC strains is clustered with avian isolates. Some E. coli strains could acquire a combination of mobile genetic elements via a horizontal exchange, to become a highly adapted pathogen capable of survival and causing a range of diseases in humans and animals (Shames et al., 2009). Thus, the APEC might be virulence genes and antibiotic-resistant genes reservoir for human ExPEC strains. It is necessary to consider the zoonotic potential of APEC (Manges and Johnson, 2012). Although the pathogenic mechanisms of APEC have not yet been completely elucidated, insights into virulence factors of APEC are increasing, which helps to develop novel strategies for controlling APEC infections. In this review, we highlight the recent advances in zoonoses’ potential characteristics, antibiotic resistance, and control strategies of APEC to provide guidance for the prevention and control of avian colibacillosis.
Avian colibacillosis is an assembly of many extraintestinal infections in chickens and other birds with APEC as etiological agents (Barbieri et al., 2015). APEC is susceptible to inducing localized and systemic types of colibacillosis with two important infection stages. The primary stage of colibacillosis was identified as infections of the reproductive tract, omphalitis, and yolk sac. Salpingitis-peritonitis-salpingoperitonitis syndrome (SPS) causes reproductive tract infections with multiple and specific symptoms (Johnson et al., 2008). Omphalitis and yolk sac infection, which are caused by fecal contamination of eggs or egg formation, affect chicks with high mortality in poultry (Matthijs et al., 2009). APEC, in the secondary colibacillosis infections stage, assures an important role in bone and joint infections affecting poultry flocks (Nolan et al., 2003). Among the several types of colibacillosis, colisepticemia was identified as the most important systemic form. Colisepticemia occurs in birds under stress and weak immunosystems through the degradation of certain biotic and abiotic factors mostly high humidity, excess temperature, high dust, viral infections, and vaccines or virulent infectious bronchitis virus in the poultry (Matthijs et al., 2009).
Avian pathogenic E. coli has been known and reported as a principal etiologic agent of avian colibacillosis, responsible for significant morbidity and mortality, with resultant serious economic losses to the poultry industry in the world (Ronco et al., 2017). According to its impact worldwide, serotyping was the most approved method used frequently to estimate the pathogenic potential of APEC strains. E. coli strains belonging to somatic (O), capsular (K), and flagellar (H) antigens. The specific O-serotypes have close correlations with pathogenic E. coli strains (Kauffmann, 1947). Previous studies indicated that O78, O1, and O2 were the predominant serotypes of APEC, whereas, there are different prevalent serotypes in diverse countries according to geographic distribution (Ewers et al., 2004). A recent report that O145 may be emerging as a predominant serogroup of APEC in China (Wang et al., 2022). In addition, the E. coli strain could be assigned to one of the main phylo-groups (A, B1, B2, and D) (Clermont et al., 2000). Significantly, strains responsible for extraintestinal infection were far more likely to be members of phylo-groups B2 or D than A or B1 (Johnson and Stell, 2000). The ExPEC, including APEC strains, mainly belong to the phylogroup B2 and a lesser extent to group D (Smith et al., 2007).
Colibacillosis is an important part of a respiratory infection that evolves to generalize infection resulting in fibrinopurulent lesions of internal organs (Kathayat et al., 2021). Pathogenic bacteria use many strategies to sustain themselves and overcome host barriers with the adhesion of the microorganism to host cells (Mellata, 2013). Colonization is a common step in the pathogenesis of pathogenic bacteria through the ability to adhere to the host surfaces and the successful replication in the respiratory tract (Mellata, 2013). APEC enters through the respiratory tract and uses adhesins to attach to the epithelial cells, followed by survival, invasion, and replication via the presence of the invasins and complementary defense mechanisms (Figure 1). Then, APEC enters the bloodstream, disseminates through the vital organs, such as the lung, heart, liver, and brain, and causes significant damage and lesions (Pourbakhsh et al., 1997). Finally, APEC leads the host to death or induces illness. The resistance to phagocytosis may be an important mechanism in the development of colisepticemia when a strong correlation was observed between pathogenicity for chickens in vivo and the ability to resist the bactericidal effects of chicken macrophages in vitro (Dho-Moulin and Morris Fairbrother, 1999).
Figure 1. The pathogenic mechanism of APEC. The schematic illustrates the APEC infection progress in avians, such as chickens or ducks.
During the process of APEC infection, various virulence factors, including adhesins, iron acquisition systems, protectins, toxins, invasins, metabolism, and secretion systems (Table 1), play important roles in the colonization and survival of APEC (Li et al., 2010).
Adhesins are a cell-surface system of bacteria that adhere to the epithelial cells during the initial stages of APEC infections (Kalita et al., 2014). Initial bacterial attachment or adhesion to host cells is vital to bacterial pathogenesis and is determined by various adhesins. There are many adhesins in APEC, including type 1 fimbriae, P fimbriae, S fimbriae, flagella, curli, outer membrane protein, non-fimbrial and atypical adhesins, and temperature-sensitive hemagglutinin (Aleksandrowicz et al., 2021). Type 1 fimbriae facilitate the adherence to epithelial cells of the respiratory tract during the primary stage (Ewers et al., 2007), whereas the expression of P fimbriae and S fimbriae contribute to the late infection. The application of normal anti-type 1 fimbriae serum and D-mannose, which are the cellular receptors of the adhesin of type 1 fimbriae, could block the specific adherence of APEC strains to chicken tracheal sections (Gyimah and Panigrahy, 1988). Curli induces resistance of APEC in the host’s cecum and facilitates the bacterial invasion of the whole cells (Gophna et al., 2001). Temperature-sensitive hemagglutinin (Tsh) intervenes in the colonization in the first stage of respiratory tract infection (Kostakioti and Stathopoulos, 2004).
Iron acquisitions have a large operating system and contribute to the growth and proliferation of APEC in the host (Rodriguez-Siek et al., 2005b). Multiple siderophores, including aerobactin, salmochelin, yersiniabactin, and transporters to sequester iron from the body fluids, were reported in the APEC. In APEC, the proportion of these operons is increased and located on large plasmids (Johnson et al., 2006). APEC strains have an important frequency of aerobactin iron acquisition system compare to non-pathogenic strains (Dozois et al., 1994).
Protectins are structural factors, including the K1 capsule, lipopolysaccharide (LPS), and certain outer membrane proteins (OMPs), which protect bacteria from the host immune system under stress conditions (Mellata et al., 2003). The capsule is an important virulence factor for the spread of APEC in the bloodstream and septicemia (Biran and Ron, 2018). Furthermore, the iss gene present in the ColV plasmid plays an important role in the bacteria serum survival. In addition, protectins also mediate APEC adhesion, invasion, intracellular survival, colonization, and proliferation in the host (Huja et al., 2015).
Toxins are biologically harmful substances that intervene in the bacterial ability to invade and cause damage to the tissues. APEC produces a low quantity of toxins, including vacuolating autotransporter toxin (Vat), cytotoxic necrotizing factor 1 (CNF1), and various hemolysins (Rodriguez-Siek et al., 2005a). These toxins play role in colonization, motility, biofilm formation, agglutination, and induction of vacuolization. Vat causes cytotoxic effects in cultured cells and attenuates virulence when its deleted (Parreira and Gyles, 2003).
Invasins facilitate the entrance of APEC into the host cells during the infection. Several genes encoding invasins, ibeA, ibeB, and gimB have been identified in NMEC, which contributed to the invasion of the brain microvascular endothelial cells (BMECs) (Germon et al., 2005). These invasins were present in APEC and contributed to resistance to oxidative stress, biofilm formation, colonization, and proliferation in the host (Germon et al., 2005; Wang et al., 2012).
Secretion systems are needle-like structures used to secrete effector proteins, which contribute to bacterial survival and virulence (Wang et al., 2016a). Among the different bacterial secretion systems, two important secretion systems (types III and VI) were identified in APEC (Ma et al., 2014). Type III secretion system 2 (ETT2) is found frequently in pathogenic E. coli strains. The O1, O2, and O78 serotypes of APEC were identified as possessing important elements of ETT2 (Wang et al., 2016a). The intact and degenerate forms are identified in the O1, O2, and O78 serotypes (Wang et al., 2016b). However, the degenerative form of ETT2 may contribute to reducing the virulence and serum survival activity in bacteria (Wang et al., 2016a). Multiple components of ETT2 are involved in the pathogenicity of APEC (Wang et al., 2016a; Fu et al., 2021; Tu et al., 2021; Li et al., 2022; Xue et al., 2022; Yin et al., 2022). Our research shows that the transcriptional regulator DctR can regulate the expression of ETT2 and affect the virulence and pathogenicity of APEC (Zhang et al., 2021). In all, ETT2 has been discovered in APEC isolates and plays significant roles in bacterial virulence, adhesion, colonization, intracellular survival, serum bactericidal activity, and the downregulation of pro-inflammatory cytokine responses. The type VI secretion system (T6SS) is one of the recent nanomachine secretion systems present in Gram-negative pathogens (Yi et al., 2019). Two different forms such as multipurpose T6SS1 and conservative T6SS2 were discovered in APEC isolates. The T6SS1 intervened in the proliferation of APEC during infection, whereas T6SS2 played a role only for cerebral infection (Ma et al., 2014). Overall, the type VI secretion systems are specialized in interbacterial competition, stress sensing, biofilm formation, and virulence (Hachani et al., 2016). In addition, APEC and NMEC have similar T6SS which contributes to binding, and competition by using it to kill neighboring non-immune bacteria and pathogenesis of APEC and NMEC (Ma J. et al., 2018).
Two-component systems (TCSs) are signaling proteins that play important roles in modulating bacterial fitness in different niches. Different TCSs such as CpxA/CpxR, BarA/UvrY, RstA/RstB, and PhoB/PhoR have been identified in APEC isolates (Tu et al., 2016). The CpxA/CpxR regulates surface structure assembly and stress response system implicated in APEC. In addition, CpxA/CpxR positively controls the expression of the APEC type VI secretion system 2 (Yi et al., 2019). BarA/UvrY regulates virulence properties in APEC through the adhesion, invasion, persistence, intracellular survival, resistance to serum bactericidal activity and oxidative stress, and regulation of exopolysaccharide production and expression of type 1 and P fimbriae (Palaniyandi et al., 2012). The RstA/RstB is a nitrogen metabolism TCS that contributes to iron acquisition, acid resistance, intracellular survival, and colonization (Gao et al., 2015b). The PhoB/PhoR is present in many bacterial species that respond to external phosphate concentrations and intervene in biofilm formation, motility, adhesion, invasion, and systemic dissemination (Bertrand et al., 2010).
Several studies have shown the phylogenic similarity between APEC and human ExPEC isolates. According to phylogenetic classification, APEC isolates share significant genetic similarities with human ExPEC (Johnson et al., 2007). ExPEC (APEC, UPEC, and NMEC) share the same virulence factors and similar pathogenic mechanisms, and it may be spread between animals and humans (Johnson et al., 2008). The report demonstrated that ExPEC strains including APEC derived from specific STs may have a high zoonotic impact on humans (Johnson et al., 2008). Several specific virulence genes of the APEC strain, detected in UPEC plasmids, were shown susceptible to increasing the bacteria process to get iron in deficiency conditions (Ewers et al., 2007). Virulence genes that operate in ColV plasmids have similar functions in APEC and UPEC strains (Johnson et al., 2008). Moreover, APEC may induce high urinary infections in mice similar to UPEC and meningitis in rats similar to NMEC (Johnson et al., 2010). In addition, the ExPEC was recognized as a potential causal agent in women’s health, newborns, elderly, and immunocompromised individuals in fact of an important number of urinary tract infections (UTIs), newborn meningitis, abdominal sepsis, and septicemia (Mellata, 2013).
Poultry is one of the most widely consumed meats in the world. APEC are causative agents of colibacillosis, one of the principal causes of morbidity and mortality in poultry worldwide (Nhung et al., 2017). Since poultry is usually raised under intensive conditions, infection transmission is favored, and the animals are more susceptible to diseases. So using large quantities of antimicrobials to prevent and treat disease, if overused or misused, lead to the evolution of bacteria and the rise of drug-resistant pathogens in the long term (Rahman et al., 2022). It is precisely due to the extensive use of antibiotics that APEC is severely resistant. Antibiotic-resistant APEC can not only lead to treatment failure, resulting in economic losses, but also be a source of resistant bacteria/genes that may represent a risk to human health (Nhung et al., 2017; Kim et al., 2021).
Avian pathogenic E. coli already carries many resistance genes and resistance to a lot of important antibiotics around the world. Colistin resistance of 2.2% was detected in isolates in Senegal, and colistin resistance of 8.7% was detected in isolates in Vietnam (Vounba et al., 2019). Particularly, there was a higher prevalence of mcr-1 in isolates from chicken in Vietnam (53.2%), and the mcr-1 gene was detected in 85% of 13 phenotypically colistin-resistant isolates (Vounba et al., 2019; Le et al., 2021). In addition, all colistin-resistant isolates exhibited multidrug-resistant phenotypes (Vounba et al., 2019). In Jordan, APEC resistance rates of sulfamethoxazole–trimethoprim, florfenicol, amoxicillin, doxycycline, and spectinomycin were 95.5, 93.7 93.3, 92.2, and 92.2%, respectively. At least five antibiotic-resistance genes were found in 68% of APEC isolates. The most important genes were int1 97%, tetA 78.4%, blaTEM 72.9%, Sul1 72.4%, and Sul2 70.2%; these resistance genes are detected in human pathogens (Ibrahim et al., 2019). Under commercial conditions in Portugal, the overall 10-year antibiotic resistance of APEC strains is amoxicillin 78%, ampicillin 73.5%, tetracycline 63.3%, doxycycline 56.4%, apramycin 34.5%, neomycin 68.2%, flumequine 39.4%, cotrimoxazole 47.7%, florfenicol 46.6%, and lincospectin 66.3% (Oliveira et al., 2022). In China, the prevalence of extended-spectrum cephalosporin-resistant strains in E. coli from chicken colibacillosis and raw meat separately accounted for 66.1% and 71.2% (Wang et al., 2021).
Previous studies indicated that APEC strains found in poultry are shown to be important reservoirs for antibiotic resistance genes (Nandi et al., 2004). Antibiotic resistance occurs with high complexity in presence of resistance-encoding genes that are found inside plasmids or chromosomal genetic material (Ibrahim et al., 2019). The antibiotic resistance genes are identified on mobile genetics elements enabling their rapid transfer among the ExPEC strains. Furthermore, animal reservoirs may be responsible for human contamination or transfer of APEC antibiotic-resistant and other commensal bacteria through the contaminated food in poultry (Hannah et al., 2009). Investigations from the poultry farm revealed the use of multiple antibiotics may present significant resistance among E. coli (Johar et al., 2021). The mcr-1 gene, found in APEC, exhibited colistin resistance, demonstrating its role in colistin resistance (Eltai et al., 2018). In addition, APEC carrying the mcr-1 gene was isolated from septicemic chickens, which may increase the difficulty of prevention and control of poultry septicemia (Ewers et al., 2016). The research has shown that β-lactamase CTX-M, OXA, CMY, and TEM genes were widespread in chicken-source E. coli, and blaCTX-M was the most predominant ESBL gene (Wang et al., 2021). The studies identify resistance genes such as floR, cmlA, cat1, cat2, tetA, tetB, tetC, tetD, tetE, tetG, sul1, sul2, addA1, and addA2 among APEC isolates (Li et al., 2007; Shin et al., 2015; Ibrahim et al., 2019). The presence of the resistance gene in isolates from poultry and marketed retail meats further complicates the APEC antibiotic resistance situation and is a possible health risk for humans.
Colibacillosis infections are suspected to focus on the clinical signs and the presence of typical macroscopic lesions (Gomis et al., 1997). The laboratory diagnosis must be confirmed in the presence of such clinical signs and lesions, including traditional bacterial isolation, virulence gene detection, and serotyping. The diagnosis occurs in different regions of an infected animal such as cardiac blood and affected tissues, liver, spleen, and pericardium. Samples of the lesion were collected and prepared. Selective media like McConkey, eosinmethylene blue, or drigalki agar are used for isolation. The antigenic identification and virulence genes of isolated strains were detected by PCR; specific antigen and virulence gene detection is beneficial to identify APEC (Antão et al., 2009). Traditional biochemical reactions and ELISA methods for APEC identification are costly and time-consuming. Therefore, the PCR method has been found to be a fast and effective common technique for APEC detection (Wang et al., 2014; Lucas et al., 2022).
While some families of antibiotics used as a treatment in poultry such as tetracyclines, penicillins, and aminoglycosides are also commonly administered to humans to treat bacterial infections. Therefore, the drug resistance of APEC will threaten the choice of drugs when human beings are infected with bacteria. Other multitude methods such as biosecurity measures and vaccination are also necessary for the prevention and control of infections (Wang et al., 2017).
Effective prevention and control of APEC infections depend on the identification and elimination of predisposing causes of the disease. Maintaining flock biosecurity is difficult to control and prevent (Dziva and Stevens, 2008). The main objective is to reduce the level of APEC exposure by improving biosecurity, good litter, and ventilation conditions in poultry (Dziva and Stevens, 2008). The sanitation of the environmental system should be improved. Furthermore, the reducing of fecal contamination of eggs, cleaning nest boxes, and decreasing the number of floor eggs contribute to reducing the incidence risk of colibacillosis infections (Dziva and Stevens, 2008). The research shows that vitamin E has been able to interfere with bacterial biofilm and prevent in vitro biofilm formation (Vergalito et al., 2018). It is possible to increase the level of vitamin E in the nutrition system to prevent APEC infection.
Generally, antibiotics are wildly used to prevent and treat APEC infections. The application of these antibiotics was reported to accelerate the emergence of multidrug-resistant bacteria (Rahman et al., 2022). Seriously, APEC’s high levels of resistance to important antibiotics may pose a high risk to humans, because antibiotic-resistant bacteria and genes can be transmitted through the food chain to humans. Previous studies demonstrated that APEC isolates were resistant to multiple antibiotics. Thus, it is crucial and helpful to perform antibiotic susceptibility testing for the appropriate antibiotic in the treatment of avian colibacillosis (Bass et al., 1999).
Avian pathogenic E. coli infections of poultry result in significant morbidity and mortality with important economic losses. Therefore, many efforts have been made to develop effective vaccines, including inactivated vaccines, subunit vaccines, and live attenuated vaccines against APEC infections (Nesta and Pizza, 2018). Table 2 shows the of vaccines development against APEC infection with their main findings.
Inactivated vaccines were developed earlier to provide the effectiveness of vaccines against homologous and heterologous challenges (Gross, 1957). These vaccines were made from inactivated predominant APEC serotype strains to control colibacillosis. The inactivated vaccines were observed to provide efficacy protection against only homologous challenges (Deb and Harry, 1978). The efficacy of the inactivated vaccines is determined by diverse parameters such as the serotypes of E. coli include in the vaccine, the administration methods, age of the birds, and the dose of vaccine administered to the birds (Russo et al., 2003).
Subunit vaccines were produced to overcome the limit of inactivated vaccines, which were unable to protect chickens against the heterologous challenge (Bolin and Jensen, 1987). Several recombinant subunit antigens intervene to produce subunit vaccines for successful protection against heterologous challenges (Bolin and Jensen, 1987). The recombinant subunit vaccines generate strong antibody responses in recipient birds when administered parenterally with adjuvant-containing formulations (Kariyawasam et al., 2002). Subunit vaccines may provide broader protection to more serotypes of APEC. The understanding of APEC genome sequences and pathogenic virulence genes may contribute to the development of more new subunit vaccines (Ghunaim et al., 2014).
Live vaccines are available for numerous viral, bacterial, and coccidial organisms. Live vaccines are effective and relatively economical (Frommer et al., 1994). The most successful APEC live attenuated vaccine is the aroA gene mutant vaccine. The live Poulvac® E. coli (Zoetis) vaccine includes an aroA mutant of a strain of serotype O78:K80 and ST23, and the aroA mutation attenuates the virulence of the strain and results in a requirement for aromatic amino acids, which results in reduced survival of the strain in the chicken and the environment (La Ragione et al., 2013; Han et al., 2015). The live vaccines are generally short-lived after first or initial exposure to the immunity system. These vaccines generally reduced the systemic lesions by mass administered drinking water, spray, and oral (Barbieri et al., 2012). Even though the live attenuated vaccine provides clinical protection against the challenge, it is not able to prevent completely pathological lesions (El-Mawgoud et al., 2020). Live vaccines may induce a high risk of reversion to natural virulence via back-mutations of the attenuated organism and susceptible to causing symptomatic affection (Ghunaim et al., 2014).
After evaluating the efficacy of inactivated vaccines, subunit vaccines, and live attenuated vaccines, various recombinant vaccines have been investigated to protect chickens against APEC infections (Ghunaim et al., 2014). Within the tested vaccines, multiple vaccines such as outer membrane vesicles (OMVs), bacterial ghost (BG) vaccines, Salmonella-delivered vaccines containing APEC antigens, such as FimA and OmpC, were able to reduce the mortality and morbidity, APEC lesions as well as stimulate the antibody (immunoglobulins; IgG and IgA) responses in immunized chickens (Ebrahimi-Nik et al., 2018). In our previous study, the BG vaccine was able to achieve over 90% immune protection against virulent challenges using the same serotype O2 strain, while it showed poor cross-protection against serotypes O1 and O78 (Hu et al., 2019). Further research is needed to provide cross-protection rates between serotypes.
Avian pathogenic E. coli is considered responsible for multi-factorial illness and causes significant economic losses in the poultry industry over the world. APEC antibiotic resistance is serious, which increases the opportunity to transmit antibiotic resistance genes from APEC to human pathogens. Investigations are necessary to provide concrete evidence for the zoonotic transmission of APEC to humans. However, we highlight the crucial roles played by the different virulence factors; further investigations and studies are suggested to understand the contribution of virulence factors in APEC virulence. We should continue the efforts to identify more potential virulence factors and reveal the pathogenic mechanism, thus helping to develop a novel diagnosis method and vaccines to control avian colibacillosis. The virulence factors and drug resistance genes of APEC can be prevented from being transmitted to humans through food animals and endangering human health.
JH, DA, and SW: conceptualization and original draft writing—review and editing. BZ, HZ, LY, WG, XW, ZW, DW, HP, MT, and JQ: helped in revising. All authors have read and agreed to the published version of the manuscript.
This research was supported by the National Key Research and Development Program of China (2021YFD1800402), the National Natural Science Foundation of China (31972654 and 32172856), and the Scientific and Technical Innovation Project of the Chinese Academy of Agricultural Sciences.
The authors declare that the research was conducted in the absence of any commercial or financial relationships that could be construed as a potential conflict of interest.
All claims expressed in this article are solely those of the authors and do not necessarily represent those of their affiliated organizations, or those of the publisher, the editors and the reviewers. Any product that may be evaluated in this article, or claim that may be made by its manufacturer, is not guaranteed or endorsed by the publisher.
Aleksandrowicz, A., Khan, M. M., Sidorczuk, K., Noszka, M., and Kolenda, R. (2021). Whatever makes them stick - Adhesins of avian pathogenic Escherichia coli. Vet. Microbiol. 257:109095. doi: 10.1016/j.vetmic.2021.109095
Antão, E. M., Ewers, C., Gürlebeck, D., Preisinger, R., Homeier, T., Li, G., et al. (2009). Signature-tagged mutagenesis in a chicken infection model leads to the identification of a novel avian pathogenic Escherichia coli fimbrial adhesin. PLoS One 4:e7796. doi: 10.1371/journal.pone.0007796
Antão, E. M., Glodde, S., Li, G., Sharifi, R., Homeier, T., Laturnus, C., et al. (2008). The chicken is a natural model for extraintestinal infections caused by avian pathogenic Escherichia coli (APEC). Microb. Pathog. 45, 361–369. doi: 10.1016/j.micpath.2008.08.005
Azeem, T., Abid, S. A., Ahmad, W., Aslam, A., Sohail, M. L., Jaleel, S., et al. (2017). Host immune responses and vaccination against avian pathogenic Escherichia coli - RETRACTED. World’s Poult Sci J. 73, 29–44. doi: 10.1017/S0043933916000866
Bao, Y., Zhai, Z., Wang, S., Ma, J., Zhang, W., and Lu, C. (2013). Chaperonin GroEL: a novel phylogenetically conserved protein with strong immunoreactivity of Avian Pathogenic Escherichia coli isolates from duck identified by immunoproteomics. Vaccine 31, 2947–2953. doi: 10.1016/j.vaccine.2013.04.042
Barbieri, N. L., de Oliveira, A. L., Tejkowski, T. M., Pavanelo, D. B., Matter, L. B., Pinheiro, S. R. S., et al. (2015). Molecular characterization and clonal relationships among Escherichia coli strains isolated from broiler chickens with colisepticemia. Foodborne Pathog. Dis. 12, 74–83. doi: 10.1089/fpd.2014.1815
Barbieri, N. L., Tejkowski, T. M., de Oliveira, A. L., de Brito, B. G., and Horn, F. (2012). Characterization of extraintestinal Escherichia coli isolated from a peacock (Pavo cristatus) with colisepticemia. Avian Dis. 56, 436–440. doi: 10.1637/9921-090811-Case.1
Bass, L., Liebert, C. A., Lee, M. D., Summers, A. O., White, D. G., Thayer, S. G., et al. (1999). Incidence and characterization of integrons, genetic elements mediating multiple-drug resistance, in avian Escherichia coli. Antimicrob. Agents Chemother. 43, 2925–2929. doi: 10.1128/AAC.43.12.2925
Bertrand, N., Houle, S., LeBihan, G., Poirier, É, Dozois, C. M., and Harel, J. (2010). Increased Pho regulon activation correlates with decreased virulence of an avian pathogenic Escherichia coli O78 strain. Infect Immun. 78, 5324–5331. doi: 10.1128/IAI.00452-10
Biran, D., and Ron, E. Z. (2018). Extraintestinal Pathogenic Escherichia coli. Curr. Top Microbiol. Immunol. 416, 149–161. doi: 10.1007/82_2018_108
Bolin, C. A., and Jensen, A. E. (1987). Passive immunization with antibodies against iron-regulated outer membrane proteins protects turkeys from Escherichia coli septicemia. Infect Immun. 55, 1239–1242. doi: 10.1128/iai.55.5.1239-1242.1987
Caza, M., Lépine, F., Milot, S., and Dozois, C. M. (2008). Specific roles of the iroBCDEN genes in virulence of an avian pathogenic Escherichia coli O78 strain and in production of salmochelins. Infect Immun. 76, 3539–3549. doi: 10.1128/IAI.00455-08
Clermont, O., Bonacorsi, S., and Bingen, E. (2000). Rapid and simple determination of the Escherichia coli phylogenetic group. Appl. Environ. Microbiol. 66, 4555–4558. doi: 10.1128/AEM.66.10.4555-4558.2000
Cortes, M. A., Gibon, J., Chanteloup, N. K., Moulin-Schouleur, M., Gilot, P., and Germon, P. (2008). Inactivation of ibeA and ibeT results in decreased expression of type 1 fimbriae in extraintestinal pathogenic Escherichia coli strain BEN2908. Infect Immun. 76, 4129–4136. doi: 10.1128/iai.00334-08
Deb, J. R., and Harry, E. G. (1976). Laboratory trials with inactivated vaccines against Escherichia coli (O78 K80) infection in fowls. Res. Vet. Sci. 20, 131–138.
Deb, J. R., and Harry, E. G. (1978). Laboratory trials with inactivated vaccines against Escherichia coli (O2:K1) infection in fowls. Res. Vet. Sci. 24, 308–313.
Dho-Moulin, M., and Morris Fairbrother, J. (1999). Avian pathogenic Escherichia coli (APEC). Vet. Res. 30, 299–316.
Dozois, C. M., Chanteloup, N., Dho-Moulin, M., Brée, A., Desautels, C., and Fairbrother, J. M. (1994). Bacterial colonization and in vivo expression of F1 (type 1) fimbrial antigens in chickens experimentally infected with pathogenic Escherichia coli. Avian Dis. 38, 231–239.
Dziva, F., Hauser, H., Connor, T. R., van Diemen, P. M., Prescott, G., Langridge, G. C., et al. (2013). Sequencing and functional annotation of avian pathogenic Escherichia coli serogroup O78 strains reveal the evolution of E. coli lineages pathogenic for poultry via distinct mechanisms. Infect Immun. 81, 838–849. doi: 10.1128/IAI.00585-12
Dziva, F., and Stevens, M. P. (2008). Colibacillosis in poultry: unravelling the molecular basis of virulence of avian pathogenic Escherichia coli in their natural hosts. Avian Pathol. 37, 355–366. doi: 10.1080/03079450802216652
Ebrahimi-Nik, H., Bassami, M. R., Mohri, M., Rad, M., and Khan, M. I. (2018). Bacterial ghost of avian pathogenic E. coli (APEC) serotype O78:K80 as a homologous vaccine against avian colibacillosis. PLoS One 13:e0194888. doi: 10.1371/journal.pone.0194888
El-Mawgoud, A. I. A., El-Nahass, E. S., Shany, S. A. S., El-Sawah, A. A., Dahshan, A. M., Nasef, S. A., et al. (2020). Efficacy of live attenuated vaccine and commercially available lectin against avian pathogenic E. coli infection in broiler chickens. Vet. Sci. 7:65. doi: 10.3390/vetsci7020065
Eltai, N. O., Abdfarag, E. A., Al-Romaihi, H., Wehedy, E., Mahmoud, M. H., Alawad, O. K., et al. (2018). Antibiotic resistance profile of commensal Escherichia coli isolated from broiler chickens in Qatar. J. Food Prot. 81, 302–307. doi: 10.4315/0362-028x.jfp-17-191
Ewers, C., Göttig, S., Bülte, M., Fiedler, S., Tietgen, M., Leidner, U., et al. (2016). Genome Sequence of Avian Escherichia coli Strain IHIT25637, an Extraintestinal Pathogenic E. coli Strain of ST131 Encoding Colistin Resistance Determinant MCR-1. Genome Announc. 4, e863–e816. doi: 10.1128/genomeA.00863-16
Ewers, C., Janssen, T., Kiessling, S., Philipp, H. C., and Wieler, L. H. (2004). Molecular epidemiology of avian pathogenic Escherichia coli (APEC) isolated from colisepticemia in poultry. Vet Microbiol. 104, 91–101. doi: 10.1016/j.vetmic.2004.09.008
Ewers, C., Li, G., Wilking, H., Kiessling, S., Alt, K., Antáo, E.-M., et al. (2007). Avian pathogenic, uropathogenic, and newborn meningitis-causing Escherichia coli: how closely related are they? Int. J. Med. Microbiol. 297, 163–176. doi: 10.1016/j.ijmm.2007.01.003
Frommer, A., Freidlin, P. J., Bock, R. R., Leitner, G., Chaffer, M., and Heller, E. D. (1994). Experimental vaccination of young chickens with a live, non-pathogenic strain of Escherichia coli. Avian Pathol. 23, 425–433. doi: 10.1080/03079459408419013
Fu, D., Li, J., Wu, J., Shao, Y., Song, X., Tu, J., et al. (2021). The ETT2 transcriptional regulator EivF affects the serum resistance and pathogenicity of avian pathogenic Escherichia coli. Microb. Pathog. 161(Pt A):105261. doi: 10.1016/j.micpath.2021.105261
Gao, Q., Jia, X., Wang, X., Xiong, L., Gao, S., and Liu, X. (2015a). The avian pathogenic Escherichia coli O2 strain E058 carrying the defined aerobactin-defective iucD or iucDiutA mutation is less virulent in the chicken. Infect. Genet. Evol. 30, 267–277. doi: 10.1016/j.meegid.2014.12.038
Gao, Q., Ye, Z., Wang, X., Mu, X., Gao, S., and Liu, X. (2015b). RstA is required for the virulence of an avian pathogenic Escherichia coli O2 strain E058. Infect. Genet. Evol. 29, 180–188. doi: 10.1016/j.meegid.2014.11.022
Germon, P., Chen, Y.-H., He, L., Blanco, J. E., Brée, A., Schouler, C., et al. (2005). ibeA, a virulence factor of avian pathogenic Escherichia coli. Microbiology (Reading). 151(Pt 4), 1179–1186. doi: 10.1099/mic.0.27809-0
Ghunaim, H., Abu-Madi, M. A., and Kariyawasam, S. (2014). Advances in vaccination against avian pathogenic Escherichia coli respiratory disease: potentials and limitations. Vet. Microbiol. 172, 13–22. doi: 10.1016/j.vetmic.2014.04.019
Gomis, S. M., Watts, T., Riddell, C., Potter, A. A., and Allan, B. J. (1997). Experimental reproduction of Escherichia coli cellulitis and septicemia in broiler chickens. Avian Dis. 41, 234–240.
Gophna, U., Barlev, M., Seijffers, R., Oelschlager, T. A., Hacker, J., and Ron, E. Z. (2001). Curli fibers mediate internalization of Escherichia coli by eukaryotic cells. Infect. Immun. 69, 2659–2665. doi: 10.1128/iai.69.4.2659-2665.2001
Gross, W. B. (1957). Pathological changes of an Escherichia coli infection in chickens and turkeys. Am. J. Vet. Res. 18, 724–730.
Gyimah, J. E., and Panigrahy, B. (1985). Immunogenicity of an Escherichia coli (serotype O1) pili vaccine in chickens. Avian Dis. 29, 1078–1083.
Gyimah, J. E., and Panigrahy, B. (1988). Adhesin-receptor interactions mediating the attachment of pathogenic Escherichia coli to chicken tracheal epithelium. Avian Dis. 32, 74–78.
Hachani, A., Wood, T. E., and Filloux, A. (2016). Type VI secretion and anti-host effectors. Curr. Opin. Microbiol. 29, 81–93. doi: 10.1016/j.mib.2015.11.006
Han, X., Bai, H., Tu, J., Yang, L., Xu, D., Wang, S., et al. (2015). Deletion of luxS further attenuates the virulence of the avian pathogenic Escherichia coli aroA mutant. Microb. Pathog. 88, 39–47. doi: 10.1016/j.micpath.2015.08.003
Han, Y., Liu, Q., Willias, S., Liang, K., Li, P., Cheng, A., et al. (2018). A bivalent vaccine derived from attenuated Salmonella expressing O-antigen polysaccharide provides protection against avian pathogenic Escherichia coli O1 and O2 infection. Vaccine 36, 1038–1046. doi: 10.1016/j.vaccine.2018.01.036
Hannah, E. L., Johnson, J. R., Angulo, F., Haddadin, B., Williamson, J., and Samore, M. H. (2009). Molecular analysis of antimicrobial-susceptible and -resistant Escherichia coli from retail meats and human stool and clinical specimens in a rural community setting. Foodborne Pathog. Dis. 6, 285–295. doi: 10.1089/fpd.2008.0176
Herren, C. D., Mitra, A., Palaniyandi, S. K., Coleman, A., Elankumaran, S., and Mukhopadhyay, S. (2006). The BarA-UvrY two-component system regulates virulence in avian pathogenic Escherichia coli O78:K80:H9. Infect. Immun. 74, 4900–4909. doi: 10.1128/IAI.00412-06
Hill, M. J., and Drasar, B. S. (1975). The normal colonic bacterial flora. Gut 16, 318–323. doi: 10.1136/gut.16.4.318
Holden, K. M., Browning, G. F., Noormohammadi, A. H., Markham, P., and Marenda, M. S. (2014). Avian pathogenic Escherichia coli ΔtonB mutants are safe and protective live-attenuated vaccine candidates. Vet Microbiol. 173, 289–298. doi: 10.1016/j.vetmic.2014.07.028
Hu, J., Zuo, J., Chen, Z., Fu, L., Lv, X., Hu, S., et al. (2019). Use of a modified bacterial ghost lysis system for the construction of an inactivated avian pathogenic Escherichia coli vaccine candidate. Vet. Microbiol. 229, 48–58. doi: 10.1016/j.vetmic.2018.12.020
Huja, S., Oren, Y., Trost, E., Brzuszkiewicz, E., Biran, D., Blom, J., et al. (2015). Genomic avenue to avian colisepticemia. mBio 6, e1681–e1614. doi: 10.1128/mBio.01681-14
Ibrahim, R. A., Cryer, T. L., Lafi, S. Q., Basha, E.-A., Good, L., and Tarazi, Y. H. (2019). Identification of Escherichia coli from broiler chickens in Jordan, their antimicrobial resistance, gene characterization and the associated risk factors. BMC Vet. Res. 15:159. doi: 10.1186/s12917-019-1901-1
Johar, A., Al-Thani, N., Al-Hadidi, S. H., Dlissi, E., Mahmoud, M. H., and Eltai, N. O. (2021). Antibiotic Resistance and Virulence Gene Patterns Associated with Avian Pathogenic Escherichia coli (APEC) from Broiler Chickens in Qatar. Antibiotics (Basel) 10:564. doi: 10.3390/antibiotics10050564
Johnson, J. R., and Stell, A. L. (2000). Extended virulence genotypes of Escherichia coli strains from patients with urosepsis in relation to phylogeny and host compromise. J. Infect. Dis. 181, 261–272. doi: 10.1086/315217
Johnson, T. J., Jordan, D., Kariyawasam, S., Stell, A. L., Bell, N. P., Wannemuehler, Y. M., et al. (2010). Sequence analysis and characterization of a transferable hybrid plasmid encoding multidrug resistance and enabling zoonotic potential for extraintestinal Escherichia coli. Infect. Immun. 78, 1931–1942. doi: 10.1128/iai.01174-09
Johnson, T. J., Kariyawasam, S., Wannemuehler, Y., Mangiamele, P., Johnson, S. J., Doetkott, C., et al. (2007). The genome sequence of avian pathogenic Escherichia coli strain O1:K1:H7 shares strong similarities with human extraintestinal pathogenic E. coli genomes. J. Bacteriol. 189, 3228–3236. doi: 10.1128/jb.01726-06
Johnson, T. J., Wannemeuhler, Y. M., Scaccianoce, J. A., Johnson, S. J., and Nolan, L. K. (2006). Complete DNA sequence, comparative genomics, and prevalence of an IncHI2 plasmid occurring among extraintestinal pathogenic Escherichia coli isolates. Antimicrob. Agents Chemother. 50, 3929–3933. doi: 10.1128/aac.00569-06
Johnson, T. J., Wannemuehler, Y., Doetkott, C., Johnson, S. J., Rosenberger, S. C., and Nolan, L. K. (2008). Identification of minimal predictors of avian pathogenic Escherichia coli virulence for use as a rapid diagnostic tool. J. Clin. Microbiol. 46, 3987–3996. doi: 10.1128/JCM.00816-08
Kalita, A., Hu, J., and Torres, A. G. (2014). Recent advances in adherence and invasion of pathogenic Escherichia coli. Curr. Opin. Infect. Dis. 27, 459–464. doi: 10.1097/qco.0000000000000092
Kariyawasam, S., and Nolan, L. K. (2009). Pap mutant of avian pathogenic Escherichia coli O1, an O1:K1:H7 strain, is attenuated in vivo. Avian Dis. 53, 255–260. doi: 10.1637/8519-111708-Reg.1
Kariyawasam, S., Wilkie, B. N., and Gyles, C. L. (2004). Construction, characterization, and evaluation of the vaccine potential of three genetically defined mutants of avian pathogenic Escherichia coli. Avian Dis. 48, 287–299. doi: 10.1637/7093
Kariyawasam, S., Wilkie, B. N., Hunter, D. B., and Gyles, C. L. (2002). Systemic and mucosal antibody responses to selected cell surface antigens of avian pathogenic Escherichia coli in experimentally infected chickens. Avian Dis. 46, 668–678. doi: 10.1637/0005-20862002046[0668:SAMART]2.0.CO;2
Kathayat, D., Lokesh, D., Ranjit, S., and Rajashekara, G. (2021). Avian Pathogenic Escherichia coli (APEC): An overview of virulence and pathogenesis factors, zoonotic potential, and control strategies. Pathogens 10:467. doi: 10.3390/pathogens10040467
Kim, J.-H., Lee, H.-J., Jeong, O.-M., Kim, D.-W., Jeong, J.-Y., Kwon, Y.-K., et al. (2021). High prevalence and variable fitness of fluoroquinolone-resistant avian pathogenic isolated from chickens in Korea. Avian Pathol. 50, 151–160. doi: 10.1080/03079457.2020.1855322
Kostakioti, M., and Stathopoulos, C. (2004). Functional analysis of the Tsh autotransporter from an avian pathogenic Escherichia coli strain. Infect. Immun. 72, 5548–5554. doi: 10.1128/iai.72.10.5548-5554.2004
Kwaga, J. K., Allan, B. J., van der Hurk, J. V., Seida, H., and Potter, A. A. (1994). A carAB mutant of avian pathogenic Escherichia coli serogroup O2 is attenuated and effective as a live oral vaccine against colibacillosis in turkeys. Infect. Immun. 62, 3766–3772. doi: 10.1128/iai.62.9.3766-3772.1994
La Ragione, R. M., Cooley, W. A., and Woodward, M. J. (2000). The role of fimbriae and flagella in the adherence of avian strains of Escherichia coli O78:K80 to tissue culture cells and tracheal and gut explants. J. Med. Microbiol. 49, 327–338. doi: 10.1099/0022-1317-49-4-327
La Ragione, R. M., Woodward, M. J., Kumar, M., Rodenberg, J., Fan, H., Wales, A. D., et al. (2013). Efficacy of a live attenuated Escherichia coli O78:K80 vaccine in chickens and turkeys. Avian Dis. 57, 273–279. doi: 10.1637/10326-081512-Reg.1
Le, P. Q., Awasthi, S. P., Hatanaka, N., Hinenoya, A., Hassan, J., Ombarak, R. A., et al. (2021). Prevalence of mobile colistin resistance (mcr) genes in extended-spectrum β-lactamase-producing Escherichia coli isolated from retail raw foods in Nha Trang, Vietnam. Int. J. Food Microbiol. 346:109164. doi: 10.1016/j.ijfoodmicro.2021.109164
Le Roy, D., Crouzier, C., Dho-Moulin, M., Dumont, A. S., Bouchet, A., Lafont, J. P., et al. (1995). Results of passive and active immunization directed against ferric aerobactin in experimental enterobacterial infections in mice and chickens. Res. Microbiol. 146, 167–174. doi: 10.1016/0923-2508(96)80894-1
Li, G., Feng, Y., Kariyawasam, S., Tivendale, K. A., Wannemuehler, Y., Zhou, F., et al. (2010). AatA is a novel autotransporter and virulence factor of avian pathogenic Escherichia coli. Infect Immun. 78, 898–906. doi: 10.1128/iai.00513-09
Li, Q., Tu, J., Jiang, N., Zheng, Q., Fu, D., Song, X., et al. (2022). Serum resistance factors in avian pathogenic mediated by ETT2 gene cluster. Avian Pathol. 51, 34–44. doi: 10.1080/03079457.2021.1998361
Li, X. S., Wang, G. Q., Du, X. D., Cui, B. A., Zhang, S. M., and Shen, J. Z. (2007). Antimicrobial susceptibility and molecular detection of chloramphenicol and florfenicol resistance among Escherichia coli isolates from diseased chickens. J. Vet. Sci. 8, 243–247. doi: 10.4142/jvs.2007.8.3.243
Lucas, C., Delannoy, S., Schouler, C., Souillard, R., Le Devendec, L., Lucas, P., et al. (2022). Description and validation of a new set of PCR markers predictive of avian pathogenic Escherichia coli virulence. Vet. Microbiol. 273:109530. doi: 10.1016/j.vetmic.2022.109530
Lynne, A. M., Kariyawasam, S., Wannemuehler, Y., Johnson, T. J., Johnson, S. J., Sinha, A. S., et al. (2012). Recombinant Iss as a potential vaccine for avian colibacillosis. Avian Dis. 56, 192–199. doi: 10.1637/9861-072111-Reg.1
Ma, J., Bao, Y., Sun, M., Dong, W., Pan, Z., Zhang, W., et al. (2014). Two functional type VI secretion systems in avian pathogenic Escherichia coli are involved in different pathogenic pathways. Infect Immun. 82, 3867–3879. doi: 10.1128/iai.01769-14
Ma, J., Sun, M., Zihao, P., Song, W., Lu, C., and Yao, H. (2018). Three Hcp homologs with divergent extended loop regions exhibit different functions in avian pathogenic Escherichia coli. Curr. Microbiol. 7:49. doi: 10.1038/s41426-018-0042-0
Ma, S. T., Ding, G. J., Huang, X. W., Wang, Z. W., Wang, L., Yu, M. L., et al. (2018). Immunogenicity in chickens with orally administered recombinant chicken-borne Lactobacillus saerimneri expressing FimA and OmpC antigen of O78 avian pathogenic Escherichia coli. J. Med. Microbiol. 67, 441–451. doi: 10.1099/jmm.0.000679
Manges, A. R., and Johnson, J. R. (2012). Food-borne origins of Escherichia coli causing extraintestinal infections. Clin. Infect. Dis. 55, 712–719. doi: 10.1093/cid/cis502
Matter, L., Spricigo, D., Tasca, C., and Vargas, A. (2015). Invasin gimB found in a bovine intestinal Escherichia coli with an adherent and invasive profile. Braz. J. Microbiol. 46, 875–878. doi: 10.1590/S1517-838246320140621
Matthijs, M. G., Ariaans, M. P., Dwars, R. M., van Eck, J. H., Bouma, A., Stegeman, A., et al. (2009). Course of infection and immune responses in the respiratory tract of IBV infected broilers after superinfection with E. coli. Vet. Immunol. Immunopathol. 127, 77–84. doi: 10.1016/j.vetimm.2008.09.016
Melamed, D., Leitner, G., and Heller, E. D. (1991). A vaccine against avian colibacillosis based on ultrasonic inactivation of Escherichia coli. Avian Dis. 35, 17–22.
Mellata, M. (2013). Human and avian extraintestinal pathogenic Escherichia coli: infections, zoonotic risks, and antibiotic resistance trends. Foodborne Pathog. Dis. 10, 916–932. doi: 10.1089/fpd.2013.1533
Mellata, M., Dho-Moulin, M., Dozois, C. M., Curtiss, R. III, Lehoux, B., and Fairbrother, J. M. (2003). Role of avian pathogenic Escherichia coli virulence factors in bacterial interaction with chicken heterophils and macrophages. Infect Immun. 71, 494–503. doi: 10.1128/iai.71.1.494-503.2003
Moulin-Schouleur, M., Répérant, M., Laurent, S., Brée, A., Mignon-Grasteau, S., Germon, P., et al. (2007). Extraintestinal pathogenic Escherichia coli strains of avian and human origin: link between phylogenetic relationships and common virulence patterns. J. Clin. Microbiol. 45, 3366–3376. doi: 10.1128/JCM.00037-07
Nandi, S., Maurer, J. J., Hofacre, C., and Summers, A. O. (2004). Gram-positive bacteria are a major reservoir of Class 1 antibiotic resistance integrons in poultry litter. Proc. Natl. Acad. Sci. U.S.A. 101, 7118–7122. doi: 10.1073/pnas.0306466101
Nesta, B., and Pizza, M. (2018). Vaccines Against Escherichia coli. Curr. Top Microbiol. Immunol. 416, 213–242. doi: 10.1007/82_2018_111
Nhung, N. T., Chansiripornchai, N., and Carrique-Mas, J. J. (2017). Antimicrobial resistance in bacterial poultry pathogens: A review. Front. Vet Sci. 4:126. doi: 10.3389/fvets.2017.00126
Nolan, L. K., Horne, S. M., Giddings, C. W., Foley, S. L., Johnson, T. J., Lynne, A. M., et al. (2003). Resistance to serum complement, iss, and virulence of avian Escherichia coli. Vet. Res. Commun. 27, 101–110. doi: 10.1023/a:1022854902700
Oliveira, J. M., Cardoso, M. F., Moreira, F. A., and Müller, A. (2022). Phenotypic antimicrobial resistance (AMR) of avian pathogenic (APEC) from broiler breeder flocks between 2009 and 2018. Avian Pathol. 51, 388–394. doi: 10.1080/03079457.2022.2074816
Palaniyandi, S., Mitra, A., Herren, C. D., Lockatell, C. V., Johnson, D. E., Zhu, X., et al. (2012). BarA-UvrY two-component system regulates virulence of uropathogenic E. coli CFT073. PLoS One 7:e31348. doi: 10.1371/journal.pone.0031348
Panigraphy, B., Gyimah, J. E., Hall, C. F., and Williams, J. D. (1984). Immunogenic potency of an oil-emulsified Escherichia coli bacterin. Avian Dis. 28, 475–481.
Parreira, V. R., and Gyles, C. L. (2003). A novel pathogenicity island integrated adjacent to the thrW tRNA gene of avian pathogenic Escherichia coli encodes a vacuolating autotransporter toxin. Infect Immun. 71, 5087–5096. doi: 10.1128/iai.71.9.5087-5096.2003
Peighambari, S. M., Hunter, D. B., Shewen, P. E., and Gyles, C. L. (2002). Safety, immunogenicity, and efficacy of two Escherichia coli cya crp mutants as vaccines for broilers. Avian Dis. 46, 287–297. doi: 10.1637/0005-20862002046[0287:SIAEOT]2.0.CO;2
Pokharel, P., Habouria, H., Bessaiah, H., and Dozois, C. M. (2019). Serine Protease Autotransporters of the Enterobacteriaceae (SPATEs): Out and About and Chopping It Up. Microorganisms 7:594. doi: 10.3390/microorganisms7120594
Pourbakhsh, S. A., Boulianne, M., Martineau-Doizé, B., Dozois, C. M., Desautels, C., and Fairbrother, J. M. (1997). Dynamics of Escherichia coil infection in experimentally inoculated chickens. Avian Dis. 41, 221–233.
Rahman, M. R. T., Fliss, I., and Biron, E. (2022). Insights in the development and uses of alternatives to antibiotic growth promoters in poultry and swine production. Antibiotics (Basel) 11:766. doi: 10.3390/antibiotics11060766
Reid, C. J., McKinnon, J., and Djordjevic, S. P. (2019). Clonal ST131-22 strains from a healthy pig and a human urinary tract infection carry highly similar resistance and virulence plasmids. Microb. Genom 5:e000295. doi: 10.1099/mgen.0.000295
Rodriguez-Siek, K. E., Giddings, C. W., Doetkott, C., Johnson, T. J., Fakhr, M. K., and Nolan, L. K. (2005a). Comparison of Escherichia coli isolates implicated in human urinary tract infection and avian colibacillosis. Microbiology (Reading) 151(Pt 6), 2097–2110. doi: 10.1099/mic.0.27499-0
Rodriguez-Siek, K. E., Giddings, C. W., Doetkott, C., Johnson, T. J., and Nolan, L. K. (2005b). Characterizing the APEC pathotype. Vet. Res. 36, 241–256. doi: 10.1051/vetres:2004057
Roland, K., Curtiss, R. III, and Sizemore, D. (1999). Construction and evaluation of a delta cya delta crp Salmonella typhimurium strain expressing avian pathogenic Escherichia coli O78 LPS as a vaccine to prevent airsacculitis in chickens. Avian Dis. 43, 429–441.
Ronco, T., Stegger, M., Olsen, R. H., Sekse, C., Nordstoga, A. B., Pohjanvirta, T., et al. (2017). Spread of avian pathogenic Escherichia coli ST117 O78:H4 in Nordic broiler production. BMC Genomics 18:13. doi: 10.1186/s12864-016-3415-6
Rosenberger, J. K., Fries, P. A., and Cloud, S. S. (1985). In vitro and in vivo characterization of avian Escherichia coli. III. Immunization. Avian Dis. 29, 1108–1117.
Russo, T. A., and Johnson, J. R. (2000). Proposal for a new inclusive designation for extraintestinal pathogenic isolates of Escherichia coli: ExPEC. J. Infect Dis. 181, 1753–1754. doi: 10.1086/315418
Russo, T. A., and Johnson, J. R. (2003). Medical and economic impact of extraintestinal infections due to Escherichia coli: focus on an increasingly important endemic problem. Microbes Infect 5, 449–456. doi: 10.1016/s1286-4579(03)00049-2
Russo, T. A., McFadden, C. D., Carlino-MacDonald, U. B., Beanan, J. M., Olson, R., and Wilding, G. E. (2003). The siderophore receptor iron of extraintestinal pathogenic Escherichia coli is a potential vaccine candidate. Infect Immun. 71, 7164–7169. doi: 10.1128/iai.71.12.7164-7169.2003
Sabri, M., Caza, M., Proulx, J., Lymberopoulos, M. H., Brée, A., Moulin-Schouleur, M., et al. (2008). Contribution of the SitABCD, MntH, and FeoB metal transporters to the virulence of avian pathogenic Escherichia coli O78 strain chi7122. Infect Immun. 76, 601–611. doi: 10.1128/IAI.00789-07
Sarowska, J., Futoma-Koloch, B., Jama-Kmiecik, A., Frej-Madrzak, M., Ksiazczyk, M., Bugla-Ploskonska, G., et al. (2019). Virulence factors, prevalence and potential transmission of extraintestinal pathogenic Escherichia coli isolated from different sources: recent reports. Gut Pathog. 11:10. doi: 10.1186/s13099-019-0290-0
Shames, S. R., Auweter, S. D., and Finlay, B. B. (2009). Co-evolution and exploitation of host cell signaling pathways by bacterial pathogens. Int. J. Biochem. Cell Biol. 41, 380–389. doi: 10.1016/j.biocel.2008.08.013
Shin, S. W., Shin, M. K., Jung, M., Belaynehe, K. M., and Yoo, H. S. (2015). Prevalence of antimicrobial resistance and transfer of tetracycline resistance genes in Escherichia coli isolates from beef cattle. Appl. Environ. Microbiol. 81, 5560–5566. doi: 10.1128/AEM.01511-15
Smith, J. L., Fratamico, P. M., and Gunther, N. W. (2007). Extraintestinal pathogenic Escherichia coli. Foodborne Pathog. Dis. 4, 134–163. doi: 10.1089/fpd.2007.0087
Thomassin, J. L., Lee, M. J., Brannon, J. R., Sheppard, D. C., Gruenheid, S., and Le Moual, H. (2013). Both group 4 capsule and lipopolysaccharide O-antigen contribute to enteropathogenic Escherichia coli resistance to human α-defensin 5. PLoS One 8:e82475. doi: 10.1371/journal.pone.0082475
Tu, J., Fu, D., Gu, Y., Shao, Y., Song, X., Xue, M., et al. (2021). Transcription regulator YgeK affects the virulence of avian pathogenic. Animals (Basel) 11:3018. doi: 10.3390/ani11113018
Tu, J., Huang, B., Zhang, Y., Zhang, Y., Xue, T., Li, S., et al. (2016). Modulation of virulence genes by the two-component system PhoP-PhoQ in avian pathogenic Escherichia coli. Pol. J. Vet. Sci. 19, 31–40. doi: 10.1515/pjvs-2016-0005
van den Bosch, J. F., Hendriks, J. H., Gladigau, I., Willems, H. M., Storm, P. K., and de Graaf, F. K. (1993). Identification of F11 fimbriae on chicken Escherichia coli strains. Infect Immun. 61, 800–806. doi: 10.1128/iai.61.3.800-806.1993
Vergalito, F., Pietrangelo, L., Petronio Petronio, G., Colitto, F., Alfio Cutuli, M., Magnifico, I., et al. (2018). Vitamin E for prevention of biofilm-caused healthcare-associated infections. Open Med. 15, 14–21. doi: 10.1515/med-2020-0004
Vounba, P., Rhouma, M., Arsenault, J., Bada Alambédji, R., Fravalo, P., and Fairbrother, J. M. (2019). Prevalence of colistin resistance and mcr-1/mcr-2 genes in extended-spectrum β-lactamase/AmpC-producing Escherichia coli isolated from chickens in Canada, Senegal and Vietnam. J. Glob. Antimicrob. Resist. 19, 222–227. doi: 10.1016/j.jgar.2019.05.002
Wang, H., Liang, K., Kong, Q., and Liu, Q. (2019). Immunization with outer membrane vesicles of avian pathogenic Escherichia coli O78 induces protective immunity in chickens. Vet. Microbiol. 236:108367. doi: 10.1016/j.vetmic.2019.07.019
Wang, M., Jiang, M., Wang, Z., Chen, R., Zhuge, X., and Dai, J. (2021). Characterization of antimicrobial resistance in chicken-source phylogroup F Escherichia coli: similar populations and resistance spectrums between E. coli recovered from chicken colibacillosis tissues and retail raw meats in Eastern China. Poult. Sci. 100:101370. doi: 10.1016/j.psj.2021.101370
Wang, S., Liu, X., Xu, X., Yang, D., Wang, D., Han, X., et al. (2016a). Escherichia coli Type III Secretion System 2 ATPase EivC Is Involved in the Motility and Virulence of Avian Pathogenic Escherichia coli. Front Microbiol. 7:1387. doi: 10.3389/fmicb.2016.01387
Wang, S., Liu, X., Xu, X., Zhao, Y., Yang, D., Han, X., et al. (2016b). Escherichia coli type III secretion system 2 (ETT2) is widely distributed in avian pathogenic Escherichia coli isolates from Eastern China. Epidemiol. Infect 144, 2824–2830. doi: 10.1017/S0950268816000820
Wang, S., Meng, Q., Dai, J., Han, X., Han, Y., Ding, C., et al. (2014). Development of an allele-specific PCR assay for simultaneous sero-typing of avian pathogenic Escherichia coli predominant O1, O2, O18 and O78 strains. PLoS One 9:e96904. doi: 10.1371/journal.pone.0096904
Wang, S., Peng, Q., Jia, H. M., Zeng, X. F., Zhu, J. L., Hou, C. L., et al. (2017). Prevention of Escherichia coli infection in broiler chickens with Lactobacillus plantarum B1. Poult. Sci. 96, 2576–2586. doi: 10.3382/ps/pex061
Wang, S., Shi, Z., Xia, Y., Li, H., Kou, Y., Bao, Y., et al. (2012). IbeB is involved in the invasion and pathogenicity of avian pathogenic Escherichia coli. Vet. Microbiol. 159, 411–419. doi: 10.1016/j.vetmic.2012.04.015
Wang, Z., Zheng, X., Guo, G., Hu, Z., Miao, J., Dong, Y., et al. (2022). O145 may be emerging as a predominant serogroup of Avian pathogenic Escherichia coli (APEC) in China. Vet. Microbiol. 266:109358. doi: 10.1016/j.vetmic.2022.109358
Xue, M., Fu, D., Hu, J., Shao, Y., Tu, J., Song, X., et al. (2022). The transcription regulator YgeK affects biofilm formation and environmental stress resistance in avian pathogenic Escherichia coli. Animals (Basel) 12:1160. doi: 10.3390/ani12091160
Yamamoto, K., and Ishihama, A. (2006). Characterization of copper-inducible promoters regulated by CpxA/CpxR in Escherichia coli. Biosci. Biotechnol. Biochem. 70, 1688–1695. doi: 10.1271/bbb.60024
Yi, Z., Wang, D., Xin, S., Zhou, D., Li, T., Tian, M., et al. (2019). The CpxR regulates type VI secretion system 2 expression and facilitates the interbacterial competition activity and virulence of avian pathogenic Escherichia coli. Vet. Res. 50:40. doi: 10.1186/s13567-019-0658-7
Yin, L., Cheng, B., Tu, J., Shao, Y., Song, X., Pan, X., et al. (2022). YqeH contributes to avian pathogenic Escherichia coli pathogenicity by regulating motility, biofilm formation, and virulence. Vet. Res. 53:30. doi: 10.1186/s13567-022-01049-6
Yu, F., Addison, O., Baker, S. J., and Davenport, A. J. (2015). Lipopolysaccharide inhibits or accelerates biomedical titanium corrosion depending on environmental acidity. Int. J. Oral. Sci. 7, 179–186. doi: 10.1038/ijos.2014.76
Keywords: APEC, epidemiology, virulence, zoonotic, antibiotic resistance, control strategies
Citation: Hu J, Afayibo DJA, Zhang B, Zhu H, Yao L, Guo W, Wang X, Wang Z, Wang D, Peng H, Tian M, Qi J and Wang S (2022) Characteristics, pathogenic mechanism, zoonotic potential, drug resistance, and prevention of avian pathogenic Escherichia coli (APEC). Front. Microbiol. 13:1049391. doi: 10.3389/fmicb.2022.1049391
Received: 20 September 2022; Accepted: 21 November 2022;
Published: 13 December 2022.
Edited by:
Jianmin Zhang, South China Agricultural University, ChinaReviewed by:
Qingqing Gao, Yangzhou University, ChinaCopyright © 2022 Hu, Afayibo, Zhang, Zhu, Yao, Guo, Wang, Wang, Wang, Peng, Tian, Qi and Wang. This is an open-access article distributed under the terms of the Creative Commons Attribution License (CC BY). The use, distribution or reproduction in other forums is permitted, provided the original author(s) and the copyright owner(s) are credited and that the original publication in this journal is cited, in accordance with accepted academic practice. No use, distribution or reproduction is permitted which does not comply with these terms.
*Correspondence: Shaohui Wang, c2h3YW5nMDgyN0AxMjYuY29t
†These authors have contributed equally to this work and share first authorship
Disclaimer: All claims expressed in this article are solely those of the authors and do not necessarily represent those of their affiliated organizations, or those of the publisher, the editors and the reviewers. Any product that may be evaluated in this article or claim that may be made by its manufacturer is not guaranteed or endorsed by the publisher.
Research integrity at Frontiers
Learn more about the work of our research integrity team to safeguard the quality of each article we publish.