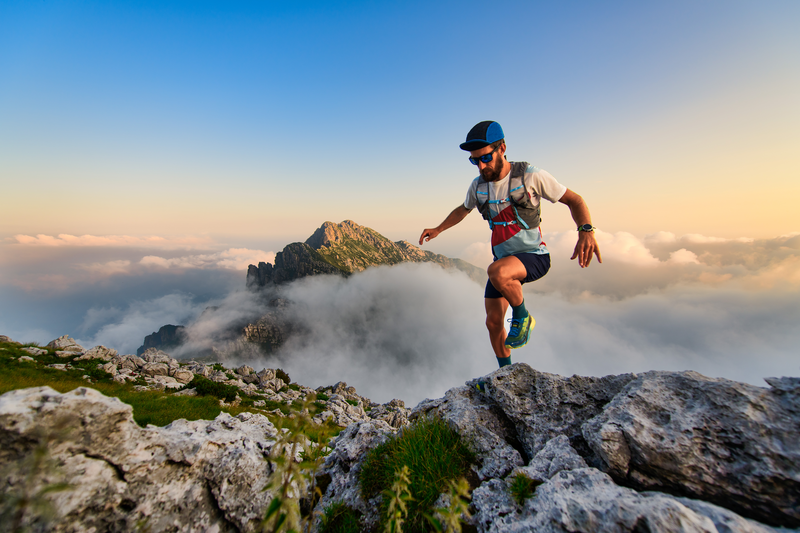
94% of researchers rate our articles as excellent or good
Learn more about the work of our research integrity team to safeguard the quality of each article we publish.
Find out more
ORIGINAL RESEARCH article
Front. Microbiol. , 08 November 2022
Sec. Infectious Agents and Disease
Volume 13 - 2022 | https://doi.org/10.3389/fmicb.2022.1048648
This article is part of the Research Topic Animal Emerging and Reemerging Diseases View all 24 articles
Mycobacterium bovis (M. bovis) is the zoonotic bacterium responsible for bovine tuberculosis. An attenuated form of M. bovis, Bacillus Calmette-Guerin (BCG), is a modified live vaccine known to provide variable protection in cattle and other species. Protection for this vaccine is defined as a reduction in disease severity rather than prevention of infection and is determined by evaluation of the characteristic lesion of tuberculosis: the granuloma. Despite its recognized ability to decrease disease severity, the mechanism by which BCG imparts protection remains poorly understood. Understanding the histopathologic differences between granulomas which form in BCG vaccinates compared to non-vaccinates may help identify how BCG imparts protection and lead to an improved vaccine. Utilizing special stains and image analysis software, we examined 88 lymph nodes obtained from BGC-vaccinated and non-vaccinated animals experimentally infected with M. bovis. We evaluated the number of granulomas, their size, severity (grade), density of multinucleated giant cells (MNGC), and the amounts of necrosis, mineralization, and fibrosis. BCG vaccinates had fewer granulomas overall and smaller high-grade granulomas with less necrosis than non-vaccinates. The relative numbers of high- and low- grade lesions were similar as were the amounts of mineralization and the density of MNGC. The amount of fibrosis was higher in low-grade granulomas from vaccinates compared to non-vaccinates. Collectively, these findings suggest that BCG vaccination reduces bacterial establishment, resulting in the formation of fewer granulomas. In granulomas that form, BCG has a protective effect by containing their size, reducing the relative amount of necrosis, and increasing fibrosis in low-grade lesions. Vaccination did not affect the amount of mineralization or density of MNGC.
Mycobacterium bovis (M. bovis) is the most host-promiscuous member of the Mycobacterium tuberculosis complex (MTBC), capable of causing tuberculous disease in over 85 species of animals, including some endangered wildlife species (Kanipe and Palmer, 2020). It is the primary cause of bovine tuberculosis (bTB) worldwide. It is a scourge to farmers, livestock, and conservationists by exacting economic tolls, as well as causing disease in both animals and humans.
Bacillus Calmette-Guérin (BCG) is an attenuated strain of Mycobacterium bovis which has been used as a vaccine for protection against Mycobacterium tuberculosis (Mtb) in humans for over 100 years. It has long been accepted as providing variable protection against virulent M. bovis in livestock and wildlife (Buddle et al., 2018; Roy et al., 2019). Importantly however, protection is often defined as a decrease in disease severity rather than protection from infection. Additionally, currently utilized diagnostic techniques based on purified protein derivative (PPD), also known as tuberculin, fail to differentiate BCG-vaccinates from M. bovis infected animals, further complicating both its use, and role in disease eradication and control efforts. Reports of protection range widely from 0 to 100% depending on age, route of vaccination, vaccine dose and uncontrollable variables such as genetics and environment (Canto Alarcon et al., 2013; Buddle et al., 2018; Bayissa et al., 2021). In naturally infected cattle results vary, but BCG vaccinates have been shown to have lower bTB prevalence (based on isolation of M. bovis), lower numbers of cattle with gross lesions, and less severe disease than non-vaccinates (Nugent et al., 2018; Bayissa et al., 2021). Because of its cross reactivity with the primary herd diagnostic, the tuberculin skin test, and the success of eradication programs in decreasing overall prevalence of bTB, interest in BCG vaccination in most countries decreased during the mid to late 20th century. Unfortunately, complete eradication has been unsuccessful in most countries for various reasons including, lack of sensitivity and specificity in current diagnostic assays, increases in the size of concentrated feeding operations (CAFOs), importation of infected animals from other countries and the presence of wildlife reservoirs of M. bovis with persistent wildlife-to-cattle transmission (de la Rua-Domenech et al., 2006; Fitzgerald and Kaneene, 2013; Ciaravino et al., 2021). Thus, there is a renewed interest in vaccines. Even with the shortcomings of BCG, thus far, no experimental bTB vaccine has consistently outperformed BCG in vaccine efficacy studies. Until a vaccine which consistently improves protection against disease is found, it is prudent to continue research on BCG’s properties and how it imparts protection. Numerous studies have explored the differences in peripheral responses between vaccinates and non-vaccinates, but this provides an incomplete picture of pathogenesis, as it is distant from the site of active host-pathogen interaction in the tissue. In order to fully elucidate how BCG works, we therefore need intralesional examination (Jones et al., 2017; Steinbach et al., 2021; Khalid et al., 2022).
The characteristic lesion of tuberculosis is the granuloma. Granulomas are collections of macrophages with multiple modifiers such as the presence or absence of varying amounts of neutrophils, lymphocytes, multinucleated giant cells (MNGC), fibrosis, necrosis, and mineralization. These are dynamic structures, with cell trafficking resulting from host- and bacterially-originating signals. Until recently, it was believed that the tuberculous granuloma was a structure which benefited both host and pathogen at different points in infection, early on by promoting dissemination of the bacteria as a result of migrating macrophages, and later by “walling off” the offending intruder through fibrosis. Although this paradigm remains popular, new advances in research create challenges for its defense (Ramakrishnan, 2012). Understanding the differences between granulomas that form in BCG vaccinates compared to non-vaccinates may not only help identify how BCG imparts protection but may also more aptly help define how it benefits pathogen as well as host. While tuberculous granulomas have been documented in numerous organs, the principal sites in cattle are the thoracic lymph nodes (tracheobronchial and mediastinal) and within the lung parenchyma. Interestingly, cattle and humans share histologic similarities in their granuloma structure only surpassed by non-human primates. Due to the financial and ethical implications with using non-human primates, this elevates the importance of work performed in cattle, which could yield valuable insight into human disease.
Previous pioneering works by Johnson, et. al have demonstrated reduced granuloma numbers, size, necrosis, peripheral fibrosis, and MNGC numbers in lymph nodes of BCG vaccinated animals compared to non-vaccinated controls when experimentally infected with virulent M. bovis (Johnson et al., 2006). The reduction in granuloma number and size, as well as decreased tissue destruction suggest improved host control over the bacteria and decreased MNGC numbers suggests a reduction in antigen persistence(Pagan and Ramakrishnan, 2018; Trout and Holian, 2020; Palmer et al., 2022). Decreased peripheral fibrosis is likely associated with decreased bacterial burden compared to non-vaccinates as demonstrated in non-human primates (Gideon et al., 2022). The aim of this study is to build, confirm and expand upon Johnson’s work in several key areas. Using histopathologic examination combined with advanced imaging software, we examine a total of 88 pulmonary lymph nodes from 22 BCG-vaccinated and 22 non-vaccinated cattle, all of which were experimentally infected with virulent M. bovis. The result of this study is a comprehensive look of the pathology that forms in the pulmonary lymph nodes of BCG-vaccinates and non-vaccinates.
Banked tissue samples from two previous studies were utilized. In both studies cattle were obtained from bTB-free herds from Iowa, United States. Briefly, in Experiment 1, 23 castrated Holstein steers of 4–5 months of age were divided into two groups: non-vaccinates (n = 11) and BCG vaccinates (n = 12). Animals were kept on pasture prior to the start of the study. As environmental bacteria are present in our area, and in order to prevent possible confounding responses to BCG vaccination, animals were confirmed non-reactive to Mycobacterium avium (M. avium) via interferon gamma release assay (IGRA) immediately before the start of the study. Animals in the vaccinated group received a subcutaneous injection 1 ml of 5 × 105 CFU of BCG Danish. Three months following vaccination, both vaccinates and non-vaccinates received 5.5 × 102 CFU of M. bovis strain 1,315 via aerosolization as described elsewhere (Palmer et al., 2002). Strain 1,315 is a virulent field strain obtained from a white-tailed deer in Michigan, United States. Animals were euthanized 13–17 weeks post-challenge. In Experiment 2, 21 newborn Holstein steers were divided into two groups: non-vaccinates (n = 11) and BCG vaccinates (n = 10). Animals were bottle-raised on a pasteurized milk product and kept in clean pens to reduce exposure to environmental mycobacteria. Due to the cleanliness of the housing situation the risk of exposure to environmental mycobacteria was considered low and IGRA testing for M. avium was not performed. Animals in the vaccinated group received a subcutaneous injection 1 ml of 1 × 106 CFU of BCG Danish at 2 weeks of age. Three months following vaccination, both vaccinates and non-vaccinates received 1 × 103 CFU of M. bovis strain 1,315 via aerosolization. Approximately 18 weeks post-challenge animals were euthanized. In both studies animals were humanely euthanized by intravenous administration of sodium pentobarbital. All animal procedures were approved prior to the experiments by the Institutional Animal Care and Use Committee (IACUC) at the National Animal Disease Center. At necropsy, both experimental groups demonstrated a significant reduction in gross lesions in the lungs of BCG-vaccinates compared to non-vaccinates and a significant decrease in CFU/gram of lymph node tissue between vaccinates and non-vaccinates (Waters et al., 2009). An approximately 2.5 × 2 × 0.4 cm randomly selected sample of both the tracheobronchial and mediastinal lymph nodes were collected from each animal resulting in a total of 88 lymph node samples, 44 from each group. Samples were fixed in 10% neutral buffered formalin, paraffin embedded following standard techniques, and sectioned 4–5 μm thick prior to staining with Harris hematoxylin and eosin (H&E). The two groups used in this study varied in age at the time of vaccination, 2 weeks vs. 4–5 mos. It was previously reported that neonatally vaccinated animals had at least as robust an immune response as adults (Hope et al., 2005). Nevertheless, to account for differences between experimental groups we evaluated the effect of group within all statistical analyses and found significance only for fibrosis metrics where group was included as a fixed effect (see stats section).
Paraffin-embedded sections were stained with H&E using a Leica 5,020 multistainer (Leica/Surgipath). Von Kossa Calcium and Masson’s Trichrome staining were performed following manufacturer’s instructions (Newcomer Supply) for calcium and collagen fiber analysis, respectively. Following staining, slides were digitally scanned using the Aperio AT2 whole slide imager, to generate bright-field whole- slide images at 20X (0.5 μm/pixel) and 40X (0.25 μm/pixel) magnification in a 24-bit color pyramid TIFF (.SVS file). The. SVS files were transferred into HALO software (HALO v3.1.1076.291, Indica labs) for annotation and analysis.
The mediastinal and tracheobronchial lymph node values for each metric were initially evaluated separately to ensure differences were not a result of anatomic location. No significant differences were noted, therefore values from the tracheobronchial and mediastinal lymph nodes were combined and averaged to yield a single value per animal.
Digitally scanned images were evaluated at 10X magnification. Using HALO annotation tools, granulomas were outlined in different colors based on grade, and the total granuloma area calculated using HALO’s annotation software. A granuloma was defined as a focus consisting of increased accumulations of macrophages admixed with varying numbers of lymphocytes, neutrophils and MNGCs. If a tissue section lacked granulomas, it was excluded from the remaining histopathologic examinations. Granulomas were separated into two grades: low and high.
Low-grade granulomas were defined as singular, with approximately ≤10% necrosis. High-grade granulomas were defined as having greater than 10% of its total area as necrosis, being multicentric, or coalescent with other granulomas. Necrosis was defined as an area of tissue destruction characterized by degenerating, fragmented cells, and structural debris with or without mineralization. When present, fibrous encapsulation was included in the total granuloma area calculated. Care was made to closely follow the directional flow of the collagen fibers when numerous, closely associated but independent granulomas were present. When fibrous bands incompletely separated granulomas or had no identifiable pattern of separation, these were counted as a single, coalescing lesion and considered high grade.
High grade granulomas were outlined in green while low grade granulomas were outlined in yellow (Figure 1). The absolute number and the number of each type of granuloma were enumerated and totaled for each animal. For high- and low-grade granulomas, the average granuloma size was calculated by dividing the total granuloma area within an animal by the number of granulomas of that grade. Necrosis was outlined using a dotted line of the corresponding grade color, and its area summated to provide the total area of necrosis. The percent necrosis was calculated, and if a low-grade granuloma was found to contain ≥10% necrosis, it was redefined as a high-grade granuloma. Enumeration of MNGCs was performed by placement of digital pins which were summated (see Figure 1). Multinucleated giant cells were defined as macrophages containing three or more nuclei within a continuous cytoplasm, identifiable at 10X magnification, in accordance with other published studies(Trout and Holian, 2020; Losslein et al., 2021).The density of MNGCs was calculated as the number of MNGC present per 100μm2 of non-necrotic area.
Figure 1. HALO imaging annotations performed on H&E tracehobronchial lymph node sections of BCG-vaccinated and non-vaccinated cattle challenged with aerosolized M. bovis. Granulomas were outlined in either green or yellow based on the amount of necrosis and/or if their boundaries were indistinguishable from the neighboring granuloma (coalescing). If there was ≤10% necrosis, it was classified as a low-grade granuloma and outlined in yellow. If there was >10% necrosis or if it was a collection of coalescing granulomas, it was outlined in green and categorized as high-grade. Necrosis, when present, was outlined by a dotted line of a matching color. MNGCs identified at 10X were marked with a pin of the same color as the grade designation. H&E section prior to annotation shown in inset.
Numerous previous studies utilized a four-stage scoring system developed by Wangoo et al to describe granuloma histopathology (Wangoo et al., 2005; Johnson et al., 2006; Waters et al., 2007; Salguero et al., 2017; Palmer et al., 2021). Granulomas staged as I and II by the Wangoo system would be categorized as low-grade in this study while those granulomas classified as stage III, and IV by Wangoo et al. would be considered high-grade. Occasionally, a Wangoo stage III granuloma would fall under the low-grade designation when small foci of mineral were present with ≤10% necrosis. The Wangoo system categorizes any granuloma containing mineral as either a III or IV depending on the other characteristics present.
Utilizing Von Kossa-stained slides, high- and low-grade granulomas were identified in two independent layers, at 10X magnification. Samples without identifiable lesions were excluded. High- and low-grade designations used for the H&E stains were followed to classify the lesions into their respective groups. These outlines served as the boundaries for tissue to be analyzed. A digital filter was formulated to identify calcium-containing areas representative of mineralization. Starting with algorithm Indica labs-Area quantification v2.1.7, a stain color was selected and optimized to identify mineralization. The percent area of mineralization for both high- and low-grade granulomas was calculated using the area of staining identified by the filter divided by the total area analyzed for each high- and low-grade granuloma.
Utilizing Masson’s trichrome-stained slides, high- and low-grade granulomas were outlined in two independent layers, at 10X magnification to ensure all granulomas were identified. Samples without identifiable lesions were excluded. High- and low-grade designations used for the H&E stains were followed to classify the lesions into their respective groups. A digital filter was formulated to identify collagenous areas highlighted by the trichrome stain and applied to the entire granuloma. Starting with algorithm Indica labs-Area quantification v2.1.7, a stain color was selected and optimized to identify fibrosis based on the color of collagen found in dense collagenous bundles. The filter was applied to each annotated layer. The percent fibrosis for high- and low-grade granulomas was calculated using the area of staining identified by the filter divided by the total area analyzed for each high- and low-grade granuloma.
For all scored granuloma metrics (count, average area, percent necrosis, MNGC frequency, percent mineralization, and percent fibrosis) data were analyzed with a simple linear regression model (lm) in R (version 4.2.1). Each scored metric was evaluated independently, fitting grade, vaccination status, and their interaction as fixed effects. A fixed effect of ‘group’, denoting the two separate experimental cohorts, was evaluated for model fit for each metric and only deemed appropriate to include for analysis of percent fibrosis. A pairwise comparison of Least Squares means (lsmeans package) was utilized to determine significant differences between specific grade and vaccination group contrasts. For all, significance was determined when p-value ≤0.05. Error bars represent standard errors.
Graph construction was performed using GraphPad Prism 9 (GraphPad Software Inc., San Diego, CA). Values for the mediastinal and tracheobronchial lymph nodes for each animal were combined to yield a single data point per animal. For all analyses performed except in the evaluation of the total number of granulomas, animals without granulomas in either lymph node were excluded. In cases where an animal lacked either high- or low-grade granulomas (and therefore their calculated values were 0), these data points were excluded with exception of granuloma count.
BCG vaccinates had fewer granulomas compared to non-vaccinates. The ratios of high- and low-grade granulomas were similar between vaccinates and non-vaccinates.
Non-vaccinated animals had higher numbers of granulomas compared to BCG-vaccinates (p < 0.0001; Figure 2A). The mean number of individual granulomas per animal in BCG-vaccinates was 18.95 (range 0–51) while the mean number of granulomas per animal in non-vaccinates was 61.5 (range 17–178). In total, 1770 granulomas were evaluated. BCG vaccinates accounted for 417 (23.6%) of these granulomas while 1,353 (76.4%) granulomas were in lymph nodes of non-vaccinates. Eight lymph node samples from BCG-vaccinates lacked identifiable granulomas, and these samples were excluded for the remainder of the study.
Figure 2. Absolute numbers of granulomas in BCG-vaccinated and non-vaccinated cattle infected with M. bovis and percentage of each granuloma grade. (A) The total number of granulomas present in vaccinates and non-vaccinates, respectively. Each dot represents one animal and the combined total of granulomas in its mediastinal and tracheobronchial lymph nodes. (B) the relative percentages of high- and low-grade granulomas. Values are presented as means ± SEM (****value of p < 0.0001).
While the number of granulomas varied markedly between BCG-vaccinates and non-vaccinates, the relative percentages of each grade of granuloma were similar (Figure 2B). There were approximately equal numbers of high- and low-grade granulomas, within each vaccinate group. Of the total granulomas present in BCG vaccinates, 58.52% of them were low grade while 43.14% were high grade. In non-vaccinates these percentages were 57.56 and 42.44%, respectively. Overall, these data indicate that there is a trend for fewer high-grade granulomas compared to low grade granulomas independent of vaccination status (p = 0.551 and p = 0.0627 for non-vaccinates and vaccinates, respectively).
BCG vaccinates had smaller high-grade granulomas and lesions with less necrosis.
The average size (area) of granulomas was compared between vaccinates and non-vaccinates. There was no significant difference (p = 0.0675) in the average size of granulomas between vaccinates and non-vaccinates, however lesions from non-vaccinated animals tended to be smaller (Figure 3A). Additionally, low-grade granulomas did not significantly vary in size between vaccinates and non-vaccinates however high-grade granulomas were larger in non-vaccinates than vaccinates (p = 0.0434; Figure 3B). Within vaccination groups, BCG vaccinates had similarly sized low- and high-grade granulomas (p = 0.6296) while non-vaccinates had larger high-grade granulomas compared to low-grade granulomas (p = 0.0064).
Figure 3. Average area of granulomas by both vaccination group and granuloma grade in BCG-vaccinated and non-vaccinated cattle infected with M. bovis. (A) Granuloma size by area (μm2) based on vaccination status. Each dot represents one animal. The average area of a granuloma was tabulated by dividing the total area occupied by all granulomas in the mediastinal and tracheobronchial lymph nodes of an animal by the total number of granulomas present. (B) Granuloma size by area (μm2) when broken down by grade (high or low). Values are presented as means ± SEM. (* = p value 0.0434, ** = p value 0.0064, ns = not significant).
In this study, to be considered low-grade, the amount of necrosis had to be ≤10% the total area. However, high grade granulomas may have ≤10% of necrosis if they were multicentric or coalescing. BCG vaccinates had lesions containing significantly (p = 0.0029) less tissue destruction as evidenced by a lower percentage of necrosis than non-vaccinates (Figure 4A). As a result of the categorization method, necrosis was expected to be substantially higher in high grade granulomas compared to low grade and this was true for both vaccinates and non-vaccinates (p < 0.001 for both). There was no significant difference in the percentage of necrosis within low-grade granulomas of vaccinates compared to non-vaccinates with values being nearly identical (p = 0.9766; Figure 4B). High-grade granulomas of non-vaccinates contained a significantly (p = 0.0080) higher percentage of necrosis than high-grade granulomas from BCG-vaccinates.
Figure 4. Percentage of granuloma area comprised of necrosis in BCG-vaccinated and non-vaccinated cattle infected with M. bovis. Values are broken down by vaccination group (A) and by vaccination group and grade (B). Each dot represents one animal and its mediastinal and tracheobronchial lymph nodes. Values are presented as means ± SEM. (** = p value 0.0029 for A, ** = p value 0.0080 for B, **** = p value <0.0001).
MNGC numbers were not dependent on vaccination status or lesion severity.
The density of MNGC was calculated as the number of MNGCs per 100 μm2 of granuloma area. No significant differences were found in the density of MNGC between vaccination groups (Figure 5A). When separated by grade, there were no significant differences in the number of MNGC between high- and low-grade granulomas, however there was a trend (p = 0.0632) for low grade granulomas of non-vaccinates to contain a higher number of MNGC than high grade granulomas of the same group (Figure 5B).
Figure 5. The density of multinucleated giant cells by vaccination group and granuloma grade in BCG-vaccinated and non-vaccinated cattle infected with M. bovis. Values represent the number per 100μm2 and are broken down by vaccination group (A) and by vaccination group and grade (B). Each dot represents one animal and its mediastinal and tracheobronchial lymph nodes. Values are presented as means ± SEM. (ns = not significant).
BCG vaccination increased the amount of fibrosis in low grade granulomas. BCG vaccination did not influence the amount of mineralization. Mineralization increased with the severity of the lesion.
Fibrosis was the only metric in which experimental group had a significant effect. The primary difference between the two groups of animals used for this study is age, where the older cohort (from Experiment 1) had higher average fibrosis than the younger animals (from Experiment 2; data not shown). That being said, there were no significant differences in the percentage of fibrosis per granuloma between vaccinates and non-vaccinates for either experimental group (Figure 6A). Collectively, when evaluated by grade, low grade granulomas of vaccinates had a significantly (p = 0.0344) higher percentage of fibrosis than low-grade granulomas of non-vaccinates (Figure 6B). When mineralization was evaluated, no significant differences were observed in the percentage of mineralization between vaccinates and non-vaccinates (Figure 7A). Additionally, mineralization was similar within grades of granuloma, despite different vaccination groups (Figure 7B). Significant differences existed between low grade granulomas and their corresponding high-grade granulomas with a p = 0.0009 in vaccinates and p < 0.0001 in non-vaccinates.
Figure 6. Percentage of granuloma area comprised of fibrosis as measured using Masson’s trichrome staining in BCG-vaccinated and non-vaccinated cattle infected with M. bovis. Values are broken down by vaccination group (A) and by vaccination group and grade (B). Each dot represents one animal and its mediastinal and tracheobronchial lymph nodes. Values are presented as means ± SEM. (* = p value 0.0344, ns = not significant).
Figure 7. Percentage of granuloma area comprised of mineralization as measured using Von Kossa staining in BCG-vaccinated and non-vaccinated cattle infected with M. bovis. Values are broken down by vaccination group (A) and by vaccination group and grade (B). Each dot represents one animal and its mediastinal and tracheobronchial lymph nodes. Values are presented as means ± SEM. (*** = p value 0.0009, **** = p value <0.0001, ns = not significant).
This study utilized 88 pulmonary lymph nodes from 44 animals (22 BCG vaccinates, 22 non-vaccinates), resulting in the evaluation of a total of 1,770 granulomas. Of these, 417 granulomas were in BCG vaccinates while 1,353 were in non-vaccinates.
Previous studies which have focused on granuloma histopathology have utilized a 4-stage scoring system created by Wangoo et al (Wangoo et al., 2005; Johnson et al., 2006). While originally created to be a consistent, easy way to describe the severity of granulomas, we found it subjective in some regards, ([ex] “minimal necrotic areas”) and inappropriately absolute in others, ([ex] mineralization not mentioned until stage III or above). These aspects could make it difficult to accurately compare results from different vaccine studies from different labs. As it pertains to vaccine efficacy in tuberculosis research, the primary concern is if disease severity is reduced. While the 4-stage system is more descriptive, in this study histopathologic lesion staging was streamlined and simplified into high- and low grades based primarily on amount of necrosis. Here, lesions were categorized into either low grade, with ≤10% necrosis and high grade, with >10% necrosis and/or coalescing lesions. This binary approach allowed for pertinent, precise evaluation while eliminating some of the subjective aspects of 4 stage scoring. While any amount of necrosis could have been selected for the high and low cutoff values, we found this new scoring system to align most closely to the Wangoo system while removing subjectivity. In this study, stage I and II lesions would be considered low grade while stage III and stage IV lesions would have been classified as high grade. Granulomas designated as low grade represent those in which cellular destruction is minimal, therefore they may still be considered under host control. In contrast, granulomas designated as high grade may be interpreted as a loss of host control as necrotic cellular accumulations and/or multicentricity are features which likely benefit the bacterium and represent irreversible tissue damage (Basaraba, 2008; Basaraba and Orme, 2011). In human tuberculosis, reactivation of latent disease is associated with increased necrosis within granulomas (Russell et al., 2010). This binary microscopic scoring system is user-friendly and can be combined with gross lesion scoring if the purpose of a given study is to evaluate vaccine efficacy. The speed at which it can be applied additionally lends itself to studies utilizing large numbers of animals.
As expected, and in accordance with previous studies, BCG decreased the lesion burden (Buddle et al., 1995; Johnson et al., 2006; Canto Alarcon et al., 2013; Dean et al., 2014; Salguero et al., 2017; Palmer et al., 2022). Vaccinated animals had an average of 19 granulomas per tissue section while non-vaccinated animals averaged 62 granulomas. Interestingly, despite this contrast, the relative breakdown of high- and low-grade granulomas did not significantly vary, with vaccinates and non-vaccinates having similar percentages of each. This suggests that the protective nature of BCG is, in part, by preventing the establishment of granulomas as opposed to necessarily preventing their progression to a higher grade. These findings are consistent with Johnson et al. and studies performed in white-tailed deer which report all stages of granulomas in lymph nodes of BCG vaccinates (Johnson et al., 2006; Nol et al., 2008; Palmer et al., 2009). Although BCG did not prevent high grade granulomas from being formed, it maintained smaller lesions on average with less necrosis. This finding is reasonable, as BCG primes the immune system for a strong cell-mediated immune response, which is believed to reduce the growth of lesions (Watanabe et al., 2006; Domingo et al., 2014). Although not quantified, “satellite” granulomas, lesions closely associated with another granuloma, were present in both vaccinates and non-vaccinates. In this study, “satellite” lesions were of both high- and low-grades, a finding in contrast to previous studies which identified only low-grade granulomas (Palmer et al., 2007; Canal et al., 2017). While differences in study design between the previous studies and the current could potentially account for the disparity in satellite granuloma grades, it does raise a curious topic to scientifically explore. It is generally believed that satellite lesions represent dissemination from the nearby “primary granuloma.” Quantifying and histologically characterizing the differences between “satellite” granulomas between vaccinates and non-vaccinates may provide insight on if a vaccine reduces dissemination of bTB within the host, and provide another metric in which to measure efficacy.
Fibrosis was the single metric in which there were statistical differences between experimental cohorts, of which the primary difference between the groups is age; older animals of Experiment 1 and the younger animals of Experiment 2. Older animals from Experiment 1 averaged more fibrosis in granulomas compared to younger animals of Experiment 2 (data not shown). This was unexpected and warrants further investigation, as it has not been reported previously. A possible explanation for the difference in fibrosis found between the two age groups could be levels of tumor growth factor beta (TGF-β), the cytokine most responsible for stimulating fibrosis. However, according to a study performed in sheep, TGF-β is lower in older animals vs. neonates (Sow et al., 2012). This would suggest other cytokines and/or immunological mechanisms are contributing to these differences. It has been demonstrated that calves vaccinated at birth with BCG produce similar levels of interferon gamma (IFN-γ) as calves 5–8 months old, however unlike the older calves, the neonatal vaccinates had a gradual decline in IFN-γ levels(Buddle et al., 2003). This might be relevant as IFN-γ can suppress TGF-β secretion by macrophages and could ultimately be responsible for the differences in fibrosis seen between age groups (Warsinske et al., 2017b). It is also possible that the older animals, being exposed for a longer period to the outdoors, may have come into contact with environmental mycobacteria, which altered their fibrotic response when exposed to virulent M. bovis (Buddle et al., 2003). Regardless, when taken as a collective group, vaccinates and non-vaccinates had similar overall percentages of fibrosis, a finding in contrast to previous studies (Johnson et al., 2006; Salguero et al., 2017). A possible explanation for this difference is that previous studies evaluated only the collagen peripherally encircling granulomas. Central fibrosis has been noted in other studies and associated with healing and containment in non-human primates, therefore it was included in our evaluation and was found to frequently be prominent (Figure 8; Flynn, 2011; Warsinske et al., 2017a; Martinot, 2018). When broken down by vaccinate group, the amount of fibrosis was significantly higher in low grade granulomas of vaccinates than non-vaccinates. By the time lesions progressed to high-grade, this significance was lost. While fibrosis has previously been considered a sign of an advanced or progressing lesion, our findings support data derived from simulated non-human primate granulomas which demonstrated fibrosis onset in lesions as early as ~10 days post infection or as late as after 200 days (Wangoo et al., 2005; Russell et al., 2009; Warsinske et al., 2017a; Palmer et al., 2021). In humans and non-human primate models, organized peripheral and central fibrosis has been associated with healing (Basaraba, 2008; Flynn, 2011; Palmer et al., 2022). These studies frequently involve post-chemotherapeutic treatment which is not routinely performed in cattle due to cost and risk of antibiotic residues in meat and milk, and the histopathologic composition of a healing bovine granuloma is therefore definitively unknown. However, in light of those studies, the finding of increased fibrosis in the low-grade lesions of vaccinates is intriguing and may represent a rapid immunological response as a result of BCG-induced memory, and an attempt at early containment of the infection.
Figure 8. Masson’s Trichrome stain of lymph node granulomas of cattle infected with M. bovis. Dark blue staining represents collagen which is found prominently surrounding the granulomas. Additionally, there are areas of centralized fibrosis throughout and immediately next to areas of necrosis (arrowheads).
Dystrophic mineralization is a common sequela to necrosis and therefore it is not surprising to see levels of mineralization increased in high grade granulomas. Nevertheless, it is interesting to note that the percentage of mineralization between high-grade granulomas were similar between vaccination groups and that small amounts of mineralization were present in the low-grade granulomas. While the Wangoo categorization system attributes mineralization with increased lesion score, and therefore, increased disease severity, studies in humans and non-human primates associate mineralization with healing, similar to fibrosis (Wangoo et al., 2005; Basaraba, 2008; Gideon et al., 2022). Indeed calcification is preferable over necrosis as necrosis can harbor viable bacteria while mineralization does not (Basaraba, 2008). Our findings of small amounts of mineral in low-grade granulomas and similar overall amounts between vaccinates and non-vaccinates, puts into question how disease severity should be defined in BCG vaccination studies in cattle.
Multinucleated giant cells are associated with persistent antigen in multiple diseases (Gupta et al., 2014; Gharun et al., 2017; Pagan and Ramakrishnan, 2018; Trout and Holian, 2020; Palmer et al., 2022). We hypothesized that MNGCs would be lower in BCG vaccinates as vaccination is typically associated with lower bacterial burden and antigen persistence(Buddle et al., 2003; Hope et al., 2005; Canto Alarcon et al., 2013; Sirak et al., 2021). Additionally, we expected to see higher numbers in high-grade granulomas as severe lesions have previously been associated with higher MNGC numbers (Menin et al., 2013). In this study we found no significant differences between groups or grade, however there was a trend for non-vaccinates to have more MNGC in their high-grade granulomas compared to their low-grade granulomas, suggesting increased bacterial burden may have played a role. Our findings differ from those of Johnson et al, which reported a significant reduction in the numbers of MNGCs in BCG vaccinated animals (Johnson et al., 2006). This difference could be due to the fact that Johnson et al, included only Langhan’s type giant cells. Although not explicitly quantified, and without a defined description of MNGC requirements, Salguero et al additionally reported a decrease in the number of MNGCs in BGC-vaccinated cattle (Salguero et al., 2017). Frequently the Langhan’s-type MNGC, with its nuclei arranged in a horseshoe shape, is considered the hallmark of tuberculosis, but in-fact, multiple other types are present and transformation from one morphology to another has been documented in cell culture (Singhal et al., 2011; Palmer et al., 2016; Pagan and Ramakrishnan, 2018). In the current study, we identified any cell containing three nuclei within a continuous cytoplasm as a multinucleated giant cell, regardless of morphology. These findings in contrast to other studies suggests this is a metric which warrants further investigation into type, function and to whom (host or microbe) this unique cell type benefits.
One limitation of this study was in interpreting coalescing lesions which had effaced the majority of the lymph node parenchyma. These cases arose occasionally in both vaccinates and non-vaccinates. This phenomenon is well document in lymph nodes of chronically infected cattle, where it may completely erase any semblance of a normal lymph node (Domingo et al., 2014). BCG provides variable protection, and animals with such severely affected lymph nodes may represent animals which failed to respond appropriately to the vaccine. It would be interesting to investigate differences present in these vaccine-non-responders and compare granuloma level cytokine and chemokine differences. As it was difficult to determine exactly how many granulomas coalesced, these lesions were counted as single, large granulomas. While minimally decreasing total granuloma numbers, these cases could dramatically alter average granulomas sizes.
Using banked samples from two previous experiments meant that treatments between the two experiments were not identical. The vaccination dose and challenge dose varied slightly between experimental groups, however as these were < 1 log, we felt it was an acceptable range. Enumeration of Mycobacterium bovis in liquid culture is difficult due to the propensity of the bacilli to clump (Gautam et al., 2022). As a result, simple optical density (OD) readings are not reliable, and due to the lengthy culture time, exact dosages are often determined retrospectively. Although the dosages between groups were not exactly the same, they were well within the range of numerous other BCG efficacy studies conducted by us and other investigators (Hope et al., 2005; Waters et al., 2007; Vordermeier et al., 2009).
Though not investigated in this study, it would be interesting to measure bacterial load between high- and low-grade granulomas between vaccination groups and how this may correlate with the metrics measured. While Ziehl-Neelson (ZN) staining for mycobacteria has historically been performed in histopathologic examinations of bTB it was not performed in this study due to limitations of the software and the relative insensitivity of ZN staining in formalin-fixed, paraffin-embedded tissues (Fukunaga et al., 2002). As quantitative culture would be impossible due to the small size of some of the lesions, techniques such as RNA in-situ hybridization, which would allow on-slide examination should be considered.
Our study provides further support to show that BCG vaccination reduced granuloma formation within the pulmonary lymph nodes of cattle following aerosol challenge with virulent M. bovis. Once established, BCG does not prevent the development of severe lesions, and the relative breakdown of high- and low- grade granulomas is similar to that of non-vaccinates. Despite this fact, BCG vaccinates have smaller granulomas with less tissue destruction than non-vaccinates. Fibrosis was higher in low-grade granulomas of vaccinates compared to non-vaccinates but this significance was lost when grade was excluded. Mineralization and MNGC density were similar between vaccinates and non-vaccinates, suggesting these may be vaccine-independent events.
The original contributions presented in the study are included in the article, further inquiries can be directed to the corresponding author.
The animal study was reviewed and approved by Institutional Animal Care and Use Committee at National Animal Disease Center.
CK, PB, and MP: experiment design. CK and MP: sample collection and experiments. CK and EP: data analysis. CK: manuscript preparation. CK, PB, EP, and MP: manuscript editing. All authors contributed to the article and approved the submitted version.
Financial support for these studies were provided by the United States Department of Agriculture, Agricultural Research Service Project (CRIS #5030–32000-222). This research did not receive any specific grant from funding agencies in the public, commercial, or not-for-profit sectors.
We would like to thank Judith Stasko and Adrienne Shircliff of the Agricultural Research Service’s Histology department for excellent and consistent slide handling and Adam Byersdorfer for HALO program support.
USDA is an equal opportunity provider and employer. Mention of trade names or commercial products in this publication is solely for the purpose of providing specific information and does not imply recommendation or endorsement by the U.S. Department of Agriculture.
The authors declare that the research was conducted in the absence of any commercial or financial relationships that could be construed as a potential conflict of interest.
All claims expressed in this article are solely those of the authors and do not necessarily represent those of their affiliated organizations, or those of the publisher, the editors and the reviewers. Any product that may be evaluated in this article, or claim that may be made by its manufacturer, is not guaranteed or endorsed by the publisher.
Basaraba, R. J. (2008). Experimental tuberculosis: the role of comparative pathology in the discovery of improved tuberculosis treatment strategies. Tuberculosis (Edinb.) 88, S35–S47. doi: 10.1016/S1472-9792(08)70035-0
Basaraba, R. J., and Orme, I. M. (2011). “Pulmonary tuberculosis in the Guinea pig” in A Color atlas of Comparative Pathology of Pulmonary Tuberculosis. eds. F. J. Leong, V. Dartois, and T. Dick (Boca Raton, FL, USA: CRC Press), 137–141.
Bayissa, B., Sirak, A., Worku, A., Zewude, A., Zeleke, Y., Chanyalew, M., et al. (2021). Evaluation of the efficacy of BCG in protecting against contact challenge with bovine tuberculosis in Holstein-Friesian and zebu crossbred calves in Ethiopia. Front. Vet. Sci. 8:702402. doi: 10.3389/fvets.2021.702402
Buddle, B. M., Keen, D., Thomson, A., Jowett, G., Mccarthy, A. R., Heslop, J., et al. (1995). Protection of cattle from bovine tuberculosis by vaccination with BCG by the respiratory or subcutaneous route, but not by vaccination with killed mycobacterium vaccae. Res. Vet. Sci. 59, 10–16. doi: 10.1016/0034-5288(95)90023-3
Buddle, B. M., Vordermeier, H. M., Chambers, M. A., and De Klerk-Lorist, L. M. (2018). Efficacy and safety of BCG vaccine for control of tuberculosis in domestic livestock and wildlife. Front. Vet. Sci. 5:259. doi: 10.3389/fvets.2018.00259
Buddle, B. M., Wedlock, D. N., Parlane, N. A., Corner, L. A., De Lisle, G. W., and Skinner, M. A. (2003). Revaccination of neonatal calves with Mycobacterium bovis BCG reduces the level of protection against bovine tuberculosis induced by a single vaccination. Infect. Immun. 71, 6411–6419. doi: 10.1128/IAI.71.11.6411-6419.2003
Canal, A. M., Pezzone, N., Cataldi, A., Zumarraga, M., Larzabal, M., Garbaccio, S., et al. (2017). Immunohistochemical detection of pro-inflammatory and anti-inflammatory cytokines in granulomas in cattle with natural Mycobacterium bovis infection. Res. Vet. Sci. 110, 34–39. doi: 10.1016/j.rvsc.2016.10.006
Canto Alarcon, G. J., Rubio Venegas, Y., Bojorquez Narvaez, L., Pizano Martinez, O. E., Garcia Casanova, L., Sosa Gallegos, S., et al. (2013). Efficacy of a vaccine formula against tuberculosis in cattle. PLoS One 8:e76418. doi: 10.1371/journal.pone.0076418
Ciaravino, G., Laranjo-Gonzalez, M., Casal, J., Saez-Llorente, J. L., and Allepuz, A. (2021). Most likely causes of infection and risk factors for tuberculosis in Spanish cattle herds. Vet. Rec. 189:e140. doi: 10.1002/vetr.140
De La Rua-Domenech, R., Goodchild, A. T., Vordermeier, H. M., Hewinson, R. G., Christiansen, K. H., and Clifton-Hadley, R. S. (2006). Ante mortem diagnosis of tuberculosis in cattle: a review of the tuberculin tests, gamma-interferon assay and other ancillary diagnostic techniques. Res. Vet. Sci. 81, 190–210. doi: 10.1016/j.rvsc.2005.11.005
Dean, G., Whelan, A., Clifford, D., Salguero, F. J., Xing, Z., Gilbert, S., et al. (2014). Comparison of the immunogenicity and protection against bovine tuberculosis following immunization by BCG-priming and boosting with adenovirus or protein based vaccines. Vaccine 32, 1304–1310. doi: 10.1016/j.vaccine.2013.11.045
Domingo, M., Vidal, E., and Marco, A. (2014). Pathology of bovine tuberculosis. Res. Vet. Sci. 97, S20–S29. doi: 10.1016/j.rvsc.2014.03.017
Fitzgerald, S. D., and Kaneene, J. B. (2013). Wildlife reservoirs of bovine tuberculosis worldwide: hosts, pathology, surveillance, and control. Vet. Pathol. 50, 488–499. doi: 10.1177/0300985812467472
Flynn, J. L. K. E. (2011). “Pulmonary tuberculosis in monkeys” in A color atlas of comparative pathology of pulmonary tuberculosis. eds. F. J. Leong, V. Dartois, and T. Dick (Boca Raton, FL, USA: CRC Press), 83–105.
Fukunaga, H., Murakami, T., Gondo, T., Sugi, K., and Ishihara, T. (2002). Sensitivity of acid-fast staining for mycobacterium tuberculosis in formalin-fixed tissue. Am. J. Respir. Crit. Care Med. 166, 994–997. doi: 10.1164/rccm.2111028
Gautam, U. S., Asrican, R., and Sempowski, G. D. (2022). Targeted dose delivery of mycobacterium tuberculosis in mice using silicon antifoaming agent via aerosol exposure system. PLoS One 17:e0276130. doi: 10.1371/journal.pone.0276130
Gharun, K., Senges, J., Seidl, M., Losslein, A., Kolter, J., Lohrmann, F., et al. (2017). Mycobacteria exploit nitric oxide-induced transformation of macrophages into permissive giant cells. EMBO Rep. 18, 2144–2159. doi: 10.15252/embr.201744121
Gideon, H. P., Hughes, T. K., Tzouanas, C. N., Wadsworth, M. H. 2nd, Tu, A. A., Gierahn, T. M., et al. (2022). Multimodal profiling of lung granulomas in macaques reveals cellular correlates of tuberculosis control. Immunity 55:e810, 827–846. doi: 10.1016/j.immuni.2022.04.004
Gupta, G., Athanikar, S. B., Pai, V. V., and Naveen, K. N. (2014). Giant cells in dermatology. Indian J. Dermatol. 59, 481–484. doi: 10.4103/0019-5154.139887
Hope, J. C., Thom, M. L., Villarreal-Ramos, B., Vordermeier, H. M., Hewinson, R. G., and Howard, C. J. (2005). Vaccination of neonatal calves with Mycobacterium bovis BCG induces protection against intranasal challenge with virulent M. bovis. Clin. Exp. Immunol. 139, 48–56. doi: 10.1111/j.1365-2249.2005.02668.x
Johnson, L., Gough, J., Spencer, Y., Hewinson, G., Vordermeier, M., and Wangoo, A. (2006). Immunohistochemical markers augment evaluation of vaccine efficacy and disease severity in bacillus Calmette-Guerin (BCG) vaccinated cattle challenged with Mycobacterium bovis. Vet. Immunol. Immunopathol. 111, 219–229. doi: 10.1016/j.vetimm.2006.01.016
Jones, G. J., Coad, M., Khatri, B., Bezos, J., Parlane, N. A., Buddle, B. M., et al. (2017). Tuberculin skin testing boosts interferon gamma responses to DIVA reagents in Mycobacterium bovis-infected cattle. Clin. Vaccine Immunol. 24:24. doi: 10.1128/CVI.00551-16
Kanipe, C., and Palmer, M. V. (2020). Mycobacterium bovis and you: a comprehensive look at the bacteria, its similarities to mycobacterium tuberculosis, and its relationship with human disease. Tuberculosis (Edinb.) 125:102006. doi: 10.1016/j.tube.2020.102006
Khalid, H., Van Hooij, A., Connelley, T. K., Geluk, A., and Hope, J. C. (2022). Protein levels of pro-inflammatory cytokines and chemokines as biomarkers of Mycobacterium bovis infection and BCG vaccination in cattle. Pathogens 11:738. doi: 10.3390/pathogens11070738
Losslein, A. K., Lohrmann, F., Scheuermann, L., Gharun, K., Neuber, J., Kolter, J., et al. (2021). Monocyte progenitors give rise to multinucleated giant cells. Nat. Commun. 12:2027. doi: 10.1038/s41467-021-22103-5
Martinot, A. J. (2018). Microbial offense vs host defense: who controls the TB granuloma? Vet. Pathol. 55, 14–26. doi: 10.1177/0300985817705177
Menin, A., Fleith, R., Reck, C., Marlow, M., Fernandes, P., Pilati, C., et al. (2013). Asymptomatic cattle naturally infected with Mycobacterium bovis present exacerbated tissue pathology and bacterial dissemination. PLoS One 8:e53884. doi: 10.1371/journal.pone.0053884
Nol, P., Palmer, M. V., Waters, W. R., Aldwell, F. E., Buddle, B. M., Triantis, J. M., et al. (2008). Efficacy of oral and parenteral routes of Mycobacterium bovis bacille Calmette-Guerin vaccination against experimental bovine tuberculosis in white-tailed deer (Odocoileus virginianus): a feasibility study. J. Wildl. Dis. 44, 247–259. doi: 10.7589/0090-3558-44.2.247
Nugent, G., Yockney, I. J., Cross, M. L., and Buddle, B. M. (2018). Low-dose BCG vaccination protects free-ranging cattle against naturally-acquired bovine tuberculosis. Vaccine 36, 7338–7344. doi: 10.1016/j.vaccine.2018.10.025
Pagan, A. J., and Ramakrishnan, L. (2018). The formation and function of granulomas. Annu. Rev. Immunol. 36, 639–665. doi: 10.1146/annurev-immunol-032712-100022
Palmer, M. V., Kanipe, C., and Boggiatto, P. M. (2022). The bovine Tuberculoid granuloma. Pathogens 11:061. doi: 10.3390/pathogens11010061
Palmer, M. V., Thacker, T. C., Kanipe, C., and Boggiatto, P. M. (2021). Heterogeneity of pulmonary granulomas in cattle experimentally infected with Mycobacterium bovis. Front. Vet. Sci. 8:671460. doi: 10.3389/fvets.2021.671460
Palmer, M. V., Thacker, T. C., and Waters, W. R. (2009). Vaccination with Mycobacterium bovis BCG strains Danish and Pasteur in white-tailed deer (Odocoileus virginianus) experimentally challenged with Mycobacterium bovis. Zoonoses Public Health 56, 243–251. doi: 10.1111/j.1863-2378.2008.01198.x
Palmer, M. V., Thacker, T. C., and Waters, W. R. (2016). Multinucleated giant cell cytokine expression in pulmonary granulomas of cattle experimentally infected with Mycobacterium bovis. Vet. Immunol. Immunopathol. 180, 34–39. doi: 10.1016/j.vetimm.2016.08.015
Palmer, M. V., Waters, W. R., and Thacker, T. C. (2007). Lesion development and immunohistochemical changes in granulomas from cattle experimentally infected with Mycobacterium bovis. Vet. Pathol. 44, 863–874. doi: 10.1354/vp.44-6-863
Palmer, M. V., Waters, W. R., and Whipple, D. L. (2002). Aerosol delivery of virulent Mycobacterium bovis to cattle. Tuberculosis (Edinb.) 82, 275–282. doi: 10.1054/tube.2002.0341
Ramakrishnan, L. (2012). Revisiting the role of the granuloma in tuberculosis. Nat. Rev. Immunol. 12, 352–366. doi: 10.1038/nri3211
Roy, A., Tome, I., Romero, B., Lorente-Leal, V., Infantes-Lorenzo, J. A., Dominguez, M., et al. (2019). Evaluation of the immunogenicity and efficacy of BCG and MTBVAC vaccines using a natural transmission model of tuberculosis. Vet. Res. 50:82. doi: 10.1186/s13567-019-0702-7
Russell, D. G., Barry, C. E. 3rd, and Flynn, J. L. (2010). Tuberculosis: what we don't know can, and does, hurt us. Science 328, 852–856. doi: 10.1126/science.1184784
Russell, D. G., Cardona, P. J., Kim, M. J., Allain, S., and Altare, F. (2009). Foamy macrophages and the progression of the human tuberculosis granuloma. Nat. Immunol. 10, 943–948. doi: 10.1038/ni.1781
Salguero, F. J., Gibson, S., Garcia-Jimenez, W., Gough, J., Strickland, T. S., Vordermeier, H. M., et al. (2017). Differential cell composition and cytokine expression within lymph node granulomas from BCG-vaccinated and non-vaccinated cattle experimentally infected with Mycobacterium bovis. Transbound. Emerg. Dis. 64, 1734–1749. doi: 10.1111/tbed.12561
Singhal, A. A. E. M., Creusy, C., Kaplan, G., and Bifani, P. (2011). “Pulmonary tuberculosis in the rat” in A color atlas of comparative pathology of pulmonary tuberculosis. eds. D. F. J. Leongv and T. Dick (Boca Raton, FL, USA: CRC Press), 166.
Sirak, A., Tulu, B., Bayissa, B., Gumi, B., Berg, S., Salguero, F. J., et al. (2021). Cellular and cytokine responses in lymph node granulomas of bacillus Calmette Guerin (BCG)-vaccinated and non-vaccinated Cross-breed calves naturally infected with Mycobacterium bovis. Front. Vet. Sci. 8:698800. doi: 10.3389/fvets.2021.698800
Sow, F. B., Gallup, J. M., Derscheid, R., Krishnan, S., and Ackermann, M. R. (2012). Ontogeny of the immune response in the ovine lung. Immunol. Investig. 41, 304–316. doi: 10.3109/08820139.2011.631657
Steinbach, S., Jalili-Firoozinezhad, S., Srinivasan, S., Melo, M. B., Middleton, S., Konold, T., et al. (2021). Temporal dynamics of intradermal cytokine response to tuberculin in Mycobacterium bovis BCG-vaccinated cattle using sampling microneedles. Sci. Rep. 11:7074. doi: 10.1038/s41598-021-86398-6
Trout, K. L., and Holian, A. (2020). Multinucleated giant cell phenotype in response to stimulation. Immunobiology 225:151952. doi: 10.1016/j.imbio.2020.151952
Vordermeier, H. M., Villarreal-Ramos, B., Cockle, P. J., Mcaulay, M., Rhodes, S. G., Thacker, T., et al. (2009). Viral booster vaccines improve Mycobacterium bovis BCG-induced protection against bovine tuberculosis. Infect. Immun. 77, 3364–3373. doi: 10.1128/IAI.00287-09
Wangoo, A., Johnson, L., Gough, J., Ackbar, R., Inglut, S., Hicks, D., et al. (2005). Advanced granulomatous lesions in Mycobacterium bovis-infected cattle are associated with increased expression of type I procollagen, gammadelta (WC1+) T cells and CD 68+ cells. J. Comp. Pathol. 133, 223–234. doi: 10.1016/j.jcpa.2005.05.001
Warsinske, H. C., Difazio, R. M., Linderman, J. J., Flynn, J. L., and Kirschner, D. E. (2017a). Identifying mechanisms driving formation of granuloma-associated fibrosis during mycobacterium tuberculosis infection. J. Theor. Biol. 429, 1–17. doi: 10.1016/j.jtbi.2017.06.017
Warsinske, H. C., Pienaar, E., Linderman, J. J., Mattila, J. T., and Kirschner, D. E. (2017b). Deletion of TGF-beta1 increases bacterial clearance by cytotoxic T cells in a tuberculosis granuloma model. Front. Immunol. 8:1843. doi: 10.3389/fimmu.2017.01843
Watanabe, Y., Watari, E., Matsunaga, I., Hiromatsu, K., Dascher, C. C., Kawashima, T., et al. (2006). BCG vaccine elicits both T-cell mediated and humoral immune responses directed against mycobacterial lipid components. Vaccine 24, 5700–5707. doi: 10.1016/j.vaccine.2006.04.049
Waters, W. R., Palmer, M. V., Nonnecke, B. J., Thacker, T. C., Scherer, C. F., Estes, D. M., et al. (2009). Efficacy and immunogenicity of Mycobacterium bovis DeltaRD1 against aerosol M. bovis infection in neonatal calves. Vaccine 27, 1201–1209. doi: 10.1016/j.vaccine.2008.12.018
Waters, W. R., Palmer, M. V., Nonnecke, B. J., Thacker, T. C., Scherer, C. F., Estes, D. M., et al. (2007). Failure of a mycobacterium tuberculosis DeltaRD1 DeltapanCD double deletion mutant in a neonatal calf aerosol M. bovis challenge model: comparisons to responses elicited by M. bovis bacille Calmette Guerin. Vaccine 25, 7832–7840. doi: 10.1016/j.vaccine.2007.08.029
Keywords: granuloma, Mycobacterium bovis, BCG, bovine tuberculosis, tuberculosis vaccine
Citation: Kanipe C, Boggiatto PM, Putz EJ and Palmer MV (2022) Histopathologic differences in granulomas of Mycobacterium bovis bacille Calmette Guérin (BCG) vaccinated and non-vaccinated cattle with bovine tuberculosis. Front. Microbiol. 13:1048648. doi: 10.3389/fmicb.2022.1048648
Received: 19 September 2022; Accepted: 24 October 2022;
Published: 08 November 2022.
Edited by:
Bernat Pérez de Val, IRTA-CReSA, Centre for Research on Animal Health, SpainReviewed by:
Joseph Cassidy, University College Dublin, IrelandCopyright © 2022 Kanipe, Boggiatto, Putz and Palmer. This is an open-access article distributed under the terms of the Creative Commons Attribution License (CC BY). The use, distribution or reproduction in other forums is permitted, provided the original author(s) and the copyright owner(s) are credited and that the original publication in this journal is cited, in accordance with accepted academic practice. No use, distribution or reproduction is permitted which does not comply with these terms.
*Correspondence: C. Kanipe, Y2FybHkua2FuaXBlQHVzZGEuZ292
Disclaimer: All claims expressed in this article are solely those of the authors and do not necessarily represent those of their affiliated organizations, or those of the publisher, the editors and the reviewers. Any product that may be evaluated in this article or claim that may be made by its manufacturer is not guaranteed or endorsed by the publisher.
Research integrity at Frontiers
Learn more about the work of our research integrity team to safeguard the quality of each article we publish.