- Department of Pediatrics, Qilu Hospital of Shandong University, Jinan, China
Gut microbiota includes a large number of microorganisms inhabiting the human gastrointestinal tract, which show a wide range of physiological functions, including digestion, metabolism, immunity, neural development, etc., and are considered to play an increasingly important role in health and disease. A large number of studies have shown that gut microbiota are closely associated with the onset and development of several diseases. In particular, the interaction between gut microbiota and cancer has recently attracted scholars’ attention. Acute leukemia (AL) is a common hematologic malignancy, especially in children. Microbiota can affect hematopoietic function, and the effects of chemotherapy and immunotherapy on AL are noteworthy. The composition and diversity of gut microbiota are important factors that influence and predict the complications and prognosis of AL after chemotherapy or hematopoietic stem cell transplantation. Probiotics, prebiotics, fecal microbiota transplantation, and dietary regulation may reduce side effects of leukemia therapy, improve response to treatment, and improve prognosis. This review concentrated on the role of the gut microbiota in the onset and development of AL, the response and side effects of chemotherapy drugs, infection during treatment, and therapeutic efficacy. According to the characteristics of gut microbes, the applications and prospects of microbial preparations were discussed.
Introduction
A large number of microorganisms, including bacteria, fungi, viruses, archaea, etc., are colonized in the human body surface and digestive tract, and the related genomes of these microorganisms are referred as microbiome (Fettweis et al., 2019). Among them, gut microbiota has the largest microbial community, including billions of types of microbes. The composition of gastrointestinal microbiota is influenced by heredity, environment, lifestyle, and other factors (Rothschild et al., 2018). Gut microbiota plays several roles in the host, such as digestion and absorption of food, synthesis of vitamins, short-chain fatty acids, essential amino acids and secondary metabolites, development and maturation of the immune system, involvement in the regulation of immune response and pathogen resistance, and even influencing our emotional and cognitive behaviors (de Vos et al., 2022).
Gut microbiota plays a fundamental role in maintaining homeostasis and intestinal integrity and is considered to play an increasingly important role in health and disease (Sekirov et al., 2010). In recent years, the gut microbiota has been reported to be closely correlated with various diseases, including intestinal diseases (e.g., inflammatory bowel disease), chronic metabolic diseases (e.g., diabetes), autoimmune diseases (e.g., rheumatoid arthritis), central nervous system diseases (e.g., Parkinson’s disease), respiratory diseases (e.g., asthma), carcinoma (e.g., hepatocellular carcinoma), etc. (Yu and Schwabe, 2017; Maeda and Takeda, 2019; Barcik et al., 2020; Gurung et al., 2020; Lavelle and Sokol, 2020; Kaur et al., 2021). Moreover, intestinal microbiota homeostasis and imbalance is an important factor affecting drug efficacy and side effects (Zhang et al., 2018). A growing body of evidence demonstrated that gut microbiota plays a role in the initiation, progression, and treatment of cancer. For instance, studies have shown that human microbiota is closely associated with the incidence of esophageal cancer, gastric cancer, hepatobiliary cancer, pancreatic cancer, colorectal cancer, lung cancer and other tapes of cancer (Vogtmann and Goedert, 2016).The present review, aimed to summarize the alterations of the gut microbiota in patients with acute leukemia (AL) and its effects on disease pathogenesis, progression, and treatment, and to discuss several approaches to improve leukemia outcomes by targeting the gut microbiota.
Possible relationships between gut microbiota and the leukemia initiation
The human gut microbiota is a complex microbial system. Previous research in the Human Microbiome Project has shown that more than 2,000 species have been isolated from the human gut microbiome (Fettweis et al., 2019), while only few of them can be found in all humans (Qin et al., 2010). The colony is composed of various types of microbiotas, whereas Bacteroides and Actinobacteria account for about 90% of the gut microbiota (Qin et al., 2010; Mandal et al., 2015). It was reported that the composition of gut microbiota in the population has certain similarities, and the onset and development of diseases many affect the composition of the gut microbiota. Studies demonstrated that pre-treatment with composition of gut microbiota can differentiate healthy individuals from cancer patients. Several sterile animal studies have shown that the microbiota promotes tumors in various organs, including skin, colon, liver, breast, lung, ect. (Sacksteder, 1976; Uronis et al., 2009; Kang et al., 2021; Matson et al., 2021). The analysis of metagenomic samples from different cancer patients showed that microbiota may be used as diagnostic bacterial markers in diverse diseases, such as Bacteroides fragilis and Fusobacterium nucleatum in colorectal cancer, and the kkermansia muciniphila, Rikenellaceae and Bacteroides et al. in non-small cell lung cancer (Cheng et al., 2020; Vernocchi et al., 2020).
Several studies have shown that microbial dysbiosis was associated with the initiation of various tapes of cancer. To explore the relationship between gut microbiota and AL, it was attempted to summarize some of the studies on microbiota in leukemia (Table 1). Several clinical studies have demonstrated a structural imbalance in the gut microbiota of acute lymphoblastic leukemia (ALL) patients before treatment compared with healthy controls. Shannon index, which summarizes taxonomic richness (i.e., the number of species represented) and evenness (i.e., the degree to which species represented are similar in frequency), is one of the most common measures of alpha diversity (i.e., within-sample diversity) reported in the gut microbiota literature and is considered as a marker of the health of gut microbiota. This conclusion was confirmed by performing a meta-analysis of the Shannon index results from six studies (Supplementary Figures 1, 2). These findings verified the interaction between gut microbiota and AL initiation and progression. However, the results regarding the alterations of specific microbiota genus were inconsistent. It may be related to the inconsistent sequencing depth of different studies and the small sample size of a single study, indicating the necessity of more standardized large-scale deep sequencing samples for analysis in the future.
When gut microbiota dysbiosis causes the destruction of intestinal epithelial barrier, some gut microbiota enters the blood or local lymph nodes, leading to an inflammatory immune response through metabolic disorders, activation of immune cells and changes in key intracellular signaling pathways, which may contribute to the development of cancer (Ivanov et al., 2008; Gaboriau-Routhiau et al., 2009; Tsilimigras et al., 2017). A study found that bacterial composition was significantly different between an xenotransplant pediatric ALL mouse model and control group in feces and small intestine, such as the increased proportion of bacteria with conversion function of dietary flavonoids in a mouse model of ALL (Song and Gyarmati, 2020). Dietary bioflavonoids were demonstrated to induce mixed lineage leukemia (MLL) gene cleavage by targeting topoisomerase II, and they may lead to childhood leukemia (Strick et al., 2000), indicating that the abnormal composition of gut microbiota is associated with the pathogenesis of leukemia. Changes in the gut microbiota may also be associated with genetic susceptibility to ALL, in mouse models of ALL, and differences in its composition were detected between healthy mice and mice genetically predisposed to leukemia (Vicente-Dueñas et al., 2020).
Relationship between gut microbiota and the treatment of acute leukemia
Chemotherapy
The correlations of chemotherapeutic drugs and gut microbiota are mutual. Chemotherapy drugs can directly act on the gut microbiota or damage the intestinal epithelial cells and destroy the intestinal barrier, resulting in the imbalance of the microbiota (Figure 1). Similarly, dysregulated gut microbiota may affect the absorption and metabolism of chemotherapeutic drugs, increasing their toxicity and reducing their efficacy.
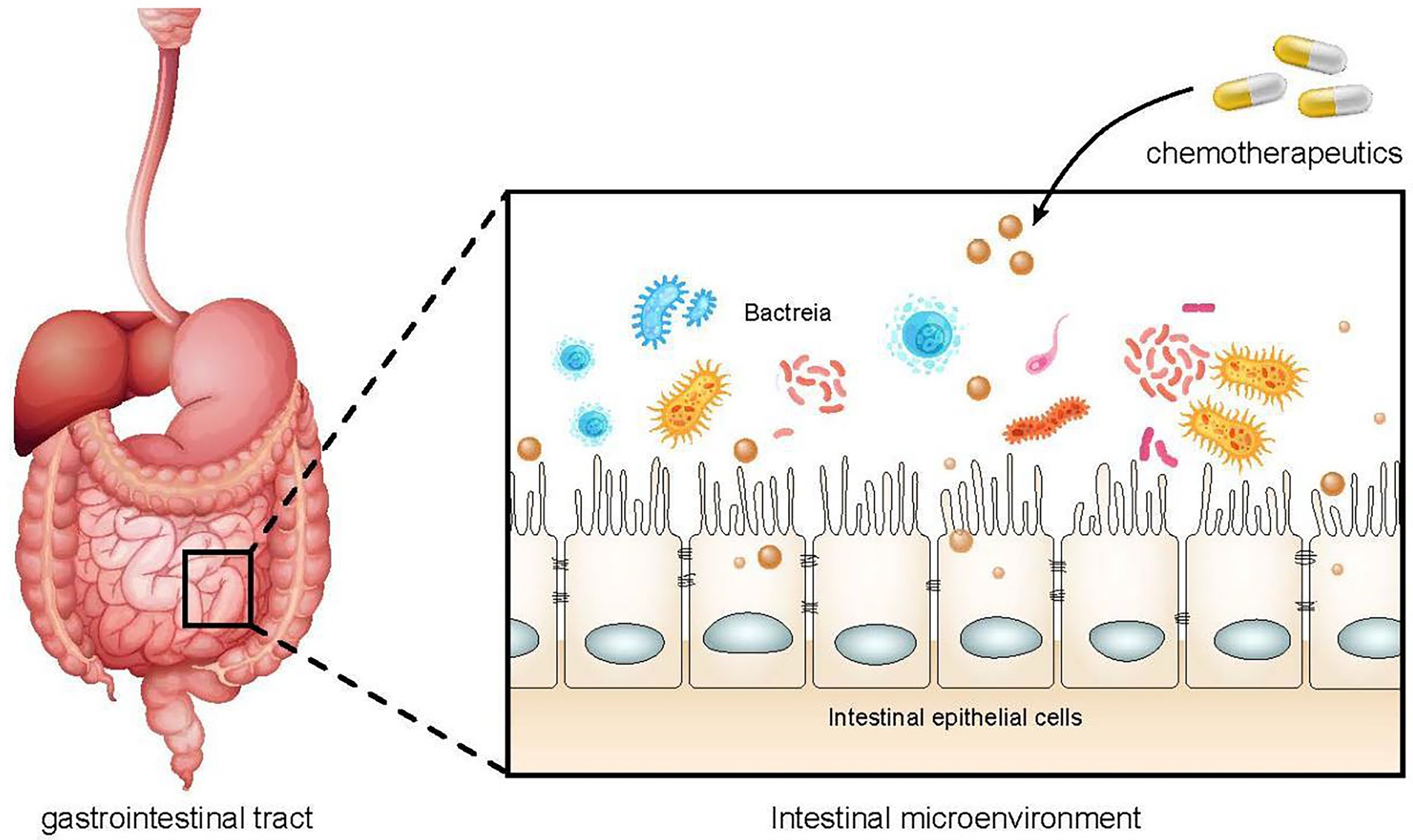
Figure 1. The effect of chemotherapy drugs on gut microbiota. The use of chemoherapeutic drugs can damage intestinal epithelial cells, alter intestinal permeability, and reduce the protective function of the intestinal barrier, resulting in intestial dysbiosis and an increased risk of infection.
A growing body of evidence demonstrated that gut microbiota is closely associated with drug efficacy and side effects, and the interaction between gut microbes and commonly used non-antibiotic drugs is mutual: the composition of the gut microbiome can be affected by drugs, and vice versa. The gut microbiome can also influence an individual’s response to a drug by enzymatically altering its structure and altering its bioavailability, bioactivity, or toxicity, which is called pharmacomicrobiology (Lucafo et al., 2020). However, gut microbiota can also directly influence an individual’s response to chemotherapeutic drugs in the treatment of AL. Methotrexate (MTX) is one of the most clinically crucial and potent anticancer drugs, which is widely used for the treatment of leukemia due to blocking folate metabolism. MTX can induce distinct intestinal impairment, including the atrophy of jejunal villi, increasing goblet cells, and collapse of muscularis mucosa. Moreover, the MTX-induced intestinal mucosal injury promotes a series of inflammatory responses, including the increase of inflammatory factors, and dysregulation of macrophages (increase of M1 phenotype and decrease of M2 phenotype) in lymph nodes and spleen. Besides, MTX can gradually induce an alteration in population, diversity, and composition of the gut microbiota in mice, especially the distinct decrease of Bacteroidales. Bacteroides fragilis also significantly decreases, whereas the Bacteroides fragilis supplementation can ameliorate the inflammatory response and polarization imbalance of macrophages induced by MTX (Zhou et al., 2018). Changes in the gut microbiota were also observed in some in vivo experiments in mice models (Huang et al., 2020; Letertre et al., 2020).
Cyclophosphamide (CTX) is another broad-spectrum antitumor drug and has a curative effect on leukemia and solid tumors. One important study demonstrated that the intestinal epithelial barrier was impaired after treatment with non-high-dose CTX in mice, which was manifested by discontinuity of epithelial barrier, shortening of villi, interstitial edema, focal accumulation of monocytes in lamina propria, and increase of goblet cells and Paneth cells (Viaud et al., 2013). Daillère et al. (2016) identified two bacterial species, Enterococcus hirae and Barnesiella intestinihominis that are involved in CTX therapy. Whereas E. hirae could be translocated from the small intestine to secondary lymphoid organs and increased the intratumoral CD8/Treg ratio. Barnesiella intestinihominis was accumulated in the colon and promoted the infiltration of interferon gamma (IFN-γ)-producing gamma delta T (γδT) cells in cancer lesions, and they can improve the efficacy of the most common alkylated immunomodulatory compounds. The above mentioned pathological changes increase the intestinal permeability, facilitate intestinal bacterial translocation, and eventually lead to bloodstream infection (BSI). Viaud et al. (2013) also found a significant change of the microbiota composition in the small intestine and a significant translocation of commensal bacteria in most mice treated with CTX, and some gram-positive bacteria species (e.g., Lactobacillus johnsonii, Lactobacillus murinus and Enterococcus hirae) were detected in lymphoid organs such as mesenteric lymph nodes and spleens. Furthermore, a previous study indicated that gut microbiota was correlated with the antitumor effect induced by chemotherapeutic agents. CTX induced the conversion of CD4+ T cells into type-1 T helper (Th1) and Th17 cells, which is closely associated with gram-positive bacterial species present in the small intestine and lymphatic organs. Antibiotic treatment can decrease the CTX-mediated inhibition of tumor growth, whereas adoptive transfer of pathogenic Th17 (pTh17) cells can eliminate this negative effect of antibiotics, suggesting that gut microbiota may contribute to CTX-mediated anticancer immune responses through pTh17 cells, and gram-positive bacteria may contribute to the formation of anticancer immune responses by stimulating Th17 cells (Viaud et al., 2013). A recent large-scale study (Zimmermann et al., 2019) involving 76 diverse human gut bacteria and 271 oral drugs indicated that the metabolic effects of specific gut bacteria on drugs have a significant influence on chemotherapy. In this study, dexamethasone was metabolized by Clostridium scindens in vitro, and it was metabolized (side-chain cleaving) by an intestinal microbe in vivo, which may influence the serum metabolism level. Other corticosteroids, such as prednisone, may be metabolized by Clostridium scindens. Their findings suggested that the metabolism of several drugs is chemically modified by microbiota. High-throughput gene sequencing was combined with mass spectrometry to systematically identify microbial gene products that metabolize drugs, and drug metabolic activities of human gut bacteria and microbiota can be predicted based on their genomic contents. When the interpersonal variation of microbiota is correlated with differences in drug metabolism, drug responses may be safer and more efficient through adjustment of exogenous microorganisms.
Immunotherapy
Although chemotherapy and hematopoietic stem cell transplantation for AL have achieved a remarkable therapeutic efficacy, some patients still encounter with troubles, such as primary or secondary drug-resistance, relapse after remission, and serious complications. In recent years, novel cancer immunotherapeutic methods with a high specificity and a low toxicity have shown a great potential for the treatment of refractory or relapsed AL. At present, the main immunotherapy for AL contains chimeric type of antigen receptor (CAR) T cell therapy, antibody-drug conjugate (ADC), bispecific T cell engager (BiTE), immune checkpoint inhibitors (ICIs), natural killer (NK) cell therapy, dendritic cell (DC) therapy, etc. A growing body of evidence showed that microbiota may alter the response to cancer immunotherapy. ICIs prevent the binding of inhibitory molecules (e.g., CTLA4, PD-1/PD-L1, LAG-3, and TIM-3) to their ligand receptors to activate the suppressed immune system and promote the removal of malignant cells (Seidel et al., 2018). A remarkable study demonstrated that the composition of gut microbiota (distinct Bacteroides species) affected the anti-tumor efficacy of anti-CTLA4 in mice with tumor. It was found that in mice and patients, T cell responses specific for B. thetaiotaomicron or B. fragilis were associated with the efficacy of CTLA-4 blockade. Tumors in antibiotic-treated or germ-free mice did not respond to CTLA blockade. This defect was overcome by gavage with B. fragilis, by immunization with B. fragilis polysaccharides, or by adoptive transfer of B. fragilis–specific T cells. Fecal microbes from individuals to mice transplanted confirmed that treatment of melanoma patients with anti-CTLA-4 antibody is beneficial to the growth of B. fragilis and has anticancer properties (Vétizou et al., 2015). Another study reported that special taxa can promote the anti-tumor efficacy of anti-PD-L1 in mice with melanoma (Sivan et al., 2015). Studies confirmed that a higher diversity of gut microbiota and a higher abundance of particular taxa were associated with positive responses to ICIs in patients with different types of cancer, such as melanoma, non-small cell lung carcinoma, renal cell carcinoma, and urothelial carcinoma (Dubin et al., 2016; Frankel et al., 2017; Routy et al., 2018; Jin et al., 2019). Kuczma et al. found that the loss of gut microbiota caused by administration of antibiotics reduced the therapeutic efficacy of adoptive T cell therapy in mice with colorectal tumors, while did not affect the efficacy of CD19-targeted CAR-T cell therapy in mice with B-cell lymphoma (Kuczma et al., 2017). Abid et al. speculated that gut microbiota may potentially enhance CAR-T response without clear evidence (Abid et al., 2019). Additional relevant studies are required to confirm the above-mentioned assumption. Establishment of a mouse model of liver cancer revealed that the altered gut microbiota induced by vancomycin might have anti-cancer effects by increasing the number of hepatic NKT cells and reducing the level of secondary bile acid metabolism (Ma et al., 2018). To explore the role of the gut microbiota in response to CD19-targeted CAR-T cell therapy, Smith et al. (2022) first conducted a multicenter retrospective study, and it was found that the antibiotic exposure of patients receiving CAR-T cell therapy was associated with worse overall survival (OS). Then, they prospectively collected stool samples from 48 patients with leukemia or lymphoma, and demonstrated that patients receiving CAR-T cell therapy with an abnormally low alpha diversity and a higher abundance of Ruminococcus, Bacteroides and Faecalibacterium were associated with the higher efficacy of CD19-targeted CAR-T cell therapy. Moreover, additional microbiota data at different treatment time points (e.g., after CAR-T cell therapy and during follow-up) may assist clinicians to better define the role of gut microbiota in CAR-T cell therapy. Further studies should also investigate specific mechanisms of fecal microbiota regulation in preclinical models, such as animal and cell experiments, to explore the interaction of bacterial taxa and bacterial metabolites in the immune system, in order to improve patient outcomes after immunotherapy.
Effects of gut microbiota on the prognosis of acute leukemia
To our knowledge, chemotherapy is one of the treatment options for AL worldwide, and all pediatric and adult cases with AL receive chemotherapy. However, intensive chemotherapy may have a long-term effect on the gastrointestinal microbiota through a direct damage to the intestinal tract and an indirect influence of the immune system, and the therapeutic or preventive antibiotics used during chemotherapy may also affect the gut microbiota. Thus, the gut microbiota of AL patients may significantly vary after chemotherapy, which has been confirmed by a number of studies. Hakim et al. (2018) found that the diversity of gut microbiota significantly decreased and the abundance of some bacteria, such as Bacteroidetes, reduced, whereas that of other bacteria, such as Clostridiaceae and Streptococcaceae, increased in 199 children with ALL who underwent intensive induction. Multiple studies have also shown that the diversity of gut microbiota decreased during induction chemotherapy in children with ALL, especially Bacteroides genus, and the composition of gut microbiota was recovered to the same as that of healthy group after cessation of thermotherapy, while partly accompanied by different levels of abundance (Chua et al., 2020; De Pietri et al., 2020). In addition, similar studies on patients with acute myelocytic leukemia (AML) have yielded consistent results. These studies have found a significant decrease in alpha diversity and beta diversity of gut microbiota in patients with AML after chemotherapy, which is associated with the use of antibiotics, particularly carbapenems (Galloway-Peña et al., 2016; Galloway-Pena et al., 2017; Galloway-Peña et al., 2020). Furthermore, a growing body of evidence demonstrated that the altered gut microbiota is associated with complications after chemotherapy, affecting the prognosis of leukemia patients. The main complications caused by chemotherapy include organ toxicity, neutropenia, infections, gastrointestinal dysfunction, etc. Among them, BSI due to chemotherapy-induced myelosuppression is a common complication and an important cause of high mortality of AL. Song et al. found an increased proportion of opportunistic pathogens (including Staphylococcus, Streptococcus, Ralstonia, and Lactococcus) in the feces of ALL mice compared with control mice (Song and Gyarmati, 2020). Once antitumor treatment is initiated, owing to the damage to intestinal epithelial barrier, these pathogens are susceptible to translocation to cause bacteremia and even resistant bacterial infections. Numerous AL-based cohort studies have found that baseline microbiota diversity and composition characteristics prior to chemotherapy are independent predictors of infections during induction chemotherapy. For instance, two studies (Galloway-Peña et al., 2016, 2020) demonstrated that baseline alpha diversity can predict infections during chemotherapy for AML patients. Febrile neutropenia is a common complication in patients with hematologic malignancies receiving chemotherapy, and is associated with high morbidity and mortality (Hakim et al., 2018). A study revealed that the abundance of Proteobacteria prior to treatment can predict febrile neutropenia. Besides, changes in gut microbiota are associated with the severity of systemic inflammation and intestinal epithelial loss during chemotherapy (De Pietri et al., 2020). The domination of Enterococcaceae or Streptococcaceae predicted a higher risk of subsequent infection in ALL patients (Hakim et al., 2018), and a decrease in alpha and beta diversities was corrected with an increased risk of infection within 6 months after therapy (Nearing et al., 2019). Increased temporal variability of gut microbiota was associated with a higher risk of infection at 90 days after induction in AML patients (Galloway-Pena et al., 2017). Therefore, assessing the risk of subsequent infection based on an individual’s microbiota characteristics and timely effective prevention or treatment may reduce mortality in AL patients.
Allogeneic hematopoietic stem cell transplantation (allo-HSCT) is a crucial treatment for AL, especially for patients with relapsed or refractory ALL. It has been found that there are associations between gut microbiota and life-threatening major complications of HSCT, such as graft-versus-host disease (GVHD), infections, and relapse. During allo-HSCT, gastrointestinal mucosa was damaged and intestinal colonizing bacteria were destroyed, resulting in the decrease of microbial diversity. In a cohort study of 80 patients undergoing HSCT, a lower diversity of gut microbiota was related to a higher mortality, suggesting that the microbial diversity was a strong independent predictor of mortality (Taur et al., 2014). Another similar study found that the increased bacterial diversity and genus Blautia were correlated with the reduced mortality (Jenq et al., 2015). Several studies have shown that the phylogenetic and functional alterations in gut microbiota were associated with the onset or severity of acute GVHD after allo-HSCT, such as the reduced diversity, some specific bacteria (Lachnospiraceae, Blautia genus, Ruminococcaceae, and anti-inflammatory Clostridia), and metabolic changes (the reduced levels of short-chain fatty acid: acetate, propionate, butyrate), which can serve as diagnostic predictors (Doki et al., 2017; Golob et al., 2017; Liu et al., 2017; Mancini et al., 2017; Simms-Waldrip et al., 2017; Payen et al., 2020; Zhou et al., 2020). An important multivariate analysis indicated that a high abundance of Lactobacillaceae and a high concentration of human beta-defensin 2 (HBD2) before HSCT were associated with moderate or severe acute GVHD and a high mortality post-HSCT; a high abundance of obligate anaerobes and a rapid recovery of B and NK cells were associated with no or mild acute GVHD and a low mortality post-HSCT; a high abundance of facultative anaerobic bacteria was associated with chronic inflammation (Ingham et al., 2019). Taur et al. pointed out that the reduced diversity of gut microbiota and dominance of opportunistic pathogens, including Enterococcus, Streptococcus, and Proteobacteria, significantly increased the risk of bacteremia during allo-HSCT (Taur et al., 2012). In a large cohort study (541 patients), Peled et al. reported that the presence or a higher abundance of a bacterial group mainly consisting of Eubacterium limosum has a significant relationship with a lower risk of relapse in patients undergoing allo-HSCT (Peled et al., 2017). Therefore, the diversity or composition of gut microbiota or specific species may be regarded as potential biomarkers to predict and prevent complications and prolong OS after allo-HSCT.
Implications of microbiota-based treatment for acute leukemia
In summary, the microbiota is altered in the onset and treatment process of leukemia, and the alterations may affect the anti-leukemia treatment and prognosis, so as to improve the prognosis of patients by restoring the altered microbiota. There are several strategies to adjust the gut microbiota, so that the altered gut microbiota may returns to a healthy state or tend to change in favor of the patient.
Probiotics
Probiotics are active microorganisms that confer health benefits on host by decreasing intestinal dysbiosis, promoting nutrient absorption, protecting intestinal mucosal barrier, and modulating immunity or inhibiting intestinal inflammation. Probiotics have been widely used to manage intestinal side effects caused by antibiotics or other factors. A study demonstrated that a probiotic (Lactobacillus) can regulate the diversity and abundance of gut microbiota in mice that received 5-fluorouracil (5-FU; Yeung et al., 2020). In a randomized controlled trial involving 60 children with AL who received chemotherapy, the group of patients who took oral probiotics (Lactobacillus rhamnosus) daily showed a significant reduction in most gastrointestinal side effects, including vomiting, nausea, abdominal distension, flatulence, constipation, and abdominal pain (Reyna-Figueroa et al., 2019). In a systematic review, the analysis of 11 studies including 1,557 patients has shown that probiotics reduce both frequency and severity of diarrhea in cancer patients (Redman et al., 2014). However, a meta-analysis of 1,091 patients showed that prophylactic probiotics were not effective in reducing diarrhea induced by any cancer therapy (Wardill et al., 2018). Therefore, there is no consistent conclusion about the side effects of probiotics in cancer patients, and further research needs to be conducted to draw definitive conclusions.
Limited supplementation of probiotics may not be enough to restore gut microbiota dysbiosis secondary to AL, while according to the patient’s specific chemotherapy regimen and changes in the gut microbiota, the selection of individualized probiotics may be beneficial to patients, and the prognosis of the patient’s disease and treatment-related side effects may be improved.
Fecal microbiota transplantation
Fecal microbiota transplantation (FMT) is an infusion of fecal suspension containing healthy donor microbiota into the gastrointestinal tract of the recipient. FMT has been given as self-administered or medically administered enemas, oral capsules, via nasogastric tube, or instilled into the duodenum by upper endoscopy or the right colon by colonoscopy or cecostomy. It can directly change the intestinal microbiota of the recipient and restore its normal composition, thus obtaining therapeutic effects. FMT has been highly regarded since it was approved by the US Food and Drug Administration in 2013 for the treatment of recurrent and refractory Clostridium difficile infections, and since then, its application has expanded rapidly and widely, not only for gastrointestinal related diseases, but also for parenteral diseases. For different hosts and diseases, FMT can be personalized to different patients and conditions (Vindigni and Surawicz, 2017; Wang J. W. et al., 2019; Tkach et al., 2022).
Studies have shown that the post-antibiotic intestinal microbiota of the human and murine can recover more quickly with autologous FMT than with multi-strain probiotics (Suez et al., 2018). Therefore, FMT is a faster and more effective method to restore the altered gut microbiota, and many clinical trials have confirmed the therapeutic effect of FMT. In a clinical trial including four acute leukemia patients undergoing allo-HSCT, who suffered from refractory diarrhea because of refractory intestinal infection or intestinal GVHD, FMT was a successful and safe treatment (Wang Q. et al., 2019). In addition, FMT is recommended for the treatment of multiple recurrent Clostridioides difficile infection (CDI) in adult and children who have failed appropriate antimicrobial therapy (McDonald et al., 2018). A case report presented a successful FMT treatment in a 27 year-old AML patient with prolonged and severe neutropenia. The patient developed fulminant CDI refractory to maximal antibiotic treatment, then appeared high fever, diffuse abdominal pain, watery diarrhea, colitis and other serious clinical symptoms, whereas his clinical symptoms improved after FMT therapy without further recurrence.(Lee et al., 2020) Two other similar case studies also reported that FMT was successful in eliminating multidrug-resistant pathogens and treating recurrent CDI after allo-HSCT in ALL patients (de Castro et al., 2015; Innes et al., 2017). In addition, FMT intervention achieved good results in restoring microbial diversity and reconstructing gut microbiota composition after HSCT (DeFilipp et al., 2018; Taur et al., 2018), as well as in treating four patients with steroid-resistant gut aGVHD (Kakihana et al., 2016). In order to evaluate the efficacy of autologous fecal microbiota transfer (AFMT) in dysbiosis correction and multidrug-resistant bacteria eradication, 25 AMl patients treated with (de Vos et al., 2022) intensive chemotherapy and antibiotics were recruited in a multicenter study, they showed that AFMT appears to be safe and effective for gut microbiota restoration in patients receiving intensive induction chemotherapy and antibiotic for AML (Malard et al., 2021).
Diet regulation and prebiotics
In 2017, the International Scientific Association for Probiotics and Prebiotics (ISAPP) consensus panel defined a prebiotic as follows: a substrate that is selectively utilized by host microorganisms conferring a health benefit (Gibson et al., 2017). Some substances present in the diet, such as soybean extracts, koji glycosylceramides, grape extracts, tea polyphenols, and seaweed extracts, can be considered as potential prebiotics, because they can selectively stimulate the proliferation of beneficial bacteria in the intestine (Cheng et al., 2022). The effects of prebiotics are indirect, and their positive effects are exerted by affecting the composition of gut microbiota.
A recent study suggested that the use of appropriate prebiotics during chemotherapy may reduce the therapeutic side effects. Establishment of a mouse model revealed that short-term dietary restriction significantly alleviated the intestinal fatal damage in mice after high-dose MTX exposure by increasing the Lactobacillus genus to reduce intestinal inflammation and protect PCNA-positive cells in basal crypt, as well as the function of intestinal stem cells (Tang et al., 2020). Importantly, Lactobacillus rhamnosus supplementation plays the same role as dietary restriction, suggesting that dietary regulation is a viable method to restore the altered microbiota after treatment. Taskin et al. (2017) found in a study on mice that lactulose significantly improved MTX-induced liver damage. Similarly, a recent study conducted by Zhou et al. (2021) pointed out that polysaccharides and ginsenosides extracted from American ginseng had a certain protective effect on CTX therapy. They restored the composition of gut microbiota and increased various beneficial mucosa-associated bacterial taxa (Clostridiales, Bifidobacterium, and Lachnospiraceae), while decreased harmful bacteria (Escherichia-Shigella and Peptococcaceae). Besides, specific nutrients may play an important role in adjusting gut microbiota. For instance, a study found that melatonin supplementation increased the diversity of gut microbiota and regulated the composition, such as enrichment of the abundance of Lactobacillus in mice (Ren et al., 2018), indicating that supplementing food enriched in melatonin is conducive to the early reconstruction of gut microbiota after chemotherapy. Another study confirmed that soy-whey blended protein can significantly increase the diversity of gut microbiota and effectively improve muscle status in AL survivors (Ren et al., 2020). In addition, enteral nutrition was given to promptly restore the gut microbiota post-HSCT (D'Amico et al., 2019). Therefore, a proper diet and supplementation of prebiotics are also an alternative treatment for the altered gut microbiota.
Conclusion
Recent studies have explored the role of gut microbiota in AL. Gut microbiota contributes to the risk of leukemia by interacting with the immune response. Gut microbiota may affect the therapeutic efficacy by influencing the metabolism of chemotherapeutic drugs and improving the patient’s response to immunotherapy. In turn, these anti-cancer treatments affect the diversity and composition of gut microbiota. The diversity and abundance of gut microbiota or specific taxa can be used as strong independent predictors for infection, recurrence, GVHD, and death in AL patients receiving therapy. It is potential to treat AL by regulating gut microbiota. Microbiota replacement therapies and supplementing microbiota with specific probiotics and dietary regulation have become effective measures to prevent or treat leukemia-associated complications and to improve prognosis of patients with AL. However, it remains elusive which specific taxa may play a key role in initiation, progression, and treatment of AL. The exploration of these special microbiota needs further study in the future.
Author contributions
CZ drafted the manuscript. YZ revised the manuscript and prepared illustrations. AZ conceived the topic and critically revised the manuscript. All authors contributed to the article and approved the submitted version.
Funding
This study was financially supported by Key Research and Development Program of Shandong Province (grant no. 2019GSF108060) and Shandong Provincial Natural Science Foundation (grant no. ZR202010220039).
Conflict of interest
The authors declare that the research was conducted in the absence of any commercial or financial relationships that could be construed as a potential conflict of interest.
Publisher’s note
All claims expressed in this article are solely those of the authors and do not necessarily represent those of their affiliated organizations, or those of the publisher, the editors and the reviewers. Any product that may be evaluated in this article, or claim that may be made by its manufacturer, is not guaranteed or endorsed by the publisher.
Supplementary material
The Supplementary material for this article can be found online at: https://www.frontiersin.org/articles/10.3389/fmicb.2022.1045497/full#supplementary-material
References
Abid, M. B., Shah, N. N., Maatman, T. C., and Hari, P. N. (2019). Gut microbiome and CAR-T therapy. Exp. Hematol. Oncol. 8:31. doi: 10.1186/s40164-019-0155-8
Bai, L., Zhou, P., Li, D., and Ju, X. (2017). Changes in the gastrointestinal microbiota of children with acute lymphoblastic leukaemia and its association with antibiotics in the short term. J. Med. Microbiol. 66, 1297–1307. doi: 10.1099/jmm.0.000568
Barcik, W., Boutin, R. C. T., Sokolowska, M., and Finlay, B. B. (2020). The role of lung and gut microbiota in the pathology of asthma. Immunity 52, 241–255. doi: 10.1016/j.immuni.2020.01.007
Bhuta, R., DeNardo, B., Wang, J., Atoyan, J., Zhang, Y., Nelson, D., et al. (2021). Durable changes in the gut microbiome in survivors of childhood acute lymphoblastic leukemia. Pediatr. Blood Cancer 68:e29308. doi: 10.1002/pbc.29308
Cheng, L., Kong, L., Xia, C., Zeng, X., Wu, Z., Guo, Y., et al. (2022). Sources, processing-related transformation, and gut Axis regulation of conventional and potential prebiotics. J. Agric. Food Chem. 70, 4509–4521. doi: 10.1021/acs.jafc.2c00168
Cheng, W. Y., Wu, C. Y., and Yu, J. (2020). The role of gut microbiota in cancer treatment: friend or foe? Gut 69, 1867–1876. doi: 10.1136/gutjnl-2020-321153
Chua, L. L., Rajasuriar, R., Azanan, M. S., Abdullah, N. K., Tang, M. S., Lee, S. C., et al. (2017). Reduced microbial diversity in adult survivors of childhood acute lymphoblastic leukemia and microbial associations with increased immune activation. Microbiome 5:35. doi: 10.1186/s40168-017-0250-1
Chua, L. L., Rajasuriar, R., Lim, Y. A. L., Woo, Y. L., Loke, P., and Ariffin, H. (2020). Temporal changes in gut microbiota profile in children with acute lymphoblastic leukemia prior to commencement-, during-, and post-cessation of chemotherapy. BMC Cancer 20:151. doi: 10.1186/s12885-020-6654-5
Daillère, R., Vétizou, M., Waldschmitt, N., Yamazaki, T., Isnard, C., Poirier-Colame, V., et al. (2016). Enterococcus hirae and Barnesiella intestinihominis facilitate cyclophosphamide-induced therapeutic immunomodulatory effects. Immunity 45, 931–943. doi: 10.1016/j.immuni.2016.09.009
D'Amico, F., Biagi, E., Rampelli, S., Fiori, J., Zama, D., Soverini, M., et al. (2019). Enteral nutrition in pediatric patients undergoing hematopoietic SCT promotes the recovery of gut microbiome homeostasis. Nutrients 11. doi: 10.3390/nu11122958
de Castro, C. G., Ganc, A. J., Ganc, R. L., Petrolli, M. S., and Hamerschlack, N. (2015). Fecal microbiota transplant after hematopoietic SCT: report of a successful case. Bone Marrow Transplant. 50:145. doi: 10.1038/bmt.2014.212
De Pietri, S., Ingham, A. C., Frandsen, T. L., Rathe, M., Krych, L., Castro Mejia, J. L., et al. (2020). Gastrointestinal toxicity during induction treatment for childhood acute lymphoblastic leukemia: the impact of the gut microbiota. Int. J. Cancer 147, 1953–1962. doi: 10.1002/ijc.32942
de Vos, W. M., Tilg, H., Van Hul, M., and Cani, P. D. (2022). Gut microbiome and health: mechanistic insights. Gut 71, 1020–1032. doi: 10.1136/gutjnl-2021-326789
DeFilipp, Z., Peled, J. U., Li, S., Mahabamunuge, J., Dagher, Z., Slingerland, A. E., et al. (2018). Third-party fecal microbiota transplantation following Allo-HCT reconstitutes microbiome diversity. Blood Adv. 2, 745–753. doi: 10.1182/bloodadvances.2018017731
Doki, N., Suyama, M., Sasajima, S., Ota, J., Igarashi, A., Mimura, I., et al. (2017). Clinical impact of pre-transplant gut microbial diversity on outcomes of allogeneic hematopoietic stem cell transplantation. Ann. Hematol. 96, 1517–1523. doi: 10.1007/s00277-017-3069-8
Dubin, K., Callahan, M. K., Ren, B., Khanin, R., Viale, A., Ling, L., et al. (2016). Intestinal microbiome analyses identify melanoma patients at risk for checkpoint-blockade-induced colitis. Nat. Commun. 7:10391. doi: 10.1038/ncomms10391
Fettweis, J. M., Serrano, M. G., Brooks, J. P., Edwards, D. J., Girerd, P. H., Parikh, H. I., et al. (2019). The vaginal microbiome and preterm birth. Nat. Med. 25, 1012–1021. doi: 10.1038/s41591-019-0450-2
Frankel, A. E., Coughlin, L. A., Kim, J., Froehlich, T. W., Xie, Y., Frenkel, E. P., et al. (2017). Metagenomic shotgun sequencing and unbiased Metabolomic profiling identify specific human gut microbiota and metabolites associated with immune checkpoint therapy efficacy in melanoma patients. Neoplasia 19, 848–855. doi: 10.1016/j.neo.2017.08.004
Gaboriau-Routhiau, V., Rakotobe, S., Lécuyer, E., Mulder, I., Lan, A., Bridonneau, C., et al. (2009). The key role of segmented filamentous bacteria in the coordinated maturation of gut helper T cell responses. Immunity 31, 677–689. doi: 10.1016/j.immuni.2009.08.020
Galloway-Peña, J. R., Shi, Y., Peterson, C. B., Sahasrabhojane, P., Gopalakrishnan, V., Brumlow, C. E., et al. (2020). Gut microbiome signatures are predictive of infectious risk following induction therapy for acute myeloid leukemia. Clin. Infect. Dis. 71, 63–71. doi: 10.1093/cid/ciz777
Galloway-Peña, J. R., Smith, D. P., Sahasrabhojane, P., Ajami, N. J., Wadsworth, W. D., Daver, N. G., et al. (2016). The role of the gastrointestinal microbiome in infectious complications during induction chemotherapy for acute myeloid leukemia. Cancer 122, 2186–2196. doi: 10.1002/cncr.30039
Galloway-Pena, J. R., Smith, D. P., Sahasrabhojane, P., Wadsworth, W. D., Fellman, B. M., Ajami, N. J., et al. (2017). Characterization of oral and gut microbiome temporal variability in hospitalized cancer patients. Genome Med. 9:21. doi: 10.1186/s13073-017-0409-1
Gao, X., Miao, R., Zhu, Y., Lin, C., Yang, X., Jia, R., et al. (2020). A new insight into acute lymphoblastic leukemia in children: influences of changed intestinal microfloras. BMC Pediatr. 20:290. doi: 10.1186/s12887-020-02192-9
Gibson, G. R., Hutkins, R., Sanders, M. E., Prescott, S. L., Reimer, R. A., Salminen, S. J., et al. (2017). Expert consensus document: the international scientific Association for Probiotics and Prebiotics (ISAPP) consensus statement on the definition and scope of prebiotics. Nat. Rev. Gastroenterol. Hepatol. 14, 491–502. doi: 10.1038/nrgastro.2017.75
Golob, J. L., Pergam, S. A., Srinivasan, S., Fiedler, T. L., Liu, C., Garcia, K., et al. (2017). Stool microbiota at neutrophil recovery is predictive for severe acute graft vs host disease after hematopoietic cell transplantation. Clin. Infect. Dis. 65, 1984–1991. doi: 10.1093/cid/cix699
Gurung, M., Li, Z., You, H., Rodrigues, R., Jump, D. B., Morgun, A., et al. (2020). Role of gut microbiota in type 2 diabetes pathophysiology. EBioMedicine 51:102590. doi: 10.1016/j.ebiom.2019.11.051
Hakim, H., Dallas, R., Wolf, J., Tang, L., Schultz-Cherry, S., Darling, V., et al. (2018). Gut microbiome composition predicts infection risk during chemotherapy in children with acute lymphoblastic leukemia. Clin. Infect. Dis. 67, 541–548. doi: 10.1093/cid/ciy153
Huang, X., Fang, Q., Rao, T., Zhou, L., Zeng, X., Tan, Z., et al. (2020). Leucovorin ameliorated methotrexate induced intestinal toxicity via modulation of the gut microbiota. Toxicol. Appl. Pharmacol. 391:114900. doi: 10.1016/j.taap.2020.114900
Ingham, A. C., Kielsen, K., Cilieborg, M. S., Lund, O., Holmes, S., Aarestrup, F. M., et al. (2019). Specific gut microbiome members are associated with distinct immune markers in pediatric allogeneic hematopoietic stem cell transplantation. Microbiome 7:131. doi: 10.1186/s40168-019-0745-z
Innes, A. J., Mullish, B. H., Fernando, F., Adams, G., Marchesi, J. R., Apperley, J. F., et al. (2017). Faecal microbiota transplant: a novel biological approach to extensively drug-resistant organism-related non-relapse mortality. Bone Marrow Transplant. 52, 1452–1454. doi: 10.1038/bmt.2017.151
Ivanov, I. I., Frutos Rde, L., Manel, N., Yoshinaga, K., Rifkin, D. B., Sartor, R. B., et al. (2008). Specific microbiota direct the differentiation of IL-17-producing T-helper cells in the mucosa of the small intestine. Cell Host Microbe 4, 337–349. doi: 10.1016/j.chom.2008.09.009
Jenq, R. R., Taur, Y., Devlin, S. M., Ponce, D. M., Goldberg, J. D., Ahr, K. F., et al. (2015). Intestinal Blautia is associated with reduced death from graft-versus-host disease. Biol. Blood Marrow Transplant. 21, 1373–1383. doi: 10.1016/j.bbmt.2015.04.016
Jin, Y., Dong, H., Xia, L., Yang, Y., Zhu, Y., Shen, Y., et al. (2019). The diversity of gut microbiome is associated with favorable responses to anti-programmed death 1 immunotherapy in Chinese patients with NSCLC. J. Thorac. Oncol. 14, 1378–1389. doi: 10.1016/j.jtho.2019.04.007
Kakihana, K., Fujioka, Y., Suda, W., Najima, Y., Kuwata, G., Sasajima, S., et al. (2016). Fecal microbiota transplantation for patients with steroid-resistant acute graft-versus-host disease of the gut. Blood 128, 2083–2088. doi: 10.1182/blood-2016-05-717652
Kang, J., Li, C., Gao, X., Liu, Z., Chen, C., and Luo, D. (2021). Metformin inhibits tumor growth and affects intestinal flora in diabetic tumor-bearing mice. Eur. J. Pharmacol. 912:174605. doi: 10.1016/j.ejphar.2021.174605
Kaur, G., Behl, T., Bungau, S., Kumar, A., Uddin, M. S., Mehta, V., et al. (2021). Dysregulation of the gut-brain Axis, Dysbiosis and influence of numerous factors on gut microbiota associated Parkinson's disease. Curr. Neuropharmacol. doi: 10.2174/1570159x18666200606233050
Kuczma, M. P., Ding, Z. C., Li, T., Habtetsion, T., Chen, T., Hao, Z., et al. (2017). The impact of antibiotic usage on the efficacy of chemoimmunotherapy is contingent on the source of tumor-reactive T cells. Oncotarget 8, 111931–111942. doi: 10.18632/oncotarget.22953
Lavelle, A., and Sokol, H. (2020). Gut microbiota-derived metabolites as key actors in inflammatory bowel disease. Nat. Rev. Gastroenterol. Hepatol. 17, 223–237. doi: 10.1038/s41575-019-0258-z
Lee, M. S. L., Ramakrishna, B., Moss, A. C., Gold, H. S., and Branch-Elliman, W. (2020). Successful treatment of fulminant Clostridioides difficile infection with emergent fecal microbiota transplantation in a patient with acute myeloid leukemia and prolonged, severe neutropenia. Transpl. Infect. Dis. 22:e13216. doi: 10.1111/tid.13216
Letertre, M. P. M., Munjoma, N., Wolfer, K., Pechlivanis, A., McDonald, J. A. K., Hardwick, R. N., et al. (2020). A two-way interaction between methotrexate and the gut microbiota of male Sprague-Dawley rats. J. Proteome Res. 19, 3326–3339. doi: 10.1021/acs.jproteome.0c00230
Liu, C., Frank, D. N., Horch, M., Chau, S., Ir, D., Horch, E. A., et al. (2017). Associations between acute gastrointestinal GvHD and the baseline gut microbiota of allogeneic hematopoietic stem cell transplant recipients and donors. Bone Marrow Transplant. 52, 1643–1650. doi: 10.1038/bmt.2017.200
Liu, X., Zou, Y., Ruan, M., Chang, L., Chen, X., Wang, S., et al. (2020). Pediatric acute lymphoblastic leukemia patients exhibit distinctive alterations in the gut microbiota. Front. Cell. Infect. Microbiol. 10:558799. doi: 10.3389/fcimb.2020.558799
Liu, X., Zou, Y., Zhang, Y., Liu, L., Duan, Y., Zhang, A., et al. (2021). Characteristics in gut microbiome is associated with chemotherapy-induced pneumonia in pediatric acute lymphoblastic leukemia. BMC Cancer 21:1190. doi: 10.1186/s12885-021-08917-y
Lucafo, M., Franzin, M., Lagatolla, C., Franca, R., Bramuzzo, M., Stocco, G., et al. (2020). Emerging insights on the interaction between anticancer and immunosuppressant drugs and intestinal microbiota in pediatric patients. Clin. Transl. Sci. 13, 238–259. doi: 10.1111/cts.12722
Ma, C., Han, M., Heinrich, B., Fu, Q., Zhang, Q., Sandhu, M., et al. (2018). Gut microbiome-mediated bile acid metabolism regulates liver cancer via NKT cells. Science 360:6391. doi: 10.1126/science.aan5931
Maeda, Y., and Takeda, K. (2019). Host-microbiota interactions in rheumatoid arthritis. Exp. Mol. Med. 51, 1–6. doi: 10.1038/s12276-019-0283-6
Malard, F., Vekhoff, A., Lapusan, S., Isnard, F., D'Incan-Corda, E., Rey, J., et al. (2021). Gut microbiota diversity after autologous fecal microbiota transfer in acute myeloid leukemia patients. Nat. Commun. 12:3084. doi: 10.1038/s41467-021-23376-6
Mancini, N., Greco, R., Pasciuta, R., Barbanti, M. C., Pini, G., Morrow, O. B., et al. (2017). Enteric microbiome markers as early predictors of clinical outcome in allogeneic hematopoietic stem cell transplant: results of a prospective study in adult patients. Open forum. Infect. Dis. 4:ofx215. doi: 10.1093/ofid/ofx215
Mandal, R. S., Saha, S., and Das, S. (2015). Metagenomic surveys of gut microbiota. Genomics Proteomics Bioinformatics 13, 148–158. doi: 10.1016/j.gpb.2015.02.005
Matson, V., Chervin, C. S., and Gajewski, T. F. (2021). Cancer and the microbiome-influence of the commensal microbiota on cancer, immune responses, and immunotherapy. Gastroenterology 160, 600–613. doi: 10.1053/j.gastro.2020.11.041
McDonald, L. C., Gerding, D. N., Johnson, S., Bakken, J. S., Carroll, K. C., Coffin, S. E., et al. (2018). Clinical practice guidelines for Clostridium difficile infection in adults and children: 2017 update by the Infectious Diseases Society of America (IDSA) and Society for Healthcare Epidemiology of America (SHEA). Clin. Infect. Dis. 66, e1–e48. doi: 10.1093/cid/cix1085
Nearing, J. T., Connors, J., Whitehouse, S., Van Limbergen, J., Macdonald, T., Kulkarni, K., et al. (2019). Infectious complications are associated with alterations in the gut microbiome in pediatric patients with acute lymphoblastic leukemia. Front. Cell. Infect. Microbiol. 9:28. doi: 10.3389/fcimb.2019.00028
Payen, M., Nicolis, I., Robin, M., Michonneau, D., Delannoye, J., Mayeur, C., et al. (2020). Functional and phylogenetic alterations in gut microbiome are linked to graft-versus-host disease severity. Blood Adv. 4, 1824–1832. doi: 10.1182/bloodadvances.2020001531
Peled, J. U., Devlin, S. M., Staffas, A., Lumish, M., Khanin, R., Littmann, E. R., et al. (2017). Intestinal microbiota and relapse after hematopoietic-cell transplantation. J. Clin. Oncol. 35, 1650–1659. doi: 10.1200/jco.2016.70.3348
Qin, J., Li, R., Raes, J., Arumugam, M., Burgdorf, K. S., Manichanh, C., et al. (2010). A human gut microbial gene catalogue established by metagenomic sequencing. Nature 464, 59–65. doi: 10.1038/nature08821
Rajagopala, S. V., Yooseph, S., Harkins, D. M., Moncera, K. J., Zabokrtsky, K. B., Torralba, M. G., et al. (2016). Gastrointestinal microbial populations can distinguish pediatric and adolescent acute lymphoblastic leukemia (ALL) at the time of disease diagnosis. BMC Genomics 17:635. doi: 10.1186/s12864-016-2965-y
Rashidi, A., Ebadi, M., Rehman, T. U., Elhusseini, H., Halaweish, H. F., Kaiser, T., et al. (2022). Lasting shift in the gut microbiota in patients with acute myeloid leukemia. Blood Adv. 6, 3451–3457. doi: 10.1182/bloodadvances.2021006783
Rashidi, A., Ebadi, M., Rehman, T. U., Elhusseini, H., Nalluri, H., Kaiser, T., et al. (2021). Altered microbiota-host metabolic cross talk preceding neutropenic fever in patients with acute leukemia. Blood Adv. 5, 3937–3950. doi: 10.1182/bloodadvances.2021004973
Rattanathammethee, T., Tuitemwong, P., Thiennimitr, P., Sarichai, P., Na Pombejra, S., Piriyakhuntorn, P., et al. (2020). Gut microbiota profiles of treatment-naïve adult acute myeloid leukemia patients with neutropenic fever during intensive chemotherapy. PLoS One 15:e0236460. doi: 10.1371/journal.pone.0236460
Redman, M. G., Ward, E. J., and Phillips, R. S. (2014). The efficacy and safety of probiotics in people with cancer: a systematic review. Ann. Oncol. 25, 1919–1929. doi: 10.1093/annonc/mdu106
Ren, W., Wang, P., Yan, J., Liu, G., Zeng, B., Hussain, T., et al. (2018). Melatonin alleviates weanling stress in mice: involvement of intestinal microbiota. J. Pineal Res. 64. doi: 10.1111/jpi.12448 [Epub ahead of print].
Ren, G., Zhang, J., Li, M., Tang, Z., Yang, Z., Cheng, G., et al. (2020). Gut microbiota composition influences outcomes of skeletal muscle nutritional intervention via blended protein supplementation in posttransplant patients with hematological malignancies. Clin. Nutr. 40, 94–102. doi: 10.1016/j.clnu.2020.04.030
Reyna-Figueroa, J., Barron-Calvillo, E., Garcia-Parra, C., Galindo-Delgado, P., Contreras-Ochoa, C., Lagunas-Martinez, A., et al. (2019). Probiotic supplementation decreases chemotherapy-induced gastrointestinal side effects in patients with acute leukemia. J. Pediatr. Hematol. Oncol. 41, 468–472. doi: 10.1097/MPH.0000000000001497
Rothschild, D., Weissbrod, O., Barkan, E., Kurilshikov, A., Korem, T., Zeevi, D., et al. (2018). Environment dominates over host genetics in shaping human gut microbiota. Nature 555, 210–215. doi: 10.1038/nature25973
Routy, B., Le Chatelier, E., Derosa, L., Duong, C. P. M., Alou, M. T., Daillère, R., et al. (2018). Gut microbiome influences efficacy of PD-1-based immunotherapy against epithelial tumors. Science 359, 91–97. doi: 10.1126/science.aan3706
Sacksteder, M. R. (1976). Occurrence of spontaneous tumors in the germfree F344 rat. J. Natl. Cancer Inst. 57, 1371–1373. doi: 10.1093/jnci/57.6.1371
Seidel, J. A., Otsuka, A., and Kabashima, K. (2018). Anti-PD-1 and anti-CTLA-4 therapies in cancer: mechanisms of action, efficacy, and limitations. Front. Oncol. 8:86. doi: 10.3389/fonc.2018.00086
Sekirov, I., Russell, S. L., Antunes, L. C., and Finlay, B. B. (2010). Gut microbiota in health and disease. Physiol. Rev. 90, 859–904. doi: 10.1152/physrev.00045.2009
Shen, Z., Gu, X., Cao, H., Mao, W., Yang, L., He, M., et al. (2021). Characterization of microbiota in acute leukemia patients following successful remission induction chemotherapy without antimicrobial prophylaxis. Int. Microbiol. 24, 263–273. doi: 10.1007/s10123-021-00163-3
Simms-Waldrip, T. R., Sunkersett, G., Coughlin, L. A., Savani, M. R., Arana, C., Kim, J., et al. (2017). Antibiotic-induced depletion of anti-inflammatory clostridia is associated with the development of graft-versus-host disease in pediatric stem cell transplantation patients. Biol. Blood Marrow Transplant. 23, 820–829. doi: 10.1016/j.bbmt.2017.02.004
Sivan, A., Corrales, L., Hubert, N., Williams, J. B., Aquino-Michaels, K., Earley, Z. M., et al. (2015). Commensal Bifidobacterium promotes antitumor immunity and facilitates anti-PD-L1 efficacy. Science 350, 1084–1089. doi: 10.1126/science.aac4255
Smith, M., Dai, A., Ghilardi, G., Amelsberg, K. V., Devlin, S. M., Pajarillo, R., et al. (2022). Gut microbiome correlates of response and toxicity following anti-CD19 CAR T cell therapy. Nat. Med. 28, 713–723. doi: 10.1038/s41591-022-01702-9
Song, Y., and Gyarmati, P. (2020). Microbiota changes in a pediatric acute lymphocytic leukemia mouse model. Microbiology 9:e982. doi: 10.1002/mbo3.982
Strick, R., Strissel, P. L., Borgers, S., Smith, S. L., and Rowley, J. D. (2000). Dietary bioflavonoids induce cleavage in the MLL gene and may contribute to infant leukemia. Proc. Natl. Acad. Sci. U. S. A. 97, 4790–4795. doi: 10.1073/pnas.070061297
Suez, J., Zmora, N., Zilberman-Schapira, G., Mor, U., Dori-Bachash, M., Bashiardes, S., et al. (2018). Post-antibiotic gut mucosal microbiome reconstitution is impaired by probiotics and improved by autologous FMT. Cells 174, 1406–1423.e16. doi: 10.1016/j.cell.2018.08.047
Tang, D., Zeng, T., Wang, Y., Cui, H., Wu, J., Zou, B., et al. (2020). Dietary restriction increases protective gut bacteria to rescue lethal methotrexate-induced intestinal toxicity. Gut Microbes 12:1714401. doi: 10.1080/19490976.2020.1714401
Taskin, B., Erdoğan, M. A., Yiğittürk, G., Günenç, D., and Erbaş, O. (2017). Antifibrotic effect of lactulose on a methotrexate-induced liver injury model. Gastroenterol. Res. Pract. 2017:7942531. doi: 10.1155/2017/7942531
Taur, Y., Coyte, K., Schluter, J., Robilotti, E., Figueroa, C., Gjonbalaj, M., et al. (2018). Reconstitution of the gut microbiota of antibiotic-treated patients by autologous fecal microbiota transplant. Sci. Transl. Med. 10:460. doi: 10.1126/scitranslmed.aap9489
Taur, Y., Jenq, R. R., Perales, M. A., Littmann, E. R., Morjaria, S., Ling, L., et al. (2014). The effects of intestinal tract bacterial diversity on mortality following allogeneic hematopoietic stem cell transplantation. Blood 124, 1174–1182. doi: 10.1182/blood-2014-02-554725
Taur, Y., Xavier, J. B., Lipuma, L., Ubeda, C., Goldberg, J., Gobourne, A., et al. (2012). Intestinal domination and the risk of bacteremia in patients undergoing allogeneic hematopoietic stem cell transplantation. Clin. Infect. Dis. 55, 905–914. doi: 10.1093/cid/cis580
Tkach, S., Dorofeyev, A., Kuzenko, I., Boyko, N., Falalyeyeva, T., Boccuto, L., et al. (2022). Current status and future therapeutic options for fecal microbiota transplantation. Medicina (Kaunas) 58:84. doi: 10.3390/medicina58010084
Tsilimigras, M. C., Fodor, A., and Jobin, C. (2017). Carcinogenesis and therapeutics: the microbiota perspective. Nat. Microbiol. 2:17008. doi: 10.1038/nmicrobiol.2017.8
Uronis, J. M., Mühlbauer, M., Herfarth, H. H., Rubinas, T. C., Jones, G. S., and Jobin, C. (2009). Modulation of the intestinal microbiota alters colitis-associated colorectal cancer susceptibility. PLoS One 4:e6026. doi: 10.1371/journal.pone.0006026
Vernocchi, P., Gili, T., Conte, F., Del Chierico, F., Conta, G., Miccheli, A., et al. (2020). Network analysis of gut microbiome and metabolome to discover microbiota-linked biomarkers in patients affected by non-small cell lung cancer. Int. J. Mol. Sci. 21:8730. doi: 10.3390/ijms21228730
Vétizou, M., Pitt, J. M., Daillère, R., Lepage, P., Waldschmitt, N., Flament, C., et al. (2015). Anticancer immunotherapy by CTLA-4 blockade relies on the gut microbiota. Science 350, 1079–1084. doi: 10.1126/science.aad1329
Viaud, S., Saccheri, F., Mignot, G., Yamazaki, T., Daillere, R., Hannani, D., et al. (2013). The intestinal microbiota modulates the anticancer immune effects of cyclophosphamide. Science 342, 971–976. doi: 10.1126/science.1240537
Vicente-Dueñas, C., Janssen, S., Oldenburg, M., Auer, F., González-Herrero, I., Casado-García, A., et al. (2020). An intact gut microbiome protects genetically predisposed mice against leukemia. Blood 136, 2003–2017. doi: 10.1182/blood.2019004381
Vindigni, S. M., and Surawicz, C. M. (2017). Fecal microbiota transplantation. Gastroenterol. Clin. N. Am. 46, 171–185. doi: 10.1016/j.gtc.2016.09.012
Vogtmann, E., and Goedert, J. J. (2016). Epidemiologic studies of the human microbiome and cancer. Br. J. Cancer 114, 237–242. doi: 10.1038/bjc.2015.465
Wang, Q., Fu, Y. W., Wang, Y. Q., Ai, H., Yuan, F. F., Wei, X. D., et al. (2019). Fecal microbiota transplantation for patients with refractory diarrhea after allogeneic hematopoietic stem cell transplantation. Zhonghua Xue Ye Xue Za Zhi 40, 853–855. doi: 10.3760/cma.j.issn.0253-2727.2019.10.011
Wang, J. W., Kuo, C. H., Kuo, F. C., Wang, Y. K., Hsu, W. H., Yu, F. J., et al. (2019). Fecal microbiota transplantation: review and update. J. Formos. Med. Assoc. 118, S23–s31. doi: 10.1016/j.jfma.2018.08.011
Wardill, H. R., Van Sebille, Y. Z. A., Ciorba, M. A., and Bowen, J. M. (2018). Prophylactic probiotics for cancer therapy-induced diarrhoea: a meta-analysis. Curr. Opin. Support. Palliat. Care 12, 187–197. doi: 10.1097/spc.0000000000000338
Yeung, C. Y., Chiang Chiau, J. S., Cheng, M. L., Chan, W. T., Chang, S. W., Chang, Y. H., et al. (2020). Modulations of probiotics on gut microbiota in a 5-fluorouracil-induced mouse model of mucositis. J. Gastroenterol. Hepatol. 35, 806–814. doi: 10.1111/jgh.14890
Yu, L. X., and Schwabe, R. F. (2017). The gut microbiome and liver cancer: mechanisms and clinical translation. Nat. Rev. Gastroenterol. Hepatol. 14, 527–539. doi: 10.1038/nrgastro.2017.72
Yu, D., Yu, X., Ye, A., Xu, C., Li, X., Geng, W., et al. (2021). Profiling of gut microbial dysbiosis in adults with myeloid leukemia. FEBS Open Bio. 11, 2050–2059. doi: 10.1002/2211-5463.13193
Zhang, J., Zhang, J., and Wang, R. (2018). Gut microbiota modulates drug pharmacokinetics. Drug Metab. Rev. 50, 357–368. doi: 10.1080/03602532.2018.1497647
Zhou, R., He, D., Xie, J., Zhou, Q., Zeng, H., Li, H., et al. (2021). The synergistic effects of polysaccharides and Ginsenosides from American ginseng (Panax quinquefolius L.) ameliorating cyclophosphamide-induced intestinal immune disorders and gut barrier dysfunctions based on microbiome-metabolomics analysis. Front. Immunol. 12:665901. doi: 10.3389/fimmu.2021.665901
Zhou, Q. H., Wu, F. T., Pang, L. T., Zhang, T. B., and Chen, Z. (2020). Role of gammadeltaT cells in liver diseases and its relationship with intestinal microbiota. World J. Gastroenterol. 26, 2559–2569. doi: 10.3748/wjg.v26.i20.2559
Zhou, B., Xia, X., Wang, P., Chen, S., Yu, C., Huang, R., et al. (2018). Induction and amelioration of methotrexate-induced gastrointestinal toxicity are related to immune response and gut microbiota. EBioMedicine 33, 122–133. doi: 10.1016/j.ebiom.2018.06.029
Keywords: gut microbiota, acute leukemia, chemotherapy, immunotherapy, hematopoietic stem cell transplantation, probiotics
Citation: Zhou Y, Zhou C and Zhang A (2022) Gut microbiota in acute leukemia: Current evidence and future directions. Front. Microbiol. 13:1045497. doi: 10.3389/fmicb.2022.1045497
Edited by:
Hu T. Huang, McKesson (United States), United StatesReviewed by:
Bikul Das, KaviKrishna Laboratory, IndiaColin J. Brislawn, Contamination Source Identification, United States
Copyright © 2022 Zhou, Zhou and Zhang. This is an open-access article distributed under the terms of the Creative Commons Attribution License (CC BY). The use, distribution or reproduction in other forums is permitted, provided the original author(s) and the copyright owner(s) are credited and that the original publication in this journal is cited, in accordance with accepted academic practice. No use, distribution or reproduction is permitted which does not comply with these terms.
*Correspondence: Aijun Zhang, emhhbmdhaWp1bkBzZHUuZXVkLmNu
†These authors have contributed equally to this work and share first authorship