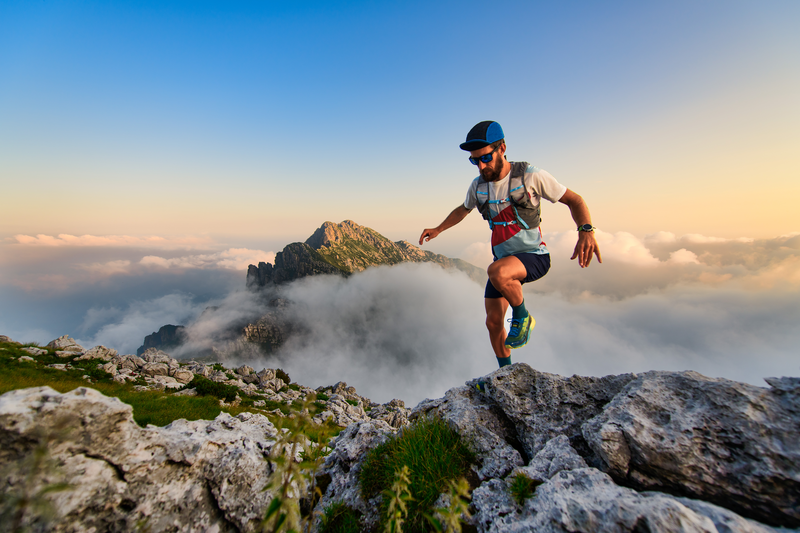
95% of researchers rate our articles as excellent or good
Learn more about the work of our research integrity team to safeguard the quality of each article we publish.
Find out more
ORIGINAL RESEARCH article
Front. Microbiol. , 03 November 2022
Sec. Antimicrobials, Resistance and Chemotherapy
Volume 13 - 2022 | https://doi.org/10.3389/fmicb.2022.1042214
Pseudomonas aeruginosa is an important opportunistic pathogen, and the emergence of drug resistance greatly increased the difficulty of treating its infection. Cell density-dependent quorum sensing (QS) system not only regulates the virulence but also associates with the drug resistance of P. aeruginosa. Screening for agents targeting QS to inhibit bacterial virulence and pathogenicity is considered a promising strategy to combat P. aeruginosa infection. In the present study, sennoside A was found to be able to inhibit the QS expression of P. aeruginosa at subinhibitory concentrations. The QS-regulated virulence factors, including protease, elastase, rhamnolipid, and pyocyanin, were also inhibited by sennoside A at both transcriptional and translational levels. Moreover, sennoside A could suppress the motility of twitching, swimming, and swarming as well as the biofilm formation, which is associated with the acute and chronic infections of P. aeruginosa in a dose-dependent manner. The attenuated pathogenicity of P. aeruginosa by sennoside A was further verified by Chinese cabbage, Drosophila melanogaster, and Caenorhabditis elegans infection analysis. Further study found that sennoside A might target the las system, mainly LasR, to interfere with QS. All the results indicate that sennoside A could inhibit the QS system to attenuate its regulated virulence and pathogenicity via mainly targeting LasR in P. aeruginosa and further research to identify its anti-QS activity for other Gram-negative bacteria is warranted.
Pseudomonas aeruginosa is a widespread important opportunistic pathogen that could cause hospital-acquired infection and severe complications, especially in patients with compromised immune systems, such as cystic fibrosis or burn patients (Stover et al., 2000; Driscoll et al., 2007; Karlberg et al., 2018). P. aeruginosa infections are very difficult to eradicate due to their intrinsic and acquired resistance to many antimicrobial agents used in clinical therapy (Levy and Marshall, 2004). Antibiotic abuse has driven a much more emergence of multiple-resistant P. aeruginosa, including those resistant to carbapenems (Basha et al., 2020). However, the development of new drugs has slowed down over the decades. The cure rate has decreased and mortality increased dramatically, resulting in worldwide public health and economic problems (Medina and Pieper, 2016). Therefore, it is urgent to explore alternative strategies for the treatment of P. aeruginosa infection.
Quorum sensing (QS) system is a cell density-dependent intercellular communication system, ubiquitously present in pathogenic bacteria (Norizan et al., 2013). Three interconnected QS systems, acyl homoserine lactone (AHL)-based las and rhl systems and quinolone-based pqs system, exist in P. aeruginosa (Li et al., 2020). lasI and rhlI encode the signaling molecules of N-3-oxo-dodecanoyl-l-homoserine lactone (3-oxo-C12-HSL) and N-butanoyl-L-homoserine lactone (C4-HSL), respectively. Transcriptional regulators of LasR and RhlR could bind with 3-oxo-C12-HSL and C4-HSL, respectively, to regulate the expression of their corresponding downstream genes, like lasA, lasB, rhlAB, toxA, and aprA (Whiteley and Greenberg, 2001; Schuster and Greenberg, 2007; Lee and Zhang, 2015). Similarly, pqsA initiates the synthesis of the signaling molecule of 2-heptyl-3-hydroxy-4-quinolone (PQS), which binds to the receptor PqsR to regulate the expression of the downstream genes, like rhlAB, phzABCDEFG, etc. (Deziel et al., 2004; Lee and Zhang, 2015). The three QS systems are interconnected with a multi-layered hierarchy, las system is at the top of the signal network and could activate the rhl and pqs systems (Wang et al., 2020); the rhl system could downregulate the pqs system, whereas pqs system could upregulate rhl system (Welsh and Blackwell, 2016).
Pseudomonas aeruginosa could cause severe acute and chronic infection in planktonic or biofilm states (Sankar Ganesh and Ravishankar Rai, 2018). The acute infection of P. aeruginosa is accompanied by the participation of many virulence factors. For instance, lasA-encoded protease and lasB-encoded elastase could destroy interstitial tissue and expand infection foci of the host (Stehling et al., 2008); rhlA-encoded rhamnolipid acts as a surfactant to stimulate motility of cells and biofilm formation (Davey et al., 2003; Caiazza et al., 2005; Tremblay et al., 2007); phzA1-encoded pyocyanin is a redox-active phenazine compound that could mediate tissue damage and necrosis of host during infection (Lau et al., 2004). Chronic infection is mainly associated with the formation of biofilms (Xu et al., 2013). The motility of swarming, swimming, and twitching mediates the movement and adhesion of cells to the infective site, initiating the acute and chronic infection of P. aeruginosa (Khan et al., 2020). The production of virulence factors, biofilm formation, and motility are all regulated by the QS system of P. aeruginosa (Adonizio et al., 2008). Therefore, inhibiting the QS system to relieve or suppress bacterial virulence and infectivity could be a promising strategy to tackle the infection caused by P. aeruginosa.
Sennoside A (SA), an anthraquinone compound, has been commonly used as a stimulant laxative to cure constipation (Le et al., 2021). Lots of physiological properties have also been reported for sennoside A, such as anti-obesity (Le et al., 2019), anti-cancer (Khan et al., 2020), hypoglycemic (Wei et al., 2020), and anti-neurodegenerative activities (Gao et al., 2021). In this study, sennoside A was found to be able to inhibit the QS and QS-regulated virulence features, including virulence factor production, biofilm formation, motility as well as pathogenicity of P. aeruginosa at subinhibitory concentrations. The probable target of sennoside A to anti-QS of P. aeruginosa was also searched by molecular docking and virulence gene expression analysis with lux-based QS quintuple mutants. Our study indicated that sennoside A could inhibit QS system of P. aeruginosa to attenuate its regulated virulence and pathogenicity by targeting the las system, mainly LasR.
The strains and plasmids used in this study are listed in Supplementary Table 1. Unless otherwise specified, bacteria strains were cultured in Luria-Bertani medium (LB, 1% NaCl, 1% peptone, and 0.5% yeast extract) at 37°C with shaking of 200 rpm. Sennoside A was purchased from Shanghai Yuanye Bio-Technology Co., Ltd. (Shanghai, China) and dissolved in dimethyl sulfoxide (DMSO).
The plasmid pMS402 carrying a promoter-less luxCDABE reporter gene cluster was used to construct promoter-luxCDABE reporter fusions of lasI and other QS-associated genes as described previously (Wang et al., 2021). Briefly, the promoter region of lasI was amplified by a polymerase chain reaction and ligated to pMS402 to obtain the plasmid pKD-lasI. Then, pKD-lasI was electroporated into PAO1 to get the lux-based reporter strain PAO1 (pKD-lasI). The lux-based reporter strains for the other genes were constructed similarly.
The gene expression was measured as the chemiluminescence produced by the luxCDABE operon, which is located in downstream of the gene promoter. Specifically, overnight cultures of the reporter strains were diluted to the appropriate cell density and cultivated for an additional 3 h. Then, 5 μl of the culture was inoculated into the parallel wells containing a total of 95 μl LB broth without or with sennoside A at different concentrations (50 or 100 μg/ml) in the black clear-bottom 96-well plate. A total of 50 μl of sterilized liquid paraffin was added to each well to prevent evaporation during the experiment. Luminescence (counts per second, CPS) as well as OD600 were measured every 30 min for 24 h by a Synergy H1 microplate reader (BioTeck, Winooski, VT, USA).
Protease activity was evaluated with 2% non-fat milk powder (Luo et al., 2017). In brief, P. aeruginosa PAO1 was cultured in the presence or absence of sennoside A at 37°C for 12 h. The supernatants were collected and then filtered by a syringe-driven filter of 0.22 μm, and 200 μl of filtered supernatant from the different groups were added to 800 μl phosphate buffer (pH = 7.8) containing 2% non-fat milk powder. The mixture was incubated at 37°C for 30 min to determine the absorbance at 440 nm.
Elastase activity was estimated by the elastin Congo red assay (Kessler and Safrin, 2014). The supernatants were prepared similarly to the protease analysis. For each sample, 200 μl of filtered supernatant was added to 800 μl of reaction buffer (1 mM CaCl2, 0.1 M Tris–HCl, pH = 7.0) containing 5 mg of Elastin-Congo Red (Sigma, Saint Louis, MO, USA) and incubated at 37°C with a shaking of 200 rpm for 3 h. Elastase activity was determined by measuring the absorbance of the Congo red solution at OD495 nm.
Secretion of rhamnolipid was evaluated using a cetyl trimethyl ammonium bromide (CTAB)-methylene blue (MB) plate (Jadhav et al., 2011). Briefly, overnight cultures of PAO1 were diluted to the appropriate cell density and then 2 μl of the diluted cultures were inoculated on the center of the CTAB-MB plate (0.7 g/L KH2PO4, 0.9 g/L Na2HPO4, 2 g/L NaNO3, 0.4 g/L MgSO4, 0.1 g/L CaCl2, 0.16 mg/L FeCl3, 1.5 mg/L ZnSO4, 0.15 mg/L CuSO4, 1.5 mg/L MnSO4, 1.5 mg/L H3BO3, 20 g/L glycerol, 0.2 g/L CTAB, 5 mg/L MB, and agar 15 g/L) with different concentrations of sennoside A. The plates were incubated at 37°C for 3 days and rhamnolipid production was confirmed by the blue halos around the colonies.
Pyocyanin was extracted and determined as described by Essar et al. (1990). Briefly, 50 μl of PAO1 overnight culture was inoculated to 5 ml Pseudomonas broth (0.14% MgCl2, 1% K2SO4, and 2% peptone, pH = 7.2) containing 0, 50, and 100 μg/ml of sennoside A and then cultivated at 37°C for 16 h. After centrifugation at 8,000 rpm, 5 ml of supernatant was mixed with 3 ml of chloroform thoroughly and then the chloroform phase was transferred to a fresh tube and mixed with 1 ml of 0.2 N HCl. Pyocyanin was quantified by determining the absorbance of the upper phase at OD520 nm.
Biofilm formation was measured using the crystal violet method (He et al., 2014). Briefly, 30 μl of PAO1 overnight culture was inoculated into the wells containing 3 ml LB broth with sennoside A at different concentrations of 0, 50, and 100 μg/ml in the 12-well polystyrene plate. The plate was cultivated at 37°C for 24 h with a static system to form biofilms. Following the removal of the supernatant and non-adherent cells, phosphate-buffered saline was used to gently rinse the wells three times and then 3.5 ml of 1% crystal violet was added into the wells for 15 min staining. The attached crystal violet was washed with anhydrous ethanol and its absorbance was measured at 595 nm to quantify biofilm formation.
The motility of swimming, swarming, and twitching of PAO1 was analyzed according to the previous report (Wang et al., 2021). For the swimming and swarming assay, 2 μl of log-phase PAO1 culture was spot inoculated onto the swimming agar plates (1% peptone, 0.5% NaCl, and 0.3% agar; pH 7.2) and swarming agar plates (0.8% nutrient broth, 0.5% glucose, and 0.5% agar; pH 7.2), which contained different concentrations of sennoside A, i.e., 0, 50, and 100 μg/ml. Twitching motility was determined by subsurface stab assays with 1% LB agar plates containing different concentrations of sennoside A. All the plates were incubated at 37°C for 24 h and the motility was investigated.
Chinese cabbage, Drosophila melanogaster, and Caenorhabditis elegans infection models were used to evaluate the anti-infection activity of sennoside A against P. aeruginosa.
The cabbage infection analysis was performed according to the previous report with slight modifications (Starkey and Rahme, 2009). Briefly, 50 μl of PAO1 overnight culture was inoculated to 5 ml LB broth with or without sennoside A and then cultured at 37°C for 12 h. After centrifugation, cells were rinsed and then resuspended in 10 mM MgSO4 to a final concentration of 107 colony-forming unit (CFU)/ml. A total of 10 μl of resuspension from the different groups was taken and injected into the cabbage stems that have been sterilized with 0.1% hydrogen peroxide. The cabbage stems were placed in Petri dishes with filter paper impregnated with 10 mM MgSO4. All the samples were incubated at 30°C for 6 days and the rotten area was determined.
The fruit fly feeding assay was carried out as described previously (Chugani et al., 2001). PAO1 was cultivated in LB broth containing sennoside A at 0, 50, and 100 μg/ml concentrations, respectively, for 12 h. The pellets from 1.5 ml cultures were suspended in 100 ml of 5% sucrose. Then, 200 μl of the resuspensions were spotted on the sterile filter disk that completely covered the surface of the sucrose agar (5% sucrose and 1% agar) contained in the flasks. Twenty 3- to 5-day-old male fruit flies that have been starved for 3 h were placed into each of the prepared flasks. The number of live fruit flies was counted every 24 h for 10 days to calculate the survival rate of the fruit flies.
The C. elegans infection analysis was performed as reported previously (Sarabhai et al., 2013). Briefly, 100 μl of overnight PAO1 culture was spread evenly on the nematode culture medium (NGM) plates (0.3% NaCl, 0.25% tryptone, 1 mM MgSO4, 1 mM CaCl2, 5 μg/ml cholesterol, 100 μg/ml 5-Fluorouracil, and 2% agar) with different concentration of sennoside A and cultivated at 37°C for 12 h to form a bacterial lawn. Plates coated with Escherichia coli OP50 served as negative controls. Synchronized C. elegans N2 hermaphrodite worms at the L4 phase were seeded onto the prepared NGM plates. The plates were incubated at 20°C and the survival rate of nematodes was recorded every 12 h for 5 days.
Molecular docking was performed according to the previous report (Dai et al., 2019). The 3D structure of the proteins, LasR, LasI, PqsA, and PqsR, were downloaded from the online Protein Data Bank.1 The structure of sennoside A was obtained from the online website.2 The binding interaction of sennoside A with the QS-associated proteins in P. aeruginosa was evaluated by Auto Dock. The docking results were displayed using the PyMOL Molecular Graphics System (v. 2.3.4). The root mean square deviation (RMSD) values of each docking task based on the ligand sennoside A were calculated by the PyMOL Molecular Graphics System (v. 2.3.4).
All the experiments were repeated three times and the values are presented as the mean ± SD. Statistical analysis was carried out by GraphPad Prism version 5.0.1 to determine significant differences (p < 0.05).
The effect of sennoside A on the growth of P. aeruginosa PAO1 was first assessed. As shown in Figure 1, sennoside A at concentrations of 50 and 100 μg/ml did not inhibit the growth of PAO1 compared with the control group with no sennoside A. Therefore, 50 and 100 μg/ml of sennoside A were used for the following studies.
Figure 1. Effect of Sennoside A (SA) on the growth of PAO1. All data were expressed as mean ± SD values with three independent experiments performed in triplicate. nsp > 0.05; **p < 0.01; ***p < 0.001.
The expression of QS genes, lasI, lasR, rhlI, rhlR, pqsA, and pqsR, was determined in the presence of 0, 50, and 100 μg/ml sennoside A. As shown in Figure 2, the expression of examined genes was all decreased by sennoside A in a dose-dependent manner during the log phase. However, the expression of the autoinducer coding gene of rhlI whose peak was at 10 h was induced later than that of the receptor coding gene of rhlR whose peak was at 4–6 h, different from the general pathway of QS signaling. A possible reason is that RhlR might respond to alternative ligands in addition to its canonical C4-HSL, like PqsE (Mukherjee et al., 2017; Groleau et al., 2020), and it needs further study.
Figure 2. Sennoside A inhibits the expression of QS-regulated genes (lasI, lasR, rhlI, rhlR, pqsA, and pqsR) in PAO1. All data were expressed as mean ± SD values with three independent experiments performed in triplicate.
Moreover, the influence of sennoside A on the expression of lasB, rhlA, and phzA1, which were the important virulence factor coding genes, and respectively, regulated by las, rhl, and pqs system, was also determined similarly. The results indicated that they were all downregulated by sennoside A in a dose-dependent manner compared with the control group (Figure 3).
Figure 3. Inhibition of SA on the expression of virulence factor encoding genes (lasB, rhlA, and phzA1). All data were expressed as mean ± SD values with three independent experiments performed in triplicate.
The production of the QS-controlled virulence factors in PAO1, including elastase, rhamnolipid, and pyocyanin, which were encoded by lasB, rhlA, and phzA1, respectively, was measured in an LB medium with and without sennoside A. As shown in Figure 4A, the absorbance at 495 nm was, respectively, decreased by 30.9 and 50.7% in the presence of 50 and 100 μg/ml sennoside A compared with the control group. The results indicated that sennoside A inhibits the production of elastase in a dose-dependent manner. Similarly, the production of other virulence factors of protease (Figure 4B), rhamnolipid (Figure 4C), and pyocyanin (Figure 4D) was also reduced significantly by sennoside A in a dose-dependent manner compared with the control group.
Figure 4. Inhibition effect of SA on the production of virulence factors in PAO1. (A) Protease; (B) elastase; (C) rhamnolipid; and (D) pyocyanin. All data were expressed as mean ± SD values with three independent experiments performed in triplicate. **p < 0.01; ***p < 0.001.
Biofilms and motility are additional crucial virulence features regulated by QS in P. aeruginosa. The impact of sennoside A on the biofilm formation and motility of swimming, swarming, and twitching was investigated in PAO1 accordingly. The results showed that sennoside A at 50 and 100 μg/ml reduced the biofilm formation by 40.3 and 46.7% (Figure 5), and inhibited swimming by 49.2 and 71%, swarming by 31.8 and 50.4%, and twitching by 39.4 and 55.8%, respectively, compared with the control (Figure 6).
Figure 5. Impact of SA on the biofilm formation of PAO1. All data were expressed as mean ± SD values with three independent experiments performed in triplicate. ***p < 0.001.
Figure 6. Effect of SA on the motility of PAO1. (A) Swimming; (B) swarming; and (C) twitching. All data were expressed as mean ± SD values with three independent experiments performed in triplicate. *p < 0.05; **p < 0.01; ***p < 0.001.
Chinese cabbage, D. melanogaster, and C. elegans infection models were employed to evaluate whether sennoside A could attenuate the pathogenicity of P. aeruginosa. The results indicated that sennoside A could decrease the infection of PAO1 in Chinese cabbage because the rotten areas caused by PAO1 were reduced by 57.3 and 68.1%, respectively, in the presence of sennoside A at 50 and 100 μg/ml compared with that in absence of sennoside A (Figure 7A). In the D. melanogaster infection model, the 10-day survival rate of fruit flies was 33.3% in the control group while those in 50 and 100 μg/ml sennoside A treatment groups were increased to 63.3 and 66.7%, respectively, suggesting that sennoside A could decrease the mortality of the fruit files caused by PAO1 (Figure 7B). Similarly, the 108-h survival rate of infected nematodes was improved by nearly 40% under sennoside A treatments compared with the control (Figure 7C). All the results illustrated that sennoside A exerts a constructive impact on reducing the pathogenicity of P. aeruginosa.
Figure 7. Effect of SA on the pathogenicity of PAO1. (A) Rotten area of Chinese cabbage; (B) survival rate of fruit flies; and (C) survival rate of nematodes. (+), with PAO1; (–), without PAO1. All data were expressed as mean ± SD values with three independent experiments performed in triplicate. **p < 0.01; ***p < 0.001.
Molecular docking analysis was performed to explore the interaction of sennoside A with the QS-related proteins of LasI, LasR, PqsA, and PqsR in P. aeruginosa. RhlI and RhlR were not used for the analysis since the crystal structures were not available. The results of AutoDock analysis showed that sennoside A could form hydrogen bonds with Gln4, Ile5, Phe11, and Asp12 of LasI (Figure 8A), and the binding energy between sennoside A and LasI was −4.67 kCal/mol. RMSD values of sennoside A binding with LasI was 0.800 Å. Sennoside A binds to LasR with Ser20, Gln24, Lys34, and Gly54 residues by hydrogen bond interactions (Figure 8B), the binding energy was −5.85 kCal/mol, RMSD of sennoside A binding with LasR was 1.295 Å. Additionally, sennoside A forms hydrogen bonds with Val10, Arg13, Asp15, and Glu388 of PqsA (Figure 8C) and Asp109 and Leu295 of PqsR (Figure 8D). The binding energy of sennoside A to PqsA and PqsR was −4.47 and −4.97 kCal/mol, respectively, and RMSD of sennoside A binding with PqsA and PqsR was 0.958 and 1.890 Å, respectively. The results indicated that sennoside A might probably inhibit the QS system of P. aeruginosa by interacting with LasR since sennoside A has the highest binding scores with LasR. However, the interaction of sennoside A with RhlI/RhlR could not be excluded.
Figure 8. Three-dimensional (left) and 3D partial enlarged graphics (right) of molecular docking analysis for SA binding to QS-related proteins of P. aeruginosa. (A) LasI, PDBID: 1RO5; (B) LasR, PDBID: 6D6A; (C) PqsA, PDBID: 5OE6; and (D) PqsR, PDBID: 4JVD.
To further explore the target of sennoside A in inhibiting QS of P. aeruginosa, three quintuple mutants, PAO1 (ΔlasIΔrhlIΔpqsAΔrhlRΔpqsR) referred to as QM-1, PAO1 (ΔlasIΔrhlIΔpqsAΔlasRΔpqsR) referred to as QM-2 and PAO1 (ΔlasIΔrhlIΔpqsAΔlasRΔrhlR) referred to as QM-3, which separately contained only one kind of receptor coding gene of lasR, rhlR, or pqsR, were constructed and used. Since LasR and RhlR could upregulate the expression of lasB, and PqsR could upregulate the expression of pqsA, three strains of QM-1 (pKD-lasB), QM-2 (pKD-lasB), and QM-3 (pKD-pqsA) were further constructed. Then, the light production from QM-1 (pKD-lasB), QM-2 (pKD-lasB), and QM-3 (pKD-pqsA) were determined in the LB medium in presence of corresponding exogenous signaling molecules of 3-oxo-C12-HSL, C4-HSL or PQS with or without sennoside A.
As a consequence, the expression of lasB in QM-1 (pKD-lasB) was reduced significantly by sennoside A in a dose-dependent manner, while the expression of lasB in QM-2 (pKD-lasB) and that of pqsA in QM-3 (pKD-pqsA) were not affected by sennoside A (Figure 9). Concurrently, sennoside A has no effect on the growth of QM-1 (pKD-lasB), QM-2 (pKD-lasB), and QM-3 (pKD-pqsA) (Figure 9). The results suggested that the inhibition of sennoside A on the QS requires the presence of the las system.
Figure 9. Effects of SA on the expression of lasB and pqsA in the QS quintuple mutants. (A) The expression of lasB in QM-1(pKD-lasB); (B) the expression of lasB in QM-2(pKD-lasB); and (C) the expression of pqsA in QM-3(pKD-pqsA). All data were expressed as mean ± SD values with three independent experiments performed in triplicate.
Furthermore, the lasR knockout mutant of PAO1 (ΔlasR) was used to determine the pathogenicity in the presence and absence of sennoside A via the cabbage infection model. The result revealed that the attenuated pathogenicity of PAO1 by sennoside A was not observed in the mutant of PAO1 (ΔlasR) in which LasR was inactivated (Supplementary Figure 1). The above results indicated that sennoside A inhibits QS of P. aeruginosa mainly by interacting with LasR.
The emergence of bacterial resistance has led to great difficulty in infection treatment and increased mortality, causing a global public health crisis (Levy and Marshall, 2004). According to a meta-analysis, multidrug-resistant P. aeruginosa infection showed a >2-fold higher risk of mortality than infection caused by susceptible P. aeruginosa (Yang et al., 2018). QS is a gene regulation system that is widely present in bacteria and controls various physiological activities of bacteria, such as antibiotic resistance, virulence, and pathogenicity (Norizan et al., 2013). Inhibition of the QS system has been reported to be able to attenuate virulence and infectivity, and is thus considered a promising strategy to tackle infections caused by multidrug-resistant bacteria including P. aeruginosa (Kalia and Purohit, 2011). Numerous natural and synthetic compounds have been reported as QS inhibitors (QSIs). However, many of them are not suitable for clinical application due to their inherent cytotoxicity.
Sennoside A, an anthraquinone compound, has been used as a laxative for a long history in China. It has also shown some other pharmacology properties including anti-bacterial (Ram Avtar, 2012). According to the subacute and chronic toxicity studies, sennoside A showed no significant toxicity to rats (max. 20 mg/kg) and dogs (max. 500 mg/kg) (Mengs, 1988). In this study, sennoside A displayed an alternative physiological role as a QSI to attenuate the virulence and pathogenicity of P. aeruginosa.
First, sennoside A at subinhibitory concentrations could decrease the expression of QS-associated genes of lasI, lasR, rhlI, rhlR, pqsA, pqsR, lasB, rhlA, and phzA1 in P. aeruginosa in a dose-dependent pattern (Figures 2, 3). Likewise, the production of the virulence factors, lasA encoded protease, lasB encoded elastase, rhlA encoded rhamnolipid, and phzA1 encoded pyocyanin, was also inhibited similarly by sennoside A. All these virulence factors play important roles during the infection of P. aeruginosa (Figure 4).
Biofilms are a community of bacteria attached to biological and abiotic surfaces (Luo et al., 2017). They are responsible for chronic infection and can decrease the sensitivity of P. aeruginosa to drugs as much as 1,000-fold (Anwar et al., 1990). In multidrug resistance P. aeruginosa isolates, the biofilm-forming ability was significantly higher (Nassar et al., 2022). Motility is another important virulence feature associated with initial acute infection and chronic infection of P. aeruginosa. It is indispensable in surface perception and adhesion, biofilm formation, and resisting host immune responses of P. aeruginosa. P. aeruginosa performs different motility, flagella-driven swimming, type IV pili impelled twitching, and co-coordinated swarming of flagella and type IV pili flagella, in accordance with environmental conditions (Yeung et al., 2009; Burrows, 2012; Khan et al., 2020). In this study, sennoside A was found to enable to restrain biofilm formation (Figure 5) and reduce the motility of swimming, swarming, and twitching significantly of P. aeruginosa (Figure 6).
Moreover, sennoside A could attenuate the pathogenicity of P. aeruginosa obviously because the rotten area in the cabbage stems caused by PAO1 was reduced (Figure 7A) and the survival rate of fruit flies and nematodes were increased significantly in the presence of sennoside A compared with those of the control group (Figures 7B,C).
To explore the target of sennoside A in QS inhibition of P. aeruginosa, the expression of lasB and pqsA was determined in QM-1, QM-2, or QM-3, respectively, in presence of corresponding exogenous signaling molecules of 3-oxo-C12-HSL, C4-HSL, and PQS with or without sennoside A. Sennoside A could suppress the gene expression only in the presence of the las system but not the rhl and pqs systems (Figure 9). Additionally, the deletion of lasR could eradicate the inhibitory effect of sennoside A on the pathogenicity of P. aeruginosa (Supplementary Figure 1). Together with the results derived from the molecular docking analysis (Figure 8), it could be concluded that LasR might be the essential target for the inhibition of sennoside A on QS-mediated virulence and pathogenicity of P. aeruginosa.
Moreover, the QS inhibition capacity of sennoside A on Gram-positive Staphylococcus aureus and Gram-negative Acinetobacter baumannii was further investigated by using a Chinese cabbage infection model. Interestingly, sennoside A could reduce the rotten area in the cabbage stems infected by A. baumannii but not those infected by S. aureus (Supplementary Figure 2), suggesting that sennoside A might show an inhibitive effect on QS of Gram-negative bacteria, but not for Gram-positive bacteria. P. aeruginosa and A. baumannii mainly use AHLs for cellular communication, whereas S. aureus uses autoinducing peptides as QS signaling molecules (Monnet and Gardan, 2015; Sun et al., 2021). An AHL-dependent QS system might be necessary for sennoside A to attenuate the pathogenicity of pathogens.
Traditional Chinese herbal medicines have been widely used for disease treatment, suggesting their safety for human consumption. Identifying potential QSIs from Chinese herbs is considered a feasible approach and some importance has been gained. Extracts from Herba patriniae (Fu et al., 2017) and Angelica dahurica (Chong et al., 2018) have been reported to enable to reduce the virulence and pathogenicity of P. aeruginosa, but without active components and targets identified. Some compounds derived from Chinese herbs have also been reported as QSIs. Falcarindiol from Notopterygium incisum could inhibit the QS of P. aeruginosa via destabilizing LasR (Zhao et al., 2021). Crude extract of Rheum palmatum L. has been previously found to be able to inhibit the QS of P. aeruginosa in our lab. Sennoside A is one of the main components of R. palmatum L. (Le et al., 2021). Sennoside A could probably respond to the inhibition of the extracts on the QS of P. aeruginosa, at least partially.
In summary, our results indicated that sennoside A could inhibit QS system of P. aeruginosa to attenuate its regulated virulence and pathogenicity via mainly targeting LasR, and further research to identify its anti-QS activity for other Gram-negative bacteria is warranted. Combining antibiotics with QSIs to synergistically treat bacterial infections would be an interesting and promising strategy.
The original contributions presented in this study are included in the article/Supplementary material, further inquiries can be directed to the corresponding author.
LS conceived and designed the experiments. XH performed the experiments and wrote the manuscript. MN and XC analyzed the experimental data. LC and BQ made some revisions. All authors contributed to the article and approved the submitted version.
This research was funded by the Provincial Natural Science Foundation of Shaanxi Province, grant number: 2018ZDXM-SF-004.
We thank Northwest University for providing the necessary materials used for experiments.
The authors declare that the research was conducted in the absence of any commercial or financial relationships that could be construed as a potential conflict of interest.
All claims expressed in this article are solely those of the authors and do not necessarily represent those of their affiliated organizations, or those of the publisher, the editors and the reviewers. Any product that may be evaluated in this article, or claim that may be made by its manufacturer, is not guaranteed or endorsed by the publisher.
The Supplementary Material for this article can be found online at: https://www.frontiersin.org/articles/10.3389/fmicb.2022.1042214/full#supplementary-material
Adonizio, A., Kong, K. F., and Mathee, K. (2008). Inhibition of quorum sensing-controlled virulence factor production in Pseudomonas aeruginosa by South Florida plant extracts. Antimicrob. Agents Chemother. 52, 198–203. doi: 10.1128/AAC.00612-07
Anwar, H., Dasgupta, M. K., and Costerton, J. W. (1990). Testing the susceptibility of bacteria in biofilms to antibacterial agents. Antimicrob Agents Chemother. 34, 2043–2046. doi: 10.1128/AAC.34.11.2043
Basha, A. M., El-Sherbiny, G. M., and Mabrouk, M. I. (2020). Phenotypic characterization of the Egyptian isolates “extensively drug-resistant Pseudomonas aeruginosa” and detection of their metallo-β-lactamases encoding genes. Bull. Natl. Res. Centre 44:350. doi: 10.1186/s42269-020-00350-8
Burrows, L. L. (2012). Pseudomonas aeruginosa twitching motility: Type IV pili in action. Annu Rev. Microbiol. 66, 493–520. doi: 10.1146/annurev-micro-092611-150055
Caiazza, N. C., Shanks, R. M., and O’Toole, G. A. (2005). Rhamnolipids modulate swarming motility patterns of Pseudomonas aeruginosa. J. Bacteriol. 187, 7351–7361. doi: 10.1128/JB.187.21.7351-7361.2005
Chong, Y. M., How, K. Y., Yin, W. F., and Chan, K. G. (2018). The effects of Chinese herbal medicines on the quorum sensing-regulated virulence in Pseudomonas aeruginosa PAO1. Molecules 23:972. doi: 10.3390/molecules23040972
Chugani, S. A., Whiteley, M., Lee, K. M., D’Argenio, D., Manoil, C., and Greenberg, E. P. (2001). QscR, a modulator of quorum-sensing signal synthesis and virulence in Pseudomonas aeruginosa. Proc. Natl. Acad. Sci. U.S.A. 98, 2752–2757. doi: 10.1073/pnas.051624298
Dai, L., Wu, T. Q., Xiong, Y. S., Ni, H. B., Ding, Y., Zhang, W. C., et al. (2019). Ibuprofen-mediated potential inhibition of biofilm development and quorum sensing in Pseudomonas aeruginosa. Life Sci. 237:116947. doi: 10.1016/j.lfs.2019.116947
Davey, M. E., Caiazza, N. C., and O’Toole, G. A. (2003). Rhamnolipid surfactant production affects biofilm architecture in Pseudomonas aeruginosa PAO1. J. Bacteriol. 185, 1027–1036. doi: 10.1128/JB.185.3.1027-1036.2003
Deziel, E., Lepine, F., Milot, S., He, J., Mindrinos, M. N., Tompkins, R. G., et al. (2004). Analysis of Pseudomonas aeruginosa 4-hydroxy-2-alkylquinolines (HAQs) reveals a role for 4-hydroxy-2-heptylquinoline in cell-to-cell communication. Proc. Natl. Acad. Sci. U.S.A. 101, 1339–1344. doi: 10.1073/pnas.0307694100
Driscoll, J. A., Brody, S. L., and Kollef, M. H. (2007). The epidemiology, pathogenesis and treatment of Pseudomonas aeruginosa infections. Drugs 67, 351–368. doi: 10.2165/00003495-200767030-00003
Essar, D. W., Eberly, L., Hadero, A., and Crawford, I. P. (1990). Identification and characterization of genes for a second anthranilate synthase in Pseudomonas aeruginosa: Interchangeability of the two anthranilate synthases and evolutionary implications. J Bacteriol. 172, 884–900. doi: 10.1128/jb.172.2.884-900.1990
Fu, B., Wu, Q., Dang, M., Bai, D., Guo, Q., Shen, L., et al. (2017). Inhibition of Pseudomonas aeruginosa biofilm formation by traditional chinese medicinal herb herba patriniae. Biomed Res Int. 2017:9584703. doi: 10.1155/2017/9584703
Gao, W., Jin, L., Liu, C., Zhang, N., Zhang, R., Bednarikova, Z., et al. (2021). Inhibition behavior of sennoside A and sennoside C on amyloid fibrillation of human lysozyme and its possible mechanism. Int. J. Biol. Macromol. 178, 424–433. doi: 10.1016/j.ijbiomac.2021.02.213
Groleau, M. C., de Oliveira Pereira, T., Dekimpe, V., and Deziel, E. (2020). PqsE is essential for RhlR-dependent quorum sensing regulation in Pseudomonas aeruginosa. mSystems 5:194. doi: 10.1128/mSystems.00194-20
He, X., Hwang, H. M., Aker, W. G., Wang, P., Lin, Y., Jiang, X., et al. (2014). Synergistic combination of marine oligosaccharides and azithromycin against Pseudomonas aeruginosa. Microbiol. Res. 169, 759–767. doi: 10.1016/j.micres.2014.01.001
Jadhav, M., Kalme, S., Tamboli, D., and Govindwar, S. (2011). Rhamnolipid from Pseudomonas desmolyticum NCIM-2112 and its role in the degradation of brown 3REL. J. Basic Microbiol. 51, 385–396. doi: 10.1002/jobm.201000364
Kalia, V. C., and Purohit, H. J. (2011). Quenching the quorum sensing system: Potential antibacterial drug targets. Crit. Rev. Microbiol. 37, 121–140. doi: 10.3109/1040841X.2010.532479
Karlberg, T., Hornyak, P., Pinto, A. F., Milanova, S., Ebrahimi, M., Lindberg, M., et al. (2018). 14-3-3 proteins activate Pseudomonas exotoxins-S and -T by chaperoning a hydrophobic surface. Nat. Commun. 9:3785. doi: 10.1038/s41467-018-06194-1
Kessler, E., and Safrin, M. (2014). Elastinolytic and proteolytic enzymes. Methods Mol. Biol. 1149, 135–169. doi: 10.1007/978-1-4939-0473-0_13
Khan, F., Pham, D. T. N., Oloketuyi, S. F., and Kim, Y. M. (2020). Regulation and controlling the motility properties of Pseudomonas aeruginosa. Appl. Microbiol. Biotechnol. 104, 33–49. doi: 10.1007/s00253-019-10201-w
Lau, G. W., Ran, H., Kong, F., Hassett, D. J., and Mavrodi, D. (2004). Pseudomonas aeruginosa pyocyanin is critical for lung infection in mice. Infect. Immun. 72, 4275–4278. doi: 10.1128/IAI.72.7.4275-4278.2004
Le, J., Ji, H., Zhou, X., Wei, X., Chen, Y., Fu, Y., et al. (2021). Pharmacology, toxicology, and metabolism of sennoside a, a medicinal plant-derived natural compound. Front Pharmacol. 12:714586. doi: 10.3389/fphar.2021.714586
Le, J., Zhang, X., Jia, W., Zhang, Y., Luo, J., Sun, Y., et al. (2019). Regulation of microbiota-GLP1 axis by sennoside A in diet-induced obese mice. Acta Pharm. Sin. B. 9, 758–768. doi: 10.1016/j.apsb.2019.01.014
Lee, J., and Zhang, L. (2015). The hierarchy quorum sensing network in Pseudomonas aeruginosa. Protein Cell. 6, 26–41. doi: 10.1007/s13238-014-0100-x
Levy, S. B., and Marshall, B. (2004). Antibacterial resistance worldwide: Causes, challenges and responses. Nat. Med. 10, S122–S129. doi: 10.1038/nm1145
Li, Y., Chen, L., Zhang, P., Bhagirath, A. Y., and Duan, K. (2020). ClpV3 of the H3-type VI secretion system (H3-T6SS) affects multiple virulence factors in Pseudomonas aeruginosa. Front. Microbiol. 11:1096. doi: 10.3389/fmicb.2020.01096
Luo, J., Dong, B., Wang, K., Cai, S., Liu, T., Cheng, X., et al. (2017). Baicalin inhibits biofilm formation, attenuates the quorum sensing-controlled virulence and enhances Pseudomonas aeruginosa clearance in a mouse peritoneal implant infection model. PLoS One. 12:e0176883. doi: 10.1371/journal.pone.0176883
Medina, E., and Pieper, D. H. (2016). Tackling threats and future problems of multidrug-resistant bacteria. Curr. Top. Microbiol. Immunol. 398, 3–33. doi: 10.1007/82_2016_492
Mengs, U. (1988). Toxic effects of sennosides in laboratory animals and in vitro. Pharmacology. 36, 180–187. doi: 10.1159/000138438
Monnet, V., and Gardan, R. (2015). Quorum-sensing regulators in gram-positive bacteria: ‘Cherchez le peptide’. Mol. Microbiol. 97, 181–184. doi: 10.1111/mmi.13060
Mukherjee, S., Moustafa, D., Smith, C. D., Goldberg, J. B., and Bassler, B. L. (2017). The RhlR quorum-sensing receptor controls Pseudomonas aeruginosa pathogenesis and biofilm development independently of its canonical homoserine lactone autoinducer. PLoS Pathog. 13:e1006504. doi: 10.1371/journal.ppat.1006504
Nassar, O., Desouky, S. E., El-Sherbiny, G. M., and Abu-Elghait, M. (2022). Correlation between phenotypic virulence traits and antibiotic resistance in Pseudomonas aeruginosa clinical isolates. Microb Pathog. 162:105339. doi: 10.1016/j.micpath.2021.105339
Norizan, S. N., Yin, W. F., and Chan, K. G. (2013). Caffeine as a potential quorum sensing inhibitor. Sensors (Basel). 13, 5117–5129. doi: 10.3390/s130405117
Ram Avtar, S. (2012). Antimicrobial activity of sennosides from Cassia pumila Lamk. J. Med. Plants Res. 6:335. doi: 10.5897/jmpr12.335
Sankar Ganesh, P., and Ravishankar Rai, V. (2018). Attenuation of quorum-sensing-dependent virulence factors and biofilm formation by medicinal plants against antibiotic resistant Pseudomonas aeruginosa. J. Tradit. Comple. Med. 8, 170–177. doi: 10.1016/j.jtcme.2017.05.008
Sarabhai, S., Sharma, P., and Capalash, N. (2013). Ellagic acid derivatives from Terminalia chebula retz. downregulate the expression of quorum sensing genes to attenuate Pseudomonas aeruginosa PAO1 virulence. PLoS One 8:e53441. doi: 10.1371/journal.pone.0053441
Schuster, M., and Greenberg, E. P. (2007). Early activation of quorum sensing in Pseudomonas aeruginosa reveals the architecture of a complex regulon. BMC Genom. 8:287. doi: 10.1186/1471-2164-8-287
Starkey, M., and Rahme, L. G. (2009). Modeling Pseudomonas aeruginosa pathogenesis in plant hosts. Nat Protoc. 4, 117–124. doi: 10.1038/nprot.2008.224
Stehling, E. G., Silveira, W. D., and Leite Dda, S. (2008). Study of biological characteristics of Pseudomonas aeruginosa strains isolated from patients with cystic fibrosis and from patients with extra-pulmonary infections. Braz J Infect Dis. 12, 86–88. doi: 10.1590/s1413-86702008000100018
Stover, C. K., Pham, X. Q., Erwin, A. L., Mizoguchi, S. D., Warrener, P., Hickey, M. J., et al. (2000). Complete genome sequence of Pseudomonas aeruginosa PAO1, an opportunistic pathogen. Nature. 406, 959–964. doi: 10.1038/35023079
Sun, X., Ni, Z., Tang, J., Ding, Y., Wang, X., and Li, F. (2021). The abaI/abaR quorum sensing system effects on pathogenicity in Acinetobacter baumannii. Front. Microbiol. 12:679241. doi: 10.3389/fmicb.2021.679241
Tremblay, J., Richardson, A. P., Lepine, F., and Deziel, E. (2007). Self-produced extracellular stimuli modulate the Pseudomonas aeruginosa swarming motility behaviour. Environ. Microbiol. 9, 2622–2630. doi: 10.1111/j.1462-2920.2007.01396.x
Wang, M., Zhao, L., Wu, H., Zhao, C., Gong, Q., and Yu, W. (2020). Cladodionen is a potential quorum sensing inhibitor against Pseudomonas aeruginosa. Mar. Drugs 18:205. doi: 10.3390/md18040205
Wang, S., Feng, Y., Han, X., Cai, X., Yang, L., Liu, C., et al. (2021). Inhibition of virulence factors and biofilm formation by wogonin attenuates pathogenicity of Pseudomonas aeruginosa PAO1 via targeting pqs quorum-sensing system. Int. J. Mol. Sci. 22:12699. doi: 10.3390/ijms222312699
Wei, Z., Shen, P., Cheng, P., Lu, Y., Wang, A., and Sun, Z. (2020). Gut bacteria selectively altered by sennoside A alleviate type 2 diabetes and obesity traits. Oxid. Med. Cell. Longev. 2020:2375676. doi: 10.1155/2020/2375676
Welsh, M. A., and Blackwell, H. E. (2016). Chemical probes of quorum sensing: From compound development to biological discovery. FEMS Microbiol. Rev. 40, 774–794. doi: 10.1093/femsre/fuw009
Whiteley, M., and Greenberg, E. P. (2001). Promoter specificity elements in Pseudomonas aeruginosa quorum-sensing-controlled genes. J. Bacteriol. 183, 5529–5534. doi: 10.1128/JB.183.19.5529-5534.2001
Xu, Z., Fang, X., Wood, T. K., and Huang, Z. J. (2013). A systems-level approach for investigating Pseudomonas aeruginosa biofilm formation. PLoS One 8:e57050. doi: 10.1371/journal.pone.0057050
Yang, P., Chen, Y., Jiang, S., Shen, P., Lu, X., and Xiao, Y. (2018). Association between antibiotic consumption and the rate of carbapenem-resistant gram-negative bacteria from China based on 153 tertiary hospitals data in 2014. Antimicrob. Resist. Infect. Control. 7:137. doi: 10.1186/s13756-018-0430-1
Yeung, A. T., Torfs, E. C., Jamshidi, F., Bains, M., Wiegand, I., Hancock, R. E., et al. (2009). Swarming of Pseudomonas aeruginosa is controlled by a broad spectrum of transcriptional regulators, including MetR. J. Bacteriol. 191, 5592–5602. doi: 10.1128/JB.00157-09
Keywords: Pseudomonas aeruginosa, quorum sensing, sennoside A, inhibition, virulence, pathogenicity
Citation: Han X, Nan M, Cai X, Qiao B, Chen L and Shen L (2022) Sennoside A inhibits quorum sensing system to attenuate its regulated virulence and pathogenicity via targeting LasR in Pseudomonas aeruginosa. Front. Microbiol. 13:1042214. doi: 10.3389/fmicb.2022.1042214
Received: 12 September 2022; Accepted: 12 October 2022;
Published: 03 November 2022.
Edited by:
Santi M. Mandal, Indian Institute of Technology Kharagpur, IndiaReviewed by:
Gamal Mohamed El-Said El-Sherbiny, Al-Azhar University, EgyptCopyright © 2022 Han, Nan, Cai, Qiao, Chen and Shen. This is an open-access article distributed under the terms of the Creative Commons Attribution License (CC BY). The use, distribution or reproduction in other forums is permitted, provided the original author(s) and the copyright owner(s) are credited and that the original publication in this journal is cited, in accordance with accepted academic practice. No use, distribution or reproduction is permitted which does not comply with these terms.
*Correspondence: Lixin Shen, c2hlbmx4QG53dS5lZHUuY24=
Disclaimer: All claims expressed in this article are solely those of the authors and do not necessarily represent those of their affiliated organizations, or those of the publisher, the editors and the reviewers. Any product that may be evaluated in this article or claim that may be made by its manufacturer is not guaranteed or endorsed by the publisher.
Research integrity at Frontiers
Learn more about the work of our research integrity team to safeguard the quality of each article we publish.