- 1Institute of Plant Protection, Henan Key Laboratory of Crop Pest Control/Key Laboratory of Integrated Pest Management on Crops in Southern Region of North China, Henan Academy of Agricultural Sciences, Zhengzhou, China
- 2School of Pharmaceutical Sciences, Laboratory of Synthetic Biology, Zhengzhou University, Zhengzhou, China
- 3Henan International Laboratory for Green Pest Control /College of Plant Protection, Henan Agricultural University, Zhengzhou, China
- 4College of Biological and Food Engineering, Anyang Institute of Technology, Anyang, China
Aphids are major crop pests, and they can be controlled through the application of the promising RNA interference (RNAi) techniques. However, chemical synthesis yield of dsRNA for RNAi is low and costly. Another sustainable aphid pest control strategy takes advantage of symbiont-mediated RNAi (SMR), which can generate dsRNA by engineered microbes. Aphid host the obligate endosymbiont Buchnera aphidicola and various facultative symbionts that not only have a wide host range but are also vertically and horizontally transmitted. Thus, we described the potential of facultative symbionts in aphid pest control by SMR. We summarized the community and host range of these facultative symbionts, and then reviewed their probable horizontal transmitted routes and ecological functions. Moreover, recent advances in the cultivation and genetic engineering of aphid facultative symbionts were discussed. In addition, current legislation of dsRNA-based pest control strategies and their safety assessments were reviewed.
Introduction
Aphids (Hemiptera: Aphididae) are tiny and soft-bodied insects. Over 5,000 aphid species have been identified globally, with roughly 10% of them being major agricultural and forest pests (Blackman and Eastop, 2017). In China, the harvest from 10 to 15 million hectares of wheat plantation is lost annually due to aphid infestations (Hu et al., 2016). Moreover, aphids are primary carriers of plant viruses, transmitting about half of all insect-vectored viruses (Ziegler-Graff, 2020). In addition, through their honeydew, aphids promote the growth of saprophytic ascomycetes on plants, resulting in sooty molds that hamper photosynthesis. Neonicotinoid pesticides are widely used to control aphids in agroecosystems. Due to their negative impact on the health and activity of pollinators (Henry et al., 2012; Tan et al., 2015; Tsvetkov et al., 2017), their use is restricted. Hence, more environmentally friendly aphid control techniques are of great interest.
In the revolutionary RNA interference technique (RNAi) (Bernstein et al., 2001; Bartel, 2004), double-stranded RNA (dsRNA) is delivered into host cells, triggering RNAi effects that ultimately inhibit the expression of the target gene. By specifically inhibiting the expression of essential genes in pests, RNAi is a potential pest management strategy, as it causes aberrant insect development and death (Vogel et al., 2018; Christiaens et al., 2020). Efficient delivery of intact and sufficient dsRNA into host cells and in aphids are essential for RNAi; this was realized by injection, topical application, and transgenic or artificial feed (Jaubert-Possamai et al., 2007; Abdellatef et al., 2015; Niu et al., 2019; Liu et al., 2021b). However, these delivery strategies are difficult to apply in aphid control, as they are labor-intensive and costly. Orally delivered dsRNA was degraded by aphid gut endonucleases (Christiaens et al., 2014; Ghodke et al., 2019). Bacterial symbionts of insects are potential producers and vectors of dsRNA (Guan et al., 2021). Through genetic engineering, these bacteria can be modified to constitutively synthesize and subsequently effectively deliver dsRNA within the pests, and this is referred to as symbiont-mediated RNAi (SMR) (Whitten et al., 2016).
Aphids have bacterial symbionts (Baumann, 2005), such as the obligate endosymbiont Buchnera aphidicola that supplies essential amino acids (Douglas, 1998). The co-evolution of this symbiosis is estimated to be 150–250 million years (Moran et al., 1993; Clark et al., 2000). Besides B. aphidicola, there exist other vertically transmitted symbiotic bacteria, and most are facultative symbionts (Russell et al., 2003). These facultative symbionts are potential SMR agents for the following reasons. Firstly, a few facultative symbionts are cultivable, and genetic engineering for dsRNA synthesis is possible. Secondly, facultative symbionts are not strictly associated with a specific host, enabling their infection of diverse aphid species. Thirdly, facultative symbionts are both vertically and horizontally transmitted and thus, dsRNA can be rapidly disseminated in an aphid population through genetically engineered strains.
The diversity of facultative symbionts of aphids and their occurrence were described, and the horizontal transmission routes and ecological functions of facultative symbionts in aphids potentially improved the dissemination of genetically modified symbionts in aphid populations were also discussed. Moreover, the cultivation and genetic engineering of facultative symbionts of aphids were highlighted. In this study, we reviewed recent advances of facultative symbionts as SMR agents for aphid control in insects.
Symbiont-mediated RNAi in insects
The features of dsRNA synthesis methods and dsRNA delivered routes were summarized (Supplementary Table S1). As the chemical synthesis yield of dsRNA is low and costs high, microbe-mediated strategies are developed to replace it (Guan et al., 2021). Through feeding and injecting of engineered strains, RNAi has been successfully performed in diverse insects (Tian et al., 2009; Zhu et al., 2011; Li et al., 2011c; Zhang et al., 2012; Vatanparast and Kim, 2017; Ma et al., 2020). The ubiquity of insect symbiotic bacteria (McFall-Ngai et al., 2013) makes them potential dsRNA expression agents (Whitten and Dyson, 2017). Bacterial SMR was successfully applied in kissing bugs (Rhodnius prolixus), western flower thrips (Frankliniella occidentalis), and honeybees (Apis mellifera). Rhodococcus rhodnii is a facultative gut symbiont of R. prolixus, and it supplies vitamin B to the host (Baines, 1956; Pachebat et al., 2013). The F. occidentalis bacterial symbiont strain BFo2 is closely related to the Pantoea genus. The dsRNA-expressing R. rhodnii and Bfo2 strains effectively infected R. prolixus and F. occidentalis, respectively, and subsequently triggered systemic RNAi in their hosts (Whitten et al., 2016). Snodgrassella alvi, a gut bacterium symbiont of A. mellifera, was genetically engineered to trigger RNAi of certain pathways, and it activated an antiviral response in A. mellifera. Furthermore, by inhibiting the expression of mite genes, S. alvi strains were used to kill parasitic Varroa mites in A. mellifera (Leonard et al., 2020).
Insects lack RNA-dependent RNA polymerases (RdRp) to amplify dsRNA, and they could not sustain RNAi. Hence, high amounts of dsRNA are required to trigger RNAi in insects. In aphids, a dsRNA concentration of 1,500 ng/μL was used for plant-mediated RNAi (Ding et al., 2017; Shang et al., 2020a, b). In the meanwhile, the optimum concentration of dsRNA for RNAi was ca. 350 ng/μL for Arabidopsis thaliana (Dubrovina et al., 2019). SMR ensured persistent synthesis of dsRNA and triggered sustained RNAi in insects (Whitten and Dyson, 2017), which filled the RNAi gaps between lab-scale studies and field pest control.
Facultative bacterial symbionts of aphids
Discovery of facultative symbionts of aphids
Seven groups of known facultative symbionts are PAR (pea aphid Rickettsia) (Chen et al., 1996), PASS (pea aphid secondary symbiont, R type) (Chen and Purcell, 1997), PABS (pea aphid Bemisia-like bacterium, T type) (Darby et al., 2001), PAUS (detected in US pea aphid strains, U type) (Sandström et al., 2001; Tsuchida et al., 2002), PAXS (pea aphid X-type symbiont, X type) (Guay et al., 2009), YSMS (Yamatocallis secondary mycetocyte symbiont) (Fukatsu, 2001), and SMLS (Sitobion miscanthi L type symbiont) (Li et al., 2011b). PASS is phylogenetically closely related to Serratia, and is thus called “Candidatus Serratia symbiotica.” Likewise, PABS and PAUS were designated as “Candidatus Hamiltonella defensa” and “Candidatus Regiella insecticola,” respectively (Moran et al., 2005), and PAXS as “Candidatus Fukatsuia symbiotica” (Manzano-Marin et al., 2017). F. symbiotica, H. defensa, and R. insecticola formed a clade in the family Yersiniaceae of the order Enterobacteriales (Patel et al., 2019). SMLS is a new genus of Rickettsiaceae that is closely related to Orientia tsutsugamushi (Li et al., 2011a). Three other arthropod-related symbionts were detected in aphids, i.e., Wolbachia (Jeyaprakash and Hoy, 2000), Spiroplasma (Cisak et al., 2015), and Arsenophonus (Novakova et al., 2009). Based on phylogenetic analysis, two monophyletic clades, provisionally designated as V and So-So, are proposed for facultative symbionts of aphids (Russell et al., 2003; Figure 1).
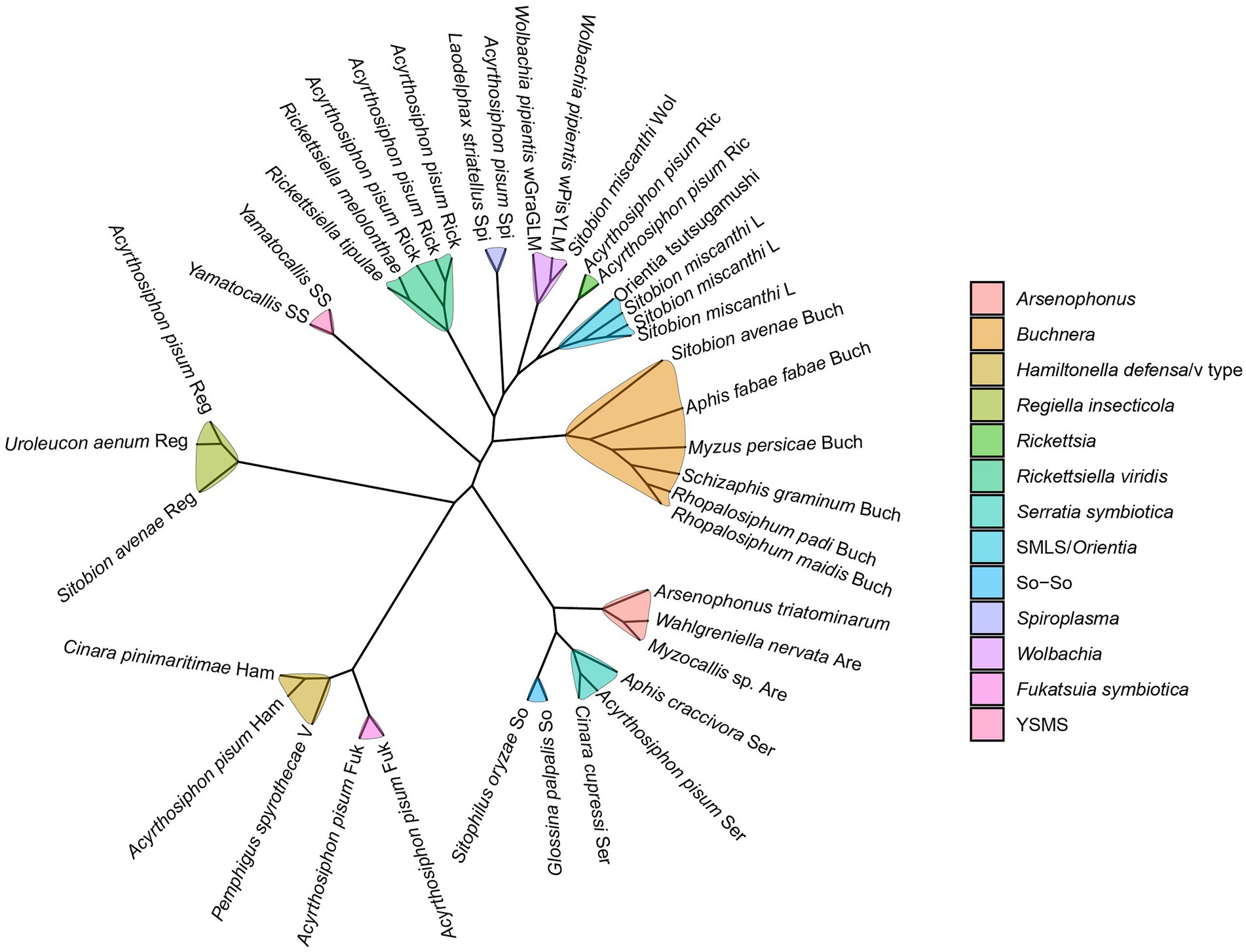
Figure 1. The cladogram of the aphid facultative symbionts. This cladogram is visualized by the ggtree R package (Yu et al., 2017). The information of the sequences used here is provided in the supplementary Supplementary Table S2.
Host range of facultative symbionts of aphids
S. symbiotica, H. defensa, and R. insecticola are the three dominant facultative symbionts of aphids, and they have been detected in 74, 53, and 38 species, respectively (Zytynska and Weisser, 2016). The Macrosiphini Aphid group is closely associated with all these three symbionts (Sandström et al., 2001; Haynes et al., 2003). Arsenophonus was detected in 1/3 tested aphids from the Aphis genus (Jousselin et al., 2012) and was widely distributed in Hormaphidinae species (Xu et al., 2021b). Wolbachia was widespread in Aphididae isolated from China, and 109 of the 114 tested aphid species (112 belonging to Aphididae) had at least a Wolbachia strain (Wang et al., 2014). In the social aphid Pseudoregma bambucicola, Wolbachia was the dominant facultative symbiont (Liu et al., 2021a). Moreover, Spiroplasma and Rickettsia were detected in several Aphis species, including A. craccivora (Brady et al., 2014), A. fabae (Zytynska et al., 2015), A. citricidus (Guidolin and Consoli, 2017), and A. aurantii from China (Deng et al., 2019). F. symbiotica was detected in Mollitrichosiphum at a prevalence of less than 1% (Qin et al., 2021). Besides S. miscanthi, SMLS was also detected in the wheat-feeding aphids Schizaphis graminum and Rhopalosiphum padi (Li et al., 2011a). It is clear that these facultative symbionts have diverse distribution patterns in aphids, which is probably correlated to climatic and geographical factors (Tsuchida et al., 2002; Russell et al., 2003; Xu et al., 2021a).
Transmission modes and ecological roles of facultative symbionts of aphids
Transmission modes
The probable horizontal transmission routes of these facultative symbionts are diverse (Figure 2). Their vertical transmission efficiency was almost 100% (Fukatsu et al., 2000; Darby and Douglas, 2003; Li et al., 2011b). However, closely related facultative symbionts were detected in distantly related aphid hosts, implying horizontal transmission was available (Sandström et al., 2001; Russell et al., 2003; Li et al., 2014). Parasitoid wasps transferred facultative symbionts to uninfected aphids via contaminated ovipositors (Gehrer and Vorburger, 2012). Parasitoid transmission efficiency depended on the titer and haplotype of facultative symbionts (Kaech and Vorburger, 2020). H. defensa was detected in the honeydew and siphuncular fluid of infected aphids, indicating probable oral horizontal transmission (Darby and Douglas, 2003). Moreover, H. defensa and S. symbiotica were transmitted horizontally via plant feeding (Li et al., 2018; Pons et al., 2019a). Cultivated S. symbiotica strains were transmitted extracellularly via honeydew of infected aphids (Pons et al., 2019b), showing the high prevalence of S. symbiotica infections in ant populations after interactions with infected aphids (Renoz et al., 2019). Although ectoparasitic mites are vectors of bacterial symbionts in fruit flies (Jaenike et al., 2007; Brown and Lloyd, 2015), symbiont transmission in black bean aphids do not occur through mites (Gehrer and Vorburger, 2012). The paternal transmission of facultative symbionts occurred in pea aphids (Moran and Dunbar, 2006), with seldom sexual transmission between different lineages (Peccoud et al., 2014). This natural transmission is proposed in laboratory environments where microinjection tools are employed to artificially transmit facultative symbionts of aphids.
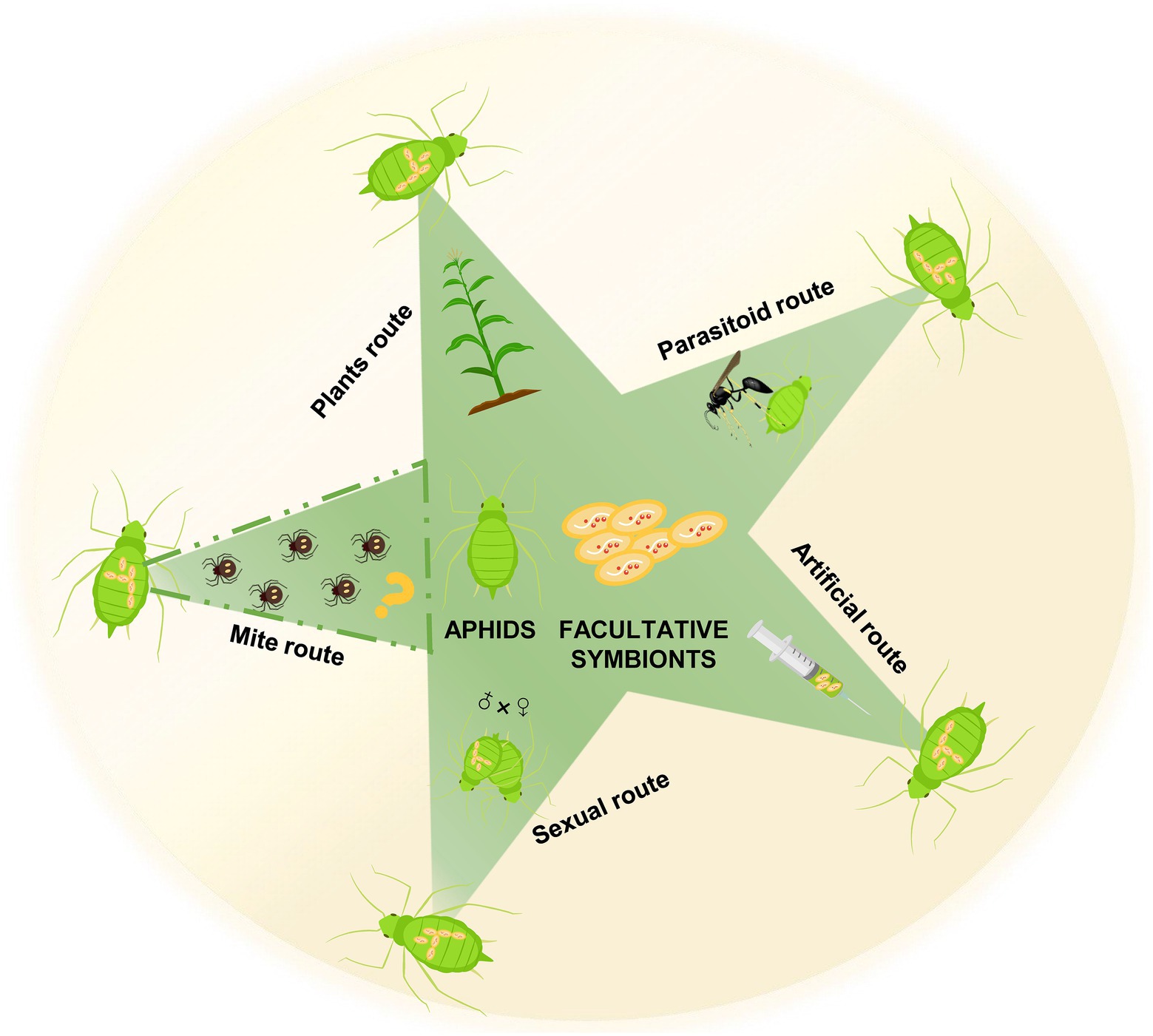
Figure 2. The potential horizontal transmission routes of aphid facultative symbionts. The question mark indicates that it is not clear whether aphid facultative symbionts can be horizontally transmitted by mites, though this route is often speculated.
Ecological roles
Temperature is a key environmental factor that affects aphids. S. symbiotica and Rickettsia infections increased the fitness of pea aphids under heat shock but decreased their fitness under normal temperature (Chen, 2000). R. insecticola and F. symbiotica infections increased the fecundity of aphids under heat stress (Heyworth et al., 2020). Parasitoid wasps and fungal pathogens are two key biotic factors affecting aphids. H. defensa infections conferred resistance to parasitoid attacks in aphids (Oliver et al., 2003). Moreover, the difference in levels of protection conferred by H. defensa strains was mainly due to co-infections by bacteriophages called APSEs (Brandt et al., 2017). R. insecticola infections increased the inclusive fitness of aphids under fungal attack by reducing the probability of fungal sporulation (Scarborough et al., 2005). Likewise, Rickettsia, Rickettsiella, and Spiroplasma spp. contributed to aphid resistance to fungal pathogens (Lukasik et al., 2012). Host specialization and body color are the main ecological characteristics of aphids. R. insecticola and Arsenophonus regulated the host usage in aphids (Tsuchida et al., 2004; Wagner et al., 2015). Specifically, Arsenophonus regulation was probably realized by changing the amino acid requirements in aphids (Tian et al., 2019). The endosymbiont R. viridis influenced prey–predator interactions by converting the body color of pea aphids from red to green (Tsuchida et al., 2010). Other endosymbionts affected the susceptibility of insects to insecticides (Kontsedalov et al., 2008; Ghanim and Kontsedalov, 2009; Liu and Guo, 2019). S. symbiotica infections increased the susceptibility of pea aphids to insecticides (Skaljac et al., 2018). Contrarily, in S. miscanthi, H. defensa infections decreased susceptibility to insecticides by probably increasing the activity of detoxifying enzymes (Li et al., 2021).
Interactions between aphids and facultative symbionts are complex. Apart from its facultative relationship with most aphid species, S. symbiotica form co-obligatory associations with B. aphidicola in members of Lachninae and Chaitophorinae (Lamelas et al., 2011; Manzano-Marin et al., 2017; Monnin et al., 2020). With the diversification of aphids, these dual symbiotic systems are replaced by those of distantly related bacterial symbionts (Manzano-Marin et al., 2017). Notably, a facultative symbiont can confer multiple fitness benefits to aphids. F. symbiotica like a “jack-of-all trades” symbiont, coinfect with other protective symbiont to provide resistance to parasitoid wasps, fungal pathogens, and heat stress (Heyworth and Ferrari, 2015). However, F. symbiotica infections cannot provide protective phenotype. These symbionts are more transmissible when other facultative symbionts infect the host (Doremus and Oliver, 2017). Furthermore, the protections conferred by facultative symbionts are costly. In the absence of stresses, these infections decrease aphid fitness (Gwynn et al., 2005; Vorburger, 2013; Kaech et al., 2022), hence, there exists a trade-off between the costs and benefits of facultative symbionts to aphids.
Cultivation and genetic engineering of aphid facultative symbionts
Following long coevolution with their hosts, the genomes of insect bacterial symbionts reduce significantly, and genes are lost in all functional categories (Moran and Bennett, 2014). Empirically, current unculturable bacteria genomes are less than 0.5 Mb. B. aphidicola genomes are between 0.4–0.6 Mb, and they contain genes encoding the biosynthesis of essential amino acids for aphids. However, they lack genes involved in the biosynthesis of lipopolysaccharides and phospholipids, which are crucial for free-living bacteria (Shigenobu et al., 2000; Wernegreen and Moran, 2000; Moran and Mira, 2001; Tamas et al., 2002). This minimal set of genes in endosymbiotic bacteria necessitates stable host-supported environments as their natural habitats, making them highly fastidious. The genomes of H. defensa and R. insecticola are 2.11 Mb and 2.07 Mb (Degnan et al., 2009a, b), respectively, whereas that of S. symbiotica is 3.0 Mb (Burke and Moran, 2011). Unlike B. aphidicola, the genomes of these three symbionts retained genes encoding pathways for the biosynthesis of cell surface components, DNA repair, and bacterial secretion systems. Thus, they are more adaptable than B. aphidicola and culturable in vitro (Masson and Lemaitre, 2020). Indeed, S. symbiotica was the first aphid facultative symbiont, to be cultured on axenic media (Sabri et al., 2010), and was followed by H. defensa and F. symbiotica (Brandt et al., 2017; Patel et al., 2019), providing the possibility to engineer microbes for SMR.
To date, a few insect bacterial symbionts have been genetically engineered. Symbionts of leafhoppers (Arora et al., 2018), mosquitoes (Wang et al., 2017), and termites (Tikhe et al., 2016) were successfully transformed with plasmids containing exogenous genes. The bacterial symbionts of tsetse flies (Kendra et al., 2020; Keller et al., 2021), and honey bees (Powell et al., 2016; Leonard et al., 2018), were genetically engineered via genome editing. Recently, one of the aphid main facultative symbionts, S. symbiotica, is successfully modified with a variety of genetic techniques. Furthermore, the modified strain of S. symbiotica can be used to infect aphids, and induce the expressed heterologous genes within its host (Elston et al., 2020).
Facultative symbionts can infect various aphid tissues, and are detected in aphid hemolymph. Moreover, the facultative symbionts can horizontally transmit among aphids. These tissue tropism and transmitted patterns of facultative symbionts, would improve the spread of the dsRNA, and then trigger the systemic RNAi in aphids. The titer of the facultative symbionts is another concern with applying SMR in aphid. The populations of three aphid facultative symbionts, i.e., S. symbiotica, Rickettsia, and SMLS are estimated to reach more than 108 per aphid (Koga et al., 2003; Sakurai et al., 2005; Li et al., 2011a). The populations of the SRM agents in F. occidentalis are estimated about 105 per insect, hence, the concentrations of facultative symbionts are enough for the SMR in aphid. The titer of the engineered S. symbiotica in aphids, would reach an average of about 108 colony-forming units (CFU) after proliferation for 5 days. The engineered facultative symbionts would also have strong colonization ability in aphids. Thus, genetically engineered facultative symbionts are possible strategies of SMR in the knockdown of aphid and plant virus genes for aphid control and paratransgenesis, respectively.
The international policies on RNAi-based pest control strategies
In U.S., RNAi-based pest control products are divided into two types: RNAi in Plant-Incorporated Protectants (PIPs), and non-PIP dsRNA end use products (dsRNA-EPs). For the PIPs, the United States Environmental Protection Agency (US-EPA) will organize a scientific advisory panel (SAP) to consider aspects of risk assessment, and evaluate whether the examined PIPs can meet the environmental safety standard of the Federal Insecticide Fungicide and Rodenticide Act (FIFRA). In the dsRNA-Eps, dsRNA is viewed as biochemical by US-EPA, and the approach used to assess the ecological risks of traditional chemical pesticides (conventional chemicals and biochemicals) are used as a basis for ecological risk assessment. Furthermore, according to the title 40 of the Code of Federal Regulations (CFR) part 158 enacted by EPA, dsRNA-Eps are required to provide evaluation about their pesticide active ingredients and products. The details for the legislation of RNAi-based pesticide in U.S. can further referred in the white paper released by US-EPA in 2013 (EPA, 2013).
In the European Union (EU), any plant protection product (PPP) needs an authorization before commercialization. The authorization process is divided in approval of the active substance and in the authorization of the PPP itself. An approval of the active substance is the precondition for the authorization of a PPP. In the EU, PPPs are divided into chemicals or microorganisms, and a biopesticide category is not available. Hence, PPPs based on dsRNA are seen as chemical PPPs (Dietz-Pfeilstetter et al., 2021). The European Food Safety Authority (EFSA) organized the risk assessments of active PPP substance. The guidance documents produced by OECD (Organization for Economic Cooperation and Development), EPPO (European and Mediterranean Plant Protection Organization), and EFSA complement the regulations of PPPs. In 2020, OECD released a report of the considerations for environmental risk assessment of the application of sprayed or externally applied dsRNA-based pesticides (OECD, 2020), which detailed the safety assessments of RNAi-based pesticides in EU.
In 2018, the New Zealand Environmental Protection Authority announced that eukaryotic cells or organisms treated with dsRNA were not new organisms, and the risk assessments of such treatments in the open environment were unnecessary (EPA, 2018). Thereafter, the New Zealand Ministry of Primary Industries classified RNA in the “Negligible Risk Register” (MPI, 2018). The regulations of dsRNA-based pest control strategies are easy in New Zealand, which will probably promote the developments of such strategies. However, the native New Zealand biosafety researchers reminded that out of the regulations of dsRNA treatments would probably cause potential environmental risks (Heinemann, 2019).
The safety assessment of RNAi-based pest control strategies
RNAi-mediated pest control strategies are the important parts of the future integrated pest management (IPM) system (Christiaens et al., 2020). To date, the risk assessment of RNAi-based genetically modified (GM) crops have been well documented. In EPA, 2013, US-EPA drafted a risk assessment guidance of RNAi-based crops (EPA, 2013). Subsequently, a scientific international workshop was organized by EFSA to evaluate the risk assessment of RNAi-based GM crops (European Food Safety Authority, 2014). As no new protein is generated by the RNAi-based GM crops, the safety assessment of RNAi-based products in EU, may not be complex as the transitional transgenic plants (Nitnavare et al., 2021). Diabrotica virgifera is a major pest during corn planting. In 2016, the commercialization of the first RNAi-based GM crop, MON87411, in the control of D. virgifera, was approved by Canadian Food Inspection Agency (CFIA), and then approved by US-EPA in 2017 (Zotti et al., 2018). In 2018–2021, EFSA evaluated the safety of MON87411 with other GM corns for food and feed uses. EFSA concluded that MON87411 was safe, and no safety concern was identified (EFSA Panel on Genetically Modified Organisms (GMO) et al., 2018, 2019, 2021). In 2021, the GMO safety certificate of MON87411 was approved by Ministry of Agriculture and Rural Affairs, PRC.
For human, insect bacterial symbionts are the friendly RNAi agents in pest control, as human have been exposed to insect bacterial symbionts for 1,000s of years (Popovici et al., 2010). To date, aphid facultative symbionts have only been identified in aphid species and their close insect relatives, such as whitefly (Darby et al., 2001; Bing et al., 2013). Although few studies evaluate the safety of aphid facultative symbionts to human, the systemic safety assessments of Wolbachia are referential. Wolbachia is the most widespread bacterial symbiont in insects, and has been used in the control of arboviruses transmission in Aedes mosquitoes. The safety assessments indicate that no Wolbachia related antigen is injected into the human volunteers, even after 1,000s of bites of Wolbachia infected mosquitoes (Popovici et al., 2010).
Increasing the specificity of dsRNA and reducing the off-target effects would strongly improve the safety of aphid SMR. The pest genomic information obtained from Genbank and the other insect genome sequencing project, such as i5k initiative, would effectively help us design aphid specific target sequences (Taning et al., 2021a, b). In the aphid SMR, employing aphid specific facultative symbiont as agents would provide a double insurance to avoid disturbing gene expressions in the non-target organisms (NTOs). Moreover, dsRNA is an environment friendly material, and the natural form of dsRNA is rapidly degraded in soil and aquatic systems within 2–7 days (Parker et al., 2019; Bachman et al., 2020). Additionally, the novel bacterial encapsulation methods would mitigate the environmental risk in aphid SMR (Arora et al., 2015).
However, some potential ecological risks should be noticed during the use of facultative symbionts in the aphid SMR. For instance, some H. defensa strains confer resistance to parasitoid attacks in aphid, hence, using of them as RNAi agents would negatively affect the biological control of parasitic wasps on aphids. Bacteriophages are known to be the key factor response to the parasitoid protection conferred by H. defensa (Brandt et al., 2017), using the bacteriophages negative H. defensa strains would avoid providing the parasitoid protection to aphids (Chuche et al., 2017). Furthermore, some species belonging to the same genus of aphid facultative symbionts are identified to be the plant pathogens (Salar et al., 2010; Bressan et al., 2012; Bressan, 2014; Dittmer et al., 2021). For aphids, the members of Spiroplasma, Arsenophonus, and Rickettsia are not recommended as the agents in SMR (Chuche et al., 2017).
Conclusions and future perspectives
In addition to nucleic and mitochondrial DNA, facultative symbionts are thought to be the third form of genetic material in aphids. In contrast to B. aphidicola, aphid facultative symbionts can infect diverse aphid tissues and species, and they are horizontally transmitted within aphid populations. Although in vitro cultivation of the symbionts was previously challenging, recent breakthroughs in culture of aphid facultative symbionts will undeniably enhance research studies in their usage. In the future, proper engineered symbionts can be potential microbial agents for aphid control via SMR, which would help in improving crop growth.
Author contributions
TL, YWe and CL conceived the study. TL, CZ, SL, SG and YZ drafted the manuscript. TL prepared the figures. CZ, SL and YWu revised the manuscript. TL, YWe and CL designed the whole study and revised the manuscript. All authors read, revised, and approved the manuscript.
Funding
This work was supported by Henan Province Key R&D and Promotion Project (grant no. 222102110317), Henan Provincial Science and Technology R&D Program Joint Fund (grant no. 222301420107), Major public welfare scientific research project of Henan Province (grant no. 201300111600), National Natural Science Foundation of China (grant no. 31601897), and Fund for Distinguished Young Scholars from Henan Academy of Agricultural Sciences (grant no. 2020JQ05).
Conflict of interest
The authors declare that the research was conducted in the absence of any commercial or financial relationships that could be construed as a potential conflict of interest.
Publisher’s note
All claims expressed in this article are solely those of the authors and do not necessarily represent those of their affiliated organizations, or those of the publisher, the editors and the reviewers. Any product that may be evaluated in this article, or claim that may be made by its manufacturer, is not guaranteed or endorsed by the publisher.
Supplementary material
The Supplementary material for this article can be found online at: https://www.frontiersin.org/articles/10.3389/fmicb.2022.1020461/full#supplementary-material
References
Abdellatef, E., Will, T., Koch, A., Imani, J., Vilcinskas, A., and Kogel, K. H. (2015). Silencing the expression of the salivary sheath protein causes transgenerational feeding suppression in the aphid Sitobion avenae. Plant Biotechnol. J. 13, 849–857. doi: 10.1111/pbi.12322
Arora, A. K., Forshaw, A., Miller, T. A., and Durvasula, R. (2015). A delivery system for field application of paratransgenic control. BMC Biotechnol. 15:59. doi: 10.1186/s12896-015-0175-3
Arora, A. K., Pesko, K. N., Quintero-Hernandez, V., Possani, L. D., Miller, T. A., and Durvasula, R. V. (2018). A paratransgenic strategy to block transmission of Xylella fastidiosa from the glassy-winged sharpshooter Homalodisca vitripennis. BMC Biotechnol. 18:50. doi: 10.1186/s12896-018-0460-z
Bachman, P., Fischer, J., Song, Z., Urbanczyk-Wochniak, E., and Watson, G. (2020). Environmental fate and dissipation of applied dsRNA in soil, aquatic systems, and plants. Front. Plant Sci. 11:21. doi: 10.3389/fpls.2020.00021
Baines, S. (1956). The role of the symbiotic bacteria in the nutrition of Rhodnius prolixus (Hemiptera). Exp. Biol. 33, 533–541. doi: 10.1242/jeb.33.3.533
Bartel, D. P. (2004). MicroRNAs: genomics, biogenesis, mechanism, and function. Cells 116, 281–297. doi: 10.1016/s0092-8674(04)00045-5
Baumann, P. (2005). Biology bacteriocyte-associated endosymbionts of plant sap-sucking insects. Annu. Rev. Microbiol. 59, 155–189. doi: 10.1146/annurev.micro.59.030804.121041
Bernstein, E., Caudy, A. A., Hammond, S. M., and Hannon, G. J. (2001). Role for a bidentate ribonuclease in the initiation step of RNA interference. Nature 409, 363–366. doi: 10.1038/35053110
Bing, X. L., Yang, J., Zchori-Fein, E., Wang, X. W., and Liu, S. S. (2013). Characterization of a newly discovered symbiont of the whitefly Bemisia tabaci (Hemiptera: Aleyrodidae). Appl. Environ. Microbiol. 79, 569–575. doi: 10.1128/AEM.03030-12
Blackman, R. L., and Eastop, V. F. (2017). Taxonomic issues, in Aphids as Crop Pests. eds. H. F. Emden van, R. Harrington (CABI), 1–36.
Brady, C. M., Asplen, M. K., Desneux, N., Heimpel, G. E., Hopper, K. R., Linnen, C. R., et al. (2014). Worldwide populations of the aphid Aphis craccivora are infected with diverse facultative bacterial symbionts. Microb. Ecol. 67, 195–204. doi: 10.1007/s00248-013-0314-0
Brandt, J. W., Chevignon, G., Oliver, K. M., and Strand, M. R. (2017). Culture of an aphid heritable symbiont demonstrates its direct role in defence against parasitoids. Proc. Biol. Sci. 284:20171925. doi: 10.1098/rspb.2017.1925
Bressan, A. (2014). Emergence and evolution of Arsenophonus bacteria as insect-vectored plant pathogens. Infect. Genet. Evol. 22, 81–90. doi: 10.1016/j.meegid.2014.01.004
Bressan, A., Terlizzi, F., and Credi, R. (2012). Independent origins of vectored plant pathogenic bacteria from arthropod-associated Arsenophonus endosymbionts. Microb. Ecol. 63, 628–638. doi: 10.1007/s00248-011-9933-5
Brown, A. N., and Lloyd, V. K. (2015). Evidence for horizontal transfer of Wolbachia by a Drosophila mite. Exp. Appl. Acarol. 66, 301–311. doi: 10.1007/s10493-015-9918-z
Burke, G. R., and Moran, N. A. (2011). Massive genomic decay in Serratia symbiotica, a recently evolved symbiont of aphids. Genome Biol. Evol. 3, 195–208. doi: 10.1093/gbe/evr002
Chen, D. Q. (2000). Fitness effects of two facultative endosymbiotic bacteria on the pea aphid, Acyrthosiphon pisum, and the blue alfalfa aphid. A. kondoi. Entomol. Exp. Appl. 95, 315–323. doi: 10.1046/j.1570-7458.2000.00670.x
Chen, D. Q., Campbell, B. C., and Purcell, A. H. (1996). A new rickettsia from a herbivorous insect, the pea aphid Acyrthosiphon pisum (Harris). Curr. Microbiol. 33, 123–128. doi: 10.1007/s002849900086
Chen, D. Q., and Purcell, A. H. (1997). Occurrence and transmission of facultative endosymbionts in aphids. Curr. Microbiol. 34, 220–225. doi: 10.1007/s002849900172
Christiaens, O., Niu, J., Taning, N. T., and C., (2020). RNAi in insects: a revolution in fundamental research and pest control applications. Insects 11:415. doi: 10.3390/insects11070415
Christiaens, O., Swevers, L., and Smagghe, G. (2014). DsRNA degradation in the pea aphid (Acyrthosiphon pisum) associated with lack of response in RNAi feeding and injection assay. Peptides 53, 307–314. doi: 10.1016/j.peptides.2013.12.014
Chuche, J., Auricau-Bouvery, N., Danet, J. L., and Thiéry, D. (2017). Use the insiders: could insect facultative symbionts control vector-borne plant diseases? J. Pest. Sci. 90, 51–68. doi: 10.1007/s10340-016-0782-3
Cisak, E., Wojcik-Fatla, A., Zajac, V., Sawczyn, A., Sroka, J., and Dutkiewicz, J. (2015). Spiroplasma -an emerging arthropod-borne pathogen? Ann. Agric. Environ. Med. 22, 589–593. doi: 10.5604/12321966.1185758
Clark, M. A., Moran, N. A., Baumann, P., and Wernegreen, J. J. (2000). Cospeciation between bacterial endosymbionts (Buchnera) and a recent radiation of aphids (Uroleucon) and pitfalls of testing for phylogenetic congruence. Evolution 54, 517–525. doi: 10.1111/j.0014-3820.2000.tb00054.x
Darby, A. C., Birkle, L. M., Turner, S. L., and Douglas, A. E. (2001). An aphid-borne bacterium allied to the secondary symbionts of whitefly. FEMS Microbiol. Ecol. 36, 43–50. doi: 10.1111/j.1574-6941.2001.tb00824.x
Darby, A. C., and Douglas, A. E. (2003). Elucidation of the transmission patterns of an insect-borne bacterium. Appl. Environ. Microbiol. 69, 4403–4407. doi: 10.1128/AEM.69.8.4403-4407.2003
Degnan, P. H., Leonardo, T. E., Cass, B. N., Hurwitz, B., Stern, D., Gibbs, R. A., et al. (2009a). Dynamics of genome evolution in facultative symbionts of aphids. Environ. Microbiol. 12, 2060–2069. doi: 10.1111/j.1462-2920.2009.02085.x
Degnan, P. H., Yu, Y., Sisneros, N., Wing, R. A., and Moran, N. A. (2009b). Hamiltonella defensa, genome evolution of protective bacterial endosymbiont from pathogenic ancestors. Proc. Natl. Acad. Sci. U. S. A. 106, 9063–9068. doi: 10.1073/pnas.0900194106
Deng, J., Liu, Q., Yu, Y. H., Li, J. J., and Huang, X. L. (2019). The detection and midgut intracellular location of rickettsia symbiont in the camellia aphid (Aphis aurantii). Appl. Ecol. Environ. Res. 17, 12203–12212. doi: 10.15666/aeer/1705_1220312212
Dietz-Pfeilstetter, A., Mendelsohn, M., Gathmann, A., and Klinkenbuss, D. (2021). Considerations and regulatory approaches in the USA and in the EU for dsRNA-based externally applied pesticides for plant protection. Front. Plant Sci. 12:682387. doi: 10.3389/fpls.2021.682387
Ding, B. Y., Shang, F., Zhang, Q., Xiong, Y., Yang, Q., Niu, J. Z., et al. (2017). Silencing of two insulin receptor genes disrupts nymph-adult transition of alate brown citrus aphid. Int. J. Mol. Sci. 18:357. doi: 10.3390/ijms18020357
Dittmer, J., Lusseau, T., Foissac, X., and Faoro, F. (2021). Skipping the insect vector: plant stolon transmission of the Phytopathogen 'Ca. Phlomobacter fragariae' from the Arsenophonus clade of insect endosymbionts. Insects 12:93. doi: 10.3390/insects12020093
Doremus, M. R., and Oliver, K. M. (2017). Aphid heritable symbiont exploits defensive mutualism. Appl. Environ. Microbiol. 83, e03276–e03216. doi: 10.1128/AEM.03276-16
Douglas, A. E. (1998). Nutritional interactions in insect-microbial symbioses: aphids and their symbiotic bacteria Buchnera. Annu. Rev. Entomol. 43, 17–37. doi: 10.1146/annurev.ento.43.1.17
Dubrovina, A. S., Aleynova, O. A., Kalachev, A. V., Suprun, A. R., Ogneva, Z. V., and Kiselev, K. V. (2019). Induction of transgene suppression in plants via external application of synthetic dsRNA. Int. J. Mol. Sci. 20:1585. doi: 10.3390/ijms20071585
EFSA Panel on Genetically Modified Organisms (GMO) Naegeli, H., Birch, A. N., Casacuberta, J., De Schrijver, A., Gralak, M. A., et al. (2018). Assessment of genetically modified maize MON 87411 for food and feed uses, import and processing, under regulation (EC) no 1829/2003 (application EFSA-GMO-NL-2015-124). EFSA J. 16:e05310. doi: 10.2903/j.efsa.2018.5310
EFSA Panel on Genetically Modified Organisms (GMO) Naegeli, H., Bresson, J. L., Dalmay, T., Dewhurst, I. C., Epstein, M. M., et al. (2019). Assessment of genetically modified maize MON 87427 x MON 89034 x MIR162 x MON 87411 and subcombinations, for food and feed uses, under regulation (EC) no 1829/2003 (application EFSA-GMO-NL-2017-144). EFSA J. 17:e05848. doi: 10.2903/j.efsa.2019.5848
EFSA Panel on Genetically Modified Organisms (GMO) Naegeli, H., Bresson, J. L., Dalmay, T., Dewhurst, I. C., Epstein, M. M., et al. (2021). Assessment of genetically modified maize MON 87427 x MON 87460 x MON 89034 x 1507 x MON 87411 x 59122 and subcombinations, for food and feed uses, under regulation (EC) no 1829/2003 (application EFSA-GMO-NL-2017-139). EFSA J. 19:e06351. doi: 10.2903/j.efsa.2021.6351
Elston, K. M., Perreau, J., Maeda, G. P., Moran, N. A., and Barrick, J. E. (2020). Engineering a culturable Serratia symbiotica strain for aphid paratransgenesis. Appl. Environ. Microbiol. 87: e02245-20. doi: 10.1128/AEM.02245-20
EPA. (2013). White paper on RNAi technology as a pesticide: problem formulation for human health and ecological risk assessment. (submitted to the FIFRA science advisory panel for review on comment. October 29, 2013). Available at: https://www.regulations.gov/document/EPA-HQ-OPP-2013-0485-0011 (accessed May 11, 2021).
EPA. (2018). Decision. Available at: https://www.epa.govt.nz/assets/FileAPI/hsno-ar/APP203395/APP203395_Decision.-Superceded-June-2021.pdf (Accessed May 1, 2018)
European Food Safety Authority (2014). International scientific workshop ‘risk assessment considerations for RNAi-based GM plants’. EFSA Support. Publ. 11:705. doi: 10.2903/sp.efsa.2014.EN-705
Fukatsu, T. (2001). Secondary intracellular symbiotic bacteria in aphids of the genus Yamatocallis (Homoptera: Aphididae: Drepanosiphinae). Appl. Environ. Microbiol. 67, 5315–5320. doi: 10.1128/AEM.67.11.5315-5320.2001
Fukatsu, T., Nikoh, N., Kawai, R., and Koga, R. (2000). The secondary endosymbiotic bacterium of the pea aphid Acyrthosiphon pisum (Insecta: homoptera). Appl. Environ. Microbiol. 66, 2748–2758. doi: 10.1128/aem.66.7.2748-2758.2000
Gehrer, L., and Vorburger, C. (2012). Parasitoids as vectors of facultative bacterial endosymbionts in aphids. Biol. Lett. 8, 613–615. doi: 10.1098/rsbl.2012.0144
Ghanim, M., and Kontsedalov, S. (2009). Susceptibility to insecticides in the Q biotype of Bemisia tabaci is correlated with bacterial symbiont densities. Pest Manag. Sci. 65, 939–942. doi: 10.1002/ps.1795
Ghodke, A. B., Good, R. T., Golz, J. F., Russell, D. A., Edwards, O., and Robin, C. (2019). Extracellular endonucleases in the midgut of Myzus persicae may limit the efficacy of orally delivered RNAi. Sci. Rep. 9:11898. doi: 10.1038/s41598-019-47357-4
Guan, R., Chu, D., Han, X., Miao, X., and Li, H. (2021). Advances in the development of microbial double-stranded RNA production systems for application of RNA interference in agricultural pest control. Front. Bioeng. Biotechnol. 9:753790. doi: 10.3389/fbioe.2021.753790
Guay, J. F., Boudreault, S., Michaud, D., and Cloutier, C. (2009). Impact of environmental stress on aphid clonal resistance to parasitoids: role of Hamiltonella defensa bacterial symbiosis in association with a new facultative symbiont of the pea aphid. J. Insect Physiol. 55, 919–926. doi: 10.1016/j.jinsphys.2009.06.006
Guidolin, A. S., and Consoli, F. L. (2017). Symbiont diversity of aphis (Toxoptera) citricidus (Hemiptera: Aphididae) as influenced by host plants. Microb. Ecol. 73, 201–210. doi: 10.1007/s00248-016-0892-8
Gwynn, D. M., Callaghan, A., Gorham, J., Walters, K. F., and Fellowes, M. D. (2005). Resistance is costly: trade-offs between immunity, fecundity and survival in the pea aphid. Proc. Biol. Sci. 272, 1803–1808. doi: 10.1098/rspb.2005.3089
Haynes, S., Darby, A. C., Daniell, T. J., Webster, G., Van Veen, F. J., Godfray, H. C., et al. (2003). Diversity of bacteria associated with natural aphid populations. Appl. Environ. Microbiol. 69, 7216–7223. doi: 10.1128/AEM.69.12.7216-7223.2003
Heinemann, J. A. (2019). Should dsRNA treatments applied in outdoor environments be regulated? Environ. Int. 132:104856. doi: 10.1016/j.envint.2019.05.050
Henry, M., Beguin, M., Requier, F., Rollin, O., Odoux, J. F., Aupinel, P., et al. (2012). A common pesticide decreases foraging success and survival in honey bees. Science 336, 348–350. doi: 10.1126/science.1215039
Heyworth, E. R., and Ferrari, J. (2015). A facultative endosymbiont in aphids can provide diverse ecological benefits. J. Evol. Biol. 28, 1753–1760. doi: 10.1111/jeb.12705
Heyworth, E. R., Smee, M. R., and Ferrari, J. (2020). Aphid facultative symbionts aid recovery of their obligate symbiont and their host after heat stress. Front. Ecol. Evol. 8, 1–10. doi: 10.3389/fevo.2020.00056
Hu, X. S., Liu, Y. J., Wang, Y. H., Wang, Z., Yu, X. L., Wang, B., et al. (2016). Resistance of wheat accessions to the english grain aphid Sitobion avenae. PLoS One 11:e0156158. doi: 10.1371/journal.pone.0156158
Jaenike, J., Polak, M., Fiskin, A., Helou, M., and Minhas, M. (2007). Interspecific transmission of endosymbiotic Spiroplasma by mites. Biol. Lett. 3, 23–25. doi: 10.1098/rsbl.2006.0577
Jaubert-Possamai, S., Le Trionnaire, G., Bonhomme, J., Christophides, G. K., Rispe, C., and Tagu, D. (2007). Gene knockdown by RNAi in the pea aphid Acyrthosiphon pisum. BMC Biotechnol. 7:63. doi: 10.1186/1472-6750-7-63
Jeyaprakash, A., and Hoy, M. A. (2000). Long PCR improves Wolbachia DNA amplification: wsp sequences found in 76% of sixty-three arthropod species. Insect Mol. Biol. 9, 393–405. doi: 10.1046/j.1365-2583.2000.00203.x
Jousselin, E., Coeur d'Acier, A., Vanlerberghe-Masutti, F., and Duron, O. (2012). Evolution and diversity of Arsenophonus endosymbionts in aphids. Mol. Ecol. 22, 260–270. doi: 10.1111/mec.12092
Kaech, H., Jud, S., and Vorburger, C. (2022). Similar cost of Hamiltonella defensa in experimental and natural aphid-endosymbiont associations. Ecol. Evol. 12:e8551. doi: 10.1002/ece3.8551
Kaech, H., and Vorburger, C. (2020). Horizontal transmission of the heritable protective endosymbiont Hamiltonella defensa depends on titre and haplotype. Front. Microbiol. 11:628755. doi: 10.3389/fmicb.2020.628755
Keller, C. M., Kendra, C. G., Bruna, R. E., Craft, D., and Pontes, M. H. (2021). Genetic modification of Sodalis species by dNA transduction. mSphere 6:6. doi: 10.1128/mSphere.01331-20
Kendra, C. G., Keller, C. M., Bruna, R. E., and Pontes, M. H. (2020). Conjugal DNA transfer in Sodalis glossinidius, a maternally inherited symbiont of tsetse flies. mSphere 5:e00864-20. doi: 10.1128/mSphere.00864-20
Koga, R., Tsuchida, T., and Fukatsu, T. (2003). Changing partners in an obligate symbiosis: a facultative endosymbiont can compensate for loss of the essential endosymbiont Buchnera in an aphid. Proc. Biol. Sci. 270, 2543–2550. doi: 10.1098/rspb.2003.2537
Kontsedalov, S., Zchori-Fein, E., Chiel, E., Gottlieb, Y., Inbar, M., and Ghanim, M. (2008). The presence of rickettsia is associated with increased susceptibility of Bemisia tabaci (Homoptera: Aleyrodidae) to insecticides. Pest Manag. Sci. 64, 789–792. doi: 10.1002/ps.1595
Lamelas, A., Gosalbes, M. J., Manzano-Marin, A., Pereto, J., Moya, A., and Latorre, A. (2011). Serratia symbiotica from the aphid Cinara cedri: a missing link from facultative to obligate insect endosymbiont. PLoS Genet. 7:e1002357. doi: 10.1371/journal.pgen.1002357
Leonard, S. P., Perutka, J., Powell, J. E., Geng, P., Richhart, D. D., Byrom, M., et al. (2018). Genetic engineering of bee gut microbiome bacteria with a toolkit for modular assembly of broad-host-range plasmids. ACS Synth. Biol. 7, 1279–1290. doi: 10.1021/acssynbio.7b00399
Leonard, S. P., Powell, J. E., Perutka, J., Geng, P., Heckmann, L. C., Horak, R. D., et al. (2020). Engineered symbionts activate honey bee immunity and limit pathogens. Science 367, 573–576. doi: 10.1126/science.aax9039
Li, Q., Fan, J., Sun, J., Wang, M. Q., and Chen, J. (2018). Plant-mediated horizontal transmission of Hamiltonella defensa in the wheat aphid Sitobion miscanthi. J. Agric. Food Chem. 66, 13367–13377. doi: 10.1021/acs.jafc.8b04828
Li, Q., Sun, J., Qin, Y., Fan, J., Zhang, Y., Tan, X., et al. (2021). Reduced insecticide susceptibility of the wheat aphid Sitobion miscanthi after infection by the secondary bacterial symbiont Hamiltonella defensa. Pest Manag. Sci. 77, 1936–1944. doi: 10.1002/ps.6221
Li, T., Xiao, J. H., Wu, Y. Q., and Huang, D. W. (2014). Diversity of bacterial symbionts in populations of Sitobion miscanthi (Hemiptera: Aphididae) in China. Environ. Entomol. 43, 605–611. doi: 10.1603/EN13229
Li, T., Xiao, J. H., Xu, Z. H., Murphy, R. W., and Huang, D. W. (2011a). Cellular tropism, population dynamics, host range and taxonomic status of an aphid secondary symbiont, SMLS (Sitobion miscanthi L type symbiont). PLoS One 6:e21944. doi: 10.1371/journal.pone.0021944
Li, T., Xiao, J. H., Xu, Z. H., Murphy, R. W., and Huang, D. W. (2011b). A possibly new rickettsia-like genus symbiont is found in Chinese wheat pest aphid, Sitobion miscanthi (Hemiptera: Aphididae). J. Invertebr. Pathol. 106, 418–421. doi: 10.1016/j.jip.2010.12.003
Li, X., Zhang, M., and Zhang, H. (2011c). RNA interference of four genes in adult Bactrocera dorsalis by feeding their dsRNAs. PLoS One 6:e17788. doi: 10.1371/journal.pone.0017788
Liu, X. D., and Guo, H. F. (2019). Importance of endosymbionts Wolbachia and rickettsia in insect resistance development. Curr. Opin. Insect Sci. 33, 84–90. doi: 10.1016/j.cois.2019.05.003
Liu, S. S., Ladera-Carmona, M. J., Poranen, M. M., van Bel, A. J. E., Kogel, K. H., and Imani, J. (2021b). Evaluation of dsRNA delivery methods for targeting macrophage migration inhibitory factor MIF in RNAi-based aphid control. J. Plant. Dis. Protect. 128, 1201–1212. doi: 10.1007/s41348-021-00464-9
Liu, Q., Zhang, H., Zeng, L., Yu, Y., Lin, X., and Huang, X. (2021a). Coexistence of three dominant bacterial symbionts in a social aphid and implications for ecological adaptation. Insects 12:416. doi: 10.3390/insects12050416
Lukasik, P., van Asch, M., Guo, H., Ferrari, J., Charles, J. G. H., and van der Putten, W. (2012). Unrelated facultative endosymbionts protect aphids against a fungal pathogen. Ecol. Lett. 16, 214–218. doi: 10.1111/ele.12031
Ma, Z. Z., Zhou, H., Wei, Y. L., Yan, S., and Shen, J. (2020). A novel plasmid-Escherichia coli system produces large batch dsRNAs for insect gene silencing. Pest Manag. Sci. 76, 2505–2512. doi: 10.1002/ps.5792
Manzano-Marin, A., Szabo, G., Simon, J. C., Horn, M., and Latorre, A. (2017). Happens in the best of subfamilies: establishment and repeated replacements of co-obligate secondary endosymbionts within Lachninae aphids. Environ. Microbiol. 19, 393–408. doi: 10.1111/1462-2920.13633
Masson, F., and Lemaitre, B. (2020). Growing ungrowable bacteria: overview and perspectives on insect symbiont culturability. Microbiol. Mol. Biol. Rev. 84: e00089-20. doi: 10.1128/MMBR.00089-20
McFall-Ngai, M., Hadfield, M. G., Bosch, T. C., Carey, H. V., Domazet-Loso, T., Douglas, A. E., et al. (2013). Animals in a bacterial world, a new imperative for the life sciences. Proc. Natl. Acad. Sci. U. S. A. 110, 3229–3236. doi: 10.1073/pnas.1218525110
Monnin, D., Jackson, R., Kiers, E. T., Bunker, M., Ellers, J., and Henry, L. M. (2020). Parallel evolution in the integration of a co-obligate aphid symbiosis. Curr. Biol. 30:e1946, 1949–1957.e6. doi: 10.1016/j.cub.2020.03.011
Moran, N. A., and Bennett, G. M. (2014). The tiniest tiny genomes. Annu. Rev. Microbiol. 68, 195–215. doi: 10.1146/annurev-micro-091213-112901
Moran, N. A., and Dunbar, H. E. (2006). Sexual acquisition of beneficial symbionts in aphids. Proc. Natl. Acad. Sci. U. S. A. 103, 12803–12806. doi: 10.1073/pnas.0605772103
Moran, N. A., and Mira, A. (2001). The process of genome shrinkage in the obligate symbiont Buchnera aphidicola. Genome Biol. 2:RESEARCH0054. doi: 10.1186/gb-2001-2-12-research0054
Moran, N. A., Munson, M. A., Baumann, P., and Ishikawa, H. (1993). A molecular clock in endosymbiotic bacteria is calibrated using the insect hosts. Proc. Biol. Sci. 253, 167–171. doi: 10.1098/rspb.1993.0098
Moran, N. A., Russell, J. A., Koga, R., and Fukatsu, T. (2005). Evolutionary relationships of three new species of Enterobacteriaceae living as symbionts of aphids and other insects. Appl. Environ. Microbiol. 71, 3302–3310. doi: 10.1128/AEM.71.6
MPI (2018). Declaration for potential risk goods in catalogues. Available at: https://www.mpi.govt. nz/dmsdocument/5134/send (Accessed June 7 2018).
Nitnavare, R. B., Bhattacharya, J., Singh, S., Kour, A., Hawkesford, M. J., and Arora, N. (2021). Next generation dsRNA-based insect control: success so far and challenges. Front. Plant Sci. 12:673576. doi: 10.3389/fpls.2021.673576
Niu, J., Yang, W. J., Tian, Y., Fan, J. Y., Ye, C., Shang, F., et al. (2019). Topical dsRNA delivery induces gene silencing and mortality in the pea aphid. Pest Manag. Sci. 75, 2873–2881. doi: 10.1002/ps.5457
Novakova, E., Hypsa, V., and Moran, N. A. (2009). Arsenophonus, an emerging clade of intracellular symbionts with a broad host distribution. BMC Microbiol. 9:143. doi: 10.1186/1471-2180-9-143
OECD (2020). Considerations for the environmental risk assessment of the application of sprayed or externally applied dsRNA-based pesticides. Series on pesticides no. 104, ENV/JM/MONO (2020) 26 (Paris).
Oliver, K. M., Russell, J. A., Moran, N. A., and Hunter, M. S. (2003). Facultative bacterial symbionts in aphids confer resistance to parasitic wasps. Proc. Natl. Acad. Sci. U. S. A. 100, 1803–1807. doi: 10.1073/pnas.0335320100
Pachebat, J. A., van Keulen, G., Whitten, M. M., Girdwood, S., Del Sol, R., Dyson, P. J., et al. (2013). Draft genome sequence of Rhodococcus rhodnii strain LMG5362, a symbiont of Rhodnius prolixus (Hemiptera, Reduviidae, Triatominae), the principle vector of Trypanosoma cruzi. Genome Announc. 1:e00329-13. doi: 10.1128/genomeA.00329-13
Parker, K. M., Borrero, V. B., van Leeuwen, D. M., Lever, M. A., Mateescu, B., and Sander, M. (2019). Environmental fate of RNA interference pesticides: adsorption and degradation of double-stranded RNA molecules in agricultural soils. Environ. Sci. Technol. 53, 3027–3036. doi: 10.1021/acs.est.8b05576
Patel, V., Chevignon, G., Manzano-Marin, A., Brandt, J. W., Strand, M. R., Russell, J. A., et al. (2019). Cultivation-assisted genome of Candidatus Fukatsuia symbiotica; the enigmatic “X-type” symbiont of aphids. Genome Biol. Evol. 11, 3510–3522. doi: 10.1093/gbe/evz252
Peccoud, J., Bonhomme, J., Maheo, F., de la Huerta, M., Cosson, O., and Simon, J. C. (2014). Inheritance patterns of secondary symbionts during sexual reproduction of pea aphid biotypes. Insect Sci. 21, 291–300. doi: 10.1111/1744-7917.12083
Pons, I., Renoz, F., Noel, C., and Hance, T. (2019a). Circulation of the cultivable symbiont Serratia symbiotica in aphids is mediated by plants. Front. Microbiol. 10:764. doi: 10.3389/fmicb.2019.00764
Pons, I., Renoz, F., Noël, C., and Hance, T. (2019b). New insights into the nature of symbiotic associations in aphids: infection process, biological effects and transmission mode of cultivable Serratia symbiotica bacteria. Appl. Environ. Microbiol. 85, e02445–e02418. doi: 10.1128/AEM.02445-18
Popovici, J., Moreira, L. A., Poinsignon, A., Iturbe-Ormaetxe, I., McNaughton, D., and O'Neill, S. L. (2010). Assessing key safety concerns of a Wolbachia-based strategy to control dengue transmission by Aedes mosquitoes. Mem. Inst. Oswaldo Cruz 105, 957–964. doi: 10.1590/s0074-02762010000800002
Powell, J. E., Leonard, S. P., Kwong, W. K., Engel, P., and Moran, N. A. (2016). Genome-wide screen identifies host colonization determinants in a bacterial gut symbiont. Proc. Natl. Acad. Sci. U. S. A. 113, 13887–13892. doi: 10.1073/pnas.1610856113
Qin, M., Chen, J., Xu, S., Jiang, L., and Qiao, G. (2021). Microbiota associated with Mollitrichosiphum aphids (Hemiptera: Aphididae: Greenideinae): diversity, host species specificity and phylosymbiosis. Environ. Microbiol. 23, 2184–2198. doi: 10.1111/1462-2920.15391
Renoz, F., Pons, I., Vanderpoorten, A., Bataille, G., Noel, C., Foray, V., et al. (2019). Evidence for gut-associated Serratia symbiotica in wild aphids and ants provides new perspectives on the evolution of bacterial mutualism in insects. Microb. Ecol. 78, 159–169. doi: 10.1007/s00248-018-1265-2
Russell, J. A., Latorre, A., Sabater-Munoz, B., Moya, A., and Moran, N. A. (2003). Side-stepping secondary symbionts: widespread horizontal transfer across and beyond the Aphidoidea. Mol. Ecol. 12, 1061–1075. doi: 10.1046/j.1365-294x.2003.01780.x
Sabri, A., Leroy, P., Haubruge, E., Hance, T., Frere, I., Destain, J., et al. (2010). Isolation, pure culture and characterization of Serratia symbiotica, the R-type of secondary endosymbionts of the black bean aphid Aphis fabae. Int. J. Syst. Evol. Microbiol. 61, 2081–2088. doi: 10.1099/ijs.0.024133-0
Sakurai, M., Koga, R., Tsuchida, T., Meng, X. Y., and Fukatsu, T. (2005). Rickettsia symbiont in the pea aphid Acyrthosiphon pisum: novel cellular tropism, effect on host fitness, and interaction with the essential symbiont Buchnera. Appl. Environ. Microbiol. 71, 4069–4075. doi: 10.1128/AEM.71.7.4069-4075.2005
Salar, P., Semetey, O., Danet, J. L., Boudon-Padieu, E., and Foissac, X. (2010). 'Candidatus Phlomobacter fragariae' and the proteobacterium associated with the low sugar content syndrome of sugar beet are related to bacteria of the arsenophonus clade detected in hemipteran insects. Eur. J. Plant Pathol. 126, 123–127. doi: 10.1007/s10658-009-9520-5
Sandström, J. P., Russell, J. A., White, J. P., and Moran, N. A. (2001). Independent origins and horizontal transfer of bacterial symbionts of aphids. Mol. Ecol. 10, 217–228. doi: 10.1046/j.1365-294x.2001.01189.x
Scarborough, C. L., Ferrari, J., and Godfray, H. C. (2005). Aphid protected from pathogen by endosymbiont. Science 310:1781. doi: 10.1126/science.1120180
Shang, F., Ding, B. Y., Ye, C., Yang, L., Chang, T. Y., Xie, J., et al. (2020a). Evaluation of a cuticle protein gene as a potential RNAi target in aphids. Pest Manag. Sci. 76, 134–140. doi: 10.1002/ps.5599
Shang, F., Niu, J., Ding, B. Y., Zhang, W., Wei, D. D., Wei, D., et al. (2020b). The miR-9b microRNA mediates dimorphism and development of wing in aphids. Proc. Natl. Acad. Sci. U. S. A. 117, 8404–8409. doi: 10.1073/pnas.1919204117
Shigenobu, S., Watanabe, H., Hattori, M., Sakaki, Y., and Ishikawa, H. (2000). Genome sequence of the endocellular bacterial symbiont of aphids Buchnera sp. APS. Nat. 407, 81–86. doi: 10.1038/35024074
Skaljac, M., Kirfel, P., Grotmann, J., and Vilcinskas, A. (2018). Fitness costs of infection with Serratia symbiotica are associated with greater susceptibility to insecticides in the pea aphid Acyrthosiphon pisum. Pest Manag. Sci. 74, 1829–1836. doi: 10.1002/ps.4881
Tamas, I., Klasson, L., Canback, B., Naslund, A. K., Eriksson, A. S., Wernegreen, J. J., et al. (2002). 50 million years of genomic stasis in endosymbiotic bacteria. Science 296, 2376–2379. doi: 10.1126/science.1071278
Tan, K., Chen, W., Dong, S., Liu, X., Wang, Y., and Nieh, J. C. (2015). A neonicotinoid impairs olfactory learning in Asian honey bees (Apis cerana) exposed as larvae or as adults. Sci. Rep. 5:10989. doi: 10.1038/srep10989
Taning, C. N. T., Gui, S. H., Schutter, K. D., Jahani, M., Castellanos, N. L., Christiaens, O., et al. (2021a). A sequence complementarity based approach for evaluating off-target transcript knock down in Bombus terrestris, following ingestion of pest specific dsRNA. J. Pest. Sci. 94, 487–503. doi: 10.1007/s10340-020-01273-z
Taning, C. N. T., Mezzetti, B., Kleter, G., Smagghe, G., and Baraldi, E. (2021b). Does RNAi-based technology fit within EU sustainability goals? Trends Biotechnol. 39, 644–647. doi: 10.1016/j.tibtech.2020.11.008
Tian, P. P., Chang, C. Y., Miao, N. H., Li, M. Y., and Liu, X. D. (2019). Infections with Arsenophonus facultative endosymbionts alter performance of aphids (Aphis gossypii) on an amino-acid-deficient diet. Appl. Environ. Microbiol. 85, e01407–e01419. doi: 10.1128/AEM.01407-19
Tian, H., Peng, H., Yao, Q., Chen, H., Xie, Q., Tang, B., et al. (2009). Developmental control of a lepidopteran pest Spodoptera exigua by ingestion of bacteria expressing dsRNA of a non-midgut gene. PLoS One 4:e6225. doi: 10.1371/journal.pone.0006225
Tikhe, C. V., Martin, T. M., Howells, A., Delatte, J., and Husseneder, C. (2016). Assessment of genetically engineered Trabulsiella odontotermitis as a “Trojan horse” for paratransgenesis in termites. BMC Microbiol. 16:202. doi: 10.1186/s12866-016-0822-4
Tsuchida, T., Koga, R., and Fukatsu, T. (2004). Host plant specialization governed by facultative symbiont. Science 303:1989. doi: 10.1126/science.1094611
Tsuchida, T., Koga, R., Horikawa, M., Tsunoda, T., Maoka, T., Matsumoto, S., et al. (2010). Symbiotic bacterium modifies aphid body color. Science 330, 1102–1104. doi: 10.1126/science.1195463
Tsuchida, T., Koga, R., Shibao, H., Matsumoto, T., and Fukatsu, T. (2002). Diversity and geographic distribution of secondary endosymbiotic bacteria in natural populations of the pea aphid, Acyrthosiphon pisum. Mol. Ecol. 11, 2123–2135. doi: 10.1046/j.1365-294x.2002.01606.x
Tsvetkov, N., Samson-Robert, O., Sood, K., Patel, H. S., Malena, D. A., Gajiwala, P. H., et al. (2017). Chronic exposure to neonicotinoids reduces honey bee health near corn crops. Science 356, 1395–1397. doi: 10.1126/science.aam7470
Vatanparast, M., and Kim, Y. (2017). Optimization of recombinant bacteria expressing dsRNA to enhance insecticidal activity against a lepidopteran insect Spodoptera exigua. PLoS One 12:e0183054. doi: 10.1371/journal.pone.0183054
Vogel, E., Santos, D., Mingels, L., Verdonckt, T. W., and Broeck, J. V. (2018). RNA interference in insects: protecting beneficials and controlling pests. Front. Physiol. 9:1912. doi: 10.3389/fphys.2018.01912
Vorburger, C. (2013). The evolutionary ecology of symbiont-conferred resistance to parasitoids in aphids. Insect Sci. 21, 251–264. doi: 10.1111/1744-7917.12067
Wagner, S. M., Martinez, A. J., Ruan, Y. M., Kim, K. L., Lenhart, P. A., Dehnel, A. C., et al. (2015). Facultative endosymbionts mediate dietary breadth in a polyphagous herbivore. Funct. Ecol. 29, 1402–1410. doi: 10.1111/1365-2435.12459
Wang, S., Dos-Santos, A. L. A., Huang, W., Liu, K. C., Oshaghi, M. A., Wei, G., et al. (2017). Driving mosquito refractoriness to plasmodium falciparum with engineered symbiotic bacteria. Science 357, 1399–1402. doi: 10.1126/science.aan5478
Wang, Z., Su, X. M., Wen, J., Jiang, L. Y., and Qiao, G. X. (2014). Widespread infection and diverse infection patterns of Wolbachia in Chinese aphids. Insect Sci. 21, 313–325. doi: 10.1111/1744-7917.12102
Wernegreen, J. J., and Moran, N. A. (2000). Decay of mutualistic potential in aphid endosymbionts through silencing of biosynthetic loci: Buchnera of Diuraphis. Proc. Biol. Sci. 267, 1423–1431. doi: 10.1098/rspb.2000.1159
Whitten, M., and Dyson, P. (2017). Gene silencing in non-model insects: overcoming hurdles using symbiotic bacteria for trauma-free sustainable delivery of RNA interference: sustained RNA interference in insects mediated by symbiotic bacteria: applications as a genetic tool and as a biocide. BioEssays 39:1600247. doi: 10.1002/bies.201600247
Whitten, M. M., Facey, P. D., Del Sol, R., Fernandez-Martinez, L. T., Evans, M. C., Mitchell, J. J., et al. (2016). Symbiont-mediated RNA interference in insects. Proc. Biol. Sci. 283:20160042. doi: 10.1098/rspb.2016.0042
Xu, T. T., Chen, J., Jiang, L. Y., and Qiao, G. X. (2021b). Diversity of bacteria associated with Hormaphidinae aphids (Hemiptera: Aphididae). Insect Sci. 28, 165–179. doi: 10.1111/1744-7917.12746
Xu, S., Chen, J., Qin, M., Jiang, L., and Qiao, G. (2021a). Geography-dependent symbiont communities in two oligophagous aphid species. FEMS Microbiol. Ecol. 97:fiab132. doi: 10.1093/femsec/fiab132
Yu, G. C., Smith, D. K., Zhu, H. C., Guan, Y., and Lam, T. T. Y. (2017). Ggtree: an R package for visualization and annotation of phylogenetic trees with their covari-ates and other associated data. Methods Ecol. Evol. 8, 28–36. doi: 10.1111/2041-210X.12628
Zhang, Y. L., Zhang, S. Z., Kulye, M., Wu, S. R., Yu, N. T., Wang, J. H., et al. (2012). Silencing of molt-regulating transcription factor gene, CiHR3, affects growth and development of sugarcane stem borer Chilo infuscatellus. J. Insect Sci. 12:91. doi: 10.1673/031.012.9101
Zhu, F., Xu, J., Palli, R., Ferguson, J., and Palli, S. R. (2011). Ingested RNA interference for managing the populations of the Colorado potato beetle Leptinotarsa decemlineata. Pest Manag. Sci. 67, 175–182. doi: 10.1002/ps.2048
Ziegler-Graff, V. (2020). Molecular insights into host and vector manipulation by plant viruses. Viruses 12:263. doi: 10.3390/v12030263
Zotti, M., Dos Santos, E. A., Cagliari, D., Christiaens, O., Taning, C. N. T., and Smagghe, G. (2018). RNA interference technology in crop protection against arthropod pests, pathogens and nematodes. Pest Manag. Sci. 74, 1239–1250. doi: 10.1002/ps.4813
Zytynska, S. E., Meyer, S. T., Sturm, S., Ullmann, W., Mehrparvar, M., and Weisser, W. W. (2015). Secondary bacterial symbiont community in aphids responds to plant diversity. Oecologia 180, 735–747. doi: 10.1007/s00442-015-3488-y
Keywords: insect bacteria, aphid symbionts, symbiont-mediated RNAi, Buchnera aphidicola, horizontal transmission
Citation: Li T, Wei Y, Zhao C, Li S, Gao S, Zhang Y, Wu Y and Lu C (2022) Facultative symbionts are potential agents of symbiont-mediated RNAi in aphids. Front. Microbiol. 13:1020461. doi: 10.3389/fmicb.2022.1020461
Edited by:
Daisuke Kageyama, National Agriculture and Food Research Organization (NARO), JapanReviewed by:
Rita Rebollo, l’alimentation et l’environnement (INRAE), FranceCopyright © 2022 Li, Wei, Zhao, Li, Gao, Zhang, Wu and Lu. This is an open-access article distributed under the terms of the Creative Commons Attribution License (CC BY). The use, distribution or reproduction in other forums is permitted, provided the original author(s) and the copyright owner(s) are credited and that the original publication in this journal is cited, in accordance with accepted academic practice. No use, distribution or reproduction is permitted which does not comply with these terms.
*Correspondence: Yongjun Wei, eW9uZ2p1bndlaUB6enUuZWR1LmNu; Chuantao Lu Y2h1YW50YW9sdUBxcS5jb20=