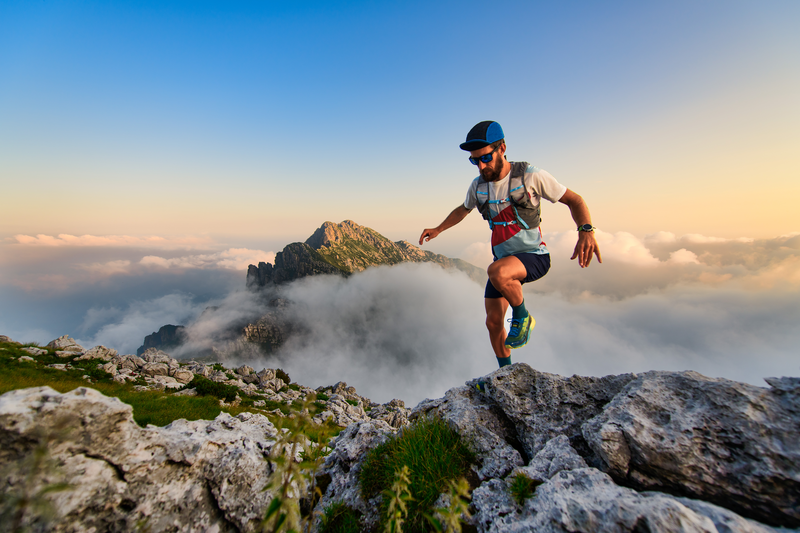
94% of researchers rate our articles as excellent or good
Learn more about the work of our research integrity team to safeguard the quality of each article we publish.
Find out more
ORIGINAL RESEARCH article
Front. Microbiol. , 10 October 2022
Sec. Food Microbiology
Volume 13 - 2022 | https://doi.org/10.3389/fmicb.2022.1014212
This article is part of the Research Topic Campylobacter in Poultry: Physiology, Genetics, and Detection Methods, Volume II View all 4 articles
Campylobacter jejuni and C. coli are one of the leading causes of gastrointestinal illnesses, and which are considered to be transmitted to humans mainly from chicken meats. Considering the less availability of quantitative contamination data in the retail chicken meats in Japan, 510 fresh chicken meats retailed at five distinct regions in Japan between June 2019 and March 2021 were examined. The quantitative testing resulted that 45.7% of the samples (254/510) were positive at mean ± standard deviation of 1.15 ± 1.03 logCFU/g, whereas 43 samples (8.4%) exceeded 3.0 logCFU/g. Seasonal comparison revealed increased bacterial counts in fall compared with spring and summer. As for the chicken slaughter age, those slaughtered at >75 days old were less contaminated than those at <75 days old. Genome sequencing analyses of 111 representative C. jejuni isolates resulted in the detection of three antimicrobial resistance genes (gyrA substitution T86I, tetO and blaOXA-61) at 25.2, 27.9 and 42.3%, respectively. In silico MLST analysis revealed the predominance of sequence types (ST)-21 clonal complex (CC), followed by ST-45CC and ST-464CC. The single nucleotide polymorphism (SNP)-based phylogenetic tree largely classified the sequenced C. jejuni isolates into two clusters (I and II), where all C. jejuni from highly contaminated samples (STs-21CC, -22CC and -45CC) belonged to cluster I, independent of both season and slaughter age. To our knowledge, this is the first example to study the current status of Campylobacter contamination levels in fresh chicken meats retailed in Japan. Our data would be contributable to future quantitative microbial risk assessment, to establish effective control measures for campylobacteriosis.
Campylobacter jejuni and C. coli are among the most reported foodborne pathogens to cause human gastrointestinal disease globally [Geissler et al., 2017; European Food Safety Authority (EFSA) and European Centre for Disease Prevention and Control (ECDC), 2021; Sher et al., 2021]. Human campylobacteriosis is usually linked to the consumption of poultry meat, particularly in fresh portioned and whole chicken meat products (Kaakoush et al., 2015; Batz et al., 2021), highlighting the necessity to control this pathogen along the poultry production chain. The focus on the risk of Campylobacter contamination from poultry meats is due to their high international consumption, estimated at 13.9 kg per capita in 2015–2017 and expected to increase up to 14.6 kg in 2027 [Food and Agriculture Organization (FAO) and Organization for Economic Co-operation and Development (OECD), 2018]. Similarly, the slaughtered weight ranges of poultry meats in Japan have been increasing, as previously reported, from 2,171,905 tonnes in 2016 to 2,331,650 tonnes in 2020 [Ministry of Agriculture, Forestry and Fisheries (MAFF), 2021]. To evaluate the risk associated with poultry consumption and its reduction measures, quantitative microbial risk assessment (QMRA) is currently employed as a structured approach that enables the estimation of the illness probability in a population; QMRA consists of the detection of the contamination levels of human/animal-related pathogens at any process, which may jeopardize human health (Chapman et al., 2016; Mikkelä et al., 2016).
In 2020, 20.5% (182/887) of the total cases of foodborne gastrointestinal illness in Japan were caused by campylobacteriosis [Ministry of Health, Labour and Welfare (MHLW), 2021]. Moreover, our previous study estimated that the source of nearly 80.3% of the detected campylobacteriosis was chicken meats (Kumagai et al., 2020). In June 2021, the MHLW in Japan adopted the national Food Hygiene Act [Ministry of Health, Labour and Welfare of Japan (MHLW), 2020], which enables all poultry slaughterhouses to apply HACCP principles, including microbiological monitoring system with validation and verification programmes [Ministry of Health, Labour and Welfare of Japan (MHLW), 2020]. However, there is a lack of a nationwide monitoring system for poultry meat products at the retail level in Japan.
European agency has suggested QMRA as a tool to reduce the impact of human campylobacteriosis emerging from broiler meat [EFSA Panel on Biological Hazards (BIOHAZ), 2010], in which the use of the QMRA model was suggested to estimate the impact of the presence of Campylobacter spp. in the broiler meat chain on human campylobacteriosis. Furthermore, the model used available quantitative data to rank/categorize the designated intervention approaches along the farm-to-fork continuum. In this context, some countries have already initiated the acquisition of a quantitative baseline data regarding Campylobacter contamination mainly at the poultry slaughter level (Bai et al., 2014; Lynch et al., 2022), while these data at the retail level were limited [Public Health England (PHE), 2019]. The Food Safety Commission of Japan (FSCJ), a national organization for risk assessment, recently revised the risk profiles of C. jejuni and C. coli in chicken meats and advised for the acquisition of such quantitative data through standardized microbiological methods [Food Safety Commission of Japan (FSCJ), 2021].
Given the background, this study aimed to quantitatively detect thermotolerant Campylobacter spp. from a total of 510 chicken thigh meat portions retailed in five distinct regions across Japan. Combined with their epidemiological history, possible factors influencing the levels of Campylobacter contamination were analyzed and discussed. Moreover, we used whole genome sequencing on the C. jejuni isolates.
Fresh-chilled chicken thigh meat products (total n = 510) were purchased from retail markets in five different regions (A–E; Supplementary Figure 1) across Japan between June 2019 and May 2021. The samples were transported at <10°C and subjected to microbiological examination within 3 h.
Considering the fact that greater dissemination of the thermophilic Campylobacter spp. relies on the skin rather than on the underlying muscle parts in retail chicken meat portions (Hansson et al., 2015), we selected skin parts as target for the bacterial testing. We quantitatively detected thermotolerant Campylobacter spp. from chicken meats essentially based on ISO 10272-2:2017 [International Organization for Standardization (ISO), 2017]. In brief, from each chicken meat product, a sample of 25 g of skin was cut using sterile scissors and tweezers, followed by morcellation and homogenisation for 1 min in 100 ml of buffered peptone water (BPW; Oxoid, Hampshire, United Kingdom). We spread aliquots of 1-mL BPW suspension with its serial dilutions on modified charcoal cefoperazone deoxycholate agar (mCCDA) plates (Oxoid), which were incubated at 42°C for 48 h under microaerophilic condition using the AnaeroPack-MicroAero system (Mitsubishi Gas Chemicals, Tokyo, Japan). We counted the number of colonies, where at least five typical or suspected colonies per plate were subjected to real-time PCR to confirm the presence of C. jejuni or C. coli, as previously described (Kawase et al., 2016). Finally, we used this number to calculate the total bacterial count in each sample. Because the theoretical limit of detection (LOD) of the aforementioned assay was estimated at 0.70 logCFU/g, we adopted 1/2 LOD (=0.35 logCFU/g) for Campylobacter-negative samples. After the test confirmation, the obtained C. jejuni isolates from the samples in regions B, C and E were microaerobically grown on Mueller–Hinton agar plates (Becton Dickinson, Franklin Lakes, NJ, United States) at 37°C for 20 h and stored in 15% glycerol in Tryptic Soy Broth (Becton Dickinson) at −80°C until further use. In this study, we did not use qualitative method for the detection and isolation of thermotolerant Campylobacter spp.
A total of 111 representatives of C. jejuni isolates from chicken meats at regions B, C and E were arbitrarily selected to include highly contaminated sample origin (>3.0 logCFU/g). The bacterial isolates were grown on Mueller–Hinton agar (Becton Dickinson) at 37°C for 20 h under microaerophilic conditions using the AnaeroPack-MicroAero system, followed by centrifugation at 4,000× g for 5 min. Accordingly, we extracted DNA from the bacterial pellets using the Maxwell Blood DNA Kit (Promega, Madison, WI, USA). We measured the concentration and quality of the extracted DNA on a TapeStation 4,150 system (Agilent Technologies, Santa Clara, CA, USA). The samples were stored at −80°C until further use.
To construct libraries of DNA, we used 1 μg of each of the bacterial extracts with the Ion Xpress Plus Fragment Library Kit (Thermo Fisher Scientific, Waltham, MA, United States). After adding barcode adaptors using the Ion Xpress Barcode Adaptors 1–16 Kit (Thermo Fisher Scientific), we purified the barcoded DNA using Agencourt AMPure XP (Beckman Coulter, Brea, CA, United States) and then combined 10 to 11 fragments. Next, we constructed a template DNA library on Ion Chef using the Ion 530 kit and 530 chip (Thermo Fisher Scientific), where we conducted sequencing by the Ion GeneStudio S5 sequencer (Thermo Fisher Scientific), according to the manufacturer’s protocols.
To remove barcode and low-quality sequences, we processed the generated fastq files using the CLC Genomic Workbench ver. 21 (Qiagen, Aarhus, Denmark). Noting that, low-quality sequences were defined with <100 bases, maximum ambiguities of 2 and homopolymers >6 bases. Then, we assembled the trimmed reads de novo to contigs under the default conditions (minimum contig length, 1,000 bases; mismatch cost, 2; insertion cost, 3; detection cost, 3; length fraction, 0.5; similarity fraction, 0.8; global alignment, yes). Accordingly, the assembled files were subjected to the DFAST programme1 (Tanizawa et al., 2018; accessed on July 1, 2022) for genomic annotation. Simultaneously, the total assemblies were also subjected to each of the following: MLST 2.0 programme2 (Larsen et al., 2012; accessed on June 27, 2022) to assign in silico sequence types (STs); ResFinder programme ver. 4.13 (Bortolaia et al., 2020; accessed on June 25, 2022) to detect acquired genes and/or chromosomal mutations mediating antimicrobial resistance; and CSI Phylogeny4 (Kaas et al., 2014; accessed on June 21, 2022) to generate the SNP-based phylogenomic tree under the following conditions: minimum depth at SNP positions, 10; relative depth at SNP positions, 10; minimum distance between SNPs (prune), 10; minimum SNP quality, 30; minimum read mapping quality, 25; and minimum Z-score, 1.96.
The sequenced C. jejuni isolates (n = 111) were used to examine their susceptibility against three antibiotics, namely, ciprofloxacin (CPFX), tetracycline (TC) and ampicillin (ABPC) via disc diffusion test using Sensi-Disc (Becton Dickinson) according to the guidelines of the Clinical and Laboratory Standard Institute (CLSI, 2015).
The statistical differences of the bacterial counts between different groups arranged by the slaughter age of chicken (<75 days and > 75 days) and the season (spring, summer, and fall; because of less sample numbers, the bacterial count data in winter was removed from the seasonal comparison) were calculated using the Mann–Whitney U test and the prevalence ratios of the AMR-related genes between the two groups (slaughter age at >75 days or < 75 days) were comparatively analysed using Pearson’s chi-squared test; the level of significance was set to p < 0.05.
Overall, Campylobacter spp. were detected in 254 samples (49.8%), in which 27 (5.3%), 120 (23.5%), 64 (12.5%) and 43 (8.4%) samples were contaminated with Campylobacter spp., respectively, at the following ranges: 0.70–0.99 logCFU/g, 1.00–1.99 logCFU/g, 2.00–2.99 logCFU/g and >3.00 logCFU/g, respectively (Table 1). In total, the mean ± standard deviation (SD) and the maximum reach of Campylobacter counts were 1.15 ± 1.03 logCFU/g and 4.27 logCFU/g, respectively (Table 1).
Table 1. Quantitative detection of Campylobacter spp. from retail chicken meats at 5 distinct regions in Japan between June 2019 and May 2021.
Seasonal comparison indicated the highest bacterial counts in the samples taken in fall (October to December, means of 4.27 logCFU/g) compared with those collected in spring (April–June, means of 3.88 logCFU/g) and summer (July–September, means of 3.78 logCFU/g), respectively (Table 1; Supplementary Figure 2A).
The comparison by slaughter age of chicken revealed that 56.7% (237/418) of meat samples from young broilers (slaughtered at <75 days old) were Campylobacter-positive with a mean ± SD of 1.29 ± 1.08 logCFU/g, which was significantly higher than the remaining 92 samples from older-aged chicken (slaughtered at >75 days old), which showed Campylobacter positivity at 18.5% (17/92) with a mean ± SD at 0.52 ± 0.41 logCFU/g (p < 0.01; Table 1; Supplementary Figure 2B).
Region comparison was excluded from the quantitative analysis because the sample numbers were not normalised between the five regions, as presented in Table 1.
Overall, we could show the current status of Campylobacter contamination levels in the chicken meat products retailed in Japan, in association with the slaughter age and season.
Throughout the quantitative detection of Campylobacter spp., a total of 218 C. jejuni and 26 C. coli were finally isolated from 52.4% (244/466) of the samples in regions B, C and E (Table 1). We excluded C. coli for further characterization because its numbers were relatively scarce compared with C. jejuni. Among C. jejuni, 111 representative isolates (68 from chicken slaughtered at <75 days old and 43 at >75 days old) were arbitrarily selected. Thereby, a WGS was designed using the Ion GeneStudio S5 sequencing platform. The sequence read numbers ranged from 1,352,730 to 2,774,038 with an average read length ranging from 205 to 279 bases, in which de novo assembled genome statistics were at 23–92 contigs. The annotation of these assemblies using the DFAST programme identified 1,493–2,215 coding sequences (CDSs), 31–43 rRNAs, 1–2 tRNAs and coverage ratio of 87.1–93.5%, respectively (Supplementary Table 1).
In silico MLST analysis classified the 111 C. jejuni isolates into 63 STs, where 45 STs were categorized in 12 clonal complexes (CC) and 9 of the remaining 18 STs were novel STs. Among the CCs, ST-21CC was the most predominant (24.3%, 27/111), followed by ST-354CC (13.5%, 15/111) and then ST-45CC (9.9%, 11/111). ST-22CC, ST-52CC and ST-607CC were detected only in the chicken meats slaughtered at <75 days old, whereas ST-353CC was detected only in the chicken meats slaughtered at >75 days old. Within ST-21CC that generally showed a broad range of host adaptation (Woodcock et al., 2017), ST-50 (n = 9), ST-21 (n = 2) and ST-9535 (n = 2) commonly originated from chicken meats slaughtered at <75 days old, whereas ST-11191 (n = 5), ST-4526 (n = 3) and ST-9776 (n = 2) were detected only in older slaughtered chicken meats (>75 days old). A total of 14 C. jejuni isolates from highly contaminated broiler chicken meat samples (>3.0 logCFU/g) were classified into ST-21CC (ST-50, n = 9), ST-22CC (ST-22, n = 2) and ST-45CC (ST-137, n = 1; ST-1326, n = 1; ST-3456, n = 1; Table 2).
Table 2. MLST and antimicrobial-resistance profiles of 111 representative C. jejuni isolates from chicken meat samples retailed in Japan.
ResFinder predicted that 42.3% (47/111) of C. jejuni isolates harboured blaOXA-61 gene, 27.9% (31/111), tetO gene; and 25.2% (28/111), gyrA mutated at T86I (Table 2). The antimicrobial susceptibility testing resulted in the complete agreement between the presence of three antimicrobial resistance (AMR)-related genes and resistance phenotype against the three antimicrobials among the sequenced isolates (Table 2). 24 isolates (18 isolates were from meats slaughtered at <75 days old and 6 from those at >75 days old) showed resistance to more than two antimicrobials, in which 14 isolates were resistant to both TC and CPFX (Table 2). The prevalence of three AMR-related genes did not exhibit statistical association with the slaughtered age of chicken (Table 2).
Combined with the MLST profiles, gyrA (T86I) was detected in 22/83 (26.5%) isolates belonging to 7 CCs [(ST-21CC (6/27), ST-22CC (2/3), ST-45CC (4/11), ST-52CC (2/2), ST-354CC (5/15), ST-443CC (1/6) and ST-464CC (2/10)] and 6/28 (21.4%) isolates unassigned (UA) to any CCs with no significant difference between CCs (p = 0.32; Table 2).
Alternately, tetO was detected in 15/83 (18.1%) isolates belonging to 3 CCs [ST-21 CC (7/27), ST-45 CC (7/11) and ST-354CC (1/15)], and 16/28 (57.1%) of UA isolates with significant difference between CCs (p < 0.01; Table 2).
Similarly, blaOXA-61 was detected in 37/83 (44.6%) isolates belonging to 8 CCs [ST-21CC (17/27), ST-22CC (3/3), ST-45CC (4/11), ST-48 CC (2/2), ST-206CC (1/1), ST-353CC (2/3), ST-443CC (6/6) and ST-464CC (2/10)], and 10/28 (35.7%) of UA isolates with significant differences between CCs (p < 0.01; Table 2).
The multidrug-resistant isolates were shown in 24/111 (21.6%) isolates, 13 of which were belonged to ST-21CC (8/27) and ST-45CC (5/11), although there were no significant differences between CCs (p = 0.33; Table 2).
In summary, we detected the MLST and AMR profiles of 111 representative C. jejuni from chicken meats retailed in Japan, with partial links to slaughter age of chicken and contamination levels.
To examine the possible association of the bacterial genomic traits with the slaughtered age of chicken and bacterial contamination levels, a single nucleotide polymorphism (SNP)-based phylogenetic tree was constructed, which was broadly divided into two clusters (I and II); the first consisted of 42 young broiler-originating isolates (red) and 28 older isolates (green), whereas cluster II consisted of 26 young (red) and 15 older ones (green; Figure 1), with no apparent statistical phylogenic bias based on the chicken slaughter age.
Figure 1. The SNPs-based phylogenetic tree of 111 C. jejuni isolates from fresh chicken meats retailed at regions B, C and E in Japan, with MLST (ST and CC) and AMR profiles (possession (+/−) of three AMR-related genes). Each isolate is highlighted in red (slaughter age of <75 days) or green (slaughter age of >75 days). C. jejuni isolates of highly contaminated samples (>3.0 logCFU/g) are underlined. CCs in which >3 isolates included are highlighted with different colours. The prevalence of AMR-related gene is shown as “+” in dark blue-highlight.
Within the MLST profiles, seven CCs (i.e., ST-21CC, -22CC and -45CC) belonged to cluster I and six (i.e., ST-354CC, ST-443CC and ST-464CC) to cluster II (Figure 1). Notably, 14 isolates that originated from samples exhibiting >3.0 logCFU/g of contamination were all distributed in cluster I and none in cluster II (isolates underlined in Figure 1).
Thus, these data indicated that the C. jejuni genotypes in cluster I were partly associated with highly contaminated chicken meat samples.
Campylobacter in poultry meat is ranked as the leading pathogen–food combination to cause health risks; therefore, it negatively impacts the national economy (Kaakoush et al., 2015; Batz et al., 2021). The ratio of highly contaminated samples (>3.0 logCFU/g) in our data was estimated at 8.4%, which was seemingly almost similar or less, compared with some recent reports from other countries; for example, Asuming-Bediako et al. reported that 12.7% (7/55) of the chicken meats retailed in Ghana were >3.0 logCFU/g (Asuming-Bediako et al., 2022). Moreover, 18.7% (59/315) of chicken meats retailed in Australia were contaminated with >2.0 logCFU/g (Habib et al., 2019).
In Japan, most of the chicken meats are retailed as portion fillets, not as whole carcass, which suggested that there might be differences due to processing. Therefore, a nationwide baseline surveillance at both slaughter and retail stages could better estimate the stage-to-stage kinetics of the bacterial contamination levels, thereby providing the QMRA data. Nevertheless, to the best of our knowledge, this is the first study to demonstrate the quantitative distribution of thermophilic Campylobacter spp. in chicken meat products retailed across Japan.
To date, it is likely that human campylobacteriosis occurred more frequently in hot seasons in summer and/or fall compared with cold seasons, as proven in Denmark (Nielsen et al., 2013), France (Bessède et al., 2014), United States (Williams et al., 2015; Sher et al., 2021), Switzerland (Baumgartner and Felleisen, 2011). Likewise, in Japan, the greater proportions of campylobacteriosis were reported in summer and fall based on the foodborne disease statistics report [Ministry of Health, Labor and Welfare of Japan (MHLW), 2021]. Our data indicated greater bacterial counts in chicken meats retailed in fall than spring and summer, which seemed to be in agreement with the foodborne statistics shown above. Several factors considerably affect the seasonal variation of bacterial counts, one of which seemed to be linked to the geography-associated atmospheric variations. For example, the mean temperature which is identified as one of the major environmental drivers for the campylobacteriosis occurrence (Cousins et al., 2020), was 22.5°C in region A and 28.4°C in region B, on August 2019, according to the Japanese meteorological agency.5 Continued surveillance at different regions with atmospheric information might improve our understanding behind the effects of temperature and/or geographical differences in the quantitative distribution of Campylobacter spp. in meat chickens on the farm. Although this study did not include human isolates, future continued monitoring and characterization of Campylobacter spp. from chicken meats together with human isolates would further unveil the seasonal dynamics of Campylobacter.
In this relation, we previously demonstrated that long-term grow-out in laying hens affects the C. jejuni colonization fitness, which is associated with altered gut microbiota and lipid compositions (Asakura et al., 2021). Considering the fact that C. jejuni cooperated and competed with diverse commensal microbiota, thereby becoming part of the gut microbial community (Han et al., 2017; Sakaridis et al., 2018), our data associated with the slaughter age also indicated that long-term feeding of chicken might reduce the colonising numbers of Campylobacter in the chicken gut.
The increasing availability of WGS datasets has significantly enhanced the analyses of bacterial population structure and diversity (Sheppard and Maiden, 2015). Several studies have attempted to trace the source of infection by identifying the genomic elements (SNPs and genes) segregated by the host (Weis et al., 2016; Thépault et al., 2018; Berthenet et al., 2019). Moreover, the WGS analysis allows the detection and characterisation of antimicrobial resistance of Campylobacter isolates (Whitehouse et al., 2018; Meistere et al., 2019).
Among the STs associated with high level of contamination in poultry meats, it is noteworthy that multiple ST-50 isolates, in particular, were identified, although chicken were slaughtered and processed in different facilities at different time points. This genotype is known as one of the major host generalists (Woodcock et al., 2017) and linked to human infection (Ramonaite et al., 2017). However, we could not conclude the association between the specific clonal complexes and contamination levels in the chicken meats, because the parts of ST-45CC and ST-22CC showed association with high level contamination. In addition, it was uncertain why C. jejuni isolates belonged to ST-21CC were phylogenetically split into two subclusters within cluster I. Considering the strain-to-strain variations of virulence property even in ST-21CC, which were assessed by multiple infection models (Humphrey et al., 2015), these strain’s virulence should be further assessed using these infection models in our future study.
Contrarily, several STs classified into cluster II originated from samples with less bacterial counts. An in vitro cell invasion property-based dose–response modelling was recently proposed (Abe et al., 2021), and it would be useful to use this approach to investigate possible differences in the virulence-associated characteristics clusters I and II. In addition, ST-4526, which previously thrived in Japan in human and chicken (Asakura et al., 2012), was isolated from meats of chicken slaughtered >75 days age. Considering that, this genotype could exhibit an increased colonisation fitness in the chicken’s gut (Asakura et al., 2013), the reason for which it was isolated from chicken meats being fed for long periods.
A recent study demonstrated that compared with infected patients with C. jejuni exhibiting TC resistance, those with CPFX resistance were more likely to be hospitalised during fall or summer seasons in the United States (Rodrigues et al., 2021). Our data demonstrating the widespread of genes conferring resistance to TC (tetO), β-lactam (blaOXA-61) and fluoroquinolone (gyrA T86I), were shown particularly among the isolates belonging to ST-21CC and ST-45CC, which are among the host generalist genotypes, thus transmissive between chicken and humans (Sheppard et al., 2014; Mourkas et al., 2020). These data suggest the necessity to monitor these CCs for antimicrobial resistance in both chicken and humans. Indeed, there were significant differences of the prevalence of tetO and blaOXA-61 genes between CCs. Moreover, a recent study demonstrated the increased conjugative efficiency of tetO-carrying plasmids between Campylobacter strains at 42°C (resembling poultry reservoir) compared with 37°C (Cuevas-Ferrando et al., 2020), which further highlighted the needs to regulate the invasion of these CCs into poultry farm in order to decrease the chance of spreading TC-resistance. The stable kinetics of fluoroquinolone and TC resistance were observed in C. jejuni from farmed animals and humans in the United States and United Kingdom (van Vliet et al., 2022) and the association of antimicrobial resistance of C. jejuni in broilers with their use at farm was recently investigated (Tenhagen et al., 2021), which showed a positive association between use of tetracycline and erythromycin and subsequent resistance in Campylobacter but no such link was found for aminoglycosides. In relation to the latter, for example 77.1% of the total amounts of oral enrofloxacin distributed in Japan (1,829.4 kg) was used in broilers until 7 days before slaughter in 2019 [National Veterinary Assay Laboratory (NVAL), 2019]. Consequently, a spatiotemporal comparative monitoring of the antimicrobial resistance of this pathogen to commonly used antibiotics in farmed animals, foods and humans is essential, where the approach of reducing antimicrobial use in farms would be advised from a healthcare viewpoint.
In this research study, we validated the current status of the Campylobacter contamination levels in retail chicken meats in Japan. Our data proposed the necessity to further reduce the contamination levels similar to European countries, which are attempting to reduce the highly contaminated chicken meats at retail (>3.0 logCFU/g) to zero (European Commission, 2017). Combining the different parameters affecting the occurrence and contamination levels of this pathogen (age of the slaughtered chicken meats and seasonal and bacterial phylogenetic variations) as well as the MLST-based source attribution study (Cody et al., 2019) might help QMRA to develop adequate control measures to reduce bacterial contamination in chicken meats, thereby leading to the reduction of the incidence of human campylobacteriosis through a continuous baseline surveillance with quantitative testing and bacterial genome characterization through the food chain.
The datasets presented in this study can be found in online repositories. The names of the repository/repositories and accession number(s) can be found in the article/Supplementary material.
HA conceptualized, designed, supervised the experiments, and wrote the original draft. SY, KY, JK, HN, KA, YS, TI, and RN conducted experiments and data analysis. HA, KY, JK, and HN conducted a writing review. All authors contributed to the article and approved the submitted version.
This research was supported in part by a grant from Ministry of Health, Labour and Welfare of Japan (grant number 20KA1007) and a grant from the Food Safety Commission of Japan (grant number JPCAFSC20222202).
We acknowledge the valuable contribution of Rika Machida and Keiko Arita for their technical support in the quantitative bacterial testing and culture preparation for the WGS analysis.
The authors declare that the research was conducted in the absence of any commercial or financial relationships that could be construed as a potential conflict of interest.
All claims expressed in this article are solely those of the authors and do not necessarily represent those of their affiliated organizations, or those of the publisher, the editors and the reviewers. Any product that may be evaluated in this article, or claim that may be made by its manufacturer, is not guaranteed or endorsed by the publisher.
The Supplementary material for this article can be found online at: https://www.frontiersin.org/articles/10.3389/fmicb.2022.1014212/full#supplementary-material
1. ^https://dfast.ddbj.nig.ac.jp/
2. ^https://cge.cbs.dtu.dk/services/MLST/
3. ^https://cge.cbs.dtu.dk/services/ResFinder/
Abe, H., Koyama, K., and Koseki, S. (2021). Modelling invasion of Campylobacter jejuni into human small intestinal epithelial-like cells by Bayesian inference. Appl. Environ. Microbiol. 87, e01551–e01520. doi: 10.1128/AEM.01551-20
Asakura, H., Brüggemann, H., Sheppard, S. K., Ekawa, T., Meyer, T. F., Yamamoto, S., et al. (2012). Molecular evidence for the thriving of Campylobacter jejuni ST-4526 in Japan. PLoS One 7:e48394. doi: 10.1371/journal.pone.0048394
Asakura, H., Nakayama, T., Yamamoto, S., Izawa, K., Kawase, J., Torii, Y., et al. (2021). Long-term grow-out affects Campylobacter jejuni colonization fitness in coincidence with altered microbiota and lipid composition in the cecum of laying hens. Front. Vet. Sci. 8:675570. doi: 10.3389/fvets.2021.675570
Asakura, H., Taguchi, M., Ekawa, T., Yamamoto, S., and Igimi, S. (2013). Continued widespread dissemination and increased poultry host fitness of Campylobacter jejuni ST-4526 and ST-4253 in Japan. J. Appl. Microbiol. 114, 1529–1538. doi: 10.1111/jam.12147
Asuming-Bediako, N., Kunadu, A. P., Jordan, D., Abraham, S., and Habib, I. (2022). Prevalence and antimicrobial susceptibility pattern of Campylobacter jejuni in raw retail chicken meat in metropolitan Accra, Ghana. Int. J. Food Microbiol. 376:109760. doi: 10.1016/j.ijfoodmicro.2022.109760
Bai, Y., Cui, S., Xu, X., and Li, F. (2014). Enumeration and characterization of Campylobacter species from retail chicken carcasses in Beijing, China. Foodborne Pathog. Dis. 11, 861–867. doi: 10.1089/fpd.2014.1785
Batz, M. B., Richardson, L. C., Bazaco, M. C., Parker, C. C., Chirtel, S. J., Cole, D., et al. (2021). Recency-weighted statistical modelling approach to attribute illnesses caused by 4 pathogens to food sources using outbreak data, United States. Emerg. Infect. Dis. 27, 214–222. doi: 10.3201/eid2701.203832
Baumgartner, A., and Felleisen, R. (2011). Market surveillance for contamination with thermotolerant Campylobacters on various categories of chicken meat in Switzerland. J. Food Prot. 74, 2048–2054. doi: 10.4315/0362-028X.JFP-11-228
Berthenet, E., Thépault, A., Chemaly, M., Rivoal, K., Ducournau, A., Buissonnière, A., et al. (2019). Source attribution of Campylobacter jejuni shows variable importance of chicken and ruminants reservoirs in non-invasive and invasive French clinical isolates. Sci. Rep. 9:8098. doi: 10.1038/s41598-019-44454-2
Bessède, E., Lehours, P., Labadi, L., Bakiri, S., and Mégraud, F. (2014). Comparison of characteristics of patients infected by Campylobacter jejuni, Campylobacter coli, and Campylobacter fetus. J. Clin. Microbiol. 52, 328–330. doi: 10.1128/JCM.03029-13
Bortolaia, V., Kaas, R. S., Ruppe, E., Roberts, M. C., Schwarz, S., Cattoir, V., et al. (2020). ResFinder 4.0 for predictions of phenotypes from genotypes. J. Antimicrob. Chemother. 75, 3491–3500. doi: 10.1093/jac/dkaa345
Chapman, B., Otten, A., Fazil, A., Ernst, N., and Smith, B. A. (2016). A review of quantitative microbial risk assessment and consumer process models for Campylobacter in broiler chickens. Microb. Risk Anal. 2-3, 3–15. doi: 10.1016/j.mran.2016.07.001
Clinical and Laboratory Standards Institute (CLSI). (2015) Methods for antimicrobial dilution and disk susceptibility testing of infrequently isolated or fastidious bacteria; approved guideline (3rd Edn). M45.
Cody, A. J., Maiden, M. C., Strachan, N. J., and McCarthy, N. D. (2019). A systematic review of source attribution of human campylobacteriosis using multilocus sequence typing. Euro Surveill. 24:1800696. doi: 10.2807/1560-7917.ES.2019.24.43.1800696
Cousins, M., Sargeant, J. M., Fisman, D. N., and Greer, A. L. (2020). Identifying the environmental drivers of Campylobacter infection risk in southern Ontario, Canada using a one health approachs. Zoonoses Public Health 67, 516–524. doi: 10.1111/zph.12715
Cuevas-Ferrando, E., Guirado, P., Miró, E., Iglesias-Torrens, Y., Navarro, F., Alioto, T. S., et al. (2020). Tetracycline resistance transmission in Campylobacter is promoted at temperatures resembling the avian reservoir. Vet. Microbiol. 244:108652. doi: 10.1016/j.vetmic.2020.108652
EFSA Panel on Biological Hazards (BIOHAZ) (2010). Scientific opinion on quantification of the risk posed by broiler meat to human campylobacteriosis in the EU. EFSA J. 8:1437. doi: 10.2903/j.efsa.2010.1437
European Commission. (2017) Commission Regulation (EU) 2017/1495 of amending regulation (EC) no. 2073/2005 as regards Campylobacter in broiler carcasses. OJ L 218 24. 8, 1–6. Available at https://www.fsai.ie/uploadedFiles/Reg2017_1495.pdf (Accessed July 25, 2022).
European Food Safety Authority (EFSA) and European Centre for Disease Prevention and Control (ECDC) (2021). The European Union one health 2019 zoonoses report. EFSA J. 19:e06406. doi: 10.2903/j.efsa.2021.6406
Food and Agriculture Organization (FAO) and Organization for Economic Co-operation and Development (OECD). (2018). Chapter 6. Meat. Available at: https://www.fao.org/publications/oecd-fao-agricultural-outlook/2018-2027/en/
Food Safety Commission of Japan (FSCJ). (2021) Revision of the risk profile of Campylobacter jejuni/coli in poultry meats. Available at: http://www.fsc.go.jp/risk_profile/index.data/210622CampylobacterRiskprofile.pdf
Geissler, A. L., Bustos Carrillo, F., Swanson, K., Patrick, M. E., Fullerton, K. E., Bennett, C., et al. (2017). Increasing Campylobacter infections, outbreaks, and antimicrobial resistance in the United States, 2004–2012. Clin. Infect. Dis. 65, 1624–1631. doi: 10.1093/cid/cix624
Habib, I., Coles, J., Fallows, M., and Goodchild, S. (2019). A baseline quantitative survey of Campylobacter spp. on retail chicken portions and carcasses in metropolitan Perth, Western Australia. Foodborne Pathog. Dis. 16, 180–186. doi: 10.1089/fpd.2018.2554
Han, Z., Willer, T., Li, L., Pielsticker, C., Rychlik, I., Velge, P., et al. (2017). Influence of the gut microbiota composition on Campylobacter jejuni colonization in chickens. Infect. Immun. 85, e00380–e00317. doi: 10.1128/IAI.00380-17
Hansson, I., Nyman, A., Lahti, E., Gustafsson, P., and Olsson Engvall, E. (2015). Associations between Campylobacter levels on chicken skin, underlying muscle, caecum and packaged fillets. Food Microbiol. 48, 178–181. doi: 10.1016/j.fm.2014.12.013
Humphrey, S., Lacharme-Lora, L., Chaloner, G., Gibbs, K., Humphrey, T., Williams, N., et al. (2015). Heterogeneity in the infection biology of Campylobacter jejuni isolates in three infection models reveals an invasive and virulent phenotype in a ST21 isolate from poultry. PLoS One 10:e0141182. doi: 10.1371/journal.pone.0141182
International Organization for Standardization (ISO). (2017) ISO 10272-2:2017. Microbiology of the Food Chain—Horizontal method for detection and enumeration of Campylobacter spp. — Part 2: Colony-count technique.
Kaakoush, N. O., Castaño-Rodríguez, N., Mitchell, H. M., and Man, S. M. (2015). Global epidemiology of Campylobacter infection. Clin. Microbiol. Rev. 28, 687–720. doi: 10.1128/CMR.00006-15
Kaas, R. S., Leekitcharoenphon, P., Aarestrup, F. M., and Lund, O. (2014). Solving the problem of comparing whole bacterial genomes across different sequencing platforms. PLoS One 9:e104984. doi: 10.1371/journal.pone.0104984
Kawase, J., Etoh, Y., Ikeda, T., Yamaguchi, K., Watahiki, M., Shima, T., et al. (2016). An improved multiplex real-time SYBR Green PCR assay for analysis of 24 target genes from 16 bacterial species in fecal DNA samples from patients with foodborne illnesses. Jpn. J. Infect. Dis. 69, 191–201. doi: 10.7883/yoken.JJID.2015.027
Kumagai, Y., Pires, S. M., Kubota, K., and Asakura, H. (2020). Attributing human foodborne diseases to food sources and water in Japan using analysis of outbreak surveillance data. J. Food Prot. 83, 2087–2094. doi: 10.4315/JFP-20-151
Larsen, M. V., Cosentino, S., Rasmussen, S., Friis, C., Hasman, H., Marvig, R. L., et al. (2012). Multilocus sequence typing of total-genome-sequenced bacteria. J. Clin. Microbiol. 50, 1355–1361. doi: 10.1128/JCM.06094-11
Lynch, H., Franklin-Hayes, P., Koolman, L., Egan, J., Gutierrez, M., Byrne, W., et al. (2022). Prevalence and levels of Campylobacter in broiler chicken batches and carcasses in Ireland in 2017–2018. Int. J. Food Microbiol. 372:109693. doi: 10.1016/j.ijfoodmicro.2022.109693
Meistere, I., Ķibilds, J., Eglīte, L., Alksne, L., Avsejenko, J., Cibrovska, A., et al. (2019). Campylobacter species prevalence, characterisation of antimicrobial resistance and analysis of whole-genome sequence of isolates from livestock and humans, Latvia, 2008 to 2016. Euro Surveill. 24:1800357. doi: 10.2807/1560-7917.ES.2019.24.31.1800357
Mikkelä, A., Ranta, J., González, M., Hakkinen, M., and Tuominen, P. (2016). Campylobacter QMRA: a bayesian estimation of prevalence and concentration in retail foods under clustering and heavy censoring. Risk Anal. 36, 2065–2080. doi: 10.1111/risa.12572
Ministry of Agriculture, Forestry and Fisheries (MAFF). (2021) Statistic report on logistics of livestock products in Japan. Available at: https://www.maff.go.jp/j/tokei/kouhyou/tikusan_ryutu/ (Accessed July 28, 2022).
Ministry of Health, Labour and Welfare of Japan (MHLW). (2021) Foodborne disease statistics report. Available at: https://www.mhlw.go.jp/stf/seisakunitsuite/bunya/kenkou_iryou/shokuhin/syokuchu/04.html (Accessed August 2, 2022).
Ministry of Health, Labour and Welfare of Japan (MHLW). (2020) Information of the current status of slaughterhouses in Japan. Available at: https://www.mhlw.go.jp/content/11130500/000689979.pdf (Accessed July 25, 2022).
Mourkas, E., Taylor, A. J., Méric, G., Bayliss, S. C., Pascoe, B., Mageiros, L., et al. (2020). Agricultural intensification and the evolution of host specialism in the enteric pathogen Campylobacter jejuni. Proc. Natl. Acad. Sci. U. S. A. 117, 11018–11028. doi: 10.1073/pnas.1917168117
National Veterinary Assay Laboratory (NVAL). (2019) Sales amounts and sales volumes (active substance) of antibiotics, synthetic antibacterials, anthelmintics and antiprotozoals. Available at: https://www.maff.go.jp/nval/iyakutou/hanbaidaka/pdf/R1_hanbaidaka.pdf (Accessed July 12, 2022).
Nielsen, H. L., Ejlertsen, T., Engberg, J., and Nielsen, H. (2013). High incidence of Campylobacter concisus in gastroenteritis in North Jutland, Denmark: a population-based study. Clin. Microbiol. Infect. 19, 445–450. doi: 10.1111/j.1469-0691.2012.03852.x
Public Health England (PHE). (2019) A microbiological survey of Campylobacter contamination in fresh whole UK-produced chilled chickens at retail sale. Available at: https://www.food.gov.uk/sites/default/files/media/document/campylobacter-contamination-uk-chickens-year-4-report.pdf (Accessed August 5, 2022).
Ramonaite, S., Tamuleviciene, E., Alter, T., Kasnauskyte, N., and Malakauskas, M. (2017). MLST genotypes of Campylobacter jejuni isolated from broiler products, dairy cattle and human campylobacteriosis cases in Lithuania. BMC Infect. Dis. 17:430. doi: 10.1186/s12879-017-2535-1
Rodrigues, J. A., Cha, W., Mosci, R. E., Mukherjee, S., Newton, D. W., Lephart, P., et al. (2021). Epidemiologic associations vary between tetracycline and fluoroquinolone resistant Campylobacter jejuni infections. Front. Public Health 9:672473. doi: 10.3389/fpubh.2021.672473
Sakaridis, I., Ellis, R. J., Cawthraw, S. A., van Vliet, A. H. M., Stekel, D. J., Penell, J., et al. (2018). Investigating the association between the caecal microbiomes of broilers and Campylobacter burden. Front. Microbiol. 9:927. doi: 10.3389/fmicb.2018.00927
Sheppard, S. K., Cheng, L., Méric, G., de Haan, C. P., Llarena, A. K., Marttinen, P., et al. (2014). Cryptic ecology among host generalist Campylobacter jejuni in domestic animals. Mol. Ecol. 23, 2442–2451. doi: 10.1111/mec.12742
Sheppard, S. K., and Maiden, M. C. (2015). The evolution of Campylobacter jejuni and Campylobacter coli. Cold Spring Harb. Perspect. Biol. 7:a018119. doi: 10.1101/cshperspect.a018119
Sher, A. A., Ashraf, M. A., Mustafa, B. E., and Raza, M. M. (2021). Epidemiological trends of foodborne Campylobacter outbreaks in the United States of America, 1998–2016. Food Microbiol. 97:103751. doi: 10.1016/j.fm.2021.103751
Tanizawa, Y., Fujisawa, T., and Nakamura, Y. (2018). DFAST: a flexible prokaryotic genome annotation pipeline for faster genome publication. Bioinformatics 34, 1037–1039. doi: 10.1093/bioinformatics/btx713
Tenhagen, B. A., Flor, M., Alt, K., Knüver, M. T., Buhler, C., Käsbohrer, A., et al. (2021). Association of antimicrobial resistance in Campylobacter spp. in broilers and turkeys with antimicrobial use. Antibiotics 10:673. doi: 10.3390/antibiotics10060673
Thépault, A., Rose, V., Quesne, S., Poezevara, T., Béven, V., Hirchaud, E., et al. (2018). Ruminant and chicken: important sources of campylobacteriosis in France despite a variation of source attribution in 2009 and 2015. Sci. Rep. 8:9305. doi: 10.1038/s41598-018-27558-z
van Vliet, A. H. M., Thakur, S., Prada, J. M., Mehat, J. W., and La Ragione, R. M. (2022). Genomic screening of antimicrobial resistance markers in UK and US Campylobacter isolates highlights stability of resistance over an 18-year period. Antimicrob. Agents Chemother. 66:e0168721. doi: 10.1128/aac.01687-21
Weis, A. M., Storey, D. B., Taff, C. C., Townsend, A. K., Huang, B. C., Kong, N. T., et al. (2016). Genomic comparison of Campylobacter spp. and their potential for zoonotic transmission between birds, primates, and livestock. Appl. Environ. Microbiol. 82, 7165–7175. doi: 10.1128/AEM.01746-16
Whitehouse, C. A., Young, S., Li, C., Hsu, C. H., Martin, G., and Zhao, S. (2018). Use of whole-genome sequencing for Campylobacter surveillance from NARMS retail poultry in the United States in 2015. Food Microbiol. 73, 122–128. doi: 10.1016/j.fm.2018.01.018
Williams, M. S., Golden, N. J., Ebel, E. D., Crarey, E. T., and Tate, H. P. (2015). Temporal patterns of Campylobacter contamination on chicken and their relationship to campylobacteriosis cases in the United States. Int. J. Food Microbiol. 208, 114–121. doi: 10.1016/j.ijfoodmicro.2015.05.018
Keywords: retailed poultry meat, contamination level, antimicrobial resistance, whole-genome sequencing, Campylobacter jejuni/coli
Citation: Asakura H, Yamamoto S, Yamada K, Kawase J, Nakamura H, Abe K-i, Sasaki Y, Ikeda T and Nomoto R (2022) Quantitative detection and genetic characterization of thermotolerant Campylobacter spp. in fresh chicken meats at retail in Japan. Front. Microbiol. 13:1014212. doi: 10.3389/fmicb.2022.1014212
Received: 08 August 2022; Accepted: 23 September 2022;
Published: 10 October 2022.
Edited by:
Michael Konkel, Washington State University, United StatesReviewed by:
Matthew Jon Sylte, Animal and Plant Health Inspection Service (USDA), United StatesCopyright © 2022 Asakura, Yamamoto, Yamada, Kawase, Nakamura, Abe, Sasaki, Ikeda and Nomoto. This is an open-access article distributed under the terms of the Creative Commons Attribution License (CC BY). The use, distribution or reproduction in other forums is permitted, provided the original author(s) and the copyright owner(s) are credited and that the original publication in this journal is cited, in accordance with accepted academic practice. No use, distribution or reproduction is permitted which does not comply with these terms.
*Correspondence: Hiroshi Asakura, aGFzYWt1cmFAbmlocy5nby5qcA==
Disclaimer: All claims expressed in this article are solely those of the authors and do not necessarily represent those of their affiliated organizations, or those of the publisher, the editors and the reviewers. Any product that may be evaluated in this article or claim that may be made by its manufacturer is not guaranteed or endorsed by the publisher.
Research integrity at Frontiers
Learn more about the work of our research integrity team to safeguard the quality of each article we publish.