- 1Antimicrobial Resistance and Phage Biocontrol Research Group, Department of Microbiology, School of Biological Sciences, Faculty of Natural and Agricultural Sciences, North-West University, Mmabatho, South Africa
- 2Department of Chemistry, Faculty of Natural and Agricultural Sciences, North-West University, Mmabatho, South Africa
- 3Indigenous Knowledge Systems Center, Faculty of Natural and Agricultural Sciences, North-West University, Mmabatho, South Africa
Diarrhea is a condition in which feces is discharged from the bowels frequently and in a liquid form. It is one of the frequent causes of morbidity and mortality in developing countries. The impact of Diarrhea is worsened by the increasing incidence of antimicrobial resistance among the causative agents, and this is now categorized as a global healthcare challenge. Antimicrobial resistance among Diarrheal pathogens also contributes to extended infection durations, and huge economic loss even in countries with advanced public health policies. The ever-increasing incidence of antimicrobial resistance including the contraindications arising from the administration of antibiotics in some Diarrheal cases highlights a crucial need for the development of novel non-antibiotic alternative agents for therapeutic and biocontrol applications. One such intervention includes the application of plant-derived nanoparticles (PDNPs) with novel antimicrobial properties. Given their small size and large surface area to volume ratio, PDNPs can attack target bacterial cell walls to generate reactive oxygen species that may simultaneously disrupt bacteria cell components such as DNA and proteins leading to cell damage or death. This potential can make it very difficult for pathogenic organisms to develop resistance against these antibacterial agents. In this review, we provide a critical overview on the antimicrobial resistance crisis among Diarrheagenic bacteria. We also discuss the evidence from the existing literature to support the potential associated with the use of PDNPs as alternative therapeutic agents for multidrug resistant and antibiotics administer contraindicated bacteria that are associated with Diarrhea.
Introduction
Diarrhea is among the most frequent cause of morbidities and mortalities in humans even in countries with advanced healthcare facilities. The global increase in antimicrobial resistance among Diarrheal pathogens is a great public health concern requiring urgent attention (Troeger et al., 2018). In 2019, the Global Burden of Disease (GDB) indicated that Diarrhea was the third and fifth leading cause of disability adjusted life years (DALYs) in children below 5 years old and all ages, respectively (Vos et al., 2020; Behera and Mishra, 2022). In South Africa, data from the National Burden of Disease (NBD) indicate that Diarrhea remains among the top 10 diseases associated with high mortalities especially among children (Nannan et al., 2019). The most frequent causative agents of Diarrhea are bacteria (Feuerstein et al., 2022), viruses (rotavirus, norovirus, astrovirus, and adenovirus), and protozoa (Giardia, Entamoeba histolytica, and Cryptosporidium species; Behera and Mishra, 2022). The transmission of Diarrheal pathogens is often through the consumption of contaminated food or water (Jung et al., 2020). The clinical signs and symptoms of Diarrhea include severe abdominal pain, vomiting, watery stool, bloody stool, and fever (Wei et al., 2020).
In some patients, Diarrhea may be self-limiting and can be treated by antibiotics to avoid life-threatening complications (Taylor et al., 2017). However, the administration of antibiotics is contraindicated for Diarrhea caused by some bacteria strains including those belonging to the E. coli serotypes O157 (Yoh et al., 1997; Wong et al., 2000; Mullish and Williams, 2018; Endtz, 2020a; Hwang et al., 2021). This limits the use of antibiotics in treating Diarrhea. In addition, there is a rapid emergence of multiple drug resistance (MDR), excessive drug resistance (XDR), and pan drug resistance (PDR) among Diarrheal pathogens (Huchin et al., 2017; Nkansa-Gyamfi et al., 2019; Ammar et al., 2021). This resistance is mainly attributed to the misuse of antimicrobial agents among humans and animals (Aminshahidi et al., 2017). The rapid increase of antimicrobial resistance among Diarrheal causative organisms is a global healthcare concern accountable for an increase in morbidity and mortality rates, extended duration of infections in susceptible individuals, and huge financial loss (IHME, 2020).
The significant increase in antimicrobial resistance coupled with the contraindications associated with the administration of antibiotics especially in Diarrheal cases highlights a crucial need for the development of novel strategies aimed at not only combating infections but also controlling the development and spread of antibiotic resistance. This review focuses on antimicrobial resistance among Diarrheagenic bacteria and highlights the public health crises it presents to both human and animal medicine. The potential antibacterial properties of PDNPs as alternative therapeutic agents for multidrug resistant bacteria and with specific focus on Diarrheal pathogens are also highlighted.
The causative agents of Diarrhea
Generally, viruses, bacteria, and protozoa are the frequent causative agents of Diarrhea among humans and animals (Figure 1). Other factors include food allergies and intolerances, long-term use of medications, and digestive disorders, such as ulcerative colitis, microscopic colitis, and small intestinal bacterial overgrowth (Troeger et al., 2018; Zhou et al., 2021). However, Diarrhea caused by bacterial pathogens is known to cause high mortality. In addition, the focus of this review on bacteria pathogens as is mainly due to the rapid increase in the development of antimicrobial resistance among bacterial species thus limiting treatment options.
Bacteria
Bacteria remain the leading cause of Diarrhea and significantly contribute to approximately 31% of all Diarrheal pathogen’s cases (IHME, 2020). Particularly, Escherichia coli, Vibrio cholerae, Shigella, Salmonella, Campylobacter, and Clostridium species (Table 1) are most frequently associated with cases of Diarrhea (Kim et al., 2015). In 2019, different bacteria pathogens were associated with global morbidities and mortalities resulting from Diarrheal complications (IHME, 2020; Murray et al., 2020).
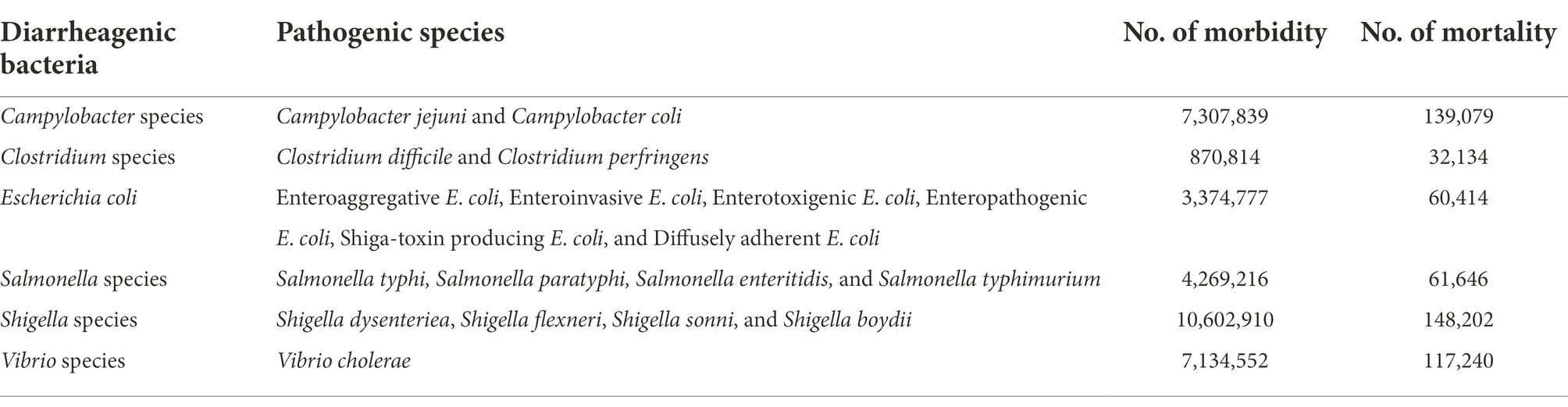
Table 1. Global illness and death due to Diarrheagenic bacteria complications in 2019 (IHME, 2020; Murray et al., 2020).
Escherichia coli
Escherichia coli forms part of the normal intestinal microbiota of humans (Gomes et al., 2016; Vidal et al., 2019). However, some E. coli strains have acquired virulence genes that allow them to cause moderate to severe diseases depending on host-pathogen interactions (Gomes et al., 2016). To date, several pathogenic E. coli strains are known to be significant causative agents of Diarrhea (Gomes et al., 2016; Vidal et al., 2019) and are collectively termed Diarrheagenic E. coli (DEC; Table 1). These DEC are grouped into different pathological types (pathotypes) based on differences in the virulence gene they harbor, namely diffusely adherent E. coli (DAEC), enterotoxigenic E. coli (ETEC), enteropathogenic E. coli (EPEC), enteroinvasive E. coli (EIEC), enteroaggregative E. coli (EAEC), and enterohemorrhagic E. coli (EHEC; Zhou et al., 2018). Enteropathogenic E. coli remains one of the most frequent causes of Diarrhea in children under the age of 5 in developing countries (Hu et al., 2015). The ability of EPEC to cause Diarrhea is associated with the potential to produce attaching and effacing lesions (Hu et al., 2015). The possession of aggregative adherence (aag) gene determinants by EAEC, invasive plasmid antigen H (ipaH) gene by EIEC and heat-stable (st) and heat-labile (lt) genes by ETEC account for the pathogenicity of these strains as well as their ability to cause Diarrhea (Mare et al., 2021). Enterohemorrhagic E. coli or STEC can cause acute inflammation of the intestine that results to bloody Diarrhea. This group of pathogenic E. coli are capable of producing Shiga toxins with two principal types, stx1 and stx2 currently known and characterized (Zhang J. et al., 2020). In addition, other putative variants of stx2 such stx2a, stx2c, and stx2d have been reported and their association with disease in human have been fully documented (Baranzoni et al., 2016).
Shigella species
Shigellosis is a common cause of Diarrhea, accounting for about 148,202 deaths globally (Vos et al., 2020). Shigella species are the second leading cause of mortality among children in low and middle-income countries, accounting for approximately 60,000 deaths per year (Rogawski McQuade et al., 2020). Shigella species have low infectious dose and thus they are recognized as pathogens of severe public health concern when compared to other enteric pathogens. Malnutrition and poor health care facilities exacerbate the impact of infections caused by Shigella species especially in developing countries (Sheikh et al., 2019). The transmission of these pathogens usually occur through the consumption of contaminated food and/or water (Abebe et al., 2020). Shigella species that are frequently associated with Diarrhea include S. boydii, S. dysenteriae, S. sonnei, and S. flexneri (Aminshahidi et al., 2017; Abbasi et al., 2019). The pathogenicity of Shigella species is also associated with their ability to produce two distinct toxins, namely, Shigella enterotoxin 1 and Shigella enterotoxin 2, which are structurally and genetically similar to the Shiga toxins produced by STECs (Yaghoubi et al., 2017). The enterotoxin 2 produced by S. dysenteriae facilitates invasion of epithelial cells of the small intestines, while the enterotoxin 1 accounts for the watery phase of Diarrhea (Yaghoubi et al., 2017).
Campylobacter species
Globally, Campylobacter species are some of the most common pathogens that cause foodborne diseases including Diarrhea (Li Y. et al., 2018). The most frequently isolated Campylobacter species from Diarrhea patients are C. jejuni and C. coli, accounting for about 500 million gastrointestinal illnesses every year (Zhang P. et al., 2020). Infections caused by Campylobacter species are generally mild but can be severe in younger children, the elderly, or immunocompromised individuals thus requiring urgent attention to avoid complications, such as bacteremia, irritable bowel syndrome, hepatitis, and pancreatitis (Same and Tamma, 2018). The complications, duration, and high incidence of Diarrhea caused by Campylobacter species make it socio-economically significant problem requiring urgent attention (Endtz, 2020b).
Salmonella species
In 2019, Diarrhea resulting from Salmonella species was estimated to be 4.2 million cases with 61,646 deaths (Vos et al., 2020). Particularly in Europe, the most common Salmonella species that causes Diarrhea is S. enteritidis, where it accounts for eight out of every 10 cases of Diarrhea caused by Salmonella species (Wei et al., 2019; Yue et al., 2020). Other commonly isolated salmonellae include S. typhimurium, S. Paratyphi, and S. Typhi (Terefe et al., 2020). The clinical symptoms that are most common in Diarrheal patients infected by Salmonella species include fever, vomiting, nausea, abdominal pains, and in some cases bloody stool (Wei et al., 2020). The most vulnerable group comprises pregnant women, children below 5 years, and elderly people as well as immunocompromised individuals (Demb et al., 2014).
Vibrio species
Vibrio species remain an important and a prevalent causative agent of Diarrheal cases that contributes to morbidity in humans of all ages globally (Ganesan et al., 2020). Vibrio cholerae is the species that is most often isolated from patients with Diarrhea and it has been responsible for a number of outbreaks worldwide making it a pathogen of significant public health concern (Bashar and Soundappan, 2022). Its socio-economic impact indicates the need for highly effect control strategies that aim to contribute to efforts made by different organizations such as WASH to curb the problem worldwide (Igere and Ekundayo, 2020). Vibrio cholerae causes an acute Diarrheal illness by invading the intestine of humans and it accounts for about 2,900,000 cases with 95,000 deaths annually (Ganesan et al., 2020). Diarrhea caused by V. cholerae is usually mild without symptoms but may progress to life-threatening complications if left untreated. With a relatively short incubation period of between 24 and 48 h before the onset of disease, cholera presents approximately a 25–50% mortality rate in humans, thus the need to prevent cross-contamination and the occurrence of disease (Igere and Ekundayo, 2020). However, cholera is often predictable and preventable. The disease can ultimately be eliminated especially during outbreaks by ensuring access to clean water and sanitation facilities, as well as good hygiene practices provided the entire population.
Diarrhea therapy and antibiotics resistance crisis
Although Diarrheal illness may be self-limiting and can be manage by oral rehydration, antimicrobial treatment is recommended for seriously-ill patients and immunocompromised individuals to avoid complications (Taylor et al., 2017). Unfortunately, a large proportion of individuals suffering from Diarrhea rarely report cases to hospitals but rather resorts to self-medication (Ferdous, 2018). This practice has been highly discouraged especially if the causative agent is E. coli strain belongs to the STEC serotype O157:H7 (Hwang et al., 2021; Ramstad et al., 2021; Tarr and Freedman, 2022). Antibiotic therapy is contraindicated in the treatment and management of Diarrhea infections caused by STEC because there is evidence that antibiotics may increase the risk of developing more life threatening complications, such as haemolytic uremic syndrome (HUS), haemorrhagic colitis, and thrombotic thrombocytopenic purpura (TTP) that have all been associated with renal failure (Hwang et al., 2021; Ramstad et al., 2021; Tarr and Freedman, 2022). This supports the view to ensure that appropriate tests are conducted to correctly determine the identities of causative agents before antibiotic therapy. In addition, the global misuse of antibiotics among individuals has led to a significant increase of antibiotic-resistant pathogens against most of the available potent antibiotics rendering them ineffective (Aminshahidi et al., 2017). Also, some of these pathogens are resistant to carbapenem antibiotics, which are considered to be a “last resort” drug for the management of infections caused by multiple antimicrobial resistant pathogens (Livermore et al., 2020). Furthermore, some of the Diarrheagenic bacteria have displayed multiple drug resistance (MDR) while others have acquired extensive drug resistance (XDR) determinants (Table 2). The Diarrhea cause by antibiotic-resistant pathogens can lead to severe illnesses, extended hospital stays, increase in healthcare costs, socio-economic burden as well as treatment failures (Rahim et al., 2021). The death rate in humans resulting from complications caused by resistant Diarrheagenic bacteria has increased globally (Vos et al., 2020; Mac Kinnon et al., 2021). In 2019, the global death caused by notable Diarrheagenic bacteria such as E. coli, V. cholerae, Shigella, Salmonella, Campylobacter, and Clostridium species were 60,414, 117,240, 148,202, 61,646, 139,079, and 32,134, respectively (IHME, 2020). This rapid increase in antimicrobial resistance couple with the contraindications associated with the administration of antibiotics in Diarrheal cases highlight a crucial need for the development of novel and alternative therapeutic antibacterial agents. This will contribute to the global action plan on antimicrobial resistance developed by WHO requiring each country to establish its own antimicrobial resistance strategic framework to curb the spread of antimicrobial resistance (Mendelson and Matsoso, 2015). Against this background, several intervention strategies have been proposed to mitigate the spread of antibiotic resistance. One such intervention includes the application of medicinal plant derivatives nanoparticles. The application of nanoparticles as alternatives for drug resistant bacteria contributes to the aim of reducing the incidence of infections and an investment in countering antimicrobial resistance.
Alternative therapeutic approaches and strategies for multidrug resistant Diarrheal pathogens
The significant and rapid increase in antimicrobial resistance among bacteria pathogens including those associated with Diarrhea pose a serious concern, especially to public health globally. This advocates for the need to develop new alternative therapeutic approaches to curb the spread of antimicrobial resistance (Harbarth et al., 2015). Recently, a number of antibacterial agents and strategies have been used to control pathogenic antibiotic-resistant bacteria (Aldayel, 2019). These attempts utilize either chemical (agents and compounds) or natural (microbes and plant extracts) methods. To date, there is evidence of the advantages that natural agents from microbes and plants possess over chemical agents, and this explains why natural agents are considered as novel alternative therapeutic approaches for combating antimicrobial resistance even among Diarrheal pathogens (Hobernik and Bros, 2018; Aldayel, 2019; Kalsoom et al., 2020). In fight against antimicrobial resistance, vaccine, bacteriophages, and nanoparticles provide some hope (Aldayel, 2019). However, more research is still needed to assess the safety and suitability of these agents. Moreover, the development of sustainable and highly effective antimicrobial agents will not only contribute to the global action plan on antimicrobial resistance, but also provide opportunities to generate data on the mechanisms of action of these natural agents.
Nanotechnology and nanoparticles as a novel approach in combating antibiotics resistant Diarrheal pathogens
Nanoparticles (NPs) are small particles that range between 1 and 100 nm in size and possess a large surface area to volume ratio. The large surface to volume ratio enhances their physicochemical properties compared to macro range particles. The small size to the large surface area to volume ratio of NPs influences their binding and reactive abilities, making them suitable antimicrobial and sensory agents. These attributes facilitate their use in biolabeling, filters, microelectronics, and catalysis (Kaabipour and Hemmati SJBJoN., 2021). Generally, NPs are produced using chemical, physical, and biological methods (Figure 2). Physical synthetic methods for NPs involve thermal decomposition, laser irradiation, and electrolysis that usually require very high temperatures, are energy-intensive and also require high-cost vacuum systems with other supporting equipments (Khan et al., 2022). Chemical methods typically involve the use of chemicals, which may be harmful to humans under certain conditions (Karimi-Maleh et al., 2021). Due to these limitations, recent studies typically focus on biological synthetic methods due to their non-toxicity, cheap, and environmentally friendly nature (Zhang et al., 2016; Nemidkanam and Chaichanawongsaroj, 2022). Plants are a major source of different types of phytochemicals with numerous biomedical applications and the extracts are used to treat various diseases. Different parts of plants including leaves, fruits, seeds, stems, flowers, roots, barks, and fruit peels have been used in the synthesis of various types of nanoparticles (Venkat Kumar and Rajeshkumar, 2018). Plant-based NP green synthesis is now regarded as a gold standard approach among the green biological techniques owing to its ease of use and the diversity of plants (Hano and Abbasi, 2022). The most popular PDNPs synthesis techniques involves the reduction of metals, such as copper, gold, silver, nickel, platinum, zinc, and titanium with the plant extracts serving as reducing, capping, and stabilizing agents (Ahmad et al., 2020). Findings from several studies have reported high efficacy of various metal-based PDNPs against a variety of multidrug resistant pathogenic bacteria (Banerjee et al., 2022). However, the development of various side effects including argyrosis, argyria, liver damage, kidney obstruction, and eye irritation, in patients limits the usage of metal-based PDNPs for oral treatment of drug resistant bacteria in human medicine (Korani et al., 2015). Metal-free NPs can be produced from plant extracts by using the differential ultracentrifugation technique (Iravani and Varma, 2020; Kim et al., 2021). The production of metal-free PDNPs has recently gained significant attention because plants contain materials such as terpenoids, alkaloids, polyphenols, phenolics, sugars, and proteins as metabolites that can be broken down to produce nanoparticles with significant antimicrobial properties. Metal-free PDNPs are environmental friendly, toxic chemical free, cheap, and energy serving (Kharissova et al., 2019; Surendran et al., 2021). The potential to derive metal-free NPs with medicinal properties from plants has gained more attention worldwide (Zhang et al., 2016; Saravanan et al., 2021; Surendran et al., 2021; Liu et al., 2022; Nemidkanam and Chaichanawongsaroj, 2022). Liu et al. (2022) reported the therapeutic ability of metal-free PDNPs on gut microbiota while Xiao et al. (2018) highlighted the therapeutic efficacy of metal-free NPs synthesized from 11 edible fruits and vegetables. Saravanan et al. (2021) reported the antibacterial efficacy of metal-free NPs derived from Curcuma longa plant against E. coli, S. aurous, K. pneumoniae, and S. epidermidis. These attributes highlight and amplify the usefulness and effectiveness of metal-free PDNPs.
Metal-free plant-derived nanoparticles as alternative therapy for the treatment of Diarrhea
The production of PDNPs involves the use of plant extracts for synthesizing these unique small-size particles that also have large surface area to volume ratios. Nanoparticles have the potential to react with bacterial cell walls and generate reactive oxygen species (ROS) that can simultaneously disrupt important bacterial cell components such as DNA and proteins, thus leading to cell death (Saravanan et al., 2021). Metal-free PDNPs have been reported to be safe for oral therapy in humans because they are derived from the plants whose components, such as carbohydrates, proteins, and lipids are also vital for survival (Xiao et al., 2018; Großkopf and Simm, 2020; Saravanan et al., 2021). The presence of these compounds makes it easy for metal-free PDNPs to be metabolized and cleared from the intestine either through absorption or defecation (Li H. et al., 2018; Großkopf and Simm, 2020). Several researchers have reported the antimicrobial efficacy of metal-free NPs synthesized from different plants against various pathogenic bacteria (Table 3). A study on NPs synthesized from Curcuma longa extracts confirmed its significant antimicrobial efficacy against multidrug-resistant bacteria (Saravanan et al., 2021). Metal-free NPs synthesized from Lawsonia inermis extracts had high antimicrobial activities against antimicrobial-resistant E. coli and Salmonella species, which are the most frequently isolated Diarrheal pathogens (Shahshahanipour et al., 2019). Similar reports on the antimicrobial efficacy of PDNPs synthesized from extracts of Citrus medica against MDR and biofilm-producing bacteria have also been documented (Selvaraju et al., 2022). The compounds in plants when present in the PDNPs, contribute in the treatment of diseases (Ajose et al., 2022), thus a valuable source for alternative non-antibiotic therapeutic agents. These attributes provide evidence of the therapeutic and biocontrol properties of PDNPs against multidrug resistant bacteria, including those associated with Diarrhea.
Factors influencing the antimicrobial activity of nanoparticles
Antimicrobial activities of metal-free NPs can be influenced by various factors which include, size, elements used in the production, shape of nanoparticle, charge of NPs (Sun et al., 2021), and target microorganisms (Figure 3).
Size of nanoparticles
The small size and large surface-to-volume ratio of smaller metal-free PDNPs enhance their physical and chemical properties when compared with larger NPs (Saravanan et al., 2021). The smaller the size, the larger the surface-area-to-volume ratio; hence, the greater the antimicrobial activity. The high antimicrobial activities of smaller PDNPs results to larger surface area to volume ratios thus improving contact with the cell wall of microorganisms (Saravanan et al., 2021). Contact with cell wall and the production of ROS results in the destruction of essential cell biomolecules, such as DNA, proteins, and lipids leading to cell death. These attributes give smaller NPs an edge over their larger counterparts in inhibiting the growth of microorganisms (Sun et al., 2021). Shahshahanipour et al. (2019) reported that small size (5 nm) of NPs derived from Lawsonia inermis plant caused damage of E. coli and S. aureus cell wall.
Shape of nanoparticles
Different shapes for PDNPs have been reported by several researchers (Saravanan et al., 2021; Sun et al., 2021). These include spherical, sheets, plates, tubes, cubes, rods, and triangles, but the most common shape is spherical. Analysis has revealed that NPs that has more exposed surface is less stable and require less energy to form oxygen vacancies, thereby has higher antimicrobial activity. Several researchers have demonstrated that NPs with cube or rod shape are more effective than other shapes, due to their exposed surface and oxidation level.
Charge of nanoparticles
The charge of NPs usually plays important role in antimicrobial activity. Studies had revealed that positively charge metal-free PDNPs, can disrupt electron transport chain function (Sun et al., 2021). Electron transport chain contains ubiquinone, which is important for aerobic respiration. Analysis on E. coli with single deletion had confirmed that mutated bacteria on ubiquinone biosynthesis related genes were more susceptible to the positively charge NPs. The exposure of the bacteria to NPs results to ROS generation that inhibits bacteria growth (Saravanan et al., 2021). In addition, positively charge NPs is more attracted electrostatically to negative charge bacterial cell wall, making it to be more antimicrobial effective than negatively charge metal-free PDNPs. Notwithstanding, some negative charge PDNPs have been reported to have more antibacterial efficacy (Manzano and Vallet-Regí, 2020).
Target organisms
Generally, bacteria are classified into Gram-positive and Gram-negative base on their cell wall structure. Peptidoglycan is the most important component of bacterial cell wall, which serves as protection against external force, and it is thicker in Gram-positive bacteria than Gram-negative (Rohde, 2019). Nanoparticles have been revealed to have higher antimicrobial activities against Gram-negative bacteria than Gram-positive (Sun et al., 2021). It had been confirmed that thickness in peptidoglycan that makeup cell wall is the reason this phenomenon exists. The cell wall of Gram-positive bacteria, such as Staphylococcus aureus composed of peptidoglycan which is 80 nm thick (Pasquina-Lemonche et al., 2020). This thickness may delay the damage of Gram-positive cell wall by PDNPs compare with cell wall of Gram-negative bacteria such as E. coli that possess peptidoglycan which is 8 nm thick (Pasquina-Lemonche et al., 2020). In addition, Gram-negative bacteria cell wall is coated with negatively charged lipopolysaccharide which has higher affinity for positive charge ion discharge by most of the metal-free PDNPs (Sun et al., 2021). This higher affinity for positive charged ion by Gram-negative bacteria cell wall results into increase in ion uptake and build-up that damage the cell wall of Gram-negative bacteria faster than Gram-positive bacteria due to absent of lipopolysaccharide (Sun et al., 2021). Wang et al. (2020) reported that NPs derived from Artemisia argyi have antimicrobial activities against only Gram-negative bacteria (E. coli and P. aeruginosa) but not Gram-positive bacteria (S. aureus and Bacillus subtilis). However, some PDNPs that have higher antimicrobial activities against Gram-positive bacteria than Gram-negative had been reported (Shahshahanipour et al., 2019). Liu et al. (2021) confirmed that positive PDNPs have more antimicrobial properties against Gram-positive bacteria (S. aureus and B. subtilis) than Gram-negative bacteria (E. coli and Salmonella).
Antibacterial activity of plant-derived nanoparticles
Owing to their small size, the effectiveness of PDNPs as an antimicrobial agent has resulted in an increasing number of studies exploring the efficacy of their antimicrobial mechanisms. The small size and large surface area to volume ratio gives PDNPs the potential to damage bacteria cell wall (Shahshahanipour et al., 2019; Wang et al., 2020) and generates ROS (Saravanan et al., 2021), which disrupt DNA, protein, and cell membrane functions (Figure 4). The ability of PDNPs to alter the metabolic activity of the bacteria signifies an enormous advantage in eradicating them (de Barros et al., 2021). The antimicrobial effectiveness of PDNPs is accomplished when they contact microbial cells via a receptor ligand, Van der Waals forces, hydrophobic interactions, or electrostatic attraction (de Barros et al., 2021). The accumulation of PDNPs across the microbial cell and its metabolic pathways influences its structure and function. It uses ROS mechanisms to interfere with the bacteria’s cell components, such as DNA, protein, and cell membrane. This interaction may lead to cell death due to increased osmotic pressure (de Barros et al., 2021; Saravanan et al., 2021). PDNPs exhibit various modes of action on target bacterial cells (Table 4), including a disruption of cell membrane function, protein inhibition, DNA synthesis, and damage to the cell wall.
Disruption of bacteria cell wall
The first and most important defense mechanism that microorganisms typically use as a mode of resistance to antimicrobial agents is their cell walls and membranes. The cell wall plays a vital role in keeping the cell viable by protecting its organelles. The cell wall comprises lipopolysaccharides (LPSs), lipoproteins, peptidoglycan, and phospholipids. The wall forms a defensive barrier and thus prevents harmful substances from the external environment from entering (Auer and Weibel, 2017). However, microorganisms have a charge on the surface of their cell walls, which can attract PDNPs when applied as an antimicrobial agent (Shahshahanipour et al., 2019; Saravanan et al., 2021). The charged PDNPs generally bind with the charged functional cell wall groups, such as carboxyl and phosphate groups, in a process called biosorption, resulting in cell walls being structurally weakened and lyses, leading to the microorganism’s cell death (Figure 5). Existing evident indicate that PDNPs have better efficacy against Gram-negative bacteria relative to Gram-positive bacteria (Wang et al., 2020). Ma et al. (2020) reported that the accumulation of Osmanthus fragrans (PDNPs) on bacterial cell wall led to the cell wall destruction.
The DNA and protein damage
The interaction of PDNPs with microbial cells may lead to cell death due to DNA denaturation, synthesis inhibition, active cell enzyme inhibition, protein synthesis blockage, and gene expression alteration through the generation of ROS (Surendran et al., 2021). Saravanan et al. (2021) confirmed that NPs derived from Curcuma longa used ROS as main antimicrobial mode of action against Escherichia coli, Klebsiella pneumonia, Staphylococcus aureus, and Staphylococcus epidermidis. When NPs react with bacterial cell wall, ROS are slowly released and absorbed through the cell membrane (Shahshahanipour et al., 2019). ROS-induced oxidative stress is an important antimicrobial mechanism of NPs that has powerful positive redox potential (Shahshahanipour et al., 2019; Saravanan et al., 2021). Different types of NPs produce different types of ROS by reducing oxygen molecules. There are four types of ROS namely superoxide radical, hydroxyl radical (OH), singlet oxygen (O2), and hydrogen peroxide (H2O2), each with different levels of antimicrobial activity. The main causes of ROS production are restructuring, defect sites, and oxygen vacancy in the crystal, which occur due to the interaction of PDNPs with microbial cells, causing an unbalanced state (Figure 6). This unbalanced state causes oxidative stress, damaging or obstructing the microorganism’s organelles’ functions. Oxidative stress has been identified as a major contributor to alteration in the permeability of the microorganism’s cell membrane, leading to cell membrane malfunction and damage. The NPs interaction with the cell membrane ultimately results in the loss of membrane integrity because of intracellular oxidative stress. Furthermore, an increasing number of studies have shown that ROS plays a key role in the disruption of bacteria DNA and protein, which is a key mechanism in microbial cell death (Shahshahanipour et al., 2019; Saravanan et al., 2021). Saravanan et al. (Saravanan et al., 2021), reported that the ROS produce by metal-free PDNPs (Curcuma longa) played a significant role in eradicating the target bacteria by disrupting their DNA and protein.
Effect of plant-derived nanoparticles on host microbiota
The usage of PDNP as an antimicrobial agent is gaining momentum among researchers, and its numerous benefits in various fields, including medicine, have been established (Akintelu and Folorunso, 2020; Manzano and Vallet-Regí, 2020). Although a lot of in vitro work has been done by researchers to prove the efficacy of PDNP as an antimicrobial agent, its application as an alternative therapy in the field of medicine has not been well established for safety purposes (Su and Kang, 2020). The assessment of PDNP is essential for a proper therapeutic result and effective clinical use to avoid complications or the potential for negative interactions with host microbiota (Vakili-Ghartavol et al., 2020). Microbiota interacts intimately with their hosts, influencing immune responses, food absorption, and energy metabolism (Hacquard et al., 2015; Brugman et al., 2018). Healthy hosts with beneficial microbiota harbor diverse mutualistic and commensal microbes. The ability of PDNP to disrupt host-associated microbiota raises serious concerns regarding its application as an alternative medicine (Missaoui et al., 2018). As a result, pharmacokinetic and toxicological investigations on the influence of PDNP on the host microbiota are required before its use. Understanding the link between the physicochemical and structural features of PDNPs and host microbiota reactivity and interaction is very important (Missaoui et al., 2018; Brinkmann et al., 2020). This information will aid in the intelligent design of PDNPs while closing the knowledge gap about their host microbiota toxicity (Missaoui et al., 2018).
Conclusion and future perspectives
This study provides a detailed overview on the antimicrobial resistance crisis among Diarrheal pathogens. Globally, there is a rapid emergence of a multidrug, extensive drug, and pan-drug resistance among Diarrheal pathogens. The calls to develop new, active, environmentally friendly alternative therapies remain pertinent. Research indicates that PDNAs lie at the frontal of possible alternative treatments. A thorough overview of recent developments in the derivation of NPs from various plant parts has been summarized. The merits of PDNPs over physical and chemical techniques of producing NPs, such as being free of harmful chemicals, devoid of metals, environmentally benign, affordable, and energy-serving, were also highlighted. This review also discussed the antibacterial mode of action of PDNPs. The PDNPs sizeable surface-area-to-volume ratio and highly charged surfaces enable them to interact with bacteria cell wall and generate ROS that simultaneously disrupt the bacterial cell membrane, proteins, and DNA. This concurrent antimicrobial action of PDNPs can make it difficult for bacteria to develop resistance. This evidence demonstrates that PDNPs could be developed and used as alternative therapeutic agents against multidrug resistant bacteria, including those associated with Diarrhea.
Despite the numerous benefits of PDNPs in biomedicine, the knowledge about their safety to human health remains limited. Up to date, there is little information about the cytotoxicity of PDNPs. In addition, several in vitro assessments of antibacterial efficacy of PDNPs have been done with little or no in vivo information. Thus, more efforts are needed for stringent investigation on the cytotoxicity and in vivo application of PDNPs to confirm their safety for therapeutic use.
Author contributions
CA, AA, and OF: conceptualization, resources, supervision, and editing. TA, DA, BO, JF, KM, and CA: writing–review and editing. TA: writing–original draft preparation. All authors contributed to the article and approved the submitted version.
Funding
We are grateful to the North-West University and the Department of Microbiology, School of Biological Sciences for the financial support.
Acknowledgments
The authors wish to appreciate the Department of Microbiology, School of Biological Sciences, North-West University, Mafikeng, South Africa.
Conflict of interest
The authors declare that the research was conducted in the absence of any commercial or financial relationships that could be construed as a potential conflict of interest.
Publisher’s note
All claims expressed in this article are solely those of the authors and do not necessarily represent those of their affiliated organizations, or those of the publisher, the editors and the reviewers. Any product that may be evaluated in this article, or claim that may be made by its manufacturer, is not guaranteed or endorsed by the publisher.
References
Abbasi, E., Abtahi, H., van Belkum, A., and Ghaznavi-Rad, E. J. I. (2019). Multidrug-resistant Shigella infection in pediatric patients with diarrhea from Central Iran. Infect. Drug Resist. 12:1535. doi: 10.2147/IDR.S203654
Abebe, E., Gugsa, G., and Ahmed, M. (2020). Review on major food-borne zoonotic bacterial pathogens. J. Trop. Med. 2020:4674235. doi: 10.1155/2020/4674235
Ahmad, S., Tauseef, I., Haleem, K. S., Khan, K., Shahzad, M., Ali, M., et al. (2020). Synthesis of silver nanoparticles using leaves of Catharanthus roseus and their antimicrobial activity. Appl. Nanosci. 10, 4459–4464. doi: 10.1007/s13204-019-01221-z
Ajose, D. J., Oluwarinde, B. O., Abolarinwa TO, Fri, J., Montso, K. P., Fayemi, O. E., et al. (2022). Combating bovine mastitis in the dairy sector in an era of antimicrobial resistance: ethno-veterinary medicinal option as a viable alternative approach. Front. Vet. Sci. 9:800322. doi: 10.3389/fvets.2022.800322
Akintelu, S. A., and Folorunso, A. S. (2020). A review on green synthesis of zinc oxide nanoparticles using plant extracts and its biomedical applications. Bio Nano Sci. 10, 848–863. doi: 10.1007/s12668-020-00774-6
Aldayel, M. (2019). Biocontrol strategies of antibiotic-resistant, highly pathogenic bacteria and fungi with potential bioterrorism risks: bacteriophage in focus. J. King Saud Univ. Sci. 31, 1227–1234. doi: 10.1016/j.jksus.2019.08.002
Aminshahidi, M., Arastehfar, A., Pouladfar, G., Arman, E., and Fani, F. J. M. D. R. (2017). Diarrheagenic Escherichia coli and Shigella with high rate of extended-spectrum Beta-lactamase production: two predominant etiological agents of acute diarrhea in shiraz. Iran. Microb. Drug Resist. 23, 1037–1044. doi: 10.1089/mdr.2017.0204
Ammar, A. M., El-Hamid, A., Marwa, I., El-Malt, R., Azab, D. S., Albogami, S., et al. (2021). Molecular detection of fluoroquinolone resistance among multidrug-, extensively drug-, and pan-drug-resistant campylobacter species in Egypt. Antibiotics 10:1342. doi: 10.3390/antibiotics10111342
Auer, G. K., and Weibel, D. B. J. B. (2017). Bacterial cell mechanics. Biochemistry 56, 3710–3724. doi: 10.1021/acs.biochem.7b00346
Banerjee, S., Vishakha, K., Das, S., Sangma, P. D., Mondal, S., Ganguli, A., et al. (2022). Oxidative stress, DNA, and membranes targets as modes of antibacterial and antibiofilm activity of facile synthesized biocompatible keratin-copper nanoparticles against multidrug resistant uro-pathogens. World J. Microbiol. Biotechnol. 38, 1–16. doi: 10.1007/s11274-021-03187-z
Baranzoni, G. M., Fratamico, P. M., Gangiredla, J., Patel, I., Bagi, L. K., Delannoy, S., et al. (2016). Characterization of Shiga toxin subtypes and virulence genes in porcine Shiga toxin-producing Escherichia coli. Front. Microbiol. 7:574. doi: 10.3389/fmicb.2016.00574
Bashar, M. A., and Soundappan, K. (2022). Outbreak investigation of acute watery diarrhea in a village of North India: timely action saved lives. J. Infect. Dev. Countr. 16, 843–849. doi: 10.3855/jidc.13113
Behera, D. K., and Mishra, S. (2022). The burden of diarrhea, etiologies, and risk factors in India from 1990 to 2019: evidence from the global burden of disease study. BMC Public Health 22:92. doi: 10.1186/s12889-022-12515-3
Brinkmann, B. W., Koch, B. E., Spaink, H. P., Peijnenburg, W. J., and Vijver, M. G. (2020). Colonizing microbiota protect zebrafish larvae against silver nanoparticle toxicity. Nanotoxicology 14, 725–739. doi: 10.1080/17435390.2020.1755469
Brugman, S., Ikeda-Ohtsubo, W., Braber, S., Folkerts, G., Pieterse, C. M., and Bakker, P. A. (2018). A comparative review on microbiota manipulation: lessons from fish, plants, livestock, and human research. Front. Nutr. 5:80. doi: 10.3389/fnut.2018.00080
de Barros, D. P. C., Reed, P., Alves, M., Santos, R., and Oliva, A. (2021). Biocompatibility and antimicrobial activity of nanostructured lipid carriers for topical applications are affected by type of oils used in their composition. Pharmaceutics 13. doi: 10.3390/pharmaceutics13111950
Demb, E. R., Konat, A., Bonkoungou, I. J. O., KagambÃ, A. T., KonatÃ, K., Serge BagrÃ, T., et al. (2014). Serotyping and antimicrobial susceptibility of salmonella isolated from children under five years of age with diarrhea in rural Burkina Faso. Afr. J. Microbiol. Res. 8, 3157–3163. doi: 10.5897/AJMR2014.7002
Deng, Z., Rong, Y., Teng, Y., Mu, J., Zhuang, X., Tseng, M., et al. (2017). Broccoli-derived nanoparticle inhibits mouse colitis by activating dendritic cell AMP-activated protein kinase. Mol. Ther. 25, 1641–1654. doi: 10.1016/j.ymthe.2017.01.025
El-Aziz, A., Norhan, K., Ammar, A. M., Hamdy, M. M., Gobouri, A. A., Azab, E., et al. (2020). First report of aac C5-aad A7Δ4 gene cassette array and phage tail tape measure protein on class 1 Integrons of campylobacter species isolated from animal and human sources in Egypt. Animals 10:2067. doi: 10.3390/ani10112067
Endtz, H. P. (2020a). “Campylobacter infections,” Hunter’s Tropical Medicine and Emerging Infectious Diseases. Elsevier, 507–511.
Endtz, H. P. (2020b). “50-campylobacter infections” in Hunter's Tropical Medicine and Emerging Infectious Diseases. eds. E. T. Ryan, D. R. Hill, T. Solomon, N. E. Aronson, and T. P. Endy 10th ed (London: Elsevier), 507–511.
El-Naenaeey, E. S. Y., El-Aziz, A., Norhan, K., Sewid, A. H., Hashem, A., and Hefny, A. A. J. S. V. R. (2021). Antimicrobial resistance, virulence-associated genes, and flagellin typing of thermophilic campylobacter species isolated from diarrheic humans, raw milk, andbroiler niches. Slov. Vet. Res. 58, 155–164. doi: 10.26873/SVR-1436-2021
Ferdous, T. (2018). Prevalence of self medication practice with antibiotics among the students of some educational institutions of Dhaka and Gazipur district. Biores. Commun. 4, 555–564.
Feuerstein, A., Scuda, N., Klose, C., Hoffmann, A., Melchner, A., Boll, K., et al. (2022). Antimicrobial resistance, serologic and molecular characterization of E. coli isolated from calves with severe or fatal enteritis in Bavaria, Germany. Antibiotics 11:23. doi: 10.3390/antibiotics11010023
Ganesan, D., Gupta, S. S., and Legros, D. J. V. (2020). Cholera surveillance and estimation of burden of cholera. Vaccine 38, A13–A17. doi: 10.1016/j.vaccine.2019.07.036
Gharieb, R., Saad, M., Abdallah, K., Khedr, M., Farag, E., El-Fattah, A., et al. (2021). Insights on toxin genotyping, virulence, antibiogram profiling, biofilm formation and efficacy of disinfectants on biofilms of Clostridium perfringens isolated from poultry, animals and humans. J. Appl. Microbiol. 130, 819–831. doi: 10.1111/jam.14838
Gomes, T. A., Elias, W. P., Scaletsky, I. C., Guth, B. E., Rodrigues, J. F., Piazza, R. M., et al. (2016). Diarrheagenic Escherichia coli. Braz. J. Microbiol. 47, 3–30. doi: 10.1016/j.bjm.2016.10.015
Großkopf, A., and Simm, A. (2020). Carbohydrates in nutrition: friend or foe? Z. Gerontol. Geriatr. 53, 290–294. doi: 10.1007/s00391-020-01726-1
Hacquard, S., Garrido-Oter, R., González, A., Spaepen, S., Ackermann, G., Lebeis, S., et al. (2015). Microbiota and host nutrition across plant and animal kingdoms. Cell Host Microbe 17, 603–616. doi: 10.1016/j.chom.2015.04.009
Hano, C., and Abbasi, B. H. (2022). Plant-based green synthesis of nanoparticles: production, characterization and applications. Biomol. Ther. 12:31. doi: 10.3390/biom12010031
Harbarth, S., Balkhy, H. H., Goossens, H., Jarlier, V., Kluytmans, J., Laxminarayan, R., et al. (2015). Antimicrobial resistance: one world, one fight! Antimicrob. Resist. Infect. Control 4:49. doi: 10.1186/s13756-015-0091-2
Hobernik, D., and Bros, M. (2018). DNA vaccines—how far from clinical use? Int. J. Mol. Sci. 19:3605. doi: 10.3390/ijms19113605
Hu, J., and Torres, A. G. J. C. M. (2015). Infection enteropathogenic Escherichia coli: foe or innocent bystander? Clin. Microbiol. Infect. 21, 729–734. doi: 10.1016/j.cmi.2015.01.015
Huchin, C., Briceño, M. A., Mendoza, T., Martínez, A. P., Ramírez, M. A., Torres, J. C., et al. (2017). Prevalence and drug-resistance patterns of Enterotoxigenic Escherichia coli and Shigella species among children with diarrhea in Merida City, Mexico. J. Biosci. Med. 6, 22–33. doi: 10.4236/jbm.2018.61004
Hwang, S.-B., Chelliah, R., Kang, J. E., Rubab, M., Banan-Mwine Daliri, E., Elahi, F., et al. (2021). Role of recent therapeutic applications and the Infection strategies of Shiga toxin-producing Escherichia coli. Front. Cell. Infect. Microbiol. 11:450. doi: 10.3389/fcimb.2021.614963
Igere, B., and Ekundayo, T. J. M. P. (2020). Global mapping of cholera vibrio and outbreaks in the pre-millennium development goals (MDG)/sustainable development goals (SDG) and MDGs/SDGs era of 1990–2019. Microb. Pathog. 149:104319. doi: 10.1016/j.micpath.2020.104319
IHME, I. f. H. M. a. E. (2020). Global Burden of Disease Study 2019. Available at: http://vizhub.healthdata.org/gbd-compare
Inbaraj, S., Agrawal, R. K., Thomas, P., Mohan, C., Rk, S. A., Verma, M. R., et al. (2022). Antimicrobial resistance in Indian isolates of non typhoidal salmonella of livestock, poultry and environmental origin from 1990 to 2017. Comp. Immunol. Microbiol. Infect. Dis. 80:101719. doi: 10.1016/j.cimid.2021.101719
Iravani, S., and Varma, R. S. J. G. C. (2020). Greener synthesis of lignin nanoparticles and their applications. Green Chem. 22, 612–636. doi: 10.1039/C9GC02835H
Jung, K., Saif, L. J., and Wang, Q. (2020). Porcine epidemic diarrhea virus (PEDV): an update on etiology, transmission, pathogenesis, and prevention and control. Virus Res. 286:198045. doi: 10.1016/j.virusres.2020.198045
Kaabipour, S., and Hemmati, S. J. B. J. O. N. (2021). A review on the green and sustainable synthesis of silver nanoparticles and one-dimensional silver nanostructures. Beilstein. J. Nanotechnol. 12, 102–136. doi: 10.3762/bjnano.12.9
Kalsoom, M., Rehman, F., Shafique, T., Junaid, S., Khalid, N., Adnan, M., et al. (2020). Biological importance of microbes in agriculture, food and pharmaceutical industry: a review. Innov. J. Life Sci. 8, 1–4. doi: 10.22159/ijls.2020.v8i6.39845
Karimi-Maleh, H., Ayati, A., Ghanbari, S., Orooji, Y., Tanhaei, B., Karimi, F., et al. (2021). Recent advances in removal techniques of Cr (VI) toxic ion from aqueous solution: a comprehensive review. J. Mol. Liq. 329:115062. doi: 10.1016/j.molliq.2020.115062
Khan, M., Khan, A. U., Rafatullah, M., Alam, M., Bogdanchikova, N., and Garibo, D. J. B. (2022). Search for effective approaches to fight microorganisms causing high losses in agriculture: application of P. lilacinum metabolites and mycosynthesised silver nanoparticles. Biomol. Ther. 12:174. doi: 10.3390/biom12020174
Kharissova, O. V., Kharisov, B. I., Oliva González, C. M., Méndez, Y. P., and López, I. (2019). Greener synthesis of chemical compounds and materials. R. Soc. Open Sci. 6:191378. doi: 10.1098/rsos.191378
Kim, N.-O., Jung, S.-M., Na, H.-Y., Chung, G. T., Yoo, C.-K., Seong, W. K., et al. (2015). Enteric bacteria isolated from diarrheal patients in Korea in 2014. Osong Public Health Res. Perspect. 6, 233–240. doi: 10.1016/j.phrp.2015.07.005
Kim, J., Li, S., Zhang, S., and Wang, J. J. A. J. O. P. S. (2021). Plant-derived exosome-like nanoparticles and their therapeutic activities. Asian J. Pharmaceut. Sci. 17, 53–69. doi: 10.1016/j.ajps.2021.05.006
Korani, M., Ghazizadeh, E., Korani, S., Hami, Z., and Mohammadi-Bardbori, A. J. E. J. O. N. (2015). Effects Silver nanoparticles on human health. Int. J. Mol. Sci. 7, 51–62. doi: 10.1515/ejnm-2014-0032
Lee, L.-H., Ab Mutalib, N.-S., Law, J. W.-F., Wong, S. H., and Letchumanan, V. J. F. (2018). Discovery on antibiotic resistance patterns of Vibrio parahaemolyticus in Selangor reveals carbapenemase producing Vibrio parahaemolyticus in marine and freshwater fish. Front. Microbiol. 9:2513. doi: 10.1016/j.foodcont.2016.06.046
Li, H., Huang, J., Song, Y., Zhang, M., Wang, H., Lu, F., et al. (2018). Degradable carbon dots with broad-Spectrum antibacterial activity. ACS Appl. Mater. Interfaces 10, 26936–26946. doi: 10.1021/acsami.8b08832
Li, Y., Zhang, S., He, M., Zhang, Y., Fu, Y., Liang, H., et al. (2018). Prevalence and molecular characterization of campylobacter spp. isolated from patients with diarrhea in Shunyi, Beijing. Front. Microbiol. 9:52.
Liu, S., Quan, T., Yang, L., Deng, L., Kang, X., Gao, M., et al. (2021). N, cl-Codoped carbon dots from Impatiens balsamina L. stems and a deep eutectic solvent and their applications for gram-positive bacteria identification, antibacterial activity, cell imaging, and ClO–sensing. ACS. Omega 6, 29022–29036. doi: 10.1021/acsomega.1c04078
Liu, Y., Tan, M.-L., Zhu, W.-J., Cao, Y.-N., Peng, L.-X., Yan, Z.-Y., et al. (2022). In vitro effects of Tartary buckwheat-derived Nanovesicles on gut microbiota. J. Agric. Food Chem. 70, 2616–2629. doi: 10.1021/acs.jafc.1c07658
Livermore, D. M., Nicolau, D. P., Hopkins, K. L., and Meunier, D. J. C. I. D. (2020). Carbapenem-resistant Enterobacterales, carbapenem resistant organisms, carbapenemase-producing Enterobacterales, and carbapenemase-producing organisms: terminology past its “sell-by date” in an era of new antibiotics and regional carbapenemase epidemiology. Clin. Infect. Dis. 71, 1776–1782. doi: 10.1093/cid/ciaa122
Ma, Y., Zhang, M., Wang, H., Wang, B., Huang, H., Liu, Y., et al. (2020). N-doped carbon dots derived from leaves with low toxicity via damaging cytomembrane for broad-spectrum antibacterial activity. Mat. Today Commun. 24:101222. doi: 10.1016/j.mtcomm.2020.101222
Mac Kinnon, M. C., McEwen, S. A., Pearl, D. L., Lyytikäinen, O., Jacobsson, G., Collignon, P., et al. (2021). Mortality in Escherichia coli bloodstream infections: a multinational population-based cohort study. BMC Infect. Dis. 21, 1–10. doi: 10.1186/s12879-021-06326-x
Manzano, M., and Vallet-Regí, M. (2020). Mesoporous silica nanoparticles for drug delivery. Adv. Funct. Mater. 30:1902634. doi: 10.1002/adfm.201902634
Mare, A. D., Ciurea, C. N., Man, A., Tudor, B., Moldovan, V., Decean, L., et al. (2021). Enteropathogenic Escherichia coli—a summary of the literature. Gastroenterol. Insights 12, 28–40. doi: 10.3390/gastroent12010004
Mendelson, M., and Matsoso, M. P. J. S. S. A. M. J. (2015). The World Health Organization global action plan for antimicrobial resistance. S. Afr. Med. J. 105:325. doi: 10.7196/SAMJ.9644
Mewada, A., Pandey, S., Shinde, S., Mishra, N., Oza, G., Thakur, M., et al. (2013). Green synthesis of biocompatible carbon dots using aqueous extract of Trapa bispinosa peel. Mater. Sci. Eng. C 33, 2914–2917. doi: 10.1016/j.msec.2013.03.018
Missaoui, W. N., Arnold, R. D., and Cummings, B. S. (2018). Toxicological status of nanoparticles: what we know and what we don't know. Chem. Biol. Interact. 295, 1–12. doi: 10.1016/j.cbi.2018.07.015
Mullish, B. H., and Williams, H. R. J. C. M. (2018). Clostridium difficile infection and antibiotic-associated Diarrhea. Clin. Med. 18:237. doi: 10.7861/clinmedicine.18-3-237
Murray, C. J. L., Aravkin, A. Y., Zheng, P., Abbafati, C., Abbas, K. M., Abbasi-Kangevari, M., et al. (2020). Global burden of 87 risk factors in 204 countries and territories, 1990–2019: a systematic analysis for the global burden of disease study 2019. Lancet 396, 1223–1249. doi: 10.1016/S0140-6736(20)30752-2
Nannan, N., Groenewald, P., Pillay-van Wyk, V., Nicol, E., Msemburi, W., Dorrington, R., et al. (2019). Child mortality trends and causes of death in South Africa, 1997-2012, and the importance of a national burden of disease study. S. Afr. Med. J. 109, 480–485. doi: 10.7196/SAMJ.2019.v109i7.13717
Nemidkanam, V., and Chaichanawongsaroj, N. (2022). Characterizing Kaempferia parviflora extracellular vesicles, a nanomedicine candidate. PLoS One 17:e0262884. doi: 10.1371/journal.pone.0262884
Nkansa-Gyamfi, N. A., Kazibwe, J., Traore, D. A., and Nji, E. J. G. H. A. (2019). Prevalence of multidrug-, extensive drug-, and pandrug-resistant commensal Escherichia coli isolated from healthy humans in community settings in low-and middle-income countries: a systematic review and meta-analysis. Glob. Health Action 12:1815272. doi: 10.1080/16549716.2020.1815272
Pasquina-Lemonche, L., Burns, J., Turner, R., Kumar, S., Tank, R., Mullin, N., et al. (2020). The architecture of the gram-positive bacterial cell wall. Nature 582, 294–297. doi: 10.1038/s41586-020-2236-6
Rahim, A. A., Ahmadissa, S. M., Muhamad, L. R., and Hama Soor, T. A. J. M. B. (2021). Antibiotic resistance: current global issue and future challenges. Microbial. Biosystems 5, 29–68. doi: 10.21608/mb.2021.55637.1029
Raina, S., Thakur, A., Sharma, A., Pooja, D., and Minhas, A. P. (2020). Bactericidal activity of Cannabis sativa phytochemicals from leaf extract and their derived carbon dots and Ag@carbon dots. Mater. Lett. 262:127122. doi: 10.1016/j.matlet.2019.127122
Ramstad, S. N., Taxt, A. M., Naseer, U., Wasteson, Y., Bjørnholt, J. V., and Brandal, L. T. J. M. P. (2021). Effects of antimicrobials on Shiga toxin production in high-virulent Shiga toxin-producing Escherichia coli. Microb. Pathog. 152:104636. doi: 10.1016/j.micpath.2020.104636
Rogawski McQuade, E. T., Shaheen, F., Kabir, F., Rizvi, A., Platts-Mills, J. A., Aziz, F., et al. (2020). Epidemiology of Shigella infections and diarrhea in the first two years of life using culture-independent diagnostics in 8 low-resource settings. PLoS Negl. Trop. Dis. 14:e0008536. doi: 10.1371/journal.pntd.0008536
Rohde, M. J. M. S. (2019). The gram-positive bacterial cell wall. Microbiol. Spectr. 7:10. doi: 10.1128/microbiolspec.GPP3-0044-2018
Same, R. G., and Tamma, P. D. J. P. I. R. (2018). Campylobacter infections in children. Pediatr. Rev. 39:533. doi: 10.1542/pir.2017-0285
Saravanan, A., Maruthapandi, M., Das, P., Luong, J. H. T., and Gedanken, A. (2021). Green synthesis of multifunctional carbon dots with antibacterial activities. Nano 11:369. doi: 10.3390/nano11020369
Selvaraju, N., Ganesh, P. S., Palrasu, V., Venugopal, G., and Mariappan, V. (2022). Evaluation of Antimicrobial and Antibiofilm Activity of Citrus medica Fruit Juice Based Carbon Dots Against Pseudomonas aeruginosa. ACS Omega.
Shahshahanipour, M., Rezaei, B., Ensafi, A. A., and Etemadifar, Z. (2019). An ancient plant for the synthesis of a novel carbon dot and its applications as an antibacterial agent and probe for sensing of an anti-cancer drug. Mater. Sci. Eng. C 98, 826–833. doi: 10.1016/j.msec.2019.01.041
Sheikh, A. F., Moosavian, M., Abdi, M., Heidary, M., Shahi, F., Jomehzadeh, N., et al. (2019). Prevalence and antimicrobial resistance of Shigella species isolated from diarrheal patients in Ahvaz, Southwest Iran. Infect. Drug Resist. 12:249. doi: 10.2147/IDR.S187861
Su, S., and Kang, P. M. (2020). Systemic review of biodegradable nanomaterials in nanomedicine. Nano 10:656. doi: 10.3390/nano10040656
Sun, B., Wu, F., Zhang, Q., Chu, X., Wang, Z., Huang, X., et al. (2021). Insight into the effect of particle size distribution differences on the antibacterial activity of carbon dots. J. Colloid Interface Sci. 584, 505–519. doi: 10.1016/j.jcis.2020.10.015
Surendran, P., Lakshmanan, A., Priya, S. S., Geetha, P., Rameshkumar, P., Kannan, K., et al. (2021). Fluorescent carbon quantum dots from Ananas comosus waste peels: a promising material for NLO behaviour, antibacterial, and antioxidant activities. Inorg. Chem. Commun. 124:108397. doi: 10.1016/j.inoche.2020.108397
Tarr, P. I., and Freedman, S. B. J. C. (2022). Why antibiotics should not be used to treat Shiga toxin-producing Escherichia coli infections. Curr. Opin. Gastroenterol. 38, 30–38. doi: 10.1097/MOG.0000000000000798
Taylor, D. N., Hamer, D. H., and Shlim, D. R. (2017). Medications for the prevention and treatment of travellers’ diarrhea. J. Travel Med. 24, S17–S22. doi: 10.1093/jtm/taw097
Terefe, Y., Deblais, L., Ghanem, M., Helmy, Y. A., Mummed, B., Chen, D., et al. (2020). Co-occurrence of campylobacter species in children from eastern Ethiopia, and their association with environmental enteric dysfunction, diarrhea, and host microbiome. Front. Public Health 8:99. doi: 10.3389/fpubh.2020.00099
Troeger, C., Blacker, B. F., Khalil, I. A., Rao, P. C., Cao, S., Zimsen, S. R., et al. (2018). Estimates of the global, regional, and national morbidity, mortality, and aetiologies of Diarrhea in 195 countries: a systematic analysis for the global burden of disease study 2016. Lancet Infect. Dis. 18, 1211–1228. doi: 10.1016/S1473-3099(18)30362-1
Vakili-Ghartavol, R., Momtazi-Borojeni, A. A., Vakili-Ghartavol, Z., Aiyelabegan, H. T., Jaafari, M. R., Rezayat, S. M., et al. (2020). Toxicity assessment of superparamagnetic iron oxide nanoparticles in different tissues. Artific. Cells Nanomed. Biotechnol. 48, 443–451. doi: 10.1080/21691401.2019.1709855
Vasimalai, N., Vilas-Boas, V., Gallo, J., Cerqueira, M. F., Menendez-Miranda, M., Costa-Fernández, J. M., et al. (2018). Green synthesis of fluorescent carbon dots from spices for in vitro imaging and tumor cell growth inhibition. Beilstein. J. Nanotechnol. 9, 530–544. doi: 10.3762/bjnano.9.51
Venkat Kumar, S., and Rajeshkumar, S. (2018). “Chapter 2-plant-based synthesis of nanoparticles and their impact” in Nanomaterials in Plants, Algae, and Microorganisms. eds. D. K. Tripathi, P. Ahmad, S. Sharma, D. K. Chauhan, and N. K. Dubey (Academic Press), 33–57.
Vidal, R. M., Muhsen, K., Tennant, S. M., Svennerholm, A.-M., Sow, S. O., Sur, D., et al. (2019). Colonization factors among enterotoxigenic Escherichia coli isolates from children with moderate-to-severe diarrhea and from matched controls in the global enteric multicenter study (GEMS). PLoS Negl. Trop. Dis. 13:e0007037. doi: 10.1371/journal.pntd.0007037
Vos, T., Lim, S. S., Abbafati, C., Abbas, K. M., Abbasi, M., Abbasifard, M., et al. (2020). Global burden of 369 diseases and injuries in 204 countries and territories, 1990–2019: a systematic analysis for the global burden of disease study 2019. Lancet 396, 1204–1222. doi: 10.1016/S0140-6736(20)30925-9
Wang, H., Zhang, M., Ma, Y., Wang, B., Shao, M., Huang, H., et al. (2020). Selective inactivation of gram-negative bacteria by carbon dots derived from natural biomass: Artemisia argyi leaves. J. Mater. Chem. B 8, 2666–2672. doi: 10.1039/C9TB02735A
Wei, X.-S., Wang, X., Niu, Y.-R., Ye, L.-L., Peng, W.-B., Wang, Z.-H., et al. (2020). Diarrhea is associated with prolonged symptoms and viral carriage in corona virus disease 2019. Clinical gastroenterology. Hepatology 18, 1753–1759. doi: 10.1016/j.cgh.2020.04.030
Wei, Z., Xu, X., Yan, M., Chang, H., Li, Y., Kan, B., et al. (2019). Salmonella typhimurium and salmonella enteritidis infections in sporadic diarrhea in children: source tracing and resistance to third-generation cephalosporins and ciprofloxacin. Foodborne Pathogens 16, 244–255. doi: 10.1089/fpd.2018.2557
Wong, C. S., Jelacic, S., Habeeb, R. L., Watkins, S. L., and Tarr, P. I. J. N. E. J. O. M. (2000). The risk of the hemolytic–uremic syndrome after antibiotic treatment of Escherichia coli O157: H7 infections. N. Engl. J. Med. 342, 1930–1936. doi: 10.1056/NEJM200006293422601
Xiao, J., Feng, S., Wang, X., Long, K., Luo, Y., Wang, Y., et al. (2018). Identification of exosome-like nanoparticle-derived micro RNAs from 11 edible fruits and vegetables. Peer 6:e5186. doi: 10.7717/peerj.5186
Yaghoubi, S., Ranjbar, R., Dallal, M. M. S., Fard, S. Y., Shirazi, M. H., Mahmoudi, M., et al. (2017). Profiling of virulence-associated factors in Shigella species isolated from acute pediatric diarrheal samples in Tehran, Iran. Osong Public Health Res. Perspect. 8:220. doi: 10.24171/j.phrp.2017.8.3.09
Yoh, M., Frimpong, E., Honda, T. J. F. I., and Microbiology, M. (1997). Effect of antimicrobial agents, especially fosfomycin, on the production and release of Vero toxin by enterohaemorrhagic Escherichia coli O157: H7. FEMS Immunol. Med. Microbiol. 19, 57–64. doi: 10.1111/j.1574-695X.1997.tb01072.x
Yue, M., Li, X., Liu, D., and Hu, X. (2020). Serotypes, antibiotic resistance, and virulence genes of salmonella in children with diarrhea. J. Clin. Lab. Anal. 34:e23525. doi: 10.1002/jcla.23525
Zhang, M., Viennois, E., Xu, C., and Merlin, D. (2016). Plant derived edible nanoparticles as a new therapeutic approach against diseases. Tissue Barriers 4:e1134415. doi: 10.1080/21688370.2015.1134415
Zhang, J., Xu, Y., Ling, X., Zhou, Y., Lin, Z., Huang, Z., et al. (2020). Identification of diarrheagenic Escherichia coli by a new multiplex PCR assay and capillary electrophoresis. Mol. Cell. Probes 49:101477. doi: 10.1016/j.mcp.2019.101477
Zhang, P., Zhang, X., Liu, Y., Jiang, J., Shen, Z., Chen, Q., et al. (2020). Multilocus sequence types and antimicrobial resistance of campylobacter jejuni and C. coli isolates of human patients from Beijing, China, 2017–2018. Front. Microbiol. 11:2542.
Zhou, H.-L., Bessey, T., Wang, S.-M., Mo, Z.-J., Barclay, L., Wang, J.-X., et al. (2021). Burden and etiology of moderate and severe diarrhea in children less than 5 years of age living in north and south of China: prospective, population-based surveillance. Gut Pathogens. 13, 1–11. doi: 10.1186/s13099-021-00428-2
Keywords: biocontrol, diarrheagenic bacteria, multidrug resistance, nanotechnology, therapeutic agent, antimicrobial agent, metal-free plant-derived nanoparticles
Citation: Abolarinwa TO, Ajose DJ, Oluwarinde BO, Fri J, Montso KP, Fayemi OE, Aremu AO and Ateba CN (2022) Plant-derived nanoparticles as alternative therapy against Diarrheal pathogens in the era of antimicrobial resistance: A review. Front. Microbiol. 13:1007115. doi: 10.3389/fmicb.2022.1007115
Edited by:
Mahendra Rai, Sant Gadge Baba Amravati University, IndiaReviewed by:
Sazzad Hossen Toushik, Gyeongsang National University, South KoreaAngela Ivask, University of Tartu, Estonia
Copyright © 2022 Abolarinwa, Ajose, Oluwarinde, Fri, Montso, Fayemi, Aremu and Ateba. This is an open-access article distributed under the terms of the Creative Commons Attribution License (CC BY). The use, distribution or reproduction in other forums is permitted, provided the original author(s) and the copyright owner(s) are credited and that the original publication in this journal is cited, in accordance with accepted academic practice. No use, distribution or reproduction is permitted which does not comply with these terms.
*Correspondence: Collins Njie Ateba, collins.ateba@nwu.ac.za