- 1Key Laboratory of Animal Genetics, Breeding and Reproduction, Ministry of Education, College of Animal Science and Technology, Huazhong Agricultural University, Wuhan, China
- 2International Joint Research Centre for Animal Genetics, Breeding and Reproduction (IJRCAGBR), Huazhong Agricultural University, Wuhan, China
- 3Animal Production Department, Faculty of Agriculture, Assiut University, Asyut, Egypt
- 4Faculty of Veterinary and Animal Sciences, Islamia University of Bahawalpur, Bahawalpur, Pakistan
- 5Bright Farming Co. Ltd., Shanghai, China
- 6Hubei Province’s Engineering Research Center in Buffalo Breeding and Products, Wuhan, China
This study aimed to determine the effect of capsicum oleoresin (CAP) on rumen fermentation and microbial abundance under different temperature and dietary conditions in vitro. The experimental design was arranged in a 2 × 2 × 3 factorial format together with two temperatures (normal: 39°C; hyperthermal: 42°C), two forage/concentrate ratios (30:70; 70:30), and two CAP concentrations in the incubation fluid at 20 and 200 mg/L with a control group. Regarding the fermentation characteristics, high temperature reduced short-chain fatty acids (SCFA) production except for molar percentages of butyrate while increasing acetate-to-propionate ratio and ammonia concentration. The diets increased total SCFA, propionate, and ammonia concentrations while decreasing acetate percentage and acetate-to-propionate ratio. CAP reduced acetate percentage and acetate-to-propionate ratio. Under hyperthermal condition, CAP could reduce acetate percentage and increase acetate-to-propionate ratio, lessening the negative effect of high heat on SCFA. Hyperthermal condition and diet altered the relative abundance of microbial abundance in cellulose-degrading bacteria. CAP showed little effect on the microbial abundance which only increased Butyrivibrio fibrisolvens. Thus, CAP could improve rumen fermentation under different conditions, with plasticity in response to the ramp of different temperature and dietary conditions, although hardly affecting rumen microbial abundance.
Introduction
In the ruminants, the rumen functioning is susceptible to climatic and dietary changes. It was reported that temperature and feed characteristics (forage: concentration) are significant parameters that affect rumen function (Sales et al., 2021). Ruminal temperature is influenced by severe changes in climatic conditions (Lees et al., 2019; Sullivan et al., 2022). Fluctuations in ruminal temperature significantly affect rumen microbial abundance and short-chain fatty acids (SCFA) production (Uyeno et al., 2010; Wang et al., 2022). Similarly, diet is the other main factor affecting rumen fermentation variables which serves as the medium for the survival of rumen microorganisms (Mavrommatis et al., 2021a). The rumen is stressed more by using a high-concentrate diet, which is the common nutritional strategy under heat stress (Nasrollahi et al., 2019). Ruminal metabolic diseases caused by a high-concentrate diet jeopardizes productivity of animal (Tian et al., 2015). Therefore, new solutions are needed to be investigated in nutritional measures to maintain normal rumen physiology under high-temperature stress and high-concentrate ratios to improve ruminant productivity.
Capsicum oleoresin (CAP) is extracted from capsicum and the active ingredient is capsaicin. As a dietary additive, CAP has a variety of functions, such as anti-inflammatory (Ghiasi et al., 2019), antioxidant (Karadas et al., 2014), regulation of rumen fermentation (Temmar et al., 2021), and alteration of gut microbiota (Grazziotin et al., 2020) which may be beneficial to dairy cows. Meanwhile, CAP has been shown to be a functional additive in the regulation of metabolic diseases caused by high-concentrate diet and resist heat stress. It has been reported that CAP could affect rumen fermentation (Fandiño et al., 2008), alter feeding behavior, and may affect ruminal acidosis (Castillo-Lopez et al., 2021) in high-concentrate diet. Additionally, CAP against overheating of the body by thermo regulating (Szolcsányi, 2015) and probably affects productivity caused by heat stress. It has been shown CAP could reduce rectal temperature and improve productive performance in dairy cows (Da Silva, 2017) and pigs (Kroscher et al., 2022). Nevertheless, the CAP effect on rumen fermentation and microbial abundance is unclear at high-temperature and different dietary compositions, which limits the further application of CAP. In response to this situation, this study investigated the CAP effect on ruminal fermentation characteristics and microbial abundance under high heat conditions and different fermentation substrates.
Materials and methods
Experimental design and treatments
The experiment conditions were arranged in a 2 × 2 × 3 factor design, including two temperatures (normal: 39°C; hyperthermal: 42°C), two diet ratios [forage/concentrate ratio, 30:70 (low forage, LF); 70:30 (high forage, HF)], and two CAP concentrations in the incubation fluid at 20 (LC) and 200 mg/L (HC) with a control group (CON). Physiological temperature and high temperature were selected based on physiological rumen temperature (Eihvalde et al., 2016) and the maximum rumen temperature under heat stress (Beatty et al., 2008). Fermentation substrates were composed of whole corn silage and commercial concentrate. Corn silage and concentrate were dried at 65°C for 48 h and passed through a 1 mm sieve. The commercial CAP products are provided by Tianxu Food Additive Co. Ltd. (MY1098, 10.0% capsaicin, Guangzhou, China). The fermentation substrates are presented in Table 1.
In vitro fermentations and sampling
Rumen fluid was collected from four cows immediately after slaughtering at a commercial slaughterhouse (Heng Xing Slaughterhouse, Wuhan, China). All cows were fed a total mixed diet mainly consisting of silage, excluding disease factors, and rumen fluid was collected individually through four layers of cheesecloth within 15 min after slaughter and reached the laboratory within 30 min. Rumen fluid was maintained in a preheated bottle without top air at 39°C after collecting until it reached the laboratory. Next, fresh buffer solutions were mixed according to Beatty et al. (2008)). Briefly, rumen fluid was mixed in equal proportion and added to the buffer at the ratio of 1:2 with 39°C under continuous CO2 flushing. Fermentation substrate (0.5 g) and incubation fluid (50 mL) were added to each fermentation flask, injected with CO2, and sealed with a butyl rubber plug and aluminum cap. Finally, the flask was placed in two constant temperature shock incubators set at 39°C and 42°C, respectively.
At the end of fermentation, the pH of the incubation fluid was measured with a pH meter (FE28 – Standard, Mettler Toledo, Switzerland). The collected culture medium was divided into 2 mL sterile tubes. Isometric samples were collected and stored at −20°C immediately for ammonium and SCFA analysis. The remaining samples were stored at −80°C with one isometric sample for further microbial abundance analysis and the remaining samples were retained.
Chemical analysis
For SCFA analysis, incubation fluid (1 mL) was added to 200 μL of 25% metaphosphoric acid and centrifuged at 12,000 g for 10 min at 4°C to obtain the supernatant. Using a gas chromatograph (7890A, Agilent Technologies, Santa Clara, CA, United States), capillary column (30 m × 0.25 mm × 00.25 μm, DB – FFAP, Agilent Technologies, Santa Clara, CA, United States), and a pyrophoric detector. The oven temperature was initially maintained at 80°C for 5 min, increased to 190°C, 12.5°C/min, and held at 190°C for 3 min. The injector and detector temperatures were 250°C and 280°C, respectively. Determination of ammonia nitrogen was performed by colorimetry according to Weatherburn (1967).
The CH4 yield was calculated according to previously reported formula (Moss et al., 2000), considering a hydrogen recovery of 90% (default):
CH4 (mmol/L) = 0.45 × Acetate (mmol/L) – 0.275 × Propionate (mmol/L) + 0.40 × Butyrate (mmol/L).
Microbial analysis
Microbial DNA was extracted from rumen fluid using a Tiangen commercial kit according to the instructions (DP328-02, Tiangen Biotechnology Co., Ltd, Beijing, China) as reported in a previous study (Ding et al., 2014), and DNA concentration and purity were determined by spectrophotometer. Microorganisms in the samples were determined by SYBR Green quantitative real-time polymerase chain reaction (qPCR) assay for different microbiota as described by Zhu et al. (2017)). Briefly, 5 μL of SYBR Green q PCR Master Taq (2×), 0.2 μL of each primer, 0.5 μL of genomic DNA (10 ng/μL), and double-distilled water were added to make a total volume of 10 μL. Specific primers were presented in Table 2. All primers amplification started with a denaturalization at 95°C for 3 min, followed by 40 cycles of 95°C for 5 s, 60°C for 30 s, and 72°C for 30 s. All qPCR assays were performed in triplicate for each sample.
The relative population sizes of total bacteria, total anaerobic fungi, total protozoa, total methanogens, Ruminococcus albus, Ruminococcus flavefaciens, Butyrivibrio fibrisolvens, Fibrobacter succinogenes, Prevotella ruminicola, and Ruminobacter amylophilus were expressed as the proportion of 16S rDNA of total rumen bacteria at 24 h. Relative abundance was expressed as a proportion of the specific gene fragments number to the total rumen bacterial 16S rDNA number, calculated according to the following equation: relative quantification = 2− (Ct target − Ct total bacteria), where Ct represents threshold cycle based on the report of Castagnino et al. (2015)).
Statistical analysis
All statistical analyses were performed using the PROC MIXED procedure of SAS 9.4 (SAS Institute Inc.). Data were analyzed according to factorial permutations in a randomized compartmentalized design. The main effects of amylases include temperature, diet, and CAP levels, their 2-way, 3-way interactions, and the random effects. For daily measurements, the data were analyzed as repeated measurements. The composite symmetry was a variance–covariance structure. All values were presented as least squares mean ± standard error of the mean (SEM), stated otherwise. Comparisons between LS means were performed according to the PDIFF function. All experiments and analyses were made in triplicate. The analysis of variance followed by a Duncan test (p < 0.05) was used for the mean comparison and the trend was set at 0.05 < p ≤ 0.10.
Results
Rumen fermentation
The fermentation characteristics influenced by culture conditions and CAP addition are indicated in Table 3. SCFA decreased by hyperthermal condition and increased by high-concentrate diets (p < 0.05), while there was no effect on CAP addition. Hyperthermal condition increased the molar percentages of butyrate, isobutyrate, and acetate-to-propionate ratio while decreasing propionate and caproate percentage (p < 0.05). The addition of CAP decreased acetate percentage and acetate-to-propionate ratio (p < 0.05) and tended to increase propionate percentage (p = 0.09). Hyperthermal condition and high concentration diet increased ammonia concentrations (p < 0.05), and there was an interaction between temperature and diet (p < 0.05). Temperature tended to increase pH in the hyperthermal group compared to the normal group (p = 0.09). Temperature, diet, and CAP addition did not affect methane.
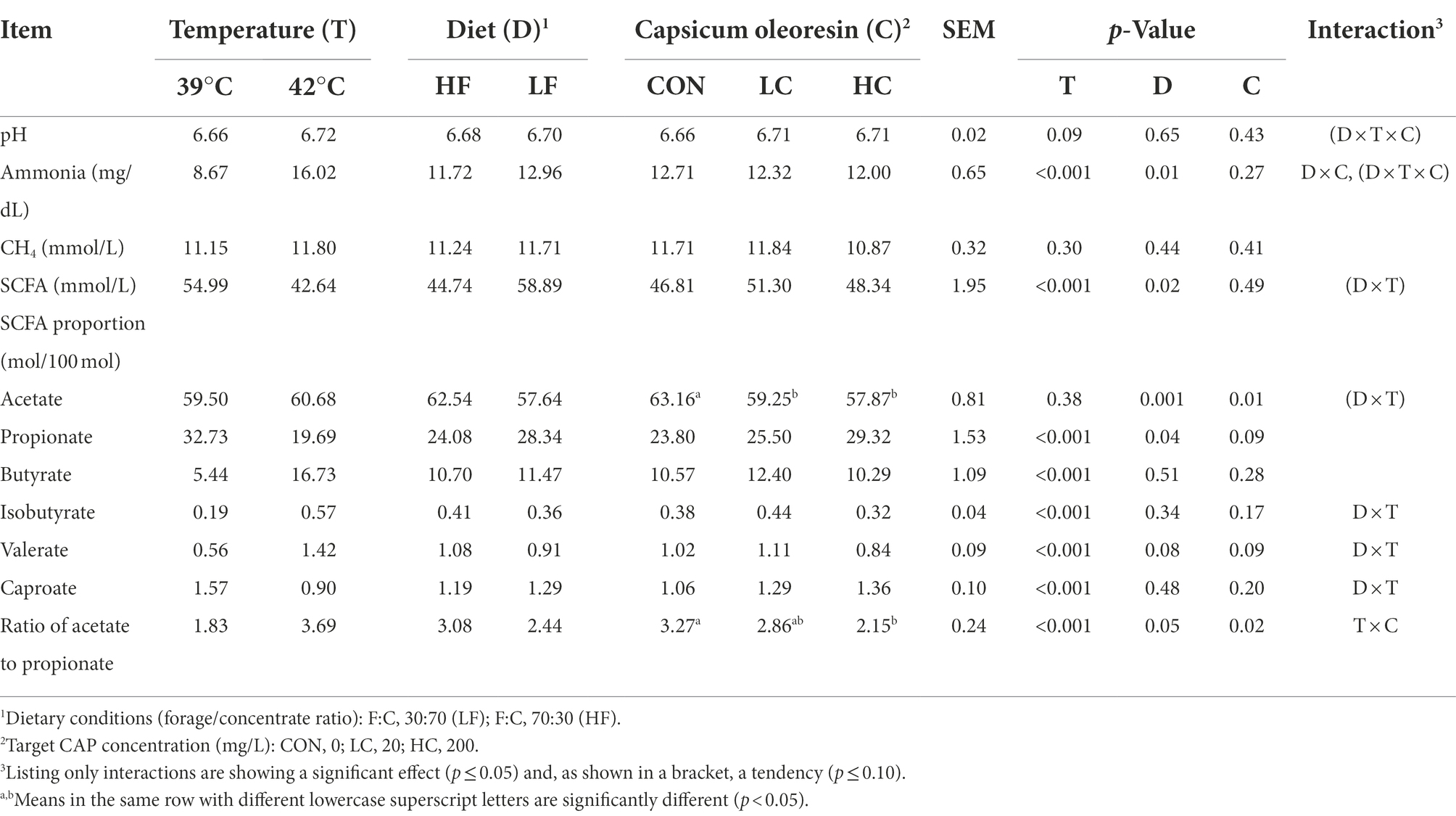
Table 3. Fermentation characteristics as affected by temperature, diet, Capsicum oleoresin (CAP) supplementation, and their interactions.
Under hyperthermal condition, the CAP group reduced the SCFA in LC (p < 0.05) compared with the CON and HC (Figure 1). The HC group decreased acetate percentage and acetate to propionate ratio relative to the CON (p < 0.05) while tending to increase propionate percentage (p ≤ 0.10). At the same time, hyperthermal condition reduced propionate percentage and increased acetate to propionate ratio in the CON and LC compared with physiological temperature (p < 0.05), while there was no difference in the HC group.
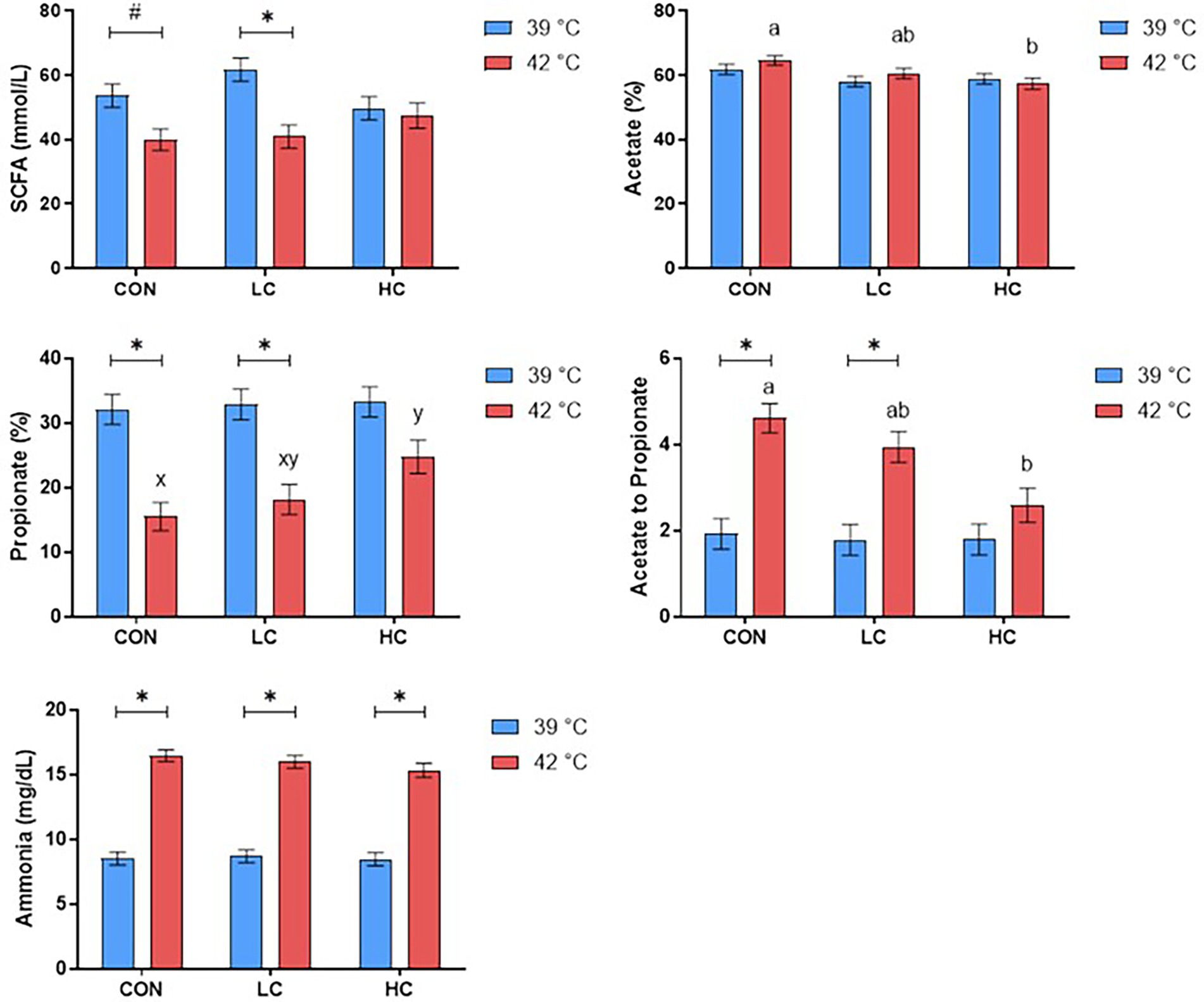
Figure 1. Concentrations of short-chain fatty acids (SCFA) and ammonia were affected by capsicum oleoresin (CAP) supplementation and incubation temperature. Least-square means of CAP groups within each temperature sharing no common letter differ at p ≤ 0.05 (a, b) or 0.05 < p ≤ 0.10 (x, y). Differences between the diet within each CAP group (p ≤ 0.05) (*) or 0.05 < p ≤ 0.10 (#). CAP levels: CON: 0 mg/L; LC: 20 mg/L; and HC: 200 mg/L.
As shown in Figure 2, the LF increased the propionate, decreased acetate percentage and acetate-to-propionate ratio (p < 0.05), and tended to decrease valerate percentage (p = 0.08). Under dietary factors, LF tended to decrease acetate concentrations in the LC (P tended to decrease valerate percentage (p = 0.08). Under dietary factors, LF tended to decrease acetate concenF (p < 0.05), and the ammonia concentration in the LF was significantly higher in the HC than in the LF (p < 0.05).
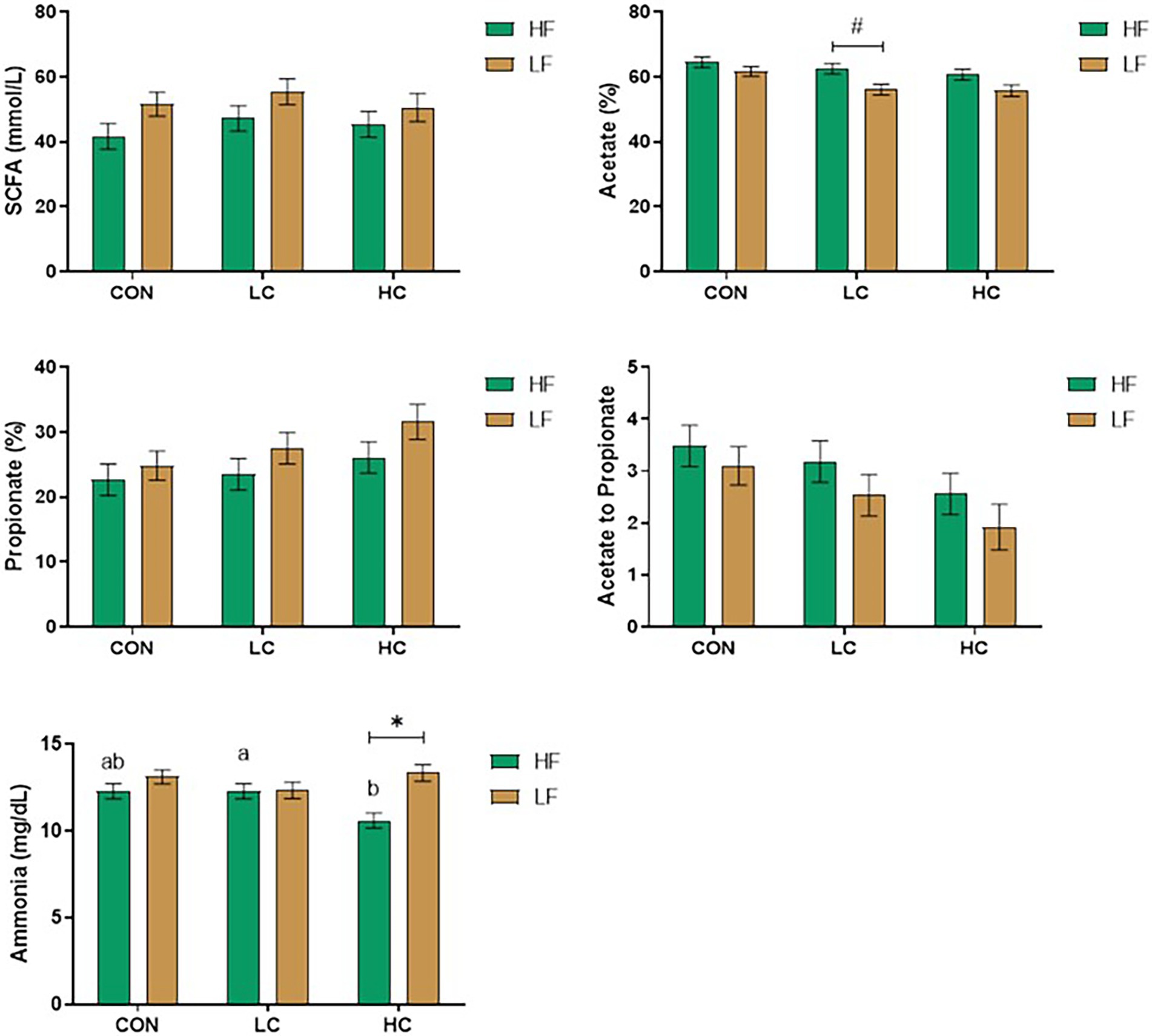
Figure 2. Concentrations of short-chain fatty acids (SCFA) and ammonia were affected by capsicum oleoresin (CAP) supplementation and diet. Least-square means of CAP groups within each diet sharing no common letter differ at P ≤ Concentrations. Differences between the dietary conditions within each CAP group (p ≤ 0.05) (*) or 0.05 < p ≤ 0.10 (#). Dietary conditions (forage/concentrate ratio): LF: 30:70; HF: 70:30. CAP levels: CON: 0 mg/L; LC: 20 mg/L; and HC: 200 mg/L.
Rumen bacteria
The results of the relative abundance of microbial populations are presented in Table 4. Hyperthermal condition increased the relative abundance of total anaerobic fungi, total methanogens, and B. fibrisolvens, while it decreased total protozoa, F. succinogenes, and P. amylophilus (p < 0.05). There is no difference in R. albus, R. flavefaciens, and R. ruminicola by the effect of hyperthermal condition. The diets significantly decreased total anaerobic fungi, B. fibrisolvens, F. succinogenes, and P. amylophilus (p < 0.05) while tended to increase total protozoa and lower Ruminococcus flavefaciens (p < 0.10). The CAP significantly increased the relative abundance of B. fibrisolvens in the LC relative to the CON (p < 0.05).
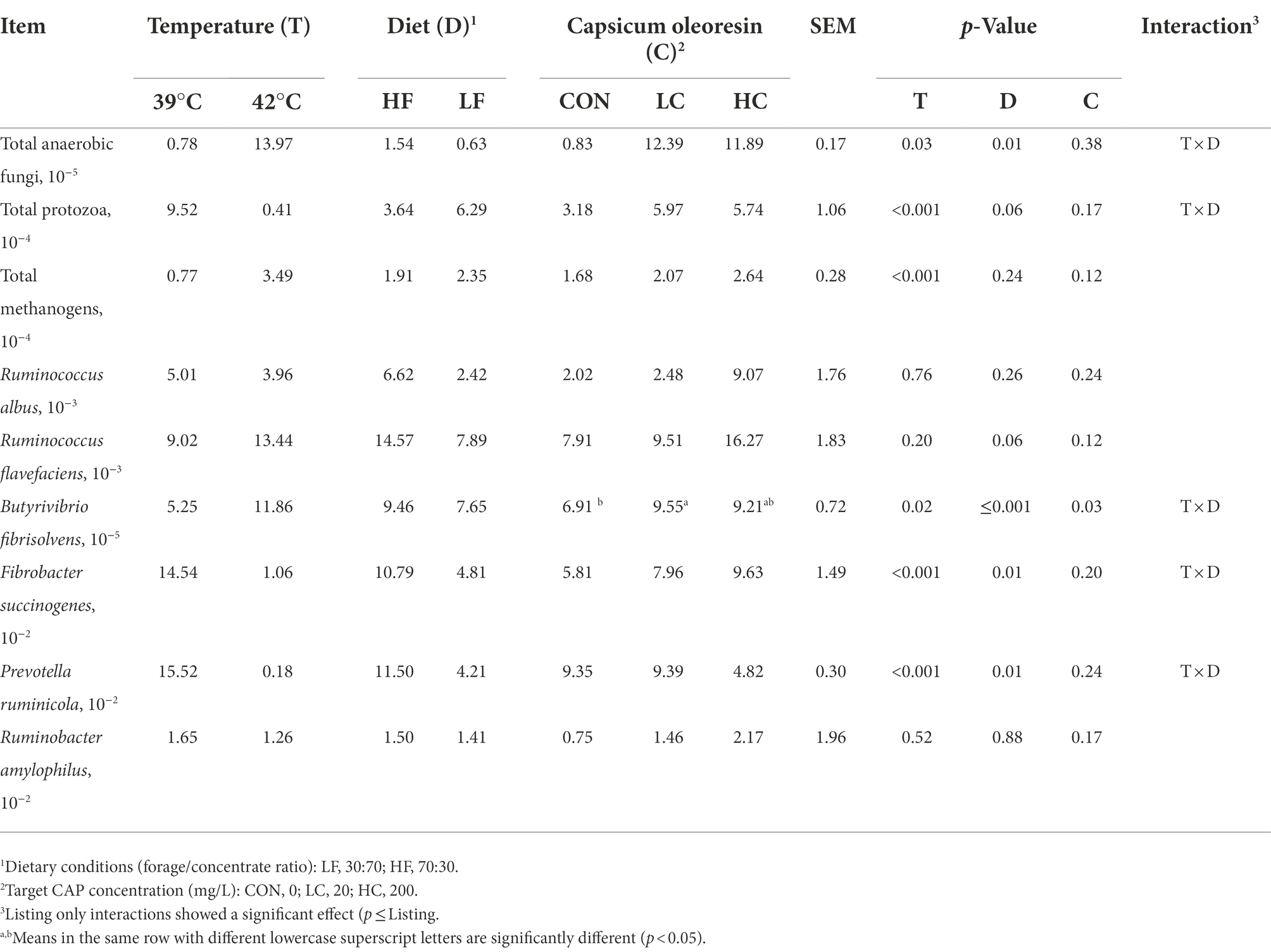
Table 4. Microbial relative abundance as affected by incubation temperature, dietary conditions, CAP supplementation, and their interactions.
Discussion
The proper achievement of rumen function would depend on temperature and diet composition and affects the ruminants’ health and productivity (Mavrommatis et al., 2021b; Phesatcha et al., 2021; Soltan et al., 2021). CAP has been extensively studied as a feed additive for ruminants in vivo (Oh et al., 2015; Castillo-Lopez et al., 2021) and in vitro (Busquet et al., 2006); however, little attention has been paid to its effect on rumen fermentation when the rumen is subjected to physiological stress. Therefore, the present study investigated whether CAP could affect rumen function and microbial abundance in the presence and/or absence of hyperthermal condition or under different temperature and dietary conditions. We hypothesized that CAP could maintain rumen fermentation characteristics and microbial abundance.
In this study, temperature and diet significantly affected SCFA concentration in incubation fluid. Although hyperthermal condition reduced SCFA due to a decrease in propionate percentage consistent with the findings of Mahmood et al. (2020)), the temperature did not affect the acetate percentage; it increased the butyrate percentage. Due to milk fat could be synthesized by butyrate (Cheng et al., 2020), there may increase in milk fat under heat stress in dairy cows (Liu et al., 2017). The LF increased SCFA due to increased propionate percentage, similar to the previous study in vitro (Natel et al., 2019) and in vivo (Ranathunga et al., 2019), and resulted in a difference in acetate-to-propionate ratio. Although CAP could not affect SCFA, it reduced the acetate percentage and tended to increase propionate percentage, consequently reducing acetate-to-propionate ratio (Cardozo et al., 2005). Previously, several studies have shown the exciting phenomenon that CAP cannot affect SCFA and propionate percentage (Busquet et al., 2006; Tager and Krause, 2010; Geron et al., 2019), while only the same effect was produced in LF (Fandiño et al., 2008, 2020). Thus, CAP can only alter SCFA composition under a high-concentrate diet. Also, CAP altered SCFA concentrations in the LC group under hyperthermal condition compared to physiological temperatures. Although CAP decreased acetate-to-propionate ratio and tended to increase the propionate percentage under hyperthermia conditions, it had no effect at physiological temperature. Therefore, it was suggested that CAP altered the fermentation pattern of the rumen under hyperthermia conditions. The effect of CAP on rumen fermentation in different diets was minimal and only trended down the acetate at LF compared to HF. However, the effect of CAP on rumen fermentation in vivo under high-concentrate diets may be more due to its stimulation of the digestive tract increasing the secretion of digestive juices, or due to the stimulating nature of capsaicin increasing the duration and frequency of feeding (Patcharatrakul et al., 2020; Castillo-Lopez et al., 2021; Shiiba et al., 2021).
Both diet and temperature increased ammonia concentration; the diet effect was attributed to the crude protein content of the fermentation substrate, while the temperature was responsible for the fermentation inhibition (Castillejos et al., 2005; Phesatcha et al., 2021). Regardless of diet and temperature, the pH of the incubation solution was within the normal range. In this study, CAP could not affect the pH and ammonia concentration of the incubation solution. Although CAP did not affect the increased ammonia concentration due to hyperthermia, ammonia concentration at HF was reduced in the HC, indicating improved microbial ammonia utilization.
Ruminal microorganisms are significant players in ruminal fermentation and are influenced by physicochemical factors, diet changes, and feed additives. Although the literature about the effect of hyperthermia on rumen microbial composition is scarce, it is one of the environmental factors that reduce ruminant performance (Lees et al., 2018; Idris et al., 2021). In the present study, hyperthermal condition increased the relative abundance of anaerobic fungi and methanogenic bacteria. Since anaerobic fungi stimulate the growth of methanogenic bacteria, this may lead to similar results to the previous study (Li et al., 2021). Also, methane production increased due to the action of methanogenic bacteria, although it was not found in this experiment.
On the other hand, hyperthermia reduced the relative abundance of total protozoa consistent with previous studies. It is generally believed that a decrease in the relative abundance of total protozoa leads to a decrease in ammonia concentration based on their phagocytosis of other bacteria (Firkins et al., 2007); however, this has not been found in the present experiment. The latter contradiction may be due to hyperthermia’s inhibition of microbial colonization. Meanwhile, hyperthermia resulted in changes in rumen microbial populations, including a reduction in the relative abundance of F. succinogenes and P. ruminicola, as reported in previous studies (Yadav et al., 2013; Sales et al., 2021). However, in contrast, hyperthermia increased the relative abundance of BF, which may lead to an increase in butyrate concentration.
The microbial community composition due to the diet is more intuitive relative to temperature, mainly regarding the relative abundance of cellulose-degrading bacteria (Kumar et al., 2015). In the present study, HF increased the relative abundance of total anaerobic fungi, B. fibrisolvens, F. succinogenes, and P. ruminicola. Ruminobacter amylophilus is the primary starch-degrading bacterium in rumen fluid and is known to contribute to propionate production (O’Herrin and Kenealy, 1993), however, HF could not affect the relative abundance of R. amylolous but could increase propionate concentration in this experiment. CAP affects various microorganisms, including cellulose-degrading bacteria of rumen origin under culture conditions in vitro (Demirtaş, 2020). In this experiment, CAP increased the relative abundance of B. fibrisolvens, which was the same as in previous studies in vivo (Oh et al., 2015). Additionally, Demirtaş (2020)) reported B. fibrisolvens could be stimulated by capsaicin in pure cultures. The role of B. fibrisolvens is mainly to degrade cellulose and consumes acetate in rumen fermentation for conversion to butyrate (Russell and Strobel, 1989). CAP did not affect butyrate percentage; however, it reduced acetate percentage in this research.
Conclusion
Hyperthermal condition and dietary factors could alter SCFA concentrations, acetate percentage, propionate percentage, acetate-to-propionate ratio, and ammonia concentrations. The CAP could influence ruminal fermentation in vitro, by reducing acetate percentage and acetate-to-propionate ratio. Microbial abundance was mainly influenced by temperature and diet, while the effect of CAP was minimal.
Data availability statement
The raw data supporting the conclusions of this article will be made available by the authors, without undue reservation.
Author contributions
ZA and GL wrote the manuscript. MA, UR, and LY revised the manuscript. SG investigated for this research. ZY, TY, and HL provided the methodology for this research. JZ and CC did the formal analysis. All authors have read and agreed to the published version of the manuscript.
Funding
This work was supported by the earmarked fund for CARS36.
Conflict of interest
CC was employed by Bright Farming Co. Ltd.
The remaining authors declare that the research was conducted in the absence of any commercial or financial relationships that could be construed as a potential conflict of interest.
Publisher’s note
All claims expressed in this article are solely those of the authors and do not necessarily represent those of their affiliated organizations, or those of the publisher, the editors and the reviewers. Any product that may be evaluated in this article, or claim that may be made by its manufacturer, is not guaranteed or endorsed by the publisher.
References
Beatty, D., Barnes, A., Taylor, E., and Maloney, S. (2008). Do changes in feed intake or ambient temperature cause changes in cattle rumen temperature relative to core temperature? J. Therm. Biol. 33, 12–19. doi: 10.1016/j.jtherbio.2007.09.002
Busquet, M., Calsamiglia, S., Ferret, A., and Kamel, C. (2006). Plant extracts affect in vitro rumen microbial fermentation. J. Dairy Sci. 89, 761–771. doi: 10.3168/jds.S0022-0302(06)72137-3
Cardozo, P., Calsamiglia, S., Ferret, A., and Kamel, C. (2005). Screening for the effects of natural plant extracts at different pH on in vitro rumen microbial fermentation of a high-concentrate diet for beef cattle. J. Anim. Sci. 83, 2572–2579. doi: 10.2527/2005.83112572x
Castagnino, P. D. S., Messana, J. D., Fiorentini, G., De Jesus, R., Vito, E. S., Carvalho, I., et al. (2015). Glycerol combined with oils did not limit biohydrogenation of unsaturated fatty acid but reduced methane production in vitro. Anim. Feed Sci. Technol. 201, 14–24. doi: 10.1016/j.anifeedsci.2014.12.004
Castillejos, L., Calsamiglia, S., Ferret, A., and Losa, R. (2005). Effects of a specific blend of essential oil compounds and the type of diet on rumen microbial fermentation and nutrient flow from a continuous culture system. Anim. Feed Sci. Technol. 119, 29–41. doi: 10.1016/j.anifeedsci.2004.12.008
Castillo-Lopez, E., Rivera-Chacon, R., Ricci, S., Petri, R. M., Reisinger, N., and Zebeli, Q. (2021). Short-term screening of multiple phytogenic compounds for their potential to modulate chewing behavior, ruminal fermentation profile, and pH in cattle fed grain-rich diets. J. Dairy Sci. 104, 4271–4289. doi: 10.3168/jds.2020-19521
Cheng, J., Zhang, Y., Ge, Y., Li, W., Cao, Y., Qu, Y., et al. (2020). Sodium butyrate promotes milk fat synthesis in bovine mammary epithelial cells via GPR41 and its downstream signalling pathways. Life Sci. 259:118375. doi: 10.1016/j.lfs.2020.118375
Demirtaş, A. (2020). Determination the effects of capsaicin on the growth of pure cultures of rumen bacteria. J. Istanbul Vet. Sci. 33.
Ding, G., Chang, Y., Zhao, L., Zhou, Z., Ren, L., and Meng, Q. (2014). Effect of Saccharomyces cerevisiae on alfalfa nutrient degradation characteristics and rumen microbial populations of steers fed diets with different concentrate-to-forage ratios. J. Anim. Sci. Biotechol. 5, 1–9. doi: 10.1186/2049-1891-5-24
Eihvalde, I., Eihvalde, D., and Sematovica, I. (2016). Long-term continuous monitoring of ruminal ph and temperature for dairy cows with indwelling and wireless data transmitting unit. Parameters 3555:4829.
Fandiño, I., Calsamiglia, S., Ferret, A., and Blanch, M. (2008). Anise and capsicum as alternatives to monensin to modify rumen fermentation in beef heifers fed a high concentrate diet. Anim. Feed Sci. Technol. 145, 409–417. doi: 10.1016/j.anifeedsci.2007.04.018
Fandiño, I., Ferret, A., and Calsamiglia, S. (2020). Dose and combinations of anise oil and capsicum oleoresin as rumen fermentation modifiers in vitro and in vivo with high concentrate diets fed to Holstein beef heifers. Anim. Feed Sci. Technol. 260:114363. doi: 10.1016/j.anifeedsci.2019.114363
Firkins, J., Yu, Z., and Morrison, M. (2007). Ruminal nitrogen metabolism: perspectives for integration of microbiology and nutrition for dairy. J. Dairy Sci. 90, E1–E16. doi: 10.3168/jds.2006-518
Geron, L. J. V., de Souza, A. L., Zanin, S. F. P., de Aguiar, S. C., de Souza Santos, I., da Silva, R. F., et al. (2019). Pepper (Capsicum ssp.) as a feed additive in sheep rations using two types of inoculum: Effects on in vitro digestibility and fermentation parameters.Semina: Cienc. Agrar. 40:3653. doi: 10.5433/1679-0359.2019v40n6Supl3p3653
Ghiasi, Z., Esmaeli, F., Aghajani, M., Ghazi-Khansari, M., Faramarzi, M. A., and Amani, A. (2019). Enhancing analgesic and anti-inflammatory effects of capsaicin when loaded into olive oil nanoemulsion: an in vivo study. Int. J. Pharm. 559, 341–347. doi: 10.1016/j.ijpharm.2019.01.043
Grazziotin, R., Halfen, J., Rosa, F., Schmitt, E., Anderson, J., Ballard, V., et al. (2020). Altered rumen fermentation patterns in lactating dairy cows supplemented with phytochemicals improve milk production and efficiency. J. Dairy Sci. 103, 301–312. doi: 10.3168/jds.2019-16996
Idris, M., Uddin, J., Sullivan, M., McNeill, D. M., and Phillips, C. J. (2021). Non-invasive physiological indicators of heat stress in cattle. Animals 11:71. doi: 10.3390/ani11010071
Karadas, F., Pirgozliev, V., Rose, S., Dimitrov, D., Oduguwa, O., and Bravo, D. (2014). Dietary essential oils improve the hepatic antioxidative status of broiler chickens. Br. Poult. Sci. 55, 329–334. doi: 10.1080/00071668.2014.891098
Kroscher, K. A., Fausnacht, D. W., McMillan, R. P., El-Kadi, S. W., Wall, E. H., Bravo, D. M., et al. (2022). Supplementation with artificial sweetener and capsaicin alters metabolic flexibility and performance in heat-stressed and feed-restricted pigs. Anim. Sci. J. 100:skac195. doi: 10.1093/jas/skac195
Kumar, S., Indugu, N., Vecchiarelli, B., and Pitta, D. W. (2015). Associative patterns among anaerobic fungi, methanogenic archaea, and bacterial communities in response to changes in diet and age in the rumen of dairy cows. Front. Microbiol. 6:781. doi: 10.3389/fmicb.2015.00781
Lees, A., Lees, J., Lisle, A., Sullivan, M., and Gaughan, J. (2018). Effect of heat stress on rumen temperature of three breeds of cattle. Int. J. Biometeorol. 62, 207–215. doi: 10.1007/s00484-017-1442-x
Lees, A. M., Sejian, V., Lees, J., Sullivan, M., Lisle, A., and Gaughan, J. (2019). Evaluating rumen temperature as an estimate of core body temperature in Angus feedlot cattle during summer. Int. J. Biometeorol. 63, 939–947. doi: 10.1007/s00484-019-01706-0
Li, Y., Meng, Z., Xu, Y., Shi, Q., Ma, Y., Aung, M., et al. (2021). Interactions between anaerobic fungi and methanogens in the rumen and their biotechnological potential in biogas production from lignocellulosic materials. Microorganisms 9:190. doi: 10.3390/microorganisms9010190
Liu, Z., Ezernieks, V., Wang, J., Arachchillage, N. W., Garner, J., Wales, W., et al. (2017). Heat stress in dairy cattle alters lipid composition of milk. Sci. Rep. 7, 1–10. doi: 10.1038/s41598-017-01120-9
Mahmood, M., Petri, R. M., Gavrău, A., Zebeli, Q., and Khiaosa-ard, R. (2020). Betaine addition as a potent ruminal fermentation modulator under hyperthermal and hyperosmotic conditions in vitro. J. Sci. Food Agric. 100, 2261–2271. doi: 10.1002/jsfa.10255
Mavrommatis, A., Skliros, D., Sotirakoglou, K., Flemetakis, E., and Tsiplakou, E. (2021a). The effect of forage-to-concentrate ratio on Schizochytrium spp.-supplemented goats: modifying rumen microbiota. Animals 11:2746. doi: 10.3390/ani11092746
Mavrommatis, A., Sotirakoglou, K., Kamilaris, C., and Tsiplakou, E. (2021b). Effects of inclusion of Schizochytrium spp. and forage-to-concentrate ratios on goats’ Milk quality and oxidative status. Foods 10:1322. doi: 10.3390/foods10061322
Moss, A. R., Jouany, J.-P., and Newbold, J. (2000). Methane production by ruminants: its contribution to global warming. Ann. zootech. 49, 231–253.
Nasrollahi, S., Zali, A., Ghorbani, G., Khani, M., Maktabi, H., and Beauchemin, K. (2019). Effects of increasing diet fermentability on intake, digestion, rumen fermentation, blood metabolites and milk production of heat-stressed dairy cows. Animal 13, 2527–2535. doi: 10.1017/S1751731119001113
Natel, A. S., Abdalla, A. L., de Araujo, R. C., McManus, C., Paim, T. D. P., de Abdalla Filho, A. L., et al. (2019). Encapsulated nitrate replacing soybean meal changes in vitro ruminal fermentation and methane production in diets differing in concentrate to forage ratio. Anim. Sci. J. 90, 1350–1361. doi: 10.1111/asj.13251
Oh, J., Giallongo, F., Frederick, T., Pate, J., Walusimbi, S., Elias, R., et al. (2015). Effects of dietary capsicum oleoresin on productivity and immune responses in lactating dairy cows. J. Dairy Sci. 98, 6327–6339. doi: 10.3168/jds.2014-9294
O’Herrin, S. M., and Kenealy, W. R. (1993). Glucose and carbon dioxide metabolism by Succinivibrio dextrinosolvens. Appl. Environ. Microbiol. 59, 748–755. doi: 10.1128/aem.59.3.748-755.1993
Patcharatrakul, T., Kriengkirakul, C., Chaiwatanarat, T., and Gonlachanvit, S. (2020). Acute effects of red chili, a natural capsaicin receptor agonist, on gastric accommodation and upper gastrointestinal symptoms in healthy volunteers and gastroesophageal reflux disease patients. Nutrients 12:3740. doi: 10.3390/nu12123740
Phesatcha, K., Phesatcha, B., Wanapat, M., and Cherdthong, A. (2021). The effect of yeast and roughage concentrate ratio on ruminal pH and protozoal population in Thai native beef cattle. Animals 12:53. doi: 10.3390/ani12010053
Ranathunga, S. D., Kalscheur, K. F., and Herrick, K. J. (2019). Ruminal fermentation, kinetics, and total-tract digestibility of lactating dairy cows fed distillers dried grains with solubles in low-and high-forage diets. J. Dairy Sci. 102, 7980–7996. doi: 10.3168/jds.2018-15771
Russell, J. B., and Strobel, H. (1989). Effect of ionophores on ruminal fermentation. Appl. Environ. Microbiol. 55, 1–6. doi: 10.1128/aem.55.1.1-6.1989
Sales, G. F. C., Carvalho, B. F., Schwan, R. F., de Figueiredo Vilela, L., Meneses, J. A. M., Gionbelli, M. P., et al. (2021). Heat stress influence the microbiota and organic acids concentration in beef cattle rumen. J. Therm. Biol. 97:102897. doi: 10.1016/j.jtherbio.2021.102897
Shiiba, Y., Takeda, K. I., and Matsushima, K. (2021). Effects of adding a commercial capsaicin fertilizer on the feeding behavior of sika deer (Cervus nippon). Anim. Sci. J. 92:e13529. doi: 10.1111/asj.13529
Soltan, Y., Morsy, A., Hashem, N., and Sallam, S. (2021). Boswellia sacra resin as a phytogenic feed supplement to enhance ruminal fermentation, milk yield, and metabolic energy status of early lactating goats. Anim. Feed Sci. Technol. 277:114963. doi: 10.1016/j.anifeedsci.2021.114963
Sullivan, M. L., Wijffels, G., George, A., Al-Hosni, Y. A., Olm, J. C., and Gaughan, J. B. (2022). Elliptical and linear relationships with rumen temperature support a homeorhetic trajectory for DMI during recovery of feedlot cattle exposed to moderate heat load. Anim. Sci. J. 100:skac127. doi: 10.1093/jas/skac127
Szolcsányi, J. (2015). Effect of capsaicin on thermoregulation: an update with new aspects. Temperature 2, 277–296. doi: 10.1080/23328940.2015.1048928
Tager, L., and Krause, K. (2010). Effects of cinnamaldehyde, eugenol, and capsicum on fermentation of a corn-based dairy ration in continuous culture. Can. J. Anim. Sci. 90, 413–420. doi: 10.4141/CJAS09080
Temmar, R., Rodríguez-Prado, M., Forgeard, G., Rougier, C., and Calsamiglia, S. (2021). Interactions among natural active ingredients to improve the efficiency of rumen fermentation in vitro. Animals 11:1205. doi: 10.3390/ani11051205
Tian, H., Wang, W., Zheng, N., Cheng, J., Li, S., Zhang, Y., et al. (2015). Identification of diagnostic biomarkers and metabolic pathway shifts of heat-stressed lactating dairy cows. J. Proteome 125, 17–28. doi: 10.1016/j.jprot.2015.04.014
Uyeno, Y., Sekiguchi, Y., Tajima, K., Takenaka, A., Kurihara, M., and Kamagata, Y. (2010). An rRNA-based analysis for evaluating the effect of heat stress on the rumen microbial composition of Holstein heifers. Anaerobe 16, 27–33. doi: 10.1016/j.anaerobe.2009.04.006
Wang, Z., Liu, L., Pang, F., Zheng, Z., Teng, Z., Miao, T., et al. (2022). Novel insights into heat tolerance using metabolomic and high-throughput sequencing analysis in dairy cows rumen fluid. Animal 16:100478. doi: 10.1016/j.animal.2022.100478
Weatherburn, M. (1967). Phenol-hypochlorite reaction for determination of ammonia. Anal. Chem. 39, 971–974. doi: 10.1021/ac60252a045
Yadav, B., Singh, G., Verma, A., Dutta, N., and Sejian, V. (2013). Impact of heat stress on rumen functions. Vet. World 6, 992–996. doi: 10.14202/vetworld.2013.992-996
Zhu, W., Wei, Z., Xu, N., Yang, F., Yoon, I., Chung, Y., et al. (2017). Effects of Saccharomyces cerevisiae fermentation products on performance and rumen fermentation and microbiota in dairy cows fed a diet containing low quality forage. J. Anim. Sci. Biotechol. 8, 1–9. doi: 10.1186/s40104-017-0167-3
Keywords: capsicum oleoresin, hyperthermal stress, dietary conditions, rumen fermentation, microbial abundance
Citation: An Z, Luo G, Abdelrahman M, Riaz U, Gao S, Yao Z, Ye T, Lv H, Zhao J, Chen C and Yang L (2022) Effects of capsicum oleoresin supplementation on rumen fermentation and microbial abundance under different temperature and dietary conditions in vitro. Front. Microbiol. 13:1005818. doi: 10.3389/fmicb.2022.1005818
Edited by:
Kun Li, Nanjing Agricultural University, ChinaReviewed by:
Montaser Elsayed Ali, Al-Azhar University, EgyptAhmed Ahmed, King Khalid University, Saudi Arabia
Copyright © 2022 An, Luo, Abdelrahman, Riaz, Gao, Yao, Ye, Lv, Zhao, Chen and Yang. This is an open-access article distributed under the terms of the Creative Commons Attribution License (CC BY). The use, distribution or reproduction in other forums is permitted, provided the original author(s) and the copyright owner(s) are credited and that the original publication in this journal is cited, in accordance with accepted academic practice. No use, distribution or reproduction is permitted which does not comply with these terms.
*Correspondence: Liguo Yang, yangliguo2006@qq.com
†These authors have contributed equally to this work