- 1College of Veterinary Medicine, Sichuan Agricultural University, Chengdu, China
- 2Sichuan Key Laboratory of Conservation Biology for Endangered Wildlife, Chengdu Research Base of Giant Panda Breeding, Sichuan Academy of Giant Panda, Chenghua, China
Multi-drug-resistant Klebsiella pneumoniae (MDR K. pneumonia) is increasingly being reported with corresponding increase in morbidity and mortality all over the world. However, limited information is available concerning MDR K. pneumonia in giant pandas. The objective of this study was to grasp the drug resistance profile of MDR K. pneumonia isolated from giant pandas. A total of 182 K. pneumoniae isolates were collected from fresh feces of 94 captive giant pandas of different ages and sex and separated by season. We performed a standard disk diffusion antimicrobial susceptibility test with the isolates and further evaluated the antibiotic resistance genes (ARGs) of multi-drug-resistant strains by high-throughput quantitative PCR. In addition, we then analyzed mobile genetic elements (MGEs), integron gene cassettes, and the multi-locus sequence typing of multi-drug-resistant strains by PCR. Antimicrobial susceptibility testing results demonstrated that a total of 30 (16.5%) K. pneumoniae isolates showed multiple drug resistances. The thirty MDR K. pneumonia isolates were mainly resistant to amoxicillin (100.0%), doxycycline (86.7%), chloramphenicol (60.0%), compound trimethoprim (60.0%) and trimethoprim (56.7%). Fifty different types of antibiotic resistance genes were found, which included a total of 671 antibiotic resistance genes, in the 30 multi-drug-resistant isolates. The top ten resistance genes were: vanTC-02, aacC, blaCTX-M-04, blaSHV-01, blaSHV-02, ampC-04, blaOXY, tetD, blaTEM and tetA-02. Thirteen mobile genetic elements were detected, of which IS26 (96.67%) and intI1 (96.67%) had the highest frequency. The thirty MDR K. pneumonia isolates were negative for the traA, traF, tnsA, IS1133, ISpa7, ISkpn6, intI2 and intI3 genes. Moreover, a further investigation of integrons revealed that two types of specific gene cassettes (dfrA12 + orfF + aadA2 and dfrA12 + orfF) were identified in class 1 integrons. Multi-locus sequence typing results showed that 22 STs in the thirty MDR K. pneumonia isolates were identified, the main type was ST37 (5/30). Our results illustrate that effective surveillance and strict biosecurity strategies should be taken to prevent the spread of multi-drug-resistant bacteria, and monitor the emergence of mobile genetic elements and integrons.
Introduction
Klebsiella Pneumonia is a gram-negative Enterobacteria, and it is also an opportunistic pathogen responsible for an important proportion of nosocomial infections in human. Multi-drug-resistant Klebsiella pneumoniae (MDR K. pneumonia) was defined as being resistant to three or more antimicrobials (Dolejska et al., 2007; Magiorakos et al., 2012), and is increasingly being reported with corresponding increase in morbidity and mortality. MDR K. pneumoniae was more and more prevalent in the tertiary teaching hospital in western Kenya from 2002 to 2013 (Apondi et al., 2016), and MDR K. pneumoniae was an increasing cause of neonatal infections in India and other developing countries, with 60.86% of MDR K. pneumoniae infections leading to death (Jeena et al., 2001). Furthermore, researchers have detected MDR K. pneumoniae isolated from raw milk from dairy farms in Jiangsu and Shandong provinces in China recent years, which was a potential threat to food safety and public health (Yang et al., 2021).
Antimicrobial abuse is a serious risk for the emergence of MDR K. pneumoniae. In addition, horizontal gene transfer (HGT) via mobile genetic elements (MGEs) such as integrons, transposons, integrative conjugative elements, genomic islands, and plasmids are another important reason for the spread of antibiotic resistance genes (ARGs) carried by MDR K. pneumonia (Partridge et al., 2009; Chen et al., 2016). Integrons can capture, convert and adapt one or more resistance gene cassettes into functionally expressed genes via a self-efficient gene expression system. Meanwhile it can also simply transfer these genes between diverse bacterial species due to their linkage with plasmids (Gillings, 2014). Class 1, 2, and 3 integrons are the three main types of MGEs associated with antimicrobial resistance (Kaushik et al., 2018).
The giant panda (Ailuropoda melanoleuca) is one of the world’s most recognized and rarest animals and is now only distributed in the mountainous areas of Sichuan, Gansu, and Shanxi provinces in China (Wang et al., 2018). Giant pandas infected with K. pneumoniae have developed hemorrhagic enteritis, and died from hemorrhagic sepsis and subsequent cases of genital hematuria, and sepsis. Infection of K. pneumoniae has increased within the captive population of giant pandas (Wang et al., 2006; Sun et al., 2013; Lu et al., 2015), generally causes mixed infection with other bacteria, and has become one of the most important pathogens of captive pandas (Peng and Xiong, 2000). According to our investigation, for the past several decades, antibiotics were widely applied to prevent and treat bacterial infectious diseases in giant pandas (Guo L. et al., 2015; Yang et al., 2017), which may have led to the emergence of antibiotic resistant bacteria (ARB) and even multi-drug-resistant (MDR) bacteria. Our previous studies have confirmed the prevalence of drug-resistance in K. pneumoniae in captive giant pandas (Su et al., 2021). However, MDR K. pneumoniae isolates from giant pandas have gained little attention and characterization so far. To the best of our knowledge, this is the first report showing the baseline characteristics of antimicrobial resistance and integron gene cassettes in K. pneumoniae isolates from captive giant pandas. The study aims to characterize the antimicrobial resistance of 182 K. pneumoniae isolates collected from the feces of giant pandas of different ages and sex separated by season, and to study the ARGs and integron gene cassettes and other MGEs in 30 MDR K. pneumonia, and further analyze the impact of age, sex and season on the antibiotic resistance of 30 MDR K. pneumonia. This study will help improve the clinical treatment and ex situ conservation of giant pandas.
Materials and Methods
Samples
To investigate the prevalence of the antimicrobial resistance of K. pneumoniae isolates, 376 fresh fecal samples were collected from 94 captive giant pandas (female: n = 47, male: n = 47) housed at the Chengdu Research Base of Giant Panda Breeding (CRBGP) in Sichuan, China during 2020. Four fecal samples were collected from each individual giant panda during each season: spring (March to May), summer (June to August), autumn (September to November) and winter (December to February). All animals were considered healthy at the time and were managed under routine CRBGP husbandry practices. A total of 182 K. pneumonia isolates were identified from the fecal samples by Gram staining, 16s rDNA, and bacterial biochemical identification. Isolates were separated and analyzed by the sex of the panda (female samples: n = 107, male samples: n = 75), the season of sampling (spring: n = 62, summer: n = 80, autumn: n = 29 and winter: n = 11), and divided into three age groups [sub-adult (aged 1.5–5 years): n = 67, adult (aged 5–19 years): n = 92, and geriatric (aged 20 years or older): n = 23]. Confirmed K. pneumonia strains were stored in Luria-Bertani (LB) broth containing 20% glycerol at –80°C for further analyses.
Antimicrobial Susceptibility Test of the Klebsiella pneumonia Isolates
The susceptibilities of all isolates to 24 antimicrobials were tested using the standard disk diffusion method recommended by the (Clinical and Laboratory Standards Institute [CLSI], 2020). The following seven classes of antibiotics of antimicrobial disks were used (Hangzhou Microbial Reagent Co., Ltd., China): β-lactams: piperacillin (PIC, 100 μg), amoxicillin (AML, 20 μg), cefotaxime (CTX, 30 μg), cefazolin (CEZ, 30 μg), ceftriaxone (CRO, 30 μg), cefuroxim (CXM, 30 μg), cefaclor (CEC, 30 μg), ampicillin/sulbactam 1:1 (SAM, 10/10 μg), aztreonam (AZM, 30 μg), meropenem (MEM, 10 μg), imipenem (IPM, 10 μg); Aminoglycosides: kanamycin (KAN, 30 μg), gentamicin (GM, 10 μg), streptomycin (STR, 10 μg); Quinolones: ofloxacin (OFX, 5 μg), norfloxacin (NOR, 10 μg), levofloxacin (LEV, 5 μg), ciprofloxacin (CIP, 5 μg); Chloramphenicol: chloramphenicol (CHL, 30 μg); Macrolides: azithromycin (AZM, 15 μg); Tetracyclines: doxycycline (DOX, 30 μg), minocycline (MH, 30 μg); Sulfonamides: compound trimethoprim (SXT, 23.75/1.25), trimethoprim (TMP, 5 μg). Results were interpreted in accordance with (Clinical and Laboratory Standards Institute [CLSI], 2020) criteria. Escherichia coli ATCC25922 was used as a control. All the antibiotics selected in this study were based on the information collected from CRBGP veterinarians and recent studies on the resistance of giant pandas (Zhang et al., 2009; Guo L. et al., 2015; Chen et al., 2018; Zou et al., 2018; Zhu et al., 2020) and antibiotics commonly resistant to K. pneumonia in clinics (Gao L. et al., 2020; Gao Q. et al., 2020). MDR was defined as being resistant to three or more classes of antibiotics (Magiorakos et al., 2012; Zhu et al., 2020).
DNA Extraction and High-Throughput Quantitative PCR for Antibiotic Resistance Genes Carried by the Multi-Drug-Resistant Klebsiella pneumonia Isolates
Total genomic DNA was extracted from the MDR K. pneumonia isolates based on the results of antimicrobial susceptibility test using the TIANamp Bacteria DNA kit (Tiangen Biotech, Beijing, China) according to the manufacturer’s instructions. DNA samples were stored at –20°C.
To evaluate the abundance of ARGs in different MDR K. pneumonia isolates, high-throughput quantitative PCR (HT-qPCR) of ARGs was performed by Yearth Biotech (Changsha, China) using the SmartChip Real-time PCR (Warfergen Inc., United States) as described previously with a slight modification (Gao Q. et al., 2020). A total of 83 primer sets (including a 16S rRNA gene primer sets, not listed, see Supplementary Table 1) were used. HT-qPCR data was preprocessed as described previously, for each primer set, amplifications efficiency beyond the range (90∼110%) were discarded, and amplification was confirmed with more than two positive replicates. The relative copy number of ARGs was calculated and transformed to absolute copy numbers by normalizing to 16S rRNA gene copy numbers which were quantified separately from the Wafergen platform.
Detection of Mobile Genetic Elements Carried by the Multi-Drug-Resistant Klebsiella pneumonia Isolates
Twenty-one pairs of primers were designed to detect MGEs in the 30 MDR K. pneumoniae isolates by PCR (Table 1; Zhu et al., 2020). PCR amplification was performed in a total volume of 25 μL: 2 × Dream Taq Green PCR master mix (Thermo Fisher Scientific, United States) 12.5 μL, forward and reverse primer each 1 μL, DNA template 2 μL, and ddH2O 8.5 μL. And ddH2O was set as a negative control. The PCR reaction conditions consisted of 5 min at 95°C, followed by 30 cycles of 95°C for 30 s, annealing temperature for 30 s, 72°C for 30 s, and a final extension at 72°C for 10 min. PCR products were subjected to electrophoresis in a 1% agarose gel stained with 4S Red Pus (Sangon Biotech, Shanghai, China), visualized under ultraviolet light and photographed using a gel documentation system (Bio-Rad, Hercules, United States).
Detection of the Integrons and Associated Cassette Arrays Carried by the Multi-Drug-Resistant Klebsiella pneumonia Isolates
All the MDR K. pneumonia isolates were also screened for the presence of int11, intI2 and intI3 genes. Moreover, strains that were positive for the intl1 or intl2 or intI3 genes were then evaluated for gene cassettes by PCR (Table 1; Zou et al., 2018). The PCR amplification, PCR reaction conditions and the analyses for PCR products were the same as described in section “Detection of Mobile Genetic Elements Carried by the Multi-Drug-Resistant Klebsiella pneumonia Isolates.”
Analysis of Clonal Relationship in the Multi-Drug-Resistant Klebsiella pneumonia Isolates Based on the Multi-Locus Sequence Typing
MLST was performed to investigate the clonal relationship of the 30 MDR K. pneumonia isolates by amplifying the seven standard housekeeping loci gapA, infB, mdh, pgi, phoE, rpoB, and tonB as described previously (Guo C. et al., 2015). The eBURST software was used to construct an evolutionary relationship map by analyzing the data of allelic sequence obtained in this study. That six of the seven sites were same was defined as a clone group. A type group containing 4 or more ST types was defined as a clonal complex (CC), of which one ST type was calculated to be the origin ST type, The other ST types was evolved based on the origin ST type. Compared with the original ST type, single-locus variant (SLV) has one different housekeeping gene locus. The single type does not belong to any clonal complex.
Sequence and Data Analysis
All positive PCR products (MGEs, integrons and MLST) were sequenced by Sangong Biotech (Shanghai, China) in both directions. Sequences of MGEs and gene cassettes were analyzed online using BLAST,1 and sequences of MLST were analyzed online using MLST.2
Statistical Analysis
Data were analyzed by a χ2-test using SPSS Statistics version 18.0. This test was used to compare the isolation rate of MDR K. pneumonia by sex in different age groups of the giant pandas by season. The difference of the number of antimicrobial resistances in MDR K. pneumonia strains from the individual giant pandas by sex, age and season groupings were tested with the “wilcox_test.” function in R package “rstatix.” The correlation between the antimicrobial resistant levels of MDR K. pneumonia and traits of the giant pandas by sex, age and season groupings using the Fisher’s test with “fisher. test” in “stats” R package. Differences among groups were considered significant at P < 0.05.
Results
The Isolation of the Multi-Drug-Resistant Klebsiella pneumonia Strains
A total of 182 K. pneumonia strains were isolated from the feces of captive giant pandas showing different degrees of resistance to 24 antimicrobials. Thirty strains which were identified as K1∼K30 were found to be multidrug resistant (MDR) (not listed, see Supplementary Table 2). The isolation rate of MDR K. pneumonia was 16.48% (30/182), of which the sub-adult group was 11.94% (8/67), adult group was 19.57% (18/92), and geriatric group was 17.39% (4/23), with the age of the giant panda having no significant effect on the isolation rate (P > 0.05). The isolation rate of MDR K. pneumonia was 16.82% (18/107) in female, and 16.00% (12/75) in male. There was no significant difference in the isolation rate based on the sex of the subject (P > 0.05); however, season had a significant effect on the isolation rate of MDR K. pneumonia (P < 0.05). The isolation rate was 14.52% (9/62) in spring, 11.25% (9/80) in summer, 24.14% (7/29) in autumn, 45.45% (5/11) in winter (Figure 1).
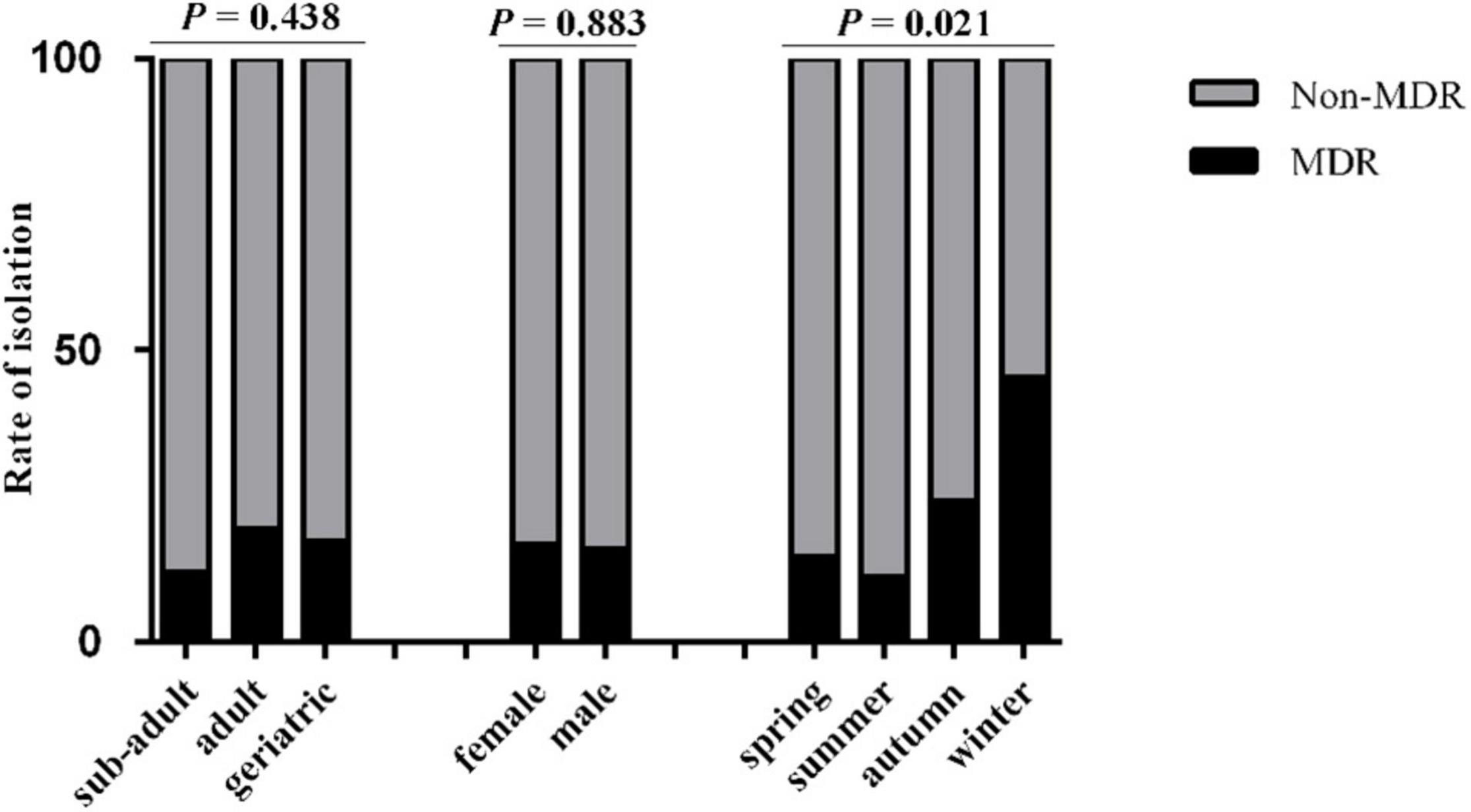
Figure 1. The rate of isolation of non-MDR and MDR K. pneumonia from giant pandas of different ages and gender in four seasons. Sub-adult: giant pandas aged 1.5–5 years; Adult: giant pandas aged 5–19 years; Geriatric: giant pandas aged 20 years or older. Spring, March to May; Summer, June to August; Autumn, September to November; Winter, December to February. Gray, the non-MDR K. pneumonia from giant pandas; Black, the MDR K. pneumonia from giant pandas. Data were analyzed by a χ2-test using SPSS Statistics version 18.0. P < 0.05: The age, sex and the season of sampling had a significant influence on the rate of isolation of non-MDR and MDR K. pneumonia from giant pandas.
The Comparison of Antimicrobial Resistances in Multi-Drug-Resistant Individual Giant Pandas
We further analyzed the effects of age, sex and season on the number of antimicrobial resistances in each MDR giant panda. It was found that the antimicrobial resistances in MDR male giant pandas was significantly higher than that of females (P < 0.05) (Figure 2A). Additionally, there was a significantly increasing trend of the number of antimicrobial resistances from spring to winter. Compared with other seasons, the antimicrobial resistance of giant pandas in winter was significantly higher (P < 0.05) (Figure 2B). However, age was not significantly correlated to the number of antimicrobial resistances (P > 0.05).
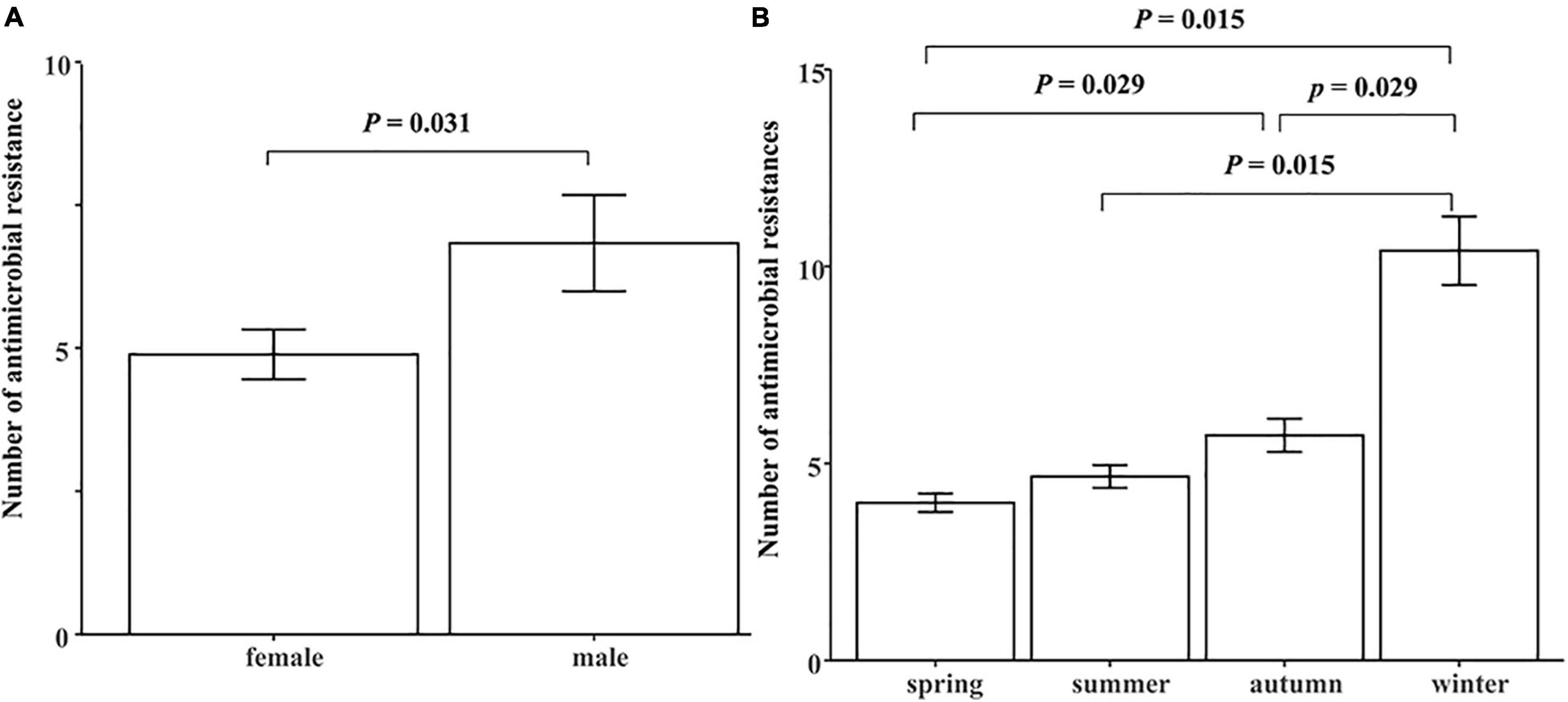
Figure 2. The comparison of number of antimicrobial resistances in 30 MDR giant pandas in different sex and season groups. (A) The effect of sex on the number of antimicrobial resistances in each MDR giant panda. (B) The effect of season on the number of antimicrobial resistances in each MDR giant panda. Spring, March to May; Summer, June to August; Autumn, September to November; Winter, December to February. Data were analyzed with the “wilcox_test.” function in R package “rstatix.” P < 0.05: The sex or the season had a significant effect on the number of antimicrobial resistances in each MDR giant panda.
The Analysis of Antimicrobial Susceptibility in the Multi-Drug-Resistant Klebsiella pneumonia Isolates
Thirty MDR K. pneumonia strains showed resistance to AML (100.00%, 30/30), DOX (86.67%, 26/30), CHL (60.00%, 18/30), SXT (60.00%, 18/30), and TMP (56.67%, 17/30); resistance to the remaining 19 antimicrobials was observed in less than 50% of the samples (Table 2). Fisher’s test showed that the antimicrobial resistance of MDR K. pneumonia strains to CRO, GM, and DOX was significantly related to age and season (P < 0.05) (Figure 3), while the remaining antimicrobials were not related to either factor (P > 0.05). Additionally, antimicrobial resistance of MDR K. pneumonia strains was not significantly correlated to sex of giant pandas (P > 0.05).
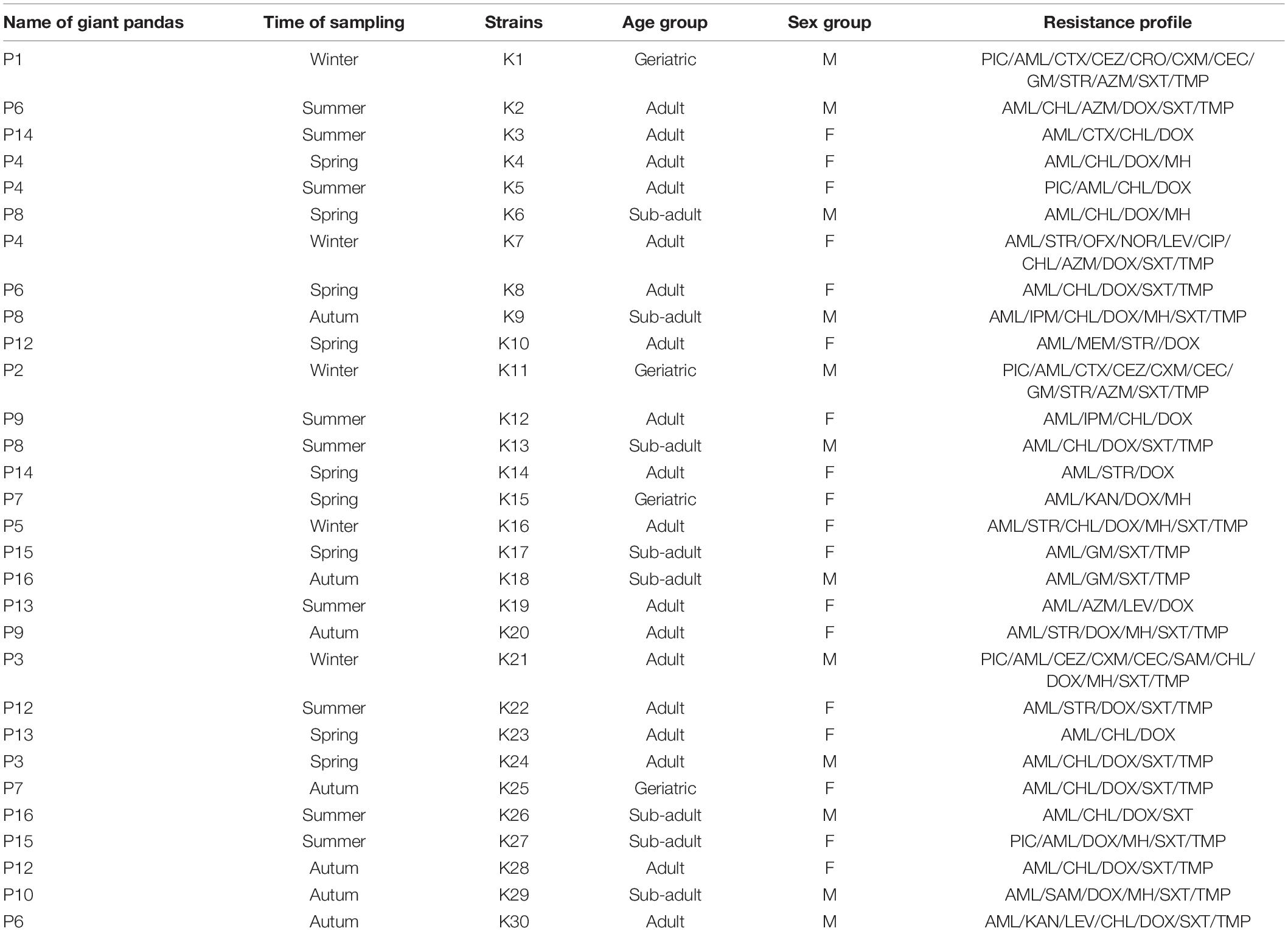
Table 2. Antimicrobial susceptibility of 30 MDR K. pneumoniae isolates from the feces of captive giant pandas in four seasons in China.
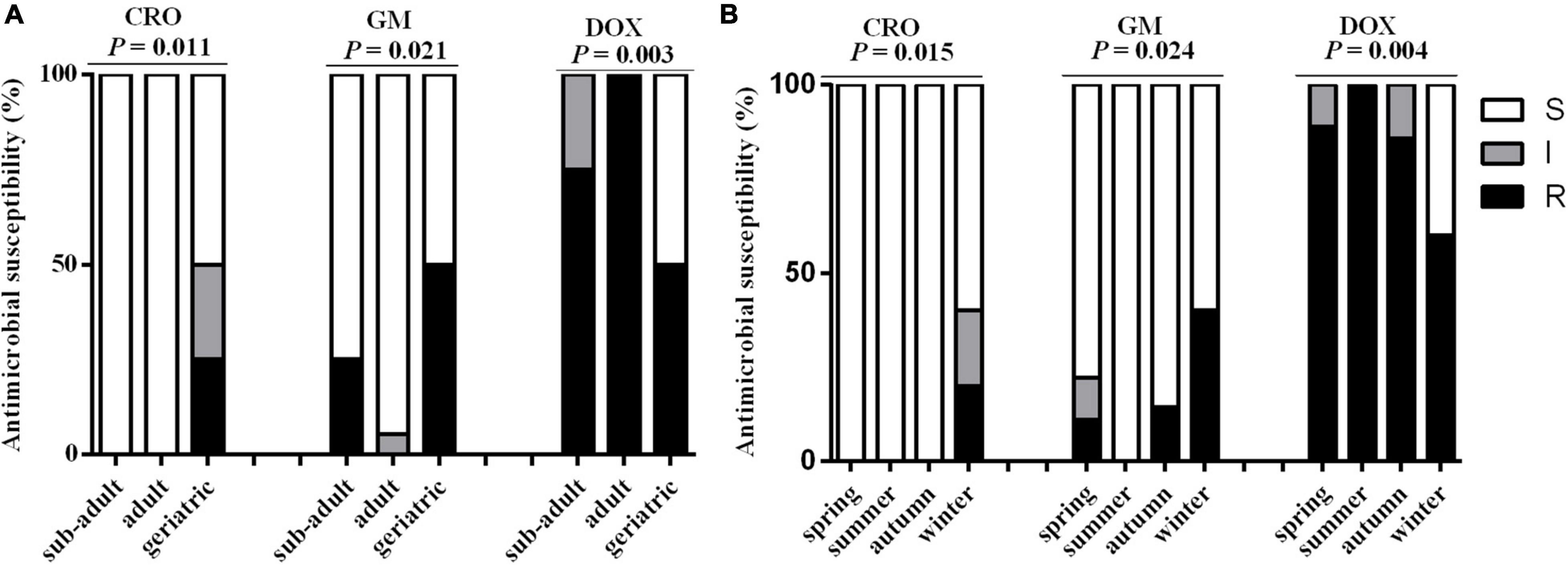
Figure 3. The comparison of antimicrobial susceptibility in the 30 MDR K. pneumonia isolates in different age and season groups. (A) The effect of age on antimicrobial susceptibility in MDR K. pneumonia isolates. Sub-adult: 1.5–5 years; Adult: 5–19 years; Geriatric: 20 years or older. (B) The effect of season on antimicrobial susceptibility in MDR K. pneumonia isolates. Spring, March to May; Summer, June to August; Autumn, September to November; Winter, December to February. CRO, ceftriaxone, GM, gentamicin, DOX, doxycycline. White, MDR K. Pneumonia isolates which were sensitive to antibiotics; Gray, MDR K. Pneumonia isolates which were intermediate to antibiotics; Dark, MDR K. Pneumonia isolates which were resistant to antibiotics. Data were analyzed with the “fisher. test” in “stats” R package. P < 0.05: The age or the season had a significant infect on antimicrobial susceptibility in MDR K. pneumonia isolates.
The Antibiotic Resistance Genes Prevalence of the Multi-Drug-Resistant Klebsiella pneumonia Isolates
We extracted DNA from the 30 MDR K. pneumoniae isolates and analyzed ARGs. Our results showed an abundance of various ARG types in the 30 MDR K. pneumoniae isolates, where a total of 50 different types of ARGs were detected, including 671 ARGs. The top ten resistance genes were: vanTC-02, aacC, blaCTX-M-04, blaSHV-01, blaSHV-02, ampC-04, blaOXY, tetD, blaTEM and tetA-02. 671 unique ARGs had potential to confer resistance against a range of antibiotics such as β-Lactamase resistance (41.58%), aminoglycosides resistance (22.21%), vancomycin resistance (11.18%), tetracycline resistance genes (18.93%), sulfonamide resistance (6.11%) (Figure 4).
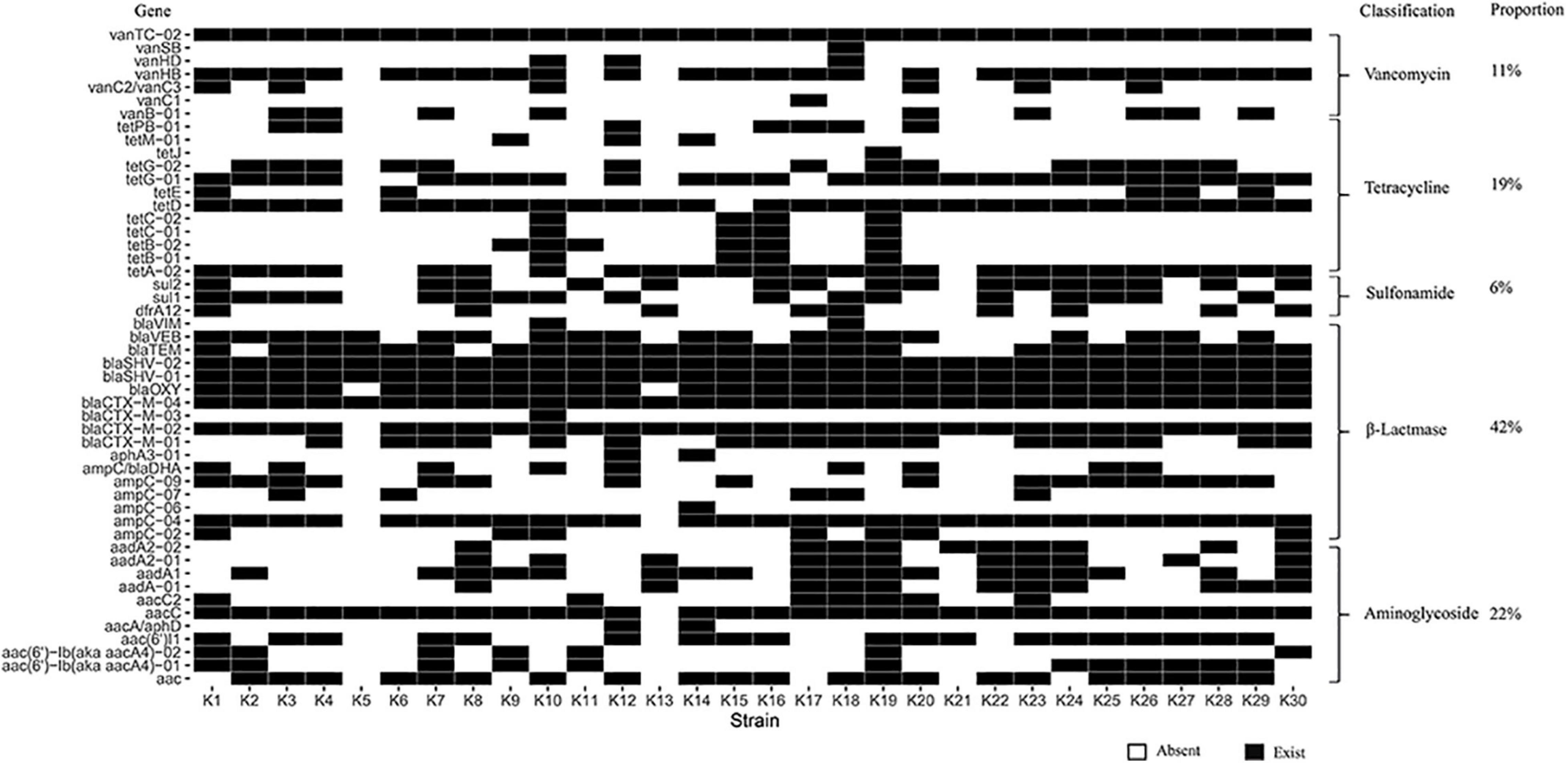
Figure 4. Heat-map for the ARGs in the 30 MDR K. pneumonia isolated from the feces of giant pandas. Columns: K1∼K30 mean 30 MDR K. pneumoniae isolates. Rows: 50 different types of ARGs, the classification and proportion. White: The K. pneumoniae isolate which did not carry the ARG; Black: The K. pneumoniae isolate which carried the ARG.
The Mobile Genetic Elements Prevalence of the Multi-Drug-Resistant Klebsiella pneumonia Isolates
We extracted DNA from the 30 MDR K. pneumoniae isolates and analyzed MGEs. The results showed that 13 MGEs were detected among the 30 MDR isolates: IS26 (96.67%, n = 29), intI1 (96.67%, n = 29), tnpU (93.33%, n = 28), tnpA/Tn21 (93.33%, n = 28), IS903 (83.33%, n = 25), tbrC (36.67%, n = 11), ISEcp1 (30.00%, n = 9), merA (20.00%, n = 6), tnp513 (10.00%, n = 3), ISkpn7 (10.00%, n = 3), ISaba1 (10.00%, n = 3), ISCR3/14 (6.67%, n = 2), and ISCR1 (3.33%, n = 1). All isolates were negative for the traA, traF, tnsA, IS1133, ISpa7, ISkpn6, intI2, and intI3 genes (Table 3).
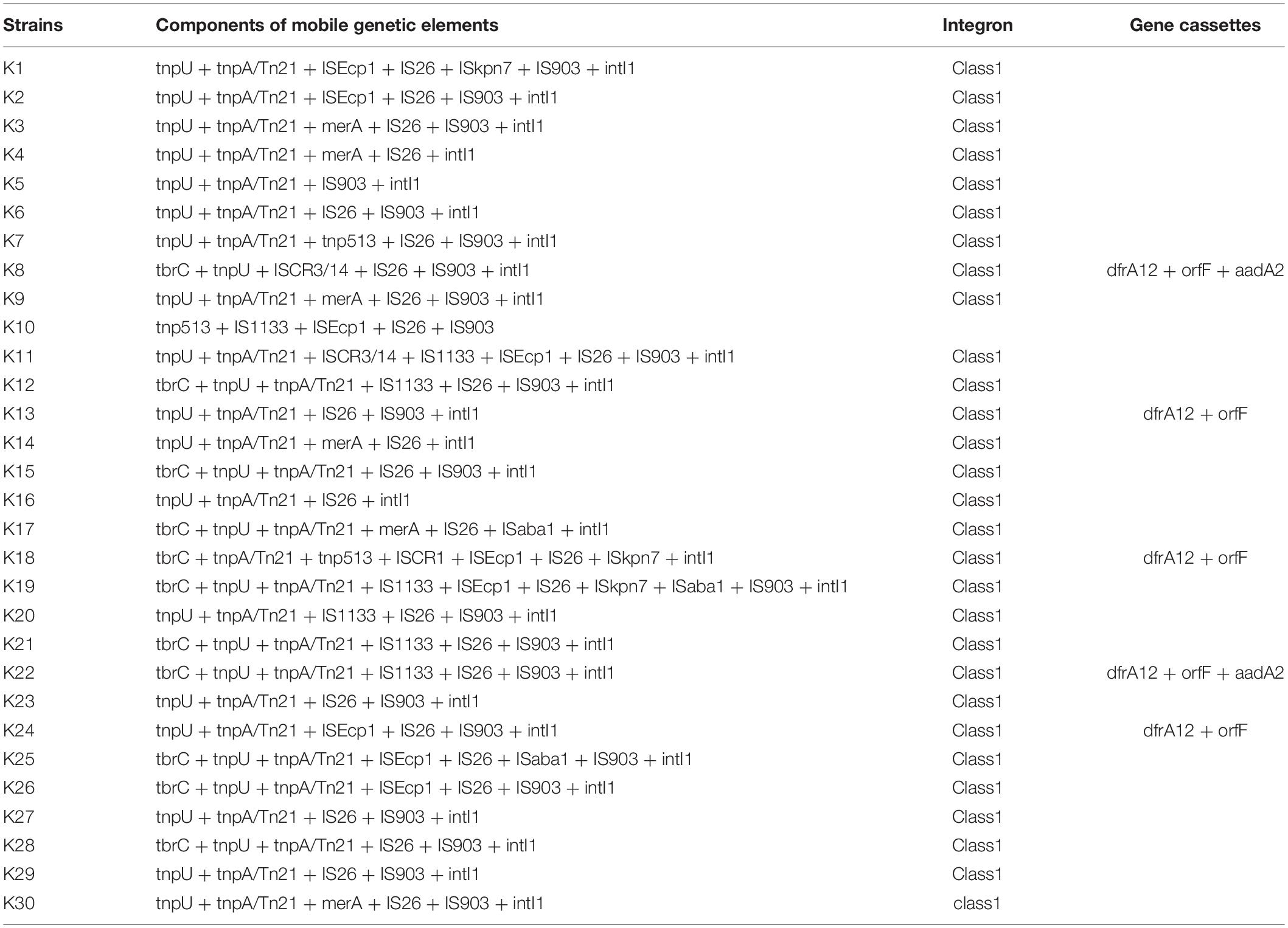
Table 3. MGEs and integron gene cassettes in 30 MDR K. pneumoniae isolates from the feces of captive giant pandas in China.
The Characterization of Integron Types and Gene Cassettes Carried by the Multi-Drug-Resistant Klebsiella pneumonia Isolates
We further studied integrons in the 30 MDR K. pneumoniae isolates: 96.67% (29 isolates) were positive for the intI1 genes, while intI2 and intI3 were not detected. We further analyzed antimicrobial resistance related to gene cassettes in the 29 intI1-positive isolates. The results showed that only in five strains were the drug resistance cassettes detected (dfrA12 + orfF + aadA2 in 2 isolates, and dfrA12 + orfF in 3 isolates) from the 29 intI1-positive isolates (Table 3).
The Multi-Locus Sequence Typing Characteristics of the Multi-Drug-Resistant Klebsiella pneumonia Isolates
We use MLST typing to understand the clonal relationship of the 30 MDR K. pneumonia strains isolated between different giant pandas. Twenty-two STs were identified, of which five strains belonged to ST37, three strains belonged to ST17, and three strains belonged to ST1558. Clonal Structure analysis through eBURST software showed that thirty MDR K. pneumoniae isolates were highly diverse, but ST37 had two SLVs (Figure 5).
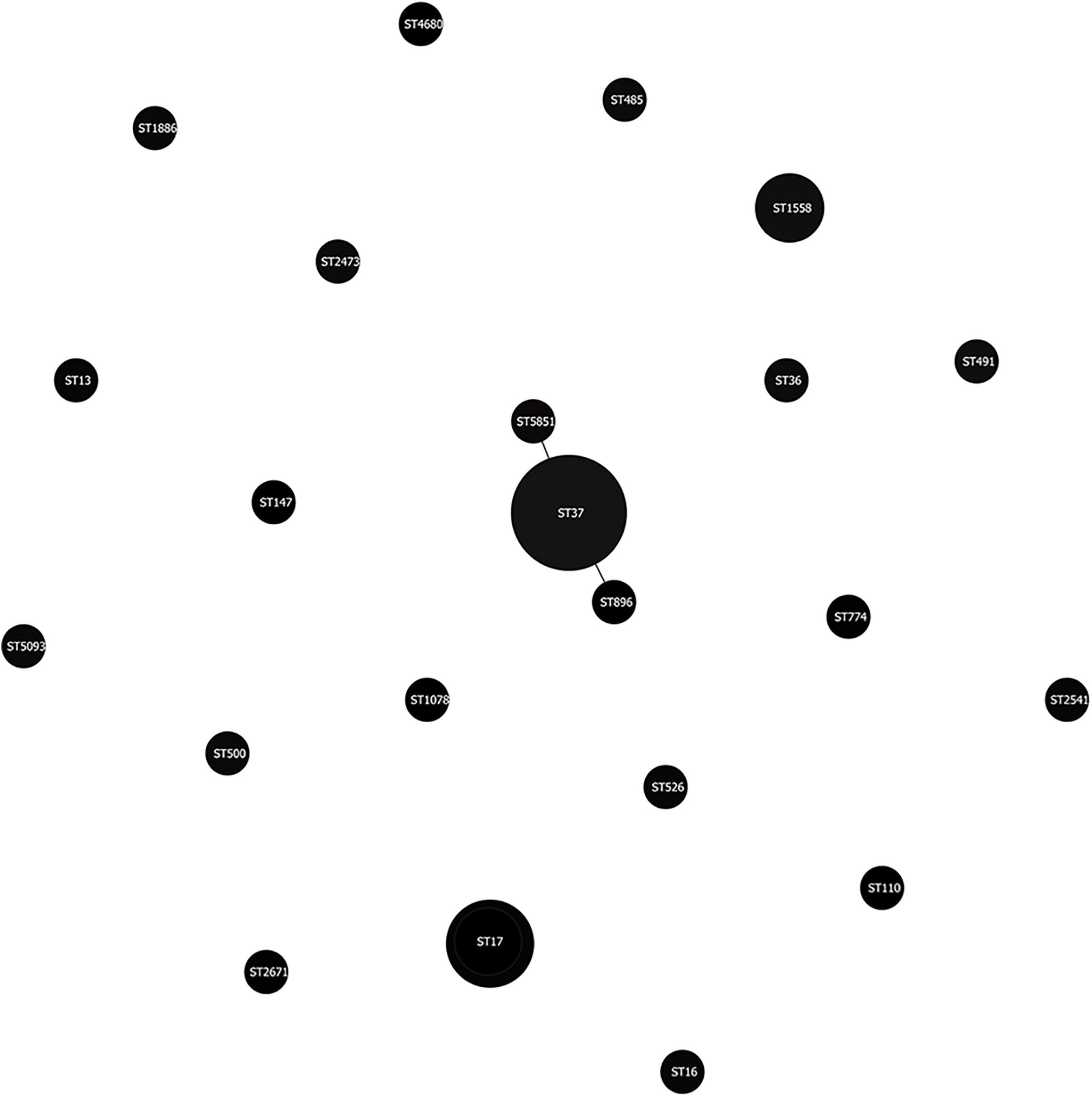
Figure 5. MLST-based clonal Structure of the 30 MDR K. pneumonia isolates from the feces of giant pandas. The scheme was constructed using eBURST analysis. STs were symbolized by dots, the size of a dot corresponded to the number of isolates belonging to an ST. SLVs (single-locus variant: has one different housekeeping gene locus compared with the original ST type) were linked by solid lines.
Discussion
MDR K. pneumoniae was first reported in the United States, followed by Europe, South America, and Asia (Winokur et al., 2001). The present study is the first to detect MDR K. pneumonia isolated from captive giant pandas, with an isolation rate of 16.48%. At the same time, our data illustrated that the isolation rate of MDR K. pneumonia was not significantly related to the age or sex of the giant panda. The isolation rate of MDR K. pneumonia was found to be 30–70% in a hospital located in Central China (Guo et al., 2017), which was higher than our study in giant pandas. The reason for the lower isolation rate of MDR K. pneumonia in giant pandas may be explained by the possibility that antibiotics were used less frequently to treat giant pandas, in addition, K. pneumoniae strains in the study were isolated from feces of healthy giant pandas, while K. pneumoniae strains in other researches were isolated from hospital materials.
In this study, MDR K. pneumonia strains showed resistance to AMX (100.00%), DOX (86.67%), CHL (60.00%), SXT (60.00%), and TMP (56.67%). Zhu et al. (2020) also found E. coli strains isolated from feces of giant pandas showed resistance to AMX, DOX, CHL, and SXT, but the resistance rates of these antibiotics were all lower than 50%. Our resistance rates to AMX, DOX, CHL, and SXT were higher than those of E. coli isolated from captive giant pandas. The antibiotics in our study were mainly distributed in β-lactams and tetracyclines, which was consistent with previous studies on E. coli (Zhu et al., 2020). We further found some strains were resistant to more than 10 antibiotics in this study, even though CHL was banned for animals, resistance to CHL was observed in 60% of the MDR strains. For these reasons, it is important to be prudent in the use of antimicrobials with giant pandas, and the supervision of antimicrobial resistance in isolates from giant pandas can be useful in choosing antibiotics for preventing K. pneumonia infections. In addition, our results also found that among the 24 antibiotics, the drug resistance rates of CRO, GM, and DOX were significantly related to the age of the giant panda. The possible reason was that the disease susceptibility of giant pandas differ by age, and the selections of antibiotics for clinical treatment were also different. At the same time, we found that the antimicrobial susceptibility of MDR K. pneumonia isolated from the geriatric groups of giant pandas to CRO and GM was significantly lower than that of the adult groups and sub-adult groups, which indicated that more attention should be paid to the rational use of antibiotics for sick elderly pandas.
HT-qPCR enables a rapid and sensitive quantification of a number of ARGs, conferring resistance to almost all major classes of antibiotics and providing comprehensive profiles of ARGs (Su et al., 2015). In this study, fifty different types of ARGs were found, which included a total of 671 ARGs, which mainly belonged to β-Lactamase (41.58%), which was consistent with the findings of Hu et al. (2021). At the same time, the results of antimicrobial susceptibility test showed that the MDR isolates also had high resistance to β-lactam antibiotics, especially the resistance rate to AML, which was 100%. The resistance mechanism of K. pneumonia to β-lactam antibiotics is to mainly produce extended-spectrum β-lactamases encoded by extended-spectrum β-lactamase genes that destroy the β-lactam ring and lead to antibiotic inactivation (Fluit et al., 2001). Although β-lactam antibiotics and genes were diverse, the resistance phenotype and genotype of isolates roughly corresponded through this study. Given that rapid distribution and occurrence of novel ARGs among bacteria are intensifying problems throughout the world, it is necessary to monitor the resistance genes carried by the bacteria of giant pandas.
MGEs play an important role in the dissemination of ARGs among K. pneumonia strains. We had detected 13 MGEs among the 30 MDR isolates. The number of MGEs detected in this study was higher than the previous research on E. coli. strains isolated from giant panda, while traA, traF, tnsA, ISpa7, ISkpn6, and intI3 genes were all negative, which was consistent with previous findings (Zhu et al., 2020). The occurrence of MDR among microbes is considered to be associated with MGEs due to their ability to transfer several ARGs instantaneously (Martinez, 2009). Horizontal transfer of ARGs mediated by MGEs is one of the main mechanisms for the dissemination of ARGs (Chen et al., 2018). MGE as a carrier of ARGs plays an important role in capturing, accumulating and spreading, this can occur between cells of the same strain or between different K. pneumonia strains, enabling the rapid and widespread growth of antibiotic-resistant bacteria. In addition, the horizontal transfer of ARGs accelerates the emergence of drug-resistant and multi-drug-resistant bacteria in clinical practice (Chamosa et al., 2017). In this study, MDR K. pneumonia strains isolated from different giant panda carried some same ARGs, and a large number of MGEs were detected. Therefore, it can be speculated that there was the possibility of sharing the ARGs between giant pandas in this study. However, further investigation is needed to determine how the ARGs are shared.
We further analyzed antimicrobial resistance related to integrons in the 30 MDR K. pneumonia isolates. Integrons are genetic units consisting of determinants of a site-specific recombination event, which can mobilize and capture genes encoded on mobile elements known as gene cassettes (Gillings, 2014). In our study, the proportion of isolates containing the intI1 gene (96.67%, 29/30) was alarmingly higher than that previously reported in giant pandas (Zou et al., 2018; Zhu et al., 2020). We speculated the reason was that the intI1 gene was detected from MDR K. pneumonia. The diversity of gene cassettes are incorporated in the integrons and are able to encode antibiotics resistance to aminoglycoside, sulfonamides and trimethoprim. Accordingly, integrons were significantly connected with MDR (San et al., 2008; Tajbakhsh et al., 2015; Akrami et al., 2017). The intI2 and intI3 genes were not detected in our study. Many studies revealed that the class 1 integron was more prevalent than the class 2 or 3 in gram-negative bacteria (Lavakhamseh et al., 2016; Chamosa et al., 2017; Rehman et al., 2017; Sidhu et al., 2017). Moreover, class 3 integrons are reported to be rare in animals and clinical samples. Therefore, the presence of only intl1 integrons and the absence of intl2 and intl3 integrons in the present study were consistent with other research (Chen et al., 2010, 2016; Rehman et al., 2017; Zou et al., 2018; Zhu et al., 2020). Sequencing analysis showed that there were only two types of gene cassettes (dfrA12 + orfF + aadA2 in 2 isolates, and dfrA12 + orfF in 3 isolates) from the 29 intI1-positive isolates in this study. The other 24 strains provided negative results for the gene cassettes likely due to retained empty or undetermined intl1 VRs, which was also reported in other studies (Zhang et al., 2019; Zhu et al., 2020). Previous studies had confirmed that the gene cassettes dfrA12-orfF-aadA2 and dfrA17-aadA5 were dominant in giant pandas. These gene cassettes have previously been reported in E. coli isolates (Koczura et al., 2015; Yu et al., 2016; Zou et al., 2018; Zhu et al., 2020), which were constituted by trimethoprim resistance genes (dfrA12, dfrA17), and also carried the aadA2 and aadA5 genes which led to resistance to aminoglycoside (example: streptomycin). In addition, these gene cassettes can code a reading frame (orfF) for a hypothetical protein with an unknown function (Zhang et al., 2019). As far as we know, the integron gene cassettes in our study exist widely in humans, animals, plants, and the environment, however, this is the first report of integron gene cassettes in K. pneumonia isolated from the feces of giant pandas. It is emphasized that we should focus more on intergrons which contribute to the effective spread of ARGs.
MLST is an unambiguous procedure for characterizing isolates of bacterial species using the sequences of internal fragments of (usually) seven house-keeping genes (Jolley et al., 2018). In this study, thirty MDR K. pneumoniae isolates were divided into 22 STs, five of which belonged to ST37. Clonal Structure analysis through eBURST software showed that there was no clonal complex in thirty MDR K. pneumoniae isolates, but ST37 had two SLVs, which indicated that although MDR Klebsiella pneumoniae strains isolated from different giant pandas were highly diverse, some strains still had the same origin.
Conclusion
In our study, 30 MDR strains among 182 K. pneumonia strains isolated from the feces of captive giant pandas. All of the MDR K. pneumonia strains carried a large number of ARGs and MGEs, especially 29 of 30 MDR K. pneumonia strains carried integrons. In addition, two different types of gene cassettes were detected. What’ more, MLST indicated that some MDR K. pneumonia strains had the same origin. To the best of our knowledge, MDR and a diversity of ARGs and MGEs existed in K. pneumonia isolates from captive giant pandas, and integrons were related to the emergence of MDR strains, and giant pandas may share the MDR K. pneumonia isolates. Therefore, it is essential that relevant precautionary measures be taken to prevent the spread of MDR bacteria, and monitor the emergence of MGEs integrons. Further studies are required to assess other factors involved in the transmission of linked resistance genes, and efforts should be made to eliminate or reduce drug resistance in captive giant pandas.
Data Availability Statement
The datasets presented in this study can be found in online repositories. The names of the repository/repositories and accession number(s) can be found in the article/Supplementary Material.
Ethics Statement
The animal study was reviewed and approved by the Institutional Animal Care and Use Committee (IACUC) of the Chengdu Research Base of Giant Panda Breeding (No. 2018017).
Author Contributions
XY, JD, ZR, XS, and RH contributed to conception and design of the study. SL and JD played a guiding role in carrying out the experiment. XY, YL, DZ, CY, and LL collected samples. XY and MY performed bacterial identification and isolation and related component testing. XY, XS, HD, YD, ZX, and XF performed the statistical analysis. XY wrote the first draft of the manuscript. All authors contributed to manuscript revision, read, and approved the submitted version.
Funding
This research was supported by the Chengdu Research Base of Giant Panda Breeding (project nos. 2021CPB-B15), the National Forestry and Grassland Administration and Sichuan Provincial Finance Department (project title: study on main epidemiological investigation and prevention of giant panda), and the Sichuan Agricultural University 2019 Disciplinary Construction Double Branch Plan (project nos. 035-1921993036).
Conflict of Interest
The authors declare that the research was conducted in the absence of any commercial or financial relationships that could be construed as a potential conflict of interest.
Publisher’s Note
All claims expressed in this article are solely those of the authors and do not necessarily represent those of their affiliated organizations, or those of the publisher, the editors and the reviewers. Any product that may be evaluated in this article, or claim that may be made by its manufacturer, is not guaranteed or endorsed by the publisher.
Acknowledgments
We would like to thank the veterinary staff and keepers of the Chengdu Panda Base for collecting samples, and James Edward Ayala for reviewing the manuscript, and Yulin Jiang and Huanxin Liu for their help in some experiments.
Supplementary Material
The Supplementary Material for this article can be found online at: https://www.frontiersin.org/articles/10.3389/fmicb.2021.801292/full#supplementary-material
Footnotes
References
Akrami, F., Shahandashti, E. F., Yahyapour, Y., Sadeghi, M., Khafri, S., Pournajaf, A., et al. (2017). Integron types, gene cassettes and antimicrobial resistance profile of Acinetobacter baumannii isolated from BAL samples in Babol, north of Iran. Microb. Pathog. 109, 35–38. doi: 10.1016/j.micpath.2017.05.005
Apondi, O. E., Oduor, O. C., Gye, B. K., and Kipkoech, M. K. (2016). High prevalence of multi-drug resistant Klebsiella pneumoniae in a tertiary teaching hospital in western kenya. Afr. J. Infect. Dis. 10, 89–95. doi: 10.21010/ajid.v10i2.3
Chamosa, L. S., Álvarez, V. E., Nardelli, M., Quiroga, M. P., Cassini, M. H., and Centrón, D. (2017). Lateral antimicrobial resistance genetic transfer is active in the open environment. Sci. Rep. 7:513. doi: 10.1038/s41598-017-00600-2
Chen, D., Zou, W., Xie, S., Kong, L., Chen, Y., Zhang, X., et al. (2018). Serotype and antimicrobial resistance of Escherichia coli isolated from feces of wild giant pandas (ailuropoda melanoleuca) in Sichuan Province, China. J. Wildl. Dis. 54, 691–699. doi: 10.7589/2017-07-165
Chen, H., Shu, W., Chang, X., Chen, J. A., Guo, Y., Tan, Y., et al. (2010). The profile of antibiotics resistance and integrons of extended-spectrum beta-lactamase producing thermotolerant coliforms isolated from the Yangtze River basin in Chongqing. Environ. Pollut. 158, 2459–2464. doi: 10.1016/j.envpol.2010.03.023
Chen, Q., An, X., Li, H., Su, J., Ma, Y., and Zhu, Y. G. (2016). Long-term field application of sewage sludge increases the abundance of antibiotic resistance genes in soil. Environ. Int. 9, 1–10. doi: 10.1016/j.envint.2016.03.026
Clinical and Laboratory Standards Institute [CLSI] (2020). Performance Standards For Antimicrobial Susceptibility Testing: Twenty - Fourth Informational Supplement CLSI document M100-S24. Wayne, PA: CLSI.
Dolejska, M., Cizek, A., and Literak, I. (2007). High prevalence of antimicrobial-resistant genes and integrons in Escherichia coli isolates from black-headed gulls in the Czech Republic. J. Appl. Microbiol. 103, 11–19. doi: 10.1111/j.1365-2672.2006.03241.x
Fluit, A. C., Visser, M. R., and Schmitz, F. J. (2001). Molecular detection of antimicrobial resistance. Clin. Microbiol. Rev. 14, 836–871. doi: 10.1128/CMR.14.4.836-871.2001
Gao, L., Lv, Y., and Li, Y. (2020). Analysis of the drug resistance of carbapenem-resistant Klebsiella pneumoniae in the China antimicrobial resistance surveillance trial program, 2007-2018. Microb. Drug Resist. 26, 944–950. doi: 10.1089/mdr.2019.0299
Gao, Q., Shen, Z., Qin, J., Liu, Y., and Li, M. (2020). Antimicrobial resistance and pathogenicity determination of community-acquired hypervirulent Klebsiella pneumoniae. Microb. Drug Resist. 26, 1195–1200. doi: 10.1089/mdr.2019.0439
Gillings, M. R. (2014). Integrons: past, present, and future. Microbiol. Mol. Biol. Rev. 78, 257–277. doi: 10.1128/MMBR.00056-13
Guo, C., Yang, X., Wu, Y., Yang, H., Han, Y., Yang, R., et al. (2015). MLST-based inference of genetic diversity and population structure of clinical klebsiella pneumoniae, China. Sci. Rep. 5:7612. doi: 10.1038/srep07612
Guo, L., Long, M., Huang, Y., Wu, G., Deng, W., Yang, X., et al. (2015). Antimicrobial and disinfectant resistance of Escherichia coli isolated from giant pandas. J. Appl. Microbiol. 119, 55–64. doi: 10.1111/jam.12820
Guo, X., Cao, Z., Dai, Z., Li, Y., He, X., Hu, X., et al. (2017). Antimicrobial susceptibility and molecular epidemiology of multidrug-resistant Klebsiella pneumoniae in central China. Jpn. J. Infect. Dis. 70, 229–234. doi: 10.7883/yoken.JJID.2016.049
Hu, T., Dai, Q., Chen, H., Zhang, Z., Dai, Q., Gu, X., et al. (2021). Geographic pattern of antibiotic resistance genes in the metagenomes of the giant panda. Microb. Biotechnol. 14, 186–197. doi: 10.1111/1751-7915.13655
Jeena, P., Thompson, E., Nchabeleng, M., and Sturm, A. (2001). Emergence of multi-drug-resistant Acinetobacter anitratus species in neonatal and paediatric intensive care units in a developing country: concern about antimicrobial policies. Ann. Trop. Paediatr. 21, 245–251. doi: 10.1080/02724930120077835
Jolley, K. A., Bray, J. E., and Maiden, M. C. J. (2018). Open-access bacterial population genomics: BIGSdb software, the PubMLST.org website and their applications. Wellcome Open Res. 24:124. doi: 10.12688/wellcomeopenres.14826.1
Kaushik, M., Kumar, S., Kapoor, R. K., Virdi, J. S., and Gulati, P. (2018). Integrons in Enterobacteriaceae: diversity, distribution and epidemiology. Int. J. Antimicrob. Agents 51, 167–176. doi: 10.1016/j.ijantimicag.2017.10.004
Koczura, R., Krysiak, N., Taraszewska, A., and Mokracka, J. (2015). Coliform bacteria isolated from recreational lakes carry class 1 and class 2 integrons and virulence-associated genes. J. Appl. Microbiol. 119, 594–603. doi: 10.1111/jam.12838
Lavakhamseh, H., Mohajeri, P., Rouhi, S., Shakib, P., Ramazanzadeh, R., Rasani, A., et al. (2016). Multidrug-Resistant Escherichia coli strains isolated from patients are associated with class 1 and 2 integrons. Chemotherapy 61, 72–76. doi: 10.1159/000438666
Lu, M. G., Jiang, J., Liu, L., Ma, A. P., and Leung, F. C. (2015). Complete genome sequence of Klebsiella pneumoniae strain HKUOPLC, a cellulose-degrading bacterium isolated from giant panda feces. Genome Announc. 3, e01318–e01415. doi: 10.1128/genomeA.01318-15
Magiorakos, A. P., Srinivasan, A., Carey, R. B., Carmeli, Y., Falagas, M. E., Giske, C. G., et al. (2012). Multidrug-resistant, extensively drug-resistant and pandrug-resistant bacteria: an international expert proposal for interim standard definitions for acquired resistance. Clin. Microbiol. Infect. 18, 268–281. doi: 10.1111/j.1469-0691.2011.03570.x
Martinez, J. L. (2009). Environmental pollution by antibiotics and by antibiotic resistance determinants. Environ. Pollut. 157, 2893–2902. doi: 10.1016/j.envpol.2009.05.051
Partridge, S. R., Tsafnat, G., Coiera, E., and Iredell, J. R. (2009). Gene cassettes and cassette arrays in mobile resistance integrons. FEMS Microbiol. Rev. 33, 757–784. doi: 10.1111/j.1574-6976.2009.00175.x
Peng, G. N., and Xiong, Y. (2000). The studying status quo on the panda Klebsiella pneumoniae. Chin. J. Sichuan Anim. Vet. Sci. 27, 86–87. doi: 10.3969/j.issn.1001-8964.2000.z1.044
Rehman, M. U., Zhang, H., Huang, S., Iqbal, M. K., Mehmood, K., Luo, H., et al. (2017). Characteristics of integrons and associated gene cassettes in antibiotic-resistant Escherichia coli isolated from free-ranging food animals in China. J. Food Sci. 82, 1902–1907. doi: 10.1111/1750-3841.13795
San, M. B., Lapierre, L., Cornejo, J., and Bucarey, S. (2008). Characterization of antibiotic resistance genes linked to class 1 and 2 integrons in strains of Salmonella spp. isolated from swine. Can. J. Microbiol. 54, 569–576. doi: 10.1139/w08-045
Sidhu, J., Jagals, P., Smith, A., and Toze, S. (2017). Comparative prevalence of Escherichia coli carrying virulence genes and class 1 and 2 integrons in sub-tropical and cool temperate freshwater. Environ. Sci. Pollut. Res. Int. 24, 18263–18272. doi: 10.1007/s11356-017-9497-0
Su, J. Q., Wei, B., Ou-Yang, W. Y., Huang, F. Y., Zhao, Y., Xu, H. J., et al. (2015). Antibiotic resistome and its association with bacterial communities during sewage sludge composting. Environ. Sci. Technol. 49, 7356–7363. doi: 10.1021/acs.est.5b01012
Su, X. Y., Li, Y. L., Yan, X., Zhang, D. S., Li, L., Hou, R., et al. (2021). Isolation and identification of extended-spectrum β-lactamases-producing Klebsiella pneumoniae in captive giant panda. Chin. J Vet. Med. 7, 1276–1281. doi: 10.16303/j.cnki.1005-4545.2021.07.08
Sun, F., Yang, S., and Chen, X. (2013). Detection of intestinal pathogenic Klebsiella pneumoniae from fecal samples of giant panda by polymerase chain reaction. J. Biotech. Res. 5, 10–15.
Tajbakhsh, E., Khamesipour, F., Ranjbar, R., and Ugwu, I. C. (2015). Prevalence of class 1 and 2 integrons in multi-drug resistant Escherichia coli isolated from aquaculture water in Chaharmahal Va Bakhtiari province, Iran. Ann. Clin. Microbiol. Antimicrob. 14:37. doi: 10.1186/s12941-015-0096-y
Wang, C. D., Lan, J. C., Luo, L., Yang, Z., and Zhang, R. R. (2006). Klebsiella pneumonia-pathogen of urogenital hematuria of giant panda. Sichuan J. Zool. 25, 83–85. doi: 10.3969/j.issn.1000-7083.2006.01.018
Wang, T., Xie, Y., Zheng, Y., Wang, C., Li, D., Koehler, A. V., et al. (2018). Parasites of the giant panda: a risk factor in the conservation of a species. Adv. Parasitol. 99, 1–33. doi: 10.1016/bs.apar.2017.12.003
Winokur, P. L., Canton, R., Casellas, J. M., and Legakis, N. (2001). Variations in the prevalence of strains expressing an extended-spectrum beta-lactamase phenotype and characterization of isolates from Europe, the Americas, and the Western Pacific region. Clin. Infect. Dis. 32(Suppl. 2), S94–S103. doi: 10.1086/320182
Yang, X., Cheng, G., Li, C., Yang, J., Li, J., Chen, D., et al. (2017). The normal vaginal and uterine bacterial microbiome in giant pandas (Ailuropoda melanoleuca). Microbiol. Res. 199, 1–9. doi: 10.1016/j.micres.2017.01.003
Yang, Y., Peng, Y., Jiang, J., Gong, Z., Zhu, H., Wang, K., et al. (2021). Isolation and characterization of multidrug-resistant Klebsiella pneumoniae from raw cow milk in Jiangsu and Shandong provinces, China. Transbound. Emerg. Dis. 68, 1033–1039. doi: 10.1111/tbed.13787
Yu, T., Zhang, J., Jiang, X., Wu, J., Dai, Z., Wu, Z., et al. (2016). Characterization and horizontal transfer of class 1 integrons in Escherichia coli isolates from cooked meat products. J. Infect. Dev. Ctries. 10, 68–73. doi: 10.3855/jidc.6858
Zhang, A. Y., Wang, H. N., Tian, G. B., Zhang, Y., Yang, X., Xia, Q. Q., et al. (2009). Phenotypic and genotypic characterisation of antimicrobial resistance in faecal bacteria from 30 Giant pandas. Int. J. Antimicrob. Agents 33, 456–460. doi: 10.1016/j.ijantimicag.2008.10.030
Zhang, S., Yang, H., Rehman, M. U., Yang, K., Dong, M., Yang, J., et al. (2019). Class 1 integrons as predominant carriers in Escherichia coli isolates from waterfowls in Hainan, China. Ecotoxicol. Environ. Saf. 183:109514. doi: 10.1016/j.ecoenv.2019.109514
Zhu, Z., Pan, S., Wei, B., Liu, H., Zhou, Z., Huang, X., et al. (2020). High prevalence of multi-drug resistances and diversity of mobile genetic elements in Escherichia coli isolates from captive giant pandas. Ecotoxicol. Environ. Saf. 198:110681. doi: 10.1016/j.ecoenv.2020.110681
Keywords: giant panda, MDR K. pneumonia, ARGS, MGEs, integron
Citation: Yan X, Su X, Ren Z, Fan X, Li Y, Yue C, Yang M, Deng H, Deng Y, Xu Z, Zhang D, Li L, Hou R, Liu S and Deng J (2022) High Prevalence of Antimicrobial Resistance and Integron Gene Cassettes in Multi-Drug-Resistant Klebsiella pneumoniae Isolates From Captive Giant Pandas (Ailuropoda melanoleuca). Front. Microbiol. 12:801292. doi: 10.3389/fmicb.2021.801292
Received: 25 October 2021; Accepted: 29 December 2021;
Published: 03 February 2022.
Edited by:
Jean-Marc Rolain, URMITE CNRS-IRD UMR 6236, FranceReviewed by:
Justin Joseph Donato, University of St. Thomas, United StatesChengming Wang, Auburn University, United States
Copyright © 2022 Yan, Su, Ren, Fan, Li, Yue, Yang, Deng, Deng, Xu, Zhang, Li, Hou, Liu and Deng. This is an open-access article distributed under the terms of the Creative Commons Attribution License (CC BY). The use, distribution or reproduction in other forums is permitted, provided the original author(s) and the copyright owner(s) are credited and that the original publication in this journal is cited, in accordance with accepted academic practice. No use, distribution or reproduction is permitted which does not comply with these terms.
*Correspondence: Songrui Liu, srui_liu@163.com; Junliang Deng, dengjl213@126.com
†These authors have contributed equally to this work and share first authorship