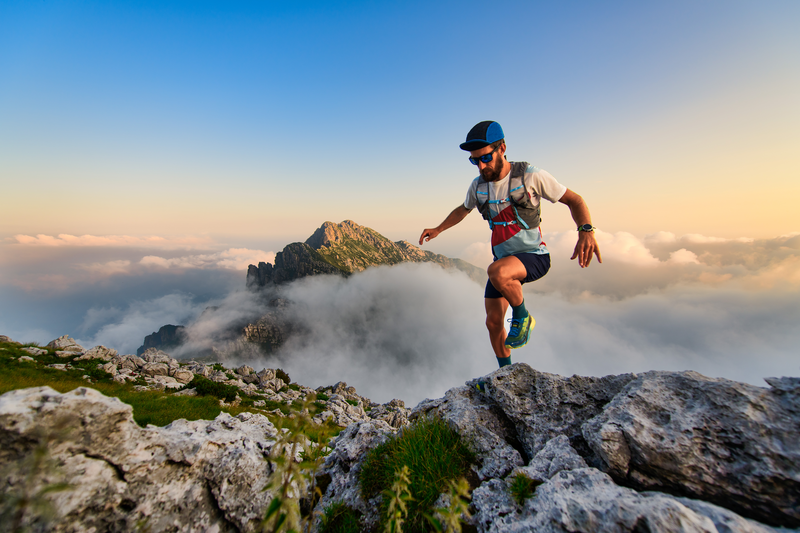
94% of researchers rate our articles as excellent or good
Learn more about the work of our research integrity team to safeguard the quality of each article we publish.
Find out more
ORIGINAL RESEARCH article
Front. Microbiol. , 03 March 2022
Sec. Microbial Symbioses
Volume 12 - 2021 | https://doi.org/10.3389/fmicb.2021.798619
This article is part of the Research Topic Portraying the Phytomicrobiome Studies during Abiotic stresses: Revisiting the past and exploring the future outcomes View all 5 articles
Bacillus spp. are the most prevalent group of bacteria in nature. Their prevalence depends upon multiple factors, namely, sporulation, antagonism, and production of secondary metabolites. The development of an eco-friendly approach to cope with edible crops diseases is very substantial for humans. In the present study, 658 isolates were obtained from wheat grown in the wheat rice cropping system and tested for their antagonistic activity against four wheat root rot pathogens, namely, Fusarium oxysporum, Fusarium moniliforme, Macrophomina phaseolina, and Rhizoctonia solani. Out of 658, 106 isolates were found antagonistic to either single or multiple fungi. Out of 106 antagonistic bacteria, 62 (23%) were rhizospheric, 28 (14%) were root endospheric, and 16 (9%) were leaf endospheric. Based on mean inhibition against all fungi, the bacterial strains SM-39 and SM-93 showed maximum antagonistic activity. The 16S rRNA gene analysis revealed that most of the antagonistic bacteria exhibiting ≥48% antagonism were Bacillus spp. (98%), except two were Klebsiella spp. (2%). The bacterial strains exhibited phylogenetic lineage with the type strains of the respective genus based on the 16S rRNA gene sequences. In the net house experiment, Bacillus velezensis (SM-39) and Bacillus cabrialesii (SM-93) significantly suppressed Fusarium root rot severity in wheat (42–62%). Plants treated with these strains had lower electrolytic leakage (29–36%), as compared to untreated (44%). Relative water content was much higher (46–58%) for plants inoculated with these strains. These antagonistic strains also considerably colonized the wheat rhizosphere with a cell population of 5.8–6.9.log CFU/g of soil. The rhizosphere of wheat grown in the wheat-rice cropping system could be the potential habitat of effective biocontrol agents.
Phytopathogenic fungi affect the field crops adversely and pose a serious threat to agriculture. They not only decrease the crop yield but also deteriorate their quality. They cause destructive damage to crops, leading to economic losses of 1 billion dollars globally (Volova et al., 2018). Among various pathogens, root rot fungi such as Macrophomina phaseolina, Fusarium oxysporum, Fusarium moniliforme, and Rhizoctonia solani are the most devastating pathogens of cereals including wheat (Islam et al., 2013). Their infection coupled with the prolonged drought conditions poses a serious threat to global food security (Fira et al., 2018).
Biological control of phytopathogens using plant growth-promoting rhizobacteria (PGPR) is an eco-friendly strategy (Parry et al., 2016; Dangi et al., 2017; Khanna et al., 2019a). A plethora of literature is available on the activity of PGPR (Khanna et al., 2019b; Sharma et al., 2020) but their commercial application is still limited. The factors responsible for their commercial limitation include lack of consistent field efficacy and poor shelf life (Valente et al., 2020).
The field performance of PGPR is typically inconsistent because of sub-optimal rhizosphere colonization and promiscuous host-specificity (Martiny et al., 2006). The higher persistence of certain microbes in the rhizosphere is a key factor that determines their consistent efficacy. Sometimes, the efficient rhizobacteria lose their efficacy due to competition with the soil microbiota. The antagonistic potential of microbes plays an important role in determining the spatial structuring of bacterial communities. This has also been formerly verified in different bacterial communities such as endophytic bacteria, whose antagonism has been described as one of the reasons for structuring the community within different plant compartments (Chiellini et al., 2019).
Rhizobacteria-host adaptation is a complex phenomenon and is regulated by multiple factors including bacterial type, host genotype, and rhizosphere environment (Brader et al., 2014). As the rhizobacteria are recognized by a certain host, crosstalk of the signaling molecules is initiated (Verma et al., 2021). The signaling also includes the chemotactic movements of microbes regulated by the root exudates of plants (Abid et al., 2018). The occurrence of certain bacterial communities is highly influenced by biotic and abiotic factors. In agriculture niches/habitats, various cultural practices like irrigation, fertilization, pathogen infestation, and cropping pattern highly influence the diversity and activity of the microbes inhabiting certain rhizosphere.
Wheat (Triticum aestivum) being a staple food is grown worldwide under different cropping systems such as fallow-wheat, wheat-maize, and wheat-rice (Koondhar et al., 2018). Wheat-rice is the most promising cropping pattern as it involves the rotation of economically important crops. The bacterial communities of multiple cropping systems have been reported as diverse than that of mono-cropping systems (Bhattacharyya and Jha, 2012).
Plant growth-promoting rhizobacteria belong to diverse genera such as Arthrobacter, Azospirillum, Bacillus, Burkholderia, Chromobacterium, Caulobacter, Erwinia, Enterobacter, Flavobacterium, Pseudomonas, and Klebsiella. The distribution of rhizobacterial communities varies with the host species, genotype, growth stage, location, and growing practices (Hakim et al., 2018). Although various studies have been conducted to know the diversity of rhizospheric bacteria using culture-dependent and independent techniques (Qaisrani et al., 2019), less attention has been paid to the prevalence of antagonistic bacteria in the rhizosphere of crops grown at the commercial scale in the specific cropping system. Based on these facts, this study aimed to recruit the antagonistic bacteria effective against wheat root rot and identify the most prevalent antagonistic genera associated with the wheat grown in the wheat-rice cropping system.
A survey was conducted to identify the major areas practicing the wheat-rice cropping system in Punjab, Pakistan. Representative districts, namely, Gujranwala, Gujrat, Sialkot, Lahore, Kasur, Sheikhupura, and Nankana Sahib, within these areas were selected for sampling. Among each district, two types of zones were identified based on their mode of irrigation, i.e., canal and tube well. Two to three fields were randomly identified in a radius of 2 km of the respective zone. Three to four plants (healthy and diseased) with variable phenotypes were sampled randomly from different corners of the field. The plants were generally uprooted manually. However, a mini spade (6′′ × 9′′) with a 1-m handle was used to uproot the plants from moisture deficient soil to minimize the risk of root damage. The spade was surface sterilized with 70% ethanol before using it on the other samples. The uprooted plants were mixed to make the representative sample of each category. The representative samples were tagged, placed in paper bags, brought to the laboratory at 4°C, and processed immediately.
Rhizospheric soil was carefully removed from the root surfaces by vigorously shaking on sterilized aluminum foil. The soil was analyzed for different physiochemical parameters such as organic matter, electrical conductivity, pH, total nitrogen, phosphorous, potassium, sodium, and sodium absorption ratio (SAR) at Fatima Sugar Mills Limited, Kot Addu, Punjab, Pakistan.
The bacterial isolates were obtained from the root rhizosphere, root, and leaf endosphere of plants by the serial dilution method (Ullah et al., 2020; Riaz et al., 2021). For endophytic isolation, the roots/leaves were washed with tap water to remove surface impurities and surface sterilized with 0.1% mercuric chloride (HgCl2) (Rais et al., 2016). Briefly, the plant tissues were dipped in solution (0.1% HgCl2) for 1–2 min, washed twice with sterile distilled water, dried, and crushed in a sterilized mortar pestle.
The rhizosphere soil or tissue homogenate (1 g) was suspended in 9 mL of sterile saline (0.9% w/v) and diluted serially (10–1–10–8). The bacterial colonies were obtained by spreading each dilution (100 μL) on Luria-Bertani (LB) agar and incubating at 37 ± 2°C overnight. The bacterial colonies appearing on agar were differentiated based on their morphology like shape, size, margins, and elevations. Different isolates were sub-cultured and preserved in 20% glycerol at −0 ± 2°C for future studies (Rais et al., 2016).
Antagonistic activity of bacteria was assessed against wheat root rot fungi such as F. oxysporum, F. moniliforme, M. phaseolina, and R. solani. Pure fungal cultures were obtained from Applied Microbiology and Biotechnology Laboratory, COMSATS University, Islamabad, Pakistan. A dual culture assay was performed as described in earlier studies (Ullah et al., 2020). Briefly, 5 mm mycelium plug of each fungus (5–7 days old) was kept at the center of the plate containing potato dextrose agar (PDA) and freshly grown bacteria were inoculated at equal distances from each fungus. Sterilized LB broth was used as a control. The plates were incubated at 28 ± 2°C and observed for zone inhibition until the 7th day. The percentage inhibition was calculated by the following formula.
where C, mycelium diameter of fungus taken as control; T, mycelium diameter of fungus treated with bacteria.
The antagonistic bacteria were identified by 16S rRNA gene analysis. Genomic DNA was extracted by CTAB method (Moore et al., 1999). The extracted DNA was qualitatively analyzed on agarose gel (1% W/V) pre-stained with ethidium bromide and electrophoresed in 1× TBE buffer at 100 V for 30–40 min.
DNA bands were visualized in the Gel documentation system (Biometra, Germany) and their quality was compared with that of the DNA ladder (Thermo Scientific). The 16S rRNA gene was amplified by using the primers P1 and P6 (Tan et al., 1997). A 50 μL PCR reaction consisted of PCR water (31 μL), 5 μL Taq buffer (10x), 4 μL MgCl2 (25 mM), 3 μL dNTPs (10 mM), 2 μL of each primer (100 pM), 0.5 μL Taq polymerase (500 U), and 2.5 μL template DNA (25 ng/μL). The reaction was amplified in a thermal cycler (Applied Biosystems, United States) using the cycling conditions, namely, initial denaturation (95°C for 5 min), 25 cycles x (denaturation at 95°C for 1 min, annealing at 56°C for 1 min, extension at 72°C for 1.5 min) and final extension at 72°C for 5 min. The PCR products were visualized by gel electrophoresis (as described above) and purified by using PCR purification kit (Thermo Scientific).
The purified PCR products of 16S rRNA gene were sequenced by Macrogen Incorporation, South Korea, and analyzed for homology with that of the closest type strain available at EZ taxon1 (Kumar et al., 2016). The 16S rRNA gene sequence of each strain was submitted to the gene bank2 under its respective accession number. For phylogenetic analysis, 16S rRNA genes of respective type strains were accessed from EZ taxon.3 These sequences were oriented in the same direction, i.e., 5′-3′, saved in FASTA format and uploaded on MEGA X software (Molecular Evolutionary Genetics Analysis X). The sequences were aligned and trimmed to the proper length to get conserved regions. These trimmed sequences were then converted into MEGA X format and saved for phylogenetic tree construction. The phylogeny was created by using the neighbor joining phylogenetic tree option in MEGA X software (Afzal et al., 2019). Evolutionary distances were calculated by using the maximum composite likelihood model and were in the units of the number of base substitutions per site.
Bio control efficacy of the Bacillus spp., i.e., Bacillus velezensis and Bacillus cabrialesii showing the best in vitro inhibition (60–63%) against root rot pathogens was assessed on wheat against Fusarium root rot. The experiment was carried out at COMSATS University, Islamabad, Pakistan (33.65°N, 73.16°) in the net house conditions during the natural growing season of wheat, i.e., November–December (2019–2020) (humidity = 65% and average temperature = 15.8°C). The seeds of wheat varieties, i.e., Galaxy and Sahar were obtained from National Agriculture Research Centre (NARC), Islamabad, Pakistan. There were five treatments, with three replications per treatment, namely, untreated plants (1), fungicide (Dimethomorph) (2), SM-39 (B. velezensis) (3), SM-93 (B. cabrialesii) (4), and a consortium of SM-39, SM-93 (5). The experiment was repeated twice during the years 2019–2020.
The soil [clay loam, phosphorous (12–14 mg/kg), total nitrogen (0.03–0.05%); organic matter (0.5–0.7%), and pH (7.6–7.8)] was obtained from a wheat field, autoclaved twice, filled in pots (17 cm × 21 cm), and fertilized with nitrogen (120 Kg/ha), phosphorous (100 Kg/ha), and potassium (60 Kg/ha) (Ramzani et al., 2016), (Ullah et al., 2020). The upper layer of soil (2–3 inches) was infested with the root rot pathogen F. moniliforme (Jaber, 2018). The fungus was grown on PDA for 7 days at 30 ± 2°C, scrapped with the spatula, and suspended in sterile water. The spore suspension was mixed with the upper layer of soil at the rate of 105 spores per g of soil.
The seeds were surface sterilized with 0.1% sodium hypo chloride (NaClO), dressed with bacterial cell suspension (4 × 109 CFU/mL), carboxy methyl cellulose (1% w/v), fungicide (Dimethomorph 90 g w/w) and sown in pots. Two plants were maintained in each pot and five plants were included in each replication. All the agronomic practices were followed as per standard recommendations (Ullah et al., 2020).
The disease was examined on the 60th day of seed sowing. Fifteen plants/treatment were randomly selected, uprooted manually, and assessed for root rot disease on a scale (0–5), i.e., 0 = healthy (0%), 1 = very slight browning (10–20%), 2 = slight to moderate browning (20–40%), 3 = moderate (40–60%), 4 = severe (>60%), and 5 = completely necrotic or dead (100%). Disease severity was determined by using the formula as described by Wildermuth and McNamara (1994).
where x is the value of disease score, n is the value of the highest disease score, and f is the number of plants for each score.
The relative water content of wheat leaves was determined as described by Guerfel et al. (2009). The fresh leaves (4–5) per replication were randomly selected, chopped, and pooled. The fresh leaves (0.5 g) were soaked in 100 mL distilled water at room temperature (27°C) for 24 h to record their turgid weight. Then, these leaves were kept at 70°C in a dry oven until the appearance of constant weight. Relative water content was calculated according to the formula used by Riaz et al. (2021).
where FW, fresh weight; DW, dry weight; and TW, turgid weight.
The electrolytic leakage of leaves was determined by the method of Ekmekci and Terzioglu (2005). Briefly, the fresh leaves were sliced and placed in deionized water (20 mL) under dark conditions for 24 h to measure the initial electrical conductivity (EC1) at room temperature with a conductivity meter. The leaves were autoclaved to release all the electrolytes. Final electrical conductivity (EC2) was measured at room temperature. The electrolytic leakage was calculated by using the following formula:
The bacterial colonization potential on wheat roots was assessed as described earlier (Ullah et al., 2020). Rhizospheric soil samples from 15 plants per replication (uprooted for diseases assessment as described in section “In planta Biocontrol Activity”) were collected and pooled together to make composite samples. The inoculated antagonistic bacteria were isolated by serial dilution method and identified based on their colony morphology and antagonism against respective fungi.
The numeric data of repeated experiments were pooled and analyzed on the statistical package Statistix 8.1. by applying the analysis of variance (ANOVA). The mean among different treatments were separated by Fisher’s least significant difference (LSD) test at p ≤ 0.05. Pearson correlation was determined by using the same statistical package. Venn diagram was constructed by using origin 6.0. Principal component analysis (PCA) was constructed by using software PRIMER 6 and PERMANOVA.
The soil texture was clay loam with organic matter (0.71–0.97%), electrical conductivity (1.0–2.2 ds/m), pH (7.2–8.2), total nitrogen (0.03–0.07%), phosphorous (10.9–13.8 ppm), potassium (170–228 ppm), sodium (146–230 ppm), and SAR (2–3.1) (Table 1). A total of 658 isolates were obtained from wheat grown in rice-wheat cropping system of Punjab, Pakistan. Out of these 658 isolates, 271 were obtained from root rhizosphere, 202 from root endosphere, and 185 from leaf endosphere, respectively.
A total of 658 bacterial isolates associated with wheat root rhizosphere, root endosphere, and leaf endosphere were tested for their antagonistic activity against wheat root rot fungi, namely, F. moniliforme, F. oxysporum, M. phaseolina, and R. solani. Out of 658 bacterial isolates, 106 isolates were found antagonistic to either single or multiple fungi, namely, F. oxysporum, F. moniliforme, M. phaseolina, and R. solani (Supplementary Table 1). Out of 106 antagonistic bacteria, 48 strains antagonized four fungi, namely, F. oxysporum, F. moniliforme, M. phaseolina, and R. solani. Based on a group of three test fungi, 22 strains antagonized M. phaseolina, F. oxysporum, and R. solani. Three strains antagonized F. oxysporum, F. moniliforme, and M. phaseolina, one strain antagonized F. oxysporum, F. moniliforme, and R. solani, and one strain antagonized F. moniliforme, M. phaseolina, and R. solani, respectively. Seven strains antagonized two fungi, namely, R. solani and F. oxysporum, five strains antagonized two fungi, namely, F. moniliforme and R. solani. One strain antagonized M. phaseolina and F. moniliforme and one strain antagonized M. phaseolina and R. solani. Eight strains antagonized only R. solani, four strains antagonized F. oxysporum, three strains antagonized F. moniliforme and two strains antagonized only M. phaseolina (Figure 1). R. solani was antagonized by a maximum number of strains (93), followed by F. oxysporum (85), M. phaseolina (78), and F. moniliforme (62), respectively. The potential of antagonistic bacteria against each fungus was highly variable (29–76%). In the case of R. solani, the maximum number of strains (42) showed antagonism between 61 and 68%. The maximum number of strains (39 each) antagonized F. oxysporum and M. phaseolina between 45 and 52%. While in the case of F. moniliforme, the maximum number of strains (25) showed antagonism between 37 and 44% (Figure 2).
Figure 1. Venn diagram representing the number of antagonists against different root rot fungi. F, Fusarium; R, Rhizoctonia; and M, Macrophomina.
Figure 2. Antagonistic bacteria with variable antagonistic potential (29–76%) against root rot fungi. F, Fusarium; R, Rhizoctonia; and M, Macrophomina.
The distribution of antagonistic bacteria was variable in different locations, plant health, irrigation source, and plant parts, i.e., rhizosphere, root, and leaf endospheres. Based on location, highest antagonistic bacteria (29%) were found in Sheikhupura followed by Sialkot (26%), Lahore (25%), Kasur (22%), Gujrat (21%), and Gujranwala (20%), respectively. In the case of irrigation source, the highest antagonism was found in canal irrigated (3–29%), followed by tube well irrigated (3–20%) (Figure 3). Healthy plants had higher antagonistic bacteria (12–30%), followed by that of diseased plants (1–20%) (Figure 4). The distribution of percent antagonistic bacteria in different plant parts was also variable. The highest number of antagonistic bacteria were observed in rhizosphere (23%), followed by that of root (14%) and leaf endospheres (9%), respectively.
Figure 3. Principal component analysis (PCA) of antagonistic bacteria isolated from tube well/canal irrigated wheat crop. Variables of soil parameters, plant parts, i.e., rhizosphere, root, and leaf endospheres are represented by base vectors. EC, electrical conductivity; SAR, sodium adsorption ratio; P, phosphorous; K, potassium; Na, sodium; N, nitrogen; OM, organic matter; R, rhizosphere; LE, leaf endosphere; and RE, root endosphere.
Figure 4. Distribution of percent antagonistic bacteria isolated from rhizosphere, root, and leaf endosphere of wheat irrigated with canal/tube well and grown in wheat-rice cropping system at different locations. TWH, tube well healthy; TWD, tube well disease; CH, canal healthy; and CD, canal disease.
Based on 16S rRNA gene analysis, the antagonistic bacteria showing ≥48% antagonism were identified as Bacillus spp. except for the strain SM-67 and SM-69 which belonged to klebsiella spp., respectively. The homology with type strains, identity, and 16S rRNA accession number is given in Table 2. The phylogenetic lineage based on 16S rRNA gene is given in Figure 5. The optimal tree with the sum of branch length = 168.4 is shown in Figure 5. Bacillus spp. strain SM-7 showed the highest bootstrap value of 100. The percentage of replicated phylogenetic tree in which the associated taxa are grouped in the bootstrap test (500 replicates) is shown next to the branches of the tree (Figure 5). Type strains are represented with black dots in front of them, while identified strains are represented with their code names. Phylogenetic lineage was calculated based on the 16S rRNA nucleotide sequences of different strains.
Figure 5. Phylogenetic lineages of antagonistic bacteria based on 16S rRNA gene. Tree leaves represent bacterial genus with accession numbers and likely top hit strain, isolated in this study. Type strains downloaded from NCBI are represented with black dots (•).
Inoculated Bacillus spp. significantly reduced Fusarium root rot severity in both wheat varieties, i.e., Sahar and Galaxy, as compared to uninoculated. A significant disease suppression over control was observed in the plants treated with consortium of B. velezensis SM-39 and B. cabrialesii SM-93 (52–62%) followed by that of B. velezensis SM-39 (49%) and B. cabrialesii SM-93 strain (42–49%) (Figure 6A). A similar trend in the performance of all treatments was observed in electrolytic leakage and relative water content (Figures 6B,C).
Figure 6. Effect of antagonistic bacteria on wheat challenged with F. moniliforme. (A) Root rot severity (%), (B) electrolytic leakage (%), (C) relative water content (%), and (D) colonization of bacteria to wheat rhizosphere. The values are the mean of three replications and having different letters within the same bar are significantly different from each other according to Fischer’s LSD test at p ≤ 0.05.
Antagonistic strains B. velezensis (SM-39) and B. cabrialesii (SM-93) considerably colonized the wheat roots either inoculated individually or in consortium (Figure 6D). Maximum root colonization was observed in B. velezensis treated plants (6.6–9.7 log CFU/g of rhizospheric soil), followed by that of B. cabrialesii (5.2–6.9 log CFU/g of rhizospheric soil) as shown in Table 3. A significant correlation was observed between the biochemical traits, root colonization, and disease severity (Table 4). Wheat variety had a significant effect on disease incidence and biochemical parameters (Supplementary Table 2).
Table 3. Habitat characteristics and root colonization of the Bacillus spp. exhibiting in planta biocontrol activity against wheat root rot.
Table 4. Pearson correlations between disease severity, biochemical traits, and root colonization of antagonistic bacteria.
Antagonistic bacteria inhibit phytopathogens to protect the plants from various diseases (Schlemper et al., 2018). The diversity of antagonistic bacteria in the root rhizosphere and endosphere depends on various factors such as cropping system, cultivar, different agricultural practices, and climatic conditions (Na et al., 2019). In this study, antagonistic Bacillus spp. were found to be the most prevalent in wheat grown in the wheat-rice cropping system.
In wheat, rhizospheric isolates were found higher in number as compared to that of root and leaf endophytes. The higher rhizosphere population may be due to the rich nutrition of the rhizosphere where the plant secretes numerous secondary metabolites including the chemoattractants of the rhizobacteria (Truyens et al., 2015). These results are also in accordance with the earlier studies in which the root rhizosphere was considered as the hub of microbes. The less endophytic population of bacteria may be due to their inability to infiltrate the internal plant tissues. Endophytic bacteria infiltrate the plant’s internal tissues through active and passive mechanisms. The active mechanism involves chemotaxis while the passive involves the movement of bacteria through water flow from injured plant tissues (Cha et al., 2016).
A higher proportion of the bacteria obtained from wheat rhizosphere/endosphere was found to be antagonistic to either one or more pathogens causing wheat root rot. This distribution of antagonistic rhizobacteria in the wheat rhizosphere/endosphere could be due to the disease-suppressive soil of the wheat-rice cropping system (Hu et al., 2018). Another reason behind the abundance of the antagonists could be the specific bacterial community that had adapted this habitat through their antagonistic behavior, which was proved in our further findings, i.e., 16S rRNA identification. The bacterial antagonism was found to be highly variable and dependent on the type of pathogen. This change in antagonistic properties of different bacterial strains is due to their genetic makeup, production of secondary metabolites, plant health, and competition with other soil microbes and type of targeted pathogen (van der Voort et al., 2016). The distribution of antagonistic bacteria was also affected by different parameters such as crop locations, crop health, irrigation source, and plant parts, i.e., rhizosphere, root, and leaf endospheres. Antagonistic bacteria produce different secondary metabolites such as antibiotics and as a result, inhibit various phytopathogens. This antagonism could be due to competition of nutrients by activating the defense mechanism of the host plant and by the production of antifungal compounds, which play an important role in the biocontrol of phytopathogens. Antibiosis and competition are the main mechanisms through which Bacillus spp. control different diseases (Cawoy et al., 2015). Bacillus spp. produce different secondary metabolites such as surfactin, iturin, fengycin, and bacillomycins (Yoon et al., 2017).
The 16S rRNA gene analysis and homology with that of type strain available at EZ Bio cloud database revealed that bacteria obtained from wheat-rice cropping system and exhibiting significant antagonism were Bacillus spp. except for a single strain of Pseudomonas spp. and two strains of Klebsiella spp. The 16S rRNA gene sequence analysis is believed to be an excellent technique for bacterial identification and EzBioCloud is an updated 16S rRNA gene sequences database in line with the latest taxonomic changes (Zhou et al., 2016).
The phylogenetic tree was constructed for all the antagonistic strains based on the 16S rRNA gene sequence on MEGA X software (Afzal et al., 2019). It is an important tool to determine the evolutionary relationship within the strains for analyzing the variation at the genus level (Afzal et al., 2019). The antagonistic bacteria showed variable phylogenetic lineage based on 16S rRNA gene. Our findings of the prevalence of antagonistic Bacillus spp. are in accordance with the earlier studies (Earl et al., 2007). The ubiquitous distribution of Bacillus spp. in the rhizosphere may be due to their unique characteristics such as adaptability to the harsh environment through desiccation tolerance and ability to regenerate through sporulation (Joshi et al., 2013). The occurrence of wheat-associated antagonistic Bacillus spp. in the wheat-rice cropping system may also be due to the specific cropping pattern and its soil environment conditions (Kumar et al., 2012).
Wheat and rice belong to generate family and secrete common root exudates, namely, benzoxazinoids, phenolic acids, and scopoletin (Zahid, 2015). The abundance of Bacillus spp. in this cropping system may also be due to the root exudates mediated recruitment of specific microbes (Winter et al., 2019). The role of root exudates in shaping the specific rhizospheric community is well documented (Lv et al., 2016). Bacillus spp., being facultative anaerobe/aerobe, may also have higher survival in the rice grown in submerged conditions. The long-standing water creates anaerobic conditions which is an environment for the adaptation of specific microbial communities like Bacillus spp. The effect of waterlogging conditions on change in the microbial community is well documented (Mentzer et al., 2006). In waterlogging conditions, oxygen quantity decreases which leads to a reduction in respiration rate and activity of the microbial community. This in turn leads to variations in the distribution of the microbial community (Fuchslueger et al., 2014). Thus, the wheat-rice cropping system could develop disease suppressive soil and wheat grown in such a system could be a potential habitat of the antagonistic Bacillus spp.
Bacillus spp. with the best in vitro antagonistic abilities also controlled Fusarium root rot in net house conditions. These findings are in accordance with earlier reports, in which rhizospheric bacteria have successfully colonized the wheat rhizosphere (Ullah et al., 2020). However, in the current study, the strains also retained their population upon their inoculation as consortia. The biocontrol efficacy of antagonistic bacteria against Fusarium root rot is well understood (Winter et al., 2019). They adopt multiple mechanisms in plant defense including induction of defense enzymes against biotic and abiotic stress (Khanna et al., 2019c,d). In consortia, our bacterial strains showed the best activity against wheat root rot as compared to the individual ones. Their effect was at par with that of earlier reports in which efficacy of Pseudomonas spp. associated with monoculture wheat was assessed against root rot (Ullah et al., 2020). Our results are also advocated by Lounaci et al. (2016) findings, in which rhizospheric bacteria has efficiently controlled root rot severity. Fungicides also effectively control root rot of wheat and barley, but their irrational use poses a serious threat to the environment. These results indicate that antagonistic bacterial strains could be an ideal candidate for the biocontrol of Fusarium root rot disease (Ngumbi and Kloepper, 2016). Based on their disease suppression abilities, they could be an excellent substitute to the chemicals, i.e., fungicides, which have serious health implications on humans and eco-systems due to their excessive usage (Upadhyay et al., 2018).
The current study states that different rhizospheric and endospheric bacteria isolated from wheat-rice cropping system of Punjab, Pakistan and identified as Bacillus spp. displayed tremendous ability to inhibit different wheat pathogens such as F. oxysporum, F. moniliforme, R. solani, and M. phaseolina. So, it can be concluded that the continuous practice of wheat-rice cropping system could develop disease suppressive soil in certain fields and wheat grown in such fields could be a potential habitat of the antagonistic Bacillus spp.
The original contributions presented in the study are included in the article/Supplementary Material, further inquiries can be directed to the corresponding author/s.
ShM: methods, experiments, writing original draft, data analysis, and review and editing. AW: methodology, experiments, review and editing, and data curation. HY: data analysis, review and editing, methodology, and resources. MH: conceptualization, supervision, resources, funding, and review and editing. SaM: methodology, data analysis, and review and editing. HAE: funding, resourced, and review and editing. NK: analysis, software, and review and editing. All authors contributed to the article and approved the submitted version.
The authors declare that the research was conducted in the absence of any commercial or financial relationships that could be construed as a potential conflict of interest.
All claims expressed in this article are solely those of the authors and do not necessarily represent those of their affiliated organizations, or those of the publisher, the editors and the reviewers. Any product that may be evaluated in this article, or claim that may be made by its manufacturer, is not guaranteed or endorsed by the publisher.
We would like to thank Higher Education Commission (HEC), Pakistan for awarding the research grant (20-2991) and the Crop Disease Research Institute (CDRI), National Agriculture Research Centre (NARC), Islamabad for providing the wheat seeds. We are also thankful to the horticulture staff of CUI Islamabad for their help in the net house experiments. The authors would also like to extend their sincere appreciation to the Researchers Supporting Project Number (RSP-2021/19), King Saud University, Riyadh, Saudi Arabia.
The Supplementary Material for this article can be found online at: https://www.frontiersin.org/articles/10.3389/fmicb.2021.798619/full#supplementary-material
Abid, S., Masood, M. A., Anwar, M. Z., Zahid, S., and Raza, I. (2018). Trends and variability of wheat crop in Pakistan. Asian J. Agric. Rural Dev. 8, 153–159. doi: 10.18488/journal.1005/2018.8.2/1005.2.153.159
Afzal, I., Shinwari, Z. K., Sikandar, S., and Shahzad, S. (2019). Plant beneficial endophytic bacteria: mechanisms, diversity, host range and genetic determinants. Microbiol. Res. 221, 36–49. doi: 10.1016/j.micres.2019.02.001
Bhattacharyya, P. N., and Jha, D. K. (2012). Plant growth-promoting rhizobacteria (PGPR): emergence in agriculture. World J. Microbiol. Biotechnol. 28, 1327–1350.
Brader, G., Compant, S., Mitter, B., Trognitz, F., and Sessitsch, A. (2014). Metabolic potential of endophytic bacteria. Curr. Opin. Biotechnol. 27, 30–37. doi: 10.1016/j.copbio.2013.09.012
Cawoy, H., Debois, D., Franzil, L., De Pauw, E., Thonart, P., and Ongena, M. (2015). Lipopeptides as main ingredients for inhibition of fungal phytopathogens by Bacillus subtilis/amyloliquefaciens. Microbial. Biotechnol. 8, 281–295. doi: 10.1111/1751-7915.12238
Cha, J.-Y., Han, S., Hong, H.-J., Cho, H., Kim, D., Kwon, Y., et al. (2016). Microbial and biochemical basis of a fusarium wilt-suppressive soil. ISME J. 10, 119–129. doi: 10.1038/ismej.2015.95
Chiellini, C., Lombardo, K., Mocali, S., Miceli, E., and Fani, R. (2019). Pseudomonas strains isolated from different environmental niches exhibit different antagonistic ability. Ethol. Ecol. Evol. 31, 399–420. doi: 10.1080/03949370.2019.1621391
Dangi, S. R., Tirado-Corbalá, R., Gerik, J., and Hanson, B. D. (2017). Effect of long-term continuous fumigation on soil microbial communities. Agronomy 7:37. doi: 10.3390/agronomy7020037
Earl, A. M., Losick, R., and Kolter, R. (2007). Bacillus subtilis genome diversity. J. Bacteriol. 189, 1163–1170. doi: 10.1128/JB.01343-06
Ekmekci, Y., and Terzioglu, S. (2005). Effects of oxidative stress induced by paraquat on wild and cultivated wheats. Pesticide Biochem. Physiol. 83, 69–81. doi: 10.1016/j.pestbp.2005.03.012
Fira, D., Dimkić, I., Berić, T., Lozo, J., and Stanković, S. (2018). Biological control of plant pathogens by Bacillus species. J. Biotechnol. 285, 44–55. doi: 10.1016/j.jbiotec.2018.07.044
Fuchslueger, L., Bahn, M., Fritz, K., Hasibeder, R., and Richter, A. (2014). Experimental drought reduces the transfer of recently fixed plant carbon to soil microbes and alters the bacterial community composition in a mountain meadow. New Phytol. 201, 916–927.
Guerfel, M., Baccouri, O., Boujnah, D., Chaïbi, W., and Zarrouk, M. (2009). Impacts of water stress on gas exchange, water relations, chlorophyll content and leaf structure in the two main Tunisian olive (olea europaea L.) cultivars. Scientia Horticul. 119, 257–263. doi: 10.1016/j.scienta.2008.08.006
Hakim, S., Mirza, B. S., Zaheer, A., Mclean, J. E., Imran, A., Yasmin, S., et al. (2018). Retrieved 16S rRNA and nif H sequences reveal co-dominance of bradyrhizobium and ensifer (sinorhizobium) strains in field-collected root nodules of the promiscuous host vigna radiata (L.) R. wilczek. Appl. Microbiol. Biotechnol. 102, 485–497. doi: 10.1007/s00253-017-8609-6
Hu, L., Robert, C. A., Cadot, S., Zhang, X., Ye, M., Li, B., et al. (2018). Root exudate metabolites drive plant-soil feedbacks on growth and defense by shaping the rhizosphere microbiota. Nat. Commun. 9, 1–13. doi: 10.1038/s41467-018-05122-7
Islam, M. R., Sultana, T., Joe, M. M., Yim, W., Cho, J. C., and Sa, T. (2013). Nitrogen-fixing bacteria with multiple plant growth-promoting activities enhance growth of tomato and red pepper. J. Basic Microbiol. 53, 1004–1015. doi: 10.1002/jobm.201200141
Jaber, L. R. (2018). Seed inoculation with endophytic fungal entomopathogens promotes plant growth and reduces crown and root rot (CRR) caused by Fusarium culmorum in wheat. Planta 248, 1525–1535. doi: 10.1007/s00425-018-2991-x
Joshi, S. J., Suthar, H., Yadav, A. K., Hingurao, K., and Nerurkar, A. (2013). Occurrence of biosurfactant producing Bacillus spp. in diverse habitats. Int. Scholarly Res. Notices 2013:652340. doi: 10.5402/2013/652340
Khanna, K., Jamwal, V. L., Kohli, S. K., Gandhi, S. G., Ohri, P., Bhardwaj, R., et al. (2019a). Plant growth promoting rhizobacteria induced Cd tolerance in Lycopersicon esculentum through altered antioxidative defense expression. Chemosphere 217, 463–474.
Khanna, K., Jamwal, V. L., Kohli, S. K., Gandhi, S. G., Ohri, P., Bhardwaj, R., et al. (2019b). Role of plant growth promoting bacteria (PGPRs) as biocontrol agents of meloidogyne incognita through improved plant defense of Lycopersicon esculentum. Plant Soil. 436, 325–345.
Khanna, K., Kohli, S. K., Ohri, P., Bhardwaj, R., Al-Huqail, A. A., Siddiqui, M. H., et al. (2019c). Microbial fortification improved photosynthetic efficiency and secondary metabolism in Lycopersicon esculentum plants under cd stress. Biomolecules 10:581.
Khanna, K., Sharma, A., Ohri, P., Bhardwaj, R., Abd-Allah, E. F., Hashem, A., et al. (2019d). Impact of plant growth promoting rhizobacteria in the orchestration of Lycopersicon esculentum mill. resistance to plant parasitic nematodes: a metabolomic approach to evaluate defense responses under field conditions. Biomolecules 9:676.
Koondhar, M. A., Qiu, L., Magsi, H., Chandio, A. A., and He, G. (2018). Comparing economic efficiency of wheat productivity in different cropping systems of sindh province, Pakistan. J. Saudi Soc. Agric. Sci. 17, 398–407. doi: 10.1016/j.jssas.2016.09.006
Kumar, P., Dubey, R., and Maheshwari, D. (2012). Bacillus strains isolated from rhizosphere showed plant growth promoting and antagonistic activity against phytopathogens. Microbiol. Res. 167, 493–499. doi: 10.1016/j.micres.2012.05.002
Kumar, S., Stecher, G., and Tamura, K. (2016). MEGA7: molecular evolutionary genetics analysis version 7.0 for bigger datasets. Mol. Biol. Evol. 33, 1870–1874. doi: 10.1093/molbev/msw054
Lounaci, L., Guemouri-Athmani, S., Boureghda, H., Achouak, W., and Heulin, T. (2016). Suppression of crown and root rot of wheat by the rhizobacterium Paenibacillus polymyxa. J. Phytopathol. Mediterranea 55, 355–365. doi: 10.14601/Phytopathol_Mediterr-18055
Lv, X.-C., Jia, R.-B., Li, Y., Chen, F., Chen, Z.-C., Liu, B., et al. (2016). Characterization of the dominant bacterial communities of traditional fermentation starters for hong qu glutinous rice wine by means of MALDI-TOF mass spectrometry fingerprinting, 16S rRNA gene sequencing and species-specific PCRs. Food Control 67, 292–302. doi: 10.1016/j.foodcont.2016.03.005
Martiny, J. B. H., Bohannan, B. J., Brown, J. H., Colwell, R. K., Fuhrman, J. A., Green, J. L., et al. (2006). Microbial biogeography: putting microorganisms on the map. Nat. Rev. Microbiol. 4, 102–112. doi: 10.1038/nrmicro1341
Mentzer, J. L., Goodman, R. M., and Balser, T. C. (2006). Microbial response over time to hydrologic and fertilization treatments in a simulated wet prairie. Plant Soil 284, 85–100. doi: 10.1007/s11104-006-0032-1
Moore, E., Arnscheidt, A., Krüger, A., Strömpl, C., and Mau, M. (1999). Simplified protocols for the preparation of genomic DNA from bacterial cultures. Mol. Microbial. Ecol. Manual 1, 1–15.
Na, X., Cao, X., Ma, C., Ma, S., Xu, P., Liu, S., et al. (2019). Plant stage, not drought stress, determines the effect of cultivars on bacterial community diversity in the rhizosphere of broomcorn millet (Panicum miliaceum L.). Front. Microbio. 10:828. doi: 10.3389/fmicb.2019.00828
Ngumbi, E., and Kloepper, J. (2016). Bacterial-mediated drought tolerance: current and future prospects. Appl. Soil Ecol. 105, 109–125. doi: 10.1016/j.apsoil.2016.04.009
Parry, J. A., Jan, S., Kamili, A. N., Qadri, R. A., Egamberdieva, D., and Ahmad, P. (2016). Current perspectives on plant growth-promoting rhizobacteria. J. Plant Growth Regul. 35, 877–902.
Qaisrani, M. M., Zaheer, A., Mirza, M. S., Naqqash, T., Qaisrani, T. B., Hanif, M. K., et al. (2019). A comparative study of bacterial diversity based on culturable and culture-independent techniques in the rhizosphere of maize (Zea mays L.). Saudi J. Biol. Sci. 26, 1344–1351. doi: 10.1016/j.sjbs.2019.03.010
Rais, A., Shakeel, M., Hafeez, F. Y., and Hassan, M. N. (2016). Plant growth promoting rhizobacteria suppress blast disease caused by Pyricularia oryzae and increase grain yield of rice. BioControl 61, 769–780. doi: 10.1007/s10526-016-9763-y
Ramzani, P. M. A., Khalid, M., Naveed, M., Ahmad, R., and Shahid, M. (2016). Iron biofortification of wheat grains through integrated use of organic and chemical fertilizers in pH affected calcareous soil. Plant Physiol. Biochem. 104, 284–293. doi: 10.1016/j.plaphy.2016.04.053
Riaz, R., Khan, A., Khan, W. J., Jabeen, Z., Yasmin, H., Naz, R., et al. (2021). Vegetable associated Bacillus spp. suppress the pea (Pisum sativum L.) root rot caused by Fusarium solani. Biol. Control 158:104610. doi: 10.1016/j.biocontrol.2021.104610
Schlemper, T. R., Van Veen, J. A., and Kuramae, E. E. (2018). Co-variation of bacterial and fungal communities in different sorghum cultivars and growth stages is soil dependent. Microb. Ecol. 76, 205–214. doi: 10.1007/s00248-017-1108-6
Sharma, N., Khanna, K., Manhas, R. K., Bhardwaj, R., Ohri, P., Alkahtani, J., et al. (2020). Insights into the role of Streptomyces hydrogenans as the plant growth promoter, photosynthetic pigment enhancer and biocontrol agent against meloidogyne incognita in Solanum lycopersicum seedlings. Plants 9:1109.
Tan, Z.-Y., Xu, X.-D., Wang, E.-T., Gao, J.-L., Martinez-Romero, E., and Chen, W.-X. (1997). Phylogenetic and genetic relationships of Mesorhizobium tianshanense and related rhizobia. Int. J. Syst. Evol. Microbiol. 47, 874–879. doi: 10.1099/00207713-47-3-874
Truyens, S., Weyens, N., Cuypers, A., and Vangronsveld, J. (2015). Bacterial seed endophytes: genera, vertical transmission and interaction with plants. Environ. Microbiol. Rep. 7, 40–50. doi: 10.1111/1758-2229.12181
Ullah, H., Yasmin, H., Mumtaz, S., Jabeen, Z., Naz, R., Nosheen, A., et al. (2020). Multitrait Pseudomonas spp. isolated from monocropped wheat (Triticum aestivum) suppress fusarium root and crown rot. Phytopathology 110, 582–592. doi: 10.1094/PHYTO-10-19-0383-R
Upadhyay, N., Singh, V. K., Dwivedy, A. K., Das, S., Chaudhari, A. K., and Dubey, N. K. (2018). Cistus ladanifer L. essential oil as a plant based preservative against molds infesting oil seeds, aflatoxin B1 secretion, oxidative deterioration and methylglyoxal biosynthesis. LWT 92, 395–403. doi: 10.1016/j.lwt.2018.02.040
Valente, J., Gerin, F., Le Gouis, J., Moënne-Loccoz, Y., and Prigent–Combaret, C. (2020). Ancient wheat varieties have a higher ability to interact with plant growth-promoting rhizobacteria. Plant Cell Environ. Microbiol. Rep. 43, 246–260. doi: 10.1111/pce.13652
van der Voort, M., Kempenaar, M., Van Driel, M., Raaijmakers, J. M., and Mendes, R. (2016). Impact of soil heat on reassembly of bacterial communities in the rhizosphere microbiome and plant disease suppression. Ecol. Lett. 19, 375–382. doi: 10.1111/ele.12567
Verma, S. K., Sahu, P. K., Kumar, K., Pal, G., Gond, S. K., Kharwar, R. N., et al. (2021). Endophyte roles in nutrient acquisition, root system architecture development and oxidative stress tolerance. J. Appl. Microbiol. 131, 2161–2177. doi: 10.1111/jam.15111
Volova, T. G., Prudnikova, S. V., and Zhila, N. O. (2018). Fungicidal activity of slow-release P (3HB)/TEB formulations in wheat plant communities infected by Fusarium moniliforme. Environ. Sci. Pollut. Res. 25, 552–561. doi: 10.1007/s11356-017-0466-4
Wildermuth, G., and McNamara, R. (1994). Testing wheat seedlings for resistance to crown rot caused by Fusarium ginearum group 1. Plant Dis. 78, 949–953.
Winter, M., Samuels, P. L., Otto-Hanson, L. K., Dill-Macky, R., and Kinkel, L. L. J. P. J. (2019). Biological control of fusarium crown and root rot of wheat by streptomyces isolates–it’s complicated. J. Phytobiomes J. 3, 52–60. doi: 10.1094/PBIOMES-11-18-0052-R
Yoon, S.-H., Ha, S.-M., Kwon, S., Lim, J., Kim, Y., Seo, H., et al. (2017). Introducing EzBioCloud: a taxonomically united database of 16S rRNA gene sequences and whole-genome assemblies. Int. J. Syst. Evol. Microbiol. 67:1613. doi: 10.1099/ijsem.0.001755
Zahid, M. (2015). Isolation and identification of indigenous plant growth promoting rhizobacteria from himalayan region of Kashmir and their effect on improving growth and nutrient contents of maize (Zea mays L.). Front. Microbiol. 6:207. doi: 10.3389/fmicb.2015.00207
Keywords: Bacillus spp., cropping system, prevalence, root rot, wheat-rice
Citation: Mulk S, Wahab A, Yasmin H, Mumtaz S, El-Serehy HA, Khan N and Hassan MN (2022) Prevalence of Wheat Associated Bacillus spp. and Their Bio-Control Efficacy Against Fusarium Root Rot. Front. Microbiol. 12:798619. doi: 10.3389/fmicb.2021.798619
Received: 20 October 2021; Accepted: 07 December 2021;
Published: 03 March 2022.
Edited by:
Kanika Khanna, Guru Nanak Dev University, IndiaReviewed by:
Javaid Akhter Bhat, Nanjing Agricultural University, ChinaCopyright © 2022 Mulk, Wahab, Yasmin, Mumtaz, El-Serehy, Khan and Hassan. This is an open-access article distributed under the terms of the Creative Commons Attribution License (CC BY). The use, distribution or reproduction in other forums is permitted, provided the original author(s) and the copyright owner(s) are credited and that the original publication in this journal is cited, in accordance with accepted academic practice. No use, distribution or reproduction is permitted which does not comply with these terms.
*Correspondence: Muhammad Nadeem Hassan, bmFkZWVtX2hhc3NhbkBjb21zYXRzLmVkdS5waw==; Humaira Yasmin, aHVtYWlyYS55YXNtaW5AY29tc2F0cy5lZHUucGs=
Disclaimer: All claims expressed in this article are solely those of the authors and do not necessarily represent those of their affiliated organizations, or those of the publisher, the editors and the reviewers. Any product that may be evaluated in this article or claim that may be made by its manufacturer is not guaranteed or endorsed by the publisher.
Research integrity at Frontiers
Learn more about the work of our research integrity team to safeguard the quality of each article we publish.