- 1Department of Nutrition and Public Health, Universidad del Bío-Bío, Chillán, Chile
- 2Science and Research Centre, Faculty of Health Sciences, Palacký University Olomouc, Olomouc, Czechia
- 3Environmental and Public Health Laboratory, Regional Secretariat of the Ministry of Health in Maule, Talca, Chile
- 4Austrian Agency for Health and Food Safety, Institute for Medical Microbiology and Hygiene, Vienna, Austria
- 5Food Quality Testing and Certification Laboratory, Universidad del Bío-Bío, Chillán, Chile
- 6School of Nutrition and Dietetics, Universidad del Bío-Bío, Chillán, Chile
- 7Intestinal Bacteriology Research Laboratory, Hospital Infantil de México Federico Gómez, Mexico City, Mexico
- 8Faculty of Medicine, Biological Sciences Graduate Program, Universidad Nacional Autónoma de México, Mexico City, Mexico
- 9Microbiology and Probiotics Laboratory, Institute of Nutrition and Food Technology, Universidad de Chile, Santiago, Chile
Listeria monocytogenes is causing listeriosis, a rare but severe foodborne infection. Listeriosis affects pregnant women, newborns, older adults, and immunocompromised individuals. Ready-to-eat (RTE) foods are the most common sources of transmission of the pathogen This study explored the virulence factors and antibiotic resistance in L. monocytogenes strains isolated from ready-to-eat (RTE) foods through in vitro and in silico testing by whole-genome sequencing (WGS). The overall positivity of L. monocytogenes in RTE food samples was 3.1% and 14 strains were isolated. L. monocytogenes ST8, ST2763, ST1, ST3, ST5, ST7, ST9, ST14, ST193, and ST451 sequence types were identified by average nucleotide identity, ribosomal multilocus sequence typing (rMLST), and core genome MLST. Seven isolates had serotype 1/2a, five 1/2b, one 4b, and one 1/2c. Three strains exhibited in vitro resistance to ampicillin and 100% of the strains carried the fosX, lin, norB, mprF, tetA, and tetC resistance genes. In addition, the arsBC, bcrBC, and clpL genes were detected, which conferred resistance to stress and disinfectants. All strains harbored hlyA, prfA, and inlA genes almost thirty-two the showed the bsh, clpCEP, hly, hpt, iap/cwhA, inlA, inlB, ipeA, lspA, mpl, plcA, pclB, oat, pdgA, and prfA genes. One isolate exhibited a type 11 premature stop codon (PMSC) in the inlA gene and another isolate a new mutation (deletion of A in position 819). The Inc18(rep25), Inc18(rep26), and N1011A plasmids and MGEs were found in nine isolates. Ten isolates showed CAS-Type II-B systems; in addition, Anti-CRISPR AcrIIA1 and AcrIIA3 phage-associated systems were detected in three genomes. These virulence and antibiotic resistance traits in the strains isolated in the RTE foods indicate a potential public health risk for consumers.
Introduction
Ready-to-eat (RTE) foods are defined as any food in a raw state or one that is handled, processed, mixed, cooked, or prepared and is consumed without any further processing (Monteiro, 2010). The RTE foods are a practical alternative to meet daily food needs; however, they are not exempt from contamination by biological hazards such as Salmonella spp., pathogenic Escherichia coli, and Listeria monocytogenes (Becker et al., 2019).
Listeria monocytogenes is a Gram-positive facultative anaerobic, ubiquitous, and persistent bacterium in food processing plants. Due to inadequate hygiene and manufacturing practices, this pathogen contaminates foods such as fresh or frozen fruits and vegetables, unpasteurized dairy products, sausages, and fish (Amajoud et al., 2018). Cheeses, sausages, meats, and fish are the most frequently associated with outbreaks of this pathogen worldwide (Kurpas et al., 2018; Ryser, 2021). L. monocytogenes causes listeriosis, a disease characterized by low morbidity but high mortality in those who are infected, and the most at risk groups are pregnant women, newborns, children, and older adults (Schlech, 2019). In Europe and North America, invasive listeriosis affects 0.3–0.6 persons per 100,000 inhabitants annually (Maertens de Noordhout et al., 2017; European Centre for Disease Prevention and Control [ECDC], 2018). In Chile, L. monocytogenes has been under mandatory laboratory notification and surveillance since 2005 (Bustamante et al., 2020). There were 97 cases in 2018 and 69 in 2019 with lethality of 22% and 26%, respectively (MINSAL: Ministerio de Salud Chile, Departamento de Estadísticas e Información en Salud [DEIS], 2019). Adults aged 65 and older and pregnant women were the most affected groups. Pregnancy was terminated in 50% of cases and abortion or fetal death was reported in 21% (MINSAL: Ministerio de Salud Chile, Departamento de Estadísticas e Información en Salud [DEIS], 2019). The RTE foods were the main source of infection associated with these listeriosis cases (MINSAL: Ministerio de Salud Chile, Departamento de Estadísticas e Información en Salud [DEIS], 2019).
The severity of L. monocytogenes infection is associated with several factors such as infecting dose, host immunity, and expression of virulence factors (adherence, invasion, immune modulator, intracellular survival, toxins, and mobile genetic elements), and the presence of CRISPR-Cas as a virulence regulator (Falavina dos Reis et al., 2011; Louwen et al., 2014; Pouillot et al., 2016; Buchanan et al., 2017; Kwon et al., 2020). In addition, there are other factors such as resistance to disinfectants and antibiotics, especially beta-lactams (Olaimat et al., 2018). The capacity to resist to adverse environmental conditions (heat and cold stress) allow the persistence and colonization throughout the food chain by forming contamination reservoirs that are difficult to control (Bolocan et al., 2016; Bucur et al., 2018). The Listeria species can be categorized into different serotypes according to the serological reactions of the listerial somatic antigen (O-antigen) and flagellar antigen (H-antigen) with specific antisera. L. monocytogenes can be classified into at least 13 serotypes (Orsi et al., 2011), three of them (1/2a, 1/2b, and 4b) are involved in over 95% of human clinical cases, and serotype 4b exhibits the strongest epidemiological association with human listeriosis (Maury et al., 2016; Lee et al., 2018).
Whole-genome sequencing (WGS) currently allows in silico generation of a wealth of information about pathogenic strains, including a more precise description of the taxonomic differences and similarities between them. The WGS technology is used to more precisely identify the pathogen and genotype it by multilocus sequence typing (MLST), clonal complex (CC) determination, core genome MLST (cgMLST), CRISPR-Cas, and serogrouping. WGS also enables the detection of antibiotic resistance and virulence genes, plasmids, and mobile genetic elements (MGEs); this information provides a more precise epidemiological relationship (Leopold et al., 2014; Ruppitsch et al., 2015a; Moura et al., 2017; Hurley et al., 2019; Kwon et al., 2020; Stessl et al., 2021). The use of WGS has been fundamental in the successful investigation of recent extensive outbreaks of L. monocytogenes in South Africa (2017–2018) and Germany (2018–2019) (Allam et al., 2018; Halbedel et al., 2020).
According to Chilean health authorities, RTE foods are the main source of infection associated with cases of this disease in Chile (MINSAL: Ministerio de Salud Chile, Departamento de Estadísticas e Información en Salud [DEIS], 2019). However, information about the diversity, pathogenicity, and virulence of L. monocytogenes in Chile is still limited and incomplete. Our study contributes to a better understanding of L. monocytogenes with respect to genotype diversity, virulence, antibiotic resistance, and cas genes by generating necessary and indispensable scientific evidence. Many of the evaluated foods in this study are marketed in the Americas. Therefore, given the need for updated information on this pathogen in Chile, we studied virulence factors and antibiotic resistance in L. monocytogenes strains isolated from RTE foods by in vitro and in silico testing using whole-genome sequencing (WGS).
Materials and Methods
Samples
A total of 436 samples of retail RTE foods were analyzed; these are regarded as at risk foods sampled as part of sampling plan the Emerging Pathogens Program of the Health Authority in the Maule Region, Chile, and which are regulated by the Chilean Food Sanitary Regulations (RSA). Samples used for our study consisted of cheeses (n = 161), cooked meats (artisanal ham, pâté, sausages, and blood sausage) (n = 186), pre-processed fruits and vegetables (chopped fruit, fruit salads with strawberries, melon, and peaches, and leafy vegetable salads) (n = 22), and meals and mixed dishes with raw and/or cooked ingredients (n = 67).
Isolation of Listeria monocytogenes
Isolation was performed on the basis of the ISO 11290–1:2017 standard. Each 25 g food sample was inoculated in 225 mL half Fraser broth (Oxoid, Basingstoke, United Kingdom) as primary selective enrichment and homogenized in a stomacher (Seward 400, Radnor, PA, United States). Incubation was performed at 30 ± 1°C for 25 ± 1 h; the second enrichment consisted of 0.1 mL of the broth culture inoculated in 10 mL of full-strength Fraser broth, which was cultured at 37°C for 24 ± 2 h. A loopful of each of the half- and full-strength Fraser broths were plated on the Listeria chromogenic agar base according to Ottaviani and Agosti (ALOA) (Merck, Darmstadt, Germany). These plates were incubated at 37°C for 24–48 h. Five typical colonies from each ALOA agar plate were restreaked on tryptic soy agar supplemented with 0.6% yeast extract (TSA-YE) (Sigma, Darmstadt, Germany) as a non-selective medium, and these were incubated at 37°C for 24–48 h. Colonies from the TSA-YE were verified by Gram staining, catalase reactions, oxidase tests, carbohydrate utilization test, CAMP tests, and motility at 20–25°C. These colonies were stored for further study.
Detection of Listeria monocytogenes
Detection of L. monocytogenes was performed with the Vitek Immunodiagnostic Assay System (VIDAS) (bioMerieux Vitek Inc., Hazelwood, MO, United States) according to the manufacturer’s instructions. The equipment automatically measured and interpreted data, reporting detection as positive or negative according to the validated AOAC (Official Method of Analysis No. 2004.2) protocol for food matrices.
Whole-Genome Sequencing
Prior to WGS, a primary species identification from single colonies was performed by matrix-assisted laser desorption ionization time-of-flight mass spectrometry (MALDI-TOF MS) (Bruker, Billerica, MA, United States) and MBT Compass IVD software 4.1.60 (Bruker) as described by Halbedel et al. (2020).
As for WGS, DNA was isolated from bacterial cultures with the MagAttract HMW DNA Kit (Qiagen, Hilden, Germany) according to the manufacturer’s instructions for Gram-positive bacteria. The amount of input DNA was quantified on a Lunatic instrument (Unchained Labs, Pleasanton, CA, United States). Nextera XT chemistry (Illumina Inc., San Diego, CA, United States) was used to prepare sequencing libraries for a 300 bp paired-end sequencing run on an Illumina MiSeq sequencer. Samples were sequenced to achieve a minimum 80-fold coverage using recommended standard protocols by Illumina. The resulting FASTQ files were quality trimmed and de novo assembled with the SPAdes version 3.9.0. Contigs were filtered for a minimum of fivefold coverage and 200 bp minimum length with SeqSphere+ software v. 7.8.0 (Ridom, Münster, Germany) (Jünemann et al., 2013).
Serotype, Sequence Type, and Core Genome Multilocus Sequence Typing of Listeria monocytogenes
From the WGS of the L. monocytogenes strains, serotypes were determined by the sequence-specific extraction of targets using the L. monocytogenes 5-plex PCR Serogroup task templates of the SeqSphere+ v. 7.8.0 (2021-7) software with fragments from five DNA regions (lmo118, lmo0737, ORF2110, ORF2829, and prs as an internal amplification control) previously described by Doumith et al. (2004) and Lee et al. (2012).
The sequence type (ST) was determined with Task templates for available MLST schemes from the SeqSphere+ v. 7.8.0 (2021-7) software (Jünemann et al., 2013). The ST was confirmed in the strains with fragments from the seven housekeeping genes abcZ, bglA, cat, dapE, dat, Idh, and ihkA (Ruppitsch et al., 2015a; Moura et al., 2016) and with the profiles from the Institut Pasteur MLST Listeria database1.
The cgMLST was performed on the basis of the profile of 1,701 loci of cgMLST complex types (CTs) (Ruppitsch et al., 2015a) with Task templates for SeqSphere+ v. 7.8.0 (2021-7). We defined a cgMLST cluster as the group of isolates with less than10 different alleles among the studied strains. We used SeqSphere in the mode that ignored pairwise missing values and an unweighted pair group method with arithmetic mean to generate phylogenetic trees (Halbedel et al., 2020).
Antibiotic Resistance Profile
The disk diffusion method was applied based on the recommendations of the Clinical and Laboratory Standards Institute (Clinical and Laboratory Standards Institute [CLSI], 2018). The commercial antibiotic disks included 10 μg ampicillin (AMP), 10 μg penicillin (PEN), 25 μg sulfamethoxazole-trimethoprim (STX), 15 μg erythromycin (ERY), 30 μg vancomycin (VAN), tetracycline (TET) 30 μg, ciprofloxacin (CIP) 5 μg, and 30 μg chloramphenicol (CHL). The resistance/susceptibility profiles of the strains were characterized by measuring the zone of inhibition and interpreting the inhibition diameters according to the manufacturer’s instructions; Streptococcus pneumoniae ATCC 49619 was used as a reference. In addition, E. coli ATCC 25922 and L. monocytogenes ATCC 7644 were used as controls.
Virulence Genes Amplification
The method described by Aznar and Alarcón (2002) was used to amplify conserved regions of the three characteristic virulence genes listeriolysin O (hlyA) (Border et al., 1990), positive regulatory factor A (prfA) (Klein and Juneja, 1997), and internalin A (inlA) (Montero et al., 2015). The genomic DNA of the suspected strains was extracted and purified with the UltraClean Microbial DNA Isolation Kit (Mo Bio Laboratories, Qiagen, Carlsbad, CA, United States) and mixed with GoTaq Green Master Mix (Promega, Madison, WI, United States) in a thermocycler (Fermelo Biotec, China). Using an agarose gel imaging system, the amplified products were stained and visualized on 1.5% agarose gel with a 1.0 mg/mL ethidium bromide solution.
In silico Detection of Virulence and Antibiotic Resistance Genes
Virulence genes were established with the task template VFDB 2.0 feature in SeqSphere+ for WGS data (Chen et al., 2016). Thresholds were set for the target scanning procedure as a required identity ≥ 90% with the reference sequence and an aligned reference sequence ≥ 99%. The Comprehensive Antibiotic Resistance Database (CARD) was used with the default “perfect” and “strict” settings for the sequence analysis of antimicrobial resistance genes (Jia et al., 2017). The Task Template AMRFinderPlus 3.2.3 available in the Ridom SeqSphere+ 7.8.0 software was used with the EXACT method at the 100% setting together with the BLAST alignment of protein sequences against the AMRFinderPlus database (Feldgarden et al., 2019).
In silico Detection of Plasmids and Mobile Genetic Elements
The PlasmidFinder 2.1 and MobileElementFinder 1.0 tools were used to detect plasmids and MGEs. The selected minimum identity was 95 and 90%, respectively2 (Carattoli et al., 2014; Johansson et al., 2021).
Bioinformatic Search of CRISPR-Cas Loci
The search for and characterization of CRISPR arrays and their association with Cas proteins was determined with CRISPRCasFinder and CRISPRminer (Couvin et al., 2018; Zhang et al., 2018), which are available at https://crisprcas.i2bc.paris-saclay.fr and http://www.microbiome-bigdata.com/CRISPRminer. The following parameters were used: 18–55 bp repeated sequence length, 25–60 bp spacer length, 0.6–2.5 spacer sequence size as a function of repeated sequence size, and 60% maximum percentage similarity between spacers. Phages associated with sequence spacers were also determined with the CRISPRminer program (Zhang et al., 2018).
The CRISPR systems were determined with the CRISPRmap program (Lange et al., 2013). The CRISPRTarget program was used to determine the protospacer adjacent motif (PAM) sequences associated with each repeated sequence of the identified arrays.
Results
Prevalence of Listeria monocytogenes
In total 3.1% (14/436) of samples were positive for L. monocytogenes when using the VIDAS system. In terms of food group, the highest positivity, 36% (8/22), occurred in pre-processed fruits and vegetables, followed by 5.9% (4/67) for prepared meals and dishes, 1.1% (2/186) for cooked meats, and 0% (0/161) for cheese and fresh cheese (Table 1).
Fourteen strains were isolated from the positive samples and confirmed as L. monocytogenes by MALDI-TOF MS.
Core Genome Multilocus Sequence Typing, Sequence Type, Complex Type, Clonal Complex, and Serotype From Whole-Genome Sequencing of Listeria monocytogenes
Whole-genome sequencing using cgMLST grouped the strains into two clusters and nine unrelated complex types (CTs) (Figure 1). In addition, the 14 strains were grouped in 10 STs and 9 CCs using average nucleotide identity (ANI), ribosomal MLST, and cgMLST complex type (CT).
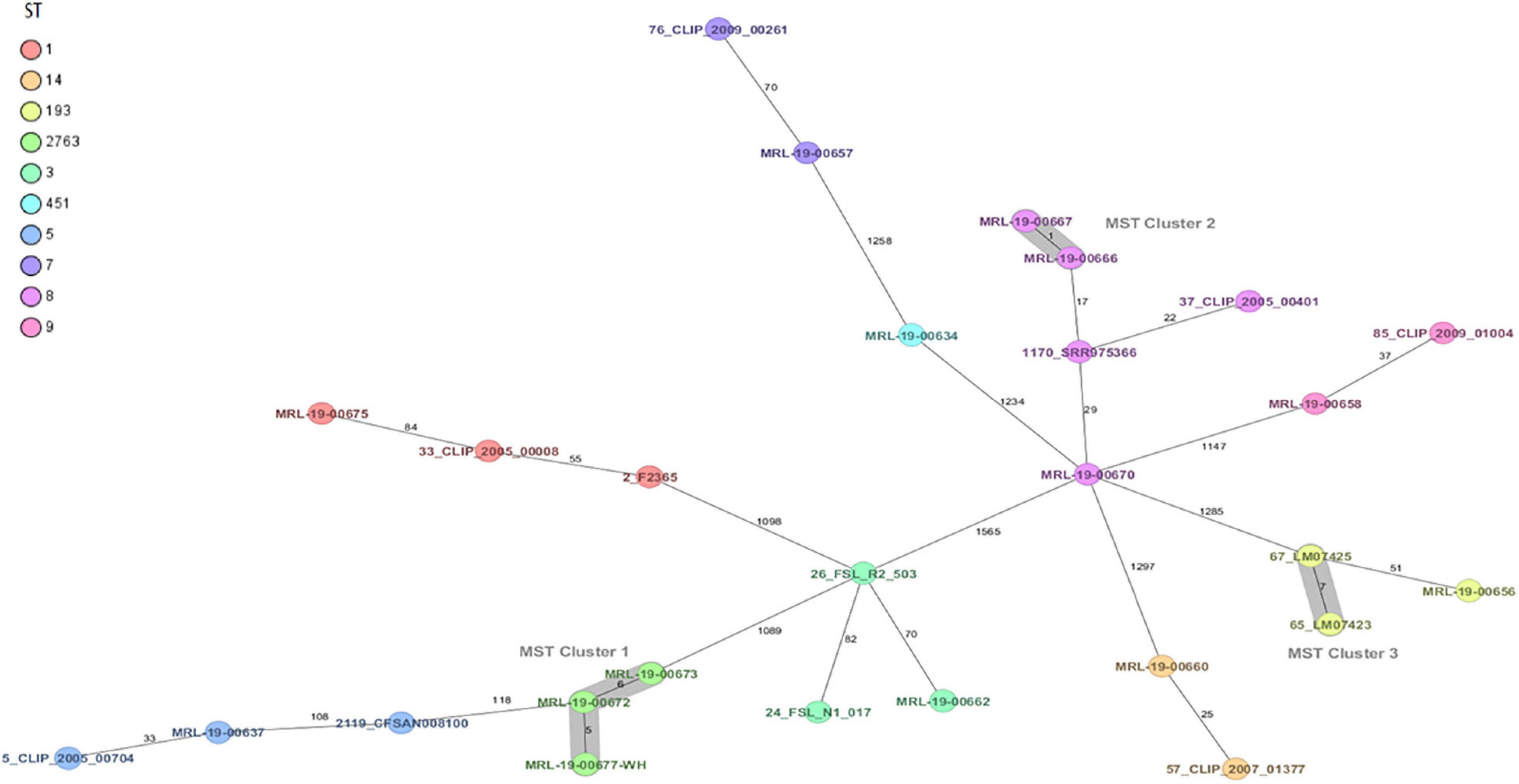
Figure 1. Minimum spanning tree (MST) of 14 Listeria monocytogenes strains from ready-to-eat foods isolated in Chile. L. monocytogenes strains with ST1, ST3, ST5, ST7, ST8, ST9, ST14, ST193, ST451, and ST2763 are of clinical and food origin. Calculation of the MST is based on the defined core genome multilocus sequence typing (cgMLST) scheme consisting of 1,701 target genes from Task templates for SeqSphere+ v. 7.8.0 (2021-7). Isolates are represented as colored circles according to the classical MLST. Black numbers are in accordance with the allelic difference between isolates. Isolates with closely related genotypes are marked as Cluster.
The dominant serotype was 1/2a in seven strains, of which three strains were identified as ST8 (CC8, CT8068, and CT8004), one ST7 (CC7 and CT8064), one ST14 (CC14 and CT8065), one ST193 (CC193 and CT8063), and one ST 451 (CC11 and CT4117).
Furthermore, the serotype 1/2b was found in five strains, one ST3 (CC3 and CT8066), one ST5 (CC5 and CT8052), and three new STs. One strain showed serotype 4b (ST1, CC1, and CT 8007), and one strain was serotype 1/2c (ST9 (CC9 and CT5231) (Table 2). A new ST was identified as ST2763 (CC5 and CT8006) in the three strains, and was uploaded to the Institute Pasteur MLST Listeria database.
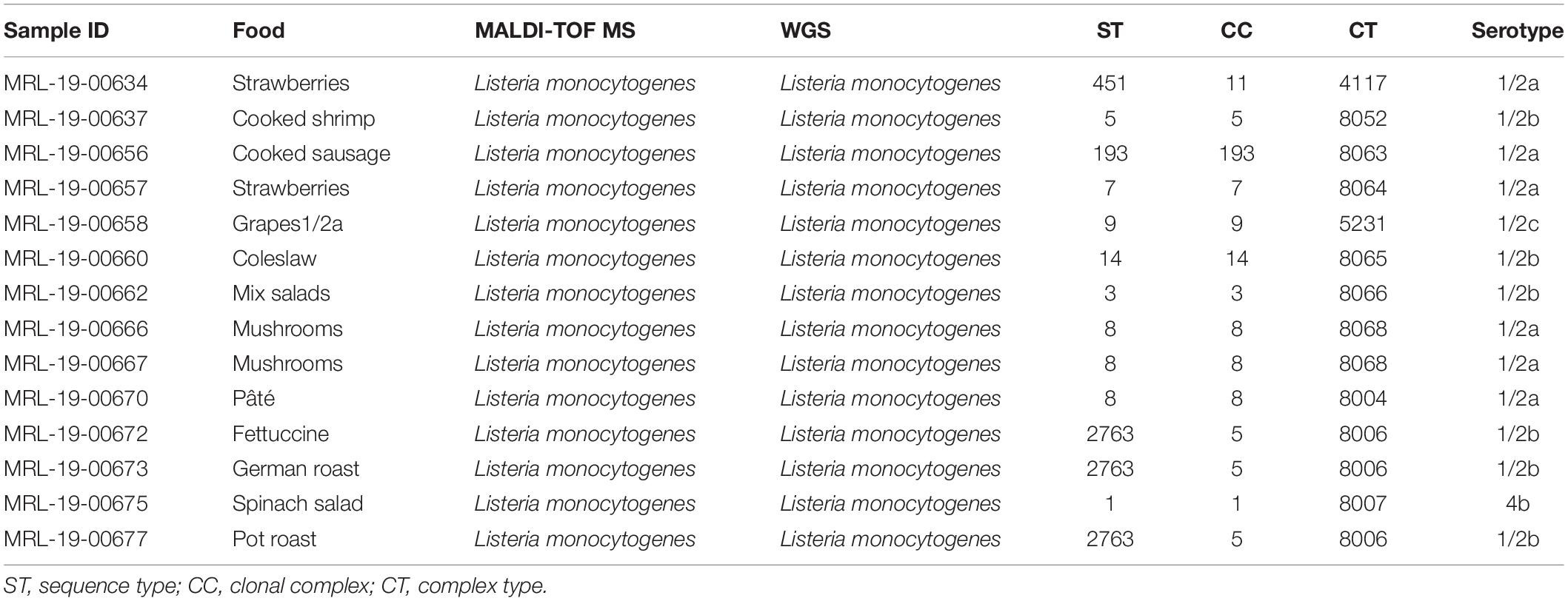
Table 2. Identification of L. monocytogenes strains by matrix-assisted laser desorption ionization time-of-flight mass spectrometry (MALDI-TOF MS) and whole-genome sequencing (WGS).
Antibiotic Resistance Profile and in vitro Detection of Virulence Genes
Most of the strains 78% (11/14) were susceptible to all the antibiotics; only the MRL-19-00656, MRL-19-006573, and MRL-19-00662 strains were resistant to ampicillin.
Regarding the virulence genes, all strains in the present study amplified hlyA, prfA, and inlA genes (Table 3).
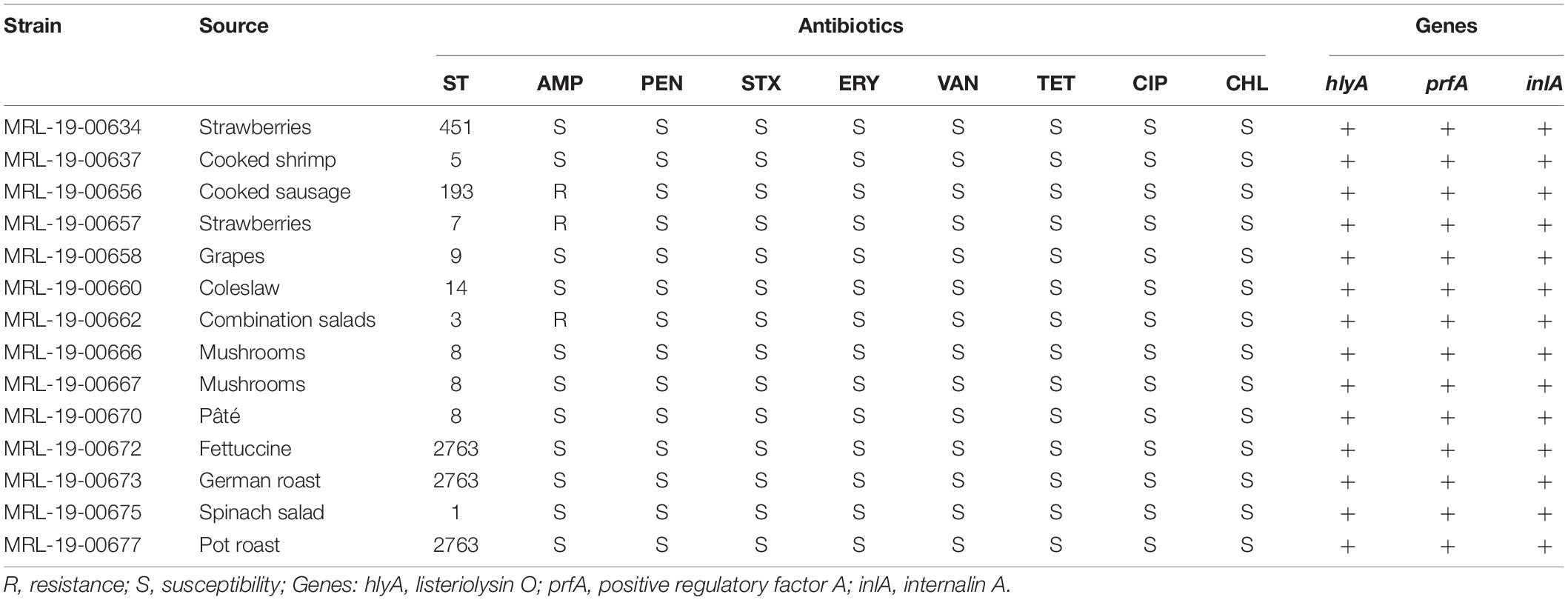
Table 3. Detection of putative virulence genes and antibiotic resistance profile of L. monocytogenes strains.
In silico Detection of Virulence and Antibiotic Resistance Genes
The virulence factor database (VFDB) was used to evaluate the 33 major virulence gene. All the strains had the following genes: bsh (bile resistance), clpCEP (stress protein), hly (toxin-listeriolysin O precursor), hpt (metabolic adaptation), iap/cwhA, inlA, inlB, and ipeA (invasion), lspA (peptidase), mpl, plcA, plcB (exoenzyme), oat, pdgA (immune evasion), and prfA (regulation) (Figure 2). Only the MRL-19-00675 genome strain exhibited the Listeria pathogenicity island 3 (LIPI-3). A mutation in position 2054 (G:A) of inlA gene was found in the MRL-19-00658 strain, known as premature stop codons (PMSC) type 11. A new mutation in position 819 of the inlA gene was encountered in the MRL-19-00662 strain, which was not identified because this mutation (deletion of A) has not yet been described in the literature or in the inlA PMSC profiles of the Institut Pasteur MLST Listeria database (see Text Footnote 1).
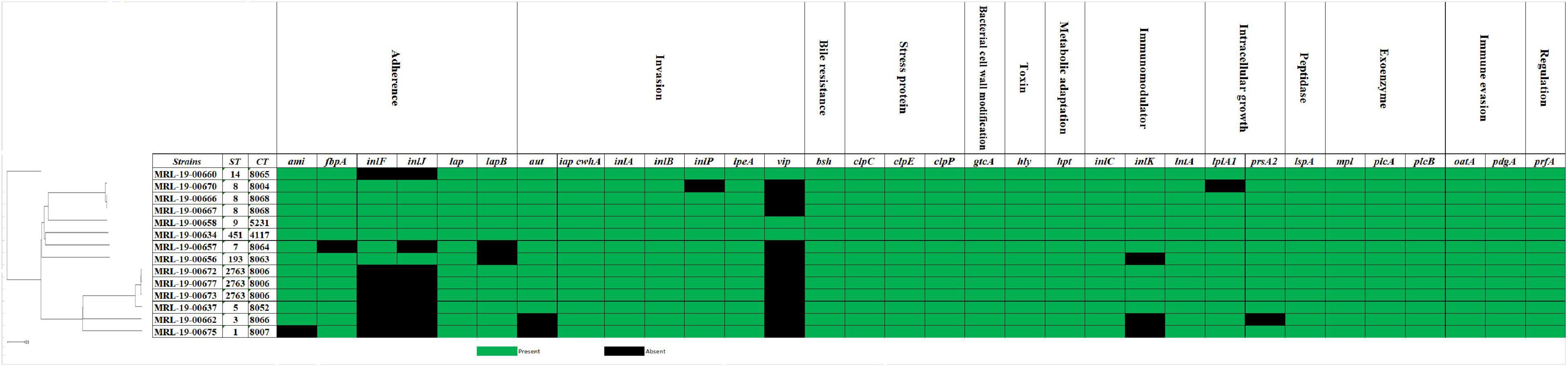
Figure 2. Distribution of virulence genes present in 14 L. monocytogenes strains isolated from ready-to-eat foods. Green boxes indicate the presence of the gene and black boxes its absence.
Furthermore, genes associated with biofilm formation such as cheY, inlL, prfA, actA, lmo0673, and lmo2504 were identified in all the strains; these genes play an important role in the survival and persistence of L. monocytogenes. The bapL, recO, and luxS genes were not found in any strain. The antimicrobial fosX, lin, norB, and mprF resistance genes were identified in all L. monocytogenes strains. These genes confer resistance to fosfomycin, lincosamides, quinolones, and cationic peptides that disrupt the cell membrane such as defensins. Regarding the genes that confer resistance to tetracycline, the tetA and tetC genes were detected and tetM and tetS were absent in all the strains. In addition, the arsBC and bcrBC genes were identified in all strains, which confer resistance to stress, and the clpL gene, which confers resistance to disinfectants.
Detection of Plasmids and Mobile Genetic Elements
Plasmids were found in 85% (12/14) of the strains. Inc18(rep25) was detected in eight strains, Inc18(rep26) in three, and N1011A in one. In addition, the ST2763 harbored the Inc18(rep25) and rep3(rep32) plasmids (Table 4).
The MGEs (insertion sequences, transposons) were found in only nine strains. The most frequent MGEs were ISLmo3, ISLmo5, ISLmo7, ISLmo9, ISLmo8, ISS1N, cn_8625_ISS1N, CN_12410_ISS1N, and CN_8566_ISS1N (Supplementary Table 1).
CRISPR-Cas Loci
Genome analysis showed the presence of CRISPR-Cas systems in 71% (10/14) of the genomes. These systems consist of at least one array; however, between two and five arrays can be observed in 50% (5/10) of the genomes in different positions. The arrays had among 3 repeated sequences and 2 spacers and up to 28 repeated sequences and 27 spacers (Table 5).
Using the CRISPRmap program, the repeated sequences and the associated cas genes enabled to determine the identified CRISPR systems to type II-B (Figure 3 and Table 1). In two of the arrays, only one cas-associated gene was identified; in contrast, the rest of the genomes showed sequences that encoded up to 16 proteins associated with these CRISPR-Cas systems.
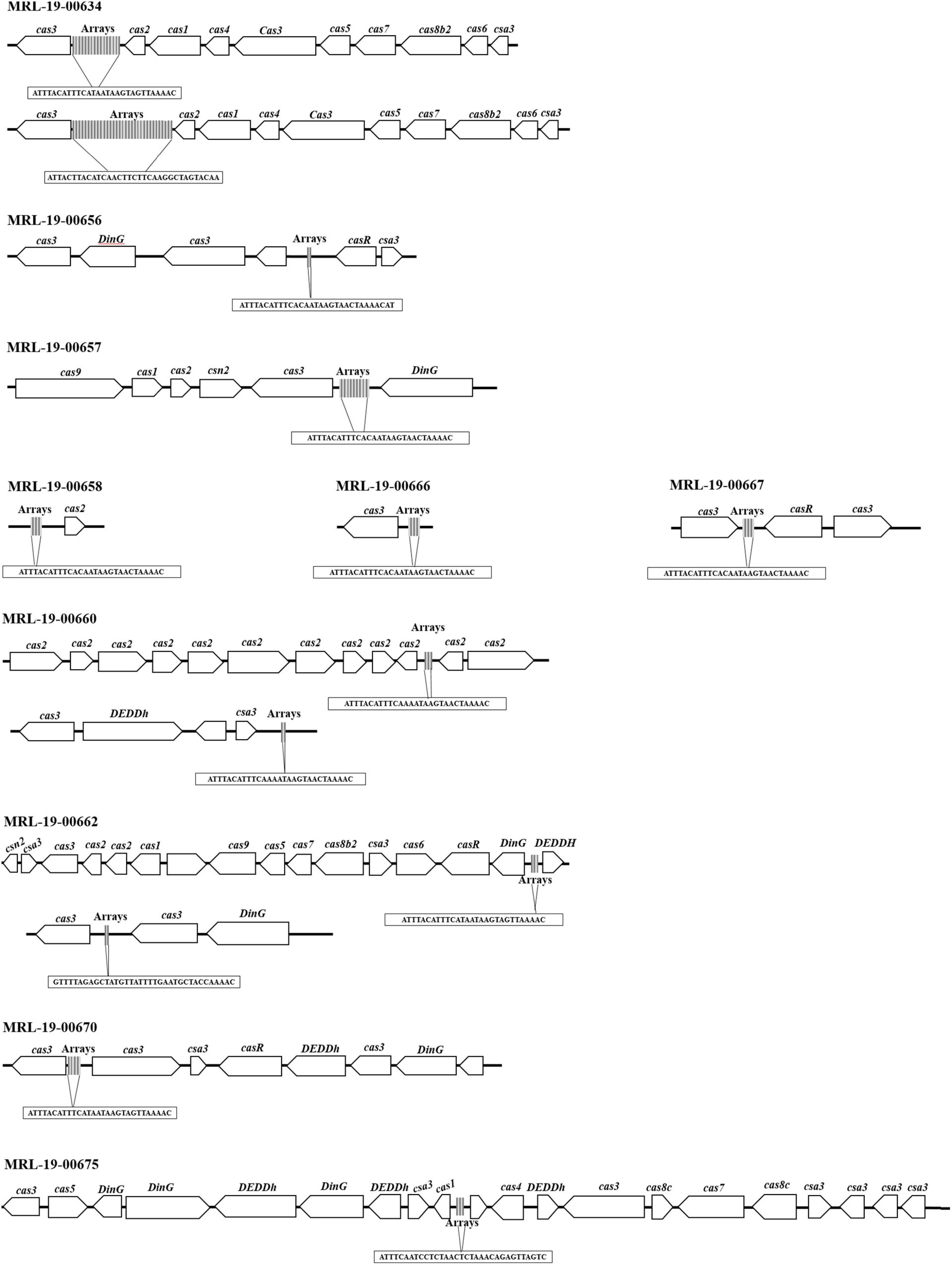
Figure 3. CRISPR-Cas systems identified in L. monocytogenes genomes. The identified systems belong to the CRISPR-Cas type II-B system, and some genomes show more than one array.
The analysis of the spacer and PAMs enabled us to associate them to sequences corresponding to different bacteriophages, which are associated with the Listeria genus (Supplementary Tables 2, 3).
The bioinformatics analysis of the genomes enabled the detection of protein sequences associated with the AcrIIA1 and AcrIIA3 Anti-CRISPR systems in the three studied genomes MRL-19-00657, MRL-19-00658, and MRL-19-00660 (Table 6), which are associated with phages present in these genomes.
Discussion
Listeria monocytogenes persists as a relevant public health and food safety risk due to its ubiquity, persistence under adverse environmental conditions, and pathogenicity (Hurley et al., 2019; Chen et al., 2020a).
In the present study, general positivity for L. monocytogenes in RTE foods was 3.1% (14/436). Positivity for L. monocytogenes in RTE foods in different countries has been reported as 5.5% in China (Li et al., 2018), 7.5% in Chile (Bustamante et al., 2020), 11.9% in Uruguay (Braga et al., 2017), 8.5% in Turkey (Sanlibaba et al., 2018), and 13.5% in Poland (Szymczak et al., 2020). Bustamante et al. (2020) reported prevalence values of 17.5, 8.6, and 8.5% in prepared meals and dishes, pre-processed fruits and vegetables, and cooked meats, respectively. Furthermore, positivity for L. monocytogenes was 0% in dairy products and cheeses, which concurs with the present study, and this situation is noteworthy because dairy products and cheeses have been associated in recent years with many outbreaks in Europe and the United States (Fretz et al., 2010; Amato et al., 2017; Martínez-Rios and Dalgaard, 2018; Churchill et al., 2019). Therefore, a better understanding of the ecology and biology of L. monocytogenes that focuses on virulence factors and stress response would further improve the control of this important foodborne pathogen (Ryser, 2021).
The L. monocytogenes strains in the present study revealed that ST8 was the most prevalent ST from samples of RTE vegetables and pork pâté. The ST8 has been found in different RTE foods such as meats, salmon, cooked meats, fried rice and noodles, and vegetables (Wang et al., 2015; Ziegler et al., 2018; Chen et al., 2020b). In addition, ST8 has been responsible for cases of human listeriosis in Canada, Italy, Switzerland, and Germany (Knabel et al., 2012; Mammina et al., 2013; Althaus et al., 2014; Ruppitsch et al., 2015b; Halbedel et al., 2020), and it has been considered to have high pathogenic potential (Fagerlund et al., 2016). Different authors state that L. monocytogenes ST 8 is one of the most persistent STs in RTE food processing plants; hence, there is a permanent risk of food recontamination by this pathogen (Knudsen et al., 2017). The second most prevalent was ST2763 (CC5), which is a new ST found in the present study and isolated in meats and RTE prepared dishes. There was a diverse distribution of the other STs, including ST1, ST3, ST5, ST7, ST9, ST14, ST193, and ST451, which have been isolated in outbreaks, clinical cases, and different foods (Althaus et al., 2014; Amajoud et al., 2018; Cabal et al., 2019; Ulloa et al., 2019; Halbedel et al., 2020). Six of the fourteen L. monocytogenes strains belonged to serogroup IIa (serotype 1/2a; ST7, ST8, ST193, and ST451), six to serogroup IIb (serotype 1/2b; ST3, ST5, ST14, and ST2763), one to serogroup IVb (serotype 4b; ST1), and one to serogroup IIc (serotype 1/2c; ST9). These four serotypes have been associated with more than 98% of reported cases of listeriosis worldwide (Gorski, 2021).
The treatment for listeriosis includes antibiotics such as ampicillin, tetracyclines, amoxicillin, and sulfamethoxazole (Thønnings et al., 2016). In the present study, 11 isolates were susceptible to all the antibiotics, while only 3 exhibited resistance to ampicillin. This is a cause for concern because previous reports in Chile have indicated the susceptibility of L. monocytogenes to ampicillin and also because ampicillin and amoxicillin are currently used to treat this infection (Seoane, 2013; Kumaraswamy et al., 2018). Several authors have encountered resistance to ampicillin in L. monocytogenes strains isolated from raw and cooked meats and fish products with a prevalence between 6 and 83% (Yucel et al., 2005; Jamali et al., 2013; Arslan and Baytur, 2019; Bustamante et al., 2020; Maćkiw et al., 2020). Emerging resistance to penicillin in clinical strains poses a major public health concern because penicillin is the standard treatment for human listeriosis (Martínez et al., 2001). Therefore, the fact that we detected ampicillin-resistant L. monocytogenes strains in the present study should alert authorities and food manufacturers to the latent risk associated with the consumption of these RTE foods contaminated by this pathogen.
We found the presence of resistance genes with mechanisms of antibiotic efflux (norB), antibiotic target alteration (mprF), and antibiotic inactivation (lin, fosX). In addition, our study reported genes that confer resistance to tetracycline (tetA and tetC). Wilson et al. (2018) reported that all strains displayed the resistance gene to fosfomycin (fosX); however, they did not detect any genes associated with tetracycline (tetA) or erythromycin (ermABC). This differs from our study in which the fosX and tetA genes were identified. Mafuna et al. (2021) encountered resistance genes in strains such as fosX, lin, mprF, and norB, and they reported an increasing global trend of resistance genes present in the food chain. L. monocytogenes is currently considered to be intrinsically resistant to fosfomycin because of the lack of expression in the membrane transport systems and a natural resistance to lincomycin due to the ribosomal protection of an ATP-binding cassette F (ABC-F) protein (Mota et al., 2020).
However, there was a difference in our study between the prediction of resistance genes and antibiotic susceptibility testing, which is due to the existence of intrinsic resistance according to some authors (Cox and Wright, 2013); in addition, the resistance genes are ancient and predate the use of antibiotics (Kashuba et al., 2017; Peterson and Kaur, 2018). Gygli et al. (2019) reported that the possible discrepancy between gene detection by WGS and antibiotic susceptibility testing of Mycobacterium tuberculosis strains could arise because the clinical concentrations established to classify it as resistant have cutoff scores that are too high, thus misclassifying strains as susceptible. Aljahdali et al. (2020), found a positive concordance between the presence of resistance genes and resistance phenotypes in various in Salmonella strains; however, they also observed that some strains with beta-lactamase resistance genes were still phenotypically susceptible to amoxicillin-clavulanic acid. Therefore, the difference between genotype and phenotype in these strains could be due to exceptional mutations that reduce gene expression and can confer susceptibility to antimicrobial agents used in susceptibility tests. With the discovery of varied antimicrobial resistance genes and gene transfer mechanisms, non-genetic mechanisms mediated by small molecules can alter the phenotypic susceptibility to antibiotics of bacterial cells (El-Halfawy and Valvano, 2012).
The presence of resistance genes to quaternary ammonium, stress, and biofilm formation have been described as key factors for the adaptation and survival in food processing plants (Horlbog et al., 2018). We identified the bcrBC cassette in three ST2763 strains and one ST5 strain, which were all serotype 1/2b associated with persistence and resistance to benzalkonium chloride, a common disinfectant used in the food industry (Cooper et al., 2021). In addition, the clpL gene was detected in one ST3 strain and one ST9 strain both isolated from RTE vegetables. This gene has been identified as an important predictor of heat resistance of L. monocytogenes (Pöntinen et al., 2017).
All our strains amplified the three evaluated virulence factors in vitro and confirmed by detection of these genes in silico. Among these strains, the most studied virulence genes are hlyA, prfA, and inlA. The hlyA gene encodes listeriolysin O, which allows pore formation for pathogen entry into the cells; it is only present in virulent species of Listeria spp. and is widely used to assess the presence of the virulence factor in L. monocytogenes isolated in RTE foods (Churchill et al., 2006; Abdollahzadeh et al., 2016). The PrfA protein is indispensable for virulence gene expression (including the prfA gene) in pathogenic species of L. monocytogenes, and it depends on environmental conditions such as high temperature and stress (Aballa et al., 2019). Internalin A (inlA) was found in all the studied isolates and is considered relevant because it participates in the adherence process between the bacteria wall and the intestinal cells (Drolia and Bhunia, 2019). Recent studies have focused on the presence of premature stop codons (PMSC) in the inlA gene, which results in impaired virulence; this type of mutation is more frequent in food isolates than in clinical cases (Van Stelten et al., 2010; Ferreira da Silva et al., 2017). Only one isolate in our study showed a PMSC with a type II mutation (G:A) in position 2054, generating a truncated internalin of 684 amino acids as describe recently (Van Stelten and Nightingale, 2008). However, we detected a new mutation in the MRL-19-00662 strain (ST3, CC3, and serotype 1/2b) in position 819 (deletion of A), resulting in a truncated internalin of 273 amino acids, which has not yet been reported in the literature. Nine strains in our study have pathogenic potential; it was confirmed that the prevalent STs, such as ST1 (CC1) and ST9 (CC9), are associated with listeriosis outbreaks in Chile and have persisted over time (Cantinelli et al., 2013; Montero et al., 2015; Toledo et al., 2018; Cabal et al., 2019; Ulloa et al., 2019).
A characteristic trait of Listeria plasmids is the presence of many MGEs encoding transposases, such as insertion sequences (IS) and transposons, and other recombinases that are determinants in the dissemination of adaptive foreign DNAs and resistance (Kuenne et al., 2010). The most common plasmids were inc18(rep25), inc18(rep26), and rep3(rep26), and only one strain showed N1-011A. The plasmid incompatibility group inc18 is naturally found in Streptococcus and Enterococcus spp. (Zhu et al., 2010) and encodes a variety of resistance to antibiotics due to their overuse in environmental and food settings (Kohler et al., 2018). In addition, plasmid N1-11A has been found in RTE seafood processing plants in France and in the food chain in South Africa: it is associated with the resistance to disinfectants such as benzalkonium chloride (Mafuna et al., 2021).
CRISPR-Cas systems are acquired immunity systems that allow bacteria and archaea to acquire exogenous material from bacteriophages and plasmids (Hupfeld et al., 2018). The CRISPR-Cas systems is a possible involved in the regulation of gene expression, including virulence genes, which have been described in a number of pathogens (Louwen et al., 2014). It was possible to determine that the repeated sequences and associated cas genes in the studied L. monocytogenes strains corresponded to type II-B systems and that the presence of the cas8b and cas9 genes allowed their classification in subtype B. However, the arrays could be related to one cas gene, likewise in the systems that only show sequences that encode for cas3 and cas2. Kuenne et al. (2013) studied CRISPR-Cas in three different loci of L. monocytogenes strains. CRISPR-Cas locus 1 was characterized by a single CRISPR matrix, locus 2 belonged to type I-B, and locus 3 was classified as type II-A. CRISPR-Cas locus 1 was previously found as being associated with the presence of a tracrRNA, which is suggested to control virulence in L. monocytogenes strain 1/2a EGD-e during growth in macrophages; however, it is still unknown how this track RNA could control virulence (Mraheil et al., 2011). Louwen et al. (2013) showed that the ability to translocate through intestinal walls was suppressed when deleting cas9 in Campylobacter jejuni isolates, which affected virulence. The same authors reported that supplementing C. jejuni isolates with cas9, which does not have a CRISPR-Cas system, significantly increased virulence in this pathogen This can also be associated with the array size because those in which these genes are absent have smaller arrays.
As for the spacer sequences, they provide us with the history of the invasive elements to which the bacterium has been subjected because these sequences are associated with exogenous material. For arrays identified in the present study, spacers were related to sequences corresponding to bacteriophages that specifically infected the Listeria genus. Therefore, those bacteria that have this information are able to evade infection by these bacteriophages, unlike those that do not. The phages have also been able to develop strategies in response to CRISPR-Cas, such as the Anti-CRISPR proteins, which were identified in the genomes under study. It has been determined for L. monocytogenes that the prophages show anti-Cas9 proteins such as AcrIIA1, which successfully blocks and inactivates Cas9 (Osuna et al., 2020). These proteins were identified in 36% of the genomes in the present study that showed CRISPR-Cas systems. Even though these strains show systems that allow the acquisition of exogenous material and the possibility of evading infection by bacteriophages, the presence of these phages with Anti-CRISPR proteins evade these immunity mechanisms acquired by the bacteria and thus counteract the acquired immunity (Hynes et al., 2018).
Conclusion
Listeria monocytogenes strains isolated from RTE foods exhibited multiple virulence factors and antibiotic resistance factors after in vitro and in silico analyses. It is therefore necessary to perform continuous genomic surveillance on these foods because of the risk associated with L. monocytogenes contamination and their consumption by populations at risk.
Data Availability Statement
The datasets presented in this study can be found in online repositories. The names of the repository/repositories and accession number(s) can be found in the article/Supplementary Material.
Author Contributions
JP-F, OH, FB, SL, AP, AC-F, AC-C, MT, GF, and WR conceived the experiments and prepared the manuscript. JP-F, FB, AC-F, JM-R, CC, CO, JX-C, MA-L, SL, and MT conducted the laboratory work. JP-F, OH, AC-C, JX-C, JM-R, SL, and WR drafted the manuscript. All authors reviewed and approved the final manuscript.
Funding
This research was funded by the Research Directorate of the Universidad del Bío-Bío, Projects 191520 4/R and GI 195420/EF.
Conflict of Interest
The authors declare that the research was conducted in the absence of any commercial or financial relationships that could be construed as a potential conflict of interest.
Publisher’s Note
All claims expressed in this article are solely those of the authors and do not necessarily represent those of their affiliated organizations, or those of the publisher, the editors and the reviewers. Any product that may be evaluated in this article, or claim that may be made by its manufacturer, is not guaranteed or endorsed by the publisher.
Acknowledgments
We wish to thank the Research Directorate of the Universidad del Bío-Bío.
Supplementary Material
The Supplementary Material for this article can be found online at: https://www.frontiersin.org/articles/10.3389/fmicb.2021.796040/full#supplementary-material
Footnotes
References
Aballa, A., Guariglia-Oropeza, V., Wiedmann, M., and Boor, K. (2019). Cross talk between SigB and PrfA in Listeria monocytogenes facilitates transitions between extra-and intracellular environments. Microbiol. Mol. Biol. Rev. 83:e00037. doi: 10.1128/MMBR.00034-19
Abdollahzadeh, E., Mahdi, S., Hosseini, H., IraJian, G., and Allah, E. (2016). Prevalence and molecular characterization of Listeria spp. and Listeria monocytogenes isolated from fish, shrimp, and cooked ready-to-eat (RTE) aquatic products in Iran. LWT 73, 205–211. doi: 10.1016/j.lwt.2016.06.020
Aljahdali, N. H., Khajanchi, B. K., Weston, K., Deck, J., Cox, J., Singh, R., et al. (2020). Genotypic and phenotypic characterization of incompatibility group FIB positive Salmonella enterica Serovar Typhimurium isolates from food animal sources. Genes 11:1307. doi: 10.3390/genes11111307
Allam, M., Tau, N., Smouse, S. L., Mtshali, P. S., Mnyameni, F., Khumalo, Z., et al. (2018). Whole-genome sequences of Listeria monocytogenes sequence type 6 isolates associated with a large foodborne outbreak in South Africa, 2017 to 2018. Genome Announc. 6:e00538-18. doi: 10.1128/genomeA.00538-18
Althaus, D., Lehner, A., Brisse, S., Maury, M., Tasara, T., and Stephan, R. (2014). Characterization of Listeria monocytogenes strains isolated during 2011–2013 from human infections in Switzerland. Foodborne Pathog. Dis. 11, 753–758. doi: 10.1089/fpd.2014.1747
Amajoud, N., Leclercq, A., Soriano, J., Bracq-Dieye, H., El Maadoudi, M., Skalli, N., et al. (2018). Prevalence of Listeria spp. and characterization of Listeria monocytogenes isolated from food products in Tetouan, Morocco. Food Control 84, 436–441. doi: 10.1016/j.foodcont.2017.08.023
Amato, E., Filipello, V., Gori, M., Lomonaco, S., Losio, M., Parisi, A., et al. (2017). Identification of a major Listeria monocytogenes outbreak clone linked to soft cheese in Northern Italy – 2009-2011. BMC Infect. Dis. 17:342. doi: 10.1186/s12879-017-2441-6
Arslan, S., and Baytur, S. (2019). Prevalence and antimicrobial resistance of Listeria species and subtyping and virulence factors of Listeria monocytogenes from retail meat. J. Food Saf. 39:e12578. doi: 10.1111/jfs.12578
Aznar, R., and Alarcón, B. (2002). On the specificity of PCR detection of Listeria monocytogenes in food: a comparison of published primers. Syst. Appl. Microbiol. 25, 109–119. doi: 10.1078/0723-2020-00079
Becker, B., Stoll, D., Schulz, P., Kulling, S., and Huch, M. (2019). Microbial contamination of organically and conventionally produced fresh vegetable salads and herbs from retail markets in southwest Germany. Foodborne Pathog. Dis. 16, 269–275. doi: 10.1089/fpd.2018.254
Bolocan, A. S., Nicolau, A. I., Alvarez-Ordóñez, A., Borda, D., Oniciuc, E. A., Stessl, B., et al. (2016). Dynamics of Listeria monocytogenes colonisation in a newly-opened meat processing facility. Meat Sci. 113, 26–34. doi: 10.1016/j.meatsci.2015.10.016
Border, P., Howard, J., Plastow, G., and Siggens, K. (1990). Detection of Listeria species and Listeria monocytogenes using polymerase chain reaction. Lett. Appl. Microbiol. 11, 158–162. doi: 10.1111/j.1472-765x.1990.tb00149.x
Braga, V., Vázquez, S., Vico, V., Pastorino, V., Mota, M. I., Legnani, M., et al. (2017). Prevalence and serotype distribution of Listeria monocytogenes isolated from foods in Montevideo-Uruguay. Braz. J. Microbiol. 48, 689–694. doi: 10.1016/j.bjm.2017.01.010
Buchanan, R., Gorris, L., Hayman, M., Jackson, T., and Whiting, R. (2017). A review of Listeria monocytogenes: an update on outbreaks, virulence, dose-response, ecology, and risk assessments. Food Control 75, 1–13. doi: 10.1016/j.foodcont.2016.12.016
Bucur, F. I., Grigore-Gurgu, L., Crauwels, P., Riedel, C. U., and Nicolau, A. I. (2018). Resistance of Listeria monocytogenes to stress conditions encountered in food and food processing environments. Front. Microbiol. 9:2700. doi: 10.3389/fmicb.2018.02700
Bustamante, F., Maury-Sintjago, E., Leal, F. C., Acuña, S., Aguirre, J., Troncoso, M., et al. (2020). Presence of Listeria monocytogenes in ready-to-eat artisanal chilean foods. Microorganisms 8:1669. doi: 10.3390/microorganisms8111669
Cabal, A., Pietzka, A., Huhulescu, S., Allerberger, F., Ruppitsch, W., and Schmid, D. (2019). Isolate-based surveillance of Listeria monocytogenes by whole genome sequencing in Austria. Front. Microbiol. 10:2282. doi: 10.3389/fmicb.2019.02282
Cantinelli, T., Chenal-Francisque, V., Diancourt, L., Frezal, L., Leclercq, A., Wirth, T., et al. (2013). “Epidemic clones” of Listeria monocytogenes are widespread and ancient clonal groups. J. Clin. Microbiol. 51, 3770–3779. doi: 10.1128/JCM.01874-13
Carattoli, A., Zankari, E., García-Fernández, A., Voldby Larsen, M., Lund, O., Villa, L., et al. (2014). In silico detection and typing of plasmids using plasmidFinder and plasmid multilocus sequence typing. Antimicrob. Agents Chemother. 58, 3895–3903. doi: 10.1128/AAC.02412-14
Chen, L., Zheng, D., Liu, B., Yang, J., and Jin, Q. (2016). VFDB 2016: hierarchical and refined dataset for big data analysis—10 years on. Nucleic Acids Res. 4, D694–D697. doi: 10.1093/nar/gkv1239
Chen, Y., Chen, M., Wang, J., Wu, Q., Cheng, J., Zhang, J., et al. (2020b). Heterogeneity, Characteristics, and public health implications of Listeria monocytogenes in ready-to-eat foods and pasteurized milk in China. Front. Microbiol. 11:642. doi: 10.3389/fmicb.2020.00642
Chen, Y., Chen, Y., Pouillot, R., Dennis, S., Xian, Z., Luchansky, J. B., et al. (2020a). Genetic diversity and profiles of genes associated with virulence and stress resistance among isolates from the 2010-2013 interagency Listeria monocytogenes market basket survey. PLoS One 15:e0231393. doi: 10.1371/journal.pone.0231393
Churchill, K. J., Sargeant, J. M., Farber, J. M., and O’Connor, A. M. (2019). Prevalence of Listeria monocytogenes in select ready-to-eat foods-deli meat, soft cheese, and packaged salad: a systematic review and meta-analysis. J. Food Prot. 82, 344–357. doi: 10.4315/0362-028X.JFP-18-158
Churchill, R., Lee, H., and Hall, C. (2006). Detection of Listeria monocytogenes and the Toxin listeriolysin O in Food. J. Microbiol. Methods. 64, 141–170. doi: 10.1016/j.mimet.2005.10.007
Clinical and Laboratory Standards Institute [CLSI] (2018). Methods for Antimicrobial Dilution and Disk Susceptibility Testing of Infrequently Isolated or Fastidious Bacteria, M45, 3rd Edn. Wayne, PA: CLSI.
Cooper, A., Carrillo, C., Deschênes, M., and Blais, B. (2021). Genomic markers for quaternary ammonium compound resistance as a persistence indicator for Listeria monocytogenes contamination in food manufacturing environments. J. Food Prot. 84, 389–398. doi: 10.4315/JFP-20-328
Couvin, D., Bernheim, A., Toffano-Nioche, C., Touchon, M., Michalik, J., Néron, B., et al. (2018). CRISPRCasFinder, an update of CRISRFinder, includes a portable version, enhanced performance and integrates search for CAS proteins. Nucleic Acids Res. 46, 246–251. doi: 10.1093/nar/gky425
Cox, G., and Wright, G. (2013). Intrinsic antibiotic resistance: mechanisms, origins, challenges and solutions. Int. J. Med. Microbiol. 303, 287–292. doi: 10.1016/j.ijmm.2013.02.009
Doumith, M., Buchrieser, C., Glaser, P., Jacquet, C., and Martin, P. (2004). Differentiation of the major Listeria monocytogenes serovars by multiplex PCR. J. Clin. Microbiol. 42, 3819–3822. doi: 10.1128/JCM.42.8.3819-3822.2004
Drolia, R., and Bhunia, A. (2019). Crossing the intestinal barrier via Listeria adhesion protein and Internalin A. Trends Microbiol. 27, 408–425. doi: 10.1016/j.tim.2018.12.007
El-Halfawy, O. M., and Valvano, M. A. (2012). Non-genetic mechanisms communicating antibiotic resistance: rethinking strategies for antimicrobial drug design. Expert Opin. Drug Discov. 7, 923–933. doi: 10.1517/17460441.2012.712512
European Centre for Disease Prevention and Control [ECDC] (2018). Surveillance Atlas of Infectious Diseases. Available onlione at: https://atlas.ecdc.europa.eu/public/index.aspx (accessed Mar 10, 2021).
Fagerlund, A., Langsrud, S., Schirmer, B. C., Møretrø, T., and Heir, E. (2016). Genome analysis of Listeria monocytogenes sequence type 8 strains persisting in salmon and poultry processing environments and comparison with related strains. PLoS One 11:e0151117. doi: 10.1371/journal.pone.0151117
Falavina dos Reis, C. M., Barbosa, A. V., Rusak, L. A., Vallim, D. C., and Hofer, E. (2011). Antimicrobial susceptibilities of Listeria monocytogenes human strains isolated from 1970 to 2008 in Brazil. Rev. Soc. Bras. Med. Trop. 44, 173–176. doi: 10.1590/s0037-86822011005000019
Feldgarden, M., Brover, V., Haft, D. H., Prasad, A. B., Slotta, D. J., Tolstoy, I., et al. (2019). Validating the AMRFinder tool and resistance gene database by using antimicrobial resistance genotype-phenotype correlations in a collection of isolates. Antimicrob. Agents Chemother. 63:e00483-19. doi: 10.1128/AAC.00483-19
Ferreira da Silva, M., Ferreira, V., Magalhães, R., Almeida, G., Alves, A., and Teixeira, P. (2017). Detection of premature stop codons leading to truncated internalin A among food and clinical strains of Listeria monocytogenes. Food Microbiol. 63, 6–11. doi: 10.1016/j.fm.2016.10.033
Fretz, R., Sagel, U., Ruppitsch, W., Pietzka, A., Stoger, A., Huhulescu, S., et al. (2010). Listeriosis outbreak caused by acid curd cheese Quargel, Austria and Germany 2009. Euro Surveill. 15:19477.
Gorski, L. (2021). “Chapter 5 – Serotype assignment by Sero-agglutination, ELISA, and PCR,” in Listeria Monocytogenes Methods and Protocols, 2nd Edn, eds E. Fox, H. Bierne, and B. Stessl (Cham: Springer Nature), 57–78. doi: 10.1007/978-1-0716-0982-8_5
Gygli, S. M., Keller, P. M., Ballif, M., Blöchliger, N., Hömke, R., Reinhard, M., et al. (2019). Whole-genome sequencing for drug resistance profile prediction in Mycobacterium tuberculosis. Antimicrob. Agents Chemother. 27:e02175-18. doi: 10.1128/AAC.02175-18
Halbedel, S., Wilking, H., Holzer, A., Kleta, S., Fischer, M. A., Lüth, S., et al. (2020). Large nationwide outbreak of invasive listeriosis associated with blood sausage, Germany, 2018-2019. Emerg. Infect. Dis. 26, 1456–1464. doi: 10.3201/eid2607.200225
Horlbog, J. A., Kent, D., Stephan, R., and Guldimann, C. (2018). Surviving host – and food relevant stresses: phenotype of L. monocytogenes strains isolated from food and clinical sources. Sci. Rep. 8:12931. doi: 10.1038/s41598-018-30723-z
Hupfeld, M., Trasanidou, D., Ramazzini, L., Klumpp, J., Loessner, M. J., and Kilcher, S. (2018). A functional type II-A CRISPR-Cas system from Listeria enables efficient genome editing of large non-integrating bacteriophage. Nucleic Acids Res. 46, 6920–6933. doi: 10.1093/nar/gky544
Hurley, D., Luque-Sastre, L., Parker, C. T., Huynh, S., Eshwar, A. K., Nguyen, S. V., et al. (2019). Whole-genome sequencing-based characterization of 100 Listeria monocytogenes isolates collected from food processing environments over a four-year period. mSphere 4:e00252-19. doi: 10.1128/mSphere.00252-19
Hynes, A. P., Rousseau, G. M., Agudelo, D., Goulet, A., Amigues, B., Loehr, J., et al. (2018). Widespread anti-CRISPR proteins in virulent bacteriophages inhibit a range of Cas9 proteins. Nat. Commun. 9:2919. doi: 10.1038/s41467-018-05092-w
Jamali, H., Paydar, M., Looi, C., and Wong, W. (2013). Prevalence of Listeria species and Listeria monocytogenes serotypes in ready mayonnaise salads and salad vegetables in Iran. Afr. J. Microbiol. Res. 2013, 1903–1906. doi: 10.5897/AJMR2013.5658
Jia, B., Raphenya, A. R., Alcock, B., Waglechner, N., Guo, P., Tsang, K., et al. (2017). CARD 2017: expansion and model-centric curation of the comprehensive antibiotic resistance database. Nucleic Acids Res. 45, D566–D573. doi: 10.1093/nar/gkw1004
Johansson, M., Bortolaia, V., Tansirichaiya, S., Aarestrup, F. M., Roberts, A. P., and Petersen, T. N. (2021). Detection of mobile genetic elements associated with antibiotic resistance in Salmonella enterica using a newly developed web tool: mobileElementFinder. J. Antimicrob. Chemother. 76, 101–109. doi: 10.1093/jac/dkaa390
Jünemann, S., Sedlazeck, F. J., Prior, K., Albersmeier, A., John, U., Kalinowski, J., et al. (2013). Updating benchtop sequencing performance comparison. Nat. Biotechnol. 31, 294–296. doi: 10.1038/nbt.2522
Kashuba, E., Dmitriev, A., Mansour, S., Melefors, O., Griva, G., Römling, U., et al. (2017). Ancient permafrost staphylococci carry antibiotic resistance genes. Microb. Ecol. Health Dis. 28:1345574. doi: 10.1080/16512235.2017.1345574
Klein, P. G., and Juneja, V. (1997). Sensitive detection of viable Listeria monocytogenes by reverse transcription-PCR. Appl. Environ. Microbiol. 63, 4441–4448. doi: 10.1128/aem.63.11.4441-4448.1997
Knabel, S. J., Reimer, A., Verghese, B., Lok, M., Ziegler, J., Farber, J., et al. (2012). Sequence typing confirms that a predominant Listeria monocytogenes clone caused human listeriosis cases and outbreaks in Canada from 1988 to 2010. J. Clin. Microbiol. 50, 1748–1751. doi: 10.1128/JCM.06185-11
Knudsen, G., Nielsen, J., Marvig, R., Ng, Y., Worning, P., Westh, H., et al. (2017). Genome-wide-analyses of Listeria monocytogenes from food-processing plants reveal clonal diversity and date the emergence of persisting sequence types. Environ. Microbiol. Rep. 9, 428–440. doi: 10.1111/1758-2229.12552
Kohler, V., Vaishampayan, A., and Grohmann, E. (2018). Broad-host-range Inc18 plasmids: occurrence, spread and transfer mechanisms. Plasmid 99, 11–21. doi: 10.1016/j.plasmid.2018.06.001
Kuenne, C., Billion, A., Mraheil, M. A., Strittmatter, A., Daniel, R., Goesmann, A., et al. (2013). Reassessment of the Listeria monocytogenes pan-genome reveals dynamic integration hotspots and mobile genetic elements as major components of the accessory genome. BMC Genomics 14:47. doi: 10.1186/1471-2164-14-47
Kuenne, C., Voget, S., Pischimarov, J., Oehm, S., Goesmann, A., Daniel, R., et al. (2010). Comparative analysis of plasmids in the genus Listeria. PLoS One 5:e12511. doi: 10.1371/journal.pone.0012511
Kumaraswamy, M., Do, C., Sakoulas, G., NoneJuie, P., Tseng, G., King, H., et al. (2018). Listeria monocytogenes endocarditis: case report, review of the literature, and laboratory evaluation of potential novel antibiotic synergies. Int. J. Antimicrob. Agents 51, 468–478. doi: 10.1016/j.ijantimicag.2017.12.032
Kurpas, M., Wieczorek, K., and Osek, J. (2018). Ready-to-eat meat products as a source of Listeria monocytogenes. J. Vet. Res. 62, 49–55. doi: 10.1515/jvetres-2018-0007
Kwon, H. J., Chen, Z., Evans, P., Meng, J., and Chen, Y. (2020). Characterization of mobile genetic elements using long-read sequencing for tracking Listeria monocytogenes from food processing environments. Pathogens 9:822. doi: 10.3390/pathogens9100822
Lange, S. J., Alkhnbashi, O. S., Rose, D., Will, S., and Backofen, R. (2013). CRISPRmap: an automated classification of repeat conservation in prokaryotic adaptive immune systems. Nucleic Acids Res. 41, 8034–8044. doi: 10.1093/nar/gkt606
Lee, S., Chen, Y., Gorski, L., Ward, T. J., Osborne, J., and Kathariou, S. (2018). Listeria monocytogenes source distribution analysis indicates regional heterogeneity and ecological niche preference among serotype 4b clones. mBio 9:e00396-18. doi: 10.1128/mBio.00396-18
Lee, S., Ward, T. J., Graves, L. M., Wolf, L. A., Sperry, K., Siletzky, R. M., et al. (2012). Atypical Listeria monocytogenes serotype 4b strains harboring a lineage II-specific gene cassette. Appl. Environ. Microbiol. 78, 660–667. doi: 10.1128/AEM.06378-11
Leopold, S. R., Goering, R. V., Witten, A., Harmsen, D., and Mellmann, A. (2014). Bacterial whole-genome sequencing revisited: portable, scalable, and standardized analysis for typing and detection of virulence and antibiotic resistance genes. J. Clin. Microbiol. 52, 2365–2370. doi: 10.1128/JCM.00262-14
Li, W., Bai, L., Fu, P., Han, H., Liu, J., and Guo, Y. (2018). The epidemiology of Listeria monocytogenes in China. Foodborne Pathog. Dis. 15, 459–466. doi: 10.1089/fpd.2017.2409
Louwen, R., Horst-Kreft, D., de Boer, A. G., van der Graaf, L., de Knegt, G., Hamersma, M., et al. (2013). A novel link between Campylobacter jejuni bacteriophage defence, virulence and Guillain–Barré syndrome. Eur. J. Clin. Microbiol. Infect. Dis. 32, 207–226. doi: 10.1007/s10096-012-1733-4
Louwen, R., Staals, R., Endtz, H., van Baarlen, P., and van der Oost, J. (2014). The role of CRISPR-Cas systems in virulence of pathogenic bacteria. Microbiol. Mol. Biol. Rev. 78, 74–88. doi: 10.1128/MMBR.00039-13
Maćkiw, E., Stasiak, M., Kowalska, J., Kucharek, K., Korsak, D., and Postupolski, J. (2020). Occurrence and characteristics of Listeria monocytogenes in ready-to-eat meat products in Poland. J. Food Prot. 83, 1002–1009. doi: 10.4315/JFP-19-525
Maertens de Noordhout, C., Devleesschauwer, B., Haagsma, J. A., Havelaar, A. H., Bertrand, S., Vandenberg, O., et al. (2017). Burden of salmonellosis, campylobacteriosis and listeriosis: a time series analysis, Belgium, 2012 to 2020. Euro Surveill. 22:30615. doi: 10.2807/1560-7917.ES.2017.22.38.30615
Mafuna, T., Matle, I., Magwedere, K., Pierneef, R. E., and Reva, O. N. (2021). Whole genome-based characterization of Listeria monocytogenes isolates recovered from the food chain in South Africa. Front. Microbiol. 12:669287. doi: 10.3389/fmicb.2021.669287
Mammina, C., Parisi, A., Guaita, A., Aleo, A., Bonura, C., Nastasi, A., et al. (2013). Enhanced surveillance of invasive listeriosis in the Lombardy region, Italy, in the years 2006-2010 reveals major clones and an increase in serotype 1/2a. BMC Infect. Dis. 13:152. doi: 10.1186/1471-2334-13-152
Martínez, L., Joyanes, P., Súarez, A., and Perea, E. (2001). Activities of gemifloxacin and five other antimicrobial agents against Listeria monocytogenes and coryneform bacteria isolated from clinical samples. Antimicrob. Agents Chemother. 45, 2390–2392. doi: 10.1128/AAC.45.8.2390-2392.2001
Martínez-Rios, V., and Dalgaard, P. (2018). Prevalence of Listeria monocytogenes in European cheeses: a systematic review and meta-analysis. Food Control 84, 205–214. doi: 10.1016/j.foodcont.2017.07.020
Maury, M., Tsai, Y. H., Charlier, C., Touchon, M., Chenal-Francisque, V., Leclercq, A., et al. (2016). Uncovering Listeria monocytogenes hypervirulence by harnessing its biodiversity. Nat. Genet. 48, 308–313. doi: 10.1038/ng.350
MINSAL: Ministerio de Salud Chile, Departamento de Estadísticas e Información en Salud [DEIS] (2019). Boletin Epidemiologico Trimestral Listeriosis SE1-52, año 2019. Available online at: http://epi.minsal.cl/wp-content/uploads/2020/01/BET_LISTERIOSIS_A%C3%91O_2019.pdf (accessed June 30, 2021).
Montero, D., Bodero, M., Riveros, G., Lapierre, L., Gaggero, A., Vidal, R., et al. (2015). Molecular epidemiology and genetic diversity of Listeria monocytogenes isolates from a wide variety of ready-to-eat foods and their relationship to clinical strains from listeriosis outbreaks in Chile. Front. Microbiol. 30:384–386. doi: 10.3389/fmicb.2015.00384
Mota, M. I., Vázquez, S., Cornejo, C., D’Alessandro, B., Braga, V., Caetano, A., et al. (2020). Does Shiga toxin-producing Escherichia coli and Listeria monocytogenes contribute significantly to the burden of antimicrobial resistance in Uruguay? Front. Vet. Sci. 7:583930. doi: 10.3389/fvets.2020.583930
Moura, A., Criscuolo, A., Pouseele, H., Maury, M., Leclerq, A., Tarr, C., et al. (2016). Whole genome-based population biology and epidemiological surveillance of Listeria monocytogenes. Nat. Microbiol. 2:16185. doi: 10.1038/nmicrobiol.2016.185
Moura, A., Tourdjman, M., Leclercq, A., Hamelin, E., Laurent, E., Fredriksen, N., et al. (2017). Real-time whole-genome sequencing for surveillance of Listeria monocytogenes, France. Emerg. Infect. Dis. 23, 1462–1470. doi: 10.3201/eid2309.170336
Mraheil, M., Billion, A., Mohamed, W., Mukherjee, K., Kuenne, C., Pischimarov, J., et al. (2011). The intracellular sRNA transcriptome of Listeria monocytogenes during growth in macrophages. Nucleic Acids Res. 39, 4235–4248. doi: 10.1093/nar/gkr033
Olaimat, A. N., Al-Holy, M. A., Shahbaz, H. M., Al-Nabulsi, A. A., Abu Ghoush, M. H., Osaili, T. M., et al. (2018). Emergence of antibiotic resistance in Listeria monocytogenes isolated from food products: a Comprehensive review. Compr. Rev. Food Sci. Food Saf. 17, 1277–1292. doi: 10.1111/1541-4337.12387
Orsi, R., den Bakker, H., and Wiedmann, M. (2011). Listeria monocytogenes lineages: genomics, evolution, ecology, and phenotypic characteristics. Int. J. Med. Microbiol. 301, 79–96. doi: 10.1016/j.ijmm.2010.05.002
Osuna, B. A., Karambelkar, S., Mahendra, C., Christie, K. A., Garcia, B., Davidson, A. R., et al. (2020). Listeria phages induce Cas9 degradation to protect lysogenic genomes. Cell Host Microbe 28, 31–40.e9. doi: 10.1016/j.chom.2020.04.0
Peterson, E., and Kaur, P. (2018). Antibiotic resistance mechanisms in bacteria: relationships between resistance determinants of antibiotic producers, environmental bacteria, and clinical pathogens. Front. Microbiol. 9:2928. doi: 10.3389/fmicb.2018.02928
Pöntinen, A., Aalto-Araneda, M., Lindström, M., and Korkeala, H. (2017). Heat resistance mediated by pLM58 plasmid-borne ClpL in Listeria monocytogenes. mSphere 2:e00364-17. doi: 10.1128/mSphere.00364-17
Pouillot, R., Klontz, K. C., Chen, Y., Burall, L. S., Macarisin, D., Doyle, M., et al. (2016). Infectious dose of Listeria monocytogenes in outbreak linked to ice cream, United States, 2015. Emerg. Infect. Dis. 22, 2113–2119. doi: 10.3201/eid2212.160165
Ruppitsch, W., Pietzka, A., Prior, K., Bletz, S., Fernandez, H. L., Allerberger, F., et al. (2015a). Defining and evaluating a core genome multilocus sequence typing scheme for whole-genome sequence-based typing of Listeria monocytogenes. J. Clin Microbiol. 53, 2869–2876. doi: 10.1128/JCM.01193-15
Ruppitsch, W., Prager, R., Halbedel, S., Hyden, P., Pietzka, A., Huhulescu, S., et al. (2015b). Ongoing outbreak of invasive listeriosis, Germany, 2012 to 2015. Euro Surveill. 20:30094. doi: 10.2807/1560-7917.ES.2015.20.50.30094
Ryser, E. (2021). “Chapter 11 – Listeria,” in The Foodborne Infections and Intoxications, 5th Edn, eds G. Morris and D. Vugia (Amsterdam: Academic Press), 201–220. doi: 10.1016/B978-0-12-819519-2.00028-1
Sanlibaba, P., Uymaz, B., and Aybige, G. (2018). Prevalence and antibiotic resistance of Listeria monocytogenes isolated from ready to eat foods in Turkey. J. Food Qual. 2018:7693782. doi: 10.1155/2018/7693782
Schlech, W. (2019). Epidemiology and clinical manifestations of Listeria monocytogenes infection. Microbiol. Spectr. 7, 1–12. doi: 10.1128/microbiolspec.GPP3-0014-2018
Seoane, M. (2013). Listeria monocytogenes. Rev. Chil. Infectol. 30, 405–406. doi: 10.4067/S0716-10182013000400009
Stessl, B., Wagner, M., and Ruppitsch, W. (2021). Multilocus sequence typing (MLST) and whole genome sequencing (WGS) of Listeria monocytogenes and Listeria innocua. Methods Mol. Biol. 2220, 89–103. doi: 10.1007/978-1-0716-0982-8_7
Szymczak, B., Szymczak, M., and Trafiałek, J. (2020). Prevalence of Listeria species and L. monocytogenes in ready-to-eat foods in the West Pomeranian region of Poland: correlations between the contamination level, serogroups, ingredients, and producers. Food Microbiol. 91:103532. doi: 10.1016/j.fm.2020.103532
Thønnings, S., Knudsen, J. D., Schønheyder, H. C., Søgaard, M., Arpi, M., Gradel, K. O., et al. (2016). Antibiotic treatment and mortality in patients with Listeria monocytogenes meningitis or bacteraemia. Clin. Microbiol. Infect. 22, 725–730. doi: 10.1016/j.cmi.2016.06.006
Toledo, V., Den Bakker, H. C., Hormazábal, J. C., González-Rocha, G., Bello-Toledo, H., Toro, M., et al. (2018). Genomic diversity of Listeria monocytogenes isolated from clinical and non-clinical samples in Chile. Genes 9:396. doi: 10.3390/genes9080396
Ulloa, S., Arata, L., Alarcón, P., Araya, P., Hormazábal, J., and Fernández, J. (2019). Caracterización genética de cepas de Listeria monocytogenes aisladas durante los años 2007–2014 en Chile. Rev. Chil. Infectol. 36, 585–590. doi: 10.4067/S0716-10182019000500585
Van Stelten, A., and Nightingale, K. K. (2008). Development and implementation of a multiplex single-nucleotide polymorphism genotyping assay for detection of virulence-attenuating mutations in the Listeria monocytogenes virulence-associated gene inlA. Appl. Environ. Microbiol. 74, 7365–7375. doi: 10.1128/AEM.01138-08
Van Stelten, A., Simpson, J. M., Ward, T. J., and Nightingale, K. K. (2010). Revelation by single-nucleotide polymorphism genotyping that mutations leading to a premature stop codon in inlA are common among Listeria monocytogenes isolates from ready-to-eat foods but not human listeriosis cases. Appl. Environ. Microbiol. 76, 2783–2790. doi: 10.1128/AEM.02651-09
Wang, G., Qian, W., Zhang, X., Wang, H., Ye, K., Bai, Y., et al. (2015). Prevalence, genetic diversity and antimicrobial resistance of Listeria monocytogenes isolated from ready-to-eat meat products in NanJing, China. Food Control 50, 202–208. doi: 10.1016/j.foodcont.2014.07.057
Wilson, A., Gray, J., Chandry, P. S., and Fox, E. M. (2018). Phenotypic and genotypic analysis of antimicrobial resistance among Listeria monocytogenes isolated from Australian food production chains. Genes 9:80. doi: 10.3390/genes9020080
Yucel, N., Citak, S., and Onder, M. (2005). Prevalence and antibiotic resistance of Listeria species in meat products in Ankara, Turkey. Food Microbiol. 22, 241–245. doi: 10.1016/j.fm.2004.03.007
Zhang, F., Zhao, S., Ren, C., Zhu, Y., Lai, Y., Zhou, F., et al. (2018). CRISPRminer is a knowledge base for exploring CRISPR-Cas systems in microbe and phage interactions. Commun. Biol. 1:180. doi: 10.1038/s42003-018-0184-6
Zhu, W., Murray, P. R., Huskins, W. C., Jernigan, J. A., McDonald, L. C., Clark, N. C., et al. (2010). Dissemination of an Enterococcus Inc18-Like vanA plasmid associated with vancomycin-resistant Staphylococcus aureus. Antimicrob. Agents Chemother. 54, 4314–4320. doi: 10.1128/AAC.00185-10
Keywords: Listeria monocytogenes, ready-to-eat foods, virulence, resistance genes, whole-genome sequencing, CRISPR-Cas
Citation: Parra-Flores J, Holý O, Bustamante F, Lepuschitz S, Pietzka A, Contreras-Fernández A, Castillo C, Ovalle C, Alarcón-Lavín MP, Cruz-Córdova A, Xicohtencatl-Cortes J, Mancilla-Rojano J, Troncoso M, Figueroa G and Ruppitsch W (2022) Virulence and Antibiotic Resistance Genes in Listeria monocytogenes Strains Isolated From Ready-to-Eat Foods in Chile. Front. Microbiol. 12:796040. doi: 10.3389/fmicb.2021.796040
Received: 15 October 2021; Accepted: 13 December 2021;
Published: 21 February 2022.
Edited by:
Yasser M. Sanad, University of Arkansas at Pine Bluff, United StatesReviewed by:
Yu Ding, Jinan University, ChinaHaiyan Zeng, Guangdong University of Technology, China
Copyright © 2022 Parra-Flores, Holý, Bustamante, Lepuschitz, Pietzka, Contreras-Fernández, Castillo, Ovalle, Alarcón-Lavín, Cruz-Córdova, Xicohtencatl-Cortes, Mancilla-Rojano, Troncoso, Figueroa and Ruppitsch. This is an open-access article distributed under the terms of the Creative Commons Attribution License (CC BY). The use, distribution or reproduction in other forums is permitted, provided the original author(s) and the copyright owner(s) are credited and that the original publication in this journal is cited, in accordance with accepted academic practice. No use, distribution or reproduction is permitted which does not comply with these terms.
*Correspondence: Julio Parra-Flores, anVwYXJyYUB1YmlvYmlvLmNs; Werner Ruppitsch, d2VybmVyLnJ1cHBpdHNjaEBhZ2VzLmF0
†These authors have contributed equally to this work