- College of Resources and Environmental Sciences, Institute of Organic Contaminant Control and Soil Remediation, Nanjing Agricultural University, Nanjing, China
Some root-associated bacteria could degrade polycyclic aromatic hydrocarbons (PAHs) in contaminated soil; however, their dynamic distribution and performance on root surface and in inner plant tissues are still unclear. In this study, greenhouse container experiments were conducted by inoculating the phenanthrene-degrading bacterium Diaphorobacter sp. Phe15, which was isolated from root surfaces of healthy plants contaminated with PAHs, with the white clover (Trifolium repens L.) via root irrigation or seed soaking. The dynamic colonization, distribution, and performance of Phe15 in white clover were investigated. Strain Phe15 could efficiently degrade phenanthrene in shaking flasks and produce IAA and siderophore. After cultivation for 30, 40, and 50 days, it could colonize the root surface of white clover by forming aggregates and enter its inner tissues via root irrigation or seed soaking. The number of strain Phe15 colonized on the white clover root surfaces was the highest, reaching 6.03 Log CFU⋅g–1 FW, followed by that in the roots and the least in the shoots. Colonization of Phe15 significantly reduced the contents of phenanthrene in white clover; the contents of phenanthrene in Phe15-inoculated plants roots and shoots were reduced by 29.92–43.16 and 41.36–51.29%, respectively, compared with the Phe15-free treatment. The Phe15 colonization also significantly enhanced the phenanthrene removal from rhizosphere soil. The colonization and performance of strain Phe15 in white clove inoculated via root inoculation were better than seed soaking. This study provides the technical support and the resource of strains for reducing the plant PAH pollution in PAH-contaminated areas.
Introduction
Polycyclic aromatic hydrocarbons (PAHs) are a class of persistent toxic organic pollutants that exist widely in the soil environment (Falciglia et al., 2016). PAHs are mainly derived from the incomplete combustion of petroleum, coal, wood, and other organic matter (Abdel-Shafy and Mansour, 2016). Moreover, studies have shown that the main source of PAHs has changed from the incomplete combustion of biomass to the use of large amounts of fossil fuels (Li et al., 2021; Yu et al., 2021). It can be seen that man-made production activities have become the main source of PAHs released into the environment. In Delhi, India, the total PAH contents hidden in PM10 and PM2.5 in winter were as high as 177.5 ng⋅m–3, and these PAHs would enter the soil eventually (Singh et al., 2011). A study performed in 2011 found that the petroleum refining soil in Bratislava contained up to 2000 μg⋅Kg−1 of PAHs (Musa Bandowe et al., 2011). And to make matters worse, recent studies have shown an increase in PAHs over the Arctic (Yu et al., 2019). PAHs in the soil can be taken up and accumulated by plants and then transferred and biomagnified through the food chains, threatening human health (Gao et al., 2013). It is of great significance to regulate the absorption and accumulation of PAHs in soil by plants, to reduce the PAH contamination in crops and produce safe agricultural products in PAH-contaminated areas.
Some surfactants can enhance the solubility and bioavailability of PAHs, and are used to regulate PAH uptake and accumulation in plants. Gao et al. (2006) founded that the addition of appropriate BRIJ35 (≤74 mg⋅L–1) could improve the absorption of pyrene and phenanthrene by plants, while the high concentration BRIJ35 (≥148 mg⋅L–1) inhibited the absorption of them due to its competitive effect and toxic effect on plants. Coincidentally, Liao et al. (2015) also pointed out that adding an appropriate concentration of surfactants to the soil could improve the bioavailability of PAHs, thus promoting the absorption and accumulation of PAHs by maize. However, due to environmentally unfriendly characteristics of most surfactants (Mueller et al., 2005), microbial methods such as endophytic bacteria and mycorrhizal fungi were more commonly used to remove PAHs from rhizosphere soil and inner plants, thus reducing the risk of plant PAH pollution in contaminated areas (Gao et al., 2011; Sun et al., 2014).
The root surface is the interface between plant roots and soil, and it is an important window for plants to absorb organic pollutants. According to Fismes et al. (2002), firstly, organic pollutants gradually spread from the surrounding environment to plant roots and were adsorbed on the root surface and then gradually absorbed by plant roots. Zhan et al. (2010) verified this conclusion when studying the absorption of phenanthrene by wheat roots: in the initial stage of rapid phenanthrene absorption by wheat roots, phenanthrene was first adsorbed to the surface of wheat roots then spread to the inner root tissue and transferred to the shoots along with transpiration flow. It is clear that reducing PAH contents adsorbed by root surfaces can effectively inhibit the uptake of PAHs by plant roots, thus reducing the plant PAH contamination.
Root exudates can attract considerable numbers of bacteria to colonize the root surface of plants and form bacterial aggregates or even bacterial biofilms (Ramey et al., 2004). Root-associated bacteria usually own various ecological functions, such as promoting plant growth, improving plant stress resistance to harsh environments, reducing plant diseases (Thimmaraju et al., 2008), and controlling even remediating environmental organic pollution (Marta et al., 2005). In 2004, Johnsen and Karlson (2004) found that three strains belonging to Sphingomonas could increase the solubility of PAHs via producing exopolysaccharides, thus enhancing the degradation efficiency of PAHs. Böltner et al. (2008) isolated two Sphingomonas strains with lindane-degrading ability from plant root surfaces and found that the two strains colonized maize roots in large numbers for a long time and could remove lindane consistently from the rhizosphere. However, there are few reports on root-associated PAH-degrading bacteria; whether their colonization on root surface can effectively reduce the accumulation and absorption of PAHs in plants remains unclear.
The PAH-polluted agricultural area exists in many countries; it is of great significance to reuse these contaminated fields to produce safe agricultural products. In these PAH-polluted soil, phenanthrene is always one of the dominant PAHs and can be absorbed by plants (Tao et al., 2004). Based on this, this study explores the isolation and recolonization of a root-associated phenanthrene-degrading bacterium into white clover via root inoculation or seed soaking. The degradation performance of phenanthrene, a model PAH, by this strain was also examined. The colonization, distribution and performance of this strain in white clover were investigated to provide a theoretical basis for the plant PAH pollution control and agricultural food safety in PAH-contaminated areas.
Materials and Methods
Reagents and Culture Media
The phenanthrene (purity ≥98%) was purchased from Fluka, Germany, and it was prepared into a highly concentrated stock solution (2.0 g⋅L–1 in acetone). The water solubility (Sw) and molecular weight (MW) of phenanthrene is 1.18 mg⋅L–1 (25°C) and 178.23, respectively, and the logarithmic transformation distribution coefficient (Log Kow) of phenanthrene octanol-water is 4.57 (Chiou et al., 1998). All solvents and reagents used were of analytical grade.
The mineral salt medium (MSM) contained 0.20 g⋅L–1 MgSO4⋅7H2O, 1.50 g⋅L–1 (NH4)2SO4, 1.91 g⋅L–1 K2HPO4⋅3H2O and 0.50 g⋅L–1 KH2PO4 (Sun et al., 2014). Phenanthrene was added to MSM to make PMM medium as described in a previous study (Liu et al., 2014). Solid medium plates were prepared by adding 18 g⋅L–1 of agar into the liquid media described above.
Isolation of the Root-Associated Phenanthrene-Degrading Bacterium Phe15
Several representative dominant plants were collected from the fields contaminated by PAHs for a long time. After removal of soil from the root surface with a sterilizing brush, the root was washed gently under running water for 15 s, cut off with sterilizing scissors, and then placed into a centrifuge tube with 5 mL sterilized deionized water to vibrate violently for 30 s, to destroy the bacterial biofilm structure and free the bacteria from the root surface (Yamaga et al., 2010).
The obtained bacterial suspension was added into PMM with the initial phenanthrene concentration of 100 mg⋅L–1 and cultivated (Liu et al., 2018). Finally, the enrichment culture was coated on PMM plates, and a bacterium named Phe15 was isolated for it could produce a clear zone around its colony. After verifying the phenanthrene-degrading ability of this strain, it was identified as mentioned previously (Sun et al., 2014).
Degradation of Phenanthrene by Strain Phe15
Strain Phe15 was inoculated in LB medium and cultured on a rotary shaker (180 r⋅min–1, 30°C) until OD600nm = 1.0. The bacterial solution was centrifuged (8000 r⋅min–1, 5 min), washed twice by MSM, resuspended to obtain 1.8 × 108 colony-forming units (cfu) ⋅ mL–1, and injected into PMM at 2% (V: V).
Then, each 20 mL of the above inoculated PMM (containing 100 mg⋅L–1 phenanthrene) was added into a 50 mL flask and cultured in a shaker (180 r⋅min–1, 30°C). The cultured samples were extracted periodically. The Phe15 cells in the flask were estimated by plate counting, and the residual phenanthrene concentration was determined by HPLC (Liu et al., 2018). Phenanthrene degradation kinetics and growth curves of Phe15 were plotted with the PMM inoculated with inactivated Phe15 as control.
The effects of pH (4.0, 5.0, 6.0, 7.0, 8.0, 9.0, 10.0), phenanthrene concentration (50, 100, 150, 200, 250 mg⋅L–1) and the incubation temperature (15, 20, 25, 30, 37, 42°C) on degradation of phenanthrene by strain Phe15 were also investigated (Sun et al., 2014).
Biological Characteristics of Strain Phe15
Antibiotic Resistance
The distribution of strain Phe15 in the inner and rhizosphere of plants could be tracked after antibiotic resistance label as following. The inoculum of strain Phe15 as above was inoculated in LB liquid medium containing different concentrations of antibiotics at 2% (V:V), and the growth of the strains was observed after 96 h of constant temperature oscillation culture (180 r⋅min–1, 30°C). Antibiotics include ampicillin, gentamicin, kanamycin, streptomycin, tetracycline, chloramphenicol, erythromycin, and spectinomycin at concentrations of 0, 10, 25, 50, 75, and 100 mg⋅L–1, respectively.
Plant Growth-Promoting Characteristics
In order to determine the plant growth promoting potential of strain Phe15, the capacity of strain Phe15 to produce indole-acetic acid (IAA) and siderophore was measured according to the methods described by Gordon and Weber (1951) and Zhang et al. (2021), respectively. Meanwhile, Phe15 pure culture (1 μL) was inoculated on the plate with LB medium supplemented with Ca5 (PO4)3OH and cultured at 30°C for 7 consecutive days. The appearance of transparent “halos” was observed to quantify the solubilizing effect of phosphate (Katznelson and Bose, 1959).
Colonization Potential on White Clover Root Surfaces
The seeds of Trifolium repens L. were purchased from Jiangsu Agricultural Science and Seed Industry Research Institute Co. Ltd. After using 1% sodium hypochlorite to disinfect the surface of plants for 10 min, the white clover seeds were immediately cleaned with sterile water to accelerate germination without light.
To determine the colonization ability of strain Phe15 on white clover root surfaces, the distribution of strain Phe15 on the white clover root surfaces was observed. After germination, the white clover was transferred to a sterile culture system (with 1/2 Hoagland nutrient solution) and cultured in a light incubator (Sun et al., 2014). When white clover grew to about 7 cm height, 2% (V: V) of Phe15 suspension (OD600nm = 1.0) was inoculated into nutrient solution for 3 days. Then the white clover was collected, and the free cells of strain Phe15 on the white clover root surfaces were gently washed off with running water. The bacterial aggregates of Phe15 on the white clover root surfaces were observed through scanning electron microscopy (SEM) detection in the Life Science Experiment Center, Nanjing Agricultural University.
Greenhouse Container Experiments
Collection and Treatment of Soil
The soil samples were collected from the topsoil of a farmland in Nanjing. The soil type was yellow-brown soil with pH value of 6.03 and organic carbon content of 14.3 g⋅kg–1, no PAHs were detected. The collected soil was air-dried, sieved through 10 meshes, and then artificially polluted with phenanthrene at a final content of 100 mg⋅kg–1 (Liu et al., 2017). After 30 days of aging (Hwang and Cutright, 2002), the phenanthrene content in the soil decreased to 62.37 mg⋅kg–1.
Potted Experiment Setup
Six treatments were set in the experiment, which were: uncontaminated soil + white clover (UW), contaminated soil (CK), contaminated soil + white clover (CW), contaminated soil + Phe15 (CP), contaminated soil + white clover + Phe15 via root irrigation (CWR) and contaminated soil + white clover + Phe15 via seed soaking (CWS). All treatments were replicated 3 times.
Greenhouse Container Experiments
After sterilizing the white clover seeds with 1% sodium hypochlorite for 10 min and then washing them with sterile water for 5 min, the white clover seeds were divided into two parts and one was planted directly in the soil (UW, CW, CWR). The other seeds were soaked in a prepared Phe15 MSM suspension (OD600nm = 1.0) for 4 h, dried, and then sown in the soil (CWS). Follow-up treatments for the CWR group: 10 mL Phe15 suspension (OD600nm = 1.0) was evenly injected into the contaminated soil when two true leaves of white clover grew after sowing. The same Phe15 suspension was also added to the unsown contaminated soil as group CP. Then, the inactivated Phe15 suspension in MSM was inoculated into CK, CW, and UW groups as the control.
Each of the six experimental groups had three pots, and each pot was evenly distributed with 8 grains. The seedlings were placed in a light incubator (25°C in the day and 20°C in the night) and cultivated for 50 days; the positions of the pots were randomly exchanged every 3 days. Destructive sampling was performed at the 30, 40 and 50 days, respectively, to determine the cell counts of colonized Phe15, the biomass of white clover, and the phenanthrene contents in plant and soil samples.
Determination of Biomass of White Clover
The samples of fresh white clover were collected and washed with sterile running water. After rinsing all surface attachments and drying the plant surface with blotting paper, the root and stem of white clover were separated, and the fresh weight of white clover was weighed with a balance. After that, the fresh plant samples were placed in a freeze-dryer and then freeze-dried for 72 h to obtain the dry weight.
Determination of Cell Count of Strain Phe15
A small number of samples (soil, plant shoots, plant roots) were ground, then suspended in sterile water and shaken to obtain bacterial suspension, which was used for dilution coating plate counting (Liu et al., 2018) on PMM plates containing 25 mg⋅L–1 chloramphenicol and 75 mg⋅L–1 ampicillin. Then, strains producing clear zones under the UV lamp were counted and then randomly selected for 16S rRNA gene sequencing. The sequencing results suggested that all the selected colonies were determined to be strain Phe15.
Determination of Phenanthrene Content in Soil and White Clover
A certain amount of freeze-dried samples (soil and white clover) were ground, homogenized extracted and analyzed for the phenanthrene residues by HPLC (Gao et al., 2017). The recovery of phenanthrene in the soil and plant samples averaged ≥93.8% (n = 5, RSD ≤ 2.47%) after the entire procedure.
Statistical Analysis
SPSS 22.0 and Microsoft Excel 2016 software packages were used for data processing and analysis. The data point is the average of at least three repetitions; the difference was considered significant when p-value < 0.05, and the error bar represented standard deviation (SD).
Results and Discussion
Isolation and Identification of Strain Phe15
A Gram-negative rod-shaped strain Phe15, with phenanthrene-degrading function, was isolated from Eleusine indica (L.) Gaertn root surfaces. This strain has a terminal flagellum and its volume is about 1.8 × 0.7 μm (Figure 1A). The colonies of strain Phe15 are milky white with neat edges and smooth and moist surfaces (Figure 1B). The biochemical and physiological characteristics of Phe15 were determined and listed in Table 1. The 16S rRNA gene sequence of Phe15 (with GenBank No. of MT361874) shows more than 99% homology with that of strains belonging to Diaphorobacter sp. (Figure 1C). The combined results of morphology, 16S rRNA gene sequence and biochemical and physiological characteristics indicated that strain Phe15 belonged to Diaphorobacter sp.
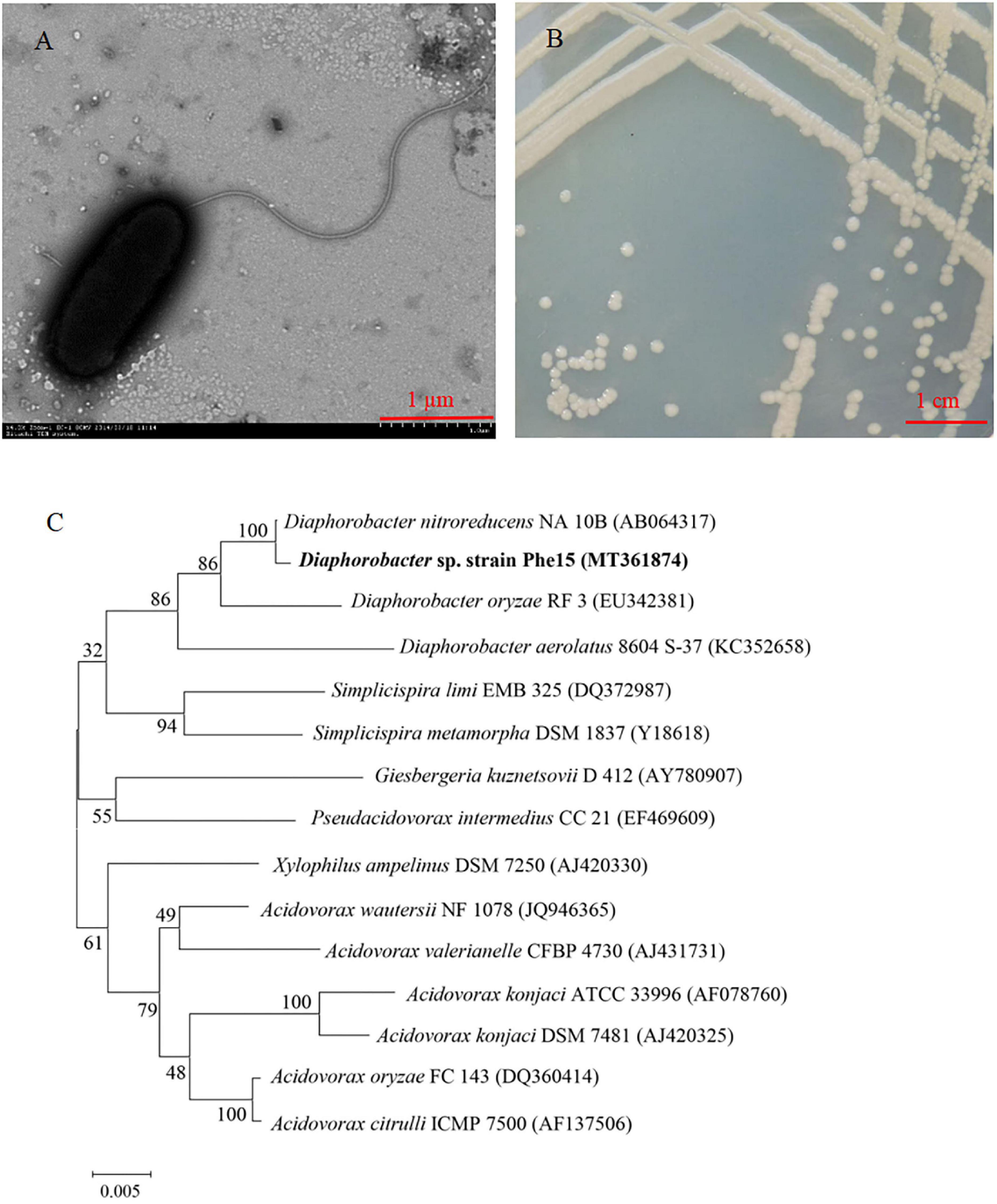
Figure 1. The identification of strain Phe15. (A) Transmission electron micrograph of strain Phe15 (× 4.0 k Zoom–1 HC–1 80 kV); (B) Colonial morphology of strain Phe15 on LB agar plate; (C) Phylogenetic analysis of strain Phe15 and related species using the neighbor joining method. Bootstrap values (%) are indicated at the nodes in a bootstrap analysis of 1000 replicates. The scale bar indicates 0.005 changes per nucleotide. The Gen Bank accession number for each bacterium used in the analysis is shown in parentheses after the species name.
Diaphorobacter sp. strains are widely found in water, soil, and sediment, it also accounted for 10% of the bacterial richness in indoor air (Miletto and Lindow, 2015). Diverse strains belonging to Diaphorobacter sp. could carry out simultaneous nitrification and denitrification under aerobic conditions, demonstrating a good potential for application in treatment of wastewater containing high nitrogen (Khardenavis et al., 2007). Furthermore, Diaphorobacter sp. strains also showed the ability to degrade various organic pollutants, such as 3-nitrotoluene (Singh and Ramanathan, 2013), 3, 4-dichloronitrobenzene (Gao et al., 2021), chloroaniline (Zhang et al., 2009), polyester polymer (Khan and Hiraishi, 2002; Qiu et al., 2015) and chlorphenuron herbicide (Zhang et al., 2018), indicating that these strains had a broad spectrum of organic pollutant degradation.
In addition, some Diaphorobacter sp. strains could also degrade PAHs. As early as 2009, Klankeo et al. (2009) isolated a strain, Diaphorobacter sp. KOTLB, from contaminated soil, which could degrade pyrene, phenanthrene, and anthracene effectively under experimental conditions. In 2016, Zhao et al. (2016) found the Diaphorobacter sp. contributed most of the dehydrogenases involved in PAH degradation in a cooperative metabolic network for fluoranthene degradation in polluted soil. Recently, strain Diaphorobacter sp. YM-6 was isolated from PAH-contaminated sediment and could degrade phenanthrene efficiently; it could degrade 96.3% of phenanthrene (with initial concentration of 100 mg⋅L–1) in liquid cultures within 52 h via phthalic acid pathway (Wang et al., 2020). In this study, strain Phe15 was isolated from the root surface of the PAH-contaminated plant, which is the first reported root-associated Diaphorobacter sp. strain with PAH-degrading ability.
Degradation of Phenanthrene by Diaphorobacter sp. Phe15
The dynamics of phenanthrene degradation by strain Phe15 were studied in liquid PMM. As shown in Figure 2A, more than 70% of the phenanthrene was degraded within 36 h and over 95% was degraded within 48 h in PMM. At the same time, the cell count of strain Phe15 increased rapidly from 12 to 36 h of cultivation, with a maximum cell count of 7.74 (Log cfu⋅mL–1).
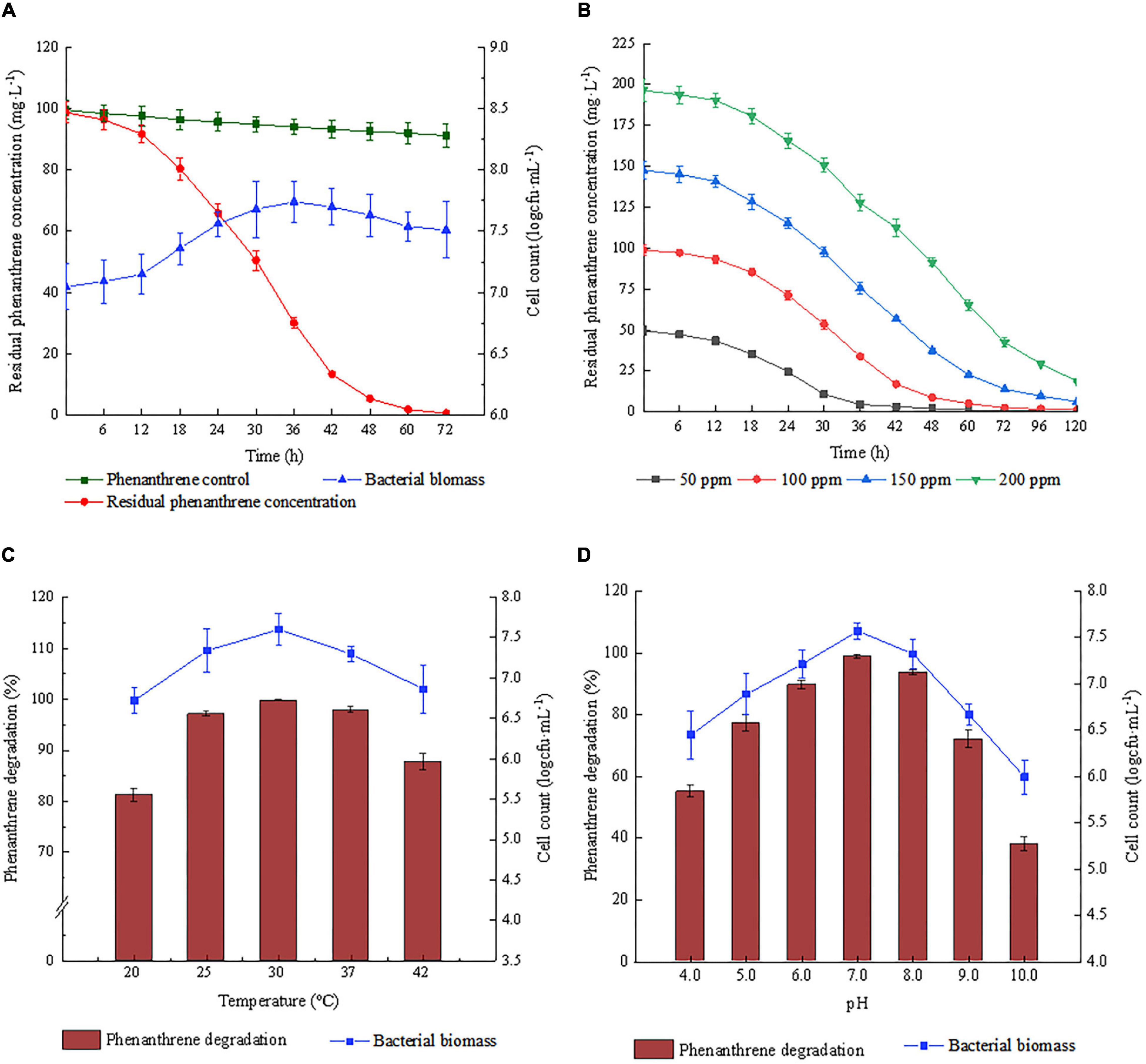
Figure 2. Degradation of phenanthrene by strain Phe15. (A) Degradation dynamics of phenanthrene and the growth curve of strain Phe15; (B) Effect of substrate concentration on phenanthrene degradation by strain Phe15; (C) Effect of temperature on phenanthrene degradation and cell growth of strain Phe15; (D) Effect of initial pH value on phenanthrene degradation and cell growth of strain Phe15. Error bars are standard deviations (SD), n = 3.
Strain Phe15 could degrade phenanthrene well at a wide range of temperatures, initial pH and phenanthrene concentrations. As shown in Figure 2B, the phenanthrene was almost completely degraded within 120 h at the concentrations of 50–200 mg⋅L–1. Strain Phe15 grew better in the temperature range of 20–42°C and could degrade more than 80% of phenanthrene. The optimum temperature is 30°C (Figure 2C). Meanwhile, Phe15 was highly stable under pH 5.0 to 9.0, and could degrade more than 70% of phenanthrene. The optimal pH was 7.0, and the degradation ratio was rapidly reduced to 40–50% when the pH value was lower than 4.0 or higher than 10.0 (Figure 2D).
Compared with the other PAH-degrading Diaphorobacter sp. strains, Phe15 demonstrated better degrading ability and higher tolerance to environmental conditions. For instance, it took 8 days for Diaphorobacter sp. KOTLB to fully degrade phenanthrene (with concentration of 100 mg⋅L–1) in liquid cultures (Klankeo et al., 2009), and 52 h for Diaphorobacter sp. YM-6 to degrade 96% of it (Klankeo et al., 2009); while for strain Phe15, it took 48 h to degrade 95% of it. When the pH value was below 6.0 or above 10.0, the phenanthrene degradation ratio by strain YM-6 quickly deceased to lower than 20%, while for Phe15, when the pH value was between 4.0–10.0, the degradation ratio was all higher than 40%. Obviously, strain Phe15 showed better potential for application in practical use in environmental remediation.
Biological Characteristics of Diaphorobacter sp. Phe15
Strain Phe15 was resistant to 25 mg⋅L–1 chloramphenicol and 75 mg⋅L–1 ampicillin, its distribution in the rhizosphere and inner plant tissues could be tracked by using the resistance to these two antibiotics as markers. Antibiotic markers, as well as the lacZ, xylE, and lux gene markers, are commonly used to track the bacterial environmental behaviors, and beneficial to the screening and counting of target strains (Prosser, 1994). For example, the ecological behavior of Pseudomonas mutants in organic soils was successfully monitored based on their resistance to rifampicin (Compeau et al., 1988). Furthermore, the environmental risk of fluoroquinolones was carefully evaluated via monitoring the resistance of Streptococcus pneumoniae to ciprofloxacin and other drugs (Sahm et al., 2000). These studies suggest that antibiotic markers are also a good option if wild strains are not suitable for genetic modification to add genetic markers.
Strain Phe15 could produce 15.89 mg⋅L–1 IAA in nitrogen medium and had a specific capacity for producing siderophores. It has been found that some nitrogen-fixing bacteria isolated from tropical grass species can easily colonize plants by producing certain factors that promote plant growth (Lugtenberg and Kamilova, 2009). Similarly, the bacteria inhibiting the growth of fungal pathogens by producing siderophores, have a high advantage in rhizosphere nutrition and niche competition (Schippers et al., 1987). These rhizosphere bacteria, which have mechanisms such as nitrogen fixation and phosphate dissolution, rhizosphere engineering and plant hormone production, are collectively referred as plant-promoting rhizosphere bacteria (PGPR), such as Rhizobia (Wang and Martínez-Romero, 2000), Pseudomonas fluorescens (Khalid et al., 2004) and Bacillus subtilis (Bhattacharyya and Jha, 2012). They can better colonize on the root surface and then migrate into inner plants.
The results of the colonization experiment showed that Phe15 could colonize well on white clover root surfaces and form aggregates (Figure 3). Studies have shown that these aggregates are conducive to the long-term existence of rhizosphere bacteria on the root surface (Singh et al., 2006), because bacteria in the aggregates have the ability to resist adverse living environments and microbial inhibitors (Davey and O’toole, 2000) and can induce the activities of some enzymes in inner plants to remove organic pollutants such as PAHs (Shehzadi et al., 2014). Therefore, rhizosphere degrading bacteria are often combined with plants to remove organic pollutants from soil, among which root-associated bacteria that could form aggregates or biofilms on the root surface are always preferred (Khan et al., 2013; Arslan et al., 2017).
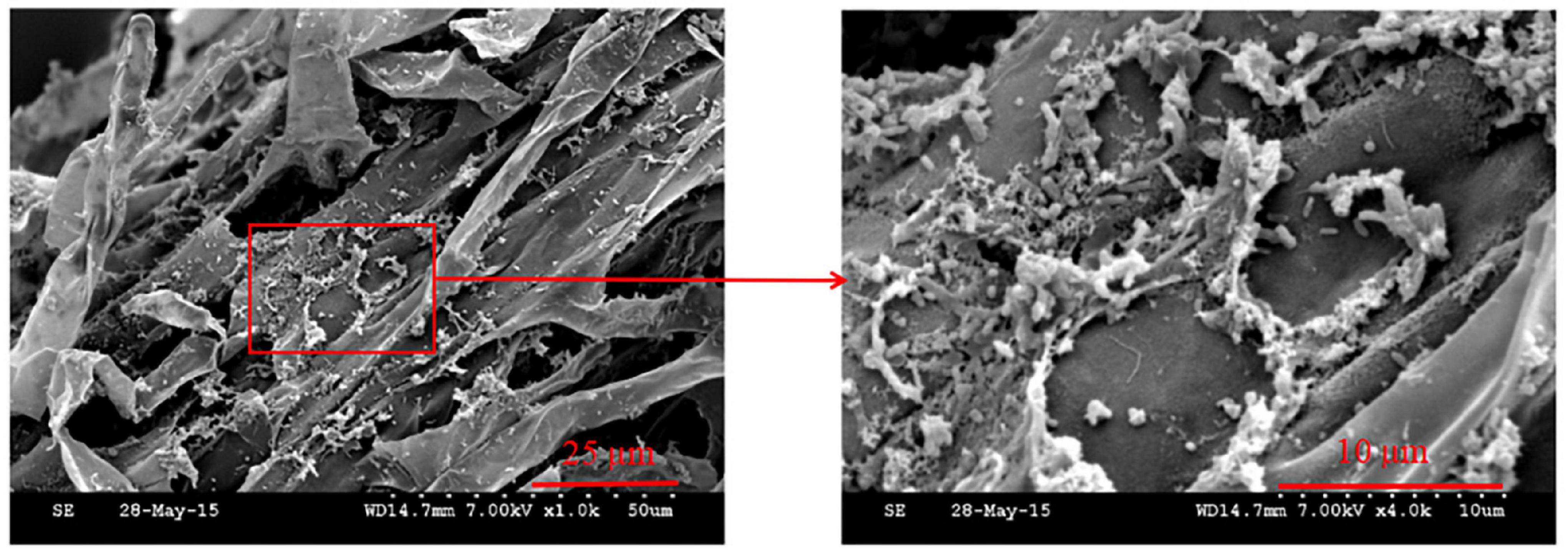
Figure 3. The colonization of strain Phe15 on the root surface of white clover observed by scanning electron microscope (WD14.7 mm 7.00 kV). The magnification of the left and right image is 1.0 and 4.0 k, respectively.
Colonization and Distribution of Diaphorobacter sp. Phe15 in White Clover
In the greenhouse container experiments, after inoculation of strain Phe15 for 30–50 days, most Phe15 cells efficiently colonized on the white clover root surfaces, and a few cells entered the inner tissues of white clover or were released into the rhizosphere soil (Table 2). For instance, the number of Phe15 cells was the highest on the root surface of white clover (5.58 Log CFU⋅g–1 FW via seed soaking and 6.03 Log CFU⋅g–1 FW via root irrigation) after inoculation for 30 d in phenanthrene contaminated soil.
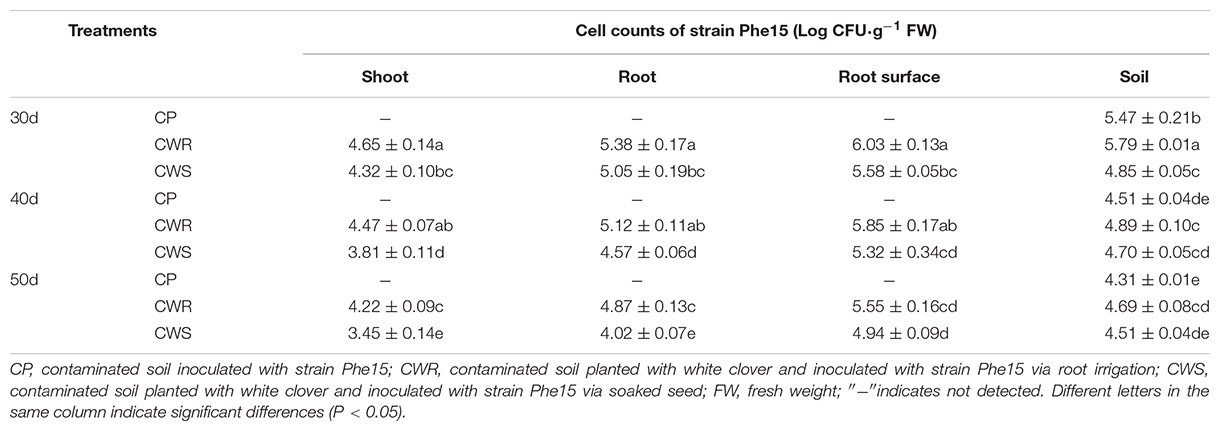
Table 2. The cell counts of strain Phe15 colonized on the root surface, in the inner tissues and soil after inoculation with white clover for 30, 40, and 50 d.
Furthermore, with the time extended from 30 to 50 days after inoculation, the number of Phe15 cells colonized in white clover and rhizosphere soil decreased obviously (P < 0.05). In most cases, the cell counts of Phe15 colonized in white clover plants via root irrigation were significantly higher than those via seed soaking (P < 0.05, Table 2). The reason may be that the cell count of Phe15 inoculated via seed soaking was much less, and the inoculation time was earlier than that via root irrigation. In addition, the decay rate of Phe15 counts in soil was also slowed down by planting white clover, indicating that white clover could provide a favorable environment for the colonization of Phe15 and facilitate its long-term survival in the system.
There are a considerable number of bacteria in the rhizosphere environment, and some of them could colonize the root surface of plants through the selection of root exudates (Bais et al., 2006; Wang et al., 2021); among which some bacteria could enter the inner tissues of roots and become endophytic bacteria (Mesa-Marin et al., 2019). In general, plant growth-promoting bacteria isolated from the root surface are more easily recolonized on the root surface of the plant and could survive in this system for a long time (Zhu et al., 2021), which is consistent with the results of this study. Strain Phe15 was isolated from the root surface of Eleusine indica (L.) Gaertn and could colonize and survive well on the root surface of white clover, some Phe15 cells could also enter the root tissue and transfer to shoot, suggesting that strain Phe15 might be a good candidate for phytoremediation of PAH-contaminated soil.
Colonization of Strain Phe15 Promoted the Growth of White Clover
As shown in Tables 3, 4, the high content of phenanthrene significantly inhibited growth of white clover during the first 40-day cultivation (P < 0.05), while the inoculation of Phe15 alleviated the inhibition obviously (P < 0.05). After cultivation for 50 days, the phenanthrene content in the soil is relatively low, basically relieving the phenanthrene stress to white clover, the inoculation of Phe15 significantly promoted the root growth of white clover via root irrigation (P < 0.05). Furthermore, compared with via seed soaking, more Phe15 cells colonized in white clover via root irrigation, demonstrating obvious advantage in relieving phenanthrene stress and promoting white clover growth.
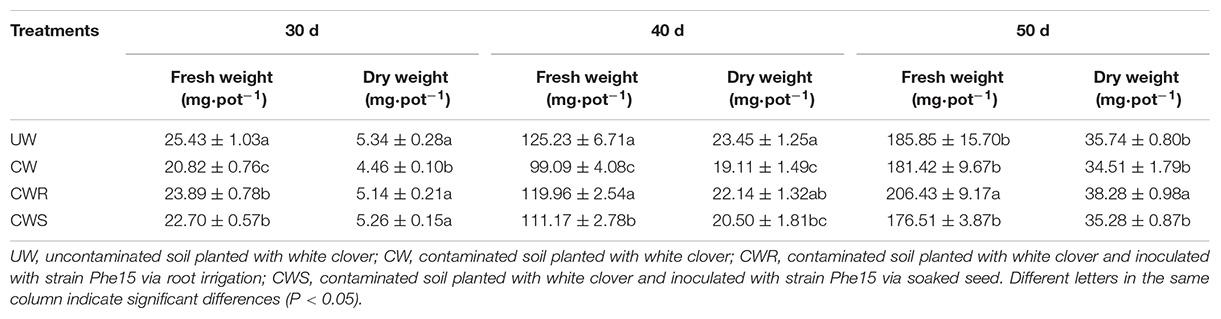
Table 3. The root biomass of white clover in different treatments (mg⋅pot–1) after inoculation with strain Phe15 for 30, 40, and 50 d.
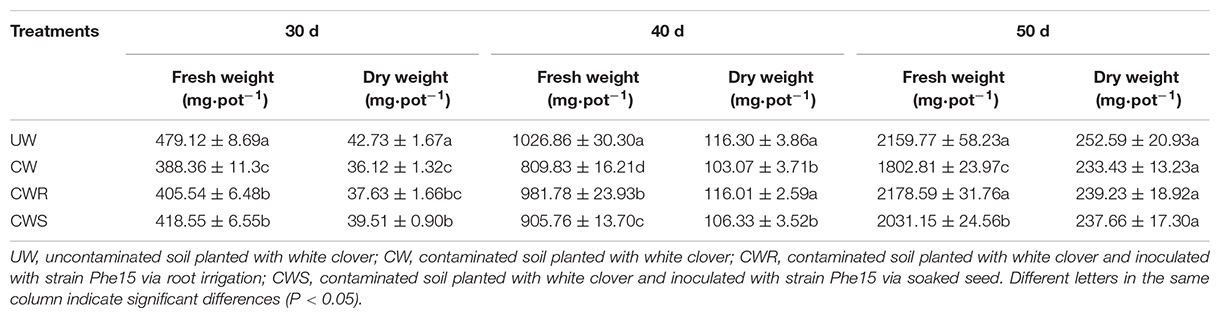
Table 4. The shoot biomass of white clover in different treatments (mg⋅pot–1) after inoculation with strain Phe15 for 30, 40, and 50 d.
Because Phe15 could degrade phenanthrene efficiently, after stable colonization on the root surface of white clover, it removed the phenanthrene from the white clover-soil system effectively and relieved the toxic stress to white clover caused by contamination. Meanwhile, Phe15 could also produce a certain amount of IAA and siderophores, significantly promoting the white clover growth after relieving the phenanthrene stress in white clover. Correspondingly, the white clover provided a stable habitat for Phe15, which could better play the role of phenanthrene degradation and plant growth promotion. Similar results were also found in previous studies (Zhou and Gao, 2019), indicating that well-constructed rhizosphere interaction between plants and bacteria could effectively remove soil organic pollutants and maintain a healthy rhizosphere environment.
Colonization of Strain Phe15 Reduced the Phenanthrene Contents in White Clover
The colonization of strain Phe15 on the white clover root surfaces effectively reduced the contents and accumulation of phenanthrene in white clover shoots and roots (Table 5). For instance, after 40 days of cultivation, compared with the Phe15-free treatments, in Phe15-inoculated treatments, the phenanthrene contents in shoots and roots of white clover were reduced by 51.29 and 43.16% via root irrigation and 48.39 and 35.40% via seed soaking, respectively (P < 0.05). Correspondingly, the accumulation of phenanthrene in white clover shoots and roots also decreased. Furthermore, the transfer and enrichment factor of phenanthrene in white clover were also obviously reduced after Phe15 inoculation. Meanwhile, compared with via seed soaking, inoculation of Phe15 with white clover via root irrigation was more effective for phenanthrene removal from white clover, and the difference was more obvious with the extension of cultivation time.
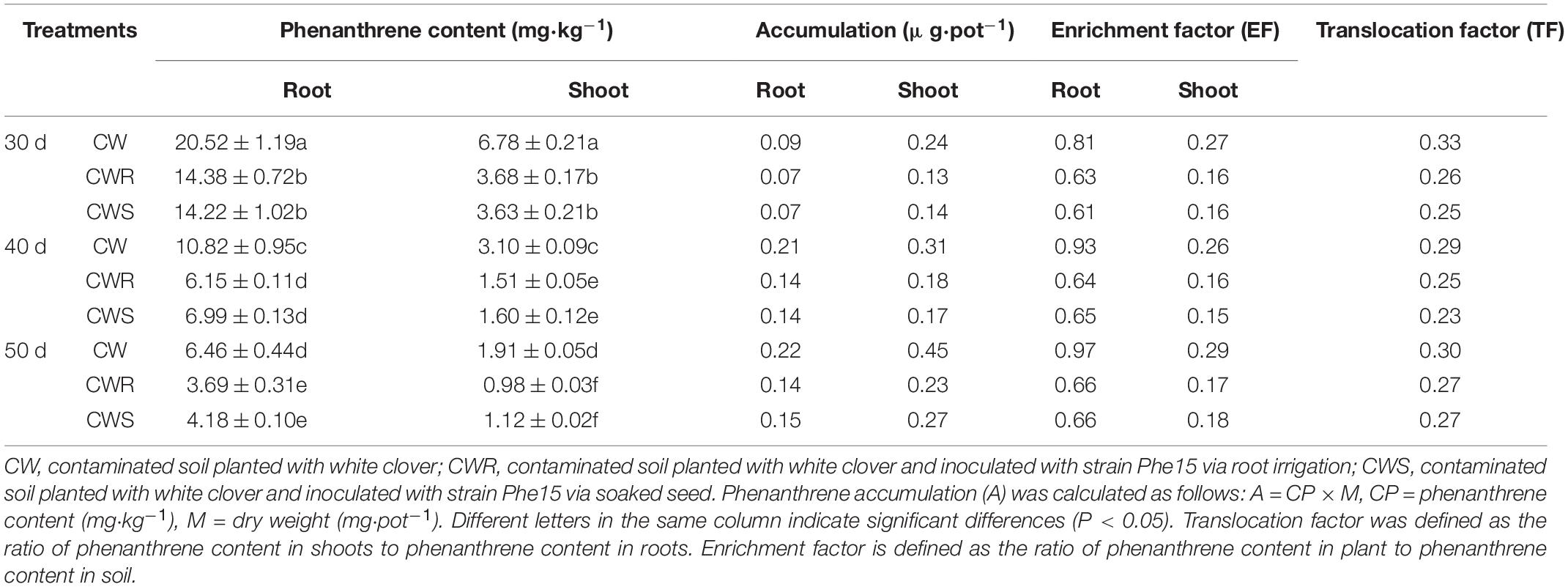
Table 5. The phenanthrene content and accumulation in white clover in different treatments after inoculation with strain Phe15 for 30, 40, and 50 d.
The root surface is a window for plants to take up organic pollutants. Degrading bacteria colonizing on plant root surfaces could quickly metabolize the organic pollutants absorbed on the root surface, thus preventing them from being taken up by plant, which is an effective method to reduce the content of organic pollutants in plant (Singh et al., 2006). In addition, because some degrading bacteria colonizing on the root surface can enter into plant and become endophytic bacteria, they could degrade organic pollutants in inner plant and reduce the pollutant accumulation (Sun et al., 2014). In this study, with phenanthrene as the representative PAHs, its uptake by white clover from the soil with or without Phe15 inoculation was investigated. The results revealed that Phe15 colonization on the white clover root surfaces could effectively remove phenanthrene from the inner white clover tissues.
Bacteria in root surfaces aggregates are often wrapped in extracellular polymers secreted by them, enhancing the solubility of phenanthrene, thus, making it easier to be utilized by bacteria (Johnsen and Karlson, 2004; Seo and Bishop, 2007). Under natural conditions, bacterial aggregates on the root surface are always formed by a variety of bacteria, which usually have different metabolic abilities and can complete a series of complex metabolic processes together, thus making the metabolism of phenanthrene more convenient and fast (Singh et al., 2006). In addition, because bacteria in the aggregation on the root surface are closely bound together, the horizontal migration of functional genes between bacterial cells are more convenient, enhancing the synergistic metabolism of phenanthrene on the root surface (Singh et al., 2006; Weyens et al., 2010; Arslan et al., 2017).
Colonization of Strain Phe15 Enhanced the Phenanthrene Removal From Soil
Compared with the untreated phenanthrene-contaminated soil (CK), the phenanthrene contents of the soil in the treated groups were significantly reduced (P < 0.05). The cooperative treatment of Phe15 and white clover (CWR and CWS) was obviously better than that of only inoculated with Phe15 (CP) or only planted white clover (CW) in removing phenanthrene from soil (P < 0.05). For example, after cultivation for 40 days, compared with the CK group, the phenanthrene content in soil inoculating with Phe15 (CP) or planting white clover (CW) decreased by 16.37 and 25.32%, respectively; while under the combination of Phe15 and white clover via root irrigation or seed soaking, the phenanthrene content in soil decreased by 39.00 and 31.71%, respectively (Figure 4).
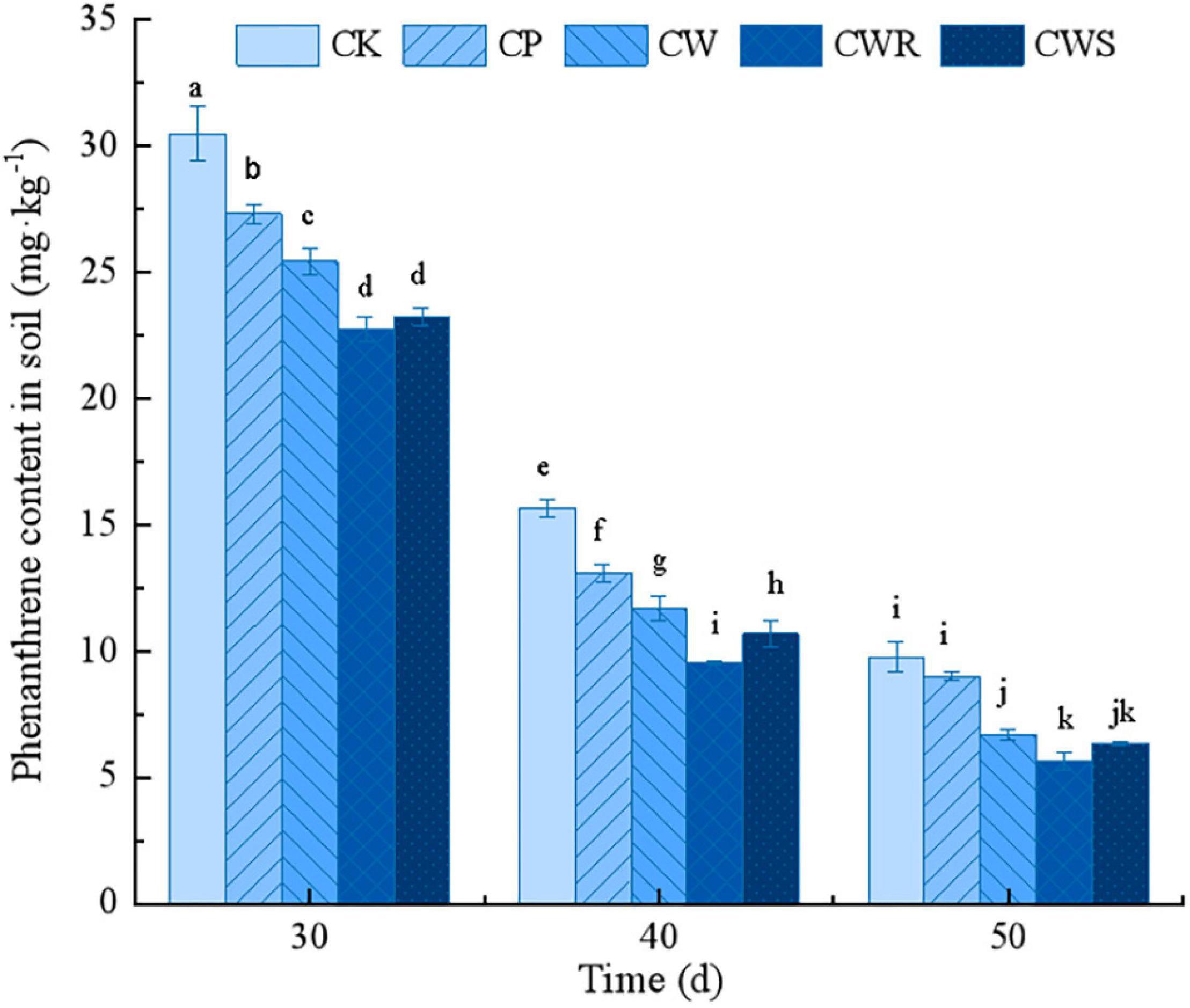
Figure 4. The phenanthrene residues in soil after inoculation for 30, 40, and 50 days. CK, contaminated soil; CP, contaminated soil inoculated with strain Phe15; CW, contaminated soil planted with white clover; CWR, contaminated soil planted with white clover and inoculated with strain Phe15 via root irrigation; CWS, contaminated soil planted with white clover and inoculated with strain Phe15 via soaked seed. The different lowercase letters on the bars indicate significant differences among treatments (P < 0.05).
The soil environment is relatively complex, there are many inhibitors limiting the activities of the enzymes involved in PAH degradation, among which the quorum sensing mechanism has a great impact on PAH degradation efficiency (Jung et al., 2020). In the practical soil bioremediation, nutrients such as nitrogen, phosphorus, and potassium are often added to the soil environment to promote the bacterial growth and PAH degradation (Arslan et al., 2014; Premnath et al., 2021). In contrast, growing plants provide a stable habitat and root exudates as the nutrient substances for root-associated PAH-degrading bacteria, which could degrade PAHs more efficiently and persistently, and plants themselves could also metabolize PAHs, thus obtaining higher removal efficiency than that via single inoculation of degrading bacteria (Dai et al., 2020). The results of this study confirmed this conclusion. At the same time, with the time extension from 30 to 50 days, the residual phenanthrene content in soil decreased much as a whole, indicating that indigenous bacteria and abiotic degradation also played essential roles in the phenanthrene removal process.
Conclusion
A root-associated phenanthrene-degrading bacterium, Diaphorobacter sp. Phe15, was isolated from Eleusine indica (L.) Gaertn and colonized on the root surface of white clover. Phe15 colonization observably reduced the phenanthrene content in white clover, promoted the white clover growth and enhanced the phenanthrene removal from soil. However, the effects of Phe15 colonization on phenanthrene metabolic enzyme activities and the molecular mechanisms involved in phenanthrene degradation in inner plants remain unclear. The richness and expression of phenanthrene-degrading genes in the soil-clover system need to be further studied. Only by supplementing and perfecting the above studies can we comprehensively and deeply analyse the mechanisms of reducing plant PAH pollution by using root-associated PAH-degrading bacteria, so as to provide a basis for the better application of this technology in practical agricultural production.
Data Availability Statement
The datasets presented in this study can be found in online repositories. The names of the repository/repositories and accession number(s) can be found below: https://www.ncbi.nlm.nih.gov/genbank/, MT361874.
Author Contributions
HZ conducted the experiments, analyzed the data, and wrote the manuscript. YG conducted the experiments and analyzed the data. XL analyzed the data and revised the manuscript. JL designed the study and wrote the manuscript. MW revised the manuscript. All authors contributed to the article and approved the submitted version.
Funding
This work was supported by the National Natural Science Foundation of China (31770549).
Conflict of Interest
The authors declare that the research was conducted in the absence of any commercial or financial relationships that could be construed as a potential conflict of interest.
Publisher’s Note
All claims expressed in this article are solely those of the authors and do not necessarily represent those of their affiliated organizations, or those of the publisher, the editors and the reviewers. Any product that may be evaluated in this article, or claim that may be made by its manufacturer, is not guaranteed or endorsed by the publisher.
References
Abdel-Shafy, H. I., and Mansour, M. S. M. (2016). A review on polycyclic aromatic hydrocarbons: source, environmental impact, effect on human health and remediation. Egypt. J. Pet. 25, 107–123. doi: 10.1016/j.ejpe.2015.03.011
Arslan, M., Afzal, M., Amin, I., Iqbal, S., and Khan, Q. M. (2014). Nutrients can enhance the abundance and expression of alkane hydroxylase CYP153 gene in the rhizosphere of ryegrass planted in hydrocarbon-polluted soil. PLoS One 9:e111208. doi: 10.1371/journal.pone.0111208
Arslan, M., Imran, A., Khan, Q. M., and Afzal, M. (2017). Plant–bacteria partnerships for the remediation of persistent organic pollutants. Environ. Sci. Pollut. Res. 24, 4322–4336. doi: 10.1007/s11356-015-4935-3
Bais, H. P., Weir, T. L., Perry, L. G., Gilroy, S., and Vivanco, J. M. (2006). The role of root exudates in rhizosphere interations with plants and other organisms. Annu. Rev. Plant Biol. 57, 233–266. doi: 10.1146/annurev.arplant.57.032905.105159
Bhattacharyya, P. N., and Jha, D. K. (2012). Plant growth-promoting rhizobacteria (PGPR): emergence in agriculture. World J. Microbiol. Biotechnol. 28, 1327–1350. doi: 10.1007/s11274-011-0979-9
Böltner, D., Godoy, P., Muñoz-Rojas, J., Duque, E., Moreno-Morillas, S., Sánchez, L., et al. (2008). Rhizoremediation of lindane by root-colonizing Sphingomonas. Microb. Biotechnol. 1, 87–93. doi: 10.1111/j.1751-7915.2007.00004.x
Chiou, C. T., McGroddy, S. E., and Kile, D. E. (1998). Partition characteristics of polycyclic aromatic hydrocarbons on soils and sediments. Environ. Sci. Technol. 32, 264–269. doi: 10.1021/es970614c
Compeau, G., Alachi, N. J., Platsouka, E., and Levy, S. B. (1988). Survival of rifampin-resistant mutants of Pseudomonas fluorescens and Pseudomonas putida in soil systems. Appl. Environ. Microbiol. 54, 2432–2438. doi: 10.1128/AEM.54.10.2432-2438.1988
Dai, Y., Liu, R., Zhou, Y., Li, N., Hou, L., Ma, Q., et al. (2020). Fire Phoenix facilitates phytoremediation of PAH-Cd co-contaminated soil through promotion of beneficial rhizosphere bacterial communities. Environ. Int. 136:105421. doi: 10.1016/j.envint.2019.105421
Davey, M. E., and O’toole, G. A. (2000). Microbial biofilms: from ecology to molecular genetics. Microbiol. Mol. Biol. Rev. 64, 847–867. doi: 10.1128/MMBR.64.4.847-867.2000
Falciglia, P. P., Guidi, G. D., Catalfo, A., and Vagliasindi, F. G. A. (2016). Remediation of soils contaminated with PAHs and nitro-PAHs using microwave irradiation. Chem. Eng. J. 296, 162–172. doi: 10.1016/j.cej.2016.03.099
Fismes, J., Perrin-Ganier, C., Empereur-Bissonnet, P., and Morel, J. L. (2002). Soil-to-root transfer and translocation of polycyclic aromatic hydrocarbons by vegetables grown on industrial contaminated soils. J. Environ. Qual. 31, 1649–1656. doi: 10.2134/jeq2002.1649
Gao, Y. Z., Hu, X., Zhou, Z., Zhang, W., Wang, Y., and Sun, B. (2017). Phytoavailability and mechanism of bound PAH residues in filed contaminated soils. Environ. Pollut. 222, 465–476. doi: 10.1016/j.envpol.2016.11.076
Gao, Y. Z., Li, Q. L., Ling, W. T., and Zhu, X. Z. (2011). Arbuscular mycorrhizal phytoremediation of soils contaminated with phenanthrene and pyrene. J. Hazard. Mater. 185, 703–709. doi: 10.1016/j.jhazmat.2010.09.076
Gao, Y. Z., Ling, W. T., and Wong, M. H. (2006). Plant-accelerated dissipation of phenanthrene and pyrene from water in the presence of a nonionic-surfactant. Chemosphere 63, 1560–1567. doi: 10.1016/j.chemosphere.2005.09.058
Gao, Y. Z., Palatucci, M. L., Waidner, L. A., Li, T., Guo, Y., Spain, J. C., et al. (2021). A nag-like dioxygenase initiates 3,4-dichloronitrobenzene degradation via 4,5-dichlorocatechol in Diaphorobacter sp. strain JS3050. Environ. Microbiol. 23, 1053–1065. doi: 10.1111/1462-2920.15295
Gao, Y. Z., Zhang, Y., Liu, J., and Kong, H. L. (2013). Metabolism and subcellular distribution of anthracene in tall fescue (Festuca arundinacea Schreb.). Plant Soil 365, 171–182. doi: 10.1007/s11104-012-1386-1
Gordon, S. A., and Weber, R. P. (1951). Colorimetric estimation of Indoleacetic Acid. Plant Physiol. 26, 192–195. doi: 10.1104/pp.26.1.192
Hwang, S., and Cutright, T. J. (2002). Biodegradability of aged pyrene and phenanthrene in a natural soil. Chemosphere 47, 891–899. doi: 10.1016/S0045-6535(02)00016-4
Johnsen, A. R., and Karlson, U. (2004). Evaluation of bacterial strategies to promote the bioavailability of polycyclic aromatic hydrocarbons. Appl. Microbiol. Biotechnol. 63, 452–459. doi: 10.1007/s00253-003-1265-z
Jung, B. K., Ibal, J. C., Pham, H. Q., Kim, M. C., Park, G. S., Hong, S. J., et al. (2020). Quorum sensing system affects the plant growth promotion traits of Serratia fonticola GS2. Front. Microbiol. 11:536865. doi: 10.3389/fmicb.2020.536865
Katznelson, H., and Bose, B. (1959). Metabolic activity and phosphate-dissolving capability of bacterial isolates from wheat roots, rhizosphere, and non-rhizosphere soil. Can. J. Microbiol. 5, 79–85. doi: 10.1139/m59-010
Khalid, A., Arshad, M., and Zahir, Z. A. (2004). Screening plant growth-promoting rhizobacteria for improving growth and yield of wheat. J. Appl. Microbiol. 96, 473–480. doi: 10.1046/j.1365-2672.2003.02161.x
Khan, S., Afzal, M., Iqbal, S., and Khan, Q. M. (2013). Plant–bacteria partnerships for the remediation of hydrocarbon contaminated soils. Chemosphere 90, 1317–1332. doi: 10.1016/j.chemosphere.2012.09.045
Khan, S. T., and Hiraishi, A. (2002). Diaphorobacter nitroreducens gen. nov., sp nov., a poly(3-hydroxybutyrate)-degrading denitrifying bacterium isolated from activated sludge. J. Gen. Appl. Microbiol. 48, 299–308. doi: 10.2323/jgam.48.299
Khardenavis, A. A., Kapley, A., and Purohit, H. J. (2007). Simultaneous nitrification and denitrification by diverse Diaphorobacter sp. Appl. Microbiol. Biotechnol. 77, 403–409. doi: 10.1007/s00253-007-1176-5
Klankeo, P., Nopcharoenkul, W., and Pinyakong, O. (2009). Two novel pyrene-degrading Diaphorobacter sp. and Pseudoxanthomonas sp. isolated from soil. J. Biosci. Bioeng. 108, 488–495. doi: 10.1016/j.jbiosc.2009.05.016
Li, W., Park, R., Alexandrou, N., Dryfhout-Clark, H., Brice, K., and Hung, H. (2021). Multi-year analyses reveal different trends, sources, and implications for source-related human health risks of atmospheric polycyclic aromatic hydrocarbons in the Canadian great lakes basin. Environ. Sci. Technol. 55, 2254–2264. doi: 10.1021/acs.est.0c07079
Liao, C. J., Liang, X. J., Liu, G. N., Thai, T., Xun, W. D., and Dang, Z. (2015). Effect of surfactant amendment to PAHs-contaminated soil for phytoremediation by maize (Zea mays L.). Ecotoxicol. Environ. Saf. 112, 1–6. doi: 10.1016/j.ecoenv.2014.10.025
Liu, J., Liu, S., Sun, K., Sheng, Y., Gu, Y. J., and Gao, Y. Z. (2014). Colonization on root surface by a phenanthrene-degrading endophytic bacterium and its application for reducing plant phenanthrene contamination. PLoS One 9:e108249. doi: 10.1371/journal.pone.0108249
Liu, J., Xiang, Y. B., Zhang, Z. M., Ling, W. T., and Gao, Y. Z. (2017). Inoculation of a phenanthrene-degrading endophytic bacterium reduces the phenanthrene level and alters the bacterial community structure in wheat. Appl. Microbiol. Biotechnol. 101, 5199–5212. doi: 10.1007/s00253-017-8247-z
Liu, J., Zhang, Z. M., Sheng, Y. H., Gao, Y. Z., and Zhao, Z. H. (2018). Phenanthrene-degrading bacteria on root surfaces: a natural defense that protects plants from phenanthrene contamination. Plant Soil 425, 335–350. doi: 10.1007/s11104-018-3575-z
Lugtenberg, B., and Kamilova, F. (2009). Plant-growth-promoting rhizobacteria. Annu. Rev. Microbiol. 63, 541–556. doi: 10.1146/annurev.micro.62.081307.162918
Marta, V., Clare, W., Martina, M., Jesper, M., María, S. C., Javier, L., et al. (2005). Polychlorinated biphenyl rhizoremediation by Pseudomonas fluorescens F113 derivatives, using a Sinorhizobium meliloti nod system to drive bph gene expression. Appl. Environ. Microbiol. 71, 2687–2694. doi: 10.1128/AEM.71.5.2687-2694.2005
Mesa-Marin, J., Mateos-Naranjo, E., Perez-Romero, J. A., Parra, R., Barcia-Piedras, J. M., Redondo-Gomez, S., et al. (2019). Soil phenanthrene phytoremediation capacity in bacteria-assisted Spartina Densiflora. Ecotoxicol. Environ. Saf. 182:109382. doi: 10.1016/j.ecoenv.2019.109382
Miletto, M., and Lindow, S. E. (2015). Relative and contextual contribution of different sources to the composition and abundance of indoor air bacteria in residences. Microbiome 3:61. doi: 10.1186/s40168-015-0128-z
Mueller, D. S., Jeffers, S. N., and Buck, J. W. (2005). Toxicity of fungicides to urediniospores of six rust fungi that occur on ornamental crops. Plant Dis. 89, 255–261. doi: 10.1094/PD-89-0255
Musa Bandowe, B. A., Sobocka, J., and Wilcke, W. (2011). Oxygen-containing polycyclic aromatic hydrocarbons (OPAHs) in urban soils of Bratislava, Slovakia: patterns, relation to PAHs and vertical distribution. Environ. Pollut. 159, 539–549. doi: 10.1016/j.envpol.2010.10.011
Premnath, N., Mohanrasu, K., Guru Raj Rao, R., Dinesh, G. H., Prakash, G. S., Ananthi, V., et al. (2021). A crucial review on polycyclic aromatic Hydrocarbons-Environmental occurrence and strategies for microbial degradation. Chemosphere 280:130608. doi: 10.1016/j.chemosphere.2021.130608
Prosser, J. I. (1994). Molecular marker systems for detection of genetically engineered micro-organisms in the environment. Microbiology 140, 5–17. doi: 10.1099/13500872-140-1-5
Qiu, T. L., Zuo, Z. Y., Gao, J. L., Gao, M., Han, M. L., Sun, L. J., et al. (2015). Diaphorobacter polyhydroxybutyrativorans sp. nov., a novel poly(3-hydroxybutyrate-co-3-hydroxyvalerate)-degrading bacterium isolated from biofilms. Int. J. Syst. Evol. Microbiol. 65, 2913–2918. doi: 10.1099/ijs.0.000353
Ramey, B. E., Koutsoudis, M., Von Bodman, S. B., and Fuqua, C. (2004). Biofilm formation in plant-microbe associations. Curr. Opin. Microbiol. 7, 602–609. doi: 10.1016/j.mib.2004.10.014
Sahm, D. F., Peterson, D. E., Critchley, I. A., and Thornsberry, C. F. (2000). Analysis of ciprofloxacin activity against Streptococcus pneumoniae after 10 years of use in the United States. Antimicrob. Agents Chemother. 44, 2521–2524. doi: 10.1128/AAC.44.9.2521-2524.2000
Schippers, B., Bakker, A. W., and Bakker, P. A. H. M. (1987). Interactions of deleterious and beneficial rhizosphere microorganisms and the effect of cropping practices. Annu. Rev. Phytopathol. 25, 339–358. doi: 10.1146/annurev.py.25.090187.002011
Seo, Y., and Bishop, P. L. (2007). Influence of nonionic surfactant on attached biofilm formation and phenanthrene bioavailability during simulated surfactant enhanced bioremediation. Environ. Sci. Technol. 41, 7107–7113. doi: 10.1021/es0701154
Shehzadi, M., Afzal, M., Khan, M. U., Islam, E., Mobin, A., Anwar, S., et al. (2014). Enhanced degradation of textile effluent in constructed wetland system using Typha Domingensis and textile effluent-degrading endophytic bacteria. Water Res. 58, 152–159. doi: 10.1016/j.watres.2014.03.064
Singh, D., and Ramanathan, G. (2013). Biomineralization of 3-nitrotoluene by Diaphorobacter species. Biodegradation 24, 645–655. doi: 10.1007/s10532-012-9612-3
Singh, D. P., Gadi, R., and Mandal, T. K. (2011). Characterization of particulate-bound polycyclic aromatic hydrocarbons and trace metals composition of urban air in Delhi, India. Atmos. Environ. 45, 7653–7663. doi: 10.1016/j.atmosenv.2011.02.058
Singh, R., Paul, D., and Jain, R. K. (2006). Biofilms: implications in bioremediation. Trends Microbiol. 14, 389–397. doi: 10.1016/j.tim.2006.07.001
Sun, K., Liu, J., Gao, Y. Z., Jin, L., Gu, Y. Z., and Wang, W. Q. (2014). Isolation, plant colonization potential and phenanthrene degradation performance of the endophytic bacterium Pseudomonas sp. Ph6- gfp. Sci. Rep. 4:5462. doi: 10.1038/srep05462
Tao, S., Cui, Y. H., Xu, F. L., Li, B. G., Cao, J., Liu, W. X., et al. (2004). Polycyclic aromatic hydrocarbons (PAHs) in agricultural soil and vegetables from Tianjin. Sci. Total. Environ. 320, 11–24. doi: 10.1016/S0048-9697(03)00453-4
Thimmaraju, R., Biedrzycki, M. L., and Bais, H. P. (2008). Causes and consequences of plant-associated biofilms. FEMS Microbiol. Ecol. 64, 153–166. doi: 10.1111/j.1574-6941.2008.00465.x
Wang, E. T., and Martínez-Romero, E. (2000). Sesbania herbacea-Rhizobium huautlense nodulation in flooded soils and comparative characterization of S. herbacea-Nodulating Rhizobia in different environments. Microb. Ecol. 40, 25–32. doi: 10.1007/s002480000010
Wang, H. W., Ma, C. Y., Xu, F. J., Lu, F., Zhang, W., and Dai, C. C. (2021). Root endophyte-enhanced peanut-rhizobia interaction is associated with regulation of root exudates. Microbiol. Res. 250:126765. doi: 10.1016/j.micres.2021.126765
Wang, P., Zhang, Y. M., Jin, J., Wang, T. H., Wang, J., and Jiang, B. Y. (2020). A high-efficiency phenanthrene-degrading Diaphorobacter sp. isolated from PAH-contaminated river sediment. Sci. Total Environ. 746:140455. doi: 10.1016/j.scitotenv.2020.140455
Weyens, N., Truyens, S., Dupae, J., Newman, L., Taghavi, S., van der Lelie, D., et al. (2010). Potential of the TCE-degrading endophyte Pseudomonas putida W619-TCE to improve plant growth and reduce TCE phytotoxicity and evapotranspiration in poplar cuttings. Environ. Pollut. 158, 2915–2919. doi: 10.1016/j.envpol.2010.06.004
Yamaga, F., Washio, K., and Morikawa, M. (2010). Sustainable biodegradation of phenol by Acinetobacter calcoaceticus P23 isolated from the rhizosphere of duckweed lemna aoukikusa. Environ. Sci. Technol. 44, 6470–6474. doi: 10.1021/es1007017
Yu, H. Y., Liu, Y. F., Han, C. X., Fang, H., Weng, J. H., Shu, X. Q., et al. (2021). Polycyclic aromatic hydrocarbons in surface waters from the seven main river basins of China: spatial distribution, source apportionment, and potential risk assessment. Sci. Total. Environ. 752:141764. doi: 10.1016/j.scitotenv.2020.141764
Yu, Y., Katsoyiannis, A., Bohlin-Nizzetto, P., Brorstrom-Lunden, E., Ma, J., Zhao, Y., et al. (2019). Polycyclic aromatic hydrocarbons not declining in arctic air despite global emission reduction. Environ. Sci. Technol. 53, 2375–2382. doi: 10.1021/acs.est.8b05353
Zhan, X. H., Ma, H. L., Zhou, L. X., Liang, J. R., Jiang, T. H., and Xu, G. H. (2010). Accumulation of phenanthrene by roots of intact wheat (Triticum acstivnm L.) seedlings: passive or active uptake? BMC Plant Biol. 10:52. doi: 10.1186/1471-2229-10-52
Zhang, L., Hang, P., Hu, Q., Chen, X. L., Zhou, X. Y., Chen, K., et al. (2018). Degradation of phenylurea herbicides by a novel bacterial consortium containing synergistically catabolic s and functionally complementary hydrolases. J. Agric. Food Chem. 66, 12479–12489. doi: 10.1021/acs.jafc.8b03703
Zhang, T., Ren, H. F., Liu, Y., Zhu, B. L., and Liu, Z. P. (2009). A novel degradation pathway of chloroaniline in Diaphorobacter sp. PCA039 entails initial hydroxylation. World J. Microbiol. Biotechnol. 26, 665–673. doi: 10.1007/s11274-009-0221-1
Zhang, X. G., Wang, N., Ma, G. D., Liu, Z. Y., Wei, G. X., and Liu, W. J. (2021). Preparation of siron-enriched yeast using siderophores and its effect on iron deficiency anemia in rats. Food Chem. 365:130508. doi: 10.1016/j.foodchem.2021.130508
Zhao, J. K., Li, X. M., Ai, G. M., Deng, Y., Liu, S. J., and Jiang, C. Y. (2016). Reconstruction of metabolic networks in a fluoranthene-degrading enrichments from polycyclic aromatic hydrocarbon polluted soil. J. Hazard. Mater. 318, 90–98. doi: 10.1016/j.jhazmat.2016.06.055
Zhou, Y. M., and Gao, X. R. (2019). Characterization of biofilm formed by phenanthrene-degrading bacteria on rice root surfaces for reduction of PAH contamination in rice. Int. J. Environ. Res. Public Health 16:2002. doi: 10.3390/ijerph16112002
Keywords: polycyclic aromatic hydrocarbons (PAHs), phenanthrene biodegradation, root-associated bacteria, colonization and distribution, plant uptake and accumulation
Citation: Zhao H, Gu Y, Liu X, Liu J and Waigi MG (2021) Reducing Phenanthrene Contamination in Trifolium repens L. With Root-Associated Phenanthrene-Degrading Bacterium Diaphorobacter sp. Phe15. Front. Microbiol. 12:792698. doi: 10.3389/fmicb.2021.792698
Received: 11 October 2021; Accepted: 29 October 2021;
Published: 26 November 2021.
Edited by:
Bin Ma, Zhejiang University, ChinaReviewed by:
Guangli Wang, Huaibei Normal University, ChinaYinping Zhang, Nanjing Normal University, China
Copyright © 2021 Zhao, Gu, Liu, Liu and Waigi. This is an open-access article distributed under the terms of the Creative Commons Attribution License (CC BY). The use, distribution or reproduction in other forums is permitted, provided the original author(s) and the copyright owner(s) are credited and that the original publication in this journal is cited, in accordance with accepted academic practice. No use, distribution or reproduction is permitted which does not comply with these terms.
*Correspondence: Juan Liu, bGl1anVhbkBuamF1LmVkdS5jbg==