- 1Department of Public Health, University of Naples Federico II, Naples, Italy
- 2Institute of Biostructures and Bioimaging, National Research Council, Naples, Italy
- 3Department of Molecular Medicine and Medical Biotechnology, University of Naples Federico II, Naples, Italy
The management of infections caused by Acinetobacter baumannii is hindered by its intrinsic tolerance to a wide variety of biocides. The aim of the study was to analyze the role of different A. baumannii efflux pumps (EPs) in tolerance to chlorhexidine (CHX) and benzalkonium (BZK) and identify non-toxic compounds, which can restore susceptibility to CHX and BZK in A. baumannii. A. baumannii ATCC 19606 strain was tolerant to both CHX and BZK with MIC and MBC value of 32 mg/L. CHX subMIC concentrations increased the expression of adeB and adeJ (RND superfamily), aceI (PACE family) and amvA (MFS superfamily) EP genes. The values of CHX MIC and MBC decreased by eightfold in ΔadeB and twofold in ΔamvA or ΔaceI mutants, respectively, while not affected in ΔadeJ mutant; EPs double and triple deletion mutants showed an additive effect on CHX MIC. CHX susceptibility was restored in double and triple deletion mutants with inactivation of adeB gene. BZK MIC was decreased by fourfold in ΔadeB mutant, and twofold in ΔamvA and ΔaceI mutants, respectively; EPs double and triple deletion mutants showed an additive effect on BZK MIC. BZK susceptibility was recovered in ΔadeB ΔaceI ΔadeJ and ΔamvA ΔadeB ΔadeJ triple mutants. The structural comparison of AdeB and AdeJ protomers showed a more negatively charged entrance binding site and F-loop in AdeB, which may favor the transport of CHX. The carbonyl cyanide m-chlorophenylhydrazine protonophore (CCCP) EP inhibitor reduced dose-dependently CHX MIC in A. baumannii ATCC 19606 and in ΔadeJ, ΔaceI, or ΔamvA mutants, but not in ΔadeB mutant. Either piperine (PIP) or resveratrol (RV) at non-toxic concentrations inhibited CHX MIC in A. baumannii ATCC 19606 parental strain and EPs gene deletion mutants, and CHX-induced EP gene expression. Also, RV inhibited BZK MIC and EP genes expression in A. baumannii ATCC 19606 parental strain and EPs mutants. These results demonstrate that tolerance to CHX and BZK in A. baumannii is mediated by the activation of AdeB, AceI and AmvA EPs, AdeB playing a major role. Importantly, inhibition of EP genes expression by RV restores CHX and BZK susceptibility in A. baumannii.
Introduction
Bacteria belonging to the genus Acinetobacter are glucose non-fermentative Gram-negative coccobacilli that are a frequent cause of health-care associated infections and hospital outbreaks. A. baumannii represents the most clinically relevant species among those belonging to the A. baumannii-calcoaceticus group (Wong et al., 2017). Global epidemiology of A. baumannii shows a clonal population structure dominated by two major international clonal lineages and few additional epidemic clones (Gaiarsa et al., 2019). The most successful Acinetobacter clones show resistance to a broad range of antimicrobials and tolerance to disinfectants and share virulence features such as biofilm formation on biotic and abiotic surfaces, resistance to desiccation and adherence to epithelial cells (Giannouli et al., 2013; Wong et al., 2017; Harding et al., 2018). A. baumannii strains responsible for nosocomial outbreaks are resistant to a wide range of antimicrobials, resistance to carbapenems being present in more than 90% of them and resistance to colistin emerging also (Wong et al., 2017).
A. baumannii persistence in the contaminated hospital environment is contributed also by reduced susceptibility of the bacteria to a broad range of biocides used as antiseptics or disinfectants, such as the bisphenol triclosan (TRI), the quaternary ammonium compounds benzalkonium chloride (BZK), dequalinium chloride (DQ), and cetrimide (CT), and the biguanide chlorhexidine (CHX) (McDonnell and Russell, 1999). CHX is a positively charged molecule able to react with the negatively charged microbial cell surface, thereby destroying the integrity of the cell membrane (McDonnell and Russell, 1999). CHX is a bactericidal agent, which is widely used for hand hygiene, skin antisepsis, oral care, and patient washing (Milstone et al., 2008). BZK has been widespread used as disinfectant in hospitals, food industry and commercial products, or antiseptic in antimicrobial soaps (Merchel Piovesan Pereira and Tagkopoulos, 2019). Reduced susceptibility to CHX and BZK is emerging in various nosocomial pathogens (Kampf, 2016; Merchel Piovesan Pereira and Tagkopoulos, 2019; Weber et al., 2019). Reduced susceptibility to CHX in A. baumannii has been correlated with activation of different efflux systems (Rajamohan et al., 2010a,b; Hassan et al., 2013; Tucker et al., 2014; Du et al., 2018; Harding et al., 2018; Kornelsen and Kumar, 2021). In particular, activation of AdeB and AdeJ resistance–nodulation–cell division (RND) efflux systems (Rajamohan et al., 2010a; Tucker et al., 2014), AmvA and CraA major facilitator superfamily (MFS) efflux systems (Rajamohan et al., 2010b; Foong et al., 2019) have been shown to induce tolerance to CHX and other disinfectants in clinical A. baumannii isolates. Reduced susceptibility to chlorhexidine has also been associated with activation of AceI proteobacterial antimicrobial compound efflux (PACE) system in A. baumannii ATCC17978 (Hassan et al., 2013; Tucker et al., 2014).
Non-toxic natural substances such as the alkaloid piperine (Haq et al., 2021) and the monomeric stilbenoid resveratrol (Mattio et al., 2020) are able to modulate the susceptibility to CHX in A. baumannii and other bacteria (Sharma et al., 2010; Mirza et al., 2011; Singkham-In et al., 2020).
The objectives of the present study were to: (i) study the contribution of efflux pump systems to and the molecular mechanisms responsible for tolerance to CHX and BZK in A. baumannii; (ii) identify non-toxic compounds, which can modulate and restore susceptibility to CHX and BZK in A. baumannii.
Materials and Methods
Bacterial Strain, Growth Condition, Antibiotics, and Reagents
A. baumannii ACICU (Iacono et al., 2008), A. baumannii AYE (Poirel et al., 2003), A. baumannii ATCC 19606 (Janssen et al., 1997), Escherichia coli 25922 and E. coli S17 λpir (Simon et al., 1983) strains were used for this study. E. coli ATCC 25922 was purchased from LGC Standards S.r.l., Italy). All strains were cultured under aerobic conditions at 37°C in Luria-Bertani (LB) broth/agar. LB broth, cation-adjusted Mueller-Hinton broth (CAMHB) and Tryptic soy broth (TSB) were used to perform growth curves, susceptibility tests and biofilm assays. The chemical reagents were chlorhexidine digluconate (CHX), carbonyl cyanide m-chlorophenylhydrazine (CCCP), triclosan (5-chloro-2-(2,4-dichlorophenoxy) phenol (TRI), the quaternary ammonium compounds benzalkonium chloride (alkylbenzyldimethylammonium chloride (BZK), dequalinium chloride (DQ), and cetrimide (alkyltrimethylammonium bromide (CT), piperine (1-piperoyliperidine, PIP) and resveratrol (3,5,4’-trihydroxy-trans-stilbene, RV). The antimicrobials and chemical reagents were purchased from Sigma-Aldrich (Sigma, Milan, Italy).
Construction of adeB, adeJ, aceI, and amvA Gene Knockouts
DNA and plasmid DNAs of A. baumannii ATCC 19606 and knockout mutants were extracted using the DNeasy Blood & Tissue Kit (Qiagen, Milan, Italy) and the Plasmid Mini/Midi Kits (Qiagen, Milan, Italy), respectively, according to the manufacturer’s instructions. A. baumannii ATCC 19606 was mutagenized as previously described (Amin et al., 2013; De Gregorio et al., 2015) with the following minor changes. The upstream and downstream fragments of target genes were amplified using the primers listed in Supplementary Table 1 and inserted in the TA Cloning pCR2.1 vector (Invitrogen); 100 μL of competent E. coli DH5α were transformed with TA-cloning vector. The upstream fragments were digested with NotI-BamHI and cloned into suicide vector pMo130-TelR, creating pMo130-TelR-Up. Next, the downstream fragments were digested with BamHI–SphI and inserted in pMo130-TelR-Up to obtain the plasmid pMo130-TelR-Up/Dw. The final plasmid was introduced into E. coli S17-1 λpir by CaCl2 transformation and mobilized to the A. baumannii ATCC 19606 strain or single/double mutants via conjugation as described (Amin et al., 2013), to obtain single, double and triple mutants. Transconjugants were selected in LB agar containing 30 mg/L tellurite + 50 mg/L ampicillin and 50 mg/L kanamycin + 50 mg/L ampicillin, cultured in LB broth containing 14% sucrose. Serial dilutions were spread onto LB plates containing 14% sucrose. Colonies were screened for tellurite sensitivity to monitor excision of the suicide vector. The inactivation of adeB, adeJ, aceI and amvA genes were confirmed by PCR amplification using control primers (Supplementary Table 1).
Determination of Minimum Inhibitory Concentration and Minimum Bactericidal Concentration
A. baumannii ATCC 19606 was grown overnight at 37°C on LB broth, under shaking (200 rpm). The MIC and MBC of CHX was determined by a manual microdilution method according to the recommended procedures by the European Committee for Antimicrobial Susceptibility Testing (Eucast) of the European Society of Clinical Microbiology and Infectious Diseases (Escmid) (2000) and the Clinical and Laboratory Standards (CLSI, 2019). Susceptibility was assessed to MIC value < 4 mg/L as described (Rajamohan et al., 2010a). A. baumannii ATCC 19606 and deletion mutants were grown on CAMHB at 37°C for 24 h. Afterward, 50 μL of 1 × 106 CFU/mL bacterial cells were added to each well of the microtiter plate containing 50 μL of the CAMHB with twice the final concentration of molecules studied. Then the plates were incubated at 37°C for 18–24 h. Non-treated bacteria were used as controls. All tests were performed in triplicate and repeated three times.
In vitro Combination Studies
The tests were carried out using the checkerboard method according to the previously reported method (Hall et al., 1983). Serial dilutions of CHX (0.5–164 mg/L) were prepared and combined with serial dilutions of piperine (8–128 mg/L), resveratrol (32–128 mg/L), CCCP (0.5, 1, and 2 mg/L). Subsequently, 1 × 106 CFU/mL of either A. baumannii ATCC 19606 or deletion mutants were added to each well of the microtiter plate. Then the plates were incubated at 37°C for 18–24 h. All experiments were repeated three times.
Biofilm Assay
Biofilm formation was examined using a crystal violet (CV) staining assay according to the previously reported method (De Gregorio et al., 2020). Bacterial cell suspension was prepared at 0.5 McFarland standard and it was diluted 1:100 in TSB. Subsequently, 100 μL of 1 × 106 cells/mL was transferred into a 96-well flat-bottomed polystyrene microtiter plate containing 100 μL of scalar doses of CHX (164–0.5 g mg/L) and incubated at 37°C for 24 h. Non-treated bacteria were incubated with 100 μL of broth and used as the control. The culture supernatant was gently discarded, the wells were washed twice with phosphate-buffered saline (PBS) 1 × pH 7.4 and the biofilms were stained with 200 μL of 0.1% crystal violet for 20 min. The wells were washed twice with PBS 1X, and dye was re-eluted with 100% ethanol. The absorbance was measured at 595 nm using a microplate reader (Bio-Rad Laboratories S.r.l.). The OD595/OD600 ratio was used to normalize the amount of biofilm formed to the total cell content.
RNA Purification and Real-Time RT-PCR
A. baumannii ATCC 19606 cells were grown over night on LB broth at 37°C at 200 rpm. Subsequently, ATCC 19606 was diluted 1:100 in LB broth alone or LB broth with subMIC of CHX or RV or PIP or CHX plus RV or CHX plus PIP and grown at 37°C at 200 rpm for a further 3 h to reach the exponential phase (OD600 = 0.5). Total RNA was isolated from three independent cultures according to the previously reported method (De Gregorio et al., 2018). The cDNAs were synthesized using QuantiTect Reverse Transcription Kit (Qiagen, Milan, Italy), according to the manufacturer’s protocol. Real-time RT-PCR assays were performed using SYBR Green master mix (Applied Biosystems) (Martinucci et al., 2016). The rpoB gene (the housekeeping gene) was used to normalize the expressions of target genes. The fold-change of the gene expression level was calculated using the 2–ΔΔct method (Livak and Schmittgen, 2001). All experiments were performed three times in triplicate. The primers used in the qRT-PCR experiments were reported in Supplementary Table 2.
Statistical Analysis
All statistical analyses were carried out using GraphPad Prism version 8.0 for Windows (GraphPad Software, San Diego, CA, United States). All experiments were performed at least three times and the results are shown as means ± SD. Differences between mean values were tested for significance using ANOVA. A P < 0.05 was considered to be statistically significant.
Structural Analysis
Comparison of cryo EM structures of AdeB (PDB code 7 kgd) and AdeJ (PDB code 7 m4q) were conducted using the DALI platform for pairwise alignment (Holm, 2020) and the software Coot (Emsley and Cowtan, 2004) and PyMol (Seeliger and de Groot, 2010).
Results
Effect of Chlorhexidine Digluconate on A. baumannii ATCC 19606
A. baumannii ATCC 19606, AYE, ACICU strains having different antimicrobial susceptibility profiles and classified as susceptible, multidrug-resistant (MDR) and extensively drug-resistant (XDR) as described (Magiorakos et al., 2012), respectively, invariably showed both CHX MIC and MBC values of 32 mg/L and were considered tolerant to CHX (Table 1). Instead, E. coli ATCC 25922 showed a CHX MIC/MBC value of 2 mg/L and was considered susceptible (Table 1). A. baumannii ATCC 19606 was able to grow and retain viability in the presence of 4–16 mg/L subMIC concentrations of CHX, while A. baumannii ATCC 19606 growth was abolished at 32 mg/L CHX (Figure 1). Also, CHX subMIC concentrations of 8 and 16 mg/L decreased stationary phase cell density of A. baumannii ATCC 19606 by three and fourfold, respectively (Figure 1).
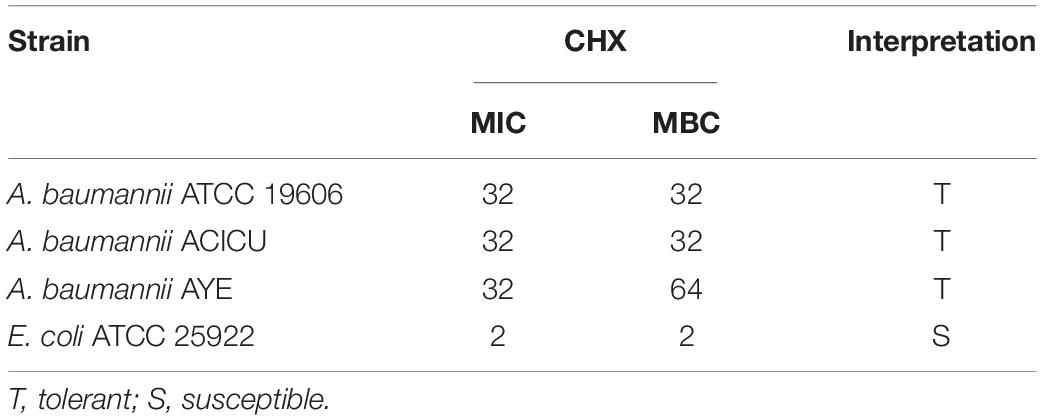
Table 1. MIC (mg/L) and MBC (mg/L) values of CHX against A. baumannii strains and E. coli reference strain.
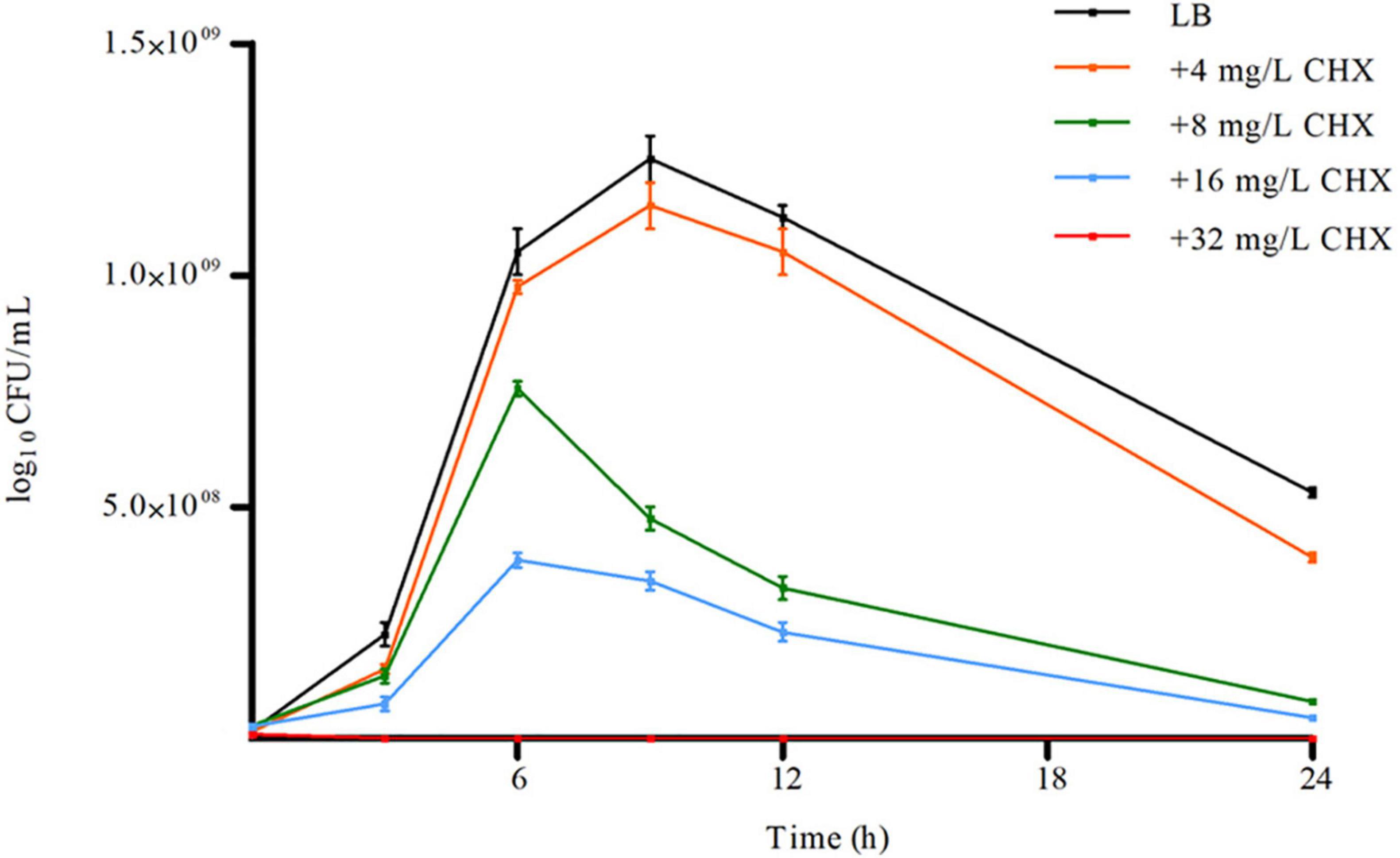
Figure 1. Effect of increasing concentration of CHX on A. baumannii ATCC 19606 planktonic growth. Error bars represent standard deviations based on three independent experiments. CFU, colony-forming units.
Because it has been demonstrated that CHX increased the expression of aceI efflux pump (EP) gene in A. baumannii ATCC 17978 (Hassan et al., 2013), we asked if CHX was able to regulate the expression of EPs genes in ATCC 19606. Preliminary data showed that basal level of expression of adeB, adeG, adeJ, belonging to RND superfamily, amvA and craA belonging to MFS superfamily, aceI, belonging to PACE superfamily, and abeS and abeM, belonging to the SMR superfamily were different in A. baumannii ATCC 19606. In particular, aceI, adeJ, adeB, and amvA were expressed at high levels, with expression levels normalized on rpoB of 0.49, 0.34, 0.25, and 0.28, respectively, while craA, abeS, and abeM at low levels (Supplementary Figure 1). As shown in Figure 2, CHX at subMIC concentrations (4 and 8 mg/L) increased the expression of adeB and adeJ EPs genes by 6x and 2x, respectively, while the expression of adeG EP gene and adeR and adeS regulatory genes were not affected. Moreover, subMIC concentrations of CHX increased the expression of aceI EP gene and amvA EP gene 5x by 4 mg/mL and 9x by 8 mg/mL, and 2x by 4 mg/mL, respectively (Figure 2). amvA EP gene expression was not induced in the presence of 8 mg/mL CHX. On the other hand, subMIC concentrations of CHX decreased the expression of craA EP gene 4x by 4 mg/L and 8x by 8 mg/L (Figure 2). The above data indicated that adeB, aceI and to lesser extent adeJ and amvA EP genes are activated by CHX in A. baumannii ATCC 19606.
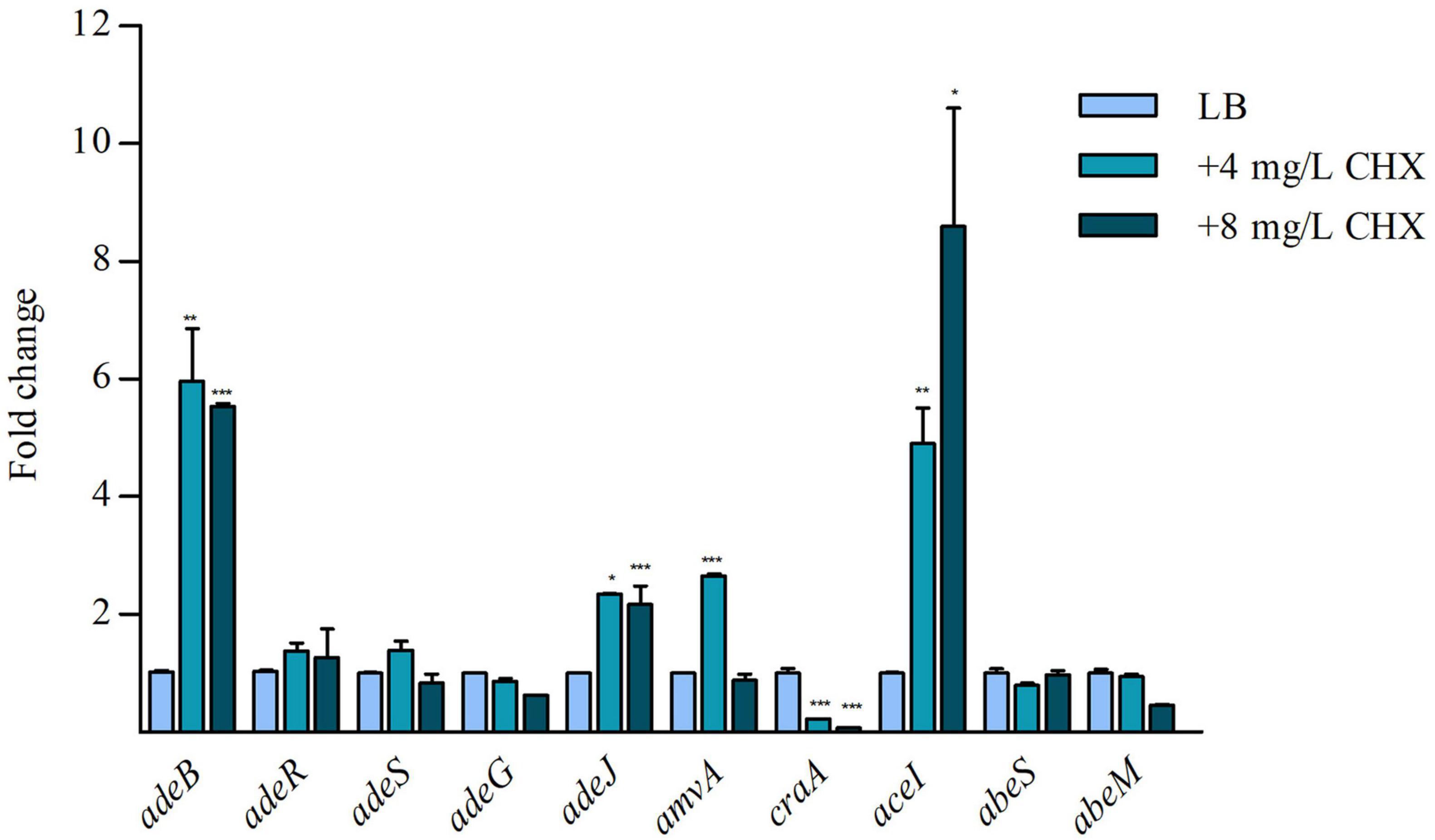
Figure 2. RT-qPCR assay of adeB, adeR, adeS, adeG, adeJ, amvA, craA, aceI, abeS, and abeM expression in the presence of LB and 4 mg/L and 8 mg/L CHX. Relative number of transcripts of each gene was normalized in each condition and calculated using the 2–ΔΔct method compared to the expression level in LB control. The mean + standard deviation of relative number of transcripts is shown for each gene. All experiments were performed in triplicate. p-values were calculated using ANOVA (*p < 0.05; **p < 0.01; ***p < 0.001).
Effect of Efflux Pumps Inactivation on Chlorhexidine Minimum Inhibitory Concentration and Minimum Bactericidal Concentration, Planktonic and Sessile Growth in A. baumannii ATCC 19606
To study the molecular mechanisms responsible for tolerance to CHX in A. baumannii, we analyzed the effect of inactivation of AdeB and AdeJ, AceI, and AmvA EPs, which are abundantly expressed and positively regulated by CHX in A. baumannii ATCC19606, on susceptibility to CHX. To this aim, CHX MIC and MBC were analyzed in A. baumannii ATCC 19606 marker-less mutants of adeB, adeJ, aceI and amvA EPs genes. As shown in Table 2, CHX MIC and MBC values were decreased by eight and twofold in ΔadeB and in ΔaceI mutant, respectively, compared with A. baumannii ATCC19606; in ΔamvA mutant CHX MIC was also decreased by twofold but CHX MBC was not affected. Instead, CHX MIC and MBC in ΔadeJ mutant were similar to A. baumannii ATCC19606 (Table 2). Furthermore, CHX MIC and MBC values were decreased by 16-fold in ΔadeB ΔaceI and ΔadeB ΔadeJ double mutants, eightfold in ΔamvA ΔadeB, and fourfold in ΔamvA ΔaceI double mutant, while CHX MIC was decreased by two fold, but CHX MBC not affected in ΔamvA ΔadeJ and ΔaceI ΔadeJ double mutants. Moreover, CHX MIC and MBC were decreased by 32-fold in ΔadeB ΔaceI ΔadeJ, 16-fold in ΔamvA ΔadeB ΔaceI and ΔamvA ΔadeB ΔadeJ, and twofold in ΔamvA ΔaceI ΔadeJ triple mutants (Table 2). CHX susceptibility with MIC and MBC values of 2–1 was recovered in ΔadeB ΔaceI and ΔadeB ΔadeJ double mutants, and ΔadeB ΔaceI ΔadeJ, ΔamvA ΔadeB ΔaceI, and ΔamvA ΔadeB ΔadeJ triple mutants (Table 2). The above data indicated that CHX MIC and MBC in A. baumannii ATCC 19606 were mainly sustained by the expression of adeB and that aceI, amvA and to a lesser extent adeJ played an additive effect.
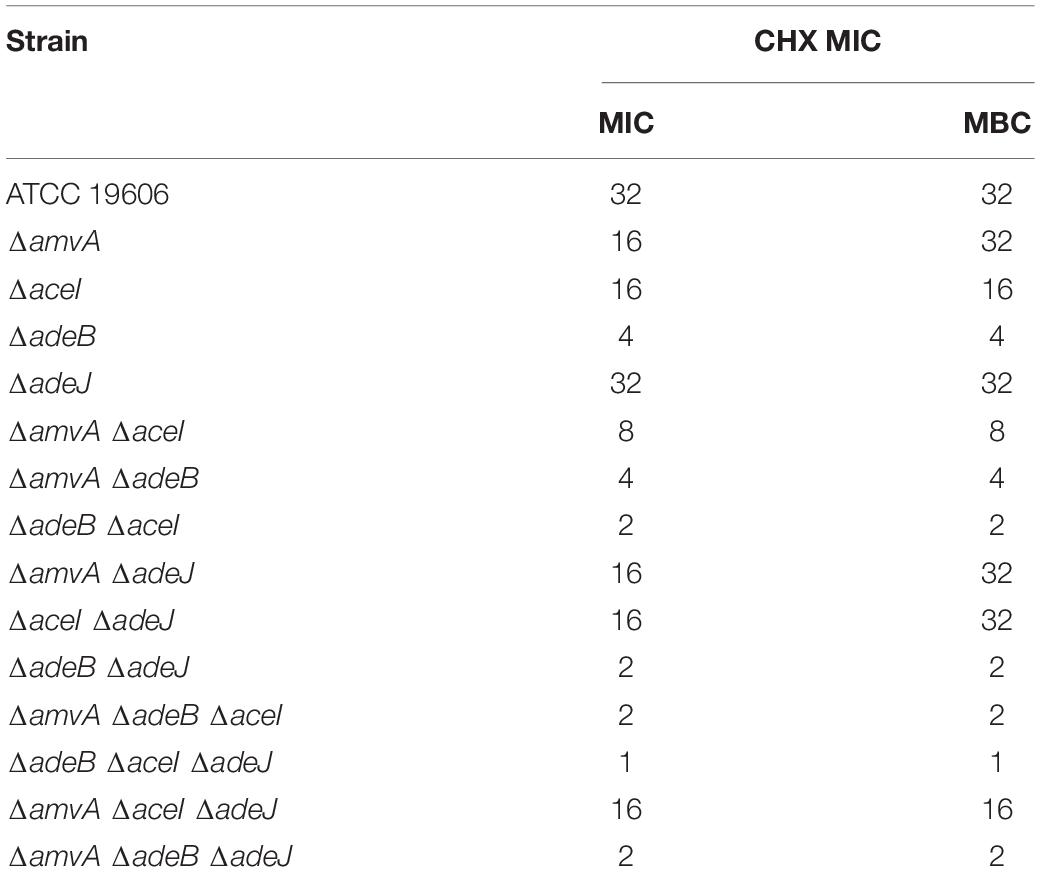
Table 2. CHX MIC (mg/L) and MBC (mg/L) of A. baumannii ATCC 19606 parental strain and EP deletion mutants.
To further study the role of EPs on CHX susceptibility in A. baumannii, we analyzed the effect the EP inhibitor CCCP in A. baumannii ATCC 19606 and EPs marker-less mutants. As shown in Table 3, CCCP reduced dose-dependently CHX MIC in A. baumannii ATCC 19606 and in ΔadeJ, ΔaceI, or ΔamvA single, double or triple mutants. CCCP reduced CHX MIC in ΔadeB, single, double or triple mutants but the effect was not dose-dependent. This indicates that inhibition of efflux pump activity restores susceptibility to CHX in A. baumannii ATCC 19606 and in ΔadeJ, ΔaceI, or ΔamvA, but not in ΔadeB mutants.
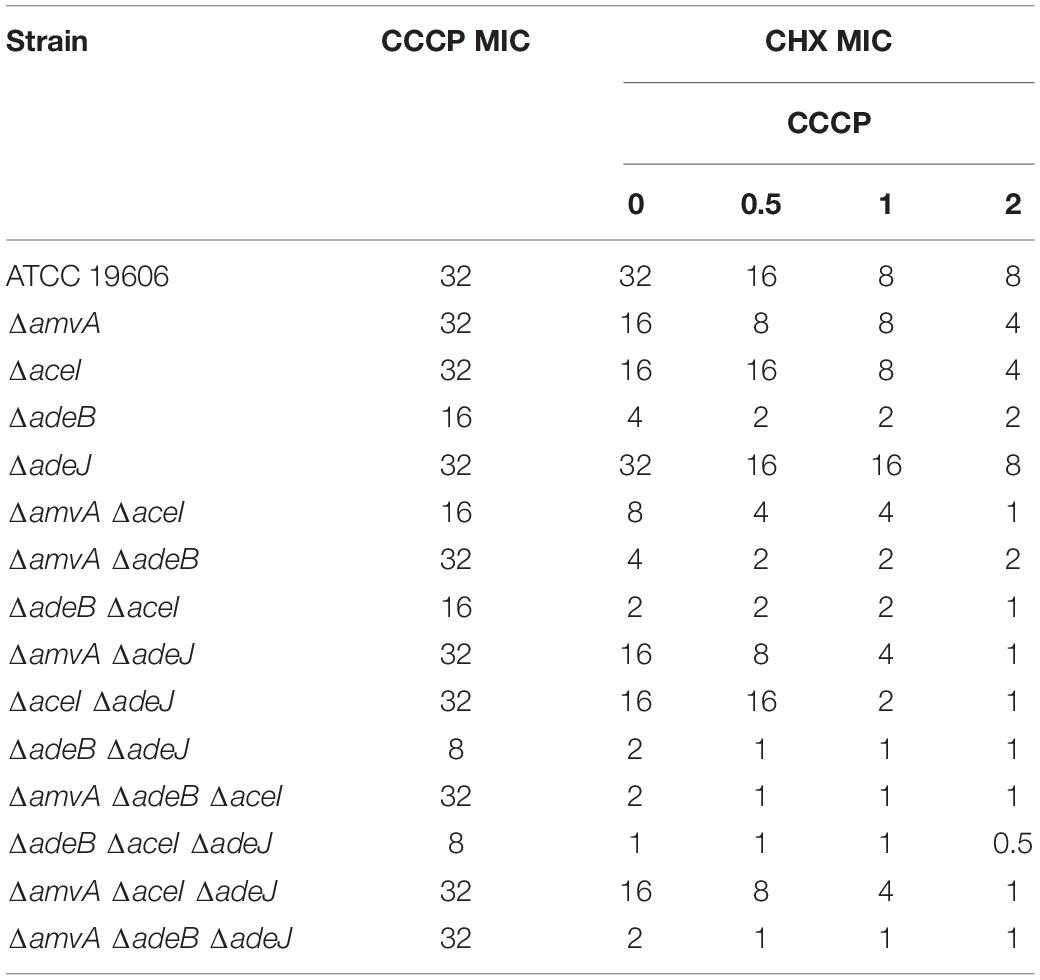
Table 3. MIC of CHX (mg/L) in combination with CCCP of A. baumannii ATCC 19606 parental strain and EP deletion mutants.
We next asked whether EPs knockout gene inactivation might affect the in vitro planktonic and sessile growth of A. baumannii ATCC 19606. A. baumannii ATCC 19606 and single, double or triple ΔadeJ, ΔaceI, ΔamvA, ΔadeB mutants showed similar sigmoid growth curves and no difference in growth rates, despite ΔamvA ΔadeB ΔaceI and ΔamvA ΔadeB ΔadeJ triple mutants showed a longer lag phase than A. baumannii ATCC 19606 and other deletion mutants (Supplementary Figure 2). We analyzed also biofilm growth of A. baumannii ATCC 19606 and single, double or triple EP mutants. As shown in Figure 3, biofilm formation of single, double or triple ΔadeJ, ΔaceI, ΔamvA, ΔadeB mutants grown in the absence or in the presence of 1/2 MIC CHX was decreased by 30–50% compared with A. baumannii ATCC 19606 parental cells. On the other hand, 1/2 MIC CHX decreased biofilm growth in ATCC 19606 parental, ΔadeJ, ΔaceI, ΔamvA, ΔadeB single mutants, ΔamvA ΔaceI, ΔamvA ΔadeB, ΔadeB ΔaceI, and ΔaceI ΔadeJ double mutants and ΔamvA ΔadeB ΔadeJ triple mutants, while induced biofilm growth in ΔadeB ΔadeJ or ΔamvA ΔadeJ double mutants, and ΔamvA ΔadeB ΔaceI, ΔadeB ΔaceI ΔadeJ, or ΔamvA ΔaceI ΔadeJ triple mutants (Figure 3).
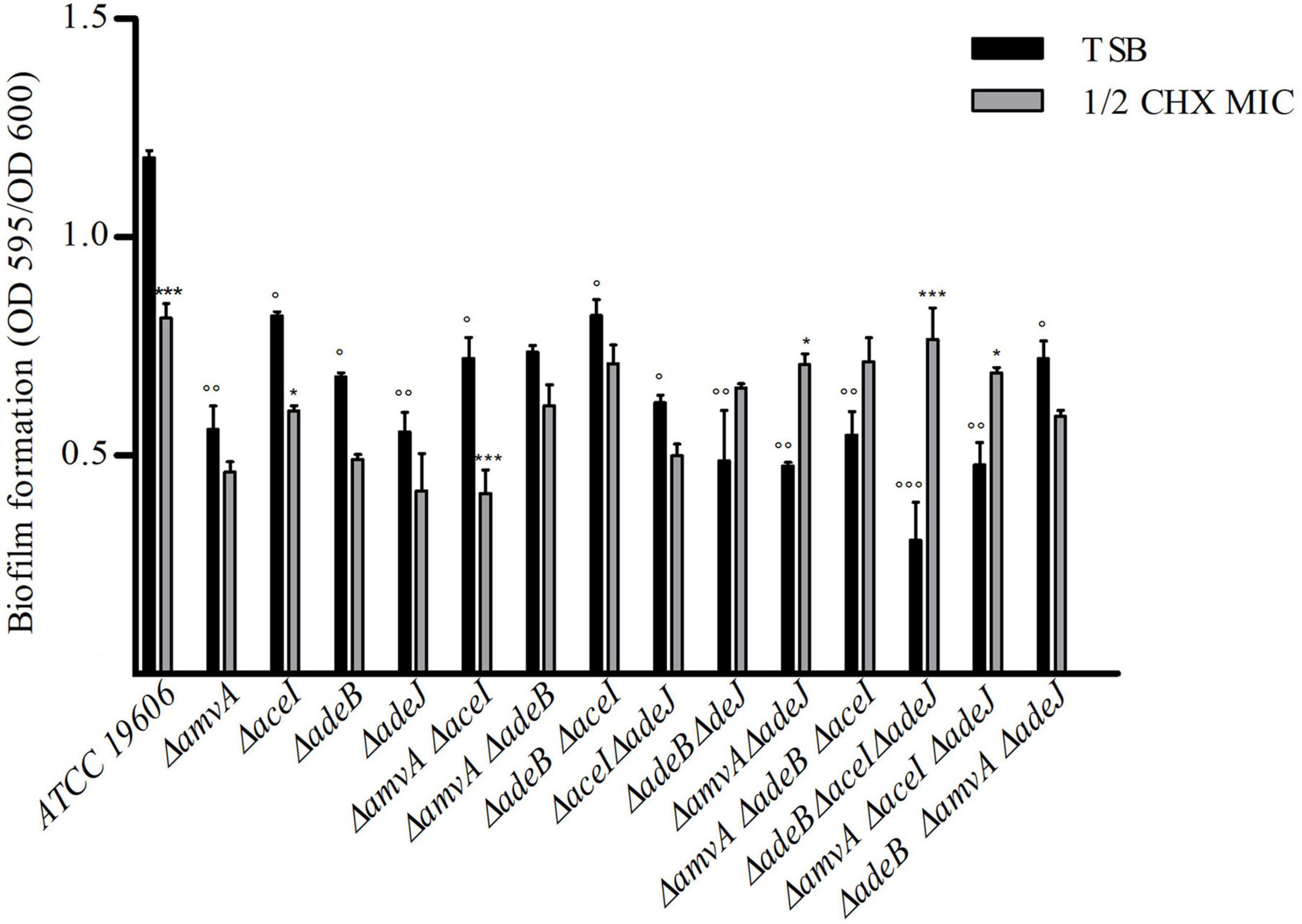
Figure 3. Biofilm formation of A. baumannii ATCC 19606 parental strain and single, double and triple deletion mutants in the absence (TSB) or the presence of ½ CHX MIC. P-values were calculated using ANOVA (°p < 0.05, °°p < 0.01, or °°°p < 0.001 vs. A. baumannii ATCC 19606 parental strain; *p < 0.05 or ***p < 0.001 vs. ½ CHX MIC).
Susceptibility to Benzalkonium Chloride, Dequalinium Chloride, Cetrimide and Triclosan in A. baumannii ATCC 19606 Wild Type and Efflux Pump Deletion Mutants
The susceptibility to other biocides, which are used as antiseptics or disinfectants (McDonnell and Russell, 1999), was analyzed in A. baumannii ATCC 19606 wild type and EP deletion mutants. In accordance with previous findings (Chen et al., 2009), A. baumannii ATCC19606 and single EP deletion mutants showed TRI MIC and MBC of 0.06 and 0.125 mg/L, respectively, and were considered susceptible to TRI (Supplementary Table 3). On the contrary, A. baumannii ATCC19606 and single EP deletion mutants were tolerant to quaternary ammonium compounds DQ and CT, showing MIC and MBC values of 32–256 and 16–64 mg/L, respectively (Supplementary Table 3).
The mechanisms responsible for tolerance to BZK was studied in detail in A. baumannii ATCC 19606 parental strain and marker-less mutants of adeB, adeJ, aceI and amvA EPs genes. As shown in Table 4, BZK MIC and MBC values were decreased by four, two, and onefold in ΔadeB, ΔamvA, and ΔaceI mutants, respectively, compared with A. baumannii ATCC19606; BZK MIC and MBC were not affected in ΔadeJ mutant. Also, BZK MIC and MBC values were decreased by eightfold in ΔamvA ΔadeB, ΔadeB ΔaceI, and ΔadeB ΔadeJ double mutants, and twofold in ΔamvA ΔaceI, ΔamvA ΔadeJ, and ΔaceI ΔadeJ double mutants. Moreover, BZK MIC and MBC were decreased by 16-fold in ΔadeB ΔaceI ΔadeJ and ΔamvA ΔadeB ΔadeJ, eightfold in ΔamvA ΔadeB ΔaceI, fourfold in ΔamvA ΔaceI ΔadeJ triple mutants (Table 4). BZK susceptibility with MIC and MBC values of 2 was recovered in ΔadeB ΔaceI ΔadeJ and ΔamvA ΔadeB ΔadeJ triple mutants (Table 4). The above data indicated that BZK MIC and MBC in A. baumannii ATCC 19606 were mainly regulated by the functioning of adeB and to a lesser extent amvA, aceI, and adeJ EPs.
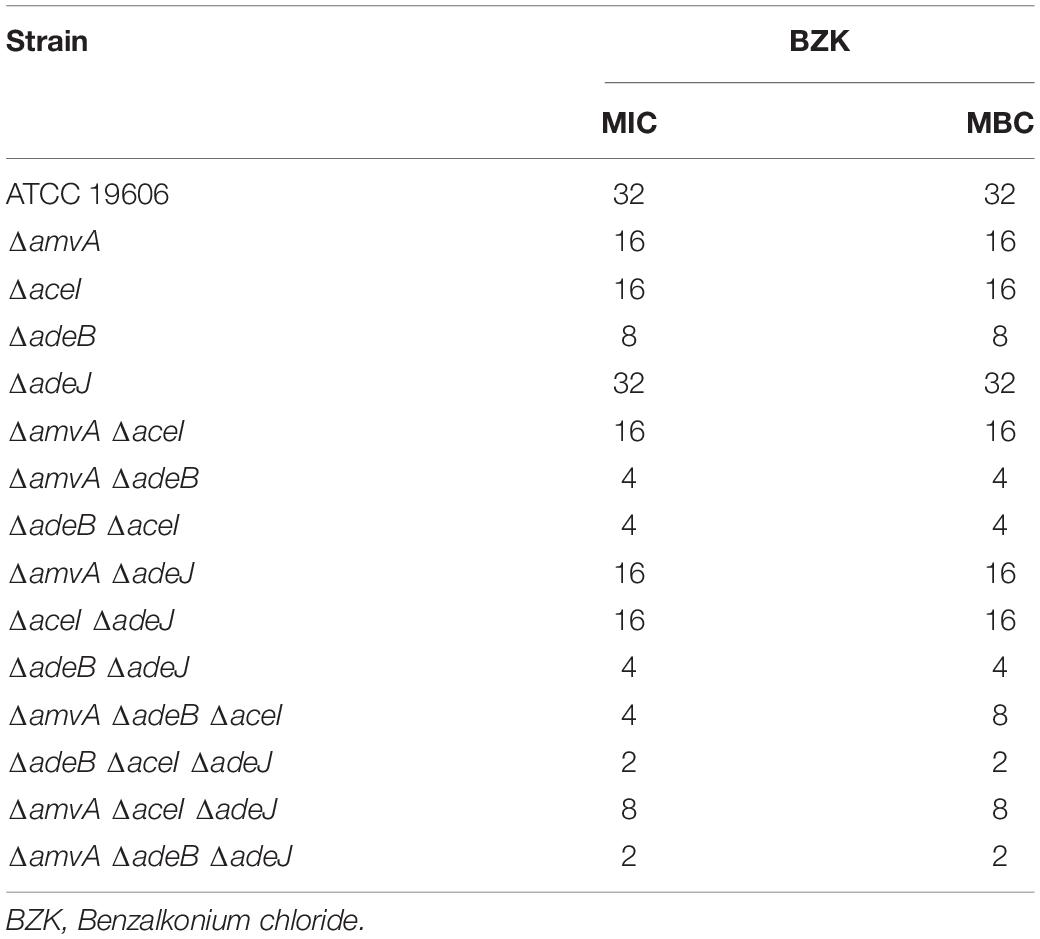
Table 4. BZK MIC (mg/L) and MBC (mg/L) of A. baumannii ATCC 19606 parental strain and EP deletion mutants.
Structural Comparison of AdeB and AdeJ Protomers
Overall, AdeB and AdeJ are two highly homologous proteins sharing a sequence identity of 49%. Both AdeB and AdeJ adopt a homotrimeric structure, with the typical RND-like fold (Su et al., 2019; Morgan et al., 2021; Zhang et al., 2021). Similar to AcrB of E. coli (seqid 50%), they are composed of a transmembrane domain formed by 12 transmembrane (TM) helices and a large periplasmic domain (Figure 4A). In this structural organization, the periplasmic domain harbors an entrance, a proximal and a distal substrate binding pockets (PBP and DBP, respectively). The PBP is separated from the DPB by a so-called “gate-loop” (or G-loop). Another conserved flexible loop (F-loop) connects the cleft entrance to the proximal drug-binding pocket. These loops are crucial to substrate discrimination in AcrB (Schuster et al., 2016). During substrate extrusion, AdeB and AcrB are thought to pass through a conformational change that forces the substrate to move from the PBP to the DBP for final extrusion (Schuster et al., 2016; Morgan et al., 2021).
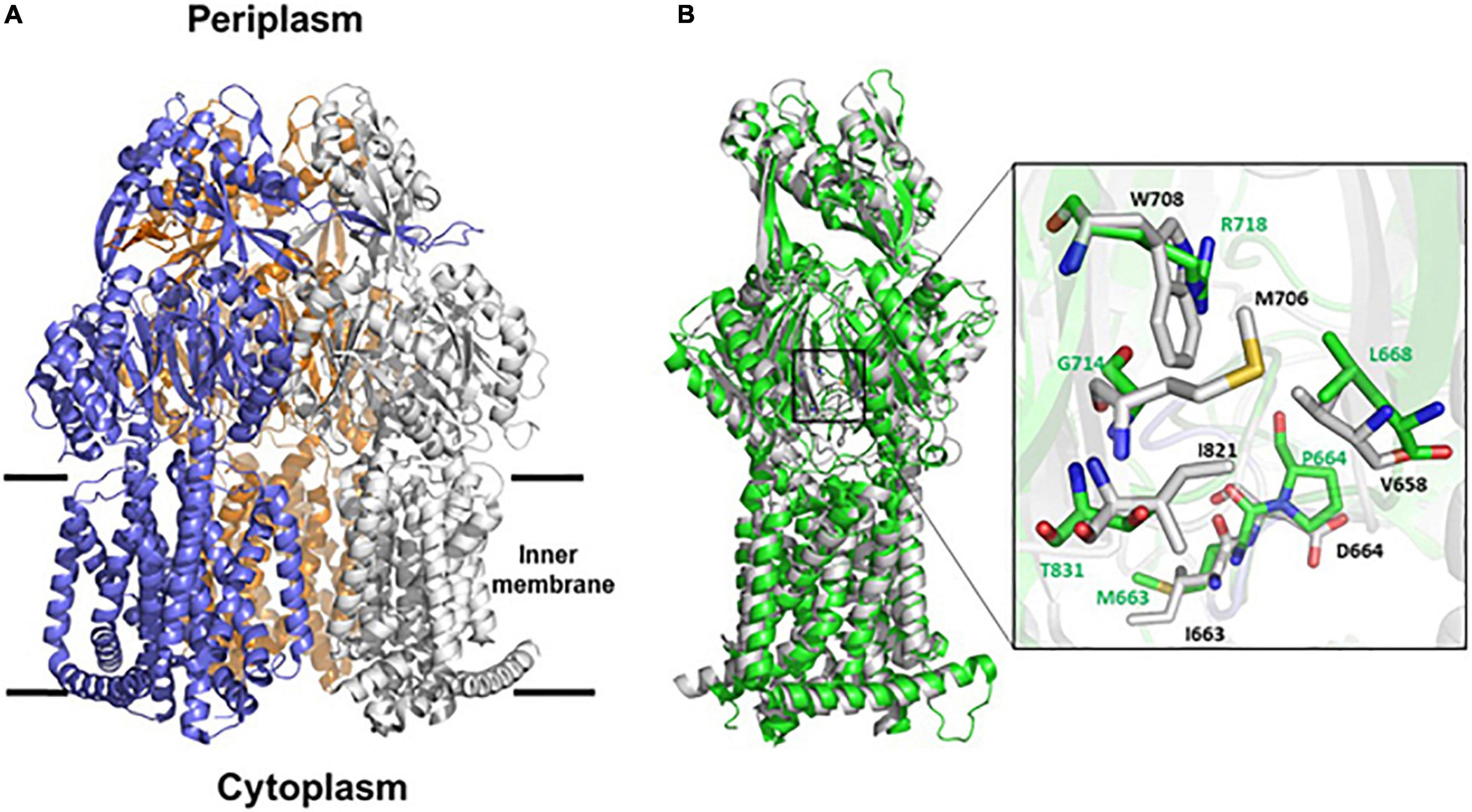
Figure 4. Structural representation of AdeB and AdeJ pumps of A. baumannii. (A) Cartoon representation of AdeB heterotrimer (pdb code 7 kgd); the three protomers are represented in blue, white and orange. (B) Superposition between the structures of AdeB and AdeJ (pdb code 7m4q) protomers. The two structures superpose with a backbone root mean square deviations (rmsd) of 2.5, 2.9, 3.0 Å on chains A, B, and C, respectively. The inset shows a zoom of the entrance sites of AdeB (white) and AdeJ (green). AdeB residues are labeled black whereas corresponding AdeJ residues are labeled green.
A structural comparison of AdeB and AdeJ protomers was performed to analyze whether differences in the structural features of the two pumps may account for the major role observed for AdeB, compared to AdeJ, on CHX extrusion and susceptibility. As shown in Figure 4B, AdeB and AdeJ share a strictly conserved fold, with root mean square deviations (rmsd) ranging between 2.5 and 3.0 Å on the three chains. The analysis of the entrance binding sites of AdeB and AdeJ suggests different features that may explain a different involvement in CHX transport. Most relevant, the conserved W708 of AdeB is replaced by an arginine residue (R718) in AdeJ (Figure 4B). Other residues belonging to this cavity also differ. Specifically, V658, M706, I861 are replaced by L668, G714, and T831, respectively. These differences in the composition of the entrance site of AdeJ, compared to AdeB, make the pocked positively charged and not prone to bind the positively charged CHX. Significant differences are also observed in the F loops of the two pumps. In AdeB, the F-loop (661-PAIDELGT-668) resembles that of AcrB (669-PAIVELGT-676) of E. coli, in which residue I671 has been shown to be important for drug discrimination (Schuster et al., 2016). Differently, the F-loop of AdeJ does not contain this key isoleucine (669-PAMPELGV-676), which is thought to be part of a preferential small-drug entrance pathway. Additionally, a more negatively charged F-loop (due to the charge contribution of D664) in AdeB may also contribute to its stronger involvement in the transport of the positively charged CHX.
Effect of Piperine and Resveratrol on Chlorhexidine and Benzalkonium Susceptibility and Expression of Efflux Pumps Genes in A. baumannii ATCC 19606 Wild Type and Deletion Mutants
We next screened two natural compounds, RV and PIP, which have shown promising activity as EPs inhibitors (Sharma et al., 2010; Mirza et al., 2011; Singkham-In et al., 2020). We tested if these non-toxic compounds can decrease CHX MIC in A. baumannii ATCC 19606 and EPs gene knockout mutants and restore susceptibility to CHX. Both PIP and RV showed no antimicrobial activity against A. baumannii ATCC 19606 and ΔadeJ, ΔaceI, ΔamvA, ΔadeB mutants (MIC > 1,024 mg/L) (Tables 5, 6). We then determined the antimicrobial activity of PIP in combination with CHX by in vitro combination assay. As shown in Table 5, increasing doses of PIP up to 128 mg/L decreased CHX MIC and MBC by four fold in A. baumannii ATCC 19606 and by two to eightfold in ΔadeJ, ΔaceI, ΔamvA, ΔadeB mutants, being able to restore CHX susceptibility in single, double and triple mutants with inactivation of adeB gene. Furthermore, RV from 32 to 128 mg/L decreased dose-dependently CHX MIC and MBC and restored CHX susceptibility in A. baumannii ATCC 19606 and ΔadeJ, ΔaceI, ΔamvA, ΔadeB single, double and triple mutants. In particular, CHX susceptibility was restored by RV at 128 mg/L in A. baumannii ATCC 19606 and ΔaceI, ΔamvA, ΔadeB, or ΔadeJ single mutants, 64 mg/L in all double or EP triple mutants, 32 mg/L in double or triple EP mutants harboring deletion of adeB (Table 6).
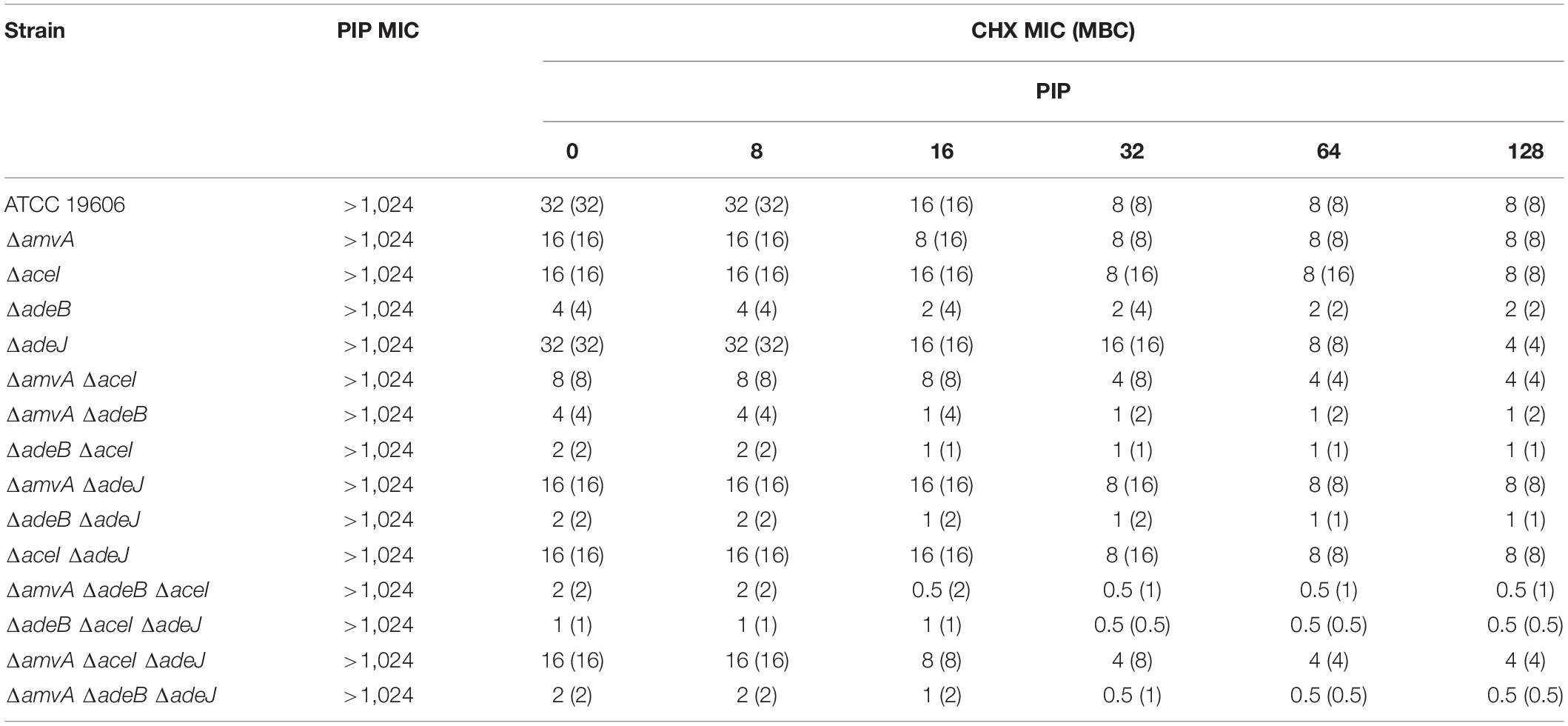
Table 5. MIC (mg/L) and MBC of CHX (mg/L) in combination with PIP in A. baumannii ATCC 19606 parental strain and EP deletion mutants.
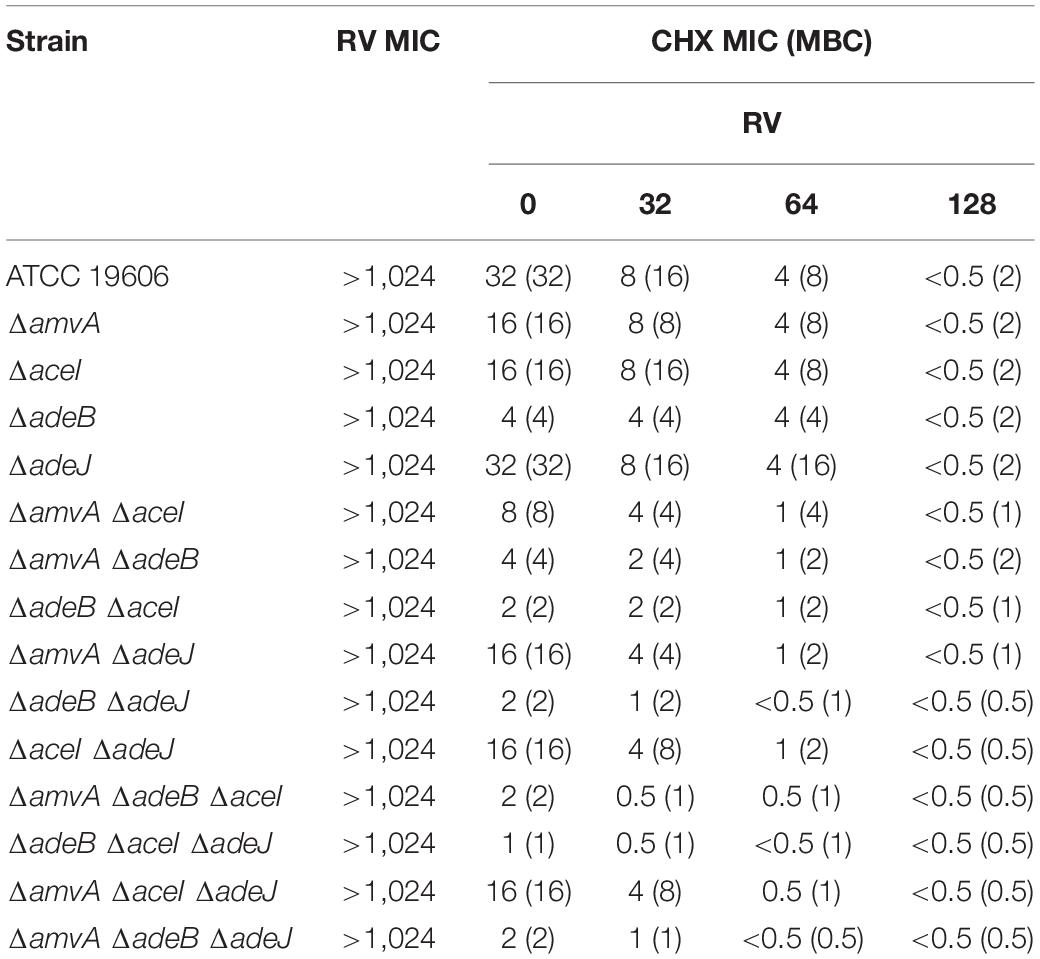
Table 6. RV effect on CHX MIC (mg/L) and CHX MBC (mg/L) in A. baumannii ATCC 19606 parental strain and EP deletion mutants.
To assess whether the effect of PIP and RV on CHX susceptibility was mediated by inhibition of EPs expression, we analyzed amvA, aceI, adeB, and adeJ expression in A. baumannii ATCC 19606 in the presence of 4 mg/L subMIC CHX in combination with 32 mg/L PIP or 32 mg/L RV. As shown in Figure 5A, PIP counteracted CHX-dependent increased expression of amvA, aceI, adeB, and adeJ, while it did not affect basal EP gene expression. On the other hand, resveratrol inhibited both basal and CHX-dependent increased expression of amvA, aceI, adeB, and adeJ, the highest effect found for adeB and amvA (Figure 5B). The above data suggested that different effects of PIP and RV on CHX MIC in A. baumannii ATCC 19606 were mediated by distinct regulation of amvA, aceI, adeB, and adeJ expression.
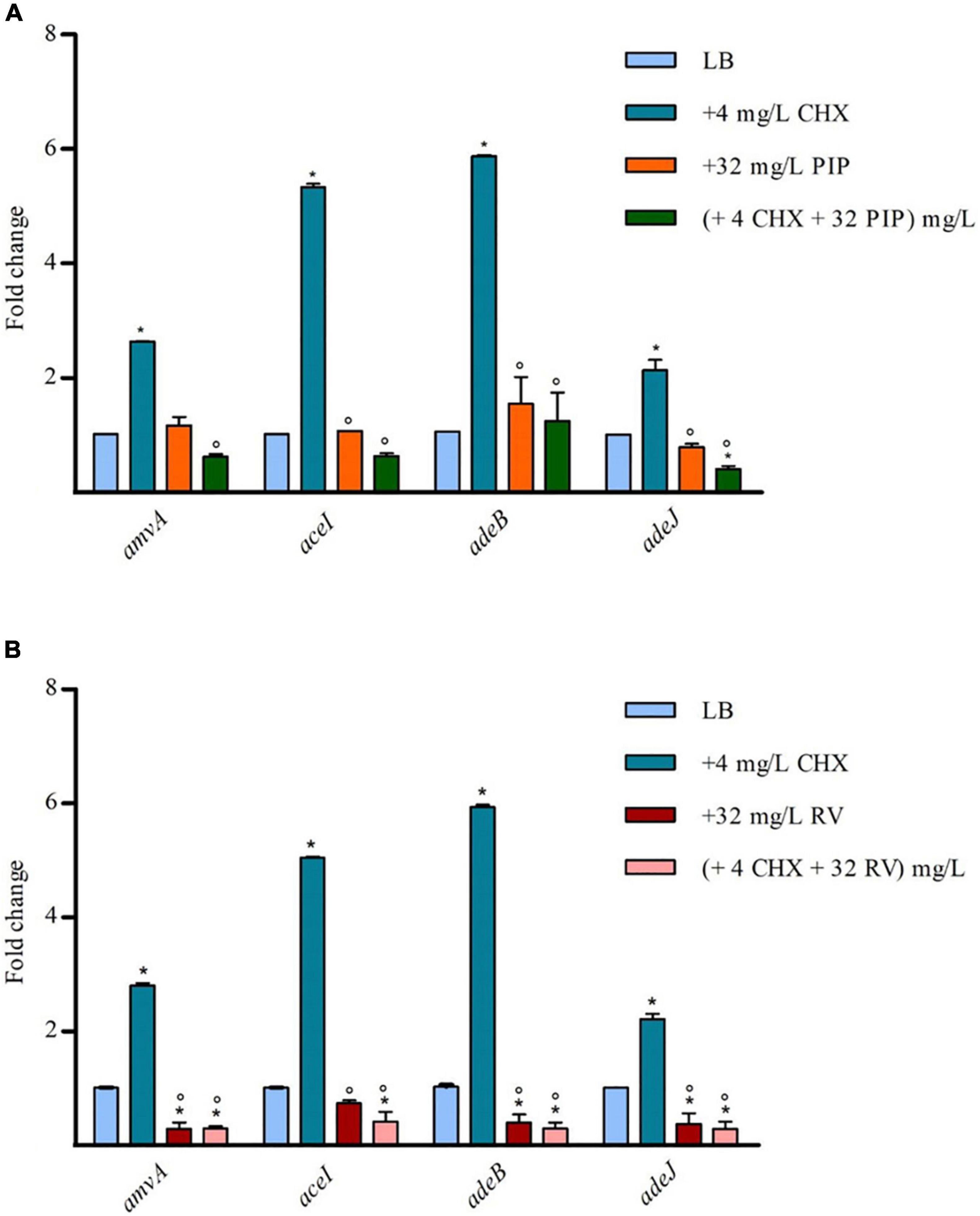
Figure 5. RT-qPCR assay of amvA, aceI, adeB, and adeJ genes expression in the absence (LB) or presence of 4 mg/L CHX alone or in combination with 32 mg/L PIP (A) or 32 mg/L RV (B). Relative number of transcripts of each gene was normalized in each condition and calculated using the 2–ΔΔct method compared to the expression level in LB control. The mean ± standard deviation of relative number of transcripts is shown for each gene. All experiments were performed in triplicate. P-values were calculated using ANOVA (*p < 0.01 vs. LB;°p < 0.01 vs. 4 mg/L CHX).
The effect of RV was also analyzed on BZK MIC and MBC in A. baumannii ATCC 19606 parental strain and EP deletion mutants. As shown in Table 7, RV from 32 mg/L to 128 mg/L decreased dose-dependently BZK MIC and MBC and restored BZK susceptibility in A. baumannii ATCC 19606 and single, double and triple EP deletion mutants. BZK susceptibility was restored by RV at 128 mg/L in A. baumannii ATCC 19606 and ΔaceI or ΔamvA, single mutants, 64 mg/L in ΔadeB, or ΔadeJ single mutants and in all double or EP triple mutants, 32 mg/L in ΔadeB single mutant and in all, but not ΔamvA ΔadeJ, double mutants (Table 7).
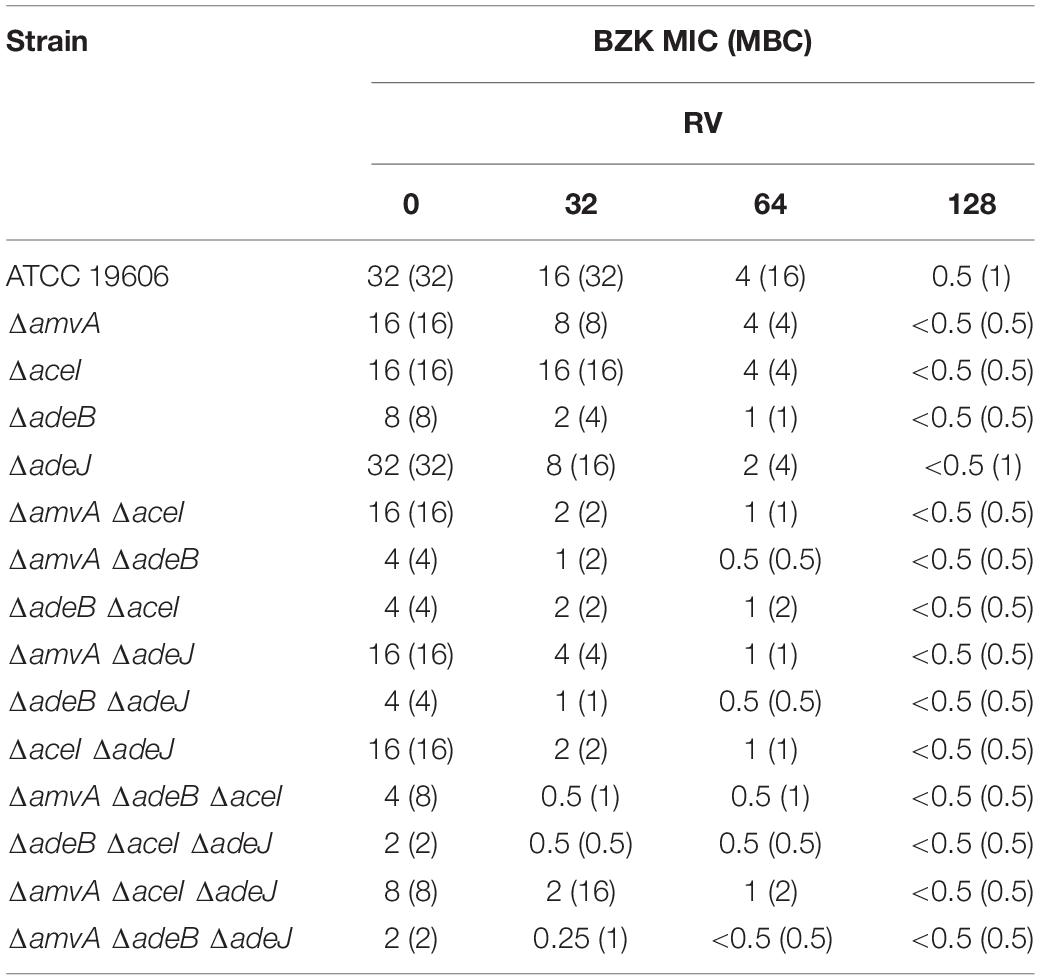
Table 7. RV effect on BZK MIC (mg/L) and MBC (mg/L) in A. baumannii ATCC 19606 parental strain and EP deletion mutants.
We analyzed also the effect of 2 mg/L BZK alone or in combination with 32 mg/L RV on amvA, aceI, adeB, and adeJ expression. As shown in Figure 6, two mg/L BZK alone inhibited in a non-significant way EP gene expression, and 2 mg/L BZK in combination with 32 mg/L RV significantly inhibited amvA, adeB, and adeJ expression by 10–15-fold and aceI expression by twofold respect to untreated cells. The above data indicated that the effect of RV on BZK susceptibility was mediated by inhibition of amvA, adeB, adeJ, and to a lesser extent aceI expression.
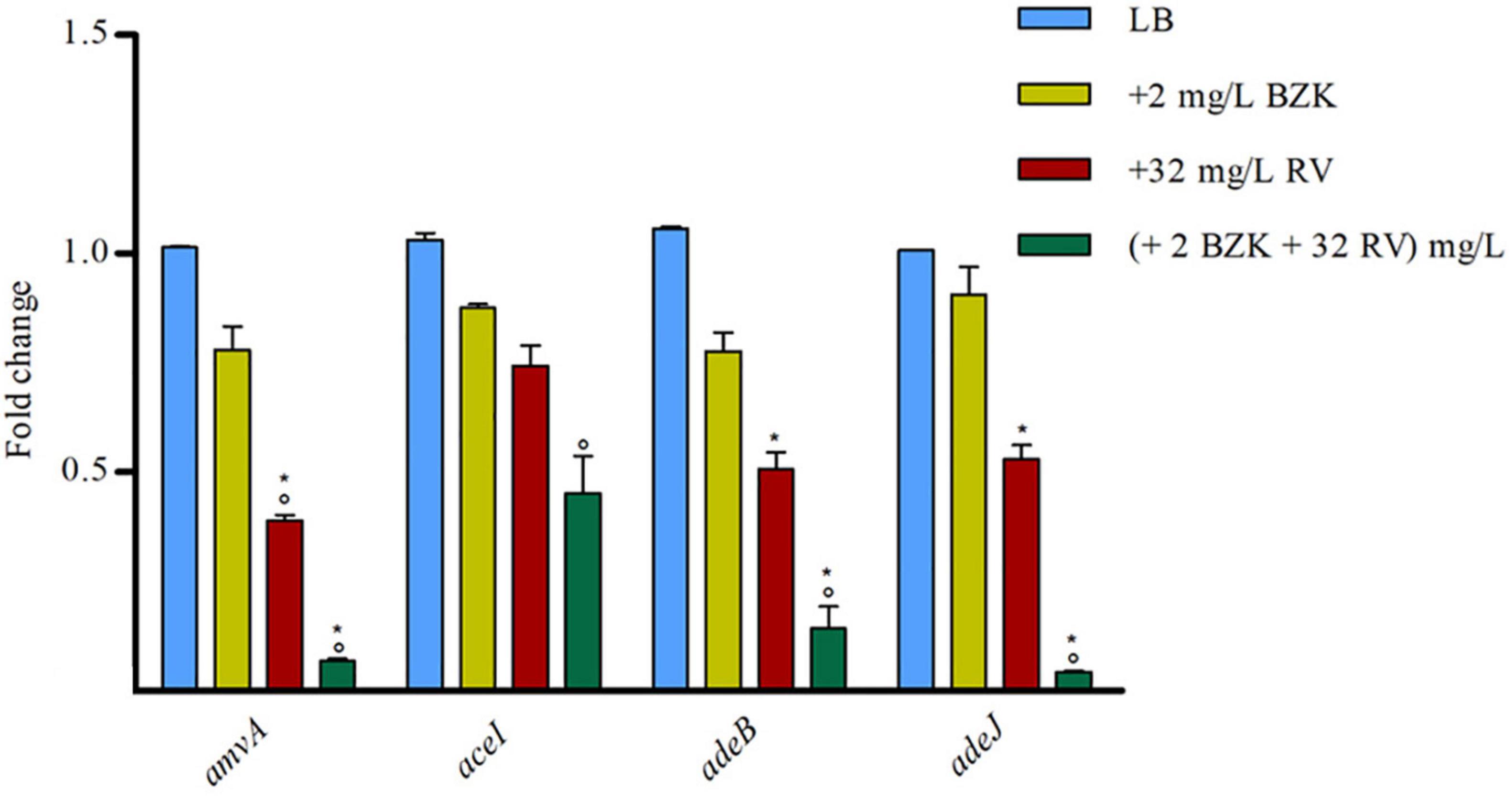
Figure 6. RT-qPCR assay of amvA, aceI, adeB, and adeJ genes expression in the absence (LB) or presence of 2 mg/L BZK alone or in combination with 32 mg/L RV. Relative number of transcripts of each gene was normalized in each condition and calculated using the 2–ΔΔct method compared to the expression level in LB control. The mean ± standard deviation of relative number of transcripts is shown for each gene. All experiments were performed in triplicate. P-values were calculated using ANOVA (*p < 0.01 vs. LB; °p < 0.01 vs. 2 mg/L BZK).
Discussion
The present study analyzes the molecular mechanism responsible for adaptation and tolerance of A. baumannii to CHX and BZK. Our data demonstrate that adeB, aceI and to lesser extent adeJ and amvA EP genes are activated by CHX in A. baumannii ATCC 19606 and that inactivation of EP genes decreases CHX MIC and MBC and restores CHX susceptibility in A. baumannii ATCC 19606. We show that subMIC concentrations of CHX enhance the expression of aceI efflux pump gene five to nine-fold, whereas that of adeB is enhanced sixfold. Despite this observation, CHX MIC and MBC decrease is significantly higher (eightfold) in ΔadeB compared to ΔamvA or ΔaceI mutant (two fold), or ΔadeJ mutant (no decrease). Single, double and triple mutants with inactivation of adeB gene showed an additive effect on CHX MIC and MBC (Table 2). Our data are in agreement with and extend previous studies showing that resistance to CHX in A. baumannii ATCC 17978 is dependent on increased expression of aceI in A. baumannii ATCC17978 (Hassan et al., 2013) and that inactivation of AceI EP (Hassan et al., 2013), AdeB or AdeJ RND EPs (Rajamohan et al., 2010a) or AmvA MFS EP (Rajamohan et al., 2010b), AceI or AdeB (Tucker et al., 2014) restores susceptibility to CHX and other disinfectants in A. baumannii. In accordance with previous findings (Tucker et al., 2014), data reported herein suggest a major involvement of AdeB in CHX transport compared to AceI. Susceptibility to CHX suggests an even lower involvement of the other pumps (AdeJ, AmvA) in CHX efflux, with no effect on MIC nor on MBC observed upon ΔadeJ mutation. In accordance with previous study (Yoon et al., 2015), we showed that inactivation of either AmvA, AceI, AdeB, or AdeJ alone or in combination did not affect planktonic growth but reduced biofilm formation by 30–50% in the absence and in the presence of 1/2 MIC CHX. However, subMIC CHX concentrations increase biofilm formation in ΔamvA ΔadeJ, ΔadeB ΔaceI ΔadeJ, and ΔamvA ΔaceI ΔadeJ mutants compared to untreated cells, thus suggesting that CHX positively regulate the phenomenon. Overall, our data indicates that EPs have pleiotropic effect and regulate multiple functions in addition to tolerance to disinfectants (Yoon et al., 2015; Du et al., 2018; Kornelsen and Kumar, 2021).
Our data demonstrate that tolerance to BZK in A. baumannii ATCC 19606 is regulated by AdeB EP and that AmvA, AceI and AdeJ EPs play a role also. BZK MIC was decreased by fourfold in ΔadeB mutant, and twofold in ΔamvA and ΔaceI mutants, respectively; EPs double and triple deletion mutants showed an additive effect on BZK MIC (Table 4). BZK susceptibility is recovered in ΔadeB ΔaceI ΔadeJ and ΔamvA ΔadeB ΔadeJ triple mutants. This is in partial agreement with previous study showing that inactivation of AmvA MFS EP decreases BZK MIC by fourfold in A. baumannii but not restores full susceptibility to biocide (Rajamohan et al., 2010b). In keeping with this, the data shown herein demonstrate that simultaneous inactivation of AdeB, AmvA, and AdeJ or AceI is necessary to restore BZK susceptibility in A. baumannii.
Importantly, AdeB and AdeJ are two highly homologous proteins sharing a sequence identity of 49%. Both adeB and adeJ genes are abundantly expressed at basal level, showing normalized expression level of 0.25 and 0.34, respectively (Supplementary Figure 1), but adeB is 3x higher expressed than adeJ in the presence of CHX (Figure 2). Also, A. baumannii ATCC 19606 does not possess the adeC gene of the adeABC operon and may use an alternate outer membrane protein (OMP), likely AdeK, of the constitutive efflux pump, AdeIJK, as described in other A. baumannii strains (Sugawara and Nikaido, 2014). However, we observe a completely different involvement of the two RND-type efflux pumps in CHX extrusion and tolerance, with AdeABC playing a central role and AdeIJK being only marginal in this mechanism (Table 2) and we postulate that differences in the structure between AdeB and AdeJ protomers may be responsible for this. The structural comparison of AdeB and AdeJ shows different features at the entrance binding site, such as W708, V658, M706, I861 in AdeB, which are replaced by R718, L668, G714, and T831 in AdeJ, respectively. Overall, a more positive electrostatic potential surface at the entrance site of AdeJ, due to R817, may render this pump not prone to bind the positively charged CHX. Additionally, the F-loop of AdeB presents different features than that of AdeJ, as it is more negatively charged (due to the charge contribution of D664) and contains a key isoleucine residue, I671, which was shown to be important in AcrB (Schuster et al., 2016). These features may contribute to its stronger involvement in the transport of the positively charged CHX by AdeB (Figure 4B). Future experimental data will be necessary to validate the impact of specific residues in AdeB protomer on CHX efflux in A. baumannii.
In this work, we also searched for EP inhibitors that restore CHX susceptibility, to tackle A. baumannii tolerance to CHX and BZK induced by EP pumps. As a first compound, CCCP showed a significant effect on CHX MIC (Table 3). However, due to the toxicity of this compound, we analyzed the effects on CHX susceptibility of two antioxidant molecules, the non-toxic PIP and RV. As a result, both PIP and RV were able to decrease CHX MIC and MBC in A. baumannii ATCC 19606 and EP deletion mutants. In particular, PIP was able to restore CHX susceptibility only in single, double and triple mutants with inactivation of adeB gene. In partial agreement with our data, PIP inhibited rifampicin-induced expression of Rv1258c multidrug efflux pump and rifampicin MIC in Mycobacterium tuberculosis (Sharma et al., 2010). Similarly, PIP has been demonstrated to inhibit ethidium bromide efflux and mupirocin resistance in methicillin-resistant S. aureus (Mirza et al., 2011). Our data also demonstrated that RV has higher efficacy than PIP on CHX susceptibility, being resveratrol able to restore CHX susceptibility dose-dependently both in A. baumannii ATCC 19606 and in EP deletion mutants. Coherent with this finding, we show that PIP inhibits CHX-induced, though not basal, expression of EP genes. In addition, consistent with previous data (Singkham-In et al., 2020) we find that RV is able to inhibit both basal levels and CHX-induced expression of amvA, aceI, adeB, and adeJ genes in A. baumannii ATCC 19606. The differential effects of PIP and RV on CHX MIC is likely to be ascribed to their different ability to inhibit EPs gene expression.
Our data also demonstrated that RV restored BZK susceptibility both in A. baumannii ATCC 19606 and in EP deletion mutants. Although unlike CHX, BZK does not induce the expression of EPs genes, RV alone or in the presence of BZK inhibited amvA, aceI, adeB and adeJ expression, the effect of RV and BZK being synergic for amvA, adeB, adeJ. Based on this, we hypothesize that the effect of RV on BZK susceptibility in A. baumannii is mediated by the inhibition of expression of EPs.
Conclusion
The data reported in this study demonstrate that tolerance to CHX and BZK in A. baumannii is mediated by the activation of EPs. In particular, adeB, adeJ, aceI, and amvA expression is induced by CHX; EPs gene inactivation inhibits both CHX and BZK MIC in an additive manner, with AdeB EP playing a major role. We also identified PIP and RV as non-toxic compounds able to inhibit EPs gene expression and CHX or BZK tolerance in A. baumannii. Our data demonstrate that co-treatments of RV and CHX or RV and BZK restore susceptibility to biocides in A. baumannii.
A. baumannii ATCC19606 and EP inactivation mutants described herein may represent a useful model system to study the molecular mechanisms responsible for tolerance to biocides other than CHX and BZK in A. baumannii and to identify innovative molecules and combination regimens, which are able to restore susceptibility to disinfectants in A. baumannii. The combination of RV may represent a useful strategy to maintain susceptibility to biocides in A. baumannii and other nosocomial pathogens.
Data Availability Statement
The original contributions presented in the study are included in the article/Supplementary Material, further inquiries can be directed to the corresponding author/s.
Author Contributions
ED and RZ conceived the study and participated in its design and coordination. AM, EE, and MB performed laboratory experiments. RB, MT, ED, and RZ performed data analyses. AM, EE, RB, ED, and RZ wrote the manuscript. All authors read and approved the final manuscript.
Funding
This work was supported in part by grant from the Italian Ministry of Education, University and Research (MIUR): PRIN2017 (Grant No. 2017SFBFER to RZ and RB).
Conflict of Interest
The authors declare that the research was conducted in the absence of any commercial or financial relationships that could be construed as a potential conflict of interest.
Publisher’s Note
All claims expressed in this article are solely those of the authors and do not necessarily represent those of their affiliated organizations, or those of the publisher, the editors and the reviewers. Any product that may be evaluated in this article, or claim that may be made by its manufacturer, is not guaranteed or endorsed by the publisher.
Acknowledgments
We thank all colleagues who generously provided strains included in the study: Alessandra Carattoli, Patrice Nordmann, and Paolo Visca.
Supplementary Material
The Supplementary Material for this article can be found online at: https://www.frontiersin.org/articles/10.3389/fmicb.2021.790263/full#supplementary-material
References
Amin, I. M., Richmond, G. E., Sen, P., Koh, T. H., Piddock, L. J. V., and Chua, K. L. (2013). A method for generating marker-less gene deletions in multidrug-resistant Acinetobacter baumannii. BMC Microbiol. 13:158. doi: 10.1186/1471-2180-13-158
Chen, Y., Pi, B., Zhou, H., Yu, Y., and Li, L. (2009). Triclosan resistance in clinical isolates of Acinetobacter baumannii. J. Med. Microbiol. 58, 1086–1091. doi: 10.1099/jmm.0.008524-0
CLSI (2019). Performance Standards for Antimicrobial Susceptibility Testing M100S. Wayne, PA: Clinical and Laboratory Standards Institute.
De Gregorio, E., Del Franco, M., Martinucci, M., Roscetto, E., Zarrilli, R., and Di Nocera, P. (2015). Biofilm-associated proteins: news from Acinetobacter. BMC Genomics. 16:933. doi: 10.1186/s12864-015-2136-6
De Gregorio, E., Esposito, A., Vollaro, A., De Fenza, M., D’Alonzo, D., Migliaccio, A., et al. (2020). N-Nonyloxypentyl-l-Deoxynojirimycin inhibits growth, biofilm formation and virulence factors expression of Staphylococcus aureus. Antibiotic (Basel) 9, 362. doi: 10.3390/antibiotics9060362
De Gregorio, E., Esposito, E. P., Zarrilli, R., and Di Nocera, P. P. (2018). Contact-dependent growth inhibition proteins in Acinetobacter baylyi ADP1. Curr. Microbiol. 75, 1434–1440. doi: 10.1007/s00284-018-1540-y
Du, D., Wang-Kan, X., Neuberger, A., van Veen, H. W., Pos, K. M., Piddock, L. J. V., et al. (2018). Multidrug efflux pumps: structure, function and regulation. Nat. Rev. Microbiol. 16, 523–539. doi: 10.1038/s41579-018-0048-6
Emsley, P., and Cowtan, K. (2004). Coot: model-building tools for molecular graphics. Acta Crystallogr. D Biol. Crystallogr. 60(Pt 12 Pt 1), 2126–2132. doi: 10.1107/S0907444904019158
European Committee for Antimicrobial Susceptibility Testing (Eucast) of the European Society of Clinical Microbiology and Infectious Diseases (Escmid) (2000). Terminology relating to methods for the determination of susceptibility of bacteria to antimicrobial agents. Clin. Microbiol. Infect. 6, 503–508. doi: 10.1046/j.1469-0691.2000.00149.x
Foong, W. E., Tam, H. K., Crames, J. J., Averhoff, B., and Pos, K. M. (2019). The chloramphenicol/H+ antiporter CraA of Acinetobacter baumannii AYE reveals a broad substrate specificity. J. Antimicrob. Chemother. 74, 1192–1201. doi: 10.1093/jac/dkz024
Gaiarsa, S., Batisti Biffignandi, G., Esposito, E. P., Castelli, M., Jolley, K. A., Brisse, S., et al. (2019). Comparative analysis of the two Acinetobacter baumannii multilocus sequence typing (MLST) schemes. Front Microbiol. 10:930. doi: 10.3389/fmicb.2019.00930
Giannouli, M., Antunes, L. C. S., Marchetti, V., Triassi, M., Visca, P., and Zarrilli, R. (2013). Virulence-related traits of epidemic Acinetobacter baumannii strains belonging to the international clonal lineages I-III and to the emerging genotypes ST25 and ST78. BMC Infect. Dis. 13:282. doi: 10.1186/1471-2334-13-282
Hall, M., Middleton, R., and Westmacott, D. (1983). The fractional inhibitory concentration (FIC) index as a measure of synergy. J. Antimicrob. Chemother. 11, 427–433. doi: 10.1093/jac/11.5.427
Haq, I. U., Imran, M., Nadeem, M., Tufail, T., Gondal, T. A., and Mubarak, M. S. (2021). Piperine: a review of its biological effects. Phytother. Res. 35, 680–700. doi: 10.1002/ptr.6855
Harding, C. M., Hennon, S. W., and Feldman, M. F. (2018). Uncovering the mechanisms of Acinetobacter baumannii virulence. Nat. Rev. Microbiol. 16, 91–102. doi: 10.1038/nrmicro.2017.148
Hassan, K. A., Jackson, S. M., Penesyan, A., Patching, S. G., Tetu, S. G., Eijkelkamp, B. A., et al. (2013). Transcriptomic and biochemical analyses identify a family of chlorhexidine efflux proteins. Proc. Natl. Acad. Sci. U.S.A. 110, 20254–20259. doi: 10.1073/pnas.1317052110
Holm, L. (2020). Using dali for protein structure comparison. Methods Mol. Biol. 2112, 29–42. doi: 10.1007/978-1-0716-0270-6_3
Iacono, M., Villa, L., Fortini, D., Bordoni, R., Imperi, F., Bonnal, R. J., et al. (2008). Whole-genome pyrosequencing of an epidemic multidrug-resistant Acinetobacter baumannii strain belonging to the European clone II group. Antimicrob. Agents Chemother. 52, 2616–2625. doi: 10.1128/AAC.01643-07
Janssen, P., Maquelin, K., Coopman, R., Tjernberg, I., Bouvet, P., Kersters, K., et al. (1997). Discrimination of Acinetobacter genomic species by AFLP fingerprinting. Int. J. Syst. Bacteriol. 47, 1179–1187. doi: 10.1099/00207713-47-4-1179
Kampf, G. (2016). Acquired resistance to chlorhexidine - is it time to establish an ‘antiseptic stewardship’ initiative? J. Hosp. Infect. 94, 213–227. doi: 10.1016/j.jhin.2016.08.018
Kornelsen, V., and Kumar, A. (2021). Update on multidrug resistance efflux pumps in Acinetobacter spp. Antimicrob. Agents Chemother. 65, e514–e521. doi: 10.1128/AAC.00514-21 e00514–21,
Livak, K. J., and Schmittgen, T. D. (2001). Analysis of relative gene expression data using real-time quantitative PCR and the 2(-Delta Delta C(T)) method. Methods 25, 402–408. doi: 10.1006/meth.2001.1262
Magiorakos, A. P., Srinivasan, A., Carey, R. B., Carmeli, Y., Falagas, M. E., Giske, C. G., et al. (2012). Multidrug-resistant, extensively drug-resistant and pandrug-resistant bacteria: an international expert proposal for interim standard definitions for acquired resistance. Clin. Microbiol. Infect. 18, 268–281. doi: 10.1111/j.1469-0691.2011.03570.x
Martinucci, M., Roscetto, E., Iula, V. D., Votsi, A., Catania, M. R., and De Gregorio, E. (2016). Accurate identification of members of the Burkholderia cepacia complex in cystic fibrosis sputum. Lett. Appl. Microbiol. 62, 221–229. doi: 10.1111/lam.12537
Mattio, L. M., Catinella, G., Dallavalle, S., and Pinto, A. (2020). Stilbenoids: a natural arsenal against bacterial pathogens. Antibiotics (Basel) 9:336. doi: 10.3390/antibiotics9060336
McDonnell, G., and Russell, A. D. (1999). Antiseptics and disinfectants: activity, action, and resistance. Clin. Microbiol. Rev. 12, 147–179. doi: 10.1128/CMR.12.1.147
Merchel Piovesan Pereira, B., and Tagkopoulos, I. (2019). Benzalkonium chlorides: uses, regulatory status, and microbial resistance. Appl. Environ. Microbiol. 85:e00377-19. doi: 10.1128/AEM.00377-19
Milstone, A. M., Passaretti, C. L., and Perl, T. M. (2008). Chlorhexidine: expanding the armamentarium for infection control and prevention. Clin. Infect. Dis. 46, 274–281. doi: 10.1086/524736
Mirza, Z. M., Kumar, A., Kalia, N. P., Zargar, A., and Khan, I. A. (2011). Piperine as an inhibitor of the MdeA efflux pump of Staphylococcus aureus. J. Med. Microbiol. 60(Pt 10), 1472–1478. doi: 10.1099/jmm.0.033167-0
Morgan, C. E., Glaza, P., Leus, I. V., Trinh, A., Su, C. C., Cui, M., et al. (2021). Cryoelectron microscopy structures of AdeB illuminate mechanisms of simultaneous binding and exporting of substrates. mBio 12:e03690–20. doi: 10.1128/mBio.03690-20
Poirel, L., Manuteau, O., Agoli, N., Cattoen, C., and Nordmann, P. (2003). Outbreak of extended-spectrum β-lactamase VEB-1-producing isolates of Acinetobacter baumannii in a French hospital. J. Clin. Microbiol. 41, 3542–3547. doi: 10.1128/JCM.41.8
Rajamohan, G., Srinivasan, V. B., and Gebreyes, W. A. (2010a). Novel role of Acinetobacter baumannii RND efflux transporters in mediating decreased susceptibility to biocides. J. Antimicrob. Chemother. 65, 228–232. doi: 10.1093/jac/dkp427
Rajamohan, G., Srinivasan, V. B., and Gebreyes, W. A. (2010b). Molecular and functional characterization of a novel efflux pump, AmvA, mediating antimicrobial and disinfectant resistance in Acinetobacter baumannii. J. Antimicrob. Chemother. 65, 1919–1925. doi: 10.1093/jac/dkq195
Schuster, S., Vavra, M., and Kern, W. V. (2016). Evidence of a substrate discriminating entrance channel in the lower porter domain of the multidrug resistance efflux pump AcrB. Antimicrob. Agents Chemother. 60, 4315–4323. doi: 10.1128/AAC.00314-16
Seeliger, D., and de Groot, B. L. (2010). Ligand docking and binding site analysis with PyMOL and Autodock/Vina. J. Comput. Aided Mol. Des. 24, 417–422. doi: 10.1007/s10822-010-9352-6
Sharma, S., Kumar, M., Sharma, S., Nargotra, A., Koul, S., and Khan, I. A. (2010). Piperine as an inhibitor of Rv1258c, a putative multidrug efflux pump of Mycobacterium tuberculosis. J. Antimicrob. Chemother. 65, 1694–1701. doi: 10.1093/jac/dkq186
Simon, R., Priefer, U., and Piihler, A. (1983). A broad host range mobilization system for in vivo genetic engineering: transposon mutagenesis in gram-negative bacteria. Biotechnology 1, 784–791.
Singkham-In, U., Higgins, P. G., Wannigama, D. L., Hongsing, P., and Chatsuwan, T. (2020). Rescued chlorhexidine activity by resveratrol against carbapenem-resistant Acinetobacter baumannii via down-regulation of AdeB efflux pump. PLoS One 15:e0243082. doi: 10.1371/journal.pone.0243082
Su, C. C., Morgan, C. E., Kambakam, S., Rajavel, M., Scott, H., Huang, et al. (2019). Cryo-electron microscopy structure of an Acinetobacter baumannii multidrug efflux pump. mBio 10:e01295-19. doi: 10.1128/mBio.01295-19
Sugawara, E., and Nikaido, H. (2014). Properties of AdeABC and AdeIJK efflux systems of Acinetobacter baumannii compared with those of the AcrAB-TolC system of Escherichia coli. Antimicrob. Agents Chemother. 58, 7250–7257. doi: 10.1128/AAC.03728-14
Tucker, A. T., Nowicki, E. M., Boll, J. M., Knauf, G. A., Burdis, N. C., Trent, M. S., et al. (2014). Defining gene-phenotype relationships in Acinetobacter baumannii through one-step chromosomal gene inactivation. mBio 5:e01313-14. doi: 10.1128/mBio.01313-14
Weber, D. J., Rutala, W. A., and Sickbert-Bennett, E. E. (2019). Use of germicides in health care settings-is there a relationship between germicide use and antimicrobial resistance: a concise review. Am. J. Infect. Contr. 47, A106–A109. doi: 10.1016/j.ajic.2019.03.023
Wong, D., Nielsen, T. B., Bonomo, R. A., Pantapalangkoor, P., Luna, B., and Spellberg, B. (2017). Clinical and pathophysiological overview of Acinetobacter infections: a century of challenges. Clin. Microbiol. Rev. 30, 409–447. doi: 10.1128/CMR.00058-16
Yoon, E. J., Chabane, Y. N., Goussard, S., Snesrud, E., Courvalin, P., Dé, E., et al. (2015). Contribution of resistance-nodulation-cell division efflux systems to antibiotic resistance and biofilm formation in Acinetobacter baumannii. mBio 6:e00309-15. doi: 10.1128/mBio.00309-15
Keywords: Acinetobacter baumannii, chlorhexidine susceptibility, efflux pumps, AdeB, biofilm growth, resveratrol, piperine, benzalkonium
Citation: Migliaccio A, Esposito EP, Bagattini M, Berisio R, Triassi M, De Gregorio E and Zarrilli R (2022) Inhibition of AdeB, AceI, and AmvA Efflux Pumps Restores Chlorhexidine and Benzalkonium Susceptibility in Acinetobacter baumannii ATCC 19606. Front. Microbiol. 12:790263. doi: 10.3389/fmicb.2021.790263
Received: 06 October 2021; Accepted: 23 December 2021;
Published: 07 February 2022.
Edited by:
Henrietta Venter, University of South Australia, AustraliaReviewed by:
Karl Hassan, The University of Newcastle, AustraliaWilliam T. Doerrler, Louisiana State University, United States
Copyright © 2022 Migliaccio, Esposito, Bagattini, Berisio, Triassi, De Gregorio and Zarrilli. This is an open-access article distributed under the terms of the Creative Commons Attribution License (CC BY). The use, distribution or reproduction in other forums is permitted, provided the original author(s) and the copyright owner(s) are credited and that the original publication in this journal is cited, in accordance with accepted academic practice. No use, distribution or reproduction is permitted which does not comply with these terms.
*Correspondence: Eliana De Gregorio, ZWRlZ3JlZ29AdW5pbmEuaXQ=; Raffaele Zarrilli, cmFmemFycmlAdW5pbmEuaXQ=
†These authors have contributed equally to this work and share first authorship