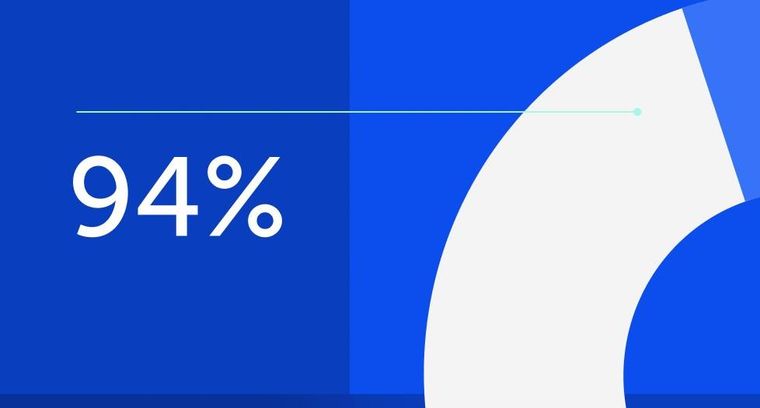
94% of researchers rate our articles as excellent or good
Learn more about the work of our research integrity team to safeguard the quality of each article we publish.
Find out more
ORIGINAL RESEARCH article
Front. Microbiol., 04 January 2022
Sec. Infectious Agents and Disease
Volume 12 - 2021 | https://doi.org/10.3389/fmicb.2021.779538
Cronobacter sakazakii is an opportunistic pathogen causing a lethality rate as high as 80% in infants. Desiccation tolerance ensures its survival in powdered infant formula (PIF) and contributes to the increased exposure to neonates, resulting in neonatal meningitis, septicemia, and necrotizing enterocolitis. This study showed that a food-isolated C. sakazakii G4023 strain exhibited a stronger desiccation tolerance than C. sakazakii ATCC 29544 strain. Considering the proven pathogenicity of G4023, it could be a big threat to infants. Transcriptome and proteome were performed to provide new insights into the desiccation adaptation mechanisms of G4023. Integrated analyses of these omics suggested that 331 genes were found regulated at both transcriptional and protein levels (≥2.0- and ≥1.5-fold, respectively). Deletion of chemotaxis system encoded genes cheA and cheW resulted in decreased tolerance in both short- and long-term desiccation. Reduced O-antigen chain length contributed to the biofilm formation and desiccation tolerance in the short term rather than the long term. In addition, biosynthesis of flagella, arginine and its transport system, and Fe/S cluster were also observed regulated in desiccated G4023. A better understanding of desiccation adaptation mechanisms of G4023 could in turn guide the operations during production and preservation of PIF or other food to reduce survival odds of G4023 and lower its exposure to get to infants.
Cronobacter sakazakii is a Gram-negative facultatively anaerobic pathogenic bacterium belonging to the family Enterobacteriaceae. Although the bacterium had been classified as Enterobacter sakazakii in 1980 (Farmer et al., 1980), it was later reclassified as a new genus, Cronobacter, containing seven species, namely, C. sakazakii, Cronobacter malonaticus, Cronobacter muytjensii, Cronobacter turicensis, Cronobacter dublinensis, Cronobacter universalis, and Cronobacter condimenti (Iversen et al., 2007, 2008; Joseph et al., 2012). Although the environmental reservoir of C. sakazakii is not evidently understood yet, reconstituted powdered infant formula (PIF) is reported to be the most associated vehicle for transmission (Chauhan et al., 2020; Parra-Flores et al., 2020). The desiccation tolerance of C. sakazakii ensures its survival in PIF and contributes to the increased exposure of neonates to the organism (Bennour Hennekinne et al., 2018). C. sakazakii-contaminated PIF has been epidemiologically linked to infections and illnesses and can cause neonatal meningitis, septicemia, and necrotizing enterocolitis in infants with a lethality rate as high as 80% (Yan et al., 2012; Almajed and Forsythe, 2016; Parra-Flores et al., 2018; Srikumar et al., 2019).
Bacteria can develop distinct strategies to tolerate desiccation stress. Salmonella can survive desiccation via the filamentation properties involving outer membrane antigen of lipopolysaccharides (LPSs), fimbriae, and cellulose (Gibson et al., 2006; White et al., 2006; Garmiri et al., 2008; Vanderlinde et al., 2010). Listeria monocytogenes was found to accumulate betaine, carnitine, and proline as osmolytes to cope with desiccation (Bayles and Wilkinson, 2000). The resistance of C. sakazakii to desiccation has been of research interest recently. A positive correlation between biofilm formation ability and desiccation tolerance was suggested, while the sequence type showed no significant correlation (Du et al., 2018). In addition, the physiological importance of trehalose in the desiccation survival of C. sakazakii cells has been confirmed (Srikumar et al., 2019). Although several factors have been reported influencing the desiccation tolerance of C. sakazakii, the pathways by which they regulate still lack sufficient experimental evidences.
Recently, the integration of transcriptome and proteome analyses has been an effective strategy to promote a better understanding of mechanisms in response to environmental stress (Lai et al., 2020). mRNA expression profile alone was insufficient for understanding the protein expression levels, since plenty of factors, such as RNA structure, regulatory proteins and sRNAs, codon bias, protein stability, protein degradation, and protein secretion could influence the level of protein expression (Maier et al., 2009; Haider and Pal, 2013; Wang X. et al., 2019; Buccitelli and Selbach, 2020). The analysis of mRNA and protein expression data usually failed to show a high correlation even under similar conditions (Chen et al., 2002; MacKay et al., 2004; Ghazalpour et al., 2011; Haider and Pal, 2013). Although RNA-seq and iTRAQ-based quantitative proteomics had been applied to reveal the potential desiccation resistance factors of C. sakazakii in recent years (Hu et al., 2017; Srikumar et al., 2019), the strains used and desiccation conditions were totally different between the two independent experiments. In order to gain a higher correlation between transcriptomic and proteomic data, and for a better understanding of desiccation tolerance mechanisms of C. sakazakii, we performed transcriptome and proteome analyses of C. sakazakii wild-type (WT) strain G4023 under the same desiccation conditions. G4023 is a food-isolated C. sakazakii strain that had been previously sequenced by us and experimentally proven to be associated with meningitis in neonates and infants (Wang M. et al., 2019). Considering label-free quantitation experiments show more accuracy and higher efficiency in quantitation of spiked-in standards than iTRAQ-labeled ones (Wang H. et al., 2012; Trinh et al., 2013; Sandberg et al., 2014), we chose the label-free quantitative proteome analysis in this study over the iTRAQ-labeled one.
The WT C. sakazakii G4023, a food-isolated strain, has been proved to belong to sequence type 4 (ST4), and the pathogenicity has also been confirmed in our previous work (Wang M. et al., 2019). In this study, we compared the whole-genome sequence (WGS) of C. sakazakii G4023 with that of ATCC 29544. ATCC 29544 was clinically isolated and identified as a type strain of C. sakazakii (Farmer et al., 1980), and detailed information of both strains are summarized in Supplementary Table 1. The WGS comparisons showed there were 3,648 homologous genes, which account for 90.9 and 83.3% of total genes in G4023 and ATCC 29544, respectively (Figure 1A). Further analyses suggested the specific genes in G4023 were found mainly localized in genomic islands (Figure 1B), which were normally horizontally acquired to provided accessory genes for niche adaptation and pathogenicity (Schmidt and Hensel, 2004). In addition, whole-genome average nucleotide identity (ANI), average amino acid identity (AAI), and tetranucleotide usage deviation (TUD) between C. sakazakii G4023 and ATCC 29544 also demonstrated they belong to the same bacterial species (97.86%, 98.77%, and 0.999, respectively).
Figure 1. Genomic and biochemical comparisons between C. sakazakii G4023 and ATCC 29544. (A) Venn diagram shows the numbers of homologous and specific gene in genomes of C. sakazakii G4023 and ATCC 29544. (B) Genomic comparison of C. sakazakii G4023 and ATCC 29544 suggests the specific genes in G4023 mainly localized in genomic islands. (C) Bacteria colonies on DFI agar (left) and LB agar (right). ATCC 29544 forms green/blue colonies on DFI agar and produces yellow pigment on LB agar (a), which are identical to that of G4023 (b); Salmonella Typhimurium ATCC 14028 (c) and EHEC O157: H7 EDL933 (d) present white colonies on both agar mediums.
In addition to the genomic comparisons, biochemical identifications of C. sakazakii G4023 were also performed, with ATCC 29544 strain as a control. Both the typical features of C. sakazakii, green/blue colonies on selective Druggan Forsythe Iversen (DFI) agar and yellow pigment production on Luria–Bertani (LB) agar (Cawthorn et al., 2008), were observed in G4023 and ATCC 29544 bacteria; EHEC O157: H7 EDL933 and Salmonella Typhimurium ATCC 14028 bacteria presented white colonies on both DFI agar and LB agar (Figure 1C and Table 1). Meanwhile, a commercial kit for C. sakazakii identification was applied to G4023 bacteria, with ATCC 29544 as a control. Both of the strains reacted exactly like the typical C. sakazakii according to instruction (Table 1 and Supplementary Figure 1), which further proved that G4023 belongs to C. sakazakii.
Table 1. The results obtained from biochemical methods for the detection and identification of Cronobacter sakazakii G4023.
Growth curves of C. sakazakii G4023 and ATCC 29544 strains cultured in LB medium exhibited no significant differences [approximately 2 × 105 colony forming units (CFU) were initially inoculated; Figure 2A]. To measure the desiccation tolerance of C. sakazakii G4023 strain, we desiccated the bacteria for up to 50 days and set C. sakazakii ATCC 29544 as a control. We dispensed the bacteria into individual wells of 12-well microtiter plates separately for desiccation treatment. Every 5 days, the viable count (CFU/ml) of each strain was documented to assess the desiccation tolerance ability. As shown in Figure 2B, viable counts of both G4023 and ATCC 29544 were sharply decreased during the first 5-day interval and tended to be stable after then. These results indicated that G4023 and ATCC 29544 strains might have adapted to desiccation during the first 5 days, and G4023 has better resistance to desiccation than ATCC 29544. Due to the strong desiccation tolerance and pathogenicity of G4023 (Wang M. et al., 2019), it could be a potential threat to infants. Therefore, we performed transcriptomic and proteomic analyses of desiccated C. sakazakii G4023 to provide insights into the desiccation adaption mechanisms.
Figure 2. Growth curves and desiccation tolerance of C. sakazakii G4023 and ATCC 29544. (A) Growth curves of C. sakazakii G4023 and ATCC 29544 cultured in LB medium. The overnight cultured bacteria were diluted in the ratio of 1:1,000 (initial inoculum size was approximately 2 × 105 CFU) and with shaking. OD600 measurements were performed every 10 min. Both strains entered the logarithmic phase at about 2 h and reached the stationary phase at about 9 h. (B) Progress curve of culturable cell counts during the desiccation of C. sakazakii G4023 and ATCC 29544. The CFU were counted every 5 days, and 50 days of desiccation tolerance was studied in total. ATCC 29544 barely survived, whereas quite a number of G4023 bacteria survived for at least 50 days in desiccation.
As suggested above, C. sakazakii G4023 bacteria have adapted to desiccation during the first 5 days, and similar desiccation treatment for 4 days has been reported earlier; so samples used for RNA-seq and quantitative proteomic analyses were desiccated for 4 days as previously described (Shaker et al., 2008; Chaibenjawong and Foster, 2011). Bacteria cultured in LB medium were collected as the control group. High-throughput Illumina RNA-seq system (Novaseq 6000) was used to systematically catalogue the transcripts. After filtering, a total of 18,914,098 and 20,738,460 clean reads were obtained for the desiccated and control sets of C. sakazakii G4023, respectively. Approximately 98.11% of the total reads for desiccated C. sakazakii G4023 and 97.64% of those for control set were uniquely mapped to the respective reference genome. We observed 1,573 genes to be differentially expressed (≥2-fold change), out of which 609 were upregulated and 964 were downregulated (Figure 3A). Detailed differential expression profiles are shown in Supplementary Table 2.
Figure 3. Transcriptome analysis. (A) Volcano plots of upregulated and downregulated (≥2-fold) mRNAs in desiccated C. sakazakii G4023. The red dots represent upregulated genes, green dots represent downregulated genes, and blue dots represent no significantly differentially expressed gene. (B) Confirmation of the RNA-seq results by qRT-PCR analysis; the expressions were normalized with the internal control gene glnS. The qRT-PCR fold-change tendencies agreed with the RNA-seq results. The error bars represent mean ± SD; n = 3.
The RNA-seq results were further confirmed by quantitative real-time PCR (qRT-PCR) analysis. The housekeeping gene glnS was used as the internal control gene as its expression did not change significantly under desiccation (Supplementary Figure 2). Ten genes were selected randomly to detect the fold-changes under desiccation. Although the fold-change values were not exactly alike, the regulation trends agreed with the RNA-seq results (Figure 3B), indicating the validity of the RNA-seq data.
The differentially expressed genes (DEGs) in the transcriptome were assigned annotations to three primary gene ontology (GO) domains: biological process (BP), cellular component (CC), and molecular function (MF). A total of 1,140 DEGs were successfully annotated against the GO database, of which 77 GO terms were significantly enriched (36 terms assigned to BP, 19 to CC, and 22 to MF, listed in Supplementary Table 2), and the most enriched 30 GO terms are shown in Figure 4. Genes involved with gene expression, macromolecule biosynthetic process, intracellular components, and cofactor binding were observed the most significantly differentially expressed in desiccated C. sakazakii G4023.
Figure 4. The most enriched 30 GO terms of desiccation C. sakazakii G4023. Twelve terms belong to biological process; eleven terms belong to cellular component, and five terms belong to molecular function.
The DEGs were also subjected to Kyoto Encyclopedia of Genes and Genomes (KEGG) analysis. Only three pathways, namely, citrate cycle, oxidative phosphorylation, and carbon metabolism, were significantly enriched out of the 84 enriched ones (Supplementary Table 2), which suggested that the basal metabolisms were the most challenged in desiccated C. sakazakii G4023.
Label-free quantitative proteomic analyses were simultaneously performed to investigate the regulations that happened at the protein level of desiccated C. sakazakii G4023, with LB-cultured bacteria set as the control group as well. Proteomics analysis showed that a total of 209,357 spectra were matched to 18,763 peptides, and 2,225 proteins were identified, out of which 1,754 were quantifiable. After data filtering (≥1.5-fold change), 360 proteins were observed upregulated and 322 were downregulated (Figure 5A). All the differentially expressed proteins (DEPs) are listed in Supplementary Table 3.
Figure 5. Proteomic analysis of desiccated C. sakazakii G4023. (A) Volcano plots of upregulated and downregulated (≥1.5-fold) proteins in desiccated C. sakazakii G4023. The red dots represent upregulated proteins, blue dots represent downregulated proteins, and gray dots represent no significantly differentially regulated protein. (B) COG analysis of DEPs. Bars represent the number of DEPs in each COG category. Besides the ones with unknown function, DEPs were categorized mostly in translation, ribosomal structure, and biogenesis, followed by in cell wall/membrane/envelope biogenesis and amino acid transport and metabolism. (C) Significantly enriched GO terms of DEPs in desiccated C. sakazakii G4023; 14 terms belong to BP, 8 terms belong to CC, and 8 terms belong to MF. (D) Seven KEGG pathways of DEPs were significantly enriched in desiccated C. sakazakii G4023. (E) Ten protein domains of DEPs were significantly enriched in desiccated C. sakazakii G4023.
In order to obtain a better understanding of DEPs in desiccated C. sakazakii G4023, we performed the GO annotation and functional classification, subcellular location classification, and functional classification of clusters of orthologous groups of proteins (COG). Five hundred forty-seven identified DEPs (80.20% of the total) were annotated to GO terms (details shown in Supplementary Figure 3), and 30 hierarchically structured GO classes were significantly enriched (Figure 5B and Supplementary Table 3). According to the GO enrichment results, proteins related to proteolysis regulation, pyrimidine biosynthesis, propionate metabolic process, and acid-ammonia (or amide) ligase activity achieved the greatest fold enrichment. Subcellular location analysis of the 682 DEPs revealed that most of them (63%) are located in the cytoplasm, whereas outer membranal DEPs and extracellular DEPs were only 3.37 and 2.49%, respectively (Supplementary Figure 3). COG analyses showed that except for the functionally unknown proteins, DEPs were mostly categorized into translation, ribosomal structure and biogenesis (70/74 proteins were upregulated), cell wall/membrane/envelope biogenesis (50/73 proteins were downregulated), and amino acid transport and metabolism (49/71 proteins were upregulated) (Figure 5C and Supplementary Table 4). These indicated that a large abundance of proteins was needed by C. sakazakii G4023 to cope with desiccation, which was also revealed by Hu et al. (2017), and transport and metabolism of amino acids might contribute to the G4023 bacterial survival under desiccation stress.
Enrichment of KEGG pathways and protein domains of DEPs were performed as well. Three hundred sixty-three DEPs were allocated to the reference pathways in KEGG, and seven pathways were significantly enriched (p ≤ 0.05; Figure 5D and Supplementary Table 3). Three of the seven (42.86%) were related to biosynthesis and metabolism of amino acids (arginine, alanine, aspartate, glutamate, taurine, and hypotaurine). Correspondingly, amino acid biosynthesis and metabolism-related protein domains were also significantly enriched (Figure 5E and Supplementary Table 3).
Based on the transcriptome and proteome of desiccated C. sakazakii G4023, we performed an integrated analysis of these two omics to promote a better understanding of bacterial responses to desiccation. Overall, 331 genes were found to be regulated at both transcriptional level (≥2.0-fold) and protein levels (≥1.5-fold) under desiccation stress (Figure 6A). COG analysis of these 331 genes suggested that 325 genes were classified into 18 COG categories (Figure 6B, left). Besides those whose functions are unknown, genes were mostly involved in translation (44 genes), amino acid metabolism and transport (39 genes), energy production and conversion (30 genes), cell wall/membrane/envelope biogenesis (27 genes), and carbohydrate metabolism and transport (27 genes) categories, which were highly consistent with COG categorizing of DEPs. Further analysis suggested that 191 out of the 331 genes were regulated in matching tendencies, which were upregulated or downregulated at both transcriptional and protein levels (87 up/upregulated and 104 down/downregulated; Supplementary Table 5), and the fold changes are shown in the heatmap (Supplementary Figure 4). The COG classifications showed the up/upregulated group was dominantly sub-categorized into the translation category (Figure 6B, middle), and the down/downregulated group was mostly sub-categorized into the cell wall/membrane/envelope biogenesis.
Figure 6. Integrated analysis of transcriptomic and proteomic data from desiccated C. sakazakii G4023. (A) Comparison between the numbers of differentially transcribed genes and differentially expressed proteins of desiccated C. sakazakii G4023. Genes with mRNA showing at least 2-fold change and proteins with at least 1.5-fold change are shown in the Venn diagram. In total, 1,573 DEGs and 682 DEPs were detected. The overlapped area represents 331 genes that were found differentially regulated at both transcriptional and protein levels. Adjusted p ≤ 0.05 for all data selected. (B) COG analyses of the 331 genes regulated at both transcriptional level and protein level (left); 44 were related to translation. In the up/upregulated group (genes were upregulated at both transcriptional and protein levels); genes were mostly categorized in translation (middle). In the down/downregulated group (genes were downregulated at both transcriptional and protein levels), genes were relatively related to the cell wall/membrane/envelop biogenesis (right).
In desiccated C. sakazakii G4023, protein levels of CheA, CheW, CheY, CheB, and CheR were upregulated 1. 78-, 1. 25-, 2. 00-, 3. 22-, and 1.23-fold, respectively, while the transcriptional expressions of the encoding genes (cheA, cheW, cheB, and cheR) were decreased (2. 22-, 3. 37-, 3. 54-, and 4.25-fold, respectively), and expression of gene cheY was not detected in transcriptome (Table 2). CheA is a histidine kinase and plays a central role in signal transduction of bacterial chemotaxis. The coupling protein CheW, cognate response regulator CheY, methyltransferase CheR, and methylesterase CheB are also involved (Kehry et al., 1984; Stephens et al., 2006; Muok et al., 2020).
Table 2. Transcriptional and protein expression changes of proteins involved in maltose/maltodextrin metabolism, ABC transporters, and arginine biosynthesis in desiccated C. sakazakii G4023.
To further investigate what role che system plays in desiccated C. sakazakii G4023, we constructed cheA, cheW, cheY, cheB, and cheR mutant strains of G4023, and their growth rates exhibited no significant differences in vitro (Figure 7A). Desiccation tolerance tests were then performed. Four-day desiccation results suggested that the G4023ΔcheR strain exhibited stronger desiccation tolerance than C. sakazakii G4023, while G4023ΔcheA and G4023ΔcheW showed significantly decreased tolerance (Figure 7B). The 50-day desiccation tests showed that G4023ΔcheA, G4023ΔcheW, and G4023ΔcheB exhibited significantly decreased resistance to desiccation compared with that of C. sakazakii G4023, especially G4023ΔcheA (Figure 7C). These results together with the upregulations in CheAWYBR protein levels suggested that the chemotaxis signaling cascade, especially the core protein CheA, might be important in the desiccation survival of C. sakazakii G4023.
Figure 7. Desiccation tolerance of chemotaxis gene mutant strains of C. sakazakii G4023. (A) Growth curves of C. sakazakii WT strain G4023 and chemotaxis gene-mutant strains (G4023ΔcheY, G4023ΔcheB, G4023ΔcheR, G4023ΔcheW, and G4023ΔcheA) cultured in LB medium with shaking; OD600 measurements were done every 10 min. There was no growth deficiency in the che mutant strains compared to that in WT. (B) Re-cultured cell counts (CFU/ml) of C. sakazakii WT strain G4023 and the chemotaxis gene-mutant strains (G4023ΔcheY, G4023ΔcheB, G4023ΔcheR, G4023ΔcheW, and G4023ΔcheA) in LB agar medium after being desiccated for 4 days. The error bars represent mean ± SD; n = 3. Differences between two groups were evaluated by a two-tailed Student’s t-test. A p-value of < 0.05 was considered statistically significant. Asterisks are used to indicate significant differences (*p < 0.05; **p < 0.01; NS, not significant). (C) Progress curve of culturable cell counts during the desiccation of WT G4023, G4023ΔcheY, G4023ΔcheB, G4023ΔcheR, G4023ΔcheW, and G4023ΔcheA. The CFU were counted every 5 days, and 50 days of desiccation tolerance was studied in total.
It has been reported that flagellum synthesis can be regulated by a chemotaxis signal transduction pathway to optimize swim cell–swarm cell differentiation in response to changing environmental conditions in Rhodospirillum centenum (Berleman and Bauer, 2005). Since the chemotaxis system relevant proteins were upregulated in desiccated C. sakazakii G4023, we wondered whether the flagellar synthesis was affected as well. Flagellar biosynthesis genes are organized in a three-tiered cascade allowing for regulated expression (Romilly et al., 2020). The products of class I operon, FlhD and FlhC, are regulators positively controlling the expression of class II operons. However, the transcriptional expressions of genes flhD and flhC showed no significant changes (0.89- and 1.01-fold), and regulations at protein levels were not determined as only FlhD was detectable in the desiccated sample, which could result from the fact that the protein amount is not enough to reach the detection threshold of label-free quantitative proteome analysis.
Although expression regulations of class I operon were not suggested in the omics analyses, FliA and FlgM encoded by class II genes were found upregulated 1.86- and 2.59-fold, respectively at protein level; transcriptional expressions of fliA and flgM genes were downregulated 4.43- and 1.12-fold, respectively. FliA is a sigma factor σ28, whereas FlgM is an anti-sigma factor which binds to FliA to prevent its association with RNA polymerase core enzyme (Hughes and Mathee, 1998). The FliA–FlgM regulatory system is involved not only in the expression of class III but also in that of class II operons, and the relative concentration of FliA and FlgM may play an important role in the production of flagella (Kutsukake and Iino, 1994). In addition to these two regulators, class II genes also encode basal body and hook components (Kapatral et al., 1996). Unlike the upregulations of FliA and FlgM, the hook-associated proteins FlgK, FlgL, FliC, and FliD were all found downregulated over 2-fold at both transcriptional and protein levels (Table 2). The downregulation of basal body and hook components of flagella suggested that flagellum demand in C. sakazakii G4023 was decreased in desiccation, and energies could be saved for better survival.
Class III genes regulated by FliA–FlgM regulatory system encode flagellar motor switch proteins and flagellin, such as FliG, FliM, and FliN, which were found increased 1. 44-, 2. 03-, and 2.86-fold, respectively, at protein level (Table 2). It has been reported that FliG, FliM, and FliN interact with one another to form a switch complex, which in turn is thought to interact with chemotaxis protein CheY, to induce clockwise rotation of the flagellar motors and tumbling of the cell (Toker and Macnab, 1997). From these results, we guessed that when C. sakazakii G4023 bacteria encounter the desiccation, flagellum numbers needed by each cell is decreased, but the flagellar motors and tumbling ability of bacteria are needed to be increased to favor survival in desiccation stress.
LPS, which is composed of lipid A, a core oligosaccharide, and O-antigen repeats (Liu et al., 2019), anchors in the outer membrane of gram-negative bacteria and serves as an effective barrier between the bacterium and its environment (Sun et al., 2011). It has been reported that cleavage of LPS offered C. sakazakii bacteria higher resistance to the hostile environment as it caused outer membrane defects and increased biofilm formation (Wang L. et al., 2012).
In this study, we focused on the O-antigen, which is generally not necessary for the survival of the microorganism (Gronow and Brade, 2001), to investigate its role in adaptation to desiccation stress of C. sakazakii G4023. The O-antigen biosynthesis genes in the genome of C. sakazakii are normally located between galF and gnd (Sun et al., 2011), and the transcriptional expressions of these genes were found downregulated in desiccated C. sakazakii G4023 (Table 2). However, most of the proteins encoded by these genes were not able to be quantified at the protein level; this may be due to their low abundance as they were mostly located on cell membranes (Okuda et al., 2016). We tried to mutate the O-antigen biosynthesis essential gene, wzy or wzx (Wang et al., 2009), in C. sakazakii G4023 but failed; we then turned to two other important genes for O-antigen biosynthesis, wzzB and fepE, which are responsible for the biosynthesis of long-chain and very-long-chain O-antigens, respectively (Murray et al., 2003). O-antigen chain length has been reported to affect survival and infection procedures in several bacteria (Murray et al., 2003; Kintz and Goldberg, 2011; da Silva et al., 2018). The expression of wzzB in this study was found decreased 1.70- and 1.72-fold at transcriptional and protein levels, respectively; while fepE was found downregulated 2.67-fold at transcriptional level but upregulated 1.74-fold at protein level.
To further investigate whether FepE and WzzB played roles in desiccation tolerance of C. sakazakii G4023, we constructed the fepE and wzzB mutant strains (G4023ΔfepE and G4023ΔwzzB). Both mutant strains showed no growth defects in vitro (Figure 8A), and the desiccation tolerance tests were performed. Results of a 4-day term of desiccation showed that both G4023ΔfepE and G4023ΔwzzB exhibited better survival than G4023 (Figure 8B), whereas the survival advantages faded with desiccation time, especially G4023ΔfepE. By the 50th day of desiccation, bacterial recovery counts of G4023ΔfepE were significantly less than those of G4023 (Figure 8C). These results indicated that shorter O-antigen chain length biosynthesis aids in the survival of C. sakazakii G4023 in the short-term rather than the long-term desiccation.
Figure 8. The role of O-antigen chain length-regulation genes in the desiccation tolerance of C. sakazakii G4023. (A) Growth curves of C. sakazakii wild-type (WT) strain G4023, G4023ΔfepE, and G4023ΔwzzB in LB medium at 37°C with shaking; OD600 measurements were done every 10 min. There were no differences in the growth of G4023, G4023ΔfepE, and G4023ΔwzzB. (B) Re-cultured cell counts (CFU/ml) of C. sakazakii WT strains G4023, G4023ΔfepE, and G4023ΔwzzB in LB agar medium after being desiccated for 4 days. All of the three strains could survive desiccation, and the desiccation tolerance of G4023ΔfepE and G4023ΔwzzB was significantly stronger than that of wild-type strain. (C) Progress curve of culturable cell counts during the desiccation of WT G4023, G4023ΔfepE, and G4023ΔwzzB. The CFU were counted every 5 days, and 50 days of desiccation tolerance was studied in total. (D) Absorbances of crystal violet at 590 nm was used to assess the biofilm formation abilities of C. sakazakii WT strain G4023, G4023ΔfepE, and G4023ΔwzzB. Biofilm formation of LB cultured strains and desiccation-treated strains were detected separately. All the three strains showed significantly increased biofilm formation under desiccation stress. Desiccated G4023ΔfepE and G4023ΔwzzB formed more biofilms than the desiccated WT strain, although the three showed no significant difference in biofilm formation when cultured in LB medium. The error bars represent mean ± SD; n = 3. Differences between two groups were evaluated by a two-tailed Student’s t-test. A p-value of < 0.05 was considered statistically significant. Asterisks are used to indicate significant differences (*p < 0.05; **p < 0.01).
Moreover, crystal violet staining assays were also performed to quantify the biofilm formed by G4023, G4023ΔfepE, and G4023ΔwzzB. There was no significant difference in biofilm formation between G4023 and the mutant strains when they were cultured in LB. However, after desiccation treatment for 4 days, all of them exhibited significant increases in biofilm formation. Notably, biofilm formed by desiccated G4023ΔfepE and G4023ΔwzzB was significantly more than that in desiccated G4023 (Figure 8D). These results indicated that C. sakazakii G4023 increased its biofilm formation to overcome desiccation, and shortening of O-antigen chain length could further facilitate the biofilm formation yet only happened when bacteria were suffering desiccation.
COG and KEGG enrichment analyses of DEPs, as well as COG classifications of genes regulated at both transcriptional and protein levels, suggested that proteins related to transport and metabolism of amino acids were significantly regulated in desiccated C. sakazakii G4023. Detailed analyses revealed that proteins involved in arginine biosynthesis, such as ArgABCDEFGH, CarAB, and the regulator ArgR (Caldara et al., 2008), were upregulated; furthermore, ArtI and ArtJ involved in arginine ABC transporter (Bai et al., 2021) were also upregulated 1.7- and 2.22-fold at protein level, respectively (Table 2). These results indicated that biosynthesis and transport of arginine may contribute to the survival of C. sakazakii G4023 in desiccation, but whether and how the arginine functions required further investigations.
During the analyses, we found the transcriptional expressions of arginine biosynthesis genes (argABCDEFGH) were downregulated (Table 2). As it has been reported that productions of all enzymes involved in arginine biosynthesis are subjected to repression by arginine, mediated by the repressor ArgR (Vyas and Maas, 1963), these downregulations could have resulted from the upregulated protein level of ArgR.
Iron/sulfur cluster is involved in all kingdoms of life to be catalysts or redox sensors in diverse BPs and is formed by Fe/S biogenesis systems NIF, ISC, and SUF (Yang et al., 2006; Roche et al., 2013), of which ISC and SUF systems allow the maturation of all Fe/S proteins in cells, while NIF is dedicated to the maturation of nitrogenase (Ayala-Castro et al., 2008). Genes encoding ISC and SUF are present in the genome of C. sakazakii G4024, while those for NIF are absent. The operon iscSUA-hscBA, encoding the ISC system (Roche et al., 2013), were observed upregulated 9. 2-, 6. 09-, 6. 40-, 7. 27-, and 5.21-fold, respectively, at the transcriptional level in desiccated C. sakazakii G4024. Meanwhile, protein amounts of IscS, IscU, and HscA were also increased 2. 31-, 2. 06-, and 1.96-fold, respectively; whereas IscA and HscB were not detectable in proteome (Table 2). Additionally, genes encoding the two sub-complexes, SufBCD and SufSE, of SUF system were found transcriptionally regulated (sufABCDS were increased 3. 59-, 4. 43-, 2. 71-, 3. 72-, and 2.83-fold, respectively, while sufE was decreased 1.22-fold). Besides, the protein levels of SufABCDSE were 0. 50-, 0. 76-, 0. 53-, 0. 82-, 0. 68-, and 1.18-fold, respectively, in desiccated C. sakazakii G4023 compared with that in LB-cultured (Table 2). Considering the ISC systems were upregulated at both transcriptional and protein levels and the SUF system was increased at the transcriptional level but decreased at the protein level, we speculated that when C. sakazakii G4023 suffered desiccation, the bacteria would prefer the ISC system instead of the SUF to synthesize F/S cluster to cope with the stress. However, these surmises need to be confirmed with further experimental investigations.
The goals of this study were to investigate the desiccation tolerance of C. sakazakii G4023 and reveal potential factors involved through the analyses of transcriptome and proteome. This study suggested C. sakazakii G4023 strain has stronger desiccation tolerance than C. sakazakii ATCC 29544 strain. This makes sense as G4023 is a food-isolated strain, while ATCC 25544 was a clinical one. Food-isolated microorganisms are exposed to a wide range of stresses, such as desiccation and osmotic stress, in food processing or preparation environments (Osaili and Forsythe, 2009), so G4023 must be equipped with strong desiccation tolerance to survive. Additionally, ATCC 29544 was reported to have a weak ability to form biofilm (Yang et al., 2016), while biofilm formation has been proved to contribute to desiccation resistance in C. sakazakii as well as other species of microorganism (Hansen and Vogel, 2011; Du et al., 2018). Therefore, the weaker desiccation tolerance of ATCC 29544 is reasonable.
iTRAQ-based comparative proteome and RNA-seq-based transcriptome were performed in 2017 and 2019, respectively, to gain insights into responses in C. sakazakii to desiccation (Hu et al., 2017; Srikumar et al., 2019). However, the C. sakazakii ATCC 29544 strain was used in the proteomic analyses and only desiccated for 24 h. Since the stronger desiccation tolerance of G4023 than ATCC 29544 was suggested in this study, using G4023 instead of ATCC 29544 to reveal desiccation resistance mechanisms seems more convincing. Additionally, although a PIF-isolated C. sakazakii SP291 strain was used in the transcriptional analyses study, the desiccated time was only 4 h, and it seems too short to reveal the regulations that happened during the long-term desiccation. So in this study, C. sakazakii G4023, which has stronger resistance to desiccation, was treated under dry stress for 4 days to perform the RNA-seq and protein quantification.
Subcellular location analyses of DEPs in this study showed that only 3.37 and 2.49% were outer membranal and extracellular proteins, respectively. The low percentages may be due to the low abundance of these proteins in samples, as solubilization, sample handing, preparation, separation, and analysis of the membranal proteins are usually complicated due to their hydrophobic nature (Han et al., 2008). Therefore, most of the proteins involved in O-antigen biosynthesis in G4023 were not able to be quantified as they were mostly located on cell membranes. Several methodologies have been developed to improve membrane protein identification and also to perform reliable quantification, which allows in-depth membrane protein studies (Helbig et al., 2010). Consequently, the membrane proteomic analysis of desiccated C. sakazakii G4023 could be performed in the future to gain further insights into the roles of O-antigen and other membranal proteins playing under desiccation stress.
Integrated analyses of transcriptome and proteome revealed that a large number of genes related to translation were upregulated at both transcriptional and protein levels in this study. Yang et al. (2016) suggested previously that C. sakazakii with a stronger biofilm formation ability contains higher levels of proteins related to 50S ribosome, 30S ribosome, and purine and pyrimidine biosynthesis. Therefore, the upregulated translation-relevant proteins may assist the biofilm formation in desiccated C. sakazakii G4023, which in turn contributes to adaptation and survival. Although this study has experimentally proved increased biofilm formation in G4023 under desiccation, the detailed mechanisms still need to be revealed in the future. Meanwhile, the observed downregulation of cell wall/membrane/envelope biogenesis genes at both transcriptional and protein levels in this study also makes sense because bacterial surface structures bear the brunt of desiccation. Instead of re-building the original surface structures, the re-organizations, such as biofilm formation, could endow C. sakazakii bacteria with better survival during desiccation (Li et al., 2012). So biogenesis of cell wall/membrane/envelope may be reduced in desiccated G4023. At the same time, the desiccated situation is harsh for bacteria to survive, and the limited energy will prioritize BPs that are more conducive to survival.
As opposite regulation trends of the chemotaxis genes were observed in transcriptome and proteome of desiccated C. sakazakii G4023, there may exist negative feedback regulations on the expression of che operons, which require further investigations. Furthermore, since 416 chemosensory systems within 245 prokaryotic species have been revealed (Wuichet and Zhulin, 2010), and several gram-negative species have been reported to utilize variations of chemotaxis signaling cascade to switch lifestyles, such as flagellar motility, type IV pili-based motility, cell development, biofilm formation, exopolysaccharide production, and flagellum biosynthesis, to survive environmental stress (He and Bauer, 2014), it is likely that C. sakazakii G4023 could also utilize a chemosensory system to switch lifestyles for better survival in desiccation.
The decreased desiccation resistance of G4023ΔfepE in the long term and the upregulated protein level of FepE suggest that FepE may play a role in the survival of C. sakazakii G4023 under desiccation stress. In view of the fact that FepE was also involved in ferric enterobactin transportation in Enterobacteriaceae bacteria (Ozenberger et al., 1987), we speculated that deficiency in fepE might result in impaired ferric enterobactin transport, which could be disadvantageous in the long-term desiccation tolerance of C. sakazakii G4023.
We are just starting to understanding the influences of the chemotaxis system and the O-antigen chain length on desiccation tolerance of C. sakazakii G4023, and plenty of work needs to be done in the future to gain further comprehension.
All the strains, plasmids, and primers used in this study are listed in Supplementary Table 6. The C. sakazakii G4023 strain is a food-isolated strain kindly offered by Beijing Entry--Exit Inspection and Quarantine Bureau and belongs to ST4. The ST type of G4023 was defined by the Cronobacter spp. multilocus sequence typing (MLST) Database.1 Genomic comparisons between G4023 and ATCC 29544 (ST8) were performed to identify G4023 as a C. sakazakii strain. The whole genome comparison was performed by local BLASTp (v2.3.0)2 with an E-value cutoff of <1e−10, identity >80%, and coverage >80%, and genomic islands were identified by software IslandViewer 4. Then the results were visualized by software BLAST Ring Image Generator (BRIG). The ANI and TUD were calculated via online server JspeciesWS3 (Richter et al., 2016); The AAI was calculated by software toolkit CompareM.4 Overnight LB-cultured bacteria of C. sakazakii G4023, C. sakazakii ATCC 29544, EHEC O157: H7 EDL933, and Salmonella Typhimurium ATCC 14028 were steak cultured on the selective DFI agar and the LB agar; the plates were then statically cultured in 37°C overnight to observe the phenotypes of colonies. Meanwhile, biochemical identifications of G4023 and ATCC 29544 were performed with commercial biochemical identification kit [Biochemical identification strip of E. sakazakii (GB), Hopebio, Qingdao, China], and the positive or negative responses were judged by the color changes of mediums according to the manufacturer’s instructions.
Bacteria in this study were grown at 37°C in LB medium under standard bacterial growth conditions unless otherwise specified. For growth curves, three replicates per strain were grown in 200 μl LB medium in a 96-well plate and inoculated aerobically in the ratio of 1:1,000 from overnight cultures at 37°C. The initial inoculum was approximately 2 × 105 CFU and monitored by measuring the OD600 value using a Multiskan GO 1510 plate reader (Thermo Fisher Scientific, Vantaa, FIN) every 10 min.
Mutant strains were generated using the λ Red recombination system described previously (Datta et al., 2006) with the plasmid pSIM17 generated by Chan et al. (2007). The plasmid pSIM17 is a temperature-sensitive, low-copy plasmid and contains blasticidin resistance. In brief, we first electroporated pSIM17 into C. sakazakii G4023 bacteria and selected on blasticidin-containing (50 μg/ml) LB agars at 30°C. Then the kanamycin cassette used to replace the target genes of C. sakazakii G4023 was amplified from pKD4 with primers listed in Supplementary Table 6. Each primer contains a 5′ end homologous to the flanking regions of the target gene and a 3′ end that primes the kanamycin cassette for PCR amplification. The amplified PCR products were purified with SanPrep Column DNA Gel Extraction Kit (Sangon Biotech, China) and electroporated into pSIM17-containing C. sakazakii G4023 strain, which had been induced for red expression by growth at 42°C for 15 min. After electroporation, 1 ml LB was added, and the bacteria were incubated at 37°C with shaking for 3–4 h. Then the bacteria were cultured on LB agar containing 50 μg/ml of kanamycin to select drug resistant recombinants at 37°C. Several colonies on each plate were picked on the following day and amplified with mutant identification primers, after which the amplified products were sequenced to verify the replacement of target genes with kanamycin cassette (Njoroge et al., 2012). The verified mutant colonies were re-cultured in LB medium supplemented with kanamycin (50 μg/ml) at 37°C and preserved in glycerin at −80°C until use.
Strains used for desiccation tolerance test were grown overnight and transferred to fresh LB medium at a ratio of 1:100 for 4 h until mid-exponential phase, and 50-μl aliquots were dispensed into individual wells of a 12-well microtiter plate, and three replicates were set for each strain. Thereafter, the bacteria were desiccated as previously described (Shaker et al., 2008; Hu et al., 2017). In brief, the plates were incubated statically in a 37°C incubator without a lid for 4 h for drying. Dehydrated silica gel was placed in the incubator. After drying, the plate was covered with a lid and kept at 30°C statically for 4 days. After desiccation, the cells were rehydrated with 1 ml sterile phosphate buffered saline (PBS, pH 7.4), and 100-μl aliquots were re-cultured in LB agar plates at 37°C for 24 h to count the cell population (CFU/ml).
RNA samples from desiccated C. sakazakii G4023 were prepared using TRIzol® LS Reagent (Invitrogen, Carlsbad, CA, United States) and treated with RNase-Free DNase I (Fermentas, Burlington, Canada) to eliminate genomic DNA contamination. C. sakazakii G4023 cultured in LB medium was set as control. Purified RNA samples were stored at -80°C till further use. The library preparation and RNA-seq were carried out commercially (Novogene, Tianjin, China). In brief, a total of 3 μg RNA per sample was used as input material for the RNA sample preparations. Sequencing libraries were generated using NEBNext® Ultra™ Directional RNA Library Prep Kit for Illumina® (NEB, United States) following manufacturer’s recommendations. In order to select cDNA fragments of preferentially 150–200 bp in length, the library fragments were purified with AMPure XP system (Beckman Coulter, Beverly, United States). Then 3 μl USER Enzyme (NEB, United States) was used with size-selected, adaptor-ligated cDNA at 37°C for 15 min followed by 5 min at 95°C before PCR. Then PCR was performed with Phusion High-Fidelity DNA polymerase, Universal PCR primers and Index (X) Primer. At last, products were purified (AMPure XP system), and library quality was assessed on the Agilent Bioanalyzer 2100 system. The clustering of the index-coded samples was performed on a cBot Cluster Generation System using TruSeq PE Cluster Kit v3-cBot-HS (Illumia) according to the manufacturer’s instructions. After cluster generation, the library preparations were sequenced on the NovaSeq 6000 (Illumina), and paired-end reads were generated.
For proteomic analysis, desiccated C. sakazakii G4023 cells were harvested and washed with ice-cold PBS five times. Cells were stored in liquid nitrogen overnight and then stored at −80°C until required. Samples were sonicated three times on ice using a high-intensity ultrasonic processor (Scientz) in lysis buffer (8 M urea, 1% Protease Inhibitor Cocktail). The remaining debris was removed by centrifugation at 12,000 g at 4°C for 10 min. Finally, the supernatant was collected, and the protein concentration was determined with BCA Protein Assay Kit (abcam, Cambridge, England) according to the manufacturer’s instructions. For digestion, the protein solution was treated as previously described (Ondrej et al., 2020). Accordingly, the protein solution was reduced with 5 mM dithiothreitol for 30 min at 56°C and alkylated with 11 mM iodoacetamide for 15 min at room temperature in darkness. The protein sample was then diluted by adding 100 mM triethylammonium bicarbonate (TEAB) to urea concentration less than 2 M. Finally, trypsin was added at 1:50 trypsin-to-protein mass ratio for the first digestion overnight and 1:100 trypsin-to-protein mass ratio for a second 4-h digestion. C. sakazakii G4023 cultured in LB medium was set as control. Three biological replicates were prepared. The tryptic peptides were then submitted to conduct the label-free quantitative mass spectrometry analyses commercially (PTM BIO, Hangzhou, China); high-performance liquid chromatography (HPLC) fractionation and liquid chromatographic-tandem mass spectrometry (LC-MS/MS) analyses were performed as previously described (Ondrej et al., 2020). Briefly, the tryptic peptides were fractionated into fractions by high pH reverse-phase HPLC using Thermo Betasil C18 column [5 μm particles, 10 mm inner diameter (ID), 250 mm length]. Peptides were first separated with a gradient of 8–32% acetonitrile (pH 9.0) over 60 min into 60 fractions. Then, the peptides were combined into six fractions and dried by vacuum centrifuging. The tryptic peptides were then dissolved in 0.1% formic acid (solvent A), directly loaded onto a home-made reversed-phase analytical column (15-cm length, 75-μm ID). The gradient comprised an increase from 6 to 23% solvent B (0.1% formic acid in 98% acetonitrile) over 26 min, 23–35% in 8 min, climbing to 80% in 3 min, and then holding at 80% for the last 3 min, all at a constant flow rate of 400 nl/min on an EASY-nLC 1000 ultra-performance liquid chromatography (UPLC) system. The peptides were subjected to nanospray ionization (NSI) source followed by MS/MS in Q ExactiveTM Plus (Thermo Fisher Scientific) coupled online to the UPLC. The electrospray voltage applied was 2.0 kV. The m/z scan range was 350–1,800 for full scan, and intact peptides were detected in the Orbitrap at a resolution of 70,000. Peptides were then selected for MS/MS using normalized collision energies (NCE) setting of 28, and the fragments were detected in the Orbitrap at a resolution of 17,500. A data-dependent procedure alternated between one MS scan and 20 MS/MS scans with 15.0 s dynamic exclusion. Automatic gain control (AGC) was set at 5E4. Fixed first mass was set at 100 m/z. Finally, the resulting MS/MS spectrums were searched against UniProt database Cronobacter_sakazakii_strain_ATCC_BAA-894_290339_PR_20191018.
RNA-seq data were validated using qRT-PCR. RNA purified from the desiccated and control C. sakazakii G4023 cells was transcribed to cDNA using the PrimeScript 1st Strand cDNA Synthesis Kit (Takara, Japan). qRT-PCR analysis was performed in an Applied Biosystems ABI 7300 Real-Time PCR System. Each qRT-PCR reaction was carried out in a total volume of 20 μl in a 96-well optical reaction plate (Applied Biosystems, Foster, CA, United States) containing 10 μl Power SYBR Green PCR Master Mix (Applied Biosystems, Foster, CA, United States), 1 μl cDNA, and two gene-specific primers with a final concentration of 0.3 mM each. The housekeeping gene glnS was used as a reference control for normalization as its transcriptional expression levels did not change dramatically when C. sakazakii G4023 bacteria suffered desiccation (Supplementary Figure 2). Relative difference in gene expression was calculated as the fold change using the 2–ΔΔCt method. At least three biological replicates were performed for each qRT-PCR analysis.
Crystal violet staining assay was performed to assess the formation of biofilm as described previously (Fernández-Gómez et al., 2020). In brief, 100-μl overnight strains were cultured in 96-well polystyrene microtiter plates and subjected to desiccation. On the third day of desiccation, the 96-well plates were taken out, and overnight-cultured strains were diluted 1:100 in fresh LB medium in the remaining blank wells. The plate was then statically cultured at 30°C for 24 h. The desiccated and LB cultured bacteria were stained with 0.5% crystal violet for 5 min after washing with double-distilled water. Next, 200 μl of 95% ethanol was added after removing the unbound crystal violet to dissolve the biofilm-bound dye, and the ability of biofilm formation was determined by measuring the absorbance at 590 nm with an enzyme-linked immunosorbent assay plate reader. Three wells of each strain were studied in parallel, and each experiment was independently performed thrice.
Desiccation tolerance test data are presented as the mean ± standard deviation (SD) of at least three trials, and the differences between two groups were evaluated in the independent samples by two-tailed Student’s t-test. A p-value < 0.05 was considered statistically significant. The asterisks indicate significant differences (*p < 0.05; **p < 0.01; ***p < 0.001).
The raw data of transcriptomes of LB cultured and desiccated C. sakazakii G4023 for this study can be found in the GenBank database. The accession number of this BioProject is PRJNA692549 (https://www.ncbi.nlm.nih.gov/bioproject/PRJNA692549).
CQ wrote the manuscript. CQ and MH designed and performed the experiments and were involved in the data analysis. YD, JS, and ZY contributed to the bioinformatic analysis. HM helped with the sample preparation for transcriptome and proteome. YW and CY validated the bioinformatic data. SZ helped with the validation of this manuscript. BL (10th author) and BL (11th author) conceptualized and supervised this study. All authors contributed to the article and approved the submitted version.
This work was supported by the National Natural Science Foundation of China (NSFC) Program (32070130, 81772148, 31820103002, 31770144, and 81871624), the Young Scholar of Tianjin (20JCJQJC00180), the Natural Science Foundation (Key Project) of Tianjin to BL (20JCZDJC00820), and the National Key Programs for Infectious Diseases of China (Nos. 2017ZX10303405 and 2017ZX10104002-001-006). The funders had no role in the study design, data collection and interpretation, or the decision to submit the work for publication.
The authors declare that the research was conducted in the absence of any commercial or financial relationships that could be construed as a potential conflict of interest.
All claims expressed in this article are solely those of the authors and do not necessarily represent those of their affiliated organizations, or those of the publisher, the editors and the reviewers. Any product that may be evaluated in this article, or claim that may be made by its manufacturer, is not guaranteed or endorsed by the publisher.
The Supplementary Material for this article can be found online at: https://www.frontiersin.org/articles/10.3389/fmicb.2021.779538/full#supplementary-material
Almajed, F. S., and Forsythe, S. J. (2016). Cronobacter sakazakii clinical isolates overcome host barriers and evade the immune response. Microb. Pathog. 90, 55–63. doi: 10.1016/j.micpath.2015.11.014
Ayala-Castro, C., Saini, A., and Outten, F. W. (2008). Fe-S cluster assembly pathways in bacteria. Microbiol. Mol. Biol. Rev. 72, 110–125. doi: 10.1128/MMBR.00034-07
Bai, H., Zhou, D., Zhang, X., Cao, Y., Xiao, X., Zhang, Y., et al. (2021). The responses of Salmonella enterica serovar Typhimurium to vanillin in apple juice through global transcriptomics. Int. J. Food Microbiol. 347:109189. doi: 10.1016/j.ijfoodmicro.2021.109189
Bayles, D. O., and Wilkinson, B. J. (2000). Osmoprotectants and cryoprotectants for Listeria monocytogenes. Lett. Appl. Microbiol. 30, 23–27. doi: 10.1046/j.1472-765x.2000.00646.x
Bennour Hennekinne, R., Guillier, L., Fazeuilh, L., Ells, T., Forsythe, S., Jackson, E., et al. (2018). Survival of Cronobacter in powdered infant formula and their variation in biofilm formation. Lett. Appl. Microbiol. 66, 496–505. doi: 10.1111/lam.12879
Berleman, J. E., and Bauer, C. E. (2005). A che-like signal transduction cascade involved in controlling flagella biosynthesis in Rhodospirillum centenum. Mol. Microbiol. 55, 1390–1402. doi: 10.1111/j.1365-2958.2005.04489.x
Buccitelli, C., and Selbach, M. (2020). mRNAs, proteins and the emerging principles of gene expression control. Nat. Rev. Genet. 21, 630–644. doi: 10.1038/s41576-020-0258-4
Caldara, M., Dupont, G., Leroy, F., Goldbeter, A., De Vuyst, L., and Cunin, R. (2008). Arginine biosynthesis in Escherichia coli: experimental perturbation and mathematical modeling. J. Biol. Chem. 283, 6347–6358. doi: 10.1074/jbc.M705884200
Cawthorn, D. M., Botha, S., and Witthuhn, R. C. (2008). Evaluation of different methods for the detection and identification of Enterobacter sakazakii isolated from South African infant formula milks and the processing environment. Int. J. Food Microbiol. 127, 129–138. doi: 10.1016/j.ijfoodmicro.2008.06.024
Chaibenjawong, P., and Foster, S. J. (2011). Desiccation tolerance in Staphylococcus aureus. Arch. Microbiol. 193, 125–135. doi: 10.1007/s00203-010-0653-x
Chan, W., Costantino, N., Li, R., Lee, S. C., Su, Q., Melvin, D., et al. (2007). A recombineering based approach for high-throughput conditional knockout targeting vector construction. Nucleic Acids Res. 35:e64. doi: 10.1093/nar/gkm163
Chauhan, R., Singh, N., Pal, G. K., and Goel, G. (2020). Trending biocontrol strategies against Cronobacter sakazakii: a recent updated review. Food Res. Int. 137:109385. doi: 10.1016/j.foodres.2020.109385
Chen, G., Gharib, T. G., Huang, C. C., Taylor, J. M., Misek, D. E., Kardia, S. L., et al. (2002). Discordant protein and mRNA expression in lung adenocarcinomas. Mol. Cell. Proteomics 1, 304–313. doi: 10.1074/mcp.m200008-mcp200
da Silva, P., Manieri, F. Z., Herrera, C. M., Trent, M. S., and Moreira, C. G. (2018). Novel role of VisP and the Wzz system during O-antigen assembly in Salmonella enterica serovar Typhimurium pathogenesis. Infect. Immun. 86:e00319-18. doi: 10.1128/iai.00319-18
Datta, S., Costantino, N., and Court, D. L. (2006). A set of recombineering plasmids for gram-negative bacteria. Gene 379, 109–115. doi: 10.1016/j.gene.2006.04.018
Du, X. J., Wang, X. Y., Dong, X., Li, P., and Wang, S. (2018). Characterization of the desiccation tolerance of Cronobacter sakazakii strains. Front. Microbiol. 9:2867. doi: 10.3389/fmicb.2018.02867
Farmer, J. J., Asbury, M., Hickman, F., and Brenner, D. J. Enterobacteriaceae Study Group (1980). Enterobacter sakazakii: a new species of “Enterobacteriaceae” isolated from clinical specimens. Int. J. Syst. Evol. Microbiol. 30, 569–584. doi: 10.1099/00207713-30-3-569
Fernández-Gómez, P., López, M., Prieto, M., González-Raurich, M., and Alvarez-Ordóñez, A. (2020). The role of the general stress response regulator RpoS in Cronobacter sakazakii biofilm formation. Food Res. Int. 136:109508. doi: 10.1016/j.foodres.2020.109508
Garmiri, P., Coles, K. E., Humphrey, T. J., and Cogan, T. A. (2008). Role of outer membrane lipopolysaccharides in the protection of Salmonella enterica serovar Typhimurium from desiccation damage. FEMS Microbiol. Lett. 281, 155–159. doi: 10.1111/j.1574-6968.2008.01093.x
Ghazalpour, A., Bennett, B., Petyuk, V. A., Orozco, L., Hagopian, R., Mungrue, I. N., et al. (2011). Comparative analysis of proteome and transcriptome variation in mouse. PLoS Genet. 7:e1001393. doi: 10.1371/journal.pgen.1001393
Gibson, D. L., White, A. P., Snyder, S. D., Martin, S., Heiss, C., Azadi, P., et al. (2006). Salmonella produces an O-antigen capsule regulated by AgfD and important for environmental persistence. J. Bacteriol. 188, 7722–7730. doi: 10.1128/JB.00809-06
Gronow, S., and Brade, H. (2001). Lipopolysaccharide biosynthesis: which steps do bacteria need to survive? J. Endotoxin Res. 7, 3–23.
Haider, S., and Pal, R. (2013). Integrated analysis of transcriptomic and proteomic data. Curr. Genom. 14, 91–110.
Han, C. L., Chien, C. W., Chen, W. C., Chen, Y. R., Wu, C. P., Li, H., et al. (2008). A multiplexed quantitative strategy for membrane proteomics: opportunities for mining therapeutic targets for autosomal dominant polycystic kidney disease. Mol. Cell Proteomics 7, 1983–1997. doi: 10.1074/mcp.M800068-MCP200
Hansen, L. T., and Vogel, B. F. (2011). Desiccation of adhering and biofilm Listeria monocytogenes on stainless steel: survival and transfer to salmon products. Int. J. Food Microbiol. 146, 88–93. doi: 10.1016/j.ijfoodmicro.2011.01.032
He, K., and Bauer, C. E. (2014). Chemosensory signaling systems that control bacterial survival. Trends Microbiol. 22, 389–398. doi: 10.1016/j.tim.2014.04.004
Helbig, A. O., Heck, A. J., and Slijper, M. (2010). Exploring the membrane proteome–challenges and analytical strategies. J. Proteomics 73, 868–878. doi: 10.1016/j.jprot.2010.01.005
Hu, S., Yu, Y., Wu, X., Xia, X., Xiao, X., and Wu, H. (2017). Comparative proteomic analysis of Cronobacter sakazakii by iTRAQ provides insights into response to desiccation. Food Res Int 100(Pt 1) 631–639. doi: 10.1016/j.foodres.2017.06.051
Hughes, K. T., and Mathee, K. (1998). The anti-sigma factors. Annu. Rev. Microbiol. 52, 231–286. doi: 10.1146/annurev.micro.52.1.231
Iversen, C., Lehner, A., Mullane, N., Bidlas, E., Cleenwerck, I., Marugg, J., et al. (2007). The taxonomy of Enterobacter sakazakii: proposal of a new genus Cronobacter gen. nov. and descriptions of Cronobacter sakazakii comb. nov. Cronobacter sakazakii subsp. sakazakii, comb. nov., Cronobacter sakazakii subsp. malonaticus subsp. nov., Cronobacter turicensis sp. nov., Cronobacter muytjensii sp. nov., Cronobacter dublinensis sp. nov. and Cronobacter genomospecies 1. BMC Evol. Biol. 7:64. doi: 10.1186/1471-2148-7-64
Iversen, C., Mullane, N., McCardell, B., Tall, B. D., Lehner, A., Fanning, S., et al. (2008). Cronobacter gen. nov., a new genus to accommodate the biogroups of Enterobacter sakazakii, and proposal of Cronobacter sakazakii gen. nov., comb. nov., Cronobacter malonaticus sp. nov., Cronobacter turicensis sp. nov., Cronobacter muytjensii sp. nov., Cronobacter dublinensis sp. nov., Cronobacter genomospecies 1, and of three subspecies, Cronobacter dublinensis subsp. dublinensis subsp. nov., Cronobacter dublinensis subsp. lausannensis subsp. nov. and Cronobacter dublinensis subsp. lactaridi subsp. nov. Int. J. Syst. Evol. Microbiol. 58(Pt 6) 1442–1447. doi: 10.1099/ijs.0.65577-0
Joseph, S., Cetinkaya, E., Drahovska, H., Levican, A., Figueras, M. J., and Forsythe, S. J. (2012). Cronobacter condimenti sp. nov., isolated from spiced meat, and Cronobacter universalis sp. nov., a species designation for Cronobacter sp. genomospecies 1, recovered from a leg infection, water and food ingredients. Int. J. Syst. Evol. Microbiol. 62(Pt 6) 1277–1283. doi: 10.1099/ijs.0.032292-0
Kapatral, V., Olson, J. W., Pepe, J. C., Miller, V. L., and Minnich, S. A. (1996). Temperature-dependent regulation of Yersinia enterocolitica class III flagellar genes. Mol. Microbiol. 19, 1061–1071. doi: 10.1046/j.1365-2958.1996.452978.x
Kehry, M. R., Doak, T. G., and Dahlquist, F. W. (1984). Stimulus-induced changes in methylesterase activity during chemotaxis in Escherichia coli. J. Biol. Chem. 259, 11828–11835.
Kintz, E. N., and Goldberg, J. B. (2011). Site-directed mutagenesis reveals key residue for O antigen chain length regulation and protein stability in Pseudomonas aeruginosa Wzz2. J. Biol. Chem. 286, 44277–44284. doi: 10.1074/jbc.M111.273979
Kutsukake, K., and Iino, T. (1994). Role of the FliA-FlgM regulatory system on the transcriptional control of the flagellar regulon and flagellar formation in Salmonella typhimurium. J. Bacteriol. 176, 3598–3605. doi: 10.1128/jb.176.12.3598-3605.1994
Lai, Y., Zhang, D., Wang, J., Wang, J., Ren, P., Yao, L., et al. (2020). Integrative transcriptomic and proteomic analyses of molecular mechanism responding to salt stress during seed germination in hulless barley. Int. J. Mol. Sci. 21:359. doi: 10.3390/ijms21010359
Li, H., Bhaskara, A., Megalis, C., and Tortorello, M. L. (2012). Transcriptomic analysis of Salmonella desiccation resistance. Foodborne Pathog. Dis. 9, 1143–1151. doi: 10.1089/fpd.2012.1254
Liu, B., Furevi, A., Perepelov, A. V., Guo, X., Cao, H., Wang, Q., et al. (2019). Structure and genetics of Escherichia coli O antigens. FEMS Microbiol. Rev. 44, 655–683. doi: 10.1093/femsre/fuz028
MacKay, V. L., Li, X., Flory, M. R., Turcott, E., Law, G. L., Serikawa, K. A., et al. (2004). Gene expression analyzed by high-resolution state array analysis and quantitative proteomics: response of yeast to mating pheromone. Mol. Cell. Proteomics 3, 478–489. doi: 10.1074/mcp.M300129-MCP200
Maier, T., Guell, M., and Serrano, L. (2009). Correlation of mRNA and protein in complex biological samples. FEBS Lett. 583, 3966–3973. doi: 10.1016/j.febslet.2009.10.036
Muok, A. R., Briegel, A., and Crane, B. R. (2020). Regulation of the chemotaxis histidine kinase CheA: a structural perspective. Biochim. Biophys. Acta Biomembr. 1862:183030. doi: 10.1016/j.bbamem.2019.183030
Murray, G. L., Attridge, S. R., and Morona, R. (2003). Regulation of Salmonella typhimurium lipopolysaccharide O antigen chain length is required for virulence; identification of FepE as a second Wzz. Mol. Microbiol. 47, 1395–1406. doi: 10.1046/j.1365-2958.2003.03383.x
Njoroge, J. W., Nguyen, Y., Curtis, M. M., Moreira, C. G., and Sperandio, V. (2012). Virulence meets metabolism: Cra and KdpE gene regulation in enterohemorrhagic Escherichia coli. mBio 3:e00280-12. doi: 10.1128/mBio.00280-12
Okuda, S., Sherman, D. J., Silhavy, T. J., Ruiz, N., and Kahne, D. (2016). Lipopolysaccharide transport and assembly at the outer membrane: the PEZ model. Nat. Rev. Microbiol. 14, 337–345. doi: 10.1038/nrmicro.2016.25
Ondrej, M., Rehulka, P., Rehulkova, H., Kupcik, R., and Tichy, A. (2020). Fractionation of enriched phosphopeptides using pH/acetonitrile-gradient-reversed-phase microcolumn separation in combination with LC-MS/MS analysis. Int. J. Mol. Sci. 21:3971. doi: 10.3390/ijms21113971
Osaili, T., and Forsythe, S. (2009). Desiccation resistance and persistence of Cronobacter species in infant formula. Int. J. Food Microbiol. 136, 214–220. doi: 10.1016/j.ijfoodmicro.2009.08.006
Ozenberger, B. A., Nahlik, M. S., and McIntosh, M. A. (1987). Genetic organization of multiple fep genes encoding ferric enterobactin transport functions in Escherichia coli. J. Bacteriol. 169, 3638–3646. doi: 10.1128/jb.169.8.3638-3646.1987
Parra-Flores, J., Aguirre, J., Juneja, V., Jackson, E. E., Cruz-Córdova, A., Silva-Sanchez, J., et al. (2018). Virulence and antibiotic resistance profiles of Cronobacter sakazakii and Enterobacter spp. Involved in the diarrheic hemorrhagic outbreak in Mexico. Front. Microbiol. 9:2206. doi: 10.3389/fmicb.2018.02206
Parra-Flores, J., Maury-Sintjago, E., Rodriguez-Fernández, A., Acuña, S., Cerda, F., Aguirre, J., et al. (2020). Microbiological quality of powdered infant formula in Latin America. J. Food Prot. 83, 534–541. doi: 10.4315/0362-028x.jfp-19-399
Richter, M., Rosselló-Móra, R., Oliver Glöckner, F., and Peplies, J. (2016). JSpeciesWS: a web server for prokaryotic species circumscription based on pairwise genome comparison. Bioinformatics 32, 929–931. doi: 10.1093/bioinformatics/btv681
Roche, B., Aussel, L., Ezraty, B., Mandin, P., Py, B., and Barras, F. (2013). Iron/sulfur proteins biogenesis in prokaryotes: formation, regulation and diversity. Biochim. Biophys. Acta 1827, 455–469. doi: 10.1016/j.bbabio.2012.12.010
Romilly, C., Hoekzema, M., Holmqvist, E., and Wagner, E. G. H. (2020). Small RNAs OmrA and OmrB promote class III flagellar gene expression by inhibiting the synthesis of anti-Sigma factor FlgM. RNA Biol. 17, 872–880. doi: 10.1080/15476286.2020.1733801
Sandberg, A., Branca, R. M., Lehtio, J., and Forshed, J. (2014). Quantitative accuracy in mass spectrometry based proteomics of complex samples: the impact of labeling and precursor interference. J. Proteomics 96, 133–144. doi: 10.1016/j.jprot.2013.10.035
Schmidt, H., and Hensel, M. (2004). Pathogenicity islands in bacterial pathogenesis. Clin. Microbiol. Rev. 17, 14–56. doi: 10.1128/cmr.17.1.14-56.2004
Shaker, R. R., Osaili, T. M., Abu Al-Hasan, A. S., Ayyash, M. M., and Forsythe, S. J. (2008). Effect of desiccation, starvation, heat, and cold stresses on the thermal resistance of Enterobacter sakazakii in rehydrated infant milk formula. J. Food Sci. 73, M354–M359. doi: 10.1111/j.1750-3841.2008.00880.x
Srikumar, S., Cao, Y., Yan, Q., Van Hoorde, K., Nguyen, S., Cooney, S., et al. (2019). RNA sequencing-based transcriptional overview of xerotolerance in Cronobacter sakazakii SP291. Appl. Environ. Microbiol. 85:e01993-18. doi: 10.1128/aem.01993-18
Stephens, B. B., Loar, S. N., and Alexandre, G. (2006). Role of CheB and CheR in the complex chemotactic and aerotactic pathway of Azospirillum brasilense. J. Bacteriol. 188, 4759–4768. doi: 10.1128/JB.00267-06
Sun, Y., Wang, M., Liu, H., Wang, J., He, X., Zeng, J., et al. (2011). Development of an O-antigen serotyping scheme for Cronobacter sakazakii. Appl. Environ. Microbiol. 77, 2209–2214. doi: 10.1128/aem.02229-10
Toker, A. S., and Macnab, R. M. (1997). Distinct regions of bacterial flagellar switch protein FliM interact with FliG, FliN and CheY. J. Mol. Biol. 273, 623–634. doi: 10.1006/jmbi.1997.1335
Trinh, H. V., Grossmann, J., Gehrig, P., Roschitzki, B., Schlapbach, R., Greber, U. F., et al. (2013). iTRAQ-based and label-free proteomics approaches for studies of human adenovirus infections. Int. J. Proteomics 2013:581862. doi: 10.1155/2013/581862
Vanderlinde, E. M., Harrison, J. J., Muszynski, A., Carlson, R. W., Turner, R. J., and Yost, C. K. (2010). Identification of a novel ABC transporter required for desiccation tolerance, and biofilm formation in Rhizobium leguminosarum bv. viciae 3841. FEMS Microbiol. Ecol. 71, 327–340. doi: 10.1111/j.1574-6941.2009.00824.x
Vyas, S., and Maas, W. K. (1963). Feedback inhibition of acetylglutamate synthetase by arginine in Escherichia coli. Arch. Biochem. Biophys. 100, 542–546. doi: 10.1016/0003-9861(63)90124-3
Wang, H., Alvarez, S., and Hicks, L. M. (2012). Comprehensive comparison of iTRAQ and label-free LC-based quantitative proteomics approaches using two Chlamydomonas reinhardtii strains of interest for biofuels engineering. J. Proteome Res. 11, 487–501. doi: 10.1021/pr2008225
Wang, L., Hu, X., Tao, G., and Wang, X. (2012). Outer membrane defect and stronger biofilm formation caused by inactivation of a gene encoding for heptosyltransferase I in Cronobacter sakazakii ATCC BAA-894. J. Appl. Microbiol. 112, 985–997. doi: 10.1111/j.1365-2672.2012.05263.x
Wang, M., Cao, B., Gao, Q., Sun, Y., Liu, P., Feng, L., et al. (2009). Detection of Enterobacter sakazakii and other pathogens associated with infant formula powder by use of a DNA microarray. J. Clin. Microbiol. 47, 3178–3184. doi: 10.1128/jcm.00366-09
Wang, X., Liang, H., Guo, D., Guo, L., Duan, X., Jia, Q., et al. (2019). Integrated analysis of transcriptomic and proteomic data from tree peony (P. ostii) seeds reveals key developmental stages and candidate genes related to oil biosynthesis and fatty acid metabolism. Hortic. Res. 6:111. doi: 10.1038/s41438-019-0194-7
Wang, M., Wang, L., Wu, P., Chen, T., Zhu, Y., Zhang, Y., et al. (2019). Genomics and experimental analysis reveal a novel factor contributing to the virulence of Cronobacter sakazakii strains associated with neonate infection. J. Infect. Dis. 220, 306–315. doi: 10.1093/infdis/jiz098
White, A. P., Gibson, D. L., Kim, W., Kay, W. W., and Surette, M. G. (2006). Thin aggregative fimbriae and cellulose enhance long-term survival and persistence of Salmonella. J. Bacteriol. 188, 3219–3227. doi: 10.1128/JB.188.9.3219-3227.2006
Wuichet, K., and Zhulin, I. B. (2010). Origins and diversification of a complex signal transduction system in prokaryotes. Sci. Signal. 3:ra50. doi: 10.1126/scisignal.2000724
Yan, Q. Q., Condell, O., Power, K., Butler, F., Tall, B. D., and Fanning, S. (2012). Cronobacter species (formerly known as Enterobacter sakazakii) in powdered infant formula: a review of our current understanding of the biology of this bacterium. J. Appl. Microbiol. 113, 1–15. doi: 10.1111/j.1365-2672.2012.05281.x
Yang, J., Bitoun, J. P., and Ding, H. (2006). Interplay of IscA and IscU in biogenesis of iron-sulfur clusters. J. Biol. Chem. 281, 27956–27963. doi: 10.1074/jbc.M601356200
Keywords: Cronobacter sakazakii, desiccation, transcriptome, proteome, O-antigen chain length, chemotaxis
Citation: Qian C, Huang M, Du Y, Song J, Mu H, Wei Y, Zhang S, Yin Z, Yuan C, Liu B and Liu B (2022) Chemotaxis and Shorter O-Antigen Chain Length Contribute to the Strong Desiccation Tolerance of a Food-Isolated Cronobacter sakazakii Strain. Front. Microbiol. 12:779538. doi: 10.3389/fmicb.2021.779538
Received: 19 September 2021; Accepted: 18 November 2021;
Published: 04 January 2022.
Edited by:
Nuno Pereira Mira, University of Lisbon, PortugalReviewed by:
Xin-jun Du, Tianjin University of Science and Technology, ChinaCopyright © 2022 Qian, Huang, Du, Song, Mu, Wei, Zhang, Yin, Yuan, Liu and Liu. This is an open-access article distributed under the terms of the Creative Commons Attribution License (CC BY). The use, distribution or reproduction in other forums is permitted, provided the original author(s) and the copyright owner(s) are credited and that the original publication in this journal is cited, in accordance with accepted academic practice. No use, distribution or reproduction is permitted which does not comply with these terms.
*Correspondence: Bin Liu (10th author), bGl1YmlubmtAbmFua2FpLmVkdS5jbg==; Bin Liu (11th author), bGl1YmluMTk4MUBuYW5rYWkuZWR1LmNu
†These authors have contributed equally to this work and share first authorship
Disclaimer: All claims expressed in this article are solely those of the authors and do not necessarily represent those of their affiliated organizations, or those of the publisher, the editors and the reviewers. Any product that may be evaluated in this article or claim that may be made by its manufacturer is not guaranteed or endorsed by the publisher.
Research integrity at Frontiers
Learn more about the work of our research integrity team to safeguard the quality of each article we publish.