- 1College of Life Sciences, Sichuan Agricultural University, Ya’an, China
- 2Sichuan Agricultural University Hospital, Ya’an, China
- 3College of Food Science, Sichuan Agricultural University, Ya’an, China
- 4Sichuan Food Fermentation Industry Research and Design Institute, Chengdu, China
The objective of this study was to evaluate the diversity of endophytic fungi of different parts of Ligusticum chuanxiong Hort (CX) and further characterize their biological activities and identify chemical compounds produced by these endophytic fungi. A total of 21 endophytic fungi were isolated and identified from CX. Penicillium oxalicum, Simplicillium sp., and Colletotrichum sp. were identified as promising strains by the color reaction. Comparing different organic extracts of the three strains, it was observed that the ethyl acetate extract of Penicillium oxalicum and Simplicillium sp. and the n-butanol extract of Colletotrichum sp. showed significant antioxidant and antibacterial activities. The ethyl acetate extracts of Penicillium oxalicum had outstanding antioxidant and antibacterial effects, and its radical scavenging rates for ABTS and DPPH were 98.43 ± 0.006% and 90.11 ± 0.032%, respectively. At the same time, their IC50 values were only 0.18 ± 0.02 mg/mL and 0.04 ± 0.003 mg/mL. The ethyl acetate extract of Penicillium oxalicum showed MIC value of only 0.5 mg/mL against Escherichia coli and Staphylococcus aureus. By liquid chromatography-mass spectrometry (LC-MS), we found that Penicillium oxalicum could produce many high-value polyphenols, such as hesperidin (36.06 μmol/g), ferulic acid (1.17 μmol/g), and alternariol (12.64 μmol/g), which can be a potential resource for the pharmaceutical industry. In conclusion, these results increase the diversity of CX endophytic fungi and the antioxidant and antibacterial activities of their secondary metabolites.
Introduction
The medicinal plant Ligusticum chuanxiong Hort (CX) belongs to the Umbelliferae family and is commonly cultivated in Sichuan Province, China. It has been used in the treatment of gynecological diseases, headache, rheumatism arthralgia, and hemiplegia (Chen et al., 2018). The major chemical constituents of CX are phthalides, terpenes, polysaccharides, alkaloids, and organic acids (Ran et al., 2011). Moreover, the young leaf and stem of CX are widely used as edible food materials in tossed salad and fried cuisines (Yuan et al., 2020a).
Endophytic fungi are a valuable resource for the detection of new natural products and have been widely used for medicinal purposes (Tanvir et al., 2017). Endophytic fungi that live with plant tissues without causing harm to hosts can produce different secondary metabolites including alkaloids, steroids, terpenoids, flavonoids, aliphatic compounds, and phenols (Aly et al., 2011). These products have various biological activities, such as antioxidant, anti-diabetic (Sharma and Sharma, 2016), anti-obesity (Noinart et al., 2017), and antimicrobial activities (Padhi et al., 2020). Moreover, plant endophytic fungi have a high species diversity, which is strongly associated with environmental variations and the taxonomy of host plants (Wu et al., 2013). The diversity of endophytic fungi will determine the production of diverse secondary metabolites promoted by endophytic fungi, which can be used as drugs for medical treatment (Jia et al., 2016). Furthermore, when the endophytic fungi was isolated in vitro and cultivated in suitable conditions, the number of fungal cells and the production of secondary metabolites would be multiplied far beyond their normal concentration in plants (Wu et al., 2018). Therefore, plant endophytic fungi are not only a source for the discovery of biologically active substances, but also a reactor for the production of drugs more easily and economically.
Currently, oxidative stress is gaining more attention. Reactive oxygen species (ROS) are continuously produced as a by-product of normal basal metabolism in humans (Valko et al., 2006). ROS is essential and beneficial to living systems, and plays a role as signaling molecules in the regulation of many biological processes (Apel and Hirt, 2004). However, oxidative stress is the result of excess unneutralized active substances in the body, which can damage cellular lipids, proteins, cells, tissues, and organs, and inhibit the normal function of DNA (Valko et al., 2007; Desai et al., 2009), and it has been reported as one of the important factors in several types of diseases, such as diabetes and even some types of cancer (Marseglia et al., 2015; Zimta et al., 2019). Although many potent antioxidants have been artificially synthesized, such as BHA and BHT, they are not safe as some natural antioxidants (Kahl and Kappus, 1993). Thus, there is a need to find more natural antioxidants to meet the demands of the food and pharmaceutical industries.
In addition, the quest for new antimicrobial drugs has always been crucial throughout human history. Bacterial infections are the result of pathogenic bacteria entering and multiplying in the human body; these are a major deleterious factor in some infections (Anderson and Kendall, 2017; Martin and Bachman, 2018). For example, the gram-positive bacterium Staphylococcus aureus is a human pathogen (Kimmig et al., 2021) that is constantly a source of skin infections, lung infections, gastroenteritis, and urinary tract infections (Thomer et al., 2016; Del Giudice, 2020). Over the years, researchers have observed that antibacterial substances can be isolated from endophytic fungi of various medicinal plants (Pinheiro et al., 2017; Nischitha and Shivanna, 2021). Hence, it is possible to discover new and effective antibacterial substances from endophytic fungi.
Medicinal plants and their endophytes are important resources for the discovery and acquisition of natural products (Huang et al., 2007). The antioxidant (Ge et al., 2018) and antimicrobial (Sim and Shin, 2008) activities of CX have been described using different pharmacological models, and CX is also well documented for production of various bioactive metabolites (Yuan et al., 2020b; Zhang et al., 2020). However, there are few studies on endophytic fungi of CX at present, and endophytic fungi resources should be further developed and utilized. In this context, the present study aimed to identify the diversity of endophytic fungi in CX and the antioxidant and antibacterial activities as well as the chemical compounds of endophytic fungus extracts.
Materials and Methods
Experimental Materials
Seedlings of CX were collected from the farm of Sichuan Agricultural University (Ya’an City, Sichuan Province, China) in October 2020 and the lingzi (nodes of the lower part of the stems) were obtained from the local market in Ya’an. The plants were identified by Dr. Hui Chen. Voucher specimens were deposited in the Herbarium “Fermentation Engineering Laboratory” with the identification numbers FEL125 and EFL126. All samples were placed in the boxes and immediately sent to the laboratory for study.
Isolation of Endophytic Fungi
The lingzi and seedlings of CX were washed thoroughly with water, and the roots, stems, and leaves systems were separated from the plant. The collected samples were cut into small 5 mm cubes and soaked in 70% ethanol for 1–2 min, followed by 5% sodium hypochlorite for 2–5 min, and then in 70% ethanol for 0.5–1 min (adapted from Zhao et al., 2012). In addition, the samples were dabbed with sterile filter paper. The surface-sterilized explant fragments were placed in Petri dishes containing PDA which was amended with ampicillin and kanamycin sulfate at a concentration of 50 μg/L to prevent bacterial contamination. The fungal strains in PDA plates were incubated at 28°C for 7–10 days and purified promising fungi were stored in the Laboratory of Molecular Biology and Biochemistry, College of Life Sciences, Sichuan Agricultural University.
Identification and Phylogenetic Analysis of the Endophytic Fungi
The endophytic fungi were identified by their morphology and DNA sequence data. Cellular morphological features including their mycelium color, pigmentation, and spore morphology, were described and investigated using a CX21 FS1 microscope (Olympus, Tokyo, Japan). Genomic DNA was isolated using the CTAB method and the ITS region of the fungal genome was amplified by PCR using the following primers: ITS1-forward primer (TCCGTAGGTGAACCTGCGG) and ITS4-reverse primer (TCCTCCGCTTATTGATATGC). The reaction mixture and amplification conditions were the same as previously described (Shah et al., 2019). The PCR products were directly sequenced by the TSINGKE Biological Technology Corporation (Shandong, China). The sequences of the ITS regions were compared with the sequences of existing species sequence in GenBank using BLASTN software. A phylogenetic tree was constructed by the neighbor joining method using MEGA 7.0 software.
Screening of Polyphenol-Producing Endophytic Fungi
Endophytic fungi were inoculated into fresh PDB and were allowed to grow for 7 days (160 rpm/min and 28°C). The culture was filtered through four layers of cheesecloth to obtain culture filtrate. Polyphenol-producing endophytic fungi were preliminarily screened by the color reaction. In brief, fermentation broth was mixed with chromogenic reagent (0.1% FeCl3:0.1% K3[Fe(CN)6] = 1:1) in a tube. If the color turns blue, it indicates that the fermentation broth contains polyphenols.
Preparation of Extracts of Fermentation Broth of Endophytic Fungi
Expanded fermentation of polyphenol-producing endophytic fungi was carried out in the same culture conditions as in Section “Screening of Polyphenol-Producing Endophytic Fungi.” The culture filtrate was extracted three times with ethyl acetate, n-butanol, petroleum ether, and chloroform (2 × 200 mL) in a separatory funnel, respectively. The crude extracts were concentrated using a lyophilize under a vacuum at –40°C and dissolved in dimethyl sulfoxide (DMSO) to evaluate their biological activities.
Determination of Total Phenolic Content
Total Phenolic Content (TPC) of the fungal extracts was determined using Folin–Ciocalteu (FC) assay as described by Minussi et al. (2003). In brief, after mixing deionized water (1 mL) and extract solution (1 mL) in a test tube, FC reagent (0.5 mL) was added to the mixture and allowed to react for 4 min. There was 20% Na2CO3 (1 mL) added thereafter and the mixture was diluted to 10 mL with distilled water. The color was developed in 2 h, and the absorbance was determined at 760 nm (A760) using a multiskan sky (Thermos Scientific, Singapore). TPC were expressed in mg equivalent in gallic acid and obtained from the regression equation: y = 0.0064x + 0.063 with R2 = 0.9979. All analyses were performed in triplicate.
Antioxidant Activity
The above-mentioned fungi extracts were adjusted to six concentrations (0.2, 0.4, 0.6, 0.8, 1.0, and 3 mg/mL) for four antioxidant activity assays [2,2-diphenyl-1-picrylhydrazyl (DPPH) radical scavenging, 2,20-azino-bis radical (ABTS+⋅) scavenging, hydroxyl radical (⋅OH) scavenging, and superoxide anion radical (⋅O2–) scavenging]. Ascorbic acid (Vc) was used as the positive control.
2,2-Diphenyl-1-Picrylhydrazyl Radical Scavenging Activity Assay
The DPPH assay was carried out using the method of Nuerxiati et al. (2019). Briefly, in each well of a 96-well microplate, test samples of different concentrations (100 μL) were mixed with DPPH solution (100 μL, 0.2 mM) in the dark for 30 min at room temperature. The absorbance of each well was recorded at 517 nm (A517). The DPPH radical scavenging activity (scavenging rate) was calculated by
where Amax is the absorbance of 100 μL of DPPH solution in 100 μL of anhydrous ethanol at 517 nm; A517 is the absorbance of 100 μL of DPPH solution mixed with 100 μL of gradient sample solution; A0 is the absorbance of 100 μL of gradient sample solution in 100 μL of anhydrous ethanol.
Hydroxyl Radical Scavenging Activity Assay
The hydroxyl radical scavenging activity of the crude extracts was measured according to Smirnoff and Cumbes (1989) with some modifications. Different concentrations of the tested sample (50 μL) were added into an enzyme label plate containing a reaction solution of 50 μL of 6.0 mM FeSO4, 50 μL of 6.0 mM salicylic acid-ethanol, and 50 μL of 0.1% H2O2. The reaction solution was cultured in a 37°C thermostat for 30 min. The absorbance of the mixture was read at 510 nm (A510). The hydroxyl radical scavenging rate was calculated using the following formula:
where A510 is the absorbance of a reaction solution containing the sample solution and H2O2 at 510 nm; Amax is the absorbance of a blank solution with anhydrous ethanol instead of the sample solution; A0 is the absorbance of a solution with distilled water instead of H2O2.
Superoxide Anion Radical Scavenging Assay
The scavenging activity of superoxide anion radical was estimated by pyrogallol autoxidation as described by Wang et al. (2009) with some modifications. Briefly, 0.05 mol/L Tris-HCl buffer (pH 8.2, 1.5 mL) and test sample with different concentration (0.5 mL) were mixed and incubated in a water bath at 25°C for 25 min. Afterward, 25 mM pyrogallol (200 μL) at 25°C was added. After 4 min of reaction, 8 mol/L HCl (0.25 mL) was added to terminate the reaction. The absorbance of the sample was measured at 325 nm (A325). The scavenging activity of superoxide anion radical scavenging rate was measured using the following equation:
where A325 is the absorbance of a reaction solution containing the sample solution and pyrogallol at 325 nm; Amax is the absorbance of a blank solution with distilled water; A0 is the absorbance of a solution with distilled water instead of pyrogallol.
ABTS Radical Scavenging Activity Assay
ABTS assay of all samples was assessed as the method described by Zhao et al. (2005). The ABTS solution was formed by mixing 7 mM ABTS (0.5 mL) with 140 mM K2S2O8 (88 μL), and the mixture was kept at room temperature for 12 h. The ABTS radical solution was diluted with distilled water and absorbance was adjusted to 0.70 ± 0.02 at 734 nm. Then, different concentrations of extracts (150 μL) were mixed with 50 μL of ABTS solution in each well of a 96-well microplate for 6 min. The absorbance of the reaction mixture was read at 734 nm (A734). The scavenging activity of ABTS radical was calculated as:
where Amax is the absorbance of ABTS solution mixed with anhydrous ethanol at 734 nm; A734 is the absorbance of ABTS solution mixed with the sample; A0 is the absorbance of the sample mixed with anhydrous ethanol.
Antibacterial Activity
Determination of the Minimum Inhibitory Concentration
To evaluate the Minimum Inhibitory Concentration (MIC) (Molla et al., 2016), two gram-negative bacteria (Escherichia coli: ATCC25922 and Pseudomonas aeruginosa: ATCC9027) and two gram-positive bacteria (Bacillus subtilis: ATCC6633 and Staphylococcus aureus: ATCC6538) were used. Initially, the bacterial strains were inoculated on a sterile nutrient agar plate and incubated at 37°C for 24 h. Subsequently, the fungal extracts (0.2–4 mg/mL) were diluted in nutrient broth using the serial micro-dilution method. Bacterium (10 μL) was inoculated in EP tubes with different concentration samples. There was 0.5% DMSO used as a negative control and chloramphenicol (50 μg/L) as a positive control. Then, all EP tubes were incubated at 37°C for 24 h. To determine the MIC, 0.1 mg/ml MTT (10 μL) was used. Any color change observed from mauve to purple was considered negative for bacterial growth. The lowest concentration of endophytic fungi extracts with no color change occurred was recorded as the MIC value. All the experiments were performed in triplicate for each bacterium.
Determination of Minimum Bactericidal Concentration
To evaluate the Minimum Bactericidal Concentration (MBC) (da Rocha et al., 2020), 10 μL positive wells of bacteria were inoculated on nutrient agar plates at 37°C for 24 h. Then the colonies on the plate were counted, and the result with growth less than 10 colonies was considered to have a bactericidal effect.
Fluorescence Microscopy
Biofilm was visualized using fluorescence microscopy according to Jardak et al. (2017). E. coli, P. aeruginosa, and S. aureus cells were treated with fungal extracts at a concentration of 2MIC. Biofilms were grown on glass slides at 37°C for 48 h. Then, the glass slides were gently washed with physiological saline 2–3 times in order to remove non-adherent bacteria. Then, acridine orange (0.1%, w/v, dissolved in PBS 1X) was used to stain biofilms formed in glass slides. Biofilms were observed under a BX53 fluorescent microscope (Olympus, Tokyo, Japan).
Detection of Bioactive Compounds by Liquid Chromatography-Mass Spectrometry Analysis
Extracts of endophytic fungi were analyzed by UPLC-HRMS (Waters, UPLC; Thermo, Q Exactive). The separation of compounds was achieved on an ACQUITY UPLC HSS T3 column (2.1 × 100 mm i.d) with bead size of 1.8 μm. The mobile phase was 0.05% formic acid in water (A)/acetonitrile (B) with a flow rate of 0.3 mL min–1. The following gradient elution program was used: 0–1 min, 5% B; 5–95% B, 2–13:50 min; 95–5% B, 13:50–16 min. For mass spectrometry (MS) detection, ionization was performed in ESI+ mode (Heater Temp 300°C; Sheath Gas Flow rate, 45arb; Aux Gas Flow Rate, 15arb; Sweep Gas Flow Rate, 1arb; spray voltage, 3.0KV; Capillary Temp, 350°C; S-Lens RF Level, 30%.) and ESI- mode (Heater Temp 300°C, Sheath Gas Flow rate,45arb; Aux Gas Flow Rate, 15arb; Sweep Gas Flow Rate, 1arb; spray voltage, 3.2KV; Capillary Temp, 350°C; S-Lens RF Level, 60%.). Full Scan (MS1) was performed from 70∼1050 m/z with a resolution of 70,000 and data dependent two-stage mass spectrometry (DD-MS2, TOPN = 10) with a resolution of 17,500. The obtained mass spectra were analyzed with Compound Discoverer 2.0 (Thermo Scientific) and the integrated mzcloud, metlin, and hmdb database were used to detect secondary metabolites in an untargeted method.
Data Analysis
All experimental data are expressed as the mean ± SD from three independent observations. The data were analyzed by one-way analysis of variance (ANOVA) followed by Duncan’s multiple range test using the SPSS24.0 software. P < 0.05 was used to define statistically significant difference between the control group and the experimental group.
Results
Isolation and Identification of Endophytic Fungi
A total of 21 strains of endophytic fungi were isolated from different parts of CX for the first time. There were 8, 2, 5, and 6 isolates obtained from lingzi, root, leaf, and stem of CX, respectively (Table 1). All these strains were preliminarily identified by their colony morphology and microscopic examination (Supplementary Figure 1 and Supplementary Table 1). Further, their molecular identification was carried out by ITS-based rDNA sequence analysis and the closest identified matching strains were listed in Table 2. All nucleotide sequences of these isolates were no less than 99% similarity to the closest matches in the nucleotide database, except for strain ZZ2, which showed only 90.14% identity with the sequence KM268690.1 retrieved from the NCBI GenBank database (Table 2). The most common genera were Fusarium sp. and Alternaria sp. (seven isolates and four isolates, respectively) (Table 2). The phylogenetic tree of the identified endophytic fungi from CX is shown in Figure 1. The 21 isolates were assigned into 11 different genus (Figure 1), including Rigidoporus sp., Aspergillus sp., Simplilium sp., Cladosporium sp., Alternaria sp., Fusarium sp., Penicillium sp., Colletotrichum sp., Nigrospora sp., Sordariomycetes sp., and Aporospora sp., showing the rich biodiversity of endophytic fungi from CX. Finally, ZZ2 was characterized as Rigidoporus sp. at the genus level based on the observation of colony morphology and phylogenetic analysis (Supplementary Figure 1, Supplementary Table 1, and Figure 1). In addition, since Colletotrichum is a very complex genus and need for identification of it at the species level by further genomics analyses (Gupta et al., 2019), strains YMY6 and YMJ13 also were preliminarily ascribed to Colletotrichum sp. at the genus level.
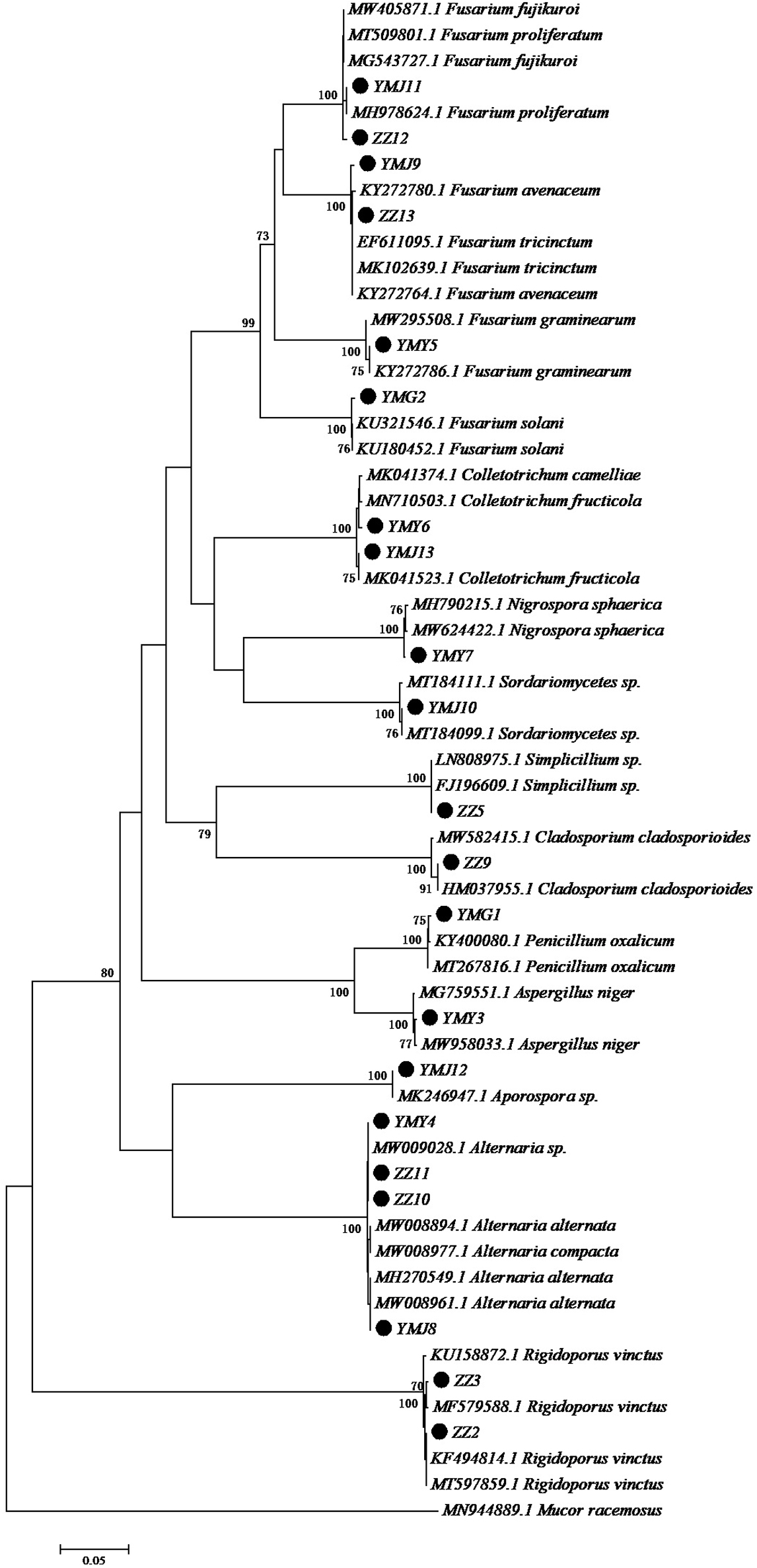
Figure 1. Neighbor-joining tree of the ITS sequences of the endophytic fungi associated with CX. Numbers at nodes are bootstrap scores obtained from 1000 replications. Mucor racemosus was used as an out group.
Screening of Polyphenol-Producing Endophytic Fungi
Polyphenol-producing strains were screened by color reaction, as shown in Supplementary Figure 2. The fermentation broth containing polyphenols reacted a blue reaction in FeCl3- K3[Fe(CN)6] solution. The color reaction of Penicillium oxalicum (YMG1), Simplilium sp. (ZZ5), and Colletotrichum sp. (YMJ13) were blue, indicating that these strains had the potential to produce polyphenols, and were further selected for chemical and pharmacological studies.
Determination of Total Phenolic Content
Extraction of Penicillium oxalicum (P. oxalicum), Simplilium sp., and Colletotrichum sp. using different solvents allowed the collection of mixture with different total phenolic contents (Table 3). The TPC of the fungal extracts ranged between 12.29 ± 3.24 and 94.58 ± 2.70 mg GAE/g with P. oxalicum possessing higher polyphenol content than Simplilium sp. and Colletotrichum sp. For P. oxalicum and Simplicillium sp., ethyl acetate was the most effective solvent for polyphenol extraction, and the recovered polyphenols are 94.58 ± 2.70 and 58.64 ± 3.61 mg GAE/g, respectively. As for Colletotrichum sp., n-butanol was the effective solvent for the extraction of polyphenols, with 58.96 ± 4.10 GAE/g polyphenols. However, the petroleum ether extract of P. oxalicum, Simplicillium sp., and Colletotrichum sp. contained considerably less polyphenols with 21.67 ± 5.91, 12.29 ± 3.24, and 26.14 ± 0.32 mg GAE/g, respectively. Therefore, for Colletotrichum sp., polar solvents allowed the recovery of more polyphenol. For P. oxalicum and Simplicillium sp., the medium polarity solvents were more suitable for polyphenol extraction.
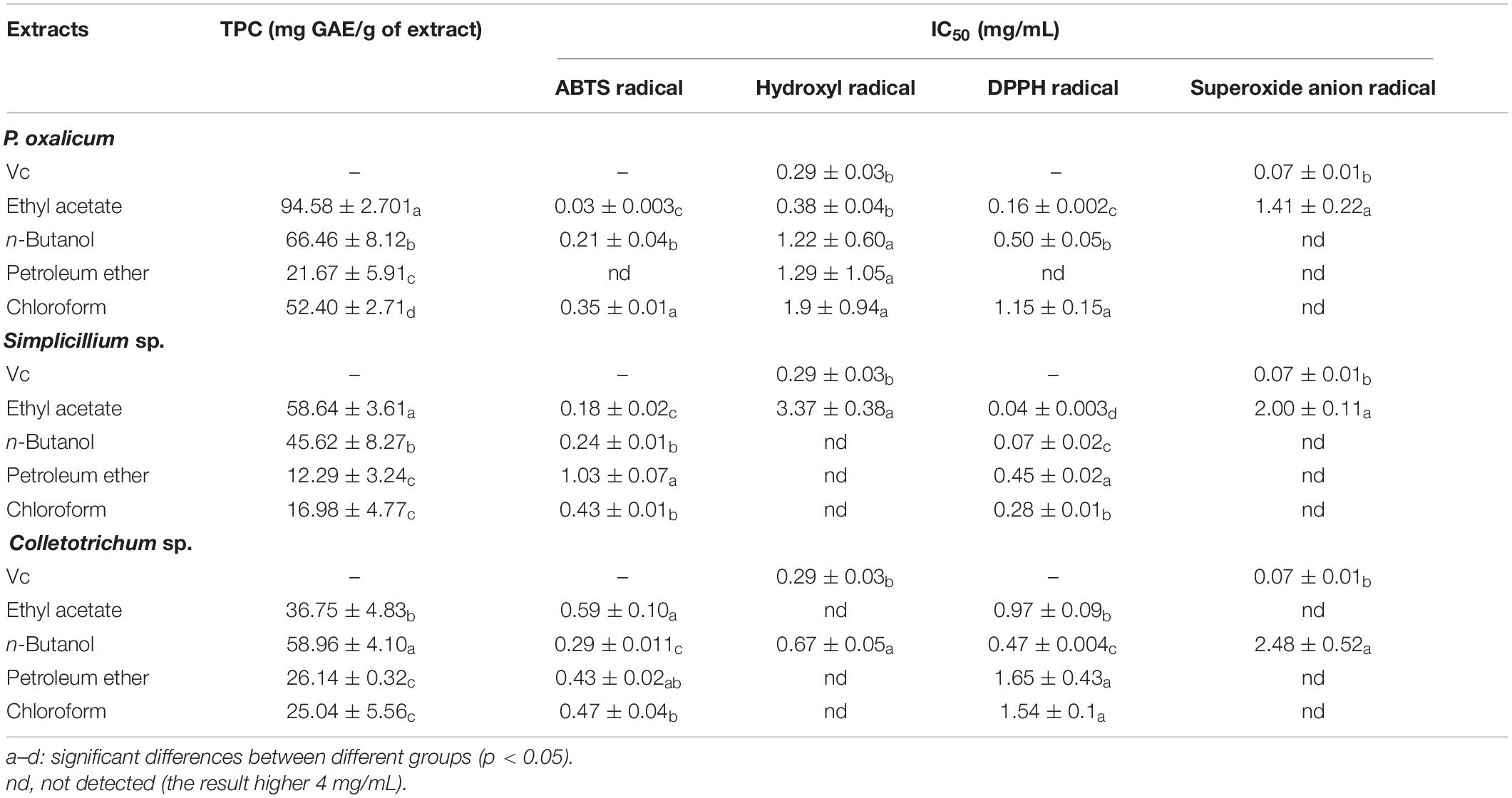
Table 3. Assessment of TPC and antioxidant activity of P. oxalicum, Simplicillium sp., and Colletotrichum sp. extracts.
Antioxidant Activity
To further explore whether the extracts possess antioxidant activity, four different methods, namely ABTS radical scavenging assay, hydroxyl radical scavenging assay, DPPH radical scavenging assay, and superoxide anion radical scavenging assay, were used to evaluate the antioxidant activities of the ethyl acetate fractions, n-butanol fractions, petroleum ether fractions, and chloroform fractions extracted from the P. oxalicum, Simplicillium sp., and Colletotrichum sp. The different extracts of the fermentation broth of the three strains showed antioxidant activity to different degrees, and the antioxidant activities seemed to be correlated with their TPC. In addition, the ethyl acetate extract of P. oxalicum and Simplicillium sp., and the n-butanol extract of Colletotrichum sp. had the highest antioxidant activity.
The results of antioxidant activity are shown in Figure 2 and Table 3. As seen in Figure 2A, all samples exhibited scavenging activity of ABTS, hydroxyl radical DPPH, and superoxide anion free radical at all concentrations in a concentration-dependent pattern. At 0.6 mg/mL, the scavenging rate of ABTS and hydroxyl radical of the P. oxalicum ethyl acetate extracts was similar to Vc and significantly higher than other extracts (Figures 2Aa,b; P < 0.05). As shown in Table 3, the ethyl acetate extracts of P. oxalicum exhibited the strongest scavenging activity (IC50 ABTS+⋅ = 0.03 ± 0.003 mg/mL; IC50 ⋅OH = 3.38 ± 0.04 mg/mL; IC50 DPPH = 0.16 ± 0.002 mg/mL; IC50 ⋅o2– = 1.41 ± 0.22 mg/mL) followed by the n-butanol extracts (IC50 ABTS+⋅ = 0.21 ± 0.04 mg/mL; IC50 ⋅OH = 1.22 ± 0.60 mg/mL; IC50 DPPH = 0.50 ± 0.05 mg/mL) (p < 0.05). Regarding the Simplicillium sp. extract, the IC50 of the ethyl acetate extracts in the four tests were significantly lower (P < 0.05) compared with the other three extracts, with IC50 of 0.18 ± 0.002, 3.37 ± 0.38, 0.04 ± 0.003, and 2.00 ± 0.11 for ABTS radical, hydroxyl radical, DPPH radical, and superoxide anion radical, respectively (Table 3). Moreover, the DPPH radical scavenging rate of the ethyl acetate extracts of Simplicillium sp. was close to Vc at the same concentration, especially at 0.2, 1, and 3 mg/mL (Figure 2Bc; p < 0.05). The results suggest that ethyl acetate extracts of P. oxalicum and Simplicillium sp. have the strongest antioxidant activity. However, at 3 mg/mL, only the n-butanol extracts from Colletotrichum sp. showed higher 50% scavenging rate of ABTS, hydroxyl radical, DPPH, and superoxide anion radical, and the best antioxidant activity was obtained with n-butanol, which IC50 was 0.29 ± 0.011, 0.67 ± 0.05, 0.67 ± 0.05, and 2.48 ± 0.52 mg/mL, respectively (Figure 2C and Table 3). The differences observed in the radical scavenging effects among the extracts could be attributed to the differences in their polyphenol content.
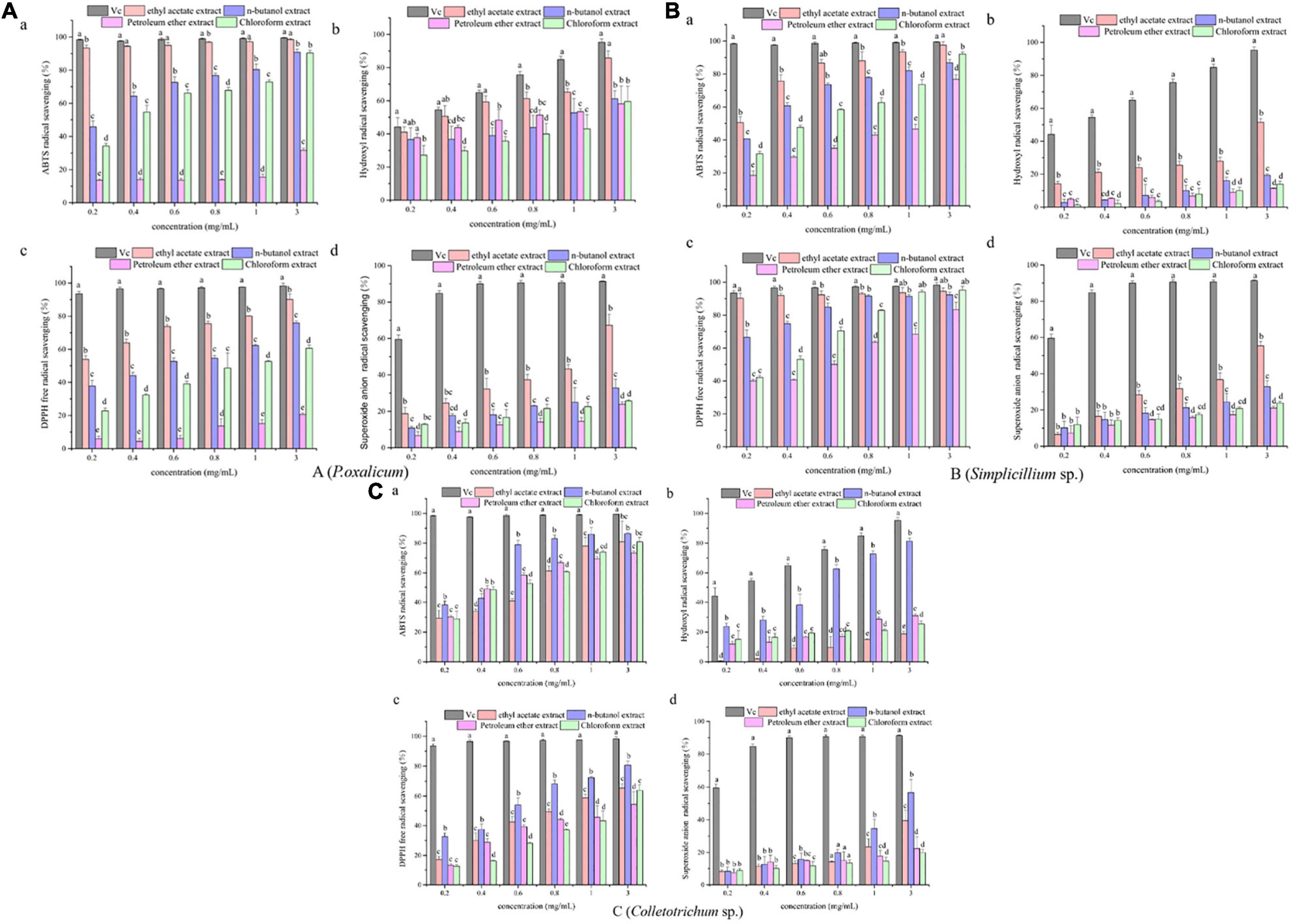
Figure 2. Antioxidant activities of the P. oxalicum (A), Simplicillium sp. (B), and Colletotrichum sp. (C) extracts. a: ABTS scavenging activity. b: Hydroxyl radical scavenging activity. c: DPPH radical scavenging activity. d: Superoxide anion radical scavenging activity. a–d: Significant differences between different groups (p < 0.05).
Antibacterial Activity
Antimicrobial activities of the extracts of P. oxalicum, Simplicillium sp., and Colletotrichum sp. extracts were tested against gram-positive and gram-negative bacteria (Tables 4, 5).
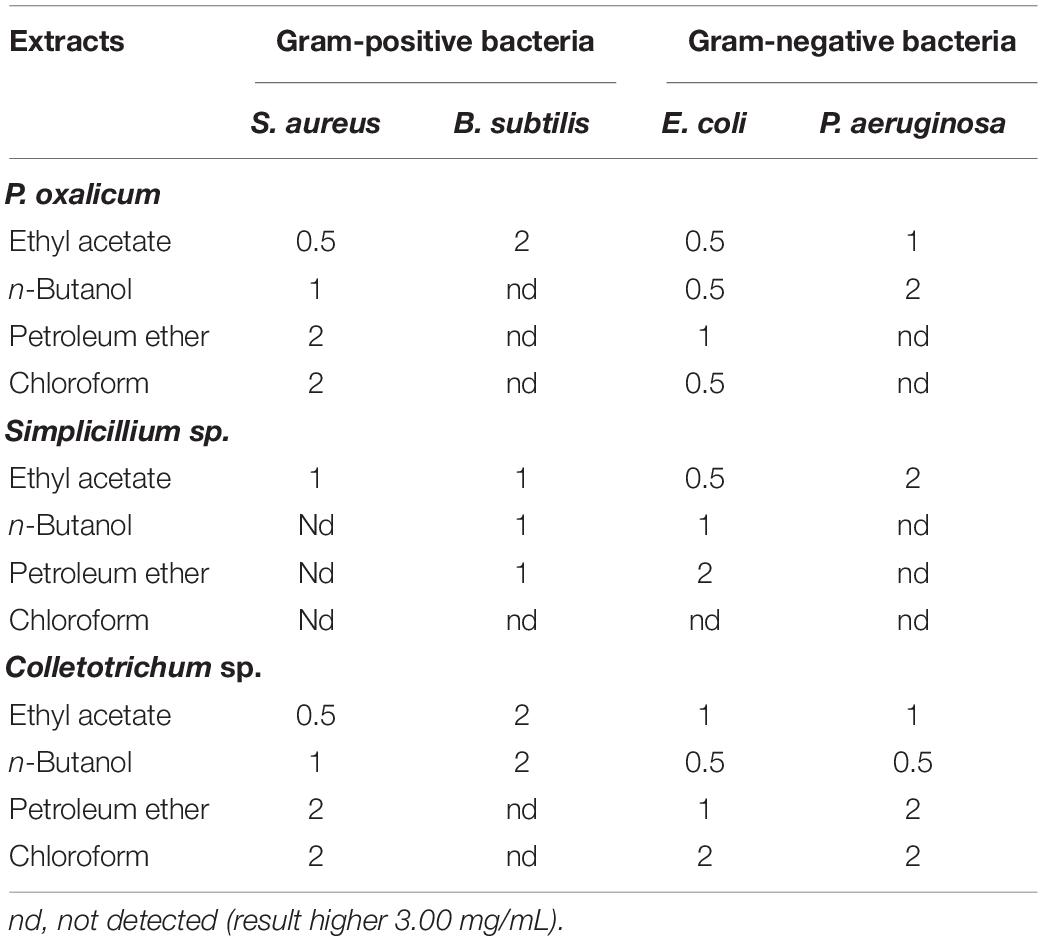
Table 4. Minimum inhibitory concentration (MIC) (mg/mL) of the P. oxalicum, Simplicillium sp., and Colletotrichum sp. extracts.
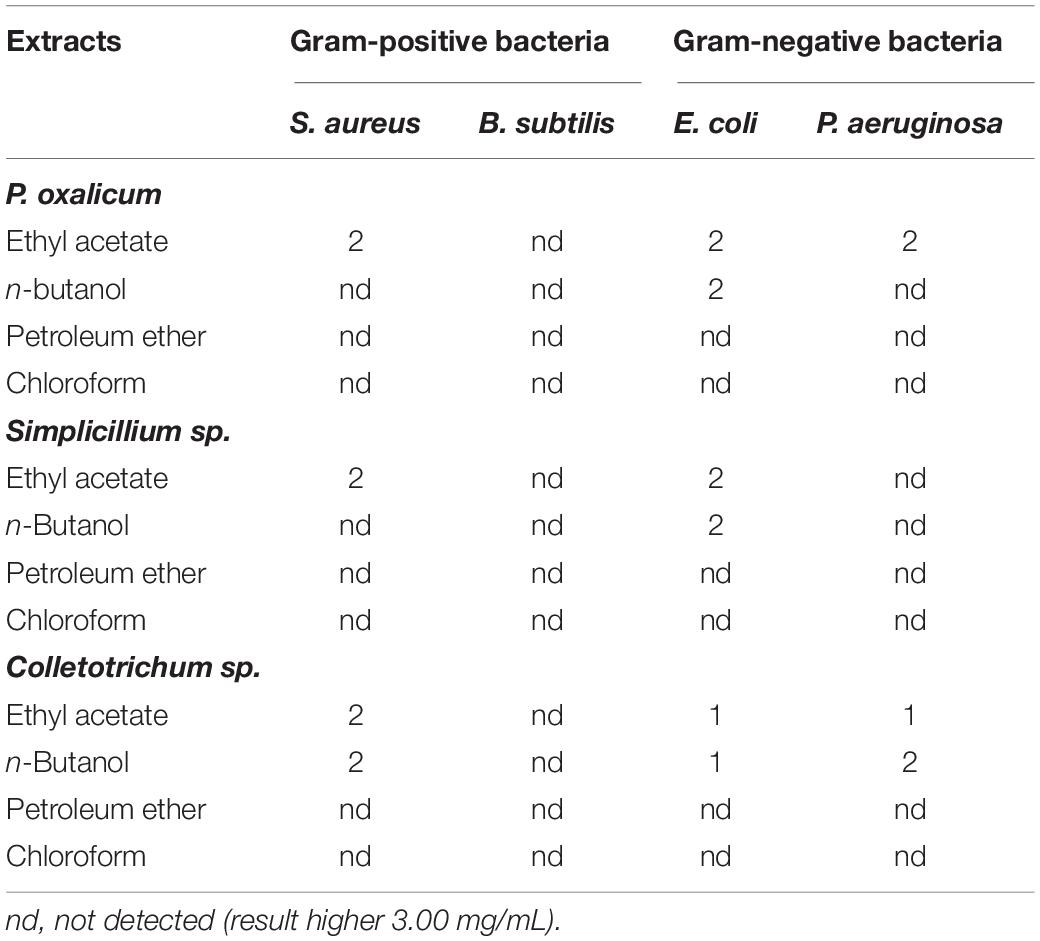
Table 5. Minimum bactericidal concentration (MBC) (mg/mL) of the P. oxalicum, Simplicillium sp., and Colletotrichum sp. extracts.
The MIC of the samples was determined using the broth micro-dilution method. P. oxalicum, Simplicillium sp., and Colletotrichum sp. showed antibacterial effects against all the tested bacteria (Table 4). However, different extracts of the three fungi showed varying degrees of antibacterial and bactericidal activity depending on the solvent used (Table 4). As shown in Table 4, the ethyl acetate extracts of P. oxalicum had antibacterial effects against all the tested bacteria with a MIC between 0.5 and 2 mg/mL. However, the n-butanol, petroleum ether, and chloroform extracts of P. oxalicum had antibacterial activity only against E. coli and S. aureus, but not against antibacterial effect of B. subtilis and P. aeruginosa. Similarly, only the ethyl acetate extracts of Simplicillium sp. showed antibacterial effect against E. coli, B. subtilis, P. aeruginosa, and S. aureus with MIC of 0.5, 1, 2, and 1 mg/mL, respectively (Table 4). Regarding Colletotrichum sp., n-butanol and ethyl acetate extracts also inhibited all tested bacteria with MICs between 0.5 and 2 mg/mL (Table 4). The above results indicated that P. oxalicum, Simplicillium sp., and Colletotrichum sp. have a certain degree of antibacterial ability and that ethyl acetate can effectively extract bioactive compounds of P. oxalicum, Simplicillium sp., and Colletotrichum sp.
The MBCs of the fungal extracts are summarized in Table 5. Against S. aureus, E. coli, and P. aeruginosa, ethyl acetate extracts of the three strains had bactericidal activity, with MBCs between 1.0 and 2.0 mg/mL. In contrast, none of the extracts exhibited bactericidal activity against B. subtilis.
Acridine orange can freely penetrate the cell membrane of bacterial cells, bind to nucleic acids, and emit green fluorescence. As shown in Figure 3, untreated bacterial cell maintained intact cell membrane and emitted intense green fluorescence (Figure 3A). In contrast, the green fluorescence significantly decreased after treatment with ethyl acetate extracts at a concentration of 2MIC, which indicated that the cell membranes of pathogenic bacteria (S. aureus, E. coli, and P. aeruginosa) induced by ethyl acetate extracts were damaged (Figures 3B–D). The above results suggested that ethyl acetate extracts can potently inhibit cell growth and differentiation by disrupting cell membranes.
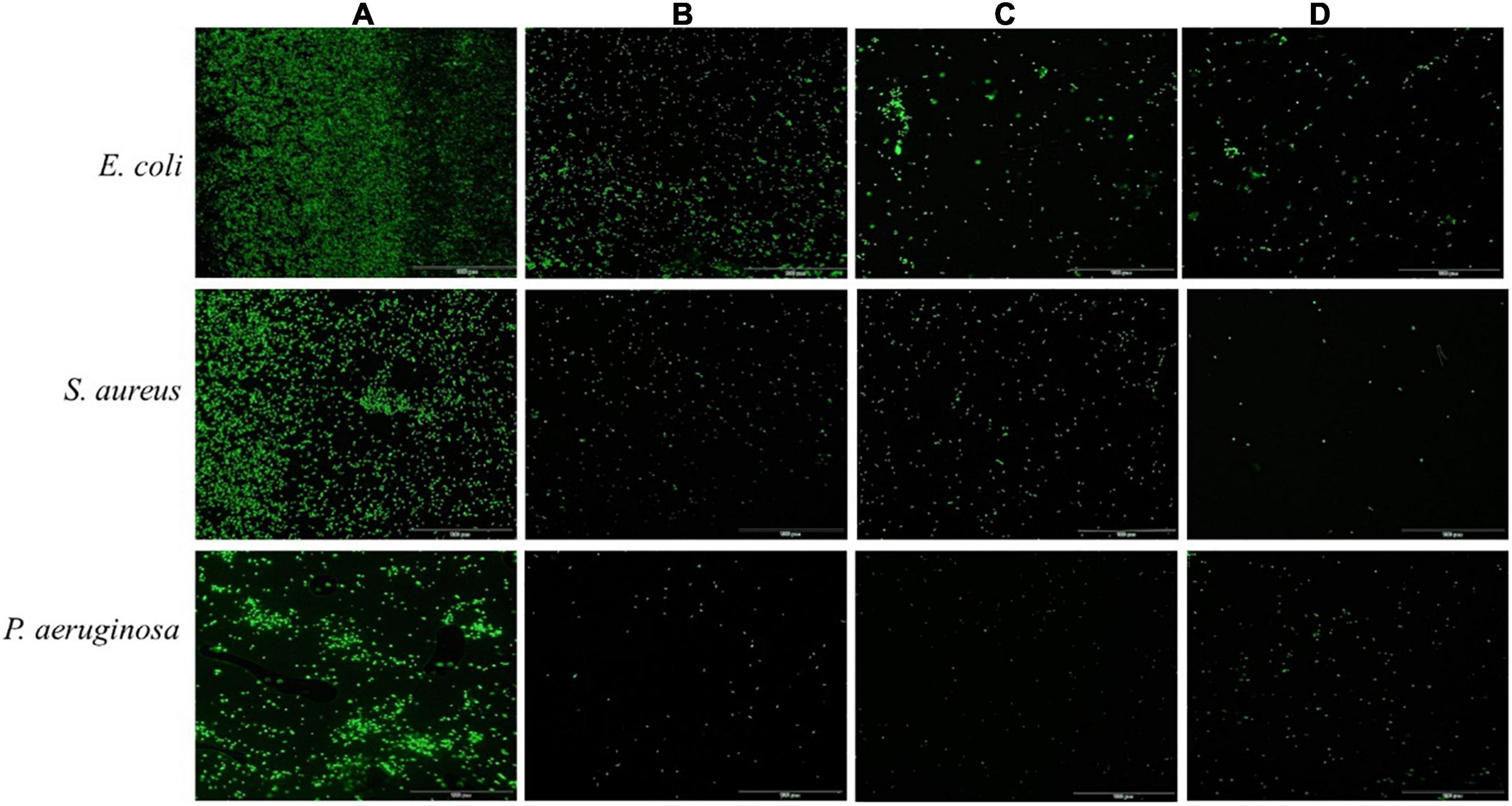
Figure 3. Effects of ethyl acetate extracts from endophytic fungi on cell membrane integrity of E. coli, S. aureus, and P. aeruginosa by fluorescence microscope. (A) Untreated bacterial cells; (B) bacterial cells treated with P. oxalicum extracts at 2MIC; (C) bacterial cells treated with Simplicillium sp. extracts at 2MIC; (D) bacterial cells treated with Colletotrichum sp. extracts at 2MIC. The scale bar was 100 μm.
Liquid Chromatography-Mass Spectrometry
The ethyl acetate extracts of P. oxalicum and Simplicillium sp. and the n-butanol extracts of Colletotrichum sp. exhibited effective anti-microbial and antioxidant activity with high polyphenol content and were selected for compound analysis by liquid chromatography-mass spectrometry (LC-MS) analytical technique. Table 6 shows the retention time, molecular weight, molecular formula, M/Z, and content of the identified chemical constituents presented in the extracts. The chromatograms are shown in Supplementary Figure 3. The identified compounds included phenolic acids (caffeic acid, chlorogenic acid, ferulic acid, etc.); flavonoids (zpigenin, phloretin, luteolin, hesperetin, etc.); fatty acids (palmitic acid, oleic acid, linoleic acid); organic acid (succinic acid, citric acid, etc.); and monosaccharide [sucrose, d-(–)-fructose, and d-(+)-glucose] (Table 6). As shown in Table 6, a total of 36 compounds were identified in P. oxalicum, of which hesperetin was the major compound with a concentration of 36.06 μmol/g. A total of 28 compounds were identified in Colletotrichum sp., and its major compound was succinic acid (8.11 μmol/g). This was followed by Simplicillium sp. extracts with 34 identified compounds. 4-hydroxyphenylpyruvic acid (17.23 μmol/g) and d (+)-phenylactic acid (4.01 μmol/g) were major compounds in Simplicillium sp. extracts. All in all, phenolic and organic acids were the most abundant compounds in the extracts of the three endophytic fungi, which may be closely related to the biological activity of the secondary metabolites of endophytic fungi.
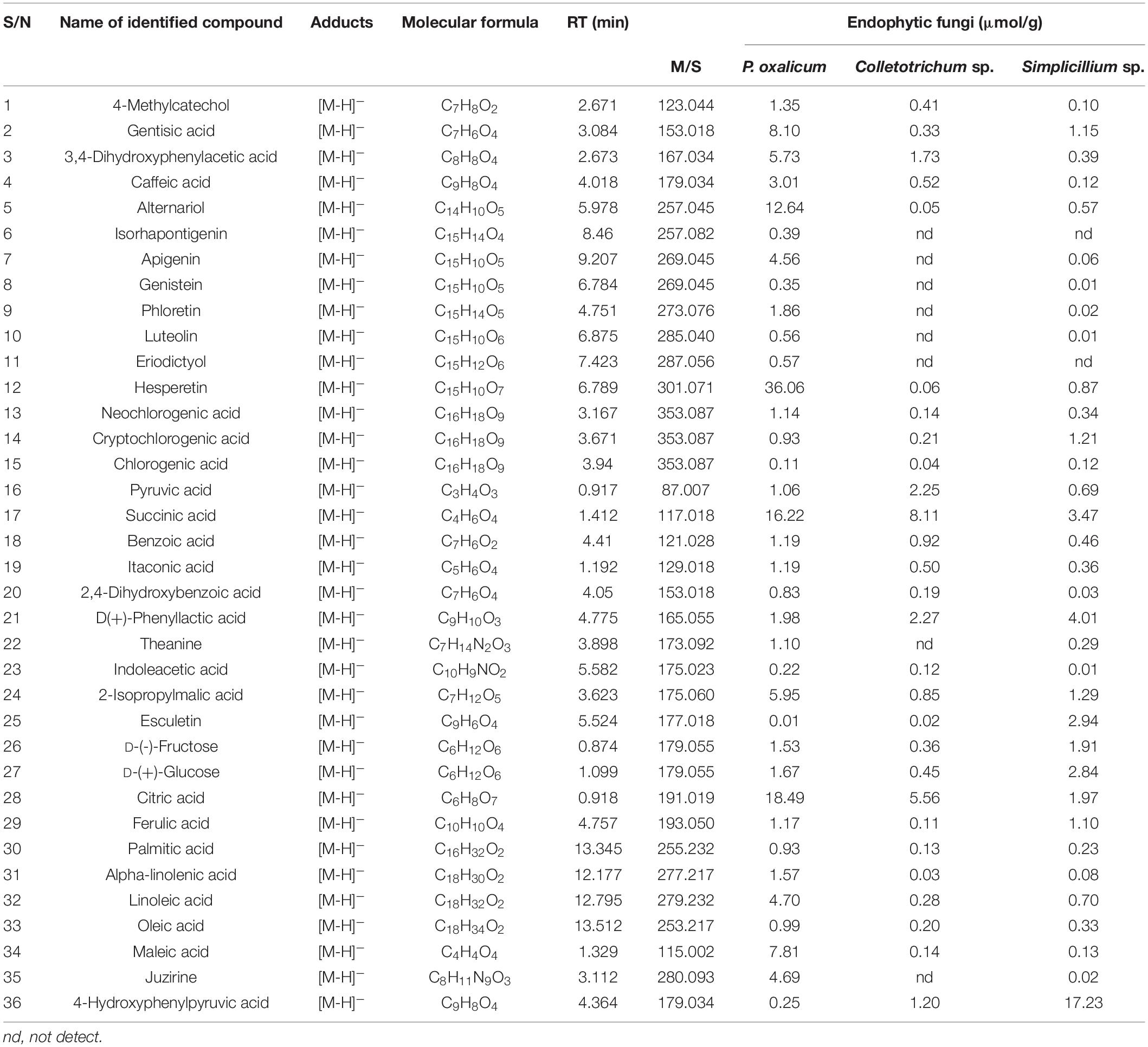
Table 6. The identification of the chemical composition of endophytic fungal extracts by LC-MS analysis.
Discussion
The CX is a well-known Chinese medicinal plant with diverse phytochemicals such as ferulic acid, ligustrazine, and ligustilide, which contribute to its medicinal properties (Yuan et al., 2020a). Endophytic fungi are not only one of the most important elements in plant micro-ecosystems but also a storehouse for natural products, including antibiotics, antioxidants, and anticancer agents, which could be an alternative source of secondary metabolites for medical and pharmacological applications (Strobel et al., 2002). A previous research showed that the different ages and tissues of host plants may influence the species composition of endophytic fungus community (Sieber, 2007). In this study, a total of 21 endophytic fungi belonging to 11 different genera were isolated from different parts of CX. This is similar to what was observed in CX by Wang et al. (2008), who also found Penicillium, Fusarium, Aspergillus, Alternaria, etc. Some species, such as Nigrospora sphaerica and Sordariomycetes sp., are exclusive to different plant parts. The tissue specificity of a particular endophyte suggests that certain species perpetuate within the specific chemistry or texture of a particular tissue due to the differences in anatomical structure and physiological conditions (Park et al., 2012). Only Fusarium sp. was present in the four sites studied. The pleiotropic colonization of Fusarium sp. may be related to its secreted metabolites (Pappas et al., 2018), which can protect host plants from biotic or abiotic stresses. The Aporospora sp. and Sordariomycetes sp. isolated from CX in this study have not been reported previously (Hu et al., 2016). These results increase the diversity of endophytic fungi in CX.
Polyphenols have hydroxyl groups and play a vital role in anti-oxidation, scavenging free radicals and other pharmacological activities (Gangwar et al., 2014). Therefore, the discovery of polyphenol-producing endophytic fungi could help meet the needs of the pharmaceutical and food industry. For instance, Govindappa et al. (2013) reported that organic extracts of Penicillium sp. present a large amount of phenolic compounds that act as antioxidants and could be used for medicinal or food preservation. Fill et al. (2010) discovered an effective antioxidant compound, namely, phenylpropanoid amide, isolated from the endophytic fungus Penicillium brasilianum. Similarly, Tian et al. (2020) found that Simplicillium sp. ethyl acetate extracts have excellent antioxidant activity, which contains various chemical components. In the present study, P. oxalicum, Simplicillium sp., and Colletotrichum sp. isolated from CX were polyphenol-producing strains, which deserve further study.
Different solvents can recover compounds of different contents and components in the current study, we found polar and medium polarity solvents are more favorable to the extraction of low and high molecular weight polyphenols in endophytic fungus fermentation broth compared to those of non-polar solvent. This finding is consistent with a previous study (Scholz and Rimpler, 1989). The highest antioxidant activity was observed in the P. oxalicum extracts, which may be linked to the concentrations of the chemical compounds and the compositions identified in the fungal extracts. Hesperidin was the main phenolic compound in the extracts of P. oxalicum, which has powerful radical scavenging activity, and it augmented the antioxidant cellular defenses via the ERK/Nrf2 signaling pathway as well (Parhiz et al., 2015). However, compared to the potency of the standard antioxidants Vc, the extracts of the endophytic fungi showed lower antioxidant activity. Moreover, the antioxidants used as standards are purified molecules, while the endophytic fungi extracts represent a group of mixtures containing different concentrations of substances.
Endophytic fungi have also been recognized as producers of antimicrobial substances, such as Simplicildone A and Botryorhodine C isolated from the fungus Simpilcillium sp. PSU-H41 displayed antibacterial against S. aureus (Saetang et al., 2017). In our study, we observed that three isolated endophytic fungi showed bacteriostatic activity against the test strain, whereas different extract parts of endophytic fungi showed varying degrees of antibacterial effect. Data from the literature demonstrating that the antibacterial effects of endophytic fungi extracts are related to the extraction solvent (Mefteh et al., 2018), which corroborates our results. Among the endophytic fungi studied, P. oxalicum stood out for the best antibacterial and bactericidal activity, which could be attributed to organic acids and phenolic compounds. Among the compounds identified in the extracts of P. oxalicum, citric acid and hesperidin were the major compounds, followed by alternariol, succinic acid, and gentisic acid, which were higher than those of Simplicillium sp. and Colletotrichum sp. Gu (2009) have reported that alternariol from endophytic fungus Alternaria brassicicola possesses antibacterial properties. The antibacterial activity of citric acid against bacteria was attributed to its physical and chemical properties, reduced production of extracellular aggregates, and hydrophobicity of the cell surface (Zhang et al., 2019).
Antibacterial compounds can act on bacterial cell walls, plasma membranes, protein synthesis, nucleic acid metabolism, and DNA, thereby killing pathogenic bacteria (Li et al., 2015). Among the numerous antibacterial compounds, we have emphasized phenolic compounds, which exhibit great structural diversity, such as the presence, number and substitution position of hydroxyl groups, and the length of saturated side chains, which confer antibacterial activity to these compounds (Cushnie and Lamb, 2011; Rempe et al., 2017). For example, Rasch (2002) found that phenolic acid inhibited the activity of ribonucleic acid reductase, a key enzyme required for DNA synthesis, and that bacterial DNA synthesis was blocked. Our findings provided further evidence that extracts of P. oxalicum, Simplicillium sp., and Colletotrichum sp. displayed anti-biofilm activity against the tested strains with an almost complete destruction of the biofilm matrix.
The P. oxalicum, Simplicillium sp., and Colletotrichum sp. isolated from CX are promising endophytic fungi, and the chemical composition of endophytic fungus extracts have been identified by LC-MS. Some of the identified chemical components have been reported to have significant antimicrobial, antioxidant, and other biological activities, while others have not been reported, such as juzirine. In addition, some of these compounds with biological activity were isolated and identified from plants rather than microorganisms. For example, hesperidin, as an anti-inflammatory and antioxidant drug (Li and Schluesener, 2017), is traditionally extracted from abundant natural citrus fruits using large amounts of ethanol or methanol solvents, which is not conducive to sustainable development in the pharmaceutical industry. In our study, P. oxalicum can produce abundant secondary metabolites including phenolic acids, flavonoids, and fatty acids. Phenolic acids and flavonoids are important secondary metabolites that exert therapeutic effects (Winter et al., 2017; Adamczak et al., 2019). Fatty acids are able to reduce and resist the oxidative stress of free radicals through a series of physiological and biochemical reactions (Gülçin, 2012; Mocking et al., 2018). Previous studies have reported the isolation and characterization of Penicilisorin (Arunpanichlert et al., 2010) and P-hydroxybenzaldehyde (Schmeda-Hirschmann et al., 2005), an antibacterial compound from the endophytic fungi Penicillium sclerotiorum PSU-A13 and Penicillium janczewskii, respectively. Similarly, a coumarone antioxidant, pestacin and isopestacin, were isolated from the endophytic fungus Pestalotiopsis microspora (Strobel et al., 2002). These results suggested that P. oxalicum from CX containing many active compounds makes it a promising source of molecules for future studies. Interestingly, we also found that P. oxalicum strain can produce ferulic acid, chlorogenic acid, and caffeic acid, just like their host plant, indicating that P. oxalicum may play an important role in affecting the quality and quantity of the CX through a specific fungus-host interaction (Ding et al., 2018). In the future, because of the fast growth rate of endophytic fungi and the advantage of easy operation during fermentation (Ludwig-Müller, 2015), these active substances can be obtained by fermentation rather than from the plant tissues itself. Moreover, the production capacity of secondary metabolites of P. oxalicum and their biological effects could be increased by optimizing cultivation conditions or genetic engineering. In conclusion, in order to use the endophytic fungus of CX in medicine, more detailed studies are needed in future research.
Conclusion
In conclusion, the present study expanded the diversity of endophytic fungal species in CX and confirmed antioxidant and antimicrobial activities of several endophytic fungi extracts, among which the ethyl acetate extract of the endophytic fungus P. oxalicum stood out. The antioxidant and antibacterial activities of the P. oxalicum extracts are attributed to the phenolic compounds. Together, this study suggests that P. oxalicum from CX is a promising source for detection of natural antioxidants and antibacterial compounds. However, in order to use this endophytic fungus in medicine, more detailed studies need to be conducted.
Data Availability Statement
The original contributions presented in the study are included in the article/Supplementary Material, further inquiries can be directed to the corresponding author/s.
Author Contributions
ZT and YQ conceived and designed the experiments, performed the experiments, and prepared figures and/or tables. WC, ZZ, WL, QL, HY, YL, and YW performed the experiments, prepared the figures and/or tables. HuC conceived and designed the experiments, drafted the work, and approved the final draft. YX and YC analyzed the data and drafted the work. TB and HoC analyzed the data and revised it critically for important content. All authors contributed to the article and approved the submitted version.
Funding
This work was supported by the Scientific Research Foundation of the Education Department of Sichuan Province, China (Grant No. 18ZB0455) and Sichuan Sharing and Service Platform of Scientific and Technological Resource (Enzyme Resource) of Sichuan Province, China (Platform Project No. 2020JDPT0018).
Conflict of Interest
The authors declare that the research was conducted in the absence of any commercial or financial relationships that could be construed as a potential conflict of interest.
Publisher’s Note
All claims expressed in this article are solely those of the authors and do not necessarily represent those of their affiliated organizations, or those of the publisher, the editors and the reviewers. Any product that may be evaluated in this article, or claim that may be made by its manufacturer, is not guaranteed or endorsed by the publisher.
Supplementary Material
The Supplementary Material for this article can be found online at: https://www.frontiersin.org/articles/10.3389/fmicb.2021.771000/full#supplementary-material
References
Adamczak, A., Ożarowski, M., and Karpiński, T. M. (2019). Antibacterial activity of some flavonoids and organic acids widely distributed in plants. J. Clin. Med. 9:109. doi: 10.3390/jcm9010109
Aly, A. H., Debbab, A., and Proksch, P. (2011). Fungal endophytes: unique plant inhabitants with great promises. Appl. Microbiol. Biotechnol. 90, 1829–1845. doi: 10.1007/s00253-011-3270-y
Anderson, C. J., and Kendall, M. M. (2017). Salmonella enterica serovar typhimurium strategies for host adaptation. Front. Microbiol. 8:1983. doi: 10.3389/fmicb.2017.01983
Apel, K., and Hirt, H. (2004). Reactive oxygen species: metabolism, oxidative stress, and signal transduction. Annu. Rev. Plant Biol. 55, 373–399. doi: 10.1146/annurev.arplant.55.031903.141701
Arunpanichlert, J., Rukachaisirikul, V., Sukpondma, Y., Phongpaichit, S., Tewtrakul, S., Rungjindamai, N., et al. (2010). Azaphilone and isocoumarin derivatives from the endophytic fungus Penicillium sclerotiorum PSU-A13. Chem. Pharm. Bull. 58, 1033–1036. doi: 10.1248/cpb.58.1033
Chen, Z., Zhang, C., Gao, F., Fu, Q., Fu, C., He, Y., et al. (2018). A systematic review on the rhizome of Ligusticum chuanxiong Hort. (Chuanxiong). Food Chem. Toxicol. 119, 309–325. doi: 10.1016/j.fct.2018.02.050
Cushnie, T. P. T., and Lamb, A. J. (2011). Recent advances in understanding the antibacterial properties of flavonoids. Int. J. Antimicrob. Agents 38, 99–107. doi: 10.1016/j.ijantimicag.2011.02.014
da Rocha, P. D. S., Paula, V. M. B., Olinto, S. C. F., Dos Santos, E. L., Souza, K., de, P., et al. (2020). Diversity, chemical constituents and biological activities of endophytic fungi isolated from Schinus terebinthifolius raddi. Microorganisms 8, 1–13. doi: 10.3390/microorganisms8060859
Del Giudice, P. (2020). Skin infections caused by Staphylococcus aureus. Acta Derm. Venereol. 100, 208–215. doi: 10.2340/00015555-3466
Desai, N., Sharma, R., Makker, K., Sabanegh, E., and Agarwal, A. (2009). Physiologic and pathologic levels of reactive oxygen species in neat semen of infertile men. Fertil. Steril. 92, 1626–1631. doi: 10.1016/j.fertnstert.2008.08.109
Ding, C. H., Wang, Q. B., Guo, S., and Wang, Z. Y. (2018). The improvement of bioactive secondary metabolites accumulation in Rumex gmelini Turcz through co-culture with endophytic fungi. Braz. J. Microbiol. 49, 362–369. doi: 10.1016/j.bjm.2017.04.013
Fill, T. P., da Silva, B. F., and Rodrigues-Fo, E. (2010). Biosynthesis of phenylpropanoid amides by an endophytic Penicillium brasilianum found in root bark of Melia azedarach. J. Microbiol. Biotechnol. 20, 622–629.
Gangwar, M., Gautam, M. K., Sharma, A. K., Tripathi, Y. B., Goel, R. K., and Nath, G. (2014). Antioxidant capacity and radical scavenging effect of polyphenol rich Mallotus philippenensis fruit extract on human erythrocytes: an in vitro study. Sci. World J. 2014:279451. doi: 10.1155/2014/279451
Ge, H., Chen, Y., Chen, J., Tian, J., Liang, X., and Chen, L. (2018). Evaluation of antioxidant activities of ethanol extract from Ligusticum subjected to in-vitro gastrointestinal digestion. Food Chem. Toxicol. 119, 417–424. doi: 10.1016/j.fct.2017.12.035
Govindappa, M., Channabasava, R., Sunil Kumar, K. R., and Pushpalatha, K. C. (2013). Antioxidant activity and phytochemical screening of crude endophytes extracts of Tabebuia argentea Bur. & K. Sch. Am. J. Plant Sci. 3, 1641–1652. doi: 10.4236/ajps.2013.48198
Gu, W. (2009). Bioactive metabolites from alternaria brassicicola ML-P08, an endophytic fungus residing in malus halliana. World J. Microbiol. Biotechnol. 25, 1677–1683. doi: 10.1007/s11274-009-0062-y
Gülçin, I. (2012). Antioxidant activity of food constituents: an overview. Arch. Toxicol. 86, 345–391. doi: 10.1007/s00204-011-0774-2
Gupta, D. R., Kabir, M. K., Hassan, O., Sabir, A. A., Mahmud, N. U., Surovy, M. Z., et al. (2019). First report of anthracnose crown rot of strawberry caused by Colletotrichum siamense in Rajshahi District of Bangladesh. Plant Dis. 103:1775.
Hu, R., Gong, G., Ye, H., Yao, L., and Huang, X. (2016). Isolation and identification of Ligusticum chuanxiong rhizome Fungi of Dujiangyan in Sichuan. Southwest China J. Agric. Sci. 29, 1238–1240. doi: 10.16213/j
Huang, W. Y., Cai, Y. Z., Xing, J., Corke, H., and Sun, M. (2007). A potential antioxidant resource: endophytic fungi from medicinal plants. Econ. Bot. 61, 14–30.
Jardak, M., Elloumi-Mseddi, J., Aifa, S., and Mnif, S. (2017). Chemical composition, anti-biofilm activity and potential cytotoxic effect on cancer cells of Rosmarinus officinalis L. essential oil from Tunisia. Lipids Health Dis. 16, 1–10. doi: 10.1186/s12944-017-0580-9
Jia, M., Chen, L., Xin, H. L., Zheng, C. J., Rahman, K., Han, T., et al. (2016). A friendly relationship between endophytic fungi and medicinal plants: a systematic review. Front. Microbiol. 7:906. doi: 10.3389/fmicb.2016.00906
Kahl, R., and Kappus, H. (1993). Toxikologie der synthetischen antioxidantien BHA und BHT im vergleich mit dem natürlichen antioxidans Vitamin E. Z. Lebensmittel Untersuchung Forschung 196, 329–338. doi: 10.1007/BF01197931
Kimmig, A., Hagel, S., Weis, S., Bahrs, C., Löffler, B., and Pletz, M. W. (2021). Management of Staphylococcus aureus bloodstream infections. Front. Med. 7:616524. doi: 10.3389/fmed.2020.616524
Li, C., and Schluesener, H. (2017). Health-promoting effects of the citrus flavanone hesperidin. Crit. Rev. Food Sci. Nutr. 57, 613–631. doi: 10.1080/10408398.2014.906382
Li, G., Kusari, S., Kusari, P., Kayser, O., and Spiteller, M. (2015). Endophytic Diaporthe sp. LG23 produces a potent antibacterial tetracyclic triterpenoid. J. Nat. Prod. 78, 2128–2132. doi: 10.1021/acs.jnatprod.5b00170
Ludwig-Müller, J. (2015). Plants and endophytes: equal partners in secondary metabolite production? Biotechnol. Lett. 37, 1325–1334. doi: 10.1007/s10529-015-1814-4
Marseglia, L., Manti, S., D’Angelo, G., Nicotera, A., Parisi, E., Di Rosa, G., et al. (2015). Oxidative stress in obesity: a critical component in human diseases. Int. J. Mol. Sci. 16, 378–400. doi: 10.3390/ijms16010378
Martin, R. M., and Bachman, M. A. (2018). Colonization, infection, and the accessory genome of Klebsiella pneumoniae. Front. Cell. Infect. Microbiol. 8:4. doi: 10.3389/fcimb.2018.00004
Mefteh, F. B., Daoud, A., Bouket, A. C., Thissera, B., Kadri, Y., Cherif-Silini, H., et al. (2018). Date palm trees root-derived endophytes as fungal cell factories for diverse bioactive metabolites. Int. J. Mol. Sci. 19:1986. doi: 10.3390/ijms19071986
Minussi, R. C., Rossi, M., Bologna, L., Cordi, L., Rotilio, D., Pastore, G. M., et al. (2003). Phenolic compounds and total antioxidant potential of commercial wines. Food Chem. 82, 409–416. doi: 10.1016/S0308-8146(02)00590-3
Mocking, R. J. T., Assies, J., Ruhé, H. G., and Schene, A. H. (2018). Focus on fatty acids in the neurometabolic pathophysiology of psychiatric disorders. J. Inherit. Metab. Dis. 41, 597–611. doi: 10.1007/s10545-018-0158-3
Molla, Y., Nedi, T., Tadesse, G., Alemayehu, H., and Shibeshi, W. (2016). Evaluation of the in vitro antibacterial activity of the solvent fractions of the leaves of Rhamnus prinoides L’Herit (Rhamnaceae) against pathogenic bacteria. BMC Complement. Altern. Med. 16:287. doi: 10.1186/s12906-016-1279-6
Nischitha, R., and Shivanna, M. B. (2021). Antimicrobial activity and metabolite profiling of endophytic fungi in Digitaria bicornis (Lam) Roem. and Schult. and Paspalidium flavidum (Retz.) A. Camus. 3 Biotech 11:53. doi: 10.1007/s13205-020-02590-x
Noinart, J., Buttachon, S., Dethoup, T., Gales, L., Pereira, J. A., Urbatzka, R., et al. (2017). A new ergosterol analog, a new bis-anthraquinone and anti-obesity activity of anthraquinones from the marine sponge-associated fungus Talaromyces stipitatus KUFA 0207. Mar. Drugs 15:139. doi: 10.3390/md15050139
Nuerxiati, R., Abuduwaili, A., Mutailifu, P., Wubulikasimu, A., Rustamova, N., Jingxue, C., et al. (2019). Optimization of ultrasonic-assisted extraction, characterization and biological activities of polysaccharides from Orchis chusua D. Don (Salep). Int. J. Biol. Macromol. 141, 431–443. doi: 10.1016/j.ijbiomac.2019.08.112
Padhi, S., Masi, M., Panda, S. K., Luyten, W., Cimmino, A., Tayung, K., et al. (2020). Antimicrobial secondary metabolites of an endolichenic Aspergillus niger isolated from lichen thallus of Parmotrema ravum. Nat. Prod. Res. 34, 2573–2580. doi: 10.1080/14786419.2018.1544982
Pappas, M. L., Liapoura, M., Papantoniou, D., Avramidou, M., Kavroulakis, N., Weinhold, A., et al. (2018). The beneficial endophytic fungus Fusarium solani strain K alters tomato responses against spider mites to the benefit of the plant. Front. Plant Sci. 9:1603. doi: 10.3389/fpls.2018.01603
Parhiz, H., Roohbakhsh, A., Soltani, F., Rezaee, R., and Iranshahi, M. (2015). Antioxidant and anti-inflammatory properties of the citrus flavonoids hesperidin and hesperetin: an updated review of their molecular mechanisms and experimental models. Phytother. Res. 29, 323–331. doi: 10.1002/ptr.5256
Park, Y. H., Lee, S. G., Ahn, D. J., Kwon, T. R., Park, S. U., Lim, H. S., et al. (2012). Diversity of Fungal endophytes in various tissues of panax ginseng meyer cultivated in Korea. J. Ginseng Res. 36, 211–217. doi: 10.5142/jgr.2012.36.2.211
Pinheiro, E. A. A., Pina, J. R. S., Feitosa, A. O., Carvalho, J. M., Borges, F. C., Marinho, P. S. B., et al. (2017). Bioprospecting of antimicrobial activity of extracts of endophytic fungi from Bauhinia guianensis. Rev. Argent. Microbiol. 49, 3–6. doi: 10.1016/j.ram.2016.08.005
Ran, X., Ma, L., Peng, C., Zhang, H., and Qin, L. P. (2011). Ligusticum chuanxiong Hort: a review of chemistry and pharmacology. Pharm. Biol. 49, 1180–1189. doi: 10.3109/13880209.2011.576346
Rasch, M. (2002). The influence of temperature, salt and pH on the inhibitory effect of reuterin on Escherichia coli. Int. J. Food Microbiol. 72, 225–231. doi: 10.1016/S0168-1605(01)00637-7
Rempe, C. S., Burris, K. P., Lenaghan, S. C., and Stewart, C. N. (2017). The potential of systems biology to discover antibacterial mechanisms of plant phenolics. Front. Microbiol. 8:422. doi: 10.3389/fmicb.2017.00422
Saetang, P., Rukachaisirikul, V., Phongpaichit, S., Preedanon, S., Sakayaroj, J., Borwornpinyo, S., et al. (2017). Depsidones and an α-pyrone derivative from Simpilcillium sp. PSU-H41, an endophytic fungus from Hevea brasiliensis leaf. Phytochemistry 143, 115–123. doi: 10.1016/j.phytochem.2017.08.002
Schmeda-Hirschmann, G., Hormazabal, E., Astudillo, L., Rodriguez, J., and Theoduloz, C. (2005). Secondary metabolites from endophytic fungi isolated from the Chilean gymnosperm Prumnopitys andina (Lleuque). World J. Microbiol. Biotechnol. 21, 27–32. doi: 10.1007/s11274-004-1552-6
Scholz, E., and Rimpler, H. (1989). Proanthocyanidins from Krameria triandra root. Planta Med. 55, 379–384. doi: 10.1055/s-2006-962032
Shah, S., Shrestha, R., Maharjan, S., Selosse, M. A., and Pant, B. (2019). Isolation and characterization of plant growth-promoting endophytic fungi from the roots of Dendrobium moniliforme. Plants 8:5. doi: 10.3390/plants8010005
Sharma, M., and Sharma, R. (2016). Drugs and drug intermediates from fungi: striving for greener processes. Crit. Rev. Microbiol. 42, 322–338. doi: 10.3109/1040841X.2014.947240
Sieber, T. N. (2007). Endophytic fungi in forest trees: are they mutualists? Fungal Biol. Rev. 21, 75–89. doi: 10.1016/j.fbr.2007.05.004
Sim, Y., and Shin, S. (2008). Combinatorial anti-Trichophyton effects of Ligusticum chuanxiong essential oil components with antibiotics. Arch. Pharm. Res. 31, 497–502. doi: 10.1007/s12272-001-1184-7
Smirnoff, N., and Cumbes, Q. J. (1989). Hydroxyl radical scavenging activity of compatible solutes. Phytochemistry 28, 1057–1060. doi: 10.1016/0031-9422(89)80182-7
Strobel, G., Ford, E., Worapong, J., Harper, J. K., Arif, A. M., Grant, D. M., et al. (2002). Isopestacin, an isobenzofuranone from Pestalotiopsis microspora, possessing antifungal and antioxidant activities. Phytochemistry 60, 179–183. doi: 10.1016/S0031-9422(02)00062-6
Tanvir, R., Javeed, A., and Bajwa, A. G. (2017). Endophyte bioprospecting in South Asian medicinal plants: an attractive resource for biopharmaceuticals. Appl. Microbiol. Biotechnol. 101, 1831–1844. doi: 10.1007/s00253-017-8115-x
Thomer, L., Schneewind, O., and Missiakas, D. (2016). Pathogenesis of Staphylococcus aureus bloodstream infections. Annu. Rev. Pathol. 11, 343–364. doi: 10.1146/annurev-pathol-012615-044351
Tian, T. T., Li, Q. R., Gan, S. Q., Chang, C. R., and Shen, X. C. (2020). Protective effect of Simplicillium sp. Ethyl acetate extract against high glucose-induced oxidative stress in HUVECs. Evid. Based Complement. Alternat. Med. 2020:5172765. doi: 10.1155/2020/5172765
Valko, M., Leibfritz, D., Moncol, J., Cronin, M. T. D., Mazur, M., and Telser, J. (2007). Free radicals and antioxidants in normal physiological functions and human disease. Int. J. Biochem. Cell Biol. 39, 44–84. doi: 10.1016/j.biocel.2006.07.001
Valko, M., Rhodes, C. J., Moncol, J., Izakovic, M., and Mazur, M. (2006). Free radicals, metals and antioxidants in oxidative stress-induced cancer. Chem. Biol. Interact. 160, 1–40. doi: 10.1016/j.cbi.2005.12.009
Wang, A. N., Yi, X. W., Yu, H. F., Dong, B., and Qiao, S. Y. (2009). Free radical scavenging activity of Lactobacillus fermentum in vitro and its antioxidative effect on growing-finishing pigs. J. Appl. Microbiol. 107, 1140–1148. doi: 10.1111/j.1365-2672.2009.04294.x
Wang, Y. L., Yan, Z. Y., Guo, X. H., Song, J., Chen, X., and Wan, D. G. (2008). Isolation and identification of endophytic fungi from Ligusticum. China J. Chin. Mater. Med. 33, 999–1001. doi: 10.3321/j
Winter, A. N., Brenner, M. C., Punessen, N., Snodgrass, M., Byars, C., Arora, Y., et al. (2017). Comparison of the neuroprotective and anti-inflammatory effects of the anthocyanin metabolites, protocatechuic acid and 4-hydroxybenzoic acid. Oxid. Med. Cell. Longev. 2017:6297080. doi: 10.1155/2017/6297080
Wu, L., Han, T., Li, W., Jia, M., Xue, L., Rahman, K., et al. (2013). Geographic and tissue influences on endophytic fungal communities of taxus chinensis var. mairei in China. Curr. Microbiol. 66, 40–48. doi: 10.1007/s00284-012-0235-z
Wu, Y. Z., Zhang, H. W., Sun, Z. H., Dai, J. G., Hu, Y. C., Li, R., et al. (2018). Bysspectin A, an unusual octaketide dimer and the precursor derivatives from the endophytic fungus Byssochlamys spectabilis IMM0002 and their biological activities. Eur. J. Med. Chem. 145, 717–725. doi: 10.1016/j.ejmech.2018.01.030
Yuan, X., Han, B., Feng, Z. M., Jiang, J. S., Yang, Y. N., and Zhang, P. C. (2020a). Chemical constituents of Ligusticum chuanxiong and their anti-inflammation and hepatoprotective activities. Bioorgan. Chem. 101:104016. doi: 10.1016/j.bioorg.2020.104016
Yuan, X., Han, B., Feng, Z. M., Jiang, J. S., Yang, Y. N., and Zhang, P. C. (2020b). Three new compounds from the rhizome of Ligusticum chuanxiong and their anti-inflammation activities. J. Asian Nat. Prod. Res. 22, 920–926. doi: 10.1080/10286020.2020.1803291
Zhang, Q. Q., Zhang, Y. H., Cai, F. Y., Liu, X. L., Chen, X. H., and Jiang, M. (2019). Comparative antibacterial and antibiofilm activities of garlic extracts, nisin, ε−polylysine, and citric acid on Bacillus subtilis. J. Food Process. Preserv. 43:e14179. doi: 10.1111/jfpp.14179
Zhang, X., Yan, H. W., Feng, Z. M., Yang, Y. N., Jiang, J. S., and Zhang, P. C. (2020). Neophathalides A and B, two pairs of unusual phthalide analog enantiomers from: Ligusticum chuanxiong. Organ. Biomol. Chem. 18, 5453–5457. doi: 10.1039/d0ob01014f
Zhao, L. X., Xu, L. H., and Jiang, C. L. (2012). Methods for the Study of Endophytic Microorganisms from Traditional Chinese Medicine Plants, 1st Edn. Amsterdam: Elsevier Inc., doi: 10.1016/B978-0-12-404634-4.00001-2
Zhao, X., Sun, H., Hou, A., Zhao, Q., Wei, T., and Xin, W. (2005). Antioxidant properties of two gallotannins isolated from the leaves of Pistacia weinmannifolia. Biochim. Biophys. Acta Gen. Subj. 1725, 103–110. doi: 10.1016/j.bbagen.2005.04.015
Keywords: chuanxiong, phenolic compounds, endophytic fungi, antioxidant activity, antimicrobial activity
Citation: Tang Z, Qin Y, Chen W, Zhao Z, Lin W, Xiao Y, Chen H, Liu Y, Chen H, Bu T, Li Q, Cai Y, Yao H and Wan Y (2021) Diversity, Chemical Constituents, and Biological Activities of Endophytic Fungi Isolated From Ligusticum chuanxiong Hort. Front. Microbiol. 12:771000. doi: 10.3389/fmicb.2021.771000
Received: 05 September 2021; Accepted: 19 October 2021;
Published: 17 November 2021.
Edited by:
Tofazzal Islam, Bangabandhu Sheikh Mujibur Rahman Agricultural University, BangladeshReviewed by:
Ali Chenari Bouket, Agricultural Research, Education and Extension Organization (AREEO), IranJayanta Kumar Patra, Dongguk University, South Korea
Copyright © 2021 Tang, Qin, Chen, Zhao, Lin, Xiao, Chen, Liu, Chen, Bu, Li, Cai, Yao and Wan. This is an open-access article distributed under the terms of the Creative Commons Attribution License (CC BY). The use, distribution or reproduction in other forums is permitted, provided the original author(s) and the copyright owner(s) are credited and that the original publication in this journal is cited, in accordance with accepted academic practice. No use, distribution or reproduction is permitted which does not comply with these terms.
*Correspondence: Zizhong Tang, MTQxMjZAc2ljYXUuZWR1LmNu; Hui Chen, Y2hlbjYyaHVpQDE2My5jb20=
†These authors have contributed equally to this work and share first authorship