- 1School of Applied Sciences, Edinburgh Napier University, Edinburgh, United Kingdom
- 2Red Sea Research Center, King Abdullah University of Science and Technology, Thuwal, Saudi Arabia
Introduction
The transition from an aquatic to a terrestrial lifestyle has evolved multiple times, and in numerous different phyla, in earth's history. In many crab species, this process is still underway (Bliss and Mantel, 1968), providing a unique opportunity to study the evolution of terrestrialization, as well as the role of associated microbiomes during this process (Cannicci et al., 2020). Recently, Cannicci et al. (2020) reported on the potential importance of microbiomes in the transition of crabs, formally wholly aquatic species, to life, fully or in part, in terrestrial environments. The authors argue that symbiotic bacteria, such as those of gill and gut microbiomes, may play a key role in easing this transition, by helping crabs to overcome physiological and morphological challenges associated with conquering the terrestrial environment, such as impaired respiration and osmotic regulation, and a new, often primary plant-based low nitrogen diet. Here we focus on the microbiomes of crab larvae and their potential role for the evolution of terrestrialization.
Crabs that are transitioning to life on land fall into two broad categories: terrestrial species that spend their whole adult life (except for larval release) on land independent of tidal inundation or freshwater bodies, and semi-terrestrial species that spend their adult life on land but are dependent on tidal inundation or freshwater (Burggren and McMahon, 1988; Anger, 1995). Many marine organisms form symbiotic relationships with microorganisms to aid life in extreme environments (Sogin et al., 2020). In line with the hologenome theory, this suggests that host-microbe interactions play an important role in an organism's evolution, where the genes of both the host and its microbes co-evolve in the collective “holobiont” (Zilber-Rosenberg and Rosenberg, 2008), potentially allowing the colonization of formerly hostile environments (Bang et al., 2018). Microbial symbionts, as individual species or in mixed-species assemblages, are present in many crustaceans, such as the marine isopod Idotea balthica (diet-specific gut microbiomes, Mattila et al., 2014), the intertidal brachyuran crab Eriocheir sinensis (gill and gut microbiomes, Zhang et al., 2016), and the freshwater signal crayfish Pacifastacus leniusculus (intestinal bacteria, Hernández-Pérez et al., 2021). Given that microbial assemblages are often specific to certain organs of their hosts (Chomicki et al., 2020), symbioses have likely evolved in support of a specific function.
The microbial assemblages associated with the guts of semi-terrestrial crabs have been proposed to aid in the adaptation of a low nitrogen, herbivorous diet during terrestrialization (Bui and Lee, 2015), like microbial assemblages of other aquatic invertebrates, e.g., isopods, where they enable the digestion of cellulose (Zimmer et al., 2002; O'Connor et al., 2014). The bacteria specifically associated with crab gills (Zhang et al., 2016, 2017) may facilitate ammonia excretion (Weihrauch et al., 2004), utilize gaseous CO2 (Morris, 2001), and buffer exposure to oxygen, which occurs at a concentration 30 times higher (Hsia et al., 2013) in the terrestrial compared to the marine environment where the host organism evolved. The microbiomes of both gut and gills could therefore provide terrestrial and semi-terrestrial crabs (here collectively called semi-/terrestrial) with means to cope with life in marine as well as in terrestrial environments. Whilst the presence of microbiomes and their role in buffering the stresses imposed on crabs by terrestrialization is beginning to be discussed (Bui and Lee, 2015; Cannicci et al., 2020), there are many unknowns. For example, the mode of bacterial acquisition, bacterial diversity, topological association, and the precise functions of their organ-specific microbial assemblages are still poorly understood, both for adult semi-/terrestrial crabs and their early life stages.
Most semi-/terrestrial crab species, like their aquatic counterparts, have a biphasic life cycle including fully aquatic larvae, via which they transition to semi-/terrestrial juvenile/adult life (Anger, 1995; Hartnoll et al., 2014). Understanding microbial colonization of the larvae would likely provide critical insights into how these crabs have been able to move from water to land, and whether the bacteria themselves facilitate this transition.
Larvae, the Vector Between Water and Land, and Their Potential Microbiome Acquisition Pathways
Most semi-/terrestrial crab species are broadcast spawners with pelagic larvae that develop for 3–6 weeks in the marine environment (Bliss and Mantel, 1968; Anger, 1995), undergoing multiple zoeal stages and one megalopal stage, mirroring the life history characteristics of fully aquatic crabs. In some semi-/terrestrial species, larval development is abbreviated (Anger, 1995; González-Gordillo et al., 2010; Vogt, 2013) and/or maternal brood care increased. For example, the larvae of the fiddler crab Uca subcylindrica, a species adapted to semiarid habitats, develop inside non-permanent water bodies to megalopae within as little as 2.5 days (Rabalais and Cameron, 1983). The Jamaican freshwater bromeliad crab Metopaulias depressus also undergoes abbreviated development; its larvae are released into phytothelmes and are actively guarded by their mothers (Diesel, 1989; Diesel and Schuh, 1993). Semi-/terrestrial crabs with larvae that (still) need to develop in the sea act as vectors at the marine-terrestrial interface. Some species travel considerable distances over land from their inland habitats to the coast, e.g., the inland forest-dwelling Christmas Island crab Gecarcoidea natalis travels up to 4 km (Adamczewska and Morris, 2001) to release its larvae into the sea. In the sea, the offspring of semi-/terrestrial crabs are preyed upon by aquatic predators and consume plankton to acquire biomass that is brought back to the land when the megalopae settle and metamorphose into the benthic juvenile stage. Hence, semi-/terrestrial crab larvae are couplers of nutrients, and likely microbiomes, between aquatic and terrestrial ecosystems, with each successive generation having to make the transition from a life in water to a life on land. If, as recently suggested (Cannicci et al., 2020), symbiotic microbiomes facilitate the evolution from an aquatic to a terrestrial life, then these larval stages provide a unique opportunity to explore this transition firsthand.
Bacterial colonization of marine invertebrate larvae has been evidenced as an essential step in their adaptation to specific, often extreme, environments. For example, once settled, the larvae and juveniles of the gutless giant tube worm Riftia spp. are colonized by sulfur-oxidizing bacteria (Nussbaumer et al., 2006). Their acquisition promotes developmental changes in the juvenile worm, including the growth of specific tissues for the bacteria to colonize. The endosymbiotic bacteria within these tissues facilitate sulfur-oxidation (Minic and Hervé, 2004), allowing the worms to survive in hydrothermal vent ecosystems. In some species, such as the sponge Amphimedon queenslandica, settlement and metamorphosis to benthic juvenile stage are facilitated by the larvae's microbiota (Song et al., 2021). These vertically acquired symbiotic bacteria produce arginine, the substrate needed for nitric oxide synthesis and the signal that regulates settlement and metamorphosis in many invertebrate species. Bacterial colonization plays a key role in the larvae of the intertidal barnacle Semibalanus balanoides, which are colonized during the settlement phase of the cyprid stage. The cypris' microbiome composition changes upon settlement, with potential implications for growth and survival during the barnacle's benthic life stage (Aldred and Nelson, 2019). In other marine crustacean species embryos and larvae are colonized by specific symbiotic bacteria that increase protection from pathogenic fungi and bacteria (Gil-Turnes et al., 1989—Palaemon macrodactylus; Gil-Turnes and Fenical, 1992—Homarus americanus), and in Bryozoa bacteria reduce larval predation risk through the production of cytotoxins (Lopanik et al., 2004—Bugula neritina).
While microbiomes have not been found in all animal species studied (including crustacean larvae) (Hammer et al., 2019; Martin et al., 2020), there is evidence that when present in marine invertebrate larvae, symbiotic relationships are often formed. It is important to comprehend at which developmental stage these microbes are acquired if we are to understand whether and how these relationships aid the transition between life stages and contribute to the evolution toward terrestrialization.
Larval microbiomes of semi-/terrestrial crabs may be obtained horizontally from the surrounding environment, or vertically, through their parents (Figure 1). Horizontal bacterial transmission can occur either during the pelagic stages from the surrounding water (Lopanik et al., 2004; Hadfield, 2021) or upon first settlement (Nussbaumer et al., 2006; Aldred and Nelson, 2019) e.g., at the megalopa stage. Megalopae of many semi-/terrestrial crabs spend considerable time on the sediment surface, ahead of their metamorphosis into benthic juveniles (Anger, 2001; Hamasaki et al., 2015) and settlement is often driven by specific environmental and conspecific cues present in the sediment or the water layer above (Diele and Simith, 2007; Simith et al., 2013), possibly including substances emitted by bacterial biofilms (Simith et al., 2017). The period that the megalopae spend on the sediment before metamorphosing to the first juvenile crab stage may therefore provide an ideal time for the acquisition of bacteria from this substrate (Lim et al., 2019). Evidence of sediment bacteria transfer has been shown in adult fiddler crabs, Uca panacea, where the crabs' microbiome is populated by bacteria from their burrows (carapace microbiome) and the sediment surface (carapace and gut microbiome) (Cuellar-Gempeler and Leibold, 2019).
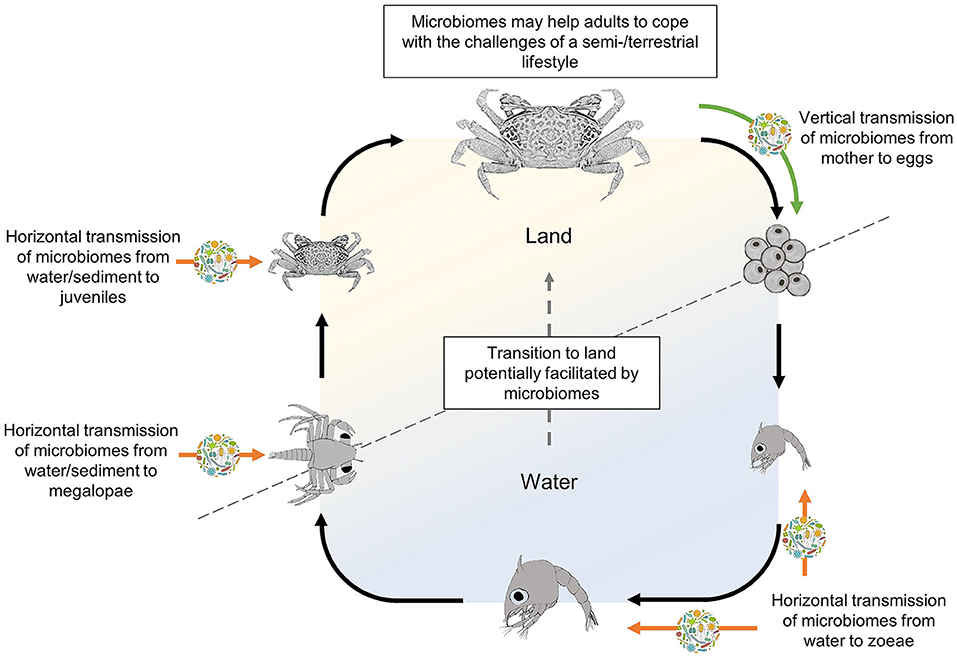
Figure 1. Life cycle of a semi-terrestrial crab (here represented as a female fiddler crab; subfamily Gelasiminae, taken as a model species; adapted after Peer et al., 2015) indicating the potential time points of vertical and horizontal acquisition of symbiotic microbiomes that may facilitate the transition from a life in water to one on land. Green and orange arrows indicate potential vertical and horizontal transmission routes of symbiotic microorganisms, respectively. Black arrows indicate the model life cycle from egg, through larvae, to adult crab.
Alternatively, vertical transmission of a larva's microbiome is generally through the mother, either from her microbiota or that received from the father during mating (Damiani et al., 2008). Given the conservative broadcast spawning and pelagic larval stages of most semi-/terrestrial crabs, vertical transmission would have to occur through symbiont association with adult gametes (Russell et al., 2018) or through an association between the microbes and the females' ovaries. Egg associated microbiomes that act as the vertical transmission point for symbionts (Nyholm, 2020) have been evidenced for the deep-sea yeti crab, Kiwa puravida (Goffredi et al., 2014), and the hydrothermal vent shrimp, Rimicaris exoculata (Methou et al., 2019). In semi-/terrestrial crabs, microbes that are transmitted through the mother's eggs would colonize their target organ upon hatching or, if such organ has not yet developed at this developmental stage (e.g., gills), persist internally or on the larvae's carapace until formed. If vertical transmission is occurring in semi-/terrestrial crabs, it would indicate a complex symbiotic relationship where the crabs' entire life cycles are intertwined with their microbiome.
Given the large number of crab species that have conquered the land but retained pelagic larvae that develop in the oceans, we advocate the importance of integrating the early life history stages into future studies on microbiomes as a paradigm for the evolution of terrestrialization in crabs. Identifying whether, when, and under which acquisition pathway microbiome transmission is occurring in the early life history of species with biphasic life cycles has the potential to significantly enhance our understanding of the contribution of animal-microbiome interactions in the crabs' transition from a life in water onto land.
Author Contributions
MW and KD conceived the idea for the paper. MW led the manuscript drafting, with input from all co-authors.
Funding
This work received funding from King Abdullah University of Science and Technology Competitive Research Grant Program 2018—OSR-CRG 2018-3739.
Conflict of Interest
The authors declare that the research was conducted in the absence of any commercial or financial relationships that could be construed as a potential conflict of interest.
Publisher's Note
All claims expressed in this article are solely those of the authors and do not necessarily represent those of their affiliated organizations, or those of the publisher, the editors and the reviewers. Any product that may be evaluated in this article, or claim that may be made by its manufacturer, is not guaranteed or endorsed by the publisher.
Acknowledgments
The authors would like to acknowledge the support of their host institutions and colleagues from the Extreme Systems Microbiology lab for helpful discussion.
References
Adamczewska, A. M., and Morris, S. (2001). Metabolic status and respiratory physiology of Gecarcoidea natalis, the Christmas Island red crab, during the annual breeding migration. Biol. Bull. 200, 321–335. doi: 10.2307/1543513
Aldred, N., and Nelson, A. (2019). Microbiome acquisition during larval settlement of the barnacle Semibalanus balanoides. Biol. Lett. 15:20180763. doi: 10.1098/rsbl.2018.0763
Anger, K. (1995). The conquest of freshwater and land by marine crabs: adaptations in life-history patterns and larval bioenergetics. J. Exp. Mar. Bio. Ecol. 193, 119–145. doi: 10.1016/0022-0981(95)00114-X
Bang, C., Dagan, T., Deines, P., Dubilier, N., Duschl, W. J., Fraune, S., et al. (2018). Metaorganisms in extreme environments: do microbes play a role in organismal adaptation? Zoology 127, 1–19. doi: 10.1016/j.zool.2018.02.004
Bliss, D. E., and Mantel, L. H. (1968). Adaptations of crustaceans to land: a summary and analysis of new findings. Integr. Comp. Biol. 8, 673–685. doi: 10.1093/icb/8.3.673
Bui, T. H. H., and Lee, S. Y. (2015). Potential contributions of gut microbiota to the nutrition of the detritivorous sesarmid crab Parasesarma erythodactyla. Mar. Biol. 162, 1969–1981. doi: 10.1007/s00227-015-2723-8
Burggren, W. W., and McMahon, B. R. (1988). Biology of Land Crabs. Cambridge: Cambridge University Press.
Cannicci, S., Fratini, S., Meriggi, N., Bacci, G., Iannucci, A., Mengoni, A., et al. (2020). To the land and beyond: crab microbiomes as a paradigm for the evolution of terrestrialization. Front. Microbiol. 11:575372. doi: 10.3389/fmicb.2020.575372
Chomicki, G., Werner, G. D. A., West, S. A., and Kiers, E. T. (2020). Compartmentalization drives the evolution of symbiotic cooperation. Philos. Trans. R. Soc. B 375:20190602. doi: 10.1098/rstb.2019.0602
Cuellar-Gempeler, C., and Leibold, M. A. (2019). Key colonist pools and habitat filters mediate the composition of fiddler crab–associated bacterial communities. Ecology 100, 1–14. doi: 10.1002/ecy.2628
Damiani, C., Ricci, I., Crotti, E., Rossi, P., Rizzi, A., Scuppa, P., et al. (2008). Paternal transmission of symbiotic bacteria in malaria vectors. Curr. Biol. 18, 1087–1088. doi: 10.1016/j.cub.2008.10.040
Diele, K., and Simith, D. J. B. (2007). Effects of substrata and conspecific odour on the metamorphosis of mangrove crab megalopae, Ucides cordatus (Ocypodidae). J. Exp. Mar. Bio. Ecol. 348, 174–182. doi: 10.1016/j.jembe.2007.04.008
Diesel, R. (1989). Parental care in an unusual environment: Metopaulias depressus (Decapoda: Grapsidae), a crab that lives in epiphytic bromeliads. Anim. Behav. 38, 561–575. doi: 10.1016/S0003-3472(89)80001-6
Diesel, R., and Schuh, M. (1993). Maternal care in the bromeliad crab Metopaulias depressus (Decapoda): maintaining oxygen, pH and calcium levels optimal for the larvae. Behav. Ecol. Sociobiol. 32, 11–15. doi: 10.1007/BF00172218
Gil-Turnes, M. S., and Fenical, W. (1992). Embryos of Homarus americanus are protected by epibiotic bacteria. Biol. Bull. 182, 105–108. doi: 10.2307/1542184
Gil-Turnes, M. S., Hay, M. E., and Fenical, W. (1989). Symbiotic marine bacteria chemically defend crustacean embryos from a pathogenic fungus. Science 246, 116–118. doi: 10.1126/science.2781297
Goffredi, S. K., Gregory, A., Jones, W. J., Morella, N. M., and Sakamoto, R. I. (2014). Ontogenetic variation in epibiont community structure in the deep-sea yeti crab, Kiwa puravida: convergence among crustaceans. Mol. Ecol. 23, 1457–1472. doi: 10.1111/mec.12439
González-Gordillo, J. I., Anger, K., and Schubart, C. D. (2010). Morphology of the larval and first juvenile stages of two Jamaican endemic crab species with abbreviated development, Sesarma windsor and Metopaulias depressus (Decapoda: Brachyura: Sesarmidae). J. Crustac. Biol. 30, 101–121. doi: 10.1651/08-3110.1
Hadfield, M. G. (2021). Developmental symbiosis: a sponge larva needs symbiotic bacteria to succeed on the benthos. Curr. Biol. 31, R88–R90. doi: 10.1016/j.cub.2020.11.007
Hamasaki, K., Ishiyama, N., and Kitada, S. (2015). Settlement behavior and substrate preference of the coconut crab Birgus latro megalopae on natural substrata in the laboratory. J. Exp. Mar. Bio. Ecol. 468, 21–28. doi: 10.1016/j.jembe.2015.03.011
Hammer, T. J., Sanders, J. G., and Fierer, N. (2019). Not all animals need a microbiome. FEMS Microbiol. Lett. 366, 1–11. doi: 10.1093/femsle/fnz117
Hartnoll, R. G., Weber, N., Régnier-Mckellar, C., and Weber, S. B. (2014). Return to the land; the stages of terrestrial recruitment in land crabs. Crustaceana 87, 531–539. doi: 10.1163/15685403-00003294
Hernández-Pérez, A., Zamora-briseño, J. A., Söderhäll, K., and Söderhäll, I. (2021). Gut microbiome alterations in the crustacean Pacifastacus leniusculus exposed to environmental concentrations of antibiotics and effects on susceptibility to bacteria challenges. Dev. Comp. Immunol. 126:104181. doi: 10.1016/j.dci.2021.104181
Hsia, C. C. W., Schmitz, A., Lambertz, M., Perry, S. F., and Maina, J. N. (2013). Evolution of air breathing: oxygen homeostasis and the transitions from water to land and sky. Compr. Physiol. 3, 849–915. doi: 10.1002/cphy.c120003
Lim, S. J., Davis, B. G., Gill, D. E., Walton, J., Nachman, E., Engel, A. S., et al. (2019). Taxonomic and functional heterogeneity of the gill microbiome in a symbiotic coastal mangrove lucinid species. ISME J. 13, 902–920. doi: 10.1038/s41396-018-0318-3
Lopanik, N., Lindquist, N., and Targett, N. (2004). Potent cytotoxins produced by a microbial symbiont protect host larvae from predation. Oecologia 139, 131–139. doi: 10.1007/s00442-004-1487-5
Martin, G. G., Natha, Z., Henderson, N., Bang, S., Hendry, H., and Loera, Y. (2020). Absence of a microbiome in the midgut trunk of six representative Crustacea. Crustac. Biol. 40, 122–130. doi: 10.1093/jcbiol/ruz087
Mattila, J. M., Zimmer, M., Vesakoski, O., and Jormalainen, V. (2014). Habitat-specific gut microbiota of the marine herbivore Idotea balthica (Isopoda). J. Exp. Mar. Bio. Ecol. 455, 22–28. doi: 10.1016/j.jembe.2014.02.010
Methou, P., Hernández-Ávila, I., Aube, J., Cueff-Gauchard, V., Gayet, N., Amand, L., et al. (2019). Is it first the egg or the shrimp? – Diversity and variation in microbial communities colonizing broods of the vent shrimp Rimicaris exoculata during embryonic development. Front. Microbiol. 10:808. doi: 10.3389/fmicb.2019.00808
Minic, Z., and Hervé, G. (2004). Biochemical and enzymological aspects of the symbiosis between the deep-sea tubeworm Riftia pachyptila and its bacterial endosymbiont. Eur. J. Biochem. 271, 3093–3102. doi: 10.1111/j.1432-1033.2004.04248.x
Morris, S. (2001). Neuroendocrine regulation of osmoregulation and the evolution of air-breathing in decapod crustaceans. J. Exp. Biol. 204, 979–989. doi: 10.1242/jeb.204.5.979
Nussbaumer, A. D., Fisher, C. R., and Bright, M. (2006). Horizontal endosymbiont transmission in hydrothermal vent tubeworms. Nature 441, 345–348. doi: 10.1038/nature04793
Nyholm, S. V. (2020). In the beginning: egg-microbe interactions and consequences for animal hosts. Philos. Trans. R. Soc. B Biol. Sci. 375:20190593. doi: 10.1098/rstb.2019.0593
O'Connor, R. M., Fung, J. M., Sharp, K. H., Benner, J. S., McClung, C., Cushing, S., et al. (2014). Gill bacteria enable a novel digestive strategy in a wood-feeding mollusk. Proc. Natl. Acad. Sci. U.S.A. 111, E5096–E5104. doi: 10.1073/pnas.1413110111
Peer, N., Miranda, N. A. F., and Perissinotto, R. (2015). A review of fiddler crabs (genus Uca Leach, 1814) in South Africa. African Zool. 50, 187–204. doi: 10.1080/15627020.2015.1055700
Rabalais, N. N., and Cameron, J. N. (1983). Abbreviated development of Uca subcylindrica (Stimpson, 1859) (Crustacea, Decapoda, Ocypodidae) reared in the laboratory. J. Crustac. Biol. 3, 519–541. doi: 10.2307/1547948
Russell, S. L., McCartney, E., and Cavanaugh, C. M. (2018). Transmission strategies in a chemosynthetic symbiosis: detection and quantification of symbionts in host tissues and their environment. Proc. R. Soc. B Biol. Sci. 285:20182157. doi: 10.1098/rspb.2018.2157
Simith, D. D. J. D. B., Abrunhosa, F. A., and Diele, K. (2013). Chemical induction in mangrove crab megalopae, Ucides cordatus (Ucididae): do young recruits emit metamorphosis-triggering odours as do conspecific adults? Estuar. Coast. Shelf Sci. 131, 264–270. doi: 10.1016/j.ecss.2013.07.015
Simith, D. D. J. D. B., Abrunhosa, F. A., and Diele, K. (2017). Metamorphosis of the edible mangrove crab Ucides cordatus (Ucididae) in response to benthic microbial biofilms. J. Exp. Mar. Bio. Ecol. 492, 132–140. doi: 10.1016/j.jembe.2017.01.022
Sogin, E. M., Leisch, N., and Dubilier, N. (2020). Chemosynthetic symbioses. Curr. Biol. 30, R1137–R1142. doi: 10.1016/j.cub.2020.07.050
Song, H., Hewitt, O. H., and Degnan, S. M. (2021). Arginine biosynthesis by a bacterial symbiont enables nitric oxide production and facilitates larval settlement in the marine-sponge host. Curr. Biol. 31, 433–437.e3. doi: 10.1016/j.cub.2020.10.051
Vogt, G. (2013). Abbreviation of larval development and extension of brood care as key features of the evolution of freshwater Decapoda. Biol. Rev. 88, 81–116. doi: 10.1111/j.1469-185X.2012.00241.x
Weihrauch, D., Morris, S., and Towle, D. W. (2004). Ammonia excretion in aquatic and terrestrial crabs. J. Exp. Biol. 207, 4491–4504. doi: 10.1242/jeb.01308
Zhang, M., Sun, Y., Chen, L., Cai, C., Qiao, F., Du, Z., et al. (2016). Symbiotic bacteria in gills and guts of Chinese mitten crab (Eriocheir sinensis) differ from the free-living bacteria in water. PLoS ONE 11:e0148135. doi: 10.1371/journal.pone.0148135
Zhang, N., Song, C., Wang, M., Liu, Y., Hui, M., and Cui, Z. (2017). Diversity and characterization of bacteria associated with the deep-sea hydrothermal vent crab Austinograea sp. comparing with those of two shallow-water crabs by 16S ribosomal DNA analysis. PLoS ONE 12:e0187842. doi: 10.1371/journal.pone.0187842
Zilber-Rosenberg, I., and Rosenberg, E. (2008). Role of microorganisms in the evolution of animals and plants: the hologenome theory of evolution. FEMS Microbiol. Rev. 32, 723–735. doi: 10.1111/j.1574-6976.2008.00123.x
Keywords: terrestrial crabs, symbiotic microbiota, hologenome theory, microbiome transmission, larval development
Citation: Wale M, Daffonchio D, Fusi M, Marasco R, Garuglieri E and Diele K (2021) The Importance of Larval Stages for Considering Crab Microbiomes as a Paradigm for the Evolution of Terrestrialization. Front. Microbiol. 12:770245. doi: 10.3389/fmicb.2021.770245
Received: 03 September 2021; Accepted: 15 September 2021;
Published: 15 October 2021.
Edited by:
Jin Sun, Ocean University of China, ChinaReviewed by:
Tyler J. Carrier, GEOMAR Helmholtz Center for Ocean Research Kiel, GermanyAriadne Hernández-Pérez, National Autonomous University of Mexico, Mexico
Copyright © 2021 Wale, Daffonchio, Fusi, Marasco, Garuglieri and Diele. This is an open-access article distributed under the terms of the Creative Commons Attribution License (CC BY). The use, distribution or reproduction in other forums is permitted, provided the original author(s) and the copyright owner(s) are credited and that the original publication in this journal is cited, in accordance with accepted academic practice. No use, distribution or reproduction is permitted which does not comply with these terms.
*Correspondence: Matthew Wale, bS53YWxlQG5hcGllci5hYy51aw==; Karen Diele, ay5kaWVsZUBuYXBpZXIuYWMudWs=