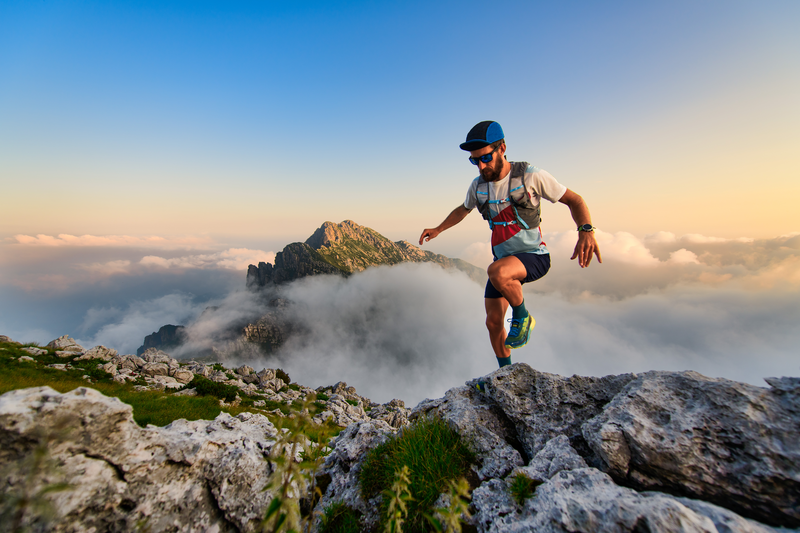
95% of researchers rate our articles as excellent or good
Learn more about the work of our research integrity team to safeguard the quality of each article we publish.
Find out more
ORIGINAL RESEARCH article
Front. Microbiol. , 21 October 2021
Sec. Microbiotechnology
Volume 12 - 2021 | https://doi.org/10.3389/fmicb.2021.767639
This article is part of the Research Topic Advances in Bioleaching and Bioremediation View all 21 articles
Various strategies to accelerate the formation of biofilms on minerals have been studied, and one of them is the use of D-galactose as an inducer of EPS production in planktonic cells of biooxidant bacteria. With the aim to evaluate the influence on the attachment and the effect over the solubilization of a polymetallic mineral concentrate, the behavior of a microbial consortium formed by Acidithiobacillus thiooxidans DSM 14887T and Leptospirillum ferrooxidans DSM 2705T previously induced with D-galactose for the early formation of EPS was studied. These microorganisms were previously adapted to 0.15 and 0.25% of D-galactose, respectively; afterward, different proportions of both strains were put in contact with the particle surface of a concentrate of polymetallic mineral. Also, to evaluate the affinity of each bacterium to the mineral, attachment tests were carried out with one of these species acting as a pre-colonizer. The same consortia were used to evaluate the solubilization of the polymetallic mineral. The results obtained show that the induction by D-galactose increases the microbial attachment percentage to the mineral by at least 10% with respect to the control of non-adapted consortia. On the other hand, the tests carried out with pre-colonization show that the order of inoculation also affects the microbial attachment percentage. From the different proportions tested, it was determined that the use of a consortium with a proportion of 50% of each species previously adapted to D-galactose and inoculated simultaneously, present a microbial attachment percentage to the mineral greater than 95% and better solubilization of a polymetallic mineral, reaching values of 9.7 and 11.7mgL−1 h−1 of Fe3+ and SO42−, respectively. Therefore, the use of D-galactose in small concentrations as inducer of EPS in acidophilic cells and the selection of an adequate strategy of inoculation can be beneficial to improve biooxidation since it would allow this process to develop in a shorter time by achieving a greater number of attached cells in a shorter time accelerating the solubilization of a sulfide mineral.
Biooxidation processes have become increasingly important for the recovery of valuable metals from low-grade minerals, where conventional processing is not feasible (Gentina and Acevedo, 2016). Chemolithoautotrophic bacteria, such as Leptospirillum (L.) ferrooxidans and Acidithiobacillus (At.) thiooxidans (Nemati and Harrison, 2000), are used for this purpose since they are capable of oxidizing iron and sulfur compounds, respectively, to obtain energy. During this process, bacteria play an important role in biooxidation through the regeneration of ferric iron (Fe3+) and the production of sulfuric acid. When they attach to the mineral and oxidize the ferrous ion (Fe2+), they generate ferric iron (Fe3+) which solubilizes the sulfur minerals in an acidic environment where the metals of interest are occluded; this occurs at the interface of the extracellular polymeric substances (EPS) produced by microorganisms and the mineral. This has been used, as an example, to release gold from sulfide matrix and its subsequent leaching with cyanide (Vera et al., 2013; Saavedra et al., 2020).
The attachment of microorganisms to the surface of minerals is a key step for the biooxidation process to take place (Bellenberg et al., 2014a). During this stage, microorganisms are organized into complex structures called biofilms (Lara et al., 2010). In a biofilm, the microorganisms are embedded in EPS that constitute a biochemical reaction space for biooxidation, which occurs in the microenvironment generated by the biofilm and the Fe3+ ions that are located between the cell walls of the microorganisms and the surface of metal sulfides (Sand and Gehrke, 2006).
In the case of biooxidant microorganisms, biofilm formation allows them to bind to the mineral surface through electrostatic forces and hydrophobic interactions, thus facilitating the oxidizing action of Fe3+ on metal sulfide (Karatan and Watnick, 2009; Aguirre Morales, 2012). In turn, EPS play an important role in the initial stages of the mineral attachment process and biofilm formation (Gehrke et al., 1998; Sand et al., 2001). Subsequently, biooxidant microorganisms which do not form EPS need a longer time to attach to the mineral surface and consolidate the biofilm. In addition, attachment rates are lower for cells that previously formed EPS (Arredondo et al., 1994; Saavedra et al., 2013). Therefore, microbial attachment and subsequent biofilm formation on the mineral surface play a fundamental role in the biooxidation processes of minerals (Rodríguez et al., 2003). In this regard, attachment strategies based on the induction of EPS have been proposed as an adaptive response to substances that present toxicity such as D-galactose (Silver et al., 1967; Barreto et al., 2005).
On the other hand, it has been observed that there are changes in the composition of the microbial communities present in the biooxidation processes; however, the interactions among the different biooxidant species that coexist in the biofilms formed on sulfur minerals are generally unknown (Bellenberg et al., 2014a). It has been shown that biofilms from different ecological habitats suffer a succession of colonizers where the former produces EPS that can promote the attachment of other species (Elifantz et al., 2013).
According to Castro et al. (2016), the attachment of the cells and the subsequent formation of the biofilm depends on both, the substrate and the species involved in the process. These researchers, in their work with pre-colonization coupled with the inoculation of a second species to generate a consortium of Acidianus spp. and Sulfolobus metallicus, suggest that both species mutually influence each other with respect to the initial adherence speed. Similarly, Liu et al. (2016) showed the existence of interspecies interactions during the initial attachment of a model consortium formed by Sulfobacillus thermosulfidooxidans DSM 9293T and Acidianus sp. DSM 29099. The work of Rawlings and Johnson (2007) has shown that mixed cultures are more efficient in the bioleaching process than pure cultures as they improve the robustness of bioleaching and resistance to disturbances. A set of relevant microbial populations could potentially reduce the operational cost in bioleaching and biooxidation processes, which would otherwise occur when unwanted microbial communities are present (Rawlings and Johnson, 2007). Thus, Falco et al. (2003) developed covellite bioleaching tests showing that mineral solubilization performed better with a microbial consortium including At. thiooxidans and L. ferrooxidans.
Given the fact that various authors have shown that for bioleaching bacteria the attachment speed depends on factors such as the substrate, the species involved, the production of EPS, among others (Harneit et al., 2006; Kock and Schippers, 2008; Yang et al., 2015), it is important to study the microbial behavior in terms of attachment percentage to the mineral and their performance when they are established as a consortium. By virtue of these antecedents, the objective of this work was to evaluate the behavior of a model microbial consortium containing the bacteria At. thiooxidans DSM 14887T and L. ferrooxidans DSM 2705T previously adapted to D-galactose for the early formation of EPS and their influence on the attachment percentage and subsequently their effect over the solubilization of iron and sulfur from a concentrate of polymetallic mineral.
The microorganisms used were At. thiooxidans DSM 14887T and L. ferrooxidans DSM 2705T. Cultivation was carried out in 250-ml Erlenmeyer flasks containing 50ml of a culture medium with the following composition in g·L−1: KCl 0.004, MgSO4·7H2O 0.005, (NH4)2HPO4 0.15, CaCl2 0.0012 (Kim et al., 2002), supplemented with 44g·L−1 FeSO4·7H2O for L. ferrooxidans DSM 2705T or 10g·L−1 S° for At. thiooxidans DSM 14887T. The culture conditions were 30°C and 180rpm, at pH 1.8 and 1.3 for At. thiooxidans DSM 14887T and L. ferrooxidans 2705T, respectively.
A polymetallic mineral concentrate was used for the attachment tests. The mineral was obtained from Bella Rica, Azuay Province (Ecuador). X-ray diffraction (XRD) analysis of the concentrate revealed the following composition in %: 30.23 quartz, 34.26 pyrite, 0.41 chalcopyrite, 0.95 galena, 3.41 sphalerite, 15.19 orthoclase, 8.24 gypsum, 0.89 apatite-(Sr-OH), 2.41 pyrrhotite, 2.61 arsenopyrite, 0.92 cobalt pentlandite, and 0.46 magnetite. The mineral used was crushed to a particle size of 75μm verified by the D80 of the ASTM # 200 sieve.
The mineral was acidified to pH 1.8 with a solution of 10N H2SO4, dried, and exposed to a N2 atmosphere to avoid chemical oxidation. Finally, it was sterilized at 121°C for 24h with dry heat (Bellenberg et al., 2014b).
At. thiooxidans DSM 14887T and L. ferrooxidans DSM 2705T were previously adapted to 0.15%, 0.25, and 0.35% of D-galactose as described in Aguirre et al. (2017 , 2018). The maximum specific growth rate (μmax) and the yield of biomass (YX/S) were calculated for each adapted strain (see Supplementary Material). The amount of EPS produced by each species was extracted using Dowex cationic exchange resin (CER DOWEX Marathon C, SIGMA) according to Bellenberg et al. (2014b), and then, it was quantified by measuring of its main components: proteins and carbohydrates. Protein concentration was determined by the Bradford method (Bradford, 1976) and carbohydrates by the phenol/sulfuric acid method (Dubois et al., 1956).
To evaluate the microbial attachment to the mineral surface, different consortia were formed with At. thiooxidans DSM 14887T and L. ferrooxidans DSM 2705T adapted to D-galactose, while non-adapted cells were used to form consortia that acted as control. Mixtures of both strains in proportions of 30:70, 50:50, and 70:30 simulated the different microbial consortia. In all cases, a total of 1·108 cells·ml−1 were initially inoculated.
Experiments were carried out in 250-ml flasks containing 50ml of Kim medium (without addition of D-galactose, iron, and elemental sulfur) supplemented with 2% w/v of a polymetallic mineral concentrate and inoculated with a total of 1·108 cells·ml−1 in accordance with the consortia established before. For the evaluation of the attachment percentage, the cultures were incubated for 7h using an orbital shaker at 30°C and 180rpm, and samples were taken at 1, 30, 180, and 420min.
To quantify microbial attachment, 5ml of each sample was centrifuged at 200rpm for 2min (Prism C2500, Labnet International, Edison, NJ, United States), and then, the supernatant was separated from the mineral solid precipitate. Number of attached cells to the minerals were determined by quantitative real-time PCR (qPCR) for each species after DNA extraction from mineral pellet. To determine the percentage of attached cells, the number of DNA copies obtained at each time was contrasted with respect to the initial number of DNA copies of each species. Additionally, the total number of planktonic cells present in the supernatant was quantified by direct counting in Neubauer chamber.
Attachment tests were carried out with pre-colonization of the mineral. The attachment method consisted of an inoculating process in two consecutive stages, in such a way that the first inoculated strain acted as a pre-colonizer. Both species were tested as pre-colonizers by independent cultures, and thus, when L. ferrooxidans DSM 2705T acted as a pre-colonizer, At. thiooxidans DSM 14887T was the secondary colonizer and vice versa. This test was carried out using adapted and non-adapted to D-galactose At. thiooxidans DSM 14887T and L. ferrooxidans DSM 2705T.
Experiments were carried out in 250-ml Erlenmeyer flasks with 50ml of Kim medium (without addition of D-galactose, iron, and elemental sulfur) supplemented with 2% w/v of a polymetallic mineral concentrate. To start the first stage of culture, 5·107 cells·ml−1 of the pre-colonizing strain were added; after 60min, the supernatant was separated from the mineral by centrifugation at 200rpm and fresh Kim medium was added together with the same number of cells of the second species. The cultures were kept for 3h at 30°C and 180rpm. Samples were taken after 1, 60, 61, 91, and 181min. For each sample, the supernatant was separated by centrifugation at 200rpm for 2min to quantify the total planktonic cells and the solid was recovered for the determination of the number of attached cells by qPCR for each species. To determine the percentage of attached cells, the number of DNA copies obtained at each time was contrasted with respect to the initial number of DNA copies of each species.
DNA extraction from the bacterial cultures was carried out with the DNA easy Ultraclean Microbial Kit (QIAGEN, Hilden, Germany) according to the protocol suggested by the manufacturer, and the quality and concentration of the extracted DNA were verified using a NanoDrop (BioSpec-Nano, Shimadzu Corporation, Kyoto, Japan). Genomic DNA extraction from colonized solid samples was performed with the Power Soil kit (Mobio Laboratories, Carlsbad, CA, United States).
Primers were designed to quantify the presence of At. thiooxidans DSM 14887T and L. ferrooxidans DSM 2705T (Table 1). For this, the 16S rRNA gene sequence of reference strains was obtained from the NCBI (National Center for Biotechnology Information) databased and used for a sequence alignment using PHYDE software (Phylogenetic Data Editor). Primer sequences of 18–22 nucleotides were selected; the Guanine/Cytosine content and the annealing temperature were verified by means of Oligocalculator software, as well as the quality and specificity by Primer-BLAST simulator. For final verification, a PCR reaction with DNA obtained from each species was carried out under the determined conditions and the resulting PCR product checked on a 1% agarose gel (see Supplementary Figure S2 in Supplementary Material).
Table 1. Designed primers for the quantification by qPCR of bacterial species of At. thiooxidans DSM 14887T and L. ferrooxidans DSM 2705T.
Using the designed primers (Table 1), a standard threshold cycle curve (Ct) (10−2–10−8) was constructed regarding the number of gene copies (Hedrich et al., 2016). All tests were performed in triplicate in a real-time PCR equipment (AriaMX, Agilent Technologies, Santa Clara, CA, United States). Reactions were performed in a total volume of 10μl containing 5μl of Brilliant II SYBR Green qPCR Master Mix, 2.7μl of double distilled water, 0.4μl of each primer, 0.5μl of BSA, and 1μl of DNA sample. The conditions of each cycle were initial denaturation at 95°C for 10min, 40 amplification cycles consisting of denaturation at 95°C for 15s and alignment for 1min at 60°C. The melting curve was constructed after reaching the qPCR runs using the following parameters: a cycle of 95°C for 30s and 72°C for 1min followed by increasing the temperature up to 95°C in 0.3°C increments. The efficiency of the amplification was verified for the precision of the tests, and it was determined by calculating the variation in the Ct values in the replicated samples and their SD.
Biooxidation test were developed in 250-ml Erlenmeyer flasks containing Kim medium with 2% w/v of previously sterilized mineral. All the conditions established for the attachment tests of the microbial consortia of the species L. ferrooxidans DSM 2705T and At. thiooxidans DSM 14887T previously adapted to D-galactose were tested, being inoculated with a total of 1·108 cells·ml−1 in the cells proportion previously defined (50:50; 30:70, and 30:70). In the experiments with pre-colonization, the inoculum for each microorganism was 5·107 cells·ml−1. The biooxidation tests were made in batch cultures during 15days at 30°C and 180rpm with an initial pH of 1.8. All experiences were contrasted with cells non-adapted to D-galactose that serving as a control and were carried out under the same conditions. The tests were considered concluded when the concentration of Fe3+ stopped increasing. In addition, pH and cell number were measured, and the volumetric productivity (Qp) was determined according to Saavedra et al. (2020).
The total number of planktonic cells was quantified by direct counting in a Neubauer chamber (depth of 0.02mm) using a phase-contrast microscope (BA410, Motic, Hong Kong, China). The concentration of ferrous iron and total iron was determined by the ferrozine method (Lovley and Phillips, 1987) and sulfate content by turbidimetry (Bernal, 1980).
The growth kinetics of At. thiooxidans DSM 14887T and L. ferrooxidans DSM 2705T were evaluated at different concentrations of D-galactose. Figure 1 resumes the kinetic parameters obtained.
Figure 1. Parameters obtained in the cultures of Acidithiobacillus thiooxidans DSM 14887T (diagonal lines) and Leptospirillum ferrooxidans DSM 2705T (horizontal lines) adapted to different concentrations of D-galactose. (A) Maximum specific growth rate (μmax). (B) Yield of biomass (YX/S). (C) EPS content (mg of EPS by g of DW cells). The data correspond to the mean values of triplicates±SD (n=6).
Acidithiobacillus thiooxidans DSM 14887T adapted to D-galactose showed a little variation in the maximum specific growth rate at different conditions. The culture without D-galactose (control) and the one with cells adapted to 0.15% of D-galactose are similar and slightly higher compared to the rest of the conditions (Figure 1A). A decrease in pH was also observed during the sulfur oxidation process, thus reaching values of 1.2 (data not shown) resulting from the formation of H2SO4. The highest biomass yield was obtained for the culture without D-galactose (Figure 1B).
Regarding L. ferrooxidans DSM 2705T, a higher specific growth rate is also observed in the culture without D-galactose and the culture using cells adapted to 0.15% D-galactose, which are also slightly higher than the values obtained in cultures with cells adapted to 0.25 and 0.35% of D-galactose (Figure 1A). Substrate depletion occurred at approximately 40h for most of the cultures run with adapted cells to D-galactose, except for the case of 0.35%, which continued to grow until approximately 48h.
When the EPS content of At. thiooxidans DSM 14887T and L. ferrooxidans DSM 2705T adapted to D-galactose was compared, both strains exhibited similar behavior, since the highest concentration of EPS was obtained with both cells adapted to 0.25% of D-galactose (Figure 1C). Leptospirillum ferrooxidans DSM 2705T achieved a content of eight times more EPS than At. thiooxidans DSM 14887T. In both strains, the culture run without D-galactose produced very little EPS, reaching a total of 30.3 and 44.6mg·g−1 of dry weight (DW) cell for At. thiooxidans DSM 14887T and L. ferrooxidans DSM 2705T, respectively.
By mixing equal number of At. thiooxidans DSM 14887T and L. ferrooxidans DSM 2705T, an inoculum was obtained to start the control culture (non-adapted cells) and the culture with adapted cells to D-galactose. In the case of adapted cells, At. thiooxidans DSM 14887T and L. ferrooxidans DSM 2705T were adapted to 0.15 and 0.25% of D-galactose, respectively. These conditions were selected, because they were the cells that showed the highest attachment percentage as pure cultures (Aguirre et al., 2017, 2018). Figure 2A shows the percentage of attached cells over time for At. thiooxidans DSM 14887T and L. ferrooxidans DSM 2705T when the consortia were formed in proportion of 50:50 of each species (5·107 cells·ml−1 for each strain), both for adapted and non-adapted microorganisms to D-galactose.
Figure 2. Attachment percentage to the mineral over time for the consortium in proportion 50:50 of At. thiooxidans DSM 14887T and L. ferrooxidans DSM 2705T, respectively. (A) Data obtained by qPCR. (B) Data obtained by counting total planktonic cells using a Neubauer chamber. The data correspond to the mean values of triplicates±SD (n=6).
In the case of the consortium previously adapted to D-galactose, both species showed an attachment percentage greater than 90% after 60min. On the other hand, in the consortium of non-adapted cells, the attachment of L. ferrooxidans DSM 2705T did not exceed 80% in the first hour of the assay; as for the At. thiooxidans DSM 148876T, it achieved approximately 90% attachment. After 60min, the microorganisms in both consortia slowly dissociated from the mineral, obtaining final attachments (after 7h) ranging from 60 to 84% for both strains in the consortia adapted and non-adapted to D-galactose, respectively. In Figure 2B, a high number of total attached cells to the mineral was observed for the two species that form both consortia (control without adaptation and with previous adaptation to D-galactose). In the case of the control, the attachment percentage reached 87% in the first hour of the experiment, while for the consortium precultured with D-galactose, the attachment percentage was 91% at the same time. After 7h, the cultures with both type of consortia showed an attachment percentage of less than 80%.
Figure 3 shows the variation in the percentage of attached cells over time for L. ferrooxidans DSM 2705T and At. thiooxidans DSM 14887T, when the inoculum concentration was in proportion of 70:30 of At. thiooxidans DSM 14887T and L. ferrooxidans DSM 2705T, respectively, as for the control (non-adapted cells) and the consortium adapted to D-galactose.
Figure 3. Attachment percentage to the mineral over time for the consortium in proportion 70:30 of At. thiooxidans DSM 14887T and L. ferrooxidans DSM 2705T, respectively. (A) Data obtained by qPCR. (B) Data obtained by counting of total planktonic cells using a Neubauer chamber. The data correspond to the mean values of triplicates±SD (n=6).
For L. ferrooxidans DSM 2705T, the adaptation to D-galactose has a greater influence on attachment to the mineral, since the attachment percentage exceeds 70% for this strain during the first hour of the experiment (Figure 3A). On the other hand, with At. thiooxidans DSM 14887T, an opposite behavior is observed. The highest attachment percentage to the mineral occurs in the control culture (non-adapted cells), reaching 69% in the first hour (Figure 3A). After 7h, the attachment percentage of both species is lower, reaching 15 and 30% for the control and adapted At. thiooxidans DSM 14887T, respectively, while for L. ferrooxidans DSM 2705T were obtained 20 and 23% for the control and the adapted, respectively. Figure 3B shows the total attachment percentage of the consortia obtained by counting planktonic cells. It can be observed that during the first hour of the experiment, approximately 80% of the microorganisms were attached to the mineral with respect to the total number of cells inoculated at the start of the experiment (1·108 cells·ml−1). Subsequently, the trend of the consortium was like that observed by qPCR, where a decrease in the attachment percentage was observed until it stabilized at approximately 50%.
Figure 4 shows the variation in the attachment percentage over time of L. ferrooxidans DSM 2705T and At. thiooxidans DSM 14887T, when the inoculum concentration was in proportion of 30:70 of At. thiooxidans DSM 14887T and L. ferrooxidans DSM 2705T, respectively, as for the control (non-adapted cells) and the consortium adapted to D-galactose.
Figure 4. Attachment percentage to the mineral over time for the consortium in proportion of 30:70 of At. thiooxidans DSM 14887T and L. ferrooxidans DSM 2705T, respectively. (A) Data obtained by qPCR. (B) Data obtained by counting of total planktonic cells using a Neubauer chamber. The data correspond to the mean values of triplicates±SD (n=6).
In the consortium adapted to D-galactose, L. ferrooxidans DSM 2705T had a higher attachment percentage (85%) with respect to At. thiooxidans DSM 14887T, which only reaches 42%. For the control, the trend was similar; however, the attachment percentage was lower for both species, reaching approximately 67% for L. ferrooxidans DSM 2705T and 30% for At. thiooxidans DSM 14887T. Figure 4B shows the total attachment percentage calculated as difference of initial total cells and the remaining planktonic cells. It is observed that approximately 83% of the total cells attach to the mineral when the consortium was adapted to D-galactose, while the control achieved approximately 70% of attachment cells, based on the total cells inoculated at the beginning of the assay (1·108 cells·ml−1).
Figure 5 shows the variation of the attachment percentage over time of L. ferrooxidans DSM 2705T and At. thiooxidans DSM 14887T to the mineral pre-colonized with L. ferrooxidans DSM 2705T, for the consortia of non-adapted and adapted cells to D-galactose.
Figure 5. Attachment percentage to the mineral over time of L. ferrooxidans DSM 2705T and At. thiooxidans DSM 2705T using L. ferrooxidans DSM 2705T as a pre-colonizer. (A) Data obtained by qPCR. (B) Data obtained by counting total planktonic cells using a Neubauer chamber. Measurements after 1, 60, 61, 91, and 181min of inoculation of the first species. The data correspond to the mean values of triplicates±SD (n=6).
After 60min, L. ferrooxidans DSM 2705T reached a higher attachment percentage (88%) when the consortia were adapted, while the control only reached 71% (Figure 5A). After 61min, when the second species (At. thiooxidans DSM 14887T) was added, the attachment percentage of L. ferrooxidans DSM 2705T decreased drastically, both in the control and in the consortium adapted to D-galactose. After 181min, the cells of non-adapted consortium had almost completely dissociated from the mineral, while cells of the adapted consortium remained attached at almost the same percentage as after the inoculation of At. thiooxidans DSM 14887T.
On the other hand, for At. thiooxidans DSM 14887T in the non-adapted consortium, the attachment percentage increased from 35 to 70% after 30min from their inoculation (91min); then, it decreased until reaching 60% at the end of the experience. In the case of the consortia adapted to D-galactose, the attachment percentage was low throughout the experiment; the observed trend was quite similar, but the maximum attachment percentage only reached 24% at 91min.
Figure 5B shows the microbial attachment of total cells adapted and non-adapted to D-galactose acting L. ferrooxidans DSM 2705T as a pre-colonizer. After 60min of incubation, a similar attachment percentage was observed compared with the results obtained by qPCR. At minute 61, when the second species (At. thiooxidans DSM 14887T) was added, it was observed that the global attachment percentage of the culture decreased, being greater for the consortium adapted to D-galactose. After 7h of culture, it was possible to maintain 40% of the attachment.
Figure 6 shows the variation of the attachment percentage over time of L. ferrooxidans DSM 2705T and At. thiooxidans DSM 14887T to mineral pre-colonized with At. thiooxidans DSM 14887T, for the consortia non-adapted and adapted to D-galactose.
Figure 6. Attachment percentage to the mineral over time of L. ferrooxidans DSM 2705T and At. thiooxidans DSM 2705T using At. thiooxidans DSM 2705T as a pre-colonizer. (A) Data obtained by qPCR. (B) Data obtained by counting total planktonic cells using a Neubauer chamber. Measurements after 1, 60, 61, 91, and 181min of inoculation of the first species. The data correspond to the mean values of triplicates±SD (n=6).
At minute 61, after inoculating At. thiooxidans DSM 14887T, the attachment percentage of L. ferrooxidans DSM 2705T was much higher in the consortium adapted to D-galactose than in the control, which only reached 13% (Figure 6A). The highest attachment percentage was observed at 91min after having inoculated the first species (At. thiooxidans DSM 14887T), reaching 83 and 96% of attachment for the control culture and the previously adapted, respectively. Finally, at 181min, it was observed that L. ferrooxidans DSM 2705T detached itself from the mineral; this phenomenon was more marked for the control.
In the case of At. thiooxidans DSM 14887T, an attachment percentage of approximately 90% was obtained during the first 60min for the consortium adapted to D-galactose, which contrasts with the control that only achieved 40% of attachment (Figure 6A). At 61min, when L. ferrooxidans DSM 2705T had been introduced, At. thiooxidans DSM 14887T began to detach. This behavior was similar in the case of the adapted consortium and in the control with cells non-adapted to D-galactose.
Figure 6B shows attachment of total cells for the consortia of non-adapted and adapted cells to D-galactose using At. thiooxidans DSM 14887T as a pre-colonizer. Up to minute 60, where only At. thiooxidans DSM 14887T was present, the percentage of microbial attachment was like the results obtained from qPCR. At minute 61, when the second species (L. ferrooxidans DSM 2705T) had been added, the trend of the consortium changed slightly, obtaining a higher attachment percentage with respect to the cultures where the pre-colonizer was L. ferrooxidans DSM 2705T.
It was also determined how to distribute the consortia on the surface of the mineral particles. Figure 7 shows the percentage of attached cells of At. thiooxidans DSM 14887T and L. ferrooxidans DSM 2705T with respect to the total cells attached to the mineral particles determined by qPCR.
Figure 7. Attachment percentage of the species At. thiooxidans DSM 14887T and L. ferrooxidans DSM 2705T on the surface of the mineral particles over time. (A) Consortia in proportion 50:50 of each specie. (B) Consortia in proportion 70:30 of At. thiooxidans DSM 14887T and L. ferrooxidans DSM 2705T, respectively. (C) Consortia in proportion 30:70 of At. thiooxidans DSM 14887T and L. ferrooxidans DSM 2705T, respectively. At. thiooxidans DSM 14887T (diagonal lines) and L. ferrooxidans DSM 2705T (horizontal lines). White Bars represent the control. Color Bars represent consortia adapted to D-galactose. The data correspond to the mean values of triplicates±SD (n=6).
According to Figure 7, the content of At. thiooxidans DSM 14887T and L. ferrooxidans DSM 2705T was close to 50% in the consortium in proportion 50:50 of both species (Figure 7A). However, the amount of L. ferrooxidans DSM 2705T increased in all consortia adapted to D-galactose, even in the consortium formed in a proportion of 70:30 of At. thiooxidans DSM 14887T and L. ferrooxidans DSM 2705T, respectively, (Figure 7B). The highest percentage of the attached cells to mineral of At. thiooxidans DSM 14887T was observed in the control consortium 70:30 of At. thiooxidans DSM 14887T and L. ferrooxidans DSM 2705T.
In the same way, it was also determined from the total number of attached cells of the consortia to the mineral how much corresponds to each species. Figure 8 shows the percentage of attached cells of each species (At. thiooxidans DSM 14887T and L. ferrooxidans DSM 2705T), with respect to the total cells attached to the mineral particles determined by qPCR using one species as pre-colonizer.
Figure 8. Attachment percentage of the species At. thiooxidans DSM 14887T and L. ferrooxidans DSM 2705T on the surface of the mineral particles determined in the time. (A) Using L. ferrooxidans DSM 2705T as a pre-colonizer. (B) Using At. thiooxidans DSM 2705T as a pre-colonizer. Measurements at 61, 91, and 181min from the inoculation of the first species. At. thiooxidans DSM 14887T (diagonal lines) and L. ferrooxidans DSM 2705T (horizontal lines). White Bars represent the control. Color Bars represent consortia adapted to D-galactose. The data correspond to the mean values of triplicates±SD (n=6).
The content of L. ferrooxidans DSM 2705T was in higher percentage in all consortia, mainly in which this microorganism acted like a pre-colonizer. The highest percentage of attached cells to mineral of At. thiooxidans DSM 14887T was observed in the non-adapted to D-galactose consortium where this microorganism was used as pre-colonizer.
Table 2 shows pH variation and the overall volumetric productivity (Qp) in the biooxidation of a polymetallic mineral concentrate carried out by consortia of different characteristics. According to these results, the consortia of non-adapted cells to D-galactose presented the lowest pH variation, being the consortia in proportion of 30:70 of At. thiooxidans DSM 14887T and L. ferrooxidans DSM 2705T, respectively, the combination that obtained the lowest differential with a ΔpH value of 0.12. On the other hand, the greater pH differential was observed in the consortia adapted to D-galactose, especially in the proportion of 50:50 of both species. ΔpH value was 0.38, reaching pH of 1.4 at the time in which biooxidation tests were completed. At the same time, this consortium registered a maximum level of 9.7mgL−1 h−1 of overall volumetric productivity of Fe3+. On the other hand, the one with the lowest overall volumetric productivity of Fe3+ was the consortium formed by L. ferrooxidans DSM 2705T and At. thiooxidans DSM 14887T non-adapted to D-galactose, using At. thiooxidans as a pre-colonizer, after a period of 15days of biooxidation.
Table 2. pH variation and overall volumetric productivities of the different consortia formed by the species At. thiooxidans DSM 14887T and L. ferrooxidans DSM 2705T.
The overall volumetric productivity of SO42− was low in consortia where the proportion of A. thiooxidans DSM 14887T was lower, such was the case of the consortium formed in proportion of 30:70 of At. thiooxidans DSM 14887T and L. ferrooxidans DSM 2705T non-adapted to D-galactose. In the same way, the highest overall volumetric productivity of SO42− occurred in the consortium formed in proportion 50:50 of At. thiooxidans DSM 14887T and L. ferrooxidans DSM 2705T adapted to D-galactose with a value of 11.7mgL−1 h−1 after 15days of biooxidation.
The tests carried out with mixed cultures of L. ferrooxidans DSM 2705T and At. thiooxidans DSM 14887T indicate that the combination of strains influences the binding capacity of each one on sulfide minerals. The results indicate that in most of the combinations of L. ferrooxidans DSM 2705T and At. thiooxidans DSM 14887T, bacteria mutually influence the mineral binding capacity, while the use of inducers of EPS synthesis, in this case D-galactose, has a potentiating effect on this binding. Similar results were obtained by other authors using different inducers like UVA radiation which promoted the formation of EPS and cell attachment (Amar et al., 2021).
In the case of L. ferrooxidans DSM 2705T when combined with At. thiooxidans DSM 14887T, the binding to sulfides is lower compared to pure cultures, which reach an attachment percentage greater than 90% (Aguirre et al., 2018). However, the attachment of At. thiooxidans DSM 14887T tends to increase in other combinations. Thus, for example, At. thiooxidans DSM 14887T was the strain with the highest attachment percentage in the consortium with proportion 50:50 and showed the highest attachment in the consortium with proportion 70:30 of At. thiooxidans DSM 14887T and L. ferrooxidans DSM 2705T, respectively. When used as a pre-colonizer, L. ferrooxidans DSM 2705T showed reduced overall attachment capacity in mixed cultures compared to pure cultures. The results suggest that At. thiooxidans DSM 14887T attach faster and in greater proportion to the mineral when it is previously adapted to D-galactose. For all cases, it is important to highlight that the moment with the highest attachment percentage occurred in the first hour of the test and then declined over time.
With respect to the variation of the attachment percentage over time with different proportions of each species, when the microbial consortium was tested in a proportion of 70:30 of At. thiooxidans DSM 14887T and L. ferrooxidans DSM 2705T (Figure 3A) showed a high attachment percentage of the first species, without neglecting that the second one had an attachment percentage of 70±5.7% when the consortium was previously adapted to D-galactose. As for the control, both species follow the same trend; however, the attachment percentage in general is lower. Respect to the attachment percentage of At. thiooxidans DSM 14887T, it seems that when this species is initially present in a greater number of cells and forming a thin layer of biofilm, this would allow that the other species to easily attach to the mineral, without having a greater impediment to the attachment of L. ferrooxidans DSM 2705T. On the other hand, when the proportion of At. thiooxidans DSM 14887T is lower (Figure 4A), it seems that L. ferrooxidans DSM 2705T occupies almost all the active sites of the mineral; in addition, having a greater amount of EPS (Figure 1C), it prevents At. thiooxidans DSM 14887T of occupying available active sites for its attachment (Florian et al., 2010; Florian, 2012). Likewise, it is known the existence of a secretion of microbial lipids called surfactant microbial compounds (SAC) that are involved in the alteration of hydrophobic surfaces. SACs secreted by bacteria into the surrounding media may be responsible for a microbially created conditioning film at an interface (Neu, 1996; Zhang et al., 2015; Castro et al., 2016). One of the first examples was reported for a species of Acidithiobacillus spp.; some compounds of this surface may be involved in the initial stages of attachment to hydrophobic surfaces (Jones and Starkey, 1961; Georgiou et al., 1992; Chandrawati and Shagufta, 2007). The presence of microbial surfactant compounds can also inhibit bacterial attachment to solid surfaces. Another cause for the decreased attachment of at least one of the strains may be competition for attractive (usable) surface areas by bacteria.
Regarding the attachment percentage of total cells when the proportion of each species is varied (Figures 2B, 3B, 4B), it is important to remark that the consortium adapted to D-galactose with a 50:50 proportion presents a higher attachment percentage compared to consortium non-adapted to D-galactose. The tests also show a similar trend to those carried out using qPCR, accounting for the behavior of the microbial species. It is interesting to highlight that the maximum attachment percentage developed up to 2h test; after such point, it slowly began to decrease.
There are studies in which the importance of EPS has been demonstrated for microbial attachment to sulfide minerals; however, little is known about the interactions generated among various species and their EPS on attachment to minerals sulfides and in turn the effect it can have on the biooxidation of sulfides. From the tests using pre-colonization with non-adapted and adapted cells, a behavior like those described in the previous cases was observed with respect to the attachment percentage of a consortium. Thus, for example, in Figure 5, it is observed that L. ferrooxidans DSM 2705T when used as a pre-colonizer after 1h of incubation, its attachment percentage is over 70±1% for the control and 90±3.25% for the consortium adapted to D-galactose, results that agree with the previously discussed pure culture data (Florian et al., 2010; Florian, 2012; Aguirre et al., 2018).
When pre-colonization started with At. thiooxidans DSM 14887T, a similar behavior of this species was observed after 1h of incubation of non-adapted and adapted to D-galactose consortium. After minute 61, when the second species was inoculated, their attachment percentage decreased. In this case, the attachment percentage of L. ferrooxidans DSM 2705T was higher in non-adapted and adapted to D-galactose consortium, the last one being higher than the control. Apparently, the forces involved in the EPS of At. thiooxidans DSM 14887T are weak. In addition, the amount of EPS formed was much lower compared with L. ferrooxidans DSM 2705T (Gilbert et al., 1993).
In the same way, the amount of each microorganism attached to the mineral particles was investigated in function of the total amount of microorganisms that only were attached to the mineral (Figure 7). When the strains are in a 50:50 ratio of each species, At. thiooxidans DSM 14887T achieves a higher attachment with respect to L. ferrooxidans DSM 2705T in the control; however, when they are previously adapted to D-galactose, the attachment of At. thiooxidans DSM 14887T decreases, while that of L. ferrooxidans DSM 2705T increases.
This phenomenon can be explained since in the consortium formed in a 50:50 ratio of each species, the amount of L. ferrooxidans DSM 2705T cells with EPS was lower compared to the consortium previously adapted to D-galactose, whose amount of microorganism with EPS was much greater, occupying most of the active sites of the mineral. Additionally, a strong EPS formation has already been described in case of atomic force microscopy (AFM) and epifluorescent microscopic (EFM) studies of Leptospirillum spp. (Noël, 2010; Florian et al., 2011; Florian, 2012). These results are agreed with Liu et al. (2016) who tested a binary mixture of Leptospirillum ferriphilum and Acidithiobacillus showed the same proportion that each represented 50% at final equilibrium stage. L. ferriphilum and At. caldus can attach on chalcopyrite simultaneously and performed mutual non-interference in their adsorption to chalcopyrite. The high proportion of L. ferriphilum at firstly stage was due to the higher affinity and EPS, which benefited the preferential attachment of L. ferriphilum.
Likewise, when the microorganisms are in the consortium in a 70:30 ratio of At. thiooxidans DSM 14887T and L. ferrooxidans DSM 2705T, respectively, in the control is observed that the predominant species is At. thiooxidans DSM 14887T. In the consortium in the same proportion (70:30) but adapted to D-galactose, it can be observed that the species with the highest attachment is L. ferrooxidans DSM 2705T. Finally, when the proportion of the consortium is reversed, both the control and the one previously adapted to D-galactose a high attachment of L. ferrooxidans DSM 2705T is observed.
In the case of consortia using pre-colonizer (Figure 8), similar phenomena happened. There was more attachment percentage of L. ferrooxidans DSM 2705T when it was used as pre-colonizer. In the other hand, when At. thiooxidans DSM 14887T was used as pre-colonizer, and once L. ferrooxidans was inoculated, it started to predominate and displaced At. thiooxidans DSM 14887T, also reaching a high attachment percentage to the mineral surface. Again, this behavior can be explained because of the higher amount of its cells EPS and probably stickier than the EPS of At. thiooxidans DSM 14887T.
On the other hand, bacterial oxidation of mineral sulfides is a process that is influenced by several factors, such as temperature, pH, availability of O2 and CO2, the presence of toxic compounds, microbial species, among others. Hence, all the consortia previously established to carry out the attachment tests were investigated as biooxidant agents. It is known that bacterial attachment is important for biooxidation processes, affecting the efficiency of dissolution of sulfur minerals. According to Makaula et al. (2020) is recognized that the effective colonization of the mineral ore bed for heap bioleaching impacts both the start-up time of the heap and its performance.
Table 2 shows the pH differential due to its decrease during the biooxidation process of the concentrate of polymetallic sulfide mineral. It should be noted that these results represent an indirect measure of the oxidation of the minerals, since it is indicative that H2SO4 was generated as a product. The highest pH differential occurs when both species are in a proportion of 50:50 and when At. thiooxidans DSM 14887T is used as a pre-colonizer, either for non-adapted or for adapted to D-galactose consortium. On the other hand, the high overall volumetric productivity of Fe3+ was determined with consortium previously adapted to D-galactose in proportion 50:50 of each species, that is, those with the highest content of both species. It should also be noted that the high concentrations of Fe3+ at the beginning of the biooxidation could have influenced the process, since this consortium probably accumulated a greater amount of iron within their EPS, which in turn allowed them to have a greater dissolution of the minerals even with respect to controls without prior adaptation to D-galactose (Sand et al., 1995).
A careful analysis that combines the results of the adhesion tests and the biooxidation experiments of the sulfide minerals would provide more in-depth information that evidences a connection between the initial bacterial binding and the mineral solubilization, since the cultures that contained greater microbial attachment of both species adapted to D-galactose were those that provided a greater dissolution of sulfur minerals. The presence of EPS on the surface of bacteria and mineral plays an important role on bioleaching (Fang et al., 2021; Ye et al., 2021).
The proportion of the species and the inoculation order of the strains in the consortium together with the EPS induction with D-galactose affected the cellular attachment percentage to the mineral. The highest attachment percentage of the microbial community was obtained when L. ferrooxidans DSM2705T and At. thiooxidans DSM 14887T were inoculated in a proportion 50:50 simultaneously and previously adapted to D-galactose. Given the high amounts of EPS in planktonic cells of the acidophilic microorganisms studied, there was an increase in the microbial attachment percentage, which would make possible to accelerate the biooxidation of sulfides minerals.
The proportion of the species and the order of inoculation of the microbial consortium influenced the biooxidation of a polymetallic mineral concentrate, obtaining the highest volumetric productivity of iron and sulfate when L. ferrooxidans DSM2705T and At. thiooxidans DSM 14887T were inoculated in a proportion of 50:50 simultaneously and previously adapted to D-galactose. Is important consider that better volumetric productivities values (ferric and sulfate) were obtained when both species were inoculated simultaneously, mainly for Fe3+, and when the attachment cell percentage to the mineral particles was closer. In the case of sulfate, high volumetric productivity values occurred with consortia adapted and non-adapted to D-galactose, meaning a certain independence on the amount of accumulated EPS.
The original contributions presented in the study are included in the article/Supplementary Material, further inquiries can be directed to the corresponding author.
JG proposed and designed the idea and the study, provided the facilities, funding, revised, commented, and contributed to writing the manuscript. PA proposed and designed the idea and the study, performed the experiments, collected, processed, and analyzed data, was involved in the study design, and contributed to writing the manuscript. AS, EM, and KG were involved in data analysis, discussed the results, and contributed to writing the manuscript. SH was involved in data analysis, discussed the results, and contributed to revision of the manuscript. All authors contributed to the article and approved the submitted version.
This work was supported by the School of Biochemical Engineering (Pontificia Universidad Católica de Valparaíso-Chile) and Universidad Técnica Particular de Loja (Loja-Ecuador).
The authors declare that the research was conducted in the absence of any commercial or financial relationships that could be construed as a potential conflict of interest.
All claims expressed in this article are solely those of the authors and do not necessarily represent those of their affiliated organizations, or those of the publisher, the editors and the reviewers. Any product that may be evaluated in this article, or claim that may be made by its manufacturer, is not guaranteed or endorsed by the publisher.
The authors would like to thank the Federal Geoscience Natural Resources Institute (Hannover-Germany), especially PhD. Axel Schippers and his group, for their important value in this work, especially in qPCR and biohydrometallurgy.
The Supplementary Material for this article can be found online at: https://www.frontiersin.org/articles/10.3389/fmicb.2021.767639/full#supplementary-material
Aguirre Morales, M. (2012). Extracellular Polymeric Substances (EPS) Production in Sulfobacillus thermosulfidooxidans and Its Relevance on Attachment to Metal Sulfides. Available at: http://www.bdigital.unal.edu.co/10705/
Aguirre, P., Guerrero, K., Sanchez-Rodriguez, A., Gentina, J. C., and Schippers, A. (2018). Making sticky cells: effect of galactose and ferrous iron on the attachment of Leptospirillum ferrooxidans to mineral surfaces. Res. Microbiol. 169, 569–575. doi: 10.1016/j.resmic.2018.08.005
Aguirre, P., Rodríguez, A. S., Gentina, J. C., and Schippers, A. (2017). Effect of galactose on EPS production and attachment of Acidithiobacillus thiooxidans to mineral surfaces. Solid State Phenom. 262, 476–481. doi: 10.4028/www.scientific.net/SSP.262.476
Amar, A., Castro, C., Bernardelli, C., Costa, C. S., and Donati, E. (2021). Influence of UVA radiation on growth, biofilm formation and bioleaching capacity of Leptospirillum ferrooxidans. Hydrometallurgy 201:105574. doi: 10.1016/j.hydromet.2021.105574
Arredondo, R., Garcia, A., and Jerez, C. A. (1994). Partial removal of lipopolysaccharide from Thiobacillus ferrooxidans affects its adhesion to solids. Appl. Environ. Microbiol. 60, 2846–2851. doi: 10.1128/aem.60.8.2846-2851.1994
Barreto, M., Jedlicki, E., and Holmes, D. S. (2005). Identification of a gene cluster for the formation of extracellular polysaccharide precursors in the chemolithoautotroph Acidithiobacillus ferrooxidans. Appl. Environ. Microbiol. 71, 2902–2909. doi: 10.1128/AEM.71.6.2902-2909.2005
Bellenberg, S., Barthen, R., Boretska, M., Zhang, R., Sand, W., and Vera, M. (2014a). Manipulation of pyrite colonization and leaching by iron-oxidizing Acidithiobacillus species. Appl. Microbiol. Biotechnol. 99, 1435–1449. doi: 10.1007/s00253-014-6180-y
Bellenberg, S., Díaz, M., Noël, N., Sand, W., Poetsch, A., Guiliani, N., et al. (2014b). Biofilm formation, communication and interactions of leaching bacteria during colonization of pyrite and sulfur surfaces. Res. Microbiol. 165, 773–781. doi: 10.1016/j.resmic.2014.08.006
Bernal, J. (1980). Analisis de aguas naturales continentales. Madrid: Instituto de Hidrología and Centro de Estudios Hidrográficos.
Bradford, M. (1976). A rapid and sensitive method for the quantitation of microgram quantities of protein utilizing the principle of protein-dye binding. Anal. Biochem. 72, 248–254. doi: 10.1016/0003-2697(76)90527-3
Castro, C., Zhang, R., Liu, J., Bellenberg, S., Neu, T. R., Donati, E., et al. (2016). Biofilm formation and interspecies interactions in mixed cultures of thermo-acidophilic archaea Acidianus spp. and Sulfolobus metallicus. Res. Microbiol. 167, 604–612. doi: 10.1016/j.resmic.2016.06.005
Chandrawati, J., and Shagufta, (2007). Environmental Biotechnology. New Delhi: APH Publishing Corporation.
Dubois, M., Gilles, K., Hamilton, J. K., Rebers, P. A., and Smith, F. (1956). A colorimetric method for the determination of sugars. Anal. Chem. 28, 350–356. doi: 10.1021/ac60111a017
Elifantz, H., Horn, G., Ayon, M., Cohen, Y., and Minz, D. (2013). Rhodobacteraceae are the key members of the microbial community of the initial biofilm formed in eastern Mediterranean coastal seawater. FEMS Microbiol. Ecol. 85, 348–357. doi: 10.1111/1574-6941.12122
Falco, L., Pogliani, C., Curutchet, G., and Donati, E. (2003). A comparison of bioleaching of covellite using pure cultures of Acidithiobacillus ferrooxidans and Acidithiobacillus thiooxidans or a mixed culture of Leptospirillum ferrooxidans and Acidithiobacillus thiooxidans. Hydrometallurgy 71, 31–36. doi: 10.1016/S0304-386X(03)00170-1
Fang, X., Sun, S., Liao, X., Li, S., Zhou, S., Gan, Q., et al. (2021). Effect of diurnal temperature range on bioleaching of sulfide ore by an artificial microbial consortium. Sci. Total Environ. 806:150234. doi: 10.1016/j.scitotenv.2021.150234
Florian, B. M. (2012). Investigation of Initial Attachment and Biofilm Formation of Mesophilic Leaching Bacteria in Pure and Mixed Cultures and Their Efficiency of Pyrite Dissolution. Available at: https://duepublico2.uni-due.de/receive/duepublico_mods_00028549
Florian, B., Noël, N., and Sand, W. (2010). Visualization of initial attachment of bioleaching bacteria using combined atomic force and epifluorescence microscopy. Miner. Eng. 23, 532–535. doi: 10.1016/j.mineng.2010.02.002
Florian, B., Noël, N., Thyssen, C., Felschau, I., and Sand, W. (2011). Some quantitative data on bacterial attachment to pyrite. Miner. Eng. 24, 1132–1138. doi: 10.1016/j.mineng.2011.03.008
Gehrke, T., Telegdi, J., Thierry, D., and Sand, W. (1998). Importance of extracellular polymeric substances from Thiobacillus ferrooxidans for bioleaching. Appl. Environ. Microbiol. 64, 2743–2747. doi: 10.1128/aem.64.7.2743-2747.1998
Gentina, J. C., and Acevedo, F. (2016). Copper bioleaching in Chile. Fortschr. Mineral. 6:23. doi: 10.3390/min6010023
Georgiou, G., Lin, S.-C., and Sharma, M. (1992). Surface-active compounds from microorganisms. Biotechnology 10, 60–65. doi: 10.1038/nbt0192-60
Gilbert, P., Evans, D., and Brown, M. R. (1993). Formation and dispersal of bacterial biofilms in vivo and in situ. J. Appl. Bacteriol. 74, 67–78. doi: 10.3917/mediu.036.0003
Harneit, K., Göksel, A., Kock, D., Klock, J. H., Gehrke, T., and Sand, W. (2006). Adhesion to metal sulfide surfaces by cells of Acidithiobacillus ferrooxidans, Acidithiobacillus thiooxidans and Leptospirillum ferrooxidans. Hydrometallurgy 83, 245–254. doi: 10.1016/j.hydromet.2006.03.044
Hedrich, S., Guézennec, A. G., Charron, M., Schippers, A., and Joulian, C. (2016). Quantitative monitoring of microbial species during bioleaching of a copper concentrate. Front. Microbiol. 7:2044. doi: 10.3389/fmicb.2016.02044
Jones, G., and Starkey, R. (1961). Surface-active substances produced by Thiobacillus thiooxidans. J. Bacteriol. 82, 788–789. doi: 10.1128/jb.82.5.788-789.1961
Karatan, E., and Watnick, P. (2009). Signals, regulatory networks, and materials that build and break bacterial biofilms. Microbiol. Mol. Biol. Rev. 73, 310–347. doi: 10.1128/MMBR.00041-08
Kim, T. W., Kim, C. J., Chang, Y. K., Ryu, H. W., and Cho, K. S. (2002). Development of an optimal medium for continuous ferrous iron oxidation by immobilized Acidothiobacillus ferrooxidans cells. Biotechnol. Prog. 18, 752–759. doi: 10.1021/bp020289j
Kock, D., and Schippers, A. (2008). Quantitative microbial community analysis of three different sulfidic mine tailing dumps generating acid mine drainage. Appl. Environ. Microbiol. 74, 5211–5219. doi: 10.1128/AEM.00649-08
Lara, R. H., Valdez-Pérez, D., Rodríguez, A. G., Navarro-Contreras, H. R., Cruz, R., and García-Meza, J. V. (2010). Interfacial insights of pyrite colonized by Acidithiobacillus thiooxidans cells under acidic conditions. Hydrometallurgy 103, 35–44. doi: 10.1016/j.hydromet.2010.02.014
Liu, J., Li, Q., Sand, W., and Zhang, R. (2016). Influence of Sulfobacillus thermosulfidooxidans on initial attachment and pyrite leaching by thermoacidophilic archaeon Acidianus sp. DSM 29099. Fortschr. Mineral. 6:76. doi: 10.3390/min6030076
Lovley, D. R., and Phillips, E. J. P. (1987). Rapid assay for microbially reducible ferric iron in aquatic sediments. Appl. Environ. Microbiol. 53, 1536–1540. doi: 10.1128/aem.53.7.1536-1540.1987
Makaula, D. X., Huddy, R. J., Fagan-Endres, M. A., and Harrison, S. T. L. (2020). Cross-correlating analyses of mineral-associated microorganisms in an unsaturated packed bed flow-through column test; cell number, activity and EPS. Res. Microbiol. 171, 222–229. doi: 10.1016/j.resmic.2020.09.002
Nemati, M., and Harrison, S. T. L. (2000). Effect of solid loading on thermophilic bioleaching of sulfide minerals. J. Chem. Technol. Biotechnol. 75, 526–532. doi: 10.1002/1097-4660(200007)75:7<526::AID-JCTB249>3.0.CO;2-4
Neu, T. R. (1996). Significance of bacterial surface-active compounds in interaction of bacteria with interfaces. Microbiol. Rev. 60, 151–166. doi: 10.1128/mr.60.1.151-166.1996
Noël, N., Florian, B., and Sand, W. (2010). AFM & EFM study on attachment of acidophilic leaching organisms. Hydrometallurgy 104, 370–375. doi: 10.1016/j.hydromet.2010.02.021
Rawlings, D. E., and Johnson, D. B. (2007). The microbiology of biomining: development and optimization of mineral-oxidizing microbial consortia. Microbiology 153, 315–324. doi: 10.1099/mic.0.2006/001206-0
Rodríguez, Y., Ballester, A., Blázquez, M. L., González, F., and Munoz, J. A. (2003). Study of bacterial attachment during the bioleaching of pyrite, chalcopyrite, and sphalerite. Geomicrobiol J. 20, 131–141. doi: 10.1080/01490450303880
Saavedra, A., Aguirre, P., and Gentina, J. C. (2020). Biooxidation of iron by Acidithiobacillus ferrooxidans in the presence of D-galactose: understanding its influence on the production of EPS and cell tolerance to high concentrations of iron. Front. Microbiol. 11:759. doi: 10.3389/fmicb.2020.00759
Saavedra, A., Pavez, B., Diaz, M., and Gentina, J. C. (2013). Galactose as inducer of the production of extracellular polymeric substances by Acidithiobacillus ferrooxidans. Adv. Mater. Res. 825, 120–124. doi: 10.4028/www.scientific.net/AMR.825.120
Sand, W., and Gehrke, T. (2006). Extracellular polymeric substances mediate bioleaching/biocorrosion via interfacial processes involving iron (III) ions and acidophilic bacteria. Res. Microbiol. 157, 49–56. doi: 10.1016/j.resmic.2005.07.012
Sand, W., Gerke, T., Hallmann, R., and Schippers, A. (1995). Sulfur chemistry, biofilm, and the (in)direct attack mechanism — a critical evaluation of bacterial leaching. Appl. Microbiol. Biotechnol. 43, 961–966. doi: 10.1007/BF00166909
Sand, W., Gehrke, T., Jozsa, P. G., and Schippers, A. (2001). (Bio)chemistry of bacterial leaching - direct vs. indirect bioleaching. Hydrometallurgy 59, 159–175. doi: 10.1016/S0304-386X(00)00180-8
Silver, M., Margalith, P., and Lundgren, D. G. (1967). Effect of glucose on carbon dioxide assimilation and substrate oxidation by Ferrobacillus ferrooxidans. J. Bacteriol. 93, 1765–1769. doi: 10.1128/jb.93.6.1765-1769.1967
Vera, M., Schippers, A., and Sand, W. (2013). Progress in bioleaching: fundamentals and mechanisms of bacterial metal sulfide oxidation-part A. Appl. Microbiol. Biotechnol. 97, 7529–7541. doi: 10.1007/s00253-013-4954-2
Yang, Y., Tan, S. N., Glenn, A. M., Harmer, S., Bhargava, S., and Chen, M. (2015). A direct observation of bacterial coverage and biofilm formation by Acidithiobacillus ferrooxidans on chalcopyrite and pyrite surfaces. Biofouling 31, 575–586. doi: 10.1080/08927014.2015.1073720
Ye, M., Liang, J., Liao, X., Li, L., Feng, X., Qian, W., et al. (2021). Bioleaching for detoxification of waste flotation tailings: relationship between EPS substances and bioleaching behavior. J. Environ. Manag. 279:111795. doi: 10.1016/j.jenvman.2020.111795
Keywords: bacterial attachment, D-galactose, At. thiooxidans, L. ferrooxidans, biooxidation
Citation: Aguirre P, Saavedra A, Moncayo E, Hedrich S, Guerrero K and Gentina JC (2021) Sticky Bacteria: Understanding the Behavior of a D-Galactose Adapted Consortium of Acidophilic Chemolithotroph Bacteria and Their Attachment on a Concentrate of Polymetallic Mineral. Front. Microbiol. 12:767639. doi: 10.3389/fmicb.2021.767639
Received: 31 August 2021; Accepted: 30 September 2021;
Published: 21 October 2021.
Edited by:
Jianyu Zhu, Central South University, ChinaReviewed by:
Arevik Vardanyan, Armenian National Academy of Sciences, ArmeniaCopyright © 2021 Aguirre, Saavedra, Moncayo, Hedrich, Guerrero and Gentina. This is an open-access article distributed under the terms of the Creative Commons Attribution License (CC BY). The use, distribution or reproduction in other forums is permitted, provided the original author(s) and the copyright owner(s) are credited and that the original publication in this journal is cited, in accordance with accepted academic practice. No use, distribution or reproduction is permitted which does not comply with these terms.
*Correspondence: Paulina Aguirre, cGlhZ3VpcnJlQHV0cGwuZWR1LmVj
Disclaimer: All claims expressed in this article are solely those of the authors and do not necessarily represent those of their affiliated organizations, or those of the publisher, the editors and the reviewers. Any product that may be evaluated in this article or claim that may be made by its manufacturer is not guaranteed or endorsed by the publisher.
Research integrity at Frontiers
Learn more about the work of our research integrity team to safeguard the quality of each article we publish.