- College of Horticulture and Plant Protection, Henan University of Science and Technology, Luoyang, China
Phytophthora sojae threatens soybean production worldwide, and the cultivation of soybean cultivars carrying Rps genes is the most effective way to control this pathogen. However, DNA mutations in the Avr genes of P. sojae can escape recognization of the corresponding Rps genes, leading to the loss of soybean resistance. In this study, we investigated sequence polymorphism and transcript level of the Avr3a gene in Chinese isolates of P. sojae. Twenty-four mutations resulting in five unique Avr3a alleles were discovered in the Avr3a coding region from 32 P. sojae isolates. The Avr3a transcripts were detectable in the isolates containing Avr3a(I), Avr3a(II), Avr3a(III), and Avr3a(IV) but not in the isolates containing Avr3a(V). Promoter and 5'-UTR sequence analysis revealed eight unique mutations in the promoter region of Avr3a(V), suggesting that the mutations could result in the loss of Avr3a(V) transcription. Virulence tests indicated the isolates containing Avr3a(II) and Avr3a(IV) were virulent, suggesting that the mutations in the coding regions of Avr3a(II) and Avr3a(IV) caused the gain of virulence to Rps3a. Based on DNA mutations of Avr3a in virulent alleles, two SNP markers and one PCR-based marker were developed successfully for detecting the virulence of P. sojae isolates to Rps3a. These findings provide new insights into escape mechanisms of Avr3a and effective support for accurate pathotype identification of P. sojae using molecular methods.
Introduction
Phytophthora sojae Kaufmann & Gerdemann is an oomycete of the Stramenopila Kingdom and a soilborne pathogen of soybean. The pathogen causes damping-off of soybean seedlings and root and stem rot of adult plants, known as Phytophthora root and stem rot (PRR) of soybean. PRR is a devastating disease of soybean worldwide and causes approximately $1–2 billion in annual agricultural losses (Tyler, 2007). Host resistance, soil drainage control, and fungicidal seed treatments, such as metalaxyl, are generally used to control the disease (Dorrance et al., 2009). The most effective way to control PRR currently involves growing soybean cultivars carrying resistance genes to P. sojae (Rps genes) (Sahoo et al., 2017; Sukumaran et al., 2018; Zhong et al., 2019).
The interaction between P. sojae and soybean follows the gene-for-gene model. The avirulence (Avr) genes of P. sojae determine the efficacy of the Rps genes in soybean. Nine Avr genes have been cloned from P. sojae to date: Avr1b-1 (Shan et al., 2004), Avr1a and Avr3a/5 (Qutob et al., 2009), Avr3c (Dong et al., 2009), Avr4/6 (Dou et al., 2010), Avr3b (Dong et al., 2011a), Avr1k (Song et al., 2013), Avr1d (Yin et al., 2013), and Avr1c (Na et al., 2014). These Avr genes encode secreted proteins with RXLR-dEER amino acid motifs. Avr proteins can be recognized by the corresponding Rps proteins in soybean, resulting in effector-triggered immunity. However, under the selective pressure of Rps genes, P. sojae can escape recognition by soybean through DNA modifications such as substitutions, frameshift mutations, large insertions, deletions, recombinations, or changes in expression of the Avr genes (Tyler and Gijzen, 2014; Zhang et al., 2019).
The large-scale deployment of Rps genes in soybean has led to the rapid evolution of the virulence profile (pathotype) of P. sojae populations, accompanying by the ineffectiveness of the deployed Rps genes (Tremblay et al., 2021). In order to determine and deploy the proper Rps genes, it is very important that the pathotypes of P. sojae are identified accurately. At present, the pathotypes of P. sojae are determined mainly through virulence tests using a soybean differential set (Cui et al., 2010; Guérin et al., 2014; Lebreton et al., 2018). As the bioassay is time-consuming and labor-intensive, it is not quite suitable for a large-scale virulence test. Hence, the development of simple and rapid molecular diagnostic methods is the main tendency for pathotype identification. More recently, Dussault-Benoit et al. (2020) developed a molecular assay that could define P. sojae pathotypes using seven Avr genes (Avr1a, Avr1b, Avr1c, Avr1d, Avr1k, Avr3a, and Avr6). The matching rate between the molecular assay and the phenotyping assay was as high as 97% (170/175). This molecular assay offers a powerful tool for the selection of germplasm resistant to P. sojae.
Although the molecular assay has been developed successfully, it is not very perfect. Five cases of discrepancy between the molecular assay and the phenotyping assay were observed in their study. Interestingly, all five cases of discrepancy were present in the Avr3a gene. The PCR amplification for the avirulent alleles of Avr3a was achieved in the five cases, but the compatible interactions with Rps3a were observed in the phenotyping assay (Dussault-Benoit et al., 2020). Avr3a is a RXLR effector gene and encodes a predicted protein of 111 amino acids that includes a signal peptide, a RXLR motif and a carboxyterminal effector domain (Qutob et al., 2009; Dong et al., 2011b). Rps3a in soybean can recognize Avr3a to generate an incompatible reaction. So far, only three alleles of Avr3a have been identified in P. sojae field populations, based on which the PCR assay was developed. The cases of discrepancy suggest that there may be unknown virulent alleles of Avr3a in natural populations that can be amplified by the PCR assay.
Therefore, it is necessary for us to do further investigations and improve the molecular assay for the perfect match between the molecular assay and the phenotyping assay. In this study, we analyzed the polymorphisms in the promoter and coding regions of Avr3a in Chinese P. sojae isolates and measured the transcript levels of each allele of Avr3a in order to: (i) discover novel alleles of Avr3a, (ii) analyze how Avr3a escape recognition by Rps3a in China, and (iii) develop novel and reliable molecular markers for accurate differentiation of virulent alleles from avirulent alleles.
Materials and Methods
Phytophthora sojae Isolates and Growth Conditions
A total of 32 P. sojae isolates were used in this study and purified by single zoospore isolation (Table 1). All isolates were recovered from soybean field soils in China (Cui et al., 2010, 2012). These isolates were grown on 10% V8 juice agar at 25°C in the dark for virulence tests and cultured in 10% V8 juice at 25°C in the dark for mycelium collection.
Virulence Tests
Soybean cultivars Williams (rps) and L83-570 (Rps3a) were used to evaluate the virulence of P. sojae isolates by hypocotyl split inoculation, as described previously (Cui et al., 2010). Phytophthora sojae isolates were grown on V8 plates for a week. Each treatment contained at least 10 plants, and each virulence test was performed in triplicate. An isolate was considered to be virulent if ≥70% of soybean seedlings were killed, avirulent if ≤30% of soybean seedlings were killed, and intermediate if 30–70% of soybean seedlings were killed (Ryley et al., 1998).
DNA Extraction, PCR Amplification, Cloning, and Sequence Analysis
Genomic DNA was isolated from mycelial cultures of P. sojae isolates following the protocol described by Tyler et al. (1995). PCR reactions were conducted in a total volume of 50μl containing 50ng template DNA, 2U PrimeSTAR HS DNA Polymerase (Takara, Dalian, China), 200μM dNTPs, 300mM of each primer, and 1×PrimeSTAR Buffer (Mg2+ Plus). The sequences of primers are shown in Supplementary Table S1. PCR was performed in a TP600 Thermal Cycler (Takara) under the following PCR program: 1cycle at 98°C for 1min for initial denaturation, 30cycles at 98°C for 10s, 56°C for 15s, 72°C for 1min, and a final 5min extension at 72°C. The PCR products were purified using a MiniBEST Agarose Gel DNA Extraction Kit (Takara), cloned into pMD19-T vectors (Takara), and sequenced by Sangon Biotech Co., Ltd. in Shanghai, China. Three clones were sequenced for each isolate. Sequence analysis was conducted using Bioedit 7.0 and Mega 7.0 softwares.
RNA Extraction and Transcript Level Analysis
Total RNA was extracted from P. sojae-infected soybean leaves as described by Ye et al. (2011). Total RNA of leaves at 12h post-inoculation was isolated and treated with RNase-free DNase I (Takara) to remove genomic DNA. The integrity of total RNA was assessed by agarose gel electrophoresis. Single-stranded cDNA was synthesized using MMLV reverse transcriptase (Takara) and oligo (dT)18 primer. Real-time reverse transcription (RT)-PCR was conducted in 20μl reactions including 20ng of cDNA, 0.2μM of a gene-specific primer for Avr3a or reference Actin gene (Supplementary Table S1), 10μl 2×TB Green Premix Ex Taq (Takara) to measure the transcript levels of five alleles of Avr3a in P. sojae isolates from China. Reactions were carried out on an ABI 7300 real-time PCR system (Applied Bio-systems, Foster City, CA, United States) under the following conditions: 95°C for 30s, 40cycles of 95°C for 5s, and 60°C for 31s to calculate cycle threshold (Ct) values, followed by a dissociation program of 95°C for 15s, 60°C for 1min, and 95°C for 15s to obtain melt curves. The relative expression level of each sample was determined using 7,300 system sequence detection software. The real-time RT-PCR assay was repeated three times, each with three independent biological replicates.
PCR Detection of Virulence
A PCR assay was conducted to detect the virulence of P. sojae to Rps3a as described by Dussault-Benoit et al. (2020). Briefly, the PCR was performed in a total volume of 25μl including 1×Taq Master Mix (Nobelab, Beijing, China), 400mM of forward and reverse primer, and 50ng template DNA. PCR amplifications were carried out in a TP600 Thermal Cycler with the following conditions: 1cycle at 94°C for 2min for initial denaturation, 32cycles at 94°C for 30s, 62°C for 30s, 72°C for 30s, and 2min of final extension at 72°C. PCR products were separated on 1.5% agarose gels in 0.5×TBE buffer, stained with 4S Green Nucleic Acid Stain (Sangon Biotech, Shanghai, China), and visualized using a Gel Doc XR+UV transilluminator (Bio-Rad, Hercules, CA, United States). The presence of an amplification product at the expected size was regarded as an avirulent isolate and vice versa.
Results
The Virulence of Phytophthora sojae Isolates From China on the Rps3a Soybean
The virulence of 32 P. sojae isolates from China was evaluated by hypocotyl split inoculation. All isolates were virulent on the soybean cultivar Williams (rps). Thirteen isolates were avirulent on the soybean cultivar L83-570 (Rps3a) and the other 19 were virulent (Table 1). Of the isolates, 59.38% were virulent on the Rps3a soybean, suggesting that most isolates are able to escape recognization of Rps3a in China and the Rps3a gene is not an effective Rps gene for controlling Phytophthora root and stem rot.
Polymorphism Analysis of Avr3a in Phytophthora sojae Isolates From China
To uncover the mechanism that Avr3a escapes recognition by Rps3a, sequence polymorphism of Avr3a was firstly investigated. A pair of Avr3a gene-specific primers, AVR3A1F/AVR3A1R (Supplementary Table S1), was used to amplify the full-length of Avr3a. The ~700bp fragments were amplified from all 32 isolates, cloned into a pMD19 vector, and sequenced. Five Avr3a alleles (GenBank accession numbers: MZ856318 to MZ856322) were identified in all sequenced isolates (Figure 1A), and two of them, Avr3a(II) and Avr3a(IV), were previously unreported alleles. Twenty-four mutations, including one deletion of six nucleotides and 23 single nucleotide polymorphisms (SNPs), were observed in the Avr3a coding region (Figure 1A). Two SNPs were synonymous substitutions, 20 SNPs were nonsynonymous substitutions, and one SNP caused a stop codon loss (Figure 1B). Among them, five SNPs were newly discovered mutations. Three new SNPs were from Avr3a(II), while the other two were from Avr3a(IV).
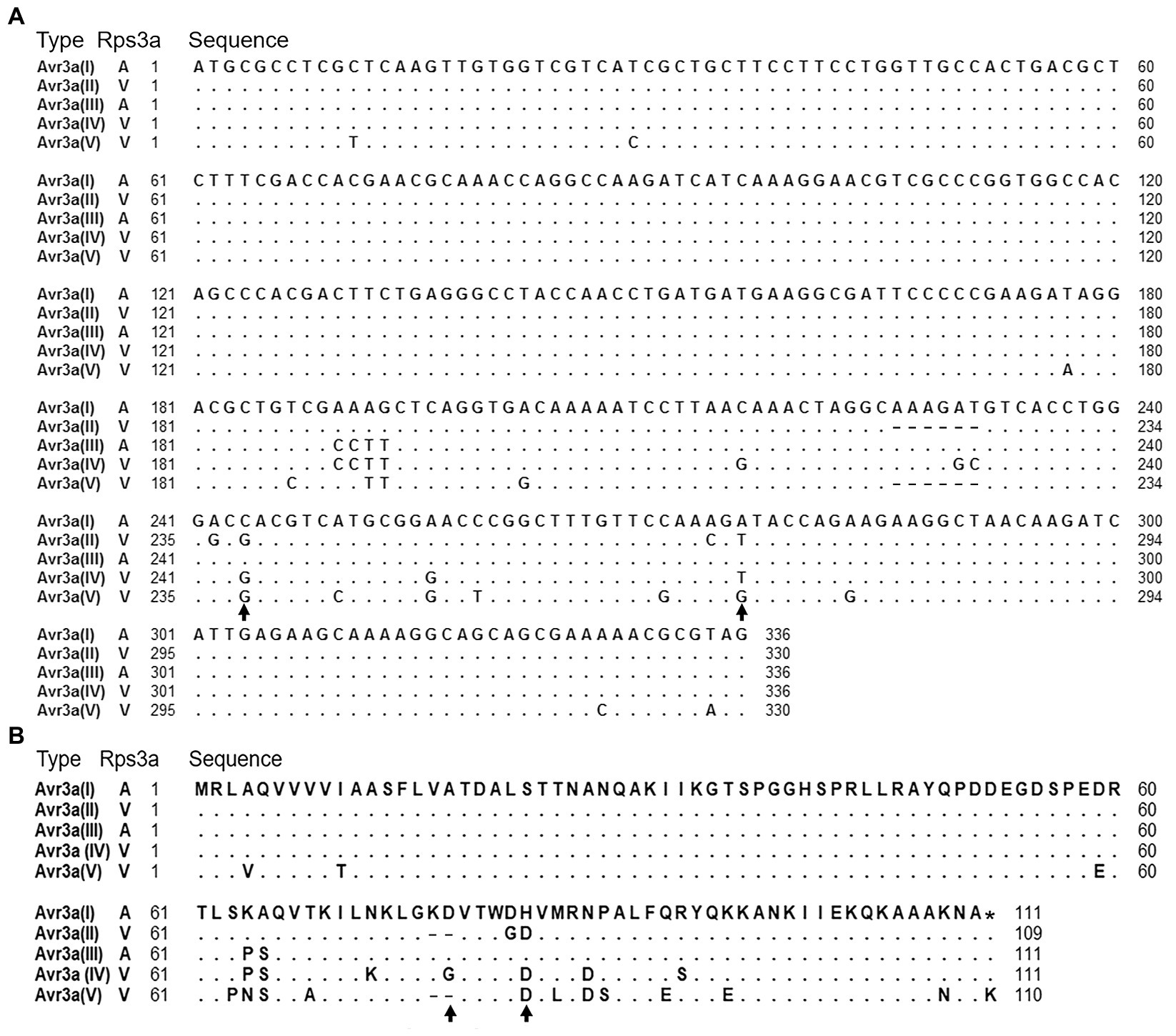
Figure 1. Sequence comparison of five Avr3a alleles. (A) Comparison of nucleotide sequences. Identities are indicated by dots and substitutions by the substituted nucleotides. Black arrows denote two nucleotides, which were different between avirulent alleles and virulent alleles. (B) Comparison of amino acid sequences. Black arrows denote two amino acids, which were different between avirulent alleles and virulent alleles.
Virulence tests showed that all the isolates containing Avr3a(I) or Avr3a(III) were avirulent to Rps3a and all the isolates containing Avr3a(II), Avr3a(IV), or Avr3a(V) were virulent to Rps3a (Table 1). In the coding regions of Avr3a(II), Avr3a(IV), and Avr3a(V), there were 5, 10, and 17 mutations, respectively, (Figure 1A). These mutations in the Avr3a coding region could cause the gain of virulence of P. sojae to Rps3a.
Transcriptional Analysis of Avr3a in Phytophthora sojae Isolates From China
The change in Avr gene expression is also an important strategy to escape recognization by Rps genes for P. sojae. To investigate whether the similar phenomenon for Avr3a occurs in this study, transcript levels of Avr3a were analyzed. A pair of gene-specific primers, AVR3A3F/AVR3A3R (Supplementary Table S1), was designed in the conserved regions of Avr3a to detect the transcript level of Avr3a using real-time RT-PCR. Ten isolates (Ps0702, Ps0708, Ps0714, Ps0301, Ps0905, Ps0705, Ps0903, Ps0904, Ps0302, and Ps0910), representative of five Avr3a alleles, were selected for transcriptional analysis. The results indicated that the Avr3a transcripts were detectable in the isolates containing Avr3a(I), Avr3a(II), Avr3a(III), and Avr3a(IV), but not in the isolates Ps0302 and Ps0910 containing Avr3a(V) (Figure 2). Transcriptional analysis suggested that the gain of virulence of the isolates Ps0302 and Ps0910 to Rps3a was due to the absence of Avr3a(V) transcript, not to DNA mutations in the coding regions.
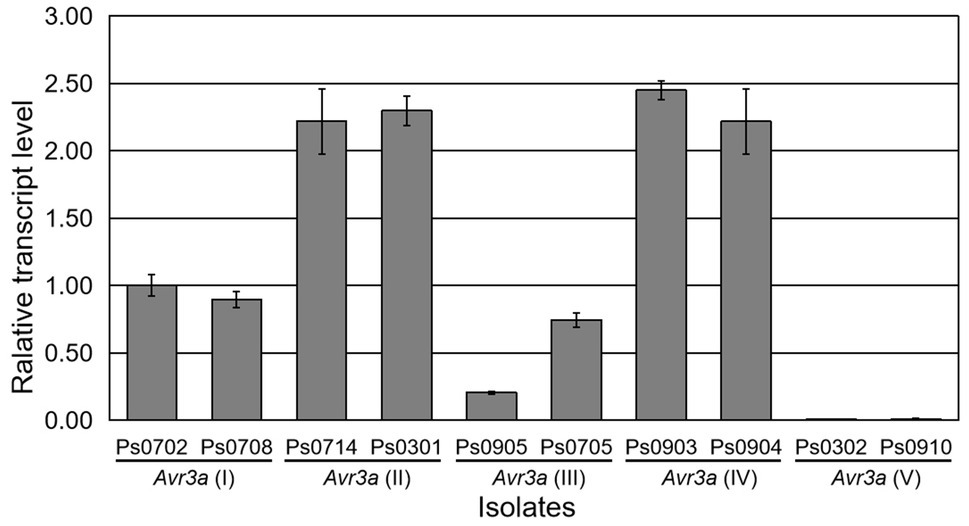
Figure 2. Transcriptional analysis of five Avr3a alleles. Expression levels relative to actin were calculated at 12h post-inoculation. The PCR reactions were replicated three times. Bars indicate standard errors.
Sequence Polymorphisms in the Promoter and 5'-Untranslated Regions of Avr3a
Considering the absence of Avr3a(V) transcript, sequence polymorphisms in the promoter and 5′-UTR regions of Avr3a were further explored. Eight representative isolates (Ps0702, Ps0708, Ps0714, Ps0301, Ps0905, Ps0705, Ps0903, and Ps0904) and all 15 isolates containing Avr3a(V) were selected for polymorphism analysis. A pair of gene-specific primers, AVR3A2F/AVR3A2R, was used to amplify the promoter and 5′-UTR regions (1,000bp upstream from the start codon) of Avr3a (Supplementary Table S1). The ~1,100bp fragments were amplified from all selected isolates and then sequenced. No mutations were found in the 5′-UTR region of Avr3a. Eleven mutations, including two deletions, four insertions, and five SNPs, were observed in the promoter region of Avr3a (Table 2). Ten mutations were observed in the promoter region of Avr3a(V) whose transcripts were not detectable, whereas only two mutations were observed in the promoter regions of Avr3a(I), Avr3a(II), Avr3a(III), and Avr3a(IV) whose transcripts were detectable. Eight mutations, including one deletion, three insertions, and four SNPs, were unique to Avr3a(V) and were found in all 15 isolates containing Avr3a(V) (Table 2), suggesting that these mutations in the promoter region could result in the loss of Avr3a(V) transcription and cause the gain of virulence to Rps3a.
Two SNP Markers for Virulent Alleles of Avr3a
Avr3a(I), Avr3a(II), Avr3a(III), and Avr3a(IV) were normally transcribed in P. sojae isolates. All the isolates containing Avr3a(I) or Avr3a(III) were avirulent to Rps3a and all the isolates containing Avr3a(II) and Avr3a(IV) were virulent (Table 1). Hence, Avr3a(I) and Avr3a(III) were avirulent alleles, while Avr3a(II) and Avr3a(IV) were virulent alleles. Transcribed virulent alleles of Avr3a were discovered for the first time. DNA mutations in the coding regions of Avr3a(II) and Avr3a(IV) caused the gain of virulence to Rps3a, which is a novel mechanism of virulence variation for Avr3a.
Although Avr3a(V) was not transcribed in P. sojae isolates, the previous study confirmed that Avr3aP7064, which is identical to Avr3a(V), did not interact with Rps3a by transient expression system, indicating that Avr3a(V) is also a virulent allele. Based on the evolutionary relationships of five Avr3a alleles (Supplementary Figure S1), these alleles were not able to be divided into avirulent and virulent groups. However, two SNPs, the 244th and 276th nucleotides, were identified which were different between two avirulent alleles and three virulent alleles (Figure 1A). The 244th nucleotide was a biallelic site, while the 276th nucleotide was a triallelic site. The 244th nucleotide was C in avirulent isolates and G in virulent isolates. Meanwhile, the 276th nucleotide was A in avirulent isolates and T/G in virulent isolates. These two SNP markers were able to differentiate virulent alleles from avirulent alleles accurately.
An Improved PCR Assay for Detecting the Virulence of Phytophthora sojae to Rps3a
Previously, a pair of allele-specific primers (Avr3aF/Avr3aR) designed by Dussault-Benoit et al. (2020) was used to detect the virulence of P. sojae to Rps3a by amplifying avirulent alleles of Avr3a (Supplementary Table S1). To determine if the primers are feasible for our isolates, five representative isolates (Ps0702, Ps0301, Ps0705, Ps0903, and Ps0302) in this study were tested. The ~600bp fragments were amplified from avirulent isolates Ps0702 and Ps0705. Regretfully, the same fragments were also amplified from virulent isolate Ps0903 with Avr3a(IV) (Figure 3B), despite using an annealing temperature of 62°C, suggesting that two SNPs of Avr3a(IV) in the middle position of the allele-specific forward primer (Avr3aF) could not prevent PCR amplification (Figure 3A). Given this, we designed a new allele-specific forward primer (Avr3aRF) to locate the two SNPs at the 3′ extremity (Figure 3A). The results from the improved PCR assay showed that the amplicons of Avr3a were present in two avirulent isolates but not in three virulent isolates (Figure 3C). The remaining 27 isolates were further tested to validate the accuracy of the improved PCR assay. The PCR results were completely consistent with the virulence of 27 isolates to Rps3a (Figure 3D), indicating that the improved PCR assay was accurate in detecting the virulence of all 32 isolates to Rps3a.
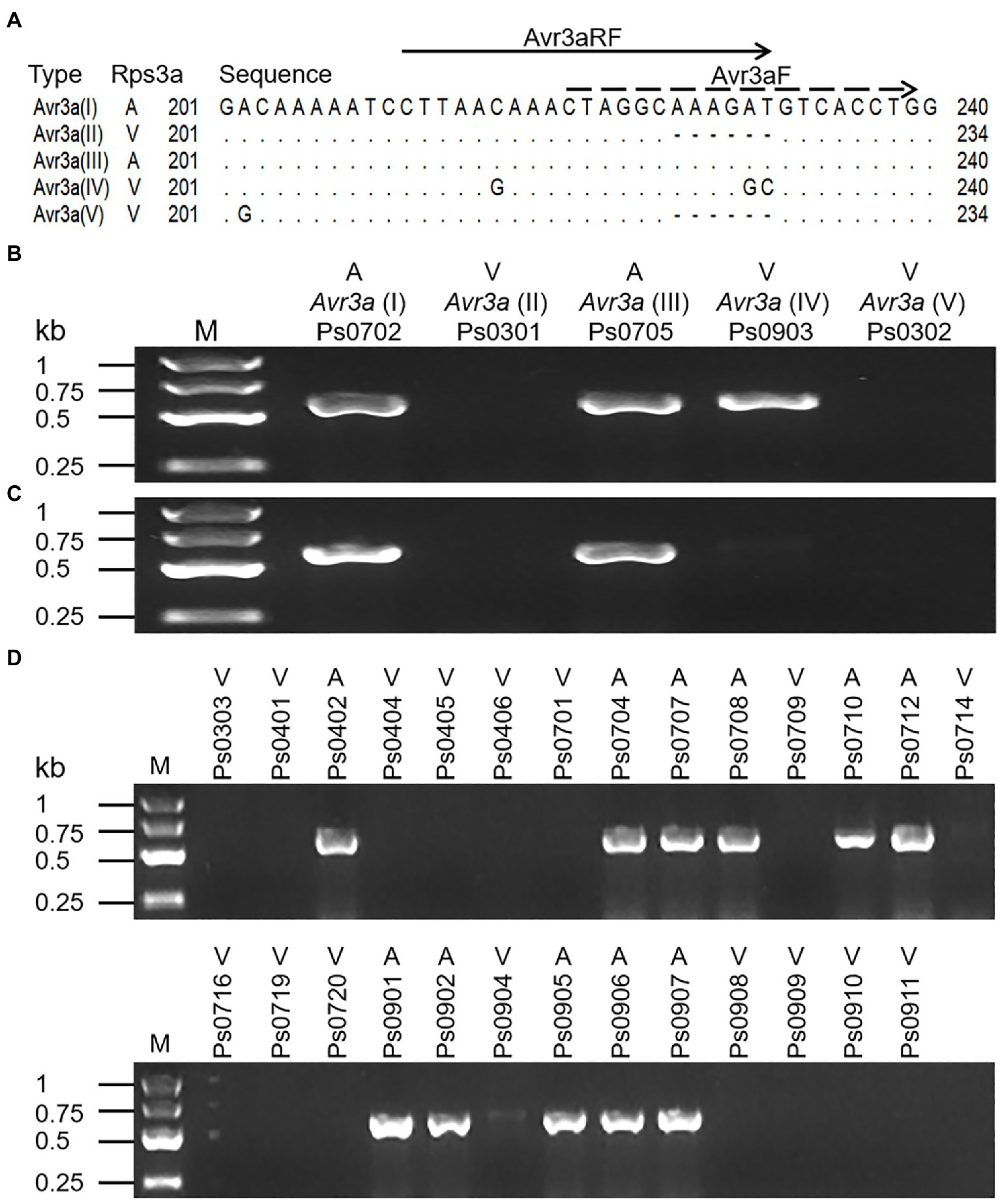
Figure 3. The PCR assays for the avirulence alleles of Avr3a. (A) Positions of the allele-specific forward primers. The dotted line shows the position of the allele-specific forward primer (Avr3aF) which designed by Dussault-Benoit et al. (2020). The solid line shows the position of the redesigned forward primer (Avr3aRF). The shared reverse primer (Avr3aR) is a gene-specific prime. A indicates an avirulent isolate or allele and V indicates a virulent isolate or allele. (B) The PCR assay of five Avr3a alleles with Avr3aF/Avr3aR. (C) The PCR assay of five Avr3a alleles with Avr3aRF/Avr3aR. (D) The PCR assay of 27 P. sojae isolates with Avr3aRF/Avr3aR.
Discussion
In this study, the polymorphism of Avr3a was analyzed detailedly in Chinese P. sojae isolates and a total of five Avr3a alleles were identified. Qutob et al. (2009) identified three Avr3a alleles in isolates P6497, ACR12 and fP7064. Avr3aP6497, Avr3aACR12, and Avr3aP7064 are the same as Avr3a(I), Avr3a(III), and Avr3a(V) identified in the present study, respectively. Arsenault-Labrecque et al. (2018) identified two Avr3a alleles from 31 Canadian isolates. The two Avr3a alleles are the same as Avr3a(I) and Avr3a(V), respectively. Avr3a(II) and Avr3a(IV) are novel alleles that have not been described previously. In the coding region of Avr3a, a total of 23 SNPs were observed, including 20 nonsynonymous SNPs and two synonymous SNPs. The ratio of the nonsynonymous substitution to synonymous substitution (dN/dS) rate was far greater than one, suggesting that the Avr3a gene was subject to strong positive selection pressure in China. The result is in line with the high virulence frequency to Rps3a in P. sojae populations from China (Zhang et al., 2008; Cui et al., 2010; Tian et al., 2016; Wu et al., 2017). Twenty-eight pathotypes were identified in the 32 isolates, indicating that there was abundant virulence diversity among these isolates (Supplementary Table S2). It might be because all isolates were isolated from soil samples. Another 30 isolates which were isolated recently in China were used to clone and analyze the avirulence gene Avr3a, but no novel alleles were discovered.
Two avirulent and three virulent alleles of Avr3a were identified in this study. By comparing the amino acid sequences between avirulent and virulent alleles, the 77th and 82nd amino acids were identified as key amino acids (Figure 1B). The 77th amino acid was aspartic acid in avirulent alleles and glutamic acid/deletion in virulent alleles. The 82nd amino acid was histidine acid in avirulent alleles and aspartic acid in virulent alleles. The two amino acids may be very important for Avr3a in the interaction between Avr3a and Rps3a. Based on the analysis, we will study the influence of key amino acid variations of Avr3a on the recognition of Rps3a in the future.
Transcriptional analysis indicated that the Avr3a transcript was non-detectable in the isolates containing Avr3a(V). Further research showed that there were 10 mutations in the promoter region of Avr3a(V). Of them, eight mutations, including one deletion, three insertions, and four SNPs, were unique to Avr3a(V) compared with other alleles and were found in all isolates containing Avr3a(V). These mutations, especially the 10bp and the 27bp insertions, could be located in the transcription factor binding sites or transcription elements and result in the loss of Avr3a(V) transcription. Dong et al. (2011b) also analyzed the polymorphisms in the promoter region of Avr3a, but only one deletion and two insertions were observed between P6497and P7064. More polymorphisms were observed in Chinese P. sojae isolates. Of the 19 virulent isolates, 15 isolates belonged to this type, which was the main escape mechanism of Avr3a in China. Avr3a(II) and Avr3a(IV) were normally transcribed in P. sojae isolates, but the isolates with Avr3a(II) and Avr3a(IV) were virulent to Rps3a. Polymorphism analysis indicated that there were five mutations in the coding region of Avr3a(II), and there were 10 mutations in the coding region of Avr3a(IV). Therefore, Avr3a(II) and Avr3a(IV) should escape recognition by Rps3a through DNA mutations in the Avr3a coding region, which is a novel escape mechanism of Avr3a. Only four virulent isolates belonged to this type which was the secondary escape mechanism of Avr3a in China. Furthermore, Qutob et al. (2013) found that isolate ACR10 escaped recognition by Rps3a through small RNA-mediated gene silencing. A total of three different mechanisms, including DNA mutations in the coding region, loss of transcription, and small RNA-mediated gene silencing, were identified up to now. This research provides new insights into escape mechanisms of the Avr3a gene.
Virulence structure of P. sojae should be constantly monitored to determine and deploy effective Rps genes (Dorrance et al., 2016; Stewart et al., 2016). Conventional virulence tests are labor-intensive and time-consuming, thus a rapid and simple PCR assay for pathotype identification of P. sojae was developed (Dussault-Benoit et al., 2020). However, when the PCR assay was used to detect the virulence of isolate Ps0903 with Avr3a(IV) to Rps3a in this study, a false positive result occurred. The existence of Avr3a(IV) could also be one of the main causes that lead to five cases of discrepancy between the phenotyping assays and the PCR assays in the previous research. The improved PCR assay was developed by redesigning the allele-specific forward primer and eliminated the false positive. Even so, false positive results can still be obtained using the improved PCR assay, because some isolates with an avirulent allele could still be virulent to Rps3a. For instance, isolate ACR10 with Avr3a(I) was virulent to Rps3a due to small RNA-mediated gene silencing (Qutob et al., 2013). Therefore, we suggest that the Avr3a transcript levels of P. sojae isolates should be analyzed firstly by real-time RT-PCR and the isolates lacking Avr3a mRNA are directly identified as virulent isolates. Subsequently, the isolates with Avr3a mRNA were selected for virulence identification by using the improved PCR assay. The combination of the two assays could be the best strategy to accurately detect the virulence of all known isolates to Rps3a.
Conclusion
In this study, two mechanisms of virulence variation for Avr3a were found in Chinese P. sojae populations. One was DNA mutations in the coding region of Avr3a that was a novel mechanism for Avr3a, and the other was the loss of transcription that was due to DNA mutations in the promoter region of Avr3a. In addition, three molecular markers were developed successfully for detecting the virulence of P. sojae to Rps3a. These findings may help us to understand how P. sojae overcome Rps3a at the molecular level in China and establish reliable molecular methods for pathotype identification of P. sojae.
Data Availability Statement
The datasets presented in this study can be found in online repositories. The names of the repository/repositories and accession number(s) can be found at: https://www.ncbi.nlm.nih.gov/, MZ856318 to MZ856322.
Author Contributions
LC designed the study, analyzed the data, and drafted the manuscript. YH and ZH performed all the experiments. YK reviewed and edited the manuscript. All authors have read and agreed to the published version of the manuscript.
Funding
This work was supported by the grant U1804104 and U1404318 of the National Science Foundation of China.
Conflict of Interest
The authors declare that the research was conducted in the absence of any commercial or financial relationships that could be construed as a potential conflict of interest.
Publisher’s Note
All claims expressed in this article are solely those of the authors and do not necessarily represent those of their affiliated organizations, or those of the publisher, the editors and the reviewers. Any product that may be evaluated in this article, or claim that may be made by its manufacturer, is not guaranteed or endorsed by the publisher.
Acknowledgments
We thank Prof. Yuanchao Wang from Nanjing Agricultural University for supplying seeds of soybean differentials and P. sojae isolates.
Supplementary Material
The Supplementary Material for this article can be found online at: https://www.frontiersin.org/articles/10.3389/fmicb.2021.759196/full#supplementary-material
References
Arsenault-Labrecque, G., Sonah, H., Lebreton, A., Labbé, C., Marchand, G., Xue, A., et al. (2018). Stable predictive markers for Phytophthora sojae avirulence genes that impair infection of soybean uncovered by whole genome sequencing of 31 isolates. BMC Biol. 16:80. doi: 10.1186/s12915-018-0549-9
Cui, L., Yin, W., Dong, S., and Wang, Y. (2012). Analysis of polymorphism and transcription of the effector gene Avr1b in Phytophthora sojae isolates from China virulent to Rps1b. Mol. Plant Pathol. 13, 114–122. doi: 10.1111/j.1364-3703.2011.00733.x
Cui, L., Yin, W., Tang, Q., Dong, S., Zheng, X., Zhang, Z., et al. (2010). Distribution, pathotypes, and metalaxyl sensitivity of Phytophthora sojae from Heilongjiang and Fujian provinces in China. Plant Dis. 94, 881–884. doi: 10.1094/PDIS-94-7-0881
Dong, S., Qutob, D., Tedman-Jones, J., Kuflu, K., Wang, Y., Tyler, B. M., et al. (2009). The Phytophthora sojae avirulence locus Avr3c encodes a multi-copy RXLR effector with sequence polymorphisms among pathogen strains. PLoS One 4:e5556. doi: 10.1371/journal.pone.0005556
Dong, S., Yin, W., Kong, G., Yang, X., Qutob, D., Chen, Q., et al. (2011a). Phytophthora sojae avirulence effector Avr3b is a secreted NADH and ADP-ribose pyrophosphorylase that modulates plant immunity. PLoS Pathog. 7:e1002353. doi: 10.1371/journal.ppat.1002353
Dong, S., Yu, D., Cui, L., Qutob, D., Tedman-Jones, J., Kale, S. D., et al. (2011b). Sequence variants of the Phytophthora sojae RXLR effector Avr3a/5 are differentially recognized by Rps3a and Rps5 in soybean. PLoS One 6:e20172. doi: 10.1371/journal.pone.0020172
Dorrance, A. E., Kurle, J., Robertson, A. E., Bradley, C. A., Giesler, L., Wise, K., et al. (2016). Pathotype diversity of Phytophthora sojae in eleven states in the United States. Plant Dis. 100, 1429–1437. doi: 10.1094/PDIS-08-15-0879-RE
Dorrance, A. E., Robertson, A. E., Cianzo, S., Giesler, L. J., Grau, C. R., Draper, M. A., et al. (2009). Integrated management strategies for Phytophthora sojae combining host resistance and seed treatments. Plant Dis. 93, 875–882. doi: 10.1094/PDIS-93-9-0875
Dou, D., Kale, S. D., Liu, T., Tang, Q., Wang, X., Arredondo, F. D., et al. (2010). Different domains of Phytophthora sojae effector Avr4/6 are recognized by soybean resistance genes Rps4 and Rps6. Mol. Plant-Microbe Interact. 23, 425–435. doi: 10.1094/MPMI-23-4-0425
Dussault-Benoit, C., Arsenault-Labrecque, G., Sonah, H., Belzile, F., and Bélanger, R. R. (2020). Discriminant haplotypes of avirulence genes of Phytophthora sojae lead to a molecular assay to predict phenotypes. Mol. Plant Pathol. 21, 318–329. doi: 10.1111/mpp.12898
Guérin, V., Lebreton, A., Cogliati, E. E., Hartley, S. E., Belzile, F., Menzies, J. G., et al. (2014). A zoospore inoculation method with Phytophthora sojae to assess the prophylactic role of silicon on soybean cultivars. Plant Dis. 98, 1632–1638. doi: 10.1094/PDIS-01-14-0102-RE
Lebreton, A., Labbe, C., de Ronne, M., Xue, A. G., Marchand, G., and Bélanger, R. R. (2018). Development of a simple hydroponic assay to study vertical and horizontal resistance of soybean and pathotypes of Phytophthora sojae. Plant Dis. 102, 114–123. doi: 10.1094/PDIS-04-17-0586-RE
Na, R., Yu, D., Chapman, B. P., Zhang, Y., Kuflu, K., Austin, R., et al. (2014). Genome re-sequencing and functional analysis places the Phytophthora sojae avirulence genes Avr1c and Avr1a in a tandem repeat at a single locus. PLoS One 9:e89738. doi: 10.1371/journal.pone.0089738
Qutob, D., Chapman, B. P., and Gijzen, M. (2013). Transgenerational gene silencing causes gain of virulence in a plant pathogen. Nat. Commun. 4:1349. doi: 10.1038/ncomms2354
Qutob, D., Tedman-Jones, J., Dong, S., Kuflu, K., Pham, H., Wang, Y., et al. (2009). Copy number variation and transcriptional polymorphisms of Phytophthora sojae RXLR effector genes Avr1a and Avr3a. PLoS One 4:e5066. doi: 10.1371/journal.pone.0005066
Ryley, M. J., Obst, N. R., Irwin, J. A. G., and Drenth, A. (1998). Changes in the racial composition of Phytophthora sojae in Australia between 1979 and 1996. Plant Dis. 82, 1048–1054. doi: 10.1094/PDIS.1998.82.9.1048
Sahoo, D. K., Abeysekara, N. S., Cianzio, S. R., Robertson, A. E., and Bhattacharyya, M. K. (2017). A novel Phytophthora sojae resistance Rps12 gene mapped to a genomic region that contains several Rps genes. PLoS One 12:e0169950. doi: 10.1371/journal.pone.0169950
Shan, W., Cao, M., Leung, D., and Tyler, B. M. (2004). The Avr1b locus of Phytophthora sojae encodes an elicitor and a regulator required for avirulence on soybean plants carrying resistance gene Rps1b. Mol. Plant-Microbe Interact. 17, 394–403. doi: 10.1094/MPMI.2004.17.4.394
Song, T., Kale, S. D., Arredondo, F. D., Shen, D., Liu, L., Wu, Y., et al. (2013). Two RxLR avirulence genes in Phytophthora sojae determine soybean Rps1k-mediated disease resistance. Mol. Plant-Microbe Interact. 26, 711–720. doi: 10.1094/MPMI-12-12-0289-R
Stewart, S., Robertson, A. E., Wickramasinghe, D., Draper, M. A., Michel, A., and Dorrance, A. E. (2016). Population structure among and within Iowa, Missouri, Ohio, and South Dakota populations of Phytophthora sojae. Plant Dis. 100, 367–379. doi: 10.1094/PDIS-04-15-0437-RE
Sukumaran, A., McDowell, T., Chen, L., Renaud, J., and Dhaubhadel, S. (2018). Isoflavonoid-specific prenyltransferase gene family in soybean: GmPT01, a pterocarpan 2-dimethylallyltransferase involved in glyceollin biosynthesis. Plant J. 96, 966–981. doi: 10.1111/tpj.14083
Tian, M., Zhao, L., Li, S., Huang, J., Sui, Z., Wen, J., et al. (2016). Pathotypes and metalaxyl sensitivity of Phytophthora sojae and their distribution in Heilongjiang, China 2011-2015. J. Gen. Plant Pathol. 82, 132–141. doi: 10.1007/s10327-016-0654-y
Tremblay, V., McLaren, D. L., Kim, Y. M., Strelkov, S., Conner, R., Wally, O., et al. (2021). Molecular assessment of pathotype diversity of Phytophthora sojae in Canada highlights declining sources of resistance in soybean. Plant Dis. [Preprint]. doi: 10.1094/PDIS-04-21-0762-RE
Tyler, B. M. (2007). Phytophthora sojae: root rot pathogen of soybean and model oomycete. Mol. Plant Pathol. 8, 1–8. doi: 10.1111/j.1364-3703.2006.00373.x
Tyler, B. M., Forster, H., and Coffey, M. D. (1995). Inheritance of avirulence factors and restriction fragment length polymorphism markers in outcrosses of the oomycete Phytophthora sojae. Mol. Plant-Microbe Interact. 8, 515–523. doi: 10.1094/MPMI-8-0515
Tyler, B. M., and Gijzen, M. (2014). “The Phytophthora sojae genome sequence: foundation for a revolution” in Genomics of Plant-Associated Fungi and Oomycetes: Dicot Pathogens. eds. R. A. Dean, A. Lichens-Park, and C. Kole (Springer), 133–157.
Wu, M., Li, B., Liu, P., Weng, Q., Zhan, J., and Chen, Q. (2017). Genetic analysis of Phytophthora sojae populations in Fujian, China. Plant Pathol. 66, 1182–1190. doi: 10.1111/ppa.12666
Ye, W., Wang, X., Tao, K., Lu, Y., Dai, T., Dong, S., et al. (2011). Digital gene expression profiling of the Phytophthora sojae transcriptome. Mol. Plant-Microbe Interact. 24, 1530–1539. doi: 10.1094/MPMI-05-11-0106
Yin, W., Dong, S., Zhai, L., Lin, Y., Zheng, X., and Wang, Y. (2013). Phytophthora sojae Avr1d gene encode an RxLR-dEER effector with presence and absence polymorphisms among pathogen strains. Mol. Plant-Microbe Interact. 26, 958–968. doi: 10.1094/MPMI-02-13-0035-R
Zhang, X., Liu, B., Zou, F., Shen, D., Yin, Z., Wang, R., et al. (2019). Whole genome re-sequencing reveals natural variation and adaptive evolution of Phytophthora sojae. Front. Microbiol. 10:2792. doi: 10.3389/fmicb.2019.02792
Zhang, S., Wu, J., Xu, P., Li, W., Zuo, Y., Qiu, L., et al. (2008). Identification of virulence of Phytophthora sojae in Heilongjiang province and the first report on race 15 in China. Chin. J. Oil Crop Sci. 30, 229–234. doi: 10.3724/SP.J.1011.2008.00534
Keywords: Phytophthora root and stem rot, avirulence gene, escape mechanism, virulence variation, DNA mutations, molecular markers
Citation: Hu Y, He Z, Kang Y and Cui L (2021) Mutations in the Promoter and Coding Regions of Avr3a Cause Gain of Virulence of Phytophthora sojae to Rps3a in Soybean. Front. Microbiol. 12:759196. doi: 10.3389/fmicb.2021.759196
Edited by:
Hossein Borhan, Agriculture and Agri-Food Canada (AAFC), CanadaReviewed by:
Yufeng Francis Fang, GreenLight Biosciences, United StatesWeixiao Yin, Huazhong Agricultural University, China
Maofeng Jing, Nanjing Agricultural University, China
Yuanchao Wang, Nanjing Agricultural University, China
Meixiang Zhang, Nanjing Agricultural University, China
Copyright © 2021 Hu, He, Kang and Cui. This is an open-access article distributed under the terms of the Creative Commons Attribution License (CC BY). The use, distribution or reproduction in other forums is permitted, provided the original author(s) and the copyright owner(s) are credited and that the original publication in this journal is cited, in accordance with accepted academic practice. No use, distribution or reproduction is permitted which does not comply with these terms.
*Correspondence: Linkai Cui, Y3VpbGtAaGF1c3QuZWR1LmNu