- Department Biological Safety, German Federal Institute for Risk Assessment, Berlin, Germany
Most strains of Vibrio parahaemolyticus are unable to utilize sucrose as carbon source, though few exceptions exist. We investigated a sucrose-positive V. parahaemolyticus strain by whole-genome sequencing (WGS) and confirmed the presences of a genomic island containing sucrose utilization genes. A 4.7 kb DNA cluster consisting of three genes: scrA encoding a sucrose uptake protein, scrK encoding a fructokinase, and scrB coding for a sucrose-6-phosphate hydrolase, was PCR amplified and inserted into the Vibrio/Escherichia coli shuttle vector pVv3. Two recombinant plasmids, only differing in the orientation of the insert with respect to the pVv3-lacZα-fragment, conferred the E. coli K12 transformants the ability to utilize sucrose. The introduction of the two plasmids into sucrose-negative V. parahaemolyticus and V. vulnificus strains also results in a change of the sucrose utilization phenotype from negative to positive. By performing a multiplex PCR targeting scrA, scrK, and scrB, 43 scr-positive V. parahaemolyticus isolates from our collection of retail strains were detected and confirmed to be able to use sucrose as carbon source. Strains unable to utilize the disaccharide were negative by PCR for the scr genes. For in-depth characterization, 17 sucrose-positive V. parahaemolyticus were subjected to WGS. A genomic island with a nucleotide identity of >95% containing scrA, scrB, scrK and three additional coding sequences (CDS) were identified in all strains. The additional genes were predicted as a gene coding for a transcriptional regulator (scrR), a porin encoding gene and a CDS of unknown function. Sequence comparison indicated that the genomic island was located in the same region of the chromosome II in all analyzed V. parahaemolyticus strains. Structural comparison of the genomes with sequences of the sucrose utilizing species V. alginolyticus revealed the same genomic island, which indicates a possible distribution of this genetic structure by horizontal gene transfer. The comparison of all genome sequences based on SNP differences reveals that the presence of sucrose utilizing genes is found in genetically diverse V. parahaemolyticus strains and is not restricted to a subset of closely related strains.
Introduction
Vibrionaceae are halophilic gram-negative bacteria and natural inhabitants of marine environments worldwide. Strains of Vibrio (V.) parahaemolyticus and V. vulnificus can cause gastrointestinal infections in humans through consumption of raw or undercooked seafood. V. vulnificus is also a notorious pathogen as it can cause wound infections with a fatal outcome (Baker-Austin et al., 2018). Vibrionaceae are present in a variety of aquatic environments and can be found in the water column, sediments or in association with other organisms (i.e., algae, fish, seafood). Due to the diversity of habitats Vibrio species are able to grow in a wide range of temperatures from 4 to 37°C (Farmer et al., 2015). They are facultative anaerobes capable of metabolizing many carbohydrates under aerobic or non-aerobic conditions. Differences in the ability to utilize sugars have been used as a general criterion for their biochemical identification and differentiation for a long time. Metabolization of the disaccharide sucrose is an important phenotype in the primary routine diagnostic of Vibrio species. Thiosulfate-Citrate-Bile-Sucrose (TCBS) agar is commonly used as first medium for the isolation of vibrios from different sources (Farmer et al., 2015; Hartnell et al., 2019). Colonies of Vibrio bacteria that utilize sucrose appear yellow on TCBS agar, while those unable to metabolize this sugar are green/blueish. Determination of sucrose utilization is also part in traditional biochemical testing series for species identification. Many food laboratories still use the biochemical identification of Vibrio isolates obtained from various seafood sources, as V. parahaemolyticus is a major cause of gastrointestinal infections worldwide (DIN EN ISO 21872-1:2017-10) (ISO 21872-1:2017-10, 2017).
Most strains of the species V. vulnificus and V. parahaemolyticus are unable to use sucrose as carbon source, although exceptions occur. While up to 15% of V. vulnificus strains are sucrose-positive, only ∼1% of the V. parahaemolyticus isolates were reported to ferment sucrose (Lam, 1985; Farmer et al., 2015). All strains of V. alginolyticus, a species frequently co-isolated with V. parahaemolyticus, are able to ferment sucrose. Thus, sucrose utilization represents an important phenotype for the differentiation of both species. Nowadays, commonly molecular techniques, like PCR, DNA sequencing or MALDI-ToF mass spectrometry are used for rapid and reliable species identification (Bauer and Roervik, 2007; Dieckmann et al., 2010). However, a sucrose-positive phenotype will not be detected by using these techniques in the current routine diagnostic.
Sucrose uptake and degradation in V. alginolyticus follow a pathway that is common for carbohydrate utilization in many eubacteria. Sucrose is taken up and translocated into the bacterial cell by phosphorylation through a sugar specific enzyme of the phosphenolpyruvate (PEP):carbohydrate phosphotransferase system (PTS) (Postma et al., 1993; Deutscher et al., 2006). Inside the bacterial cell sucrose-6-phosphate is cleaved by hydrolysis of the glycosidic bond. The two resulting monosaccharides glucose-6-phosphate and fructose enter the glycolytic pathway directly or in case of fructose are phosphorylated first (Reid and Abratt, 2005). The genetics of sucrose uptake and degradation of V. alginolyticus was characterized and all required genes were found organized in a cluster (Scholle et al., 1987, 1989; Blatch et al., 1990; Blatch and Woods, 1991). The cluster contains the gene for a sugar specific PTS gene (scrA) for uptake of the disaccharide. Downstream of scrA, a gene for fructokinase (scrK) and a gene encoding sucrose-6-phosphate hydrolase (scrB) are located. Upstream of scrA a gene encoding a regulator (scrR) is found whose transcription is in the opposite direction to scrA, scrK, and scrB. In one recent paper the genetics of sucrose metabolism in several Vibrio and Photobacterium species was addressed (Abushattal et al., 2020) and in a second paper the genes of a sucrose-positive V. parahaemolyticus isolate were identified (De Mesa et al., 2021). The arrangement of sucrose utilization genes of most species correspond to that of V. alginolyticus.
In the past, we obtained a number of sucrose-positive V. parahaemolyticus strains from different food sources. To elucidate the genetic background enabling sucrose utilization of these isolates, we performed whole-genome sequencing (WGS) and bioinformatics analysis of one isolate. We also identified a gene cluster (abbreviated scr gene cluster) which showed high similarity to the V. alginolyticus cluster. We cloned the corresponding region of genes responsible for translocation and metabolization of sucrose into a Vibrio/Escherichia coli shuttle cloning vector. Resulting recombinant plasmids were introduced into sucrose-negative strains of V. vulnificus and V. parahaemolyticus to analyze the sucrose phenotype of the transformants. A multiplex PCR targeting the individual determinants of the scr gene cluster was developed to elucidate their genetic dissection in other scr-positive V. parahaemolyticus isolates. WGS analyses on more sucrose-positive strains were performed to determine the genetic arrangement in all sequenced strains and to find out if sucrose utilization is restricted to phylogenetically closely related strains.
Materials and Methods
Bacterial Strains, Culture Conditions, and Initial Identification
Bacterial strains used for cloning and expression of the scr genes are displayed in Table 1. Information regarding the source and time of isolation of Vibrio strains are shown in Table 2. Most V. parahaemolyticus strains were sent to the Vibrio consultant laboratory hosted at the BfR from official German federal state office laboratories, which investigate seafood for microbiological safety. All strains used in this study were routinely grown on lysogeny broth (LB) containing yeast extract (5 g/L), peptone (10 g/L), NaCl (10 g/L) or LB agar (Merck, Darmstadt, Germany). For selective cultivation of strains carrying the shuttle vector pVv3 (Klevanskaa et al., 2014) or its derivatives, kanamycin sulfate (Roth, Karlsruhe, Germany) was supplemented to a final concentration of 100 μg/mL (w/v).
Identification of V. parahaemolyticus strains was carried out using phenotypical tests, e.g., cultivation on TCBS agar (Oxoid, Wesel, Germany) and Vibrio Chrom-Agar (MAST-Diagnostika, Reinfeld, Germany), growth tolerance against different NaCl concentrations (0, 2, 6, 8, and 10%) in peptone water (Bacto Peptone, Gibco/Thermo Fisher Scientific, Schwerte, Germany; NaCl Merck, Darmstadt, Germany), API20E profiling (biomérieux, Nürtingen, Germany) and oxidase test (Merck, Darmstadt, Germany). Furthermore, PCR targeting the toxR gene (Bauer and Roervik, 2007; Bechlars et al., 2015) and Matrix-Assisted-Laser-Desorption/Ionization Time-of-Flight Mass Spectrometry (MALDI-ToF MS) (Biotyper microflex, LT/SH, Bruker, Bremen, Germany) was conducted as previously described (Jäckel et al., 2020). The test of sucrose fermentation was conducted by using sucrose bouillon with bromothymol blue containing peptone from meat (10 g/L), NaCl (5 g/L), and sucrose (10 g/L) (Merck, Darmstadt, Germany). 5 mL of sucrose broth was inoculated with bacterial strains and incubated for 24–48 h at 37°C. Sucrose-positive strains turn the media from blueish green to yellow.
Molecular Amplification of the scr Genes
Genomic DNA (gDNA) extraction from V. parahaemolyticus strain 16-VB00198 was carried out by using the RTP Bacteria DNA Kit (Stratec Molecular, Berlin, Germany) according to the recommendations of the manufacturer and was used for multiplex PCR amplification of the scr gene cluster. PCR reactions for the detection of the scrA, scrB, and scrK genes were performed in a volume of 25 μl with 1x PCR buffer (2 mM MgCl2), 0.2 mM of each dNTP, 0.5 μM of each primer, and 1.5 U of Dream Taq DNA Polymerase (Fermentas, St. Leon-Rot, Germany). The following PCR running conditions were used: An initial denaturation of 94°C for 2 min, 35 cycles of 94°C for 15 s, 58°C for 30 s, and 72°C for 30 min, and a final elongation step of 72°C for 5 min. The sequences of the used oligonucleotide primers are given in Table 3.
Cloning of scr Gene Cluster
For the amplification of the complete scr gene cluster comprising scrA, scrK, and scrB and the flanking regions, the PhusionTM High-Fidelity DNA Polymerase Kit (ThermoFisher Scientific, Schwerte, Germany) was used with the primers VparascrAB-Fo and VparascrAB-Re (Table 3). In a volume of 50 μl, 1 x PCR buffer (2 mM MgCl2), 0.2 mM of each dNTP, 0.5 μM of primers and 1.5 U of PhusionTM Polymerase (Thermo Fisher Scientific, Schwerte, DE) were used for amplification. PCR conditions were applied as stated below: An initial denaturation of 98°C for 2 min, 35 cycles of 98°C for 10 s, 61.6°C for 30 s and 72°C for 2 min, and a final elongation step of 72°C for 5 min. For further downstream analysis, the PCR products were purified using the RTP Kit INVITEK according to the manufacturer’s recommendations (Stratec Molecular, Berlin, Germany). A 4,656 bp PCR fragment containing the scr operon was obtained from V. parahaemolyticus 16-VB00168. The fragment was digested with the enzymes BamHI and HindIII (Thermo Fisher Scientific, Schwerte, Germany), whose recognition sequences were included in the sequences of the primers VparascrAB-Fo and VparascrAB-Re (Table 3). The digested PCR fragment was inserted into the corresponding sites of the pUC18 cloning vector (Thermo Fisher Scientific, Schwerte, Germany) using T4 ligase (Thermo Fisher Scientific, Schwerte, Germany). After transformation of electrocompetent E. coli GeneHogsTM cells, transformants containing the recombinant plasmid consisting of pUC18 with the inserted scr operon were obtained on LB agar with ampicillin (Merck, Darmstadt, DE) (100 mg/mL). Plasmid DNA from transformants was isolated using the RTP plasmid Kit according to the manufacturer’s recommendation and subjected to Sanger sequencing (Eurofins Genomics, Ebersberg, Germany) for verification.
The verified scr region was excised from the recombinant pUC18 derivative by BamHI/HindIII cleavage and inserted into the corresponding sites of the pVv3 shuttle cloning vector (accession number HG326273) (Klevanskaa et al., 2014). To avoid re-ligation of the vector DNA, BamHI/HindIII cleaved pVv3 was incubated with alkaline phosphatase (Thermo Fisher Scientific, Schwerte, Germany) before T4 ligase treatment. Ligation mixtures were subjected to transformation to electrocompetent E. coli GeneHogs cells. Transformed bacteria were selected on LB agar supplemented with kanamycin (100 μg/mL) and the presence of the src gene cluster was confirmed by primer walking (Supplementary Table S1) and Sanger sequencing (Eurofins Genomics, Ebersberg, Germany). pVv3 plasmid or derivative isolation was conducted using Qiagen Plasmid Plus Kits (Qiagen GmbH, Hilden, Germany).
Preparation of competent cells of E. coli GeneHogsTM was done following a standard protocol (Sambrook and Russel, 2001). The preparation of electrocompetent Vibrio cells was conducted as described previously (Klevanskaa et al., 2014). For electroporation, 200–400 ng gDNA and 40–50 μl freshly prepared competent cells were mixed, and incubated on ice for 10 min. Electroporation was performed at settings of 7.5 kV cm–1, 25 μF, and 200 Ω. Thereafter, 950 μl SOC medium (Sambrook and Russel, 2001) was added, and the bacteria were incubated for 37°C for 1 h. Afterward the bacteria were plated on LB agar under selective conditions, but potential Vibrio transformants were investigated by MALDI-ToF MS before further processing.
Whole-Genome Sequence Determination and Bioinformatics Analysis
Whole-genome sequencing was performed for 17 V. parahaemolyticus isolates. Therefore, isolates were grown in LB and gDNA was extracted using the PureLink Genomic DNA Kit (Invitrogen, Karlsruhe, Germany). Paired-end, short-read WGS (MiSeq, Illumina, San Diego, CA, United States) was performed as previously described (Schwartz et al., 2019). SPAdes de novo assemblies using raw reads and genome annotation were performed using the Pathosystems Resource Integration Center (PATRIC) (release 3.5.39) (Wattam et al., 2017) and the automated Prokaryotic Genome Annotation Pipeline (PGAP) of the National Center for Biotechnology Information (NCBI), respectively.
Average nucleotide identity (ANI) prediction was used for assigning genomes at species level (Rodriguez and Konstantinidis, 2014). Genome sequences were compared pairwise to the genome of the V. parahaemolyticus type strain ATCC 17802 (accession NZ_LATW01000001-51). The ANI online calculation tool1 was used with default settings (alignment options: minimum length 700 bp, minimum identity 70%, minimum alignments 50; fragment options: window size 1,000 bp, step size 200 bp).
Multilocus sequence typing (MLST) was conducted using MLST Finder (Larsen et al., 2012), which is based on the V. parahaemolyticus scheme of the pubMLST database2 (Jolley et al., 2018). Prediction of putative prophage sequences was performed using the PHAge Search Tool Enhanced Release (PHASTER) with default settings (Arndt et al., 2016). Initial plasmid replicon prediction was performed using PlasmidFinder (v 2.03) (Carattoli et al., 2014). In addition, genomic contigs showing significantly higher sequence coverages than the rest of the contigs were screened for similarities to known plasmids using the BlastN algorithm of the NCBI database All BlastN searches were carried out using the NCBI database (https://blast.ncbi.nlm.nih.gov/Blast.cgi?PROGRAM=blastn&PAGE_TYPE=BlastSearch&LINK_LOC=blasthome) with default settings.
To determine the phylogenetic relationship of the isolates, a CSI Phylogeny (v 1.44) based single nucleotide polymorphism (SNP) tree was prepared. The tool was used under default settings and the exclusion of heterozygous SNPs. As reference for comparison of the sucrose-positive strains the WGS dataset of 16-VB00498 was used. Nucleotide variations were predicted according to the specifications provided (Kaas et al., 2014).
The relation between the sucrose region (scrA-scrK-scrB) was determined by using MEGA X (Kumar et al., 2018) with the Maximum Likelihood method and Tamura–Nei model (Tamura and Nei, 1993). Initial tree(s) were obtained automatically by applying Neighbor-Join and BioNJ algorithms. Bootstrapping was performed with 500 repetitions using all sites.
Accession Numbers
Genome sequences of the investigated V. parahaemolyticus isolates have been deposited in GenBank at the National Center for Biotechnology Information (NCBI) under the accession numbers given in Supplementary Table S2.
Results
Identification of a Sucrose Utilization (scr) Gene Cluster in Vibrio parahaemolyticus 16-VB00198
16-VB00198 was assigned to the species V. parahaemolyticus by MALDI-ToF MS and PCR targeting toxR (data not shown). The strain was positive for sucrose utilization when tested with API20E strips. Furthermore, it changed the color from blue to yellow when grown in sucrose bouillon indicating that it is able to utilize sucrose as sole carbon source.
Whole-genome sequencing and ANI calculation showed a result of 98.2% identity between 16-VB00198 and the V. parahaemolyticus type strain ATCC17805 (WDCM00037). Through BlastN searches using published genes involved in sucrose metabolism, a CDS cluster consisting of three scr genes was identified. Nucleotide sequence (accession M30194) containing the sucrose uptake region with scrA and scrB genes of V. alginolyticus (Blatch et al., 1990) showed a nucleotide identity of 97% to a DNA region (contig_16) of 16-VB00198. Further bioinformatic analysis revealed that the strain contains a sucrose utilization gene cluster containing three genes (scrA, scrK, and scrB) arranged head to tail in the order 5′-3′. The scrA gene encodes a sucrose uptake protein of the phosphoenolpyruvate dependent phosphotransferase system (PTS), scrK encodes a fructokinase and scrB a sucrose-6-phosphate hydrolase (Scholle et al., 1987, 1989; Blatch and Woods, 1991; Postma et al., 1993). Upstream of scrA a gene coding for a transcriptional regulator is located whose coding sequence is counter orientated to scrA.
Cloning of the scrAKB Gene Cluster in Escherichia coli
To examine the functionality of the scrA-scrK-scrB cluster, the region was amplified by PCR with a High FidelityTM DNA-polymerase using forward and reverse primers containing the restriction sites HindIII and BamHI, respectively. The 4,656 bp PCR fragment was digested with both restriction enzymes and ligated with BamHI/HindIII cleaved DNA of the high copy number E. coli cloning vector pUC18 (AmpR) (Norrander et al., 1983). After transformation of E. coli K12, a recombinant plasmid with the scr region was isolated from ampicillin-resistant transformants (data not shown).
The verified pUC18 (scrAKB) derivative was used for re-cloning of the 16-VB00198 scrA-scrK-scrB cluster into the Vibrio/E. coli shuttle vector pVv3 (KmR) (Klevanskaa et al., 2014). Finally, through selection of kanamycin-resistant E. coli K12 two recombinant plasmids derived from pVv3 were obtained. Both plasmids differed in the orientation of the inserted scr fragment with respect to the transcription direction of the lacZα-fragment (Figure 1) and were further verified by commercial Sanger sequencing. In the recombinant plasmid pC50, the 30 bp BamHI-HindIII fragment from the multiple cloning site of pVv3 is inserted on both sides of the scr fragment resulting in an opposite transcription direction of the scr genes and the lacZα-fragment. In contrast, pC98 exhibited the same transcription direction of scr genes and lacZα-fragment. Out of the literature (Blatch et al., 1990), two promoter and three transcription terminator sequences were deduced within the cloned fragment (Figure 1B), which are up to now not experimentally verified.
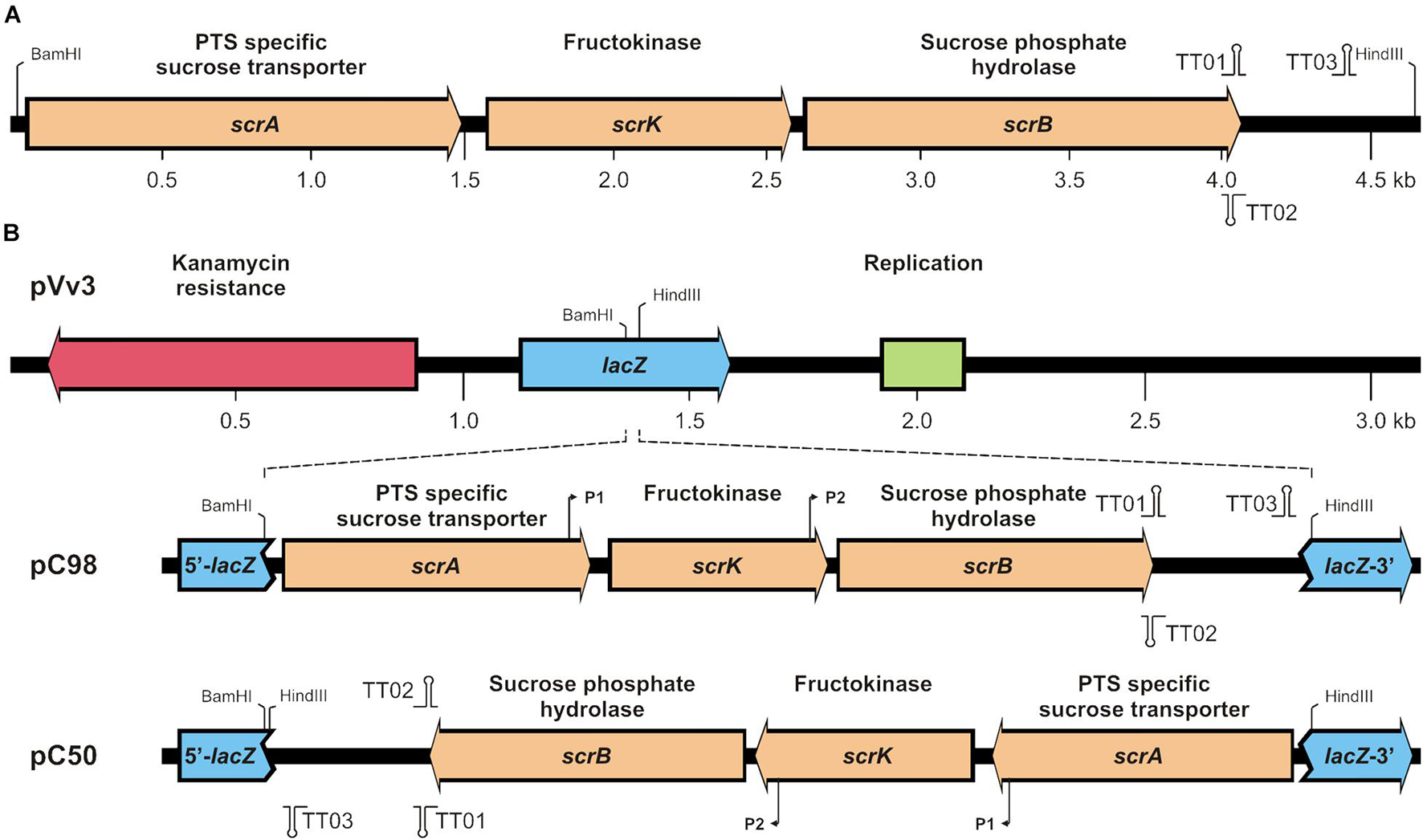
Figure 1. (A) Genetic map of the scrA-K-B region of strain V. parahaemolyticus 16-VB00198 and (B) integration of the cluster into shuttle vector pVv3. In (B) lacZ fragment containing a multiple cloning site the single restriction sites BamHI and HindIII are shown in pVv3. In recombinant plasmids pC98 and pC50 putative promoters (P1, P2) and transcription terminators (TT01, TT02, TT03) are indicated.
Expression of the scr Gene Cluster in Escherichia coli and Vibrio spp.
Escherichia coli K12 strains are unable to utilize sucrose (Schmid et al., 1988). However, it was shown that after introduction of the V. alginolyticus scr gene cluster into E. coli K12, the clones are able to metabolize sucrose (Scholle et al., 1987). The expression of the scr genes of V. parahaemolyticus 16-VB00198 in E. coli K12 was tested in sucrose bouillon. While the original strain (GeneHogsTM ) and its derivative carrying the vector pVv3 were negative for sucrose utilization, transformants harboring the recombinant plasmids pC50 or pC98 were capable of metabolizing the disaccharide (Figure 2A). To confirm the E. coli K12 transformants, a multiplex PCR targeting scrA, scrB, and scrK was applied using specific primers (Table 3). PCR amplification yielded PCR products of the expected sizes (Figure 2B).
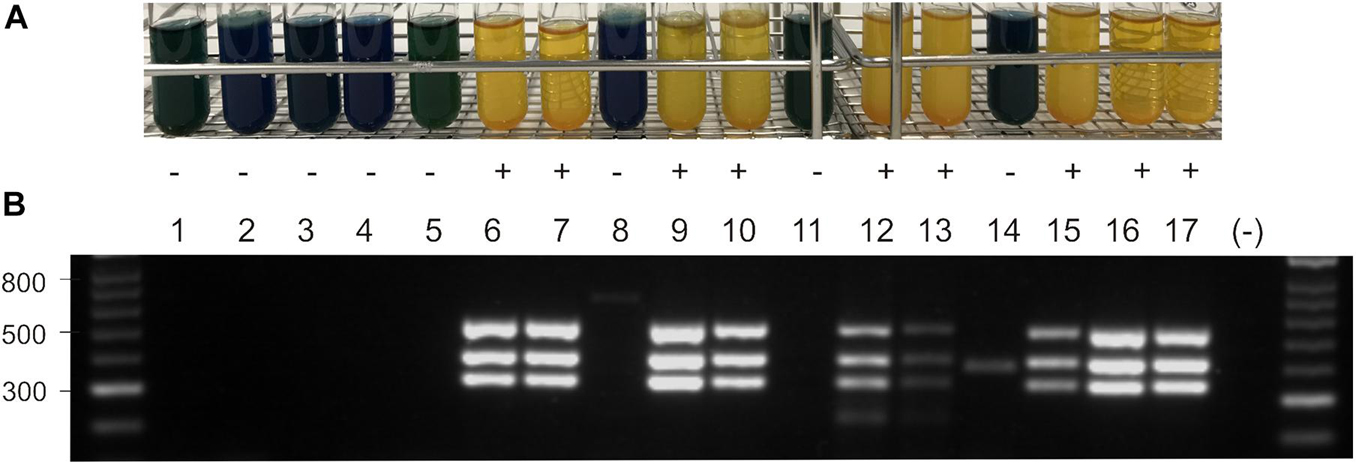
Figure 2. (A) Sucrose utilization of E. coli and Vibrio strains without plasmid, with vector pVv3, and with recombinant plasmids pC50 and pC98 in sucrose bouillon after growth at 37°C. Yellow color indicates growth (+) dark blue color no growth (–). (B) Agarose gel electrophorese of PCR products obtained with primers for scrA, scrK and scrB gene (331 bp, 410 bp, 527 bp; see Table 2). DNA ladder in bp, lane (–) shows PCR water control. Lane 1: E. coli K12, lane 2: BfR-VB00009, lane 3: BfR-VB00016, lane 4: BfR-VB00034, lane 5: E. coli K12 [pVv3], lane 6: E. coli K12 [pC50], lane 7: E.coli K12 [pC98], lane 8: BfR-VB00009 [pVv3], lane 9: BfR-VB00009 [pC50], lane 10: BfR-VB00009 [pC98], lane 11: BfR-VB00016 [pVv3], lane 12: BfR-VB00016 [pC50], lane 13: BfR-VB00016 [pC98], lane 14: BfR-VB00034 [pVv3], lane 15: BfR-VB00034 [pC50], lane 16: BfR-VB00034 [pC98], and lane 17: V. parahaemolyticus 16-VB00198.
To test if sucrose-negative V. parahaemolyticus and V. vulnificus can be converted to utilize the sugar, plasmids pC50 or pC98 were introduced into selected strains by electroporation using a protocol developed for pVv3 (Klevanskaa et al., 2014). Following transformation of three Vibrio strains (Table 1), kanamycin-resistant transformants were tested for sucrose utilization by growth in sucrose bouillon (Figure 2A). The transformants of the two V. parahaemolyticus strains (BfR-VB00009 and BfR-VB00016) and the V. vulnificus strain BfR-VB00034 were shown to be able to metabolize the sugar. The presence of the scrABK genes was also confirmed by scr multiplex PCR (Figure 2B).
Presence of scr Cluster in Sucrose-Positive Vibrio parahaemolyticus Strains
To determine the occurrence of the scr gene cluster among Vibrio strains of our culture collection, a number of sucrose-positive V. parahaemolyticus strains was subjected to PCR application using the above described scr multiplex PCR. In total, 43 sucrose-positive strains, which had been found through biochemical testing were investigated for the presence of the scr genes. From all sucrose-positive strains the three expected PCR products were obtained confirming the presence of the scr DNA region (data not shown). Additionally, 10 sucrose-negative V. parahaemolyticus strains were tested by PCR, but did not yield any visible PCR products confirming that the PCR is specific for the detection of the scr gene cluster.
Genetic Diversity of Sucrose-Positive Vibrio parahaemolyticus Strains
Whole-genome sequencing was performed to find out if the sucrose cluster is restricted to a small group of genetically related V. parahaemolyticus strains. In total, 17 sucrose-positive strains from our collection originating from different geographical origin, host animals and time point of isolation were selected for further dissection of their genetic basis. The resulting genomes of the strains were analyzed with respect to their phylogenetic relationship and their scr regions. WGS revealed that the genome sizes of the strains varied between 4.9 and 5.9 Mb (Supplementary Table S2). A MLST analysis of all 17 strains revealed that some strains belong to the same MLST sequence type (ST), however, several diverse alleles of housekeeping genes are found (see below).
The genomic region encompassing the coding sequences of the scrA, scrK, and scrB genes including the intergenic sequences (in total 4,027 bp) were analyzed using the Maximum Likelihood method. The sequences were assigned to three clusters, which are indicated by different colors (Figure 3, left half). The nucleotide identity of the scr regions of the strains ranged between 95.8 and 100%.
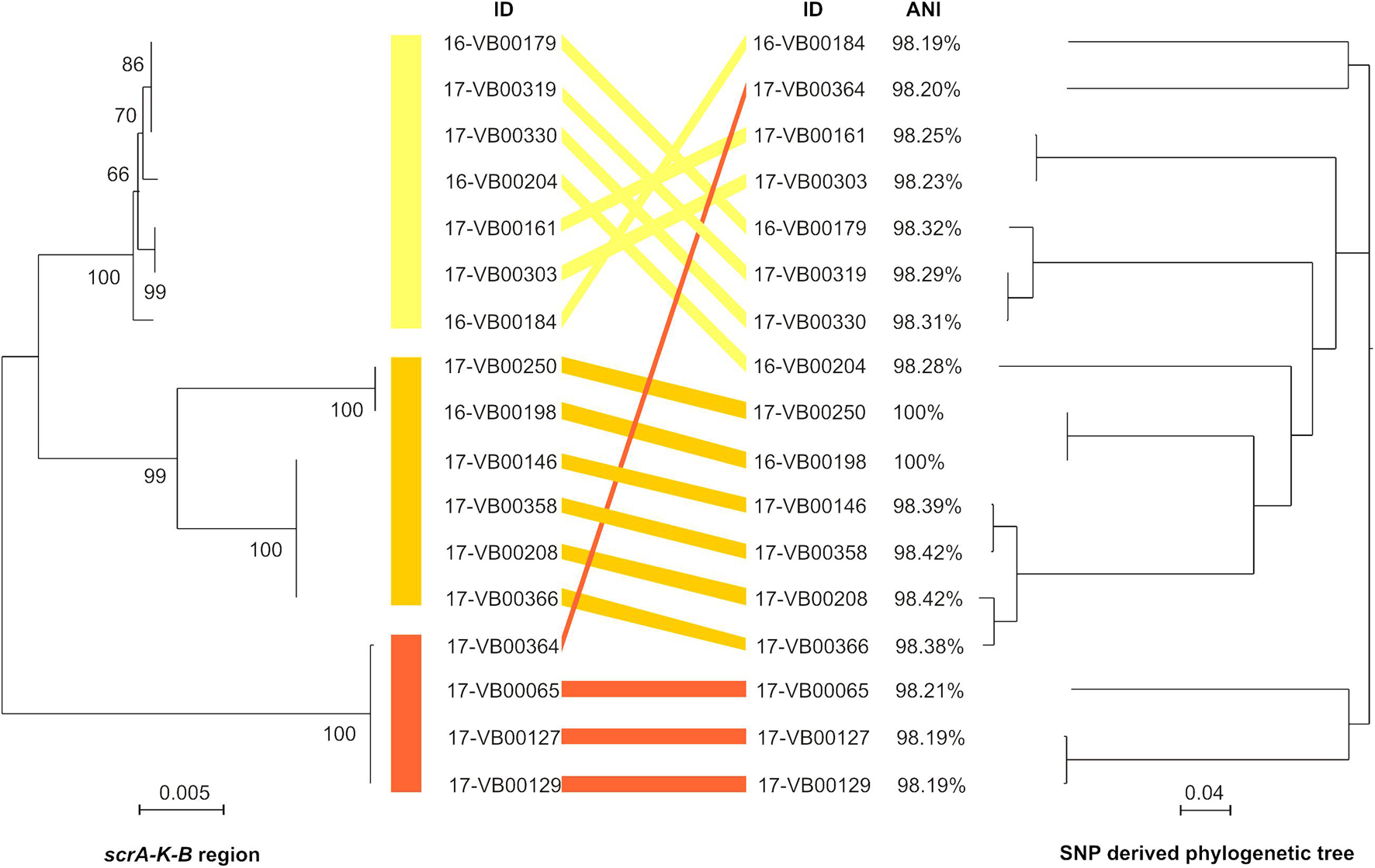
Figure 3. Comparison of sucrose scrAKB region (left half of figure) with an SNP-derived phylogeny tree (right half of figure) from 17 sucrose-positive V. parahaemolyticus strains. Left half: The evolutionary analyses for the scrAKB region was carried out by using the Maximum Likelihood method with Mega X (see section “Materials and Methods”). In the final dataset, 4,027 positions were included. The branch length is measured in number of substitutions per site and drawn to scale. Bootstrap values are shown at the nods. Right half: SNP tree was conducted using CSI Phylogeny 1.4 under default settings and the exclusion of heterozygous SNPs. Single nucleotide polymorphisms (SNPs) were called by mapping the genome of strain 16-VB00198 as reference. Scale bar represents the number of nucleotide substitutions per site. ANI value for each genome was calculated using the 16-VB00198 genome as reference.
For determination of the phylogenetic relationship of the strains, the genome of 16-VB00198 was chosen as a reference for a SNP analysis. CSI phylogeny was used for SNP tree generation out of 17 sucrose-positive strains (see Figure 3, right half). In Supplementary Table S3, the SNP counts are displayed numerically. The percentage of the reference genome covered by all isolates is 73.59%, so 4,359,330 nucleotides represent the basis of the SNP comparison.
The SNP tree shows a distinct diversity between the sucrose-positive strains, as the SNP counts range between 7 (minimum) and 40,506 (maximum). The strains 16-VB00184, 16-VB00204, 17-VB00364, and 17-VB00065 differ between each other and all other strains by more than 35,000 SNPs. WGS also revealed that a number of strains with low differences in SNP counts between each other are closely related isolates. Such strains probably belong to the same yet unassigned multilocus sequence type (ST) according to the V. parahaemolyticus pubMLST scheme. In Supplementary Table S2, the alleles of housekeeping genes and their resulting STs are given. For unknown STs, the nearest alleles were given (Jolley et al., 2018).
As expected, the strains belonging to the same ST, are highly identical and exhibit scrAKB region only differing in a few nucleotides. In conclusion, the comparison of all genome sequences based on SNP differences reveals that the presence of sucrose utilizing genes is found in genetically diverse strains and is not restricted to a subset of closely related strains.
Additionally, the ANI values of all strains were calculated using the genome of 16-VB00198 as reference. The ANI values differ between 98.19 and 98.42% when compared to the reference. The genome of strain 17-VB00250 is 100% identical to the reference genome, corresponding to the low the difference in SNPs (only seven SNPs). The ANI values are displayed in Figure 3, however, their distinctiveness is less than that of the SNP analysis.
Integration Site of scr Gene Cluster Within the Vibrio parahaemolyticus Genome
We compared the scrAKB region of strain V. parahaemolyticus 16-VB00198 to the genome of the V. alginolyticus reference strain ATCC 33787 (accession CP013485). In general, these two Vibrio species are related belonging to the Vibrio harveyi clade (Ke et al., 2018) and sucrose utilization has been used as an important phenotypic feature for biochemical differentiation of these bacteria. The comparison of the scr region of the two Vibrio strain genomes is depicted in Figure 4A. The cloned region is part of a genomic island, which may have been acquired through horizontal gene transfer from V. alginolyticus. In this species all strains are sucrose-positive (Farmer et al., 2015), whereas in V. parahaemolyticus 99% of the strains are unable to utilize sucrose. In V. parahaemolyticus 16-VB00198, the sucrose genes scrA, scrK, and scrB are part of a genomic island of approximately 8.0 kb. The island has an overall nucleotide similarity of >95% to the region of V. alginolyticus. Interestingly, the location of the scr gene cluster was determined to be at the same position in chromosome II of V. alginolyticus and V. parahaemolyticus. In the flanking sequences of the scr genomic island the similarity between the chromosomal DNA sequences of the two species drops to approximately 70%. The identified scr island contains additional coding sequences (CDS) in both species: scrR, a gene coding for a putative transcriptional regulator upstream of scrA, a gene encoding a porin protein possibly involved in disaccharide uptake and a CDS of a hypothetical protein. The direction of transcription of all island genes is depicted in Figure 4A. Downstream of the scrB gene, the genomic island ends in a short pseudogene sequence (110 bp), which is derived from a gene encoding a dihydrofolate reductase. On the 5′ end of the scr genomic island the same genes encoding metabolic enzymes are present in both strains (immediately upstream of the island is a gene for a ß-ketoacyl-ACP reductase). On the 3′ end of the V. parahaemolyticus island the first common gene with a chromosomal gene of V. alginolyticus is a gene coding for a S-glutathione transferase. Two genes present in V. alginolyticus ATCC 33787 between the scrB gene of the genomic island and the S-glutathione transferase gene are absent in V. parahaemolyticus 16-VB00198. In the 17 genomes of the sucrose-positive V. parahaemolyticus strains, the same arrangement of genes is conserved. The overall nucleotide identity of the scr genomic island and its flanking chromosomal region among the V. parahaemolyticus strains is higher than 97% (14 kb of contig_16 of 16-VB00198 from nucleotide position 25,001–40,000). The GC contents of the 8 kb region of the scr island of each V. parahaemolyticus genome (start codon of the hypothetical gene to the stop codon of scrB gene) was calculated and compared to the GC contents of the corresponding whole genome. In all 17 cases the GC contents of the scr island is 1.5% lower than that of the whole genome (see Supplementary Table S2). This result supports the hypothesis of acquisition of the scr island through horizontal gene transfer.
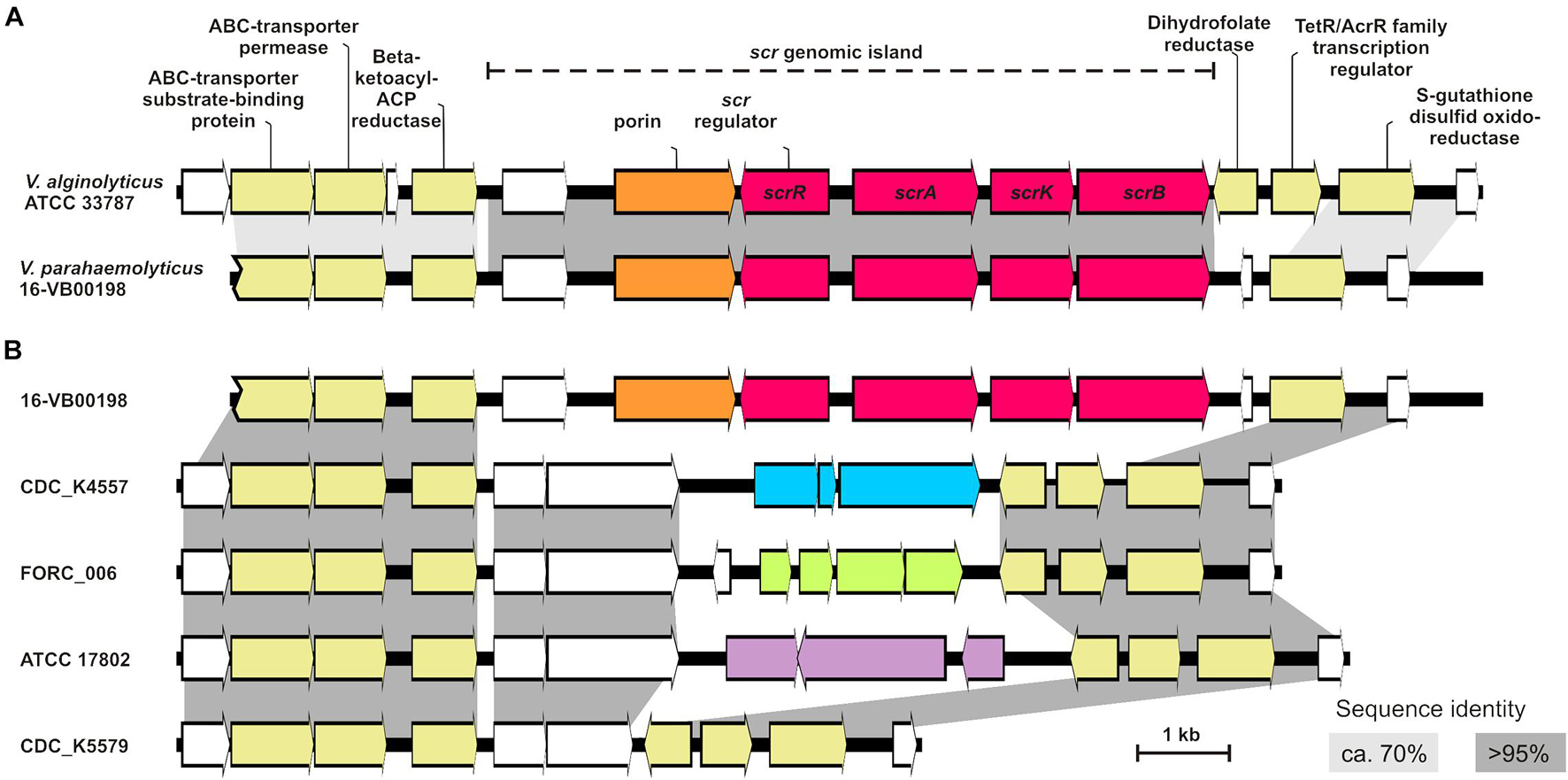
Figure 4. (A) Comparison of the genetic region with the sucrose metabolizing genes (scr island) of strain V. parahaemolyticus 16-VB00198 to the corresponding region of V. alginolyticus ATCC 33787 (accession CP013485). scr genes are colored red, porin-coding genes in orange, hypothetical genes are in white, and annotated genes are in yellow. (B) Comparison of the genetic region of chromosome II of 16-VB00198 to the corresponding region of sucrose-negative V. parahaemolyticus strains (CDC-K4557 accession CP006007, strain FORC-006 accession CP009766, strain ATCC 17802 accession LATW01000005, strain CDC_K5579 accession MIVB01000118). Gene clusters in blue, in green and in purple indicate annotated genes with different functions. Sequence identity is shown in light gray (approximately 70%) and in dark gray (>95%).
We also performed BlastN searches with the chromosomal scr region of V. alginolyticus ATCC 33878 (accession CP013485; nucleotide position 1,012,100 to 1,027,000) against other published V. alginolyticus genomes. In some V. alginolyticus strains, the region contains the same number and identical arrangement of genes as depicted in Figure 4A. However, in various other V. alginolyticus strains the two genes between scrB and the S-glutathione transferase gene are missing (just as with the sucrose-positive V. parahaemolyticus strains, data not shown).
We were furthermore interested which genes are present in the corresponding chromosome II region of sucrose-negative V. parahaemolyticus strains (Figure 4B). This genomic region displays a greater genetic variability between the strains. Downstream of the gene encoding ß-ketoacyl-ACP reductase two conserved genes encoding proteins of unknow function are common in the analyzed strains (indicated by dark gray boxes, Figure 4B). Downstream of these two hypothetical protein CDS, more genetic variability is found. Four examples of genomes of sucrose-negative strains are shown in Figure 4B: In strains CDC-K4557, FORC-006, and ATCC 17802 different gene clusters follow (see accession for details), whereas in strain CDC_K5579 nothing more than the two common hypothetical proteins CDS are present. In all genomes of sucrose-negative strains two genes for the dihydrofolate reductase gene and the transcription regulator are present upstream of the S-glutathione transferase gene as found in the genome of V. alginolyticus ATCC 33878 (Figure 4B).
Discussion
The aim of our study was to characterize the genetic background for sucrose utilization in sucrose-positive strains of the species V. parahaemolyticus, of which most strains are unable to metabolize sucrose as carbon source. By BlastN comparison with known genes of the related species V. alginolyticus (Scholle et al., 1987, 1989; Blatch et al., 1990; Blatch and Woods, 1991) the genes scrA (encoding a sucrose uptake protein), scrK (coding for a fructokinase) and scrB (coding for a sucrose-6-phosphate hydrolase) were identified. The proteins of these genes degrade the disaccharide to phosphorylated sugars (fructose-6-phosphate and glucose-6-phosphate) which enter the glycolytic pathway (Reid and Abratt, 2005). The scrA gene product is a sucrose-specific protein of the phosphoenolpyruvate dependent phosphotransferase system (PTS) which transports sucrose through the inner membrane by phosphorylation of the disaccharide (Reid and Abratt, 2005; Deutscher et al., 2006).
We inserted the three genes into the Vibrio/E. coli shuttle vector pVv3 (Klevanskaa et al., 2014) and constructed two recombinant plasmids pC50 and pC98 containing the gene cluster in different orientations. Usually E. coli K12 strains are unable to ferment sucrose (Schmid et al., 1988). However, the derivative strains carrying pC50 or pC98 grew in sucrose bouillon. A similar result was reported for the V. alginolyticus scrAKB genes (Scholle et al., 1987) when introduced into E. coli K12. After transformation of the recombinant plasmid into sucrose-negative V. parahaemolyticus and V. vulnificus strains, the transformants were able to utilize the disaccharide. In the three species, the expression of the scr genes was independent of their transcription orientation in respect to the lacZα-fragment suggesting that the expression is driven by promoters within the pVv3 plasmid. Thus, we demonstrated that these three enzymes are sufficient for the metabolization of the disaccharide for catabolic pathways. By performing a multiplex PCR targeting scrA, scrK, and scrB we confirmed that sucrose utilizing V. parahaemolyticus strains harbor this gene cluster, as all 43 sucrose-positive isolates from our strain collection were positive. The sequence similarity between all scr clusters was higher than 95.8%. Whole genome sequencing confirmed that several strains are genetically highly diverse (>35,000 SNPs) and do not belong to a genetically related subset of strains.
In two recent publications the sucrose utilization in Vibrio and the related species Photobacterium was addressed. One paper describes the presence of a sucrose utilization cluster in a sucrose utilizing V. parahaemolyticus isolate from a shrimps aquaculture (De Mesa et al., 2021). The strain was subjected to WGS and in a bioinformatic approach the genome was compared to that of a sucrose-negative strain. The authors identified the same arrangement of sucrose utilizing genes in the sucrose-positive strain, as determined in this study (De Mesa et al., 2021). Upstream of the scrA-K-B region in the analyzed V. parahaemolyticus strain also a scrR gene and a porin gene are present. The putative scrR gene encodes a LacI-GalR family transcription regulator controlling expression of the scrB gene in response to sucrose (Reid and Abratt, 2005). Upstream of scrR a gene encoding a porin is located in all strains. This porin belongs to the LamB family whose members are able to bind various sugars and to transport them through the outer membrane. A related porin is the Salmonella typhimurium sucrose-specific porin ScrY (Reid and Abratt, 2005). In our genetic constructs, the porin gene of the scr island of V. parahaemolyticus was not included. However, the metabolization of sucrose in transformants of V. parahaemolyticus and V. vulnificus show that other porins in these Vibrio strains can transport the disaccharide through the outer membrane.
The arrangement of scr genes in Photobacterium damselae, a marine species belonging to the family Vibrionaceae, is also very similar to that of V. alginolyticus and V. parahaemolyticus (Abushattal et al., 2020). The authors performed a bioinformatic analysis for scr genes in several published genomes of Vibrio and Photobacterium species which revealed the arrangement of scrA, scrK, and scrB with the putative regulator gene scrR upstream of scrA in many species of these two genera (Abushattal et al., 2020). The V. alginolyticus sucrose uptake and utilization system represents the prototype for the metabolization of this disaccharide in many species of the Vibrionaceae.
In Photobacterium damselae two direct repeat sequences flanking the four scr genes were identified and it was hypothesized that these sequences may be involved in horizontal gene transfer (Abushattal et al., 2020). We could not find repeat sequences flanking the scr genomic cluster in our V. parahaemolyticus genomes. All 17 scr clusters were integrated in the same site between genes, which are located in chromosome II. For this reason, a plasmid borne origin of the scr genomic island is in our investigated sucrose-positive stains highly unlikely. Horizontal gene transfer (HGT) is a widely used mechanism in which bacteria exchange genetic material. The close similarity of the scr genomic islands between V. alginolyticus and V. parahaemolyticus suggests that this genetic structure was acquired by HGT. This hypothesis is also supported by comparison of the GC contents of the scr islands to the GC contents of the whole genomes in all 17 sucrose-positive V. parahaemolyticus strains. The GC content of the scr islands is 1.5% lower than that of the complete genome in all strains.
In conclusion, we characterized a genomic island within V. parahaemolyticus strains, which carries genes encoding proteins for uptake and degradation of sucrose. We could verify the function of three genes scrA, scrK, and scrB by cloning and expression in V. parahaemolyticus and V. vulnificus. Recombinant pVv3 plasmids with the scr genomic island were also functional in E. coli K12. A multiplex PCR targeting the three scr genes showed that this gene cluster is present in sucrose-positive V. parahaemolyticus strains. By WGS of 17 sucrose-positive V. parahaemolyticus we could show that the genes are arranged in a conserved genomic island which is closely related to the scr genomic region of V. alginolyticus. The island is found in genetically diverse strains and not restricted to a small subgroup of V. parahaemolyticus strains. Food laboratories testing biochemical phenotypes could use the multiplex PCR targeting the scrAKB genes if sucrose-positive V. parahaemolyticus isolates are detected.
Data Availability Statement
This Whole Genome Shotgun project has been deposited at DDBJ/ENA/GenBank under the accession numbers given in Supplementary Table S2.
Author Contributions
ES and JH designed the study. CG, CJ, FS, and HG performed the experiments. JH, CG, and ES analyzed the data. ES and JH prepared the tables and figures. All the authors edited the manuscript.
Funding
This work was supported by a grant of the German Federal Institute for Risk Assessment (45-009).
Conflict of Interest
The authors declare that the research was conducted in the absence of any commercial or financial relationships that could be construed as a potential conflict of interest.
Publisher’s Note
All claims expressed in this article are solely those of the authors and do not necessarily represent those of their affiliated organizations, or those of the publisher, the editors and the reviewers. Any product that may be evaluated in this article, or claim that may be made by its manufacturer, is not guaranteed or endorsed by the publisher.
Acknowledgments
The authors thank Jonas Nekat and Nicole vom Ort for excellent technical assistance and Stephen Marino for advice on data interpretation.
Supplementary Material
The Supplementary Material for this article can be found online at: https://www.frontiersin.org/articles/10.3389/fmicb.2021.754464/full#supplementary-material
Footnotes
- ^ enve-omics.ce.gatech.edu/ani/
- ^ pubmlst.org/organisms?title=vibrio+parahemolyticus
- ^ cge.cbs.dtu.dk/services/PlasmidFinder/
- ^ cge.cbs.dtu.dk/services/CSIPhylogeny/
References
ISO 21872-1:2017-10 (2017). Microbiology of the Food Chain - Horizontal Method for the Determination of Vibrio spp. - Part 1: detection of Potentially Enteropathogenic Vibrio Parahaemolyticus, Vibrio Cholerae and Vibrio. Berlin: Beuth publishing DIN.
Abushattal, S., Vences, A., Barca, A. V., and Osorio, C. R. (2020). Diverse Horizontally-Acquired Gene Clusters Confer Sucrose Utilization to Different Lineages of the Marine Pathogen Photobacterium damselae subsp. damselae. Genes 11:1244. doi: 10.3390/genes11111244
Arndt, D., Grant, J. R., Marcu, A., Sajed, T., Pon, A., Liang, Y., et al. (2016). PHASTER: a better, faster version of the PHAST phage search tool. Nucleic Acids Res. 44, W16–W21. doi: 10.1093/nar/gkw387
Baker-Austin, C., Oliver, J. D., Alam, M., Ali, A., Waldor, M. K., Qadri, F., et al. (2018). Vibrio spp. infections. Nat. Rev. Dis. Primers. 4:8. doi: 10.1038/s41572-018-0005-8
Bauer, A., and Roervik, L. M. (2007). A novel multiplex PCR for the identification of Vibrio parahaemolyticus, Vibrio cholerae and Vibrio vulnificus. Lett. Appl. Microbiol. 45, 371–375. doi: 10.1111/j.1472-765X.2007.02195.x
Bechlars, S., Jäckel, C., Diescher, S., Wustenhagen, D. A., Kubick, S., Dieckmann, R., et al. (2015). Characterization of trh2 harbouring Vibrio parahaemolyticus strains isolated in Germany. PLoS One 10:e0118559. doi: 10.1371/journal.pone.0118559
Blatch, G. L., Scholle, R. R., and Woods, D. R. (1990). Nucleotide sequence and analysis of the Vibrio alginolyticus sucrose uptake-encoding region. Gene 95, 17–23. doi: 10.1016/0378-1119(90)90408-J
Blatch, G. L., and Woods, D. R. (1991). Nucleotide sequence and analysis of the Vibrio alginolyticus scr repressor-encoding gene (scrR). Gene 101, 45–50. doi: 10.1016/0378-1119(91)90222-W
Carattoli, A., Zankari, E., Garcia-Fernandez, A., Voldby Larsen, M., Lund, O., Villa, L., et al. (2014). In silico detection and typing of plasmids using PlasmidFinder and plasmid multilocus sequence typing. Antimicrob. Agents Chemother. 58, 3895–3903. doi: 10.1128/AAC.02412-14
De Mesa, C. A. E., Mendoza, R. M., Amar, E. C., De La Peña, L. D., and Saloma, C. P. (2021). Identification of a chromosomally-encoded sucrose operon-like gene cluster in Vibrio parahaemolyticus strain PH05 isolated from Negros Island, Philippines. Biorxiv doi: 10.1101/2021.02.27.433172
Deutscher, J., Francke, C., and Postma, P. W. (2006). How phosphotransferase system-related protein phosphorylation regulates carbohydrate metabolism in bacteria. Microbiol. Mol. Biol. Rev. 70, 939–1031. doi: 10.1128/MMBR.00024-06
Dieckmann, R., Strauch, E., and Alter, T. (2010). Rapid identification and characterization of Vibrio species using whole-cell MALDI-TOF mass spectrometry. J. Appl. Microbiol. 109, 199–211. doi: 10.1111/j.1365-2672.2009.04647.x
Farmer, J. J. III, Brenner, F. W., Cameron, D. N., Birkhead, K. M., and Janda, J. M. (2015). “Vibrio” in Bergey’s Manual of Systematics of Archaea and Bacteria 3 Edn. ed. W. B. Whitman (United States: John Wiley & Sons, Inc). doi: 10.1002/9781118960608.gbm01078
Hartnell, R. E., Stockley, L., Keay, W., Rosec, J. P., Hervio-Heath, D., Van Den Berg, H., et al. (2019). A pan-European ring trial to validate an International Standard for detection of Vibrio cholerae, Vibrio parahaemolyticus and Vibrio vulnificus in seafoods. Int. J. Food Microbiol. 288, 58–65. doi: 10.1016/j.ijfoodmicro.2018.02.008
Jäckel, C., Hammerl, J. A., Arslan, H. H., Göllner, C., Vom Ort, N., Taureck, K., et al. (2020). Phenotypic and Genotypic Characterization of Veterinary Vibrio cincinnatiensis Isolates. Microorganisms 8:739. doi: 10.3390/microorganisms8050739
Jolley, K. A., Bray, J. E., and Maiden, M. C. J. (2018). Open-access bacterial population genomics: bIGSdb software, the PubMLST.org website and their applications. Wellcome Open Res. 3:124. doi: 10.12688/wellcomeopenres.14826.1
Kaas, R. S., Leekitcharoenphon, P., Aarestrup, F. M., and Lund, O. (2014). Solving the problem of comparing whole bacterial genomes across different sequencing platforms. PLoS One 9:e104984. doi: 10.1371/journal.pone.0104984
Ke, H. M., Liu, D., Ogura, Y., Hayashi, T., Urbanczyk, H., and Tsai, I. J. (2018). Tracing Genomic Divergence of Vibrio Bacteria in the Harveyi Clade. J. Bacteriol. 200:e00001–18. doi: 10.1128/JB.00001-18
Klevanskaa, K., Bier, N., Stingl, K., Strauch, E., and Hertwig, S. (2014). PVv3, a new shuttle vector for gene expression in Vibrio vulnificus. Appl. Environ. Microbiol. 80, 1477–1481. doi: 10.1128/AEM.03720-13
Kumar, S., Stecher, G., Li, M., Knyaz, C., and Tamura, K. (2018). MEGA X: molecular Evolutionary Genetics Analysis across Computing Platforms. Mol. Biol. Evol. 35, 1547–1549. doi: 10.1093/molbev/msy096
Lam, S. (1985). A mucoid, sucrose-positive Vibrio parahaemolyticus strain isolated from diarrhoeal stool. Singapore Med. J. 26, 479–481.
Larsen, M. V., Cosentino, S., Rasmussen, S., Friis, C., Hasman, H., Marvig, R. L., et al. (2012). Multilocus sequence typing of total-genome-sequenced bacteria. J. Clin. Microbiol. 50, 1355–1361. doi: 10.1128/JCM.06094-11
Norrander, J., Kempe, T., and Messing, J. (1983). Construction of improved M13 vectors using oligodeoxynucleotide-directed mutagenesis. Gene 26, 101–106. doi: 10.1016/0378-1119(83)90040-9
Postma, P. W., Lengeler, J. W., and Jacobson, G. R. (1993). Phosphoenolpyruvate:carbohydrate phosphotransferase systems of bacteria. Microbiol. Rev. 57, 543–594. doi: 10.1128/mr.57.3.543-594.1993
Reid, S. J., and Abratt, V. R. (2005). Sucrose utilisation in bacteria: genetic organisation and regulation. Appl. Microbiol. Biotechnol. 67, 312–321. doi: 10.1007/s00253-004-1885-y
Rodriguez, R. L., and Konstantinidis, K. T. (2014). Bypassing Cultivation To Identify Bacterial Species. Microbe 9, 111–118. doi: 10.1128/microbe.9.111.1
Sambrook, J., and Russel, D. W. (2001). Molecular Cloning: a Laboratory Manual. New York: Cold Spring Harbor Laboratory Press.
Schmid, K., Ebner, R., Altenbuchner, J., Schmitt, R., and Lengeler, J. W. (1988). Plasmid-mediated sucrose metabolism in Escherichia coli K12: mapping of the scr genes of pUR400. Mol. Microbiol. 2, 1–8. doi: 10.1111/j.1365-2958.1988.tb00001.x
Scholle, R. R., Coyne, V. E., Maharaj, R., Robb, F. T., and Woods, D. R. (1987). Expression and regulation of a Vibrio alginolyticus sucrose utilization system cloned in Escherichia coli. J. Bacteriol. 169, 2685–2690. doi: 10.1128/jb.169.6.2685-2690.1987
Scholle, R. R., Robb, S. M., Robb, F. T., and Woods, D. R. (1989). Nucleotide sequence and analysis of the Vibrio alginolyticus sucrase gene (scrB). Gene 80, 49–56. doi: 10.1016/0378-1119(89)90249-7
Schwartz, K., Hammerl, J. A., Göllner, C., and Strauch, E. (2019). Environmental and Clinical Strains of Vibrio cholerae Non-O1, Non-O139 from Germany Possess Similar Virulence Gene Profiles. Front. Microbiol. 10:733. doi: 10.3389/fmicb.2019.00733
Tamura, K., and Nei, M. (1993). Estimation of the number of nucleotide substitutions in the control region of mitochondrial DNA in humans and chimpanzees. Mol. Biol. Evol. 10, 512–526.
Keywords: Vibrio parahaemolyticus, metabolic activity, cloning, shuttle vector pVv3, whole-genome sequencing
Citation: Hammerl JA, Göllner C, Jäckel C, Swidan F, Gutmann H and Strauch E (2021) The Acquisition of the scr Gene Cluster Encoding Sucrose Metabolization Enzymes Enables Strains of Vibrio parahaemolyticus and Vibrio vulnificus to Utilize Sucrose as Carbon Source. Front. Microbiol. 12:754464. doi: 10.3389/fmicb.2021.754464
Received: 06 August 2021; Accepted: 13 October 2021;
Published: 15 November 2021.
Edited by:
Dario De Medici, National Institutes of Health (ISS), ItalyReviewed by:
Aoife Boyd, National University of Ireland Galway, IrelandGraciela Dias, Federal University of Rio de Janeiro, Brazil
Copyright © 2021 Hammerl, Göllner, Jäckel, Swidan, Gutmann and Strauch. This is an open-access article distributed under the terms of the Creative Commons Attribution License (CC BY). The use, distribution or reproduction in other forums is permitted, provided the original author(s) and the copyright owner(s) are credited and that the original publication in this journal is cited, in accordance with accepted academic practice. No use, distribution or reproduction is permitted which does not comply with these terms.
*Correspondence: Eckhard Strauch, ZWNraGFyZC5zdHJhdWNoQGJmci5idW5kLmRl